- 1Key Laboratory of Meteorological Disaster, Ministry of Education (KLME) and Collaborative Innovation Center on Forecast and Evaluation of Meteorological Disasters (CIC-FEMD), Nanjing University of Information Science and Technology (NUIST), Nanjing, China
- 2College of Intelligent Science and Control Engineering, Jingling Institute of Technology, Nanjing, China
- 3College of Oceanography, Hohai University, Nanjing, China
- 4Zhejiang Early Warning Center, Zhejiang Meteorological Bureau, Hangzhou, China
In this study, the relationship between Eurasian spring snowmelt and the East Asian summer monsoon (EASM), and its related mechanisms are investigated using observational and reanalysis data. Our analyses reveal that the interannual change of spring snowmelt over Eurasia is strongly linked to the EASM circulation variation and its corresponding summer precipitation in China. It is noteworthy that soil moisture anomaly caused by the snow hydrological effect (i.e., snowmelt) plays a bridging role in connecting the Eurasian snow cover and the EASM. The results show that increased spring snowmelt over Siberia induces anomalously higher soil moisture from spring to the following summer, thus continuously lowering the local temperature. Such a cooling effect leads to a weakened meridional temperature gradient and a decreased baroclinicity between north Eurasia and the Arctic Ocean. Consequently, the variation in atmospheric baroclinicity induced by the nonuniform heating of land surface exerts a significant impact on the synoptic eddy. The eddy forcing gives a positive feedback on intensification of the wave train over north Eurasia. Finally, the atmospheric circulation responses weaken the EASM and influence summer precipitation over China, producing excessive precipitation over most regions of South China and deficient rainfall over Northeast and North China. Our study emphasizes the hydrological effect of the sub-seasonal change of spring snow cover over Siberia, which has a significant linkage to the variation of the EASM intensity. The resultant changes in the EASM lead to an abnormal meridional dipole pattern of summer precipitation over China. These findings will contribute to the seasonal prediction of monsoon precipitation.
Introduction
The weather and climate over China are evidently influenced by variations of the monsoon system. Previous studies have shown that large-scale summer drought and flood disasters over China, which can result in tremendous economic losses and human casualties (Wang, 2006; Ding et al., 2009), are closely associated with the onset and intensity of the East Asian summer monsoon (EASM). Therefore, it is essential to explore the nature and cause of the interannual variability of the EASM.
The interannual variability of the Asian monsoon system can be affected by anomalous variations of lower boundary layer properties such as sea surface temperature, snow cover/snow depth, and soil moisture (Wang et al., 2000; Wu et al., 2012; Xiao and Duan 2016; Moon and Ha 2019). Among them, snow cover exerts a great impact on the interannual variability of the monsoon owing to its abilities to alter surface albedo and regulate soil moisture (Xu and Dirmeyer 2011; Wu et al., 2014; Henderson et al., 2018). High albedo reflects more solar radiation, while melting snow absorbs heat and increases soil moisture. In addition, snow cover on the surface will also affect the release of sensible heat between land and atmosphere because of the low thermal conductivity of the snowpack. These local cooling effects, in turn, have been suggested to play a significant role in global-scale climate as well as region climate (Gong et al., 2003; Cohen et al., 2014). Eurasian snow cover has been revealed to significantly influence the Asian summer monsoon (Douville and Royer 1996; Liu and Yanai 2002; Souma and Wang 2010). For the Indian monsoon, numerous analyses have shown that there generally exists a negative correlation between the Eurasian snow cover and the subsequent Indian summer monsoon, indicating that excessive (deficient) Eurasian snow cover/snow depth from winter to spring is followed by weak (strong) Indian summer monsoon rainfall (Sankar-Rao et al., 1996; Fasullo 2004; Dash et al., 2006; Saha et al., 2012). A large amount of numerical simulations have suggested that the abnormal surface thermal states induced by such snow cover anomalies can affect the atmospheric circulation, consequently causing monsoonal precipitation anomalies (Barnett et al., 1989; Bamzai and Marx 2000; Xu and Dirmeyer 2013).
For the East Asia monsoon, previous studies showed that anomalies of Eurasian snow cover have a significant impact on the monsoon precipitation in different regions (Yang and Xu 1994; Kripalani et al., 2002; Wu et al., 2009; Zuo et al., 2014). For example, Wu and Kirtman (2007) found that the snow cover anomalies over western Siberia are associated with an obvious abnormal circulation pattern over the eastern Atlantic through Eurasia, which leads to anomalous spring rainfall in South China. By using snow cover data from National Oceanic and Atmospheric Administration (NOAA) satellites, Yim et al. (2010) noted that the variation of an abnormal dipole pattern of snow cover over Eurasia is closely related to the EASM precipitation over Korea and Japan. Shen et al. (2020) found that an anomalously lower snow water equivalent over Siberia leads to higher temperature from the surface to the mid-troposphere, forming a Rossby wave train response and then leading to below-normal summer rainfall in South-Central China.
Snow cover affects the monsoon circulation system/climate mainly through the albedo effect and snow hydrological effect (Groisman et al., 1994; Wang et al., 2015). Previous studies have emphasized a robust relationship between winter/spring Eurasian snow cover and the East Asian climate, and have attempted to illustrate the relative potential mechanism. However, whether the albedo effect or the snow hydrological effect is more important still remains unclear. Robock et al. (2003) concluded that the albedo of snow cover is more significant because the snow hydrological effect can last for only one to two months. To support this conclusion, Liu et al. (2004) performed regional climate simulations and showed that the effect of albedo dominates the process during which snow cover affects climate, while the effects of snowmelt and evaporation are relatively less important. Nevertheless, some other studies tended to show that the snow hydrological effect is more significant (Bamzai and Marx 2000; Dash et al., 2006), and the albedo effect of snow cover becomes dominant only at a certain stage or in a certain region (Ose 1996; Souma and Wang 2010). For example, Wu et al. (2014) and Zhang et al. (2008) suggested that soil moisture anomalies are likely to be caused by snowmelt in spring and then maintained to summer, finally producing abnormal variations in the summer precipitation. Halder and Dirmeyer (2017) showed that snow cover in Eurasia can affect soil moisture through the lagged hydrological effect, resulting in the abnormal Indian summer monsoon circulation and thus precipitation anomalies.
Previous studies mainly focus on the effects of snow cover on climate at the seasonal scale, while the sub-seasonal change of snow cover (i.e., snowmelt) and its influences are less investigated. It is worth mentioning that snow cover in Eurasia exhibits little change during the three months in winter but shows evident sub-seasonal variations in other seasons, especially in spring (Mu and Zhou 2010). During March, April, and May, snow cover variation can be characterized by a snowmelt starting from southern to northeastern Eurasia, and a large amount of snow cover has disappeared by May. Under such a condition, studies on spring snow cover in Eurasia relying on the seasonal averages may erase inter-monthly differences. For instance, Dey and Kummar (1982) found that an anomalously larger snow cover area in spring over Eurasia plays an important role in retarding the development of the Indian summer monsoon, while a similar change in the snowmelt area has stronger contrary effect. Thus, spring snowmelt may be more suitable to represent the sub-seasonal variation of snow cover.
