- 1Department of Vertebrate Paleontology, Florida Museum of Natural History, Gainesville, FL, United States
- 2Department of Geological Sciences, University of Florida, Gainesville, FL, United States
- 3Thompson Earth Systems Institute, Gainesville, FL, United States
- 4Department of Biological Sciences, University of Florida, Gainesville, FL, United States
- 5Department of Anthropology, University of Florida, Gainesville, FL, United States
- 6Department of Paleontology, Science Museum of Minnesota, Saint Paul, MN, United States
- 7School of Teaching and Learning, University of Florida, Gainesville, FL, United States
The past decade has seen an exponential increase of innovative applications of 3D technology in the geosciences. Here, we present a case study from the Florida Museum of Natural History applied to the multidisciplinary field of paleontology. We have deployed 3D scanning and printing techniques for the purposes of scientific research, formal education, and informal outreach. Depending on the application of the 3D file, different techniques are utilized to create high-fidelity models of physical fossil specimens or geologic field sites. These techniques include X-ray CT scans, surface scans, and photogrammetry, all of which produce 3D models that vary in resolution and scale. Novel paleontological research applied non-destructive CT scanning to explore the internal anatomy of fossil museum specimens, additionally, 3D models are being used to create K–12 curricula aligned with national and state-specific education standards that are implemented in formal classroom settings. Many of these lessons are part of the NSF-funded iDigFossils project, which aims to evaluate students’ motivation and interest towards science, technology, engineering, and mathematics after participating in integrated 3D printing and paleontology lessons. Specifically, lessons on dinosaur trackways, horse evolution, and the Great American Biotic Interchange teach geologic concepts such as deep time, taphonomy, plate tectonics, and evolutionary trends. The same 3D models developed for these K–12 lessons have been used during Florida Museum’s outreach events to engage broad audiences with hands-on exhibits and activities. All 3D files are stored on open-access, online repositories, providing accessibility to fossil specimens and field sites. The application of 3D technology for the study of fossils and paleontology will continue to expand the impact of scientific discoveries for basic research as well as for broader impacts on society.
Introduction
Museums have been using 3D technology for more than two decades to digitize field sites and preserve natural history objects, while making them more accessible to scientific researchers and the public (i.e., Gangjee et al., 2011; Fujiwara, 2012; Rahman et al., 2012; Lee, 2013; Neumüller et al., 2014; Short, 2015). Accessibility for scientific research has been driven by advancements in techniques (i.e., CT scanning, laser surface scanning, and photogrammetry) for producing 3D models and an increase in the number of open-source repositories (Boyer et al., 2016; Sutton et al., 2016; Lebrun and Orliac, 2017; Walker and Humphries, 2019). The use of 3D printing (3DP) technology expanded into households and classrooms as equipment and supportive software became more prevalent and affordable (Savini and Savini, 2015; Gokhare et al., 2017). This combination of accessibility and affordability has led to unique opportunities for collaborations between researchers and educators. At the Florida Museum of Natural History (FLMNH) 3D technology has been pivotal in scientific research, formal K–12 education, and museum outreach (e.g., Grant et al., 2016; Blackburn et al., 2020).
Within geoscience, vertebrate paleontology has benefited tremendously from the application of 3D technologies. In a research context, 3D technology is used to document field sites and preserve fossil specimens, while permitting for non-destructive analyses of internal anatomy. In many instances, fossil recovery is time-sensitive, either because the fossils are prone to destruction through natural weathering or access to the field site itself is limited. This was particularly true for the Cerro Ballena field site, a whale death assemblage in Chile, which was unearthed during the construction of a highway and prompted rapid 3D modeling of in situ specimens at this fossiliferous locality (Pyenson et al., 2014). Classically, histological studies are conducted to examine internal anatomy by cutting and polishing fossil specimens, but these techniques inevitably destroy the specimens to some degree. The advent of high-fidelity CT scanning enabled sustainable analyses of internal anatomy, while creating a digital record of the fossil (Sutton, 2008). Many institutions have made these digital records available to the public through their online repositories (e.g., Digital Atlas of Ancient Life, Idaho Virtualization Laboratory, MorphoMuseuM, MorphoSource, Phenome10K, Smithsonian National Museum of Natural History 3D Collection, and University of Michigan Online Repository of Fossils).
These virtual collections have immense educational potential in formal and informal settings. 3D technology has been applied across a wide array of K–12 subjects, but is particularly relevant within Science, Technology, Engineering, Art, and Mathematics (STEAM; Lee et al., 2015; Maloy et al., 2017; Trust and Maloy, 2017; Pai et al., 2018). The United States federal government has emphasized the importance of STEAM-integrated education to remain globally competitive (PCAST, 2012; Land, 2013; Crippen et al., 2015a). Geoscience is inherently interdisciplinary, making it ideal for incorporating STEAM-integrated lessons. Over the last decade, a wider introduction of 3DP into geoscience education has occurred, covering topics such as climate, evolution, topography, tectonics, and stratigraphy (Reyes et al., 2008; Horowitz and Schultz, 2014; Grant et al., 2016; Koehler, 2017; Kyriakopoulos, 2019).
As we have seen from the work described above, the twenty-first century has witnessed an explosion of applications of 3D scanning and printing in many aspects of STEAM, including of relevance here, fossils and paleontology. This innovation has not just advanced research, but also has positively impacted education and outreach, in both formal and informal settings. The purpose, therefore, of this paper is to describe the suite of programs, activities, and partnerships that we have developed using 3D scanning and printing in the Vertebrate Paleontology program at the FLMNH, University of Florida (UF). As we describe below, our work will hopefully serve both as a model and case study of the effective application of 3D technologies in research and education and collaborations between scientists and educators.
3D Techniques
The 3D techniques section of this paper serves as an introduction to how 3D models are created via CT, surface scans, and photogrammetry as well as the supportive software used for post-processing. This is followed up by elaborating on various open-source 3D repositories (e.g., MorphoSource, myFOSSIL, and Sketchfab) used to store and democratize FLMNH 3D data featured in this paper. Other techniques utilize malleable materials (i.e., plaster, silicon) to create artificial replicas (cast or mold) of fossil specimens. Similar to 3D scanning and printing, the creation of these artificial models helps to preserve data associated with fossil specimens that are considered one-of-a-kind (e.g., plastotype), not feasible to relocate, historically or culturally significant, or prone to erosion (Lucas and Harris, 2019). These traditional techniques are still in use today, but 3D digitization is often favored to capture high-fidelity paleontological data due to non-invasive methodologies and accessible means of distribution (Adams et al., 2010). Thus, it is important to note that this section only features a subset of techniques and complimentary hardware, software, and equipment utilized by the FLMNH to create replicas of fossil specimens and is not an exhaustive list. Rather, it is meant to describe best practices in the creation, application, and dissemination of 3D data for applicable FLMNH research, formal education, and informal outreach activities published on herein.
CT Scans
One of the highest fidelity methods for creating 3D models uses industrial high-power and high-resolution X-ray micro- and nanofocus CT scanning. CT scanning is important for non-destructive study and accessibility of proprietary objects by creating 3D virtual model replicates of paleontological specimens. Many fossilized specimens range from uncommon to one-of-a-kind and are therefore not readily accessible for loan to external researchers or for incorporation into education and outreach initiatives. At FLMNH, all faculty, staff, postdoctoral researchers, and UF graduate and undergraduate students affiliated with the museum have access to the Phoenix V|Tome|X M dual-tube nano-CT (GE Measurement and Control Solutions) scanner at UF’s Nanoscale Research Facility. Training to become a technician of this machine is available for UF affiliates and scanning rates are reasonable for internal users and external researchers (University of Florida Research Services, 2020).