Zhang et al. (2017) have already indicated that a decrease of spring snow water equivalent in Eurasia can affect the East Asian summer precipitation and its associated abnormal atmospheric circulation via triggering an anomalous midlatitude Eurasian wave train. However, its effects on the EASM circulation system, the dominant factor of summer precipitation over East Asia, still remain unclear. The purpose of the present study is to investigate the effect of spring snowmelt on the EASM system and discuss the possible physical mechanisms underlying it. The results show that a strong relationship exists between spring snowmelt over Eurasia and the EASM. To be more specific, when there is excessive snowmelt in spring over Siberia, the EASM weakens with more/less summer precipitations over southern/northern China, and vice versa. This is mainly due to the snow hydrological effect: The abnormally higher snowmelt changes the local soil moisture in spring, and such a soil moisture anomaly is sustained to summer. Consequently, a continuously cooling effect is induced over Siberia, which decreases the temperature gradient and baroclinicity between north Eurasia and the Arctic Ocean in summer. Therefore, the spring snowmelt exerts a great impact on the summer atmospheric states and the monsoon circulations.
The rest of this paper is arranged as follows. Data and Methods introduces the data and methods adopted in our study. Climatologies of Observed Snow Water Equivalent and Snowmelt in Spring Over Eurasia presents the basic features of spring snowmelt variations over Eurasia. Relationships Between the Eurasian Spring Snowmelt and the East Asian Summer Monsoon shows the relationship between the spring snowmelt over Eurasia and the EASM anomalies. The related mechanisms are investigated and demonstrated in The Effect of Snowmelt Over Eurasia on the East Asian Summer Monsoon. Summary and Discussion is the summary.
Data and Methods
The data used in this study include: 1) The Finnish Meteorological Institute’s monthly snow water equivalent (SWE) dataset (Takala et al., 2011) from 1979 to 2014 that has an original resolution of 25 km × 25 km; in this study, by adopting the method of bilinear interpolation, we produced uniform grids with a resolution of 1° × 1°. 2) Monthly soil moisture dataset provided by the Global Land Data Assimilation System (GLDAS) V2.0 for the period 1948–2012 (1° × 1°; Rodell et al., 2004), which has been used previously in studies related to East Asian land surface processes (Wu and Zhang 2013; Cheng et al., 2015). The soil moisture information for the surface layer (0–10 cm) was used in this study. 3) The monthly reanalysis data with a resolution of 1° × 1° provided by the European Center for Medium-Range Weather Forecasts (ECMWF) from 1979 to 2015, whose variables include soil moisture, 2-m surface temperature, geopotential height, temperature, and wind fields. 4) The monthly observed precipitation data with a resolution of 1° × 1° provided by the China Meteorological Administration.
In this study, the spring snowmelt amount was calculated by subtracting the SWE in May from that in March, and if the value of the defined difference is positive, it represents the spring snowmelt amount. The seasonal means of other variables were calculated by 3-months averages: spring (March to May) and summer (June to August). The study period was set to be 1981–2014.
The scheme adopted in this study to calculate the apparent heat source, Q1 (Yanai et al., 1992), is shown as follows:
in which
Climatologies of Observed Snow Water Equivalent and Snowmelt in Spring Over Eurasia
Groisman et al. (1994) proposed that snow cover exhibits the greatest influence on the Earth’s radiative balance in the spring period (especially April and May) when the incoming solar radiation is mainly concentrated in high-latitude regions (i.e., snow cover regions). Figure 1 illustrates the multi-year mean SWE and snowmelt in spring, and their interannual standard deviations for the period of 1981–2014 over Eurasia. In spring, the northern part of Eurasia is almost completely covered by snow, and it is evident that the snow cover is relatively deeper over the region north of 60° N, with the maximal amount reaching 150 mm (Figure 1A). In addition, there is little interannual variability over Siberia, while northern Europe has a large interannual variability due to the freezing/thawing of snow, suggesting that there are evident changes in the speed of local snowmelt (Figure 1C). During the springtime, the mean snowmelt is mainly concentrated in Siberia, and the maximal snowmelt amount reaches 100 mm, which is comparable to the snow cover amount in spring (Figure 1B). In western Siberia and Europe, there are strong interannual variabilities of snowmelt (Figure 1D), which means there exists significant interaction between snow cover and atmospheric circulation and related surface air temperature changes (Chen et al., 2016; Wu and Chen 2016). Thus, spring snowmelt variation over Siberia and its potential influences are worthy of attention.
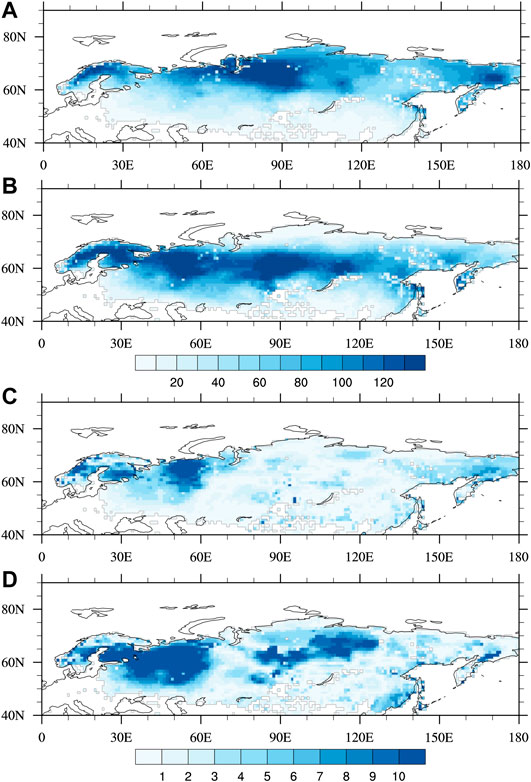
FIGURE 1. The multi-year means of (A) SWE (mm) and (B) snowmelt (mm) in spring, and (C,D) their interannual standard deviations for the period of 1981–2014.
An empirical orthogonal function (EOF) analysis was further applied to investigate the Eurasian spring snowmelt variations. The first EOF mode (EOF1) of the spring snowmelt accounts for 20.5% of the total variance. According to North et al. (1982), this mode is well separated from others. Figure 2 shows the spatiotemporal features of the first two EOF modes of Eurasian spring snowmelt. The spatial pattern of EOF1 is characterized by a west-east dipole pattern, with a negative center located in the East European Plain and a positive center in Siberia (Figure 2A). As shown by the principal component of EOF1 (PC1), the anomaly pattern exhibits significant interannual variations (Figure 2B) with a significant upward long-term trend (correlation between the time and PC1 is 0.38, p < 0.05). This further suggests that a relatively large variation center of spring snowmelt is located over Siberia. The second EOF mode (EOF2) of the spring snowmelt accounts for 15.2% of the total variance. As shown in Figure 2B, the EOF2 of Eurasian spring snowmelt shows the three-pole type, with a positive center in Northern Europe and two negative centers at Central Europe and Siberia.
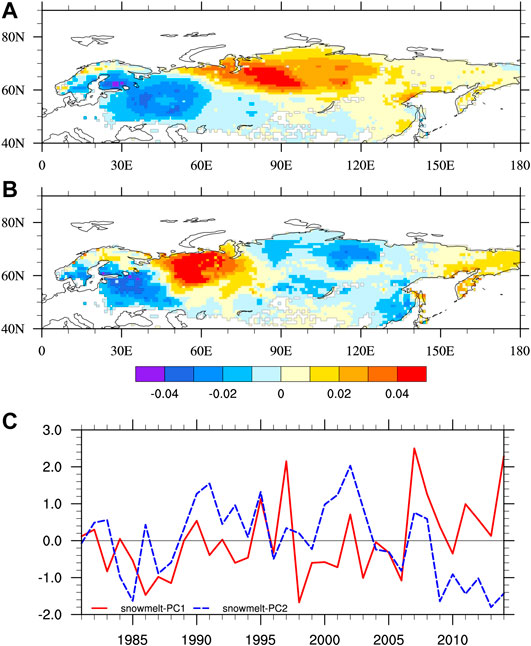
FIGURE 2. Spatial pattern of (A) the first EOF mode, (B) the second EOF mode of spring snowmelt anomaly over Eurasia, (C) and time series of the two modes of spring snowmelt.
Relationships Between the Eurasian Spring Snowmelt and the East Asian Summer Monsoon
Previous studies have presented that there are evident connections between snow cover over high-latitudes and the EASM (Wu and Kirtman 2007; Xiao and Duan 2016). Correspondingly, the East Asian summer precipitation and 850-hPa wind anomalies related to EOF1 of spring snowmelt were investigated with correlation analysis. As shown in Figure 3, the correlation coefficient pattern of wind filed with PC1 indicates that a weak cyclonic circulation exists in the south of 30° N in China, while the region around Lake Baikal is controlled by a strong anti-cyclonic circulation. When the PC1 phase is positive, there exist northeasterly (or northerly) wind anomalies from Northeast China, the Shandong Peninsula, the Bohai Gulf, and the middle to lower reaches of the Yangtze River to regions south of the Yangtze River, suggesting a weakened EASM activity. In contrast, when PC1 is negative, southwesterly wind anomalies become widely popular over East China and the Korean Peninsula, and the summer monsoon activity is strengthened. For the monsoon precipitation, the correlation distribution map of summer precipitation with PC1 shows a meridional dipole structure prevailing in the region between northern and southern China. When the snowmelt over Siberia is abnormally higher in spring, above-normal precipitation tends to be observed in south China and below-normal precipitation appears over north China, and vice versa. In addition, we recalculated our results based on the detrended data, and they are highly consistent with the results before removing the long-term trend.
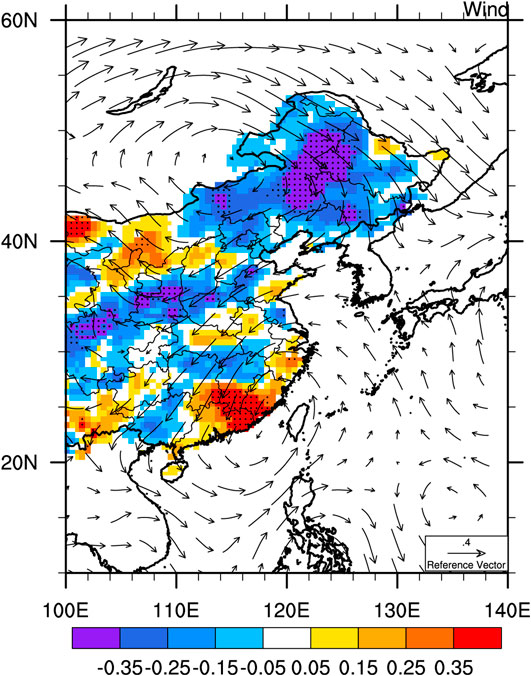
FIGURE 3. The correlation coefficients between PC1 of the spring snowmelt over Eurasia and summer 850-hPa wind field (vector) over East Asia. The correlation coefficients between PC1 of the spring snowmelt over Eurasia and precipitation in eastern China are shaded. Dotted areas denote the correlation significant at the 95% confidence level.
According to Huang et al. (2015), the anomalous activity of the EASM has multi-spatial modes, and their results suggested that its second EOF mode (EOF2) shows consistent variation of summer wind over the extensive area extending from South China, across the middle-lower reaches of the Yangtze River, the Shangdong Peninsula, the Bay of Bohai Sea, to Northeast China and the Korean Peninsula, which represents the overall variation of EASM intensity. Figure 4 shows EOF1 and EOF2 of the 850-hPa wind and summer precipitation over eastern China corresponding to the PCs of the EASM wind. The EOF1 mode of the 850-hPa wind is characterized by enhanced northeasterly wind from the middle and lower reaches of the Yangtze River to southern China and strengthened westerly wind between 10°N and 20°N. The anomalous precipitation pattern related to PC1 show negative anomalies over the middle and lower reaches of the Yangtze River, and positive anomalies over the east of northeast China, the southeast coastal area, and western Yunnan. (Figure 4A). The EOF2 mode of the 850-hPa wind is shown in Figure 4B, and it demonstrates a cyclonic circulation and an anti-cyclonic circulation over the south and north of East Asia, respectively, which is generally consistent with the anomalous wind related to PC1 of Eurasian snowmelt. The correlation coefficient between PC1 of spring snowmelt over Eurasia and PC2 of summer 850-hPa wind over East Asia reaches 0.38 (figure not shown), which is statistically significant at the 95% confidence level. Figure 4B further shows the summer precipitation over eastern China corresponding to PC2 of the EASM wind. The precipitation anomaly generally exhibits opposite phases between southern and northern China. The spatial correlation coefficient between the summer precipitation anomalies related to PC1 of spring snowmelt and PC2 of the EASM is as high as 0.65 (p < 0.001) (figure not shown). Those above findings further confirm that Eurasian spring snowmelt has evident effects on the EASM and its related precipitation.
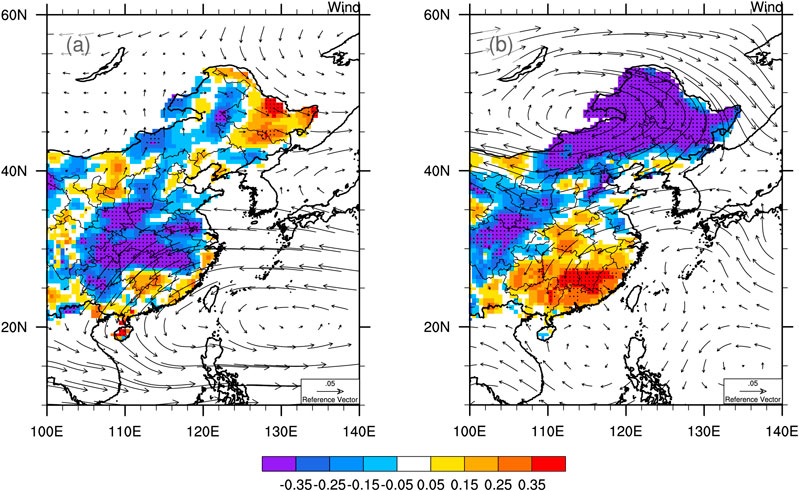
FIGURE 4. Spatial patterns (vectors) of the (A) first and (B) second mode of EASM, whichare given by the first two modes of the summer 850-hPa wind EOF analysis over East Asia. The correlation coefficients between time series of PC1 (PC2) and precipitation in eastern China are shaded in a (B). Dotted areas denote the correlation significant at the 95% confidence level.