Depending on the scanning requirements for the specimen(s) of interest, a versatile dual-tube CT scanner offers either higher-power microfocus or higher resolution nanofocus tube. The microfocus option allows for penetration of denser specimens (e.g., large fossils and rocks) while the nanofocus option can provide very fine submicron resolution for tiny (<2 mm) and fragile museum specimens, such as fireflies (Dunn et al., 2019). Most often, scanning geological and paleontological specimens requires the high power microfocus tube which allows for a maximum voltage of 240 kV. Standard operating procedures for clear scans require a current (amperage) greater than that of the voltage to remove unnecessary noise in the resulting scan. We have used currents up to 500 μA for very dense and large fossil specimens (e.g., giant armadillo-like glyptodont skull; Figure 1). Since so many X-rays are produced to penetrate dense specimens, thin sheets (0.1–2 mm) of aluminum, copper, or tin are placed at the beam source to filter out nonpenetrating lower energy X-rays which cause light scattering artifacts (du Plessis et al., 2017). Additional methods to avoid unwanted artifacts and noise in resulting scans largely depends on how specimens are packed. Packing materials should be at densities significantly lower than the specimen of interest and measures should be taken to ensure the specimen does not move during the scan. Gravity acts naturally, so allowing a specimen to sit upright in a packed state for at least an hour prior to scanning is imperative.
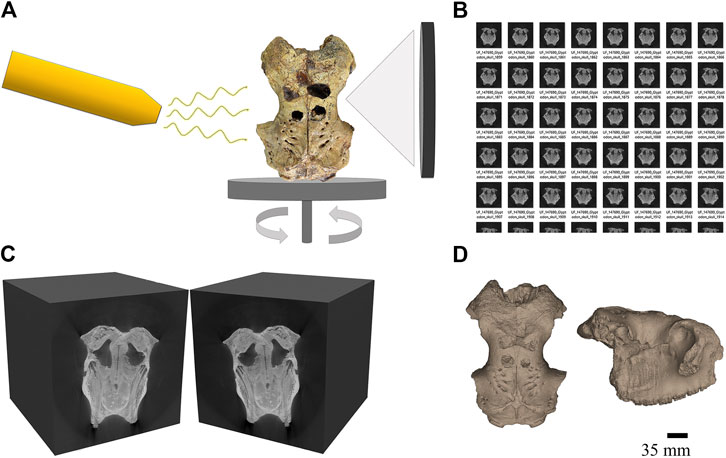
FIGURE 1. Basic CT scanning workflow of a Glyptodon sp. skull (UF-VP-147690) where the black scale bar indicates 35 mm (A) X-rays are penetrating the fossil as it rotates on a plate platform in dorsal view, anterior up. Images are captured on the detector plate opposite to the X-ray source with each rotation of the plate (B) A file folder is produced with hundreds/thousands of tomograms taken from all sides of the specimen and are typically called a TIFF stack because they are composed of. tiff image files (C) Using computer software, the. tiff images can be stacked together and sliced on x, y, or z planes. Here the TIFF stack is sliced in the x plane around the middle of the specimen (D) Using additional computer software, the TIFF stacks can be manipulated to virtually extract the specimen or specific parts of it. Here, a 3D model of the fossil specimen is featured and oriented where: left) dorsal view, anterior up and right) lateral view, anterior right.
Regardless of which tube is used, X-rays are produced from a fixed source that beam towards a rotating specimen with an image detector plate behind the specimen and opposite of the X-ray source (Figure 1). The detector plate captures information in the form of different shades of gray pixels depending on presence or absence of X-rays. Fewer X-rays are able to pass through the densest parts of a specimen while most can easily pass through air. In the produced image, the densest areas of a specimen will appear lightest in color (i.e., teeth) while the surrounding air will appear dark. Fossil specimens should not exceed a width of 350 mm but can easily exceed a length of 350 mm by using the multiscan option and stitching the resulting scans together during post-processing.
Proprietary software, Phoenix datos|x 3D, is used at UF to record digital information from the CT scanning process and reconstruct tomograms for future post-processing use. Tomograms are the two-dimensional (2D) images made up of grayscale pixels that can be stacked to create the virtual 3D model of the physical specimen (Figure 1). CT scanning methods and parameters vary depending on the nature of a specific specimen, but typically a folder is produced with hundreds to thousands of tomograms. Since each image is unique, existing in two dimensions, the specimen may be sliced anywhere in three dimensions (x, y, z planes) allowing for observation and manipulation of internal structures using specialized software programs. These software programs range from open-source to licensed and include, but are not limited to, 3D slicer (Kikinis et al., 2014), Amira/Aviso, ORS Dragonfly, Mimics, and VGSTUDIO MAX. VGSTUDIO MAX is used to post-process CT scans by researchers at the FLMNH’s Digital Discovery and Dissemination (3D) Laboratory (Florida Museum of Natural History, 2020). Several Principal Investigators at the FLMNH hold licenses for VGSTUDIO MAX software. Training is provided by the lab manager, and workshops hosted by Volume Graphics support technicians are generally available once per academic semester.