To identify the key region of spring snowmelt affecting the EASM, Figure 5 demonstrates the spatial distribution of the correlation coefficient between spring snowmelt and PC2 of the EASM. As expected, the key area is mainly located in Siberia (as shown by the black box in Figure 5), which has a large coverage of positive correlations. To quantify the Eurasian snowmelt variability and its relationship with the EASM, we defined a snowmelt index (ISM) as the normalized area-averaged spring snowmelt over Siberia (55 –75 N and 60 –120 E). Figure 6 shows the PC2 of the EASM and ISM during 1981–2014. ISM is highly consistent with PC2 of the EASM, and their correlation coefficient is 0.55, which is statistically significant at p < 0.001. After removing their long-term trends, the correlation coefficient still reaches 0.45 (p < 0.05), verifying that the variation of EASM is distinctly associated with the spring snowmelt over Eurasia. Furthermore, the correlation distribution between ISM and summer 850-hPa wind over East Asia as well as that between ISM and rainfall in eastern China is shown in Figure 7. The correlation pattern more resembles the spatial distribution of EOF2 of the EASM and its corresponding precipitation anomalies, confirming that the key region where spring snowmelt affects the EASM lies over Siberia.
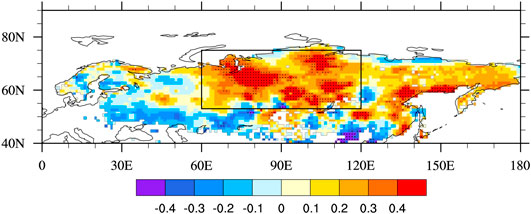
FIGURE 5. Correlation coefficients between PC2 of the EASM and the spring snowmelt over Eurasia. Dotted areas denote the correlation significant at the 95% confidence level.
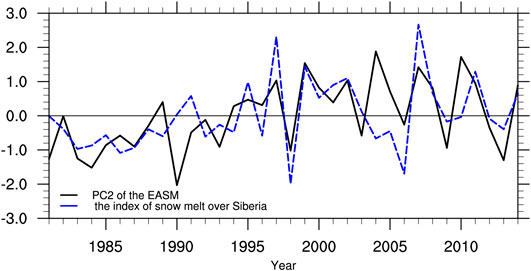
FIGURE 6. The standardized time series of PC2 of the EASM over East Asia and the standardized time series of ISM during 1981–2014.
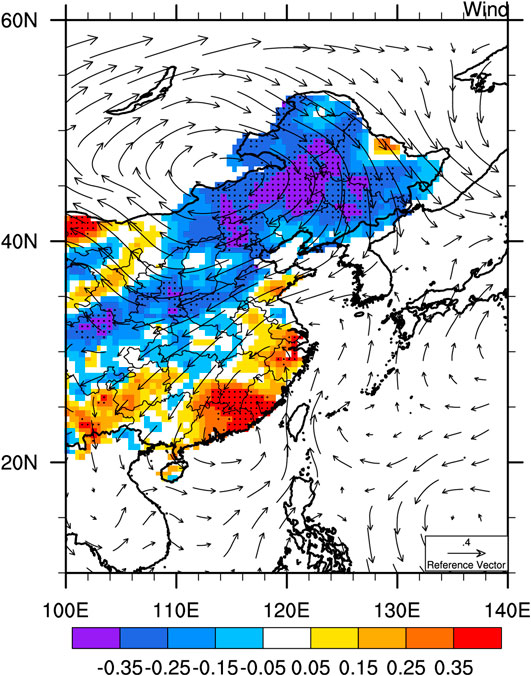
FIGURE 7. The correlation coefficients between ISM and summer 850-hPa wind field (vector) over East Asia. The correlation coefficients between ISM and precipitation in eastern China are shaded. Dotted areas denote the correlation significant at the 95% confidence level.
The Effect of Snowmelt Over Eurasia on the East Asian Summer Monsoon
SWE is a measure of water mass in the snowpack, and its characteristics determine the potential for the snow hydrological effect. In this study, we highlight the effect of spring snowmelt over Eurasia on the EASM circulation and precipitation, which can be attributed to the snow hydrological effect. It is found that the abnormal soil moisture over Eurasia induced by the spring snowmelt anomalies sustains itself to summer, affecting the local thermal state and thus the remote atmospheric circulation.
Figure 8A displays the regressed 0–10-cm soil moisture anomalies during summer onto ISM. Over Siberia, especially its western part, a significant increase in surface soil moisture occurs together with a large ISM. The connection between snowmelt and soil moisture supports the clear snow hydrological effect during the warming season: Excessive (deficient) snowmelt leads to wetter (drier) soil. It is similar to the results of Quiring and Kluver (2009), who found that an increase in snowfall during winter shows a positive correlation with the soil moisture in the subsequent summer in the northern part of the Great Plains. Along with the anomalous surface evapotranspiration associated with such a moistening (drying) of soil (figure not shown), there is a significant cooling (warming) in local air temperature in summer (Figure 8B).
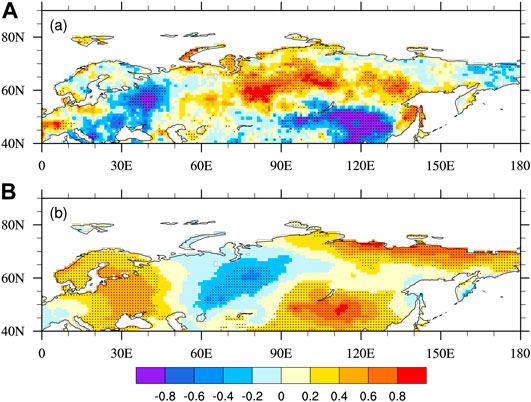
FIGURE 8. Regression of (A) GLDAS-2.0 surface soil moisture (0–10 cm; mm), and (B) 2-m temperature (K) in summer onto ISM during 1981–2014. Dotted areas are significant at the 95% confidence level.
Soil moisture anomalies generated from snowmelt during spring can modulate land-atmosphere coupling (Xu and Dirmeyer 2011) and act as a delayed snow-driven feedback to the atmosphere in summer. To analyze the effect of snowmelt on the surface thermal conditions in spring and summer, we primarily investigated the apparent heating. Figure 9 shows the height-latitude section (averaged over 40°–140° E) of the regression of spring and summer Q1 anomalies onto ISM. As shown in Figure 9A, there exists a negative anomaly of Q1 from the ground surface to the overlying atmospheric column in spring, resulting in a vertical temperature structure characterized by a “cold source” from the ground surface to the 500-hPa upper troposphere. In summer, the pattern of the apparent heating anomalies is quite similar to that in spring, but the corresponding strength in summer is only half of that in spring (Figure 9B). It is in agreement with previous studies which show that Eurasian snowmelt may directly increase soil moisture, and then affect soil temperature during warming seasons, thereby leading to the reduction of surface air temperature and tropospheric diabatic heating (Saito and Cohen 2003; Zuo et al., 2011).
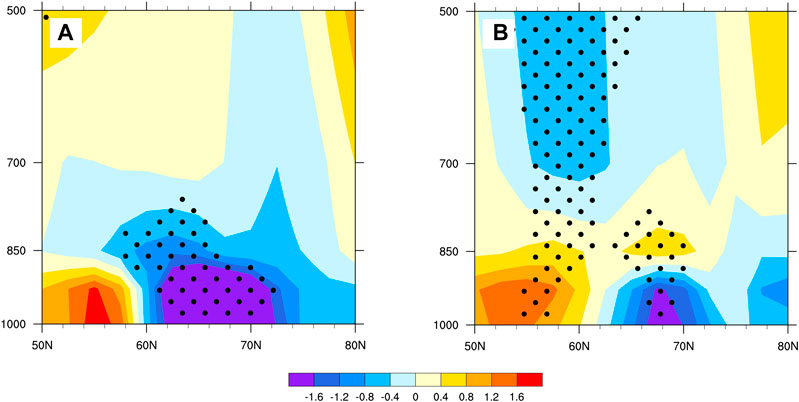
FIGURE 9. Regression of the height-latitude section (averaged over 40°–140° E) in (A) spring and (B) summer Q1 (10 −6k/mon) anomalies onto ISM during 1981–2014. Dotted areas are significant at the 95% confidence level.
Figure 10A illustrates that the near-surface thermal conditions associated with snowmelt substantially change the local temperature thickness of the lower troposphere between 700 and 1,000 hPa, with a notable cooling centered over western Siberia. The decreased summer temperature over Siberia further reduces the meridional temperature gradient between northern Eurasia and the Arctic Ocean, and simultaneously strengthens the meridional temperature gradient between the mid-latitude regions to northern Eurasia. Lindzen et al. (1980) pointed out that the atmospheric baroclinicity is closely related to the meridional temperature gradient of the atmosphere. We calculated the maximum Eady growth rate
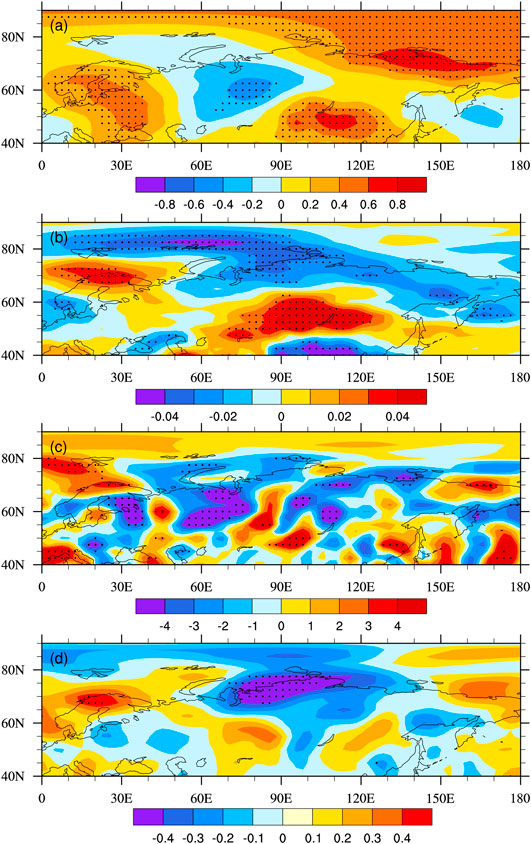
FIGURE 10. Regression of (A) tropospheric temperature (K) averaged between 1,000 and 700 hPa, (B) atmospheric baroclinicity (day-1) at 700 hPa, (C) eddy kinetic energy (m2·s−2), and (D) eddy forcing at 500 hPa (10–4 m·s−1) in summer onto ISM during 1981–2014. Dotted areas are significant at the 95% confidence level.
Further analysis indicates that the variation in the atmospheric baroclinicity induced by the nonuniform heating of the land surface can have a significant impact on the eddy kinetic energy. We used the eddy kinetic energy (EKE),
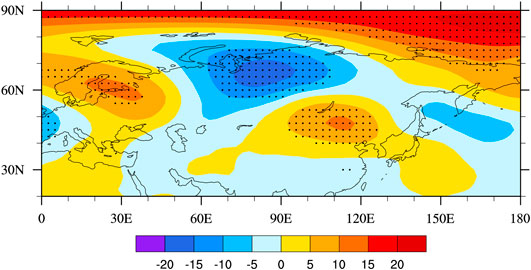
FIGURE 11. Regression of 500-hPa geopotential height anomalies (gpm) in summer onto ISM during 1981–2014. Dotted areas are significant at the 95% confidence level.
To examine the EASM responses over East Asia, we further checked the summer wind anomalies associated with ISM. Figure 12A shows the zonal wind anomalies at 200 hPa regressed onto ISM. The Asian subtropical jet is normally located along the latitudinal band at 35°–45° N in summertime. The ISM corresponds to significant positive anomalies to the north of the subtropical jet and negative anomalies over the jet zone. These zonal wind anomalies suggest that weakened westerly jet occurs in summer when there is excessive spring snowmelt over Siberia, and vice versa. Indeed, such an anomalous subtropical jet in summer over East Asia is closely linked to the strength of the EASM (Liao et al., 2004; Xie et al., 2015). Accordingly, Figure 12B demonstrates the summer meridional wind at 850 hPa regressed onto ISM. With the increase of spring snowmelt over Siberia, eastern China mainly has a northerly wind anomaly, implying that the EASM is weakened. This prevents the northward moisture transportation in summer, causing precipitation surplus and deficit over southern and northern China (Figure 7), respectively. Figure 13 shows the relationships of ISM with summer precipitation over South China (20°–30° N and 110°–120° E) and North China (40°–55° N and 110°–135° E), respectively. In the period of 1981–2014, the spring snowmelt over Siberia has a strong positive relationship with summer precipitation over South China (r = 0.35, p < 0.05; Figure 13A significant negative correlation with summer precipitation over North China (r = −0.56, p < 0.001; Figure 13B). This indicates that when there is excessive snowmelt over Siberia, the summer rain band moves northward due to a weakened EASM. On the contrary, when there is abnormally deficient snowmelt over Siberia, the EASM will be intensified, consequently causing the monsoon precipitation to shift northward.
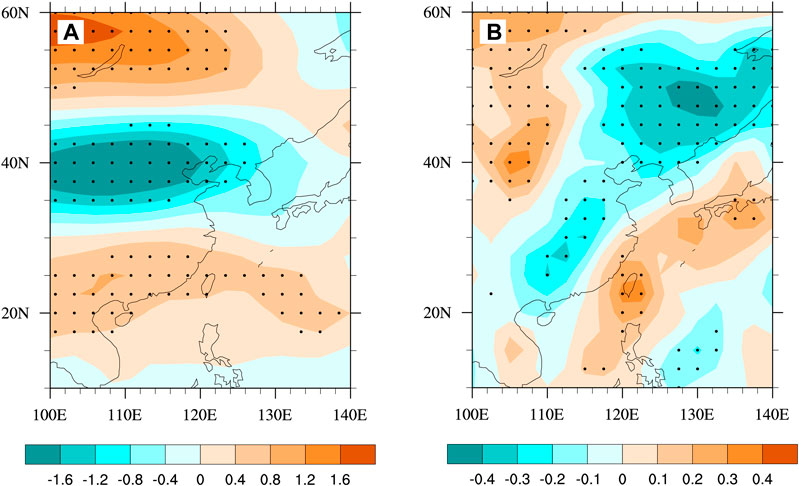
FIGURE 12. Regression of (A) 200-hPa zonal wind (ms−1) and (B) 850-hPa meridional wind (ms−1) anomalies in summer onto ISM during 1981–2014. Dotted areas are significant at the 95% confidence level.
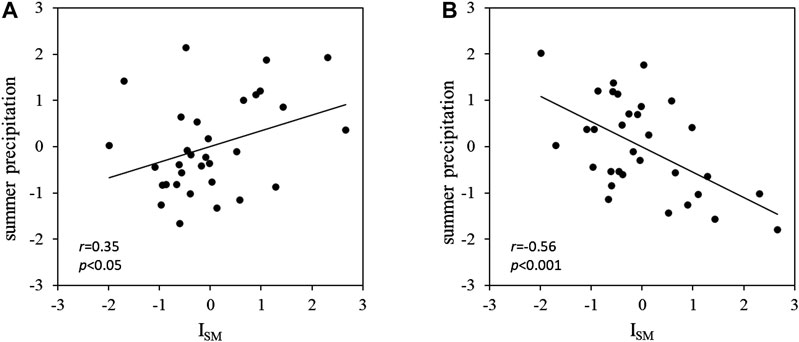
FIGURE 13. Scatter plots (A) between ISM and the summer rainfall averaged over South China (20–30° N and 110–120° E), and (B) between ISM and the summer rainfall averaged over North China (40–55° N and 110–135° E) for the period of 1981–2014. All data were standardized for the correlation analysis.
Summary and Discussion
In the current study, we explored the Eurasian spring snowmelt anomaly and its linkages to the EASM and the related summer precipitation over eastern China. Using the monthly observed SWE dataset, we defined the spring snowmelt amount to reflect the sub-seasonal change of snow cover. The basic characteristics of the anomalous variations of spring snowmelt over Eurasia were analyzed, and the possible mechanisms behind the impact of the anomalous spring snowmelt on the EASM were explored from the perspective of snow hydrological effect over Eurasia.
Results show that EOF1 of Eurasian snowmelt exhibits a west–east dipole pattern, with a negative center located over the East European Plain and a positive center over Siberia. This anomalous snowmelt pattern is significantly associated with EOF2 of the EASM, with the correlation coefficient between their principle components being 0.38. The further analysis verifies that the key region of spring snowmelt affecting the EASM is mainly located in Siberia. When there is more snowmelt in spring over Siberia, the summer monsoon activity generally becomes weakened, and there exists a dipole structure of summer precipitation in China (above-normal precipitation in the south and below-normal precipitation in the north). In contrast, associated with less spring snowmelt over Siberia, the overall summer monsoon activity becomes strengthened, and a northward movement of monsoon precipitation occurs.
Snowmelt is the major characteristic of the delayed hydrological effect, and the sub-seasonal snow melting process absorbs heat and increases soil moisture at the seasonal scale. The analysis in this study shows a robust positive correlation between snowmelt and the subsequent summer soil moisture. That is to say, the snow hydrological effect indeed exists and can hold a long-term memory via soil moisture anomaly persistency, which also plays an important role in connecting the snow cover over the mid-high latitudes and the EASM. The soil moisture is abnormally higher due to more snowmelt over Siberia in spring, and such a soil moisture anomaly is sustained to the following summer. Thus, the increased soil moisture can continuously lower the surface temperature and cool the atmosphere in summer. This cooling effect in Siberia leads to a weakened meridional temperature gradient and decreased baroclinicity between north Eurasia and the Arctic Ocean. Then, the variation in the atmospheric baroclinicity induced by the nonuniform heating of the land surface can produce a significant impact on the synoptic eddy. The synoptic eddy feedback further intensifies the wave train over north Eurasia.
The above factors create favorable physical conditions for the maintenance and enhancement of the anomalous Eurasian wave train prevailing over the region from Europe to Lake Baikal. The anomalous circulation patterns associated with an excessive snowmelt over Siberia ultimately weaken the EASM and influence the summer precipitation over China, with excessive precipitation occurring over most regions of South China and deficient precipitation over Northeast China and North China. Our findings demonstrate the role of anomalous snowmelt in triggering the Eurasian wave train, which is closely related to the EASM and summer precipitation over China. This study emphasizes the snow hydrological effect reflected by the spring snowmelt over Eurasia, and further contributes to the seasonal prediction of the EASM and summer precipitation over eastern China.
Previous studies indicate that spring Arctic Oscillation (AO) is the dominate driving factor for the spring surface air temperature and snow cover anomalies over the Eurasia (Chen et al., 2016). We adopt a spring AO index provided by the United States Climate Prediction Center (CPC) to denote the AO inter-annual variation. This index and its detailed information can be found at the following website: https://www.cpc.ncep.noaa.gov/products/precip/CWlink/daily_ao_index/ao.shtml. During our study period, the spring AO index exhibits an evident correlation with the PC1 of spring snowmelt over the Eurasia by a correlation coefficient of 0.33 (p < 0.05; Figure not shown). This suggests that the AO could also regulate the Eurasian snowmelt in spring. In addition, studies have shown that spring AO exerts evident impacts on the following EASM (Chen et al., 2015; Chen et al., 2020). Therefore, it is worth of attention that spring large-scale atmospheric circulation activities, such the AO, may contribute the Eurasian snowmelt affecting the EASM. This is beyond our current objective, which could be thoroughly investigated in the further.
Data Availability Statement
The raw data supporting the conclusions of this article will be made available by the authors, without undue reservation.
Author Contributions
HC designed the study, contributed to the idea, and revised manuscript writing. BX contributed to the data analysis and manuscript writing. CG and QH contributed to the data analysis. GZ contributed to the manuscript review.
Funding
This work was jointly supported by the National Science Fund for Distinguished Young Scholars (41625019), the National Natural Science Foundation of China (41230422, 41905054), the China Postdoctoral Science Foundation (2020T130168), the Joint Open Project of Key Laboratory of Meteorological Disaster, Ministry of Education/Collaborative Innovation Center on Forecast and Evaluation of Meteorological Disasters, Nanjing University of Information Science and Technology (KLME202006), and the Fifth “333 High Level Talents Training Project” of Jiangsu Province.
Conflict of Interest
The authors declare that the research was conducted in the absence of any commercial or financial relationships that could be construed as a potential conflict of interest.
The reviewer (AH) declared a past co-authorship with one of the authors (HC) to the handling editor.
References
Bamzai, A. S., and Marx, L. (2000). COLA AGCM simulation of the effect of anomalous spring snow over Eurasia on the Indian summer monsoon. Q.J Royal Met. Soc. 126, 2575–2584. doi:10.1002/qj.49712656811
Barnett, T. P., Dümenil, L., Schlese, U., Roeckner, E., and Latif, M. (1989). The effect of Eurasian snow cover on regional and global climate variations. J. Atmos. Sci. 46, 661–686. doi:10.1175/1520-0469(1989)046<0661:teoesc>2.0.co;2
Chen, H., Teng, F., Zhang, W., and Liao, H. (2017). Impacts of anomalous midlatitude cyclone activity over east Asia during summer on the decadal mode of East asian summer monsoon and its possible mechanism. J. Clim. 30, 739–753. doi:10.1175/jcli-d-16-0155.1
Chen, S., Chen, W., and Wu, R. (2015). An interdecadal change in the relationship between boreal spring arctic oscillation and the east asian summer monsoon around the early 1970s. J. Clim. 28, 1527–1542. doi:10.1175/jcli-d-14-00409.1
Chen, S., Chen, W., Wu, R., and Song, L. (2020). Impacts of the atlantic multidecadal oscillation on the spring arctic oscillation and the following East Asian summer monsoon relation. J. Clim. 33, 6651–6672. doi:10.1175/JCLI-D-19-0978.1
Cheng, S., Guan, X., Huang, J., Ji, F., and Guo, R. (2015). Long-term trend and variability of soil moisture over East Asia. J. Geophys. Res. Atmos. 120, 8658–8670. doi:10.1002/2015jd023206
Chen, S., Wu, R., and Liu, Y. (2016). Dominant modes of interannual variability in eurasian surface air temperature during boreal spring. J. Clim. 29, 1109–1125. doi:10.1175/jcli-d-15-0524.1
Cohen, J., Furtado, J. C., Jones, J., Barlow, M., Whittleston, D., and Entekhabi, D. (2014). Linking siberian snow cover to precursors of stratospheric variability. J. Clim. 27, 5422–5432. doi:10.1175/jcli-d-13-00779.1
Dash, S. K., Parth Sarthi, P., and Panda, S. K. (2006). A study on the effect of Eurasian snow on the summer monsoon circulation and rainfall using a spectral GCM. Int. J. Climatol. 26, 1017–1025. doi:10.1002/joc.1299
Dey, B., and Bhanu Kumar, O. S. R. U. (1982). An apparent relationship between Eurasian spring snow cover and the advance period of the Indian summer monsoon. J. Appl. Meteorol. 21, 1929–1932. doi:10.1175/1520-0450(1982)021<1929:aarbes>2.0.co;2
Ding, Y., Sun, Y., Wang, Z., Zhu, Y., and Song, Y. (2009). Inter-decadal variation of the summer precipitation in China and its association with decreasing Asian summer monsoon Part II: possible causes. Int. J. Climatol. 29, 1926–1944. doi:10.1002/joc.1759
Douville, H., and Royer, J.-F. (1996). Sensitivity of the Asian summer monsoon to an anomalous Eurasian snow cover within the Météo-France GCM. Clim. Dynam. 12, 449–466. doi:10.1007/bf02346818
Fasullo, J. (2004). A stratified diagnosis of the Indian monsoon-Eurasian snow cover relationship. J. Clim. 17, 1110–1122. doi:10.1175/1520-0442(2004)017<1110:asdoti>2.0.co;2
Gong, G., Entekhabi, D., and Cohen, J. (2003). Relative impacts of siberian and north American snow anomalies on the winter arctic oscillation. Geophys. Res. Lett. 30, 1848. doi:10.1029/2003gl017749
Groisman, P. Y., Karl, T. R., Knight, R. W., and Stenchikov, G. L. (1994). Changes of snow cover, temperature, and radiative heat balance over the northern hemisphere. J. Clim. 7, 1633–1656. doi:10.1175/1520-0442(1994)007<1633:coscta>2.0.co;2
Halder, S., and Dirmeyer, P. A. (2017). Relation of Eurasian snow cover and Indian summer monsoon rainfall: importance of the delayed hydrological effect. J. Clim. 30, 1273–1289. doi:10.1175/jcli-d-16-0033.1
Hartmann, D. L., and Lo, F. (1998). Wave-driven zonal flow vacillation in the southern hemisphere. J. Atmos. Sci. 55, 1303–1315. doi:10.1175/1520-0469(1998)055<1303:wdzfvi>2.0.co;2
Henderson, G. R., Peings, Y., Furtado, J. C., and Kushner, P. J. (2018). Snow-atmosphere coupling in the northern hemisphere. Nat. Clim. Change. 8, 954–963. doi:10.1038/s41558-018-0295-6
Huang, Y., Chen, H., Jiang, W., Xu, B., and Li, Z. (2015). Multi-spatial modes of East Asian summer monsoon activity: comparative analysis of various reanalysis data. Chin. J. Atmos. Sci. 39 (1), 145–160.
Kripalani, R. H., Kim, B.-J., Oh, J.-H., and Moon, S.-E. (2002). Relationship between Soviet snow and Korean rainfall. Int. J. Climatol. 22, 1313–1325. doi:10.1002/joc.809
Lau, N.-C., and Holopainen, E. O. (1984). Transient eddy forcing of the time-mean flow as identified by geopotential tendencies. J. Atmos. Sci. 41, 313–328. doi:10.1175/1520-0469(1984)041<0313:tefott>2.0.co;2
Lau, N.-C., and Nath, M. J. (1991). Variability of the baroclinic and barotropic transient eddy forcing associated with monthly changes in the midlatitude storm tracks. J. Atmos. Sci. 48, 2589–2613. doi:10.1175/1520-0469(1991)048<2589:votbab>2.0.co;2
Liao, Q.-H., Tao, S.-Y., and Wang, H.-J. (2004). Interannual variation of summer subtropical westerly jet in east Asia and its impacts on the climate anomalies of East Asia summer monsoon. Chin. J. Geophys. 47, 12–21. doi:10.1002/cjg2.449
Lindzen, R. S., Farrell, B., and Tung, K.-K. (1980). The concept of wave overreflection and its application to baroclinic instability. J. Atmos. Sci. 37, 44–63. doi:10.1175/1520-0469(1980)037<0044:tcowoa>2.0.co;2
Liu, H., Sun, Z., Wang, J., and Min, J. (2004). A modeling study of the effects of anomalous snow cover over the Tibetan Plateau upon the South Asian summer monsoon. Adv. Atmos. Sci. 21, 964–975.
Liu, X., and Yanai, M. (2002). Influence of Eurasian spring snow cover on Asian summer rainfall. Int. J. Climatol. 22, 1075–1089. doi:10.1002/joc.784
Lorenz, D. J., and Hartmann, D. L. (2003). Eddy-zonal flow feedback in the Northern Hemisphere winter. J. Clim. 16, 1212–1227. doi:10.1175/1520-0442(2003)16<1212:effitn>2.0.co;2
Moon, S., and Ha, K.-J. (2019). Early Indian summer monsoon onset driven by low soil moisture in the Iranian desert. Geophys. Res. Lett. 46, 10568. doi:10.1029/2019gl084520
Mu, S., and Zhou, G. (2010). Relationship between winter Northern Eurasian fresh snow extent and summer climate anomalies in China. Chin. J. Atmos. Sci. 34 (1), 213–226.
North, G. R., Bell, T. L., Cahalan, R. F., and Moeng, F. J. (1982). Sampling errors in the estimation of empirical orthogonal functions. Mon. Weather Rev. 110, 699–706. doi:10.1175/1520-0493(1982)110<0699:seiteo>2.0.co;2
Ose, T. (1996). The comparison of the simulated response to the regional snow mass anomalies over Tibet, Eastern Europe, and Siberia. J. Meteorol. Soc. Jpn. 74, 845–866. doi:10.2151/jmsj1965.74.6_845
Quiring, S., and Kluver, D. (2009). Relationship between winter/spring snowfall and summer precipitation in the Northern Great Plains of North America. J. Hydrometeorol. 10, 1203–1217. doi:10.1175/2009jhm1089.1
Robock, A., Mu, M., Vinnikov, K., and Robinson, D. (2003). Land surface conditions over Eurasia and Indian summer monsoon rainfall. J. Geophys. Res. 108, 4131. doi:10.1029/2002jd002286
Rodell, M., Houser, P. R., Jambor, U., Gottschalck, J., Mitchell, K., Meng, C.-J., et al. (2004). The global land data assimilation system. Bull. Am. Meteorol. Soc. 85, 381–394. doi:10.1175/bams-85-3-381
Saha, S. K., Pokhrel, S., and Chaudhari, H. S. (2012). Influence of Eurasian snow on Indian summer monsoon in NCEP CFSv2 freerun. Clim. Dynam. 41, 1801–1815. doi:10.1007/s00382-012-1617-4
Saito, K., and Cohen, J. (2003). The potential role of snow cover in forcing interannual variability of the major Northern Hemisphere mode. Geophys. Res. Lett. 30, 1302. doi:10.1029/2002gl016341
Sankar-Rao, M., Lau, K. M., and Yang, S. (1996). On the relationship between Eurasian snow cover and the Asian summer monsoon. Int. J. Climatol. 16, 605–616. doi:10.1002/(sici)1097-0088(199606)16:6<605::aid-joc41>3.0.co;2-p
Shen, H., Li, F., He, S., Orsolini, Y. J., and Li, J. (2020). Impact of late spring Siberian snow on summer rainfall in South-Central China. Clim. Dynam. 54, 3803–3818. doi:10.1007/s00382-020-05206-5
Song, L., Wang, L., Chen, W., and Zhang, Y. (2016). Intraseasonal variation of the strength of the east asian trough and its climatic impacts in boreal winter. J. Clim. 29, 2557–2577. doi:10.1175/jcli-d-14-00834.1
Souma, K., and Wang, Y. (2010). A comparison between the effects of snow albedo and infiltration of melting water of Eurasian snow on East Asian summer monsoon rainfall. J. Geophys. Res. 115, D02115. doi:10.1029/2009jd012189
Takala, M., Luojus, K., Pulliainen, J., Derksen, C., Lemmetyinen, J., Kärnä, J.-P., et al. (2011). Estimating northern hemisphere snow water equivalent for climate research through assimilation of space-borne radiometer data and ground-based measurements. Remote Sensing of Environment. 115, 3517–3529. doi:10.1016/j.rse.2011.08.014
Wang, B., Wu, R., and Fu, X. (2000). Pacific-East Asian teleconnection: how does ENSO affect East Asian climate? J. Clim. 13, 1517–1536. doi:10.1175/1520-0442(2000)013<1517:peathd>2.0.co;2
Wang, T., Peng, S., Ottlé, C., and Ciais, P. (2015). Spring snow cover deficit controlled by intraseasonal variability of the surface energy fluxes. Environ. Res. Lett. 10, 024018. doi:10.1088/1748-9326/10/2/024018
Wu, B., Yang, K., and Zhang, R. (2009). Eurasian snow cover variability and its association with summer rainfall in China. Adv. Atmos. Sci. 26, 31–44. doi:10.1007/s00376-009-0031-2
Wu, L., and Zhang, J. (2013). Asymmetric effects of soil moisture on mean daily maximum and minimum temperatures over eastern China. Meteorol. Atmos. Phys. 122, 199–213. doi:10.1007/s00703-013-0284-2
Wu, R., and Chen, S. (2016). Regional change in snow water equivalent-surface air temperature relationship over Eurasia during boreal spring. Clim. Dynam. 47, 2425–2442. doi:10.1007/s00382-015-2972-8
Wu, R., and Kirtman, B. P. (2007). Observed relationship of spring and summer East Asian rainfall with winter and spring Eurasian snow. J. Clim. 20, 1285–1304. doi:10.1175/jcli4068.1
Wu, R., Liu, G., and Ping, Z. (2014). Contrasting Eurasian spring and summer climate anomalies associated with western and eastern Eurasian spring snow cover changes. J. Geophys. Res. Atmos. 119, 7410–7424. doi:10.1002/2014jd021764
Wu, Z., Li, J., Jiang, Z., and Ma, T. (2012). Modulation of the Tibetan Plateau snow cover on the ENSO teleconnections: from the East Asian summer monsoon perspective. J. Clim. 25, 2481–2489. doi:10.1175/jcli-d-11-00135.1
Xiao, Z., and Duan, A. (2016). Impacts of Tibetan Plateau snow cover on the interannual variability of the East Asian summer monsoon. J. Clim. 29, 8495–8514. doi:10.1175/jcli-d-16-0029.1
Xie, Z., Du, Y., and Yang, S. (2015). Zonal extension and retraction of the subtropical westerly jet stream and evolution of precipitation over East Asia and the Western Pacific. J. Clim. 28, 6783–6798. doi:10.1175/jcli-d-14-00649.1
Xu, L., and Dirmeyer, P. (2011). Snow-atmosphere coupling strength in a global atmospheric model. Geophys. Res. Lett. 38, L13401. doi:10.1029/2011gl048049
Xu, L., and Dirmeyer, P. (2013). Snow-atmosphere coupling strength. Part II: albedo effect versus hydrological effect. J. Hydrometeorol. 14, 404–418. doi:10.1175/jhm-d-11-0103.1
Yanai, M., Chengfeng, L., and Zhengshan, S. (1992). Seasonal heating of the Tibetan Plateau and its effects on the evolution of the Asian summer monsoon. J. Meteorol. Soc. Jpn. 79, 419–434.
Yang, S., and Xu, L. (1994). Linkage between Eurasian winter snow cover and regional Chinese summer rainfall. Int. J. Climatol. 14, 739–750. doi:10.1002/joc.3370140704
Yim, S.-Y., Jhun, J.-G., Lu, R., and Wang, B. (2010). Two distinct patterns of spring Eurasian snow cover anomaly and their impacts on the East Asian summer monsoon. J. Geophys. Res. 115, D22113. doi:10.1029/2010jd013996
Zhang, J., Wang, W.-C., and Leung, L. R. (2008). Contribution of land-atmosphere coupling to summer climate variability over the contiguous United States. J. Geophys. Res. 113, D17119. doi:10.1029/2008jd009807
Zhang, R., Zhang, R., and Zuo, Z. (2017). Impact of Eurasian spring snow decrement on East Asian summer precipitation. J. Clim. 30, 3421–3437. doi:10.1175/jcli-d-16-0214.1
Zuo, Z., Yang, S., Wang, W., Kumar, A., Xue, Y., and Zhang, R. (2011). Relationship between anomalies of Eurasian snow and southern China rainfall in winter. Environ. Res. Lett. 6, 045402. doi:10.1088/1748-9326/6/4/045402
Keywords: snowmelt, snow hydrological effect, east asian summer monsoon, seasonal prediction, eurasian continent
Citation: Xu B, Chen H, Gao C, Zeng G and Huang Q (2021) Abnormal Change in Spring Snowmelt Over Eurasia and Its Linkage to the East Asian Summer Monsoon: The Hydrological Effect of Snow Cover. Front. Earth Sci. 8:594656. doi: 10.3389/feart.2020.594656
Received: 14 August 2020; Accepted: 28 September 2020;
Published: 14 January 2021.
Edited by:
Wen Chen, Institute of Atmospheric Physics (CAS), ChinaReviewed by:
Anning Huang, Nanjing University, ChinaShangfeng Chen, Institute of Atmospheric Physics (CAS), China
Copyright © 2021 Xu, Chen, Gao, Zeng and Huang. This is an open-access article distributed under the terms of the Creative Commons Attribution License (CC BY). The use, distribution or reproduction in other forums is permitted, provided the original author(s) and the copyright owner(s) are credited and that the original publication in this journal is cited, in accordance with accepted academic practice. No use, distribution or reproduction is permitted which does not comply with these terms. The author(s) is/are employees of the US Government and therefore domestic copyright protection in USA does not apply to this work. The work may be protected under the copyright laws of other jurisdictions when used in those jurisdictions.
*Correspondence: Haishan Chen, aGFpc2hhbkBudWlzdC5lZHUuY24=