Surface Scans
Surface scans of fossil specimens are an easy, and relatively affordable way to create reliable 3D replicas. Surface scans use light to create a 3D volume of a surface, effectively scanning the specimen, much like a photocopier scans a page to reproduce it. The surface scans are then converted into a mesh volume using accessory software, usually proprietary to the surface scanner. Prior to printing, the volume can be saved as an object (.obj) or stereolithographic (.stl) file and opened in mesh processing software to repair any potential holes in the mesh. Accompanied by a workflow for creating 3D surface scans (Figure 2), differences between two types of surface scanners and open-source post-processing software will be discussed below. Common troubleshooting resolutions can be found in the Supplementary Materials section of this paper.
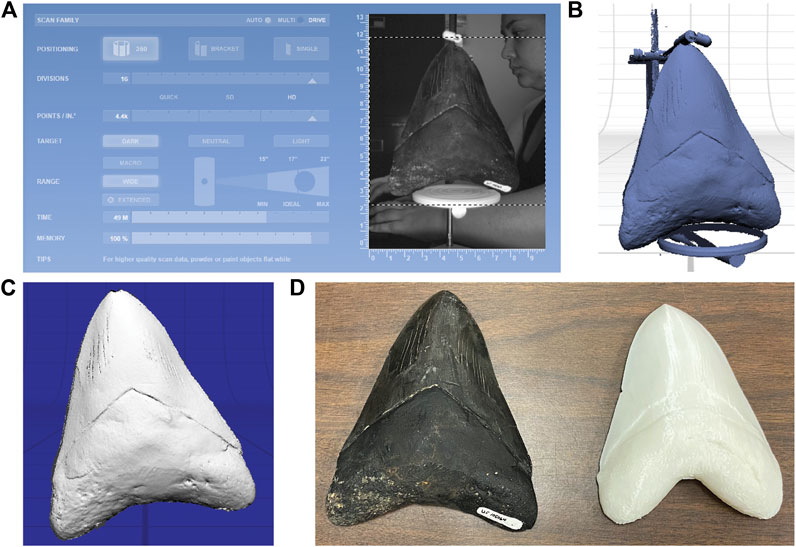
FIGURE 2. Workflow for setting up, scanning and processing a fossil specimen using a NextEngine laser scanner (A) Scan settings to surface scan a tooth of C. megalodon(B) Surface scan of tooth prior to trimming (C) Surface scan of tooth after trimming, preparing for export of surface file (D) Actual specimen (left) next to 3D print of surface scan (right) of C. megalodon tooth.
The NextEngine laser scanner is an affordable desktop scanner that has proven to be effective and accurate in scanning bone geometries (Slizewski et al., 2010). It is also travel friendly, making it a great model for museum and classroom settings. The NextEngine scanner comes with a turntable, allowing for a full 360° scan of a specimen and its own proprietary software, ScanStudio HD Pro® (NextEngine, 2020). The Artec 3D Space Spider (herein: Spider; Artec 3D, 2018) is a structured blue-light LED scanner that provides independence of movement for the researcher to work around large, or difficult to scan specimens (Tokkari et al., 2017; Ritschl et al., 2019). The Spider, although priced higher than the NextEngine, is a handheld scanner that is portable and has shown great results with both soft and hard tissues (Leipner et al., 2019; Ritschl et al., 2019). This scanner also comes with its own proprietary software, Artec Studio (Artec 3D, 2018). Both scanners produce 3D meshes with or without textures, meaning they also capture the color of a specimen, which is converted into a layer that can be applied to the mesh. Similarly, both the NextEngine and the Artec scanner have several file outputs, including. obj and. stl for manipulation and printing.
Even though many surface scanners come with their own software, there are various open-source post-processing software available for download (e.g., MeshLab, MeshMixer, and Blender). As a processing tool, MeshLab is user friendly with a gentle learning curve, but it still proves powerful enough for more experienced users (Cignoni et al., 2008). MeshLab is considered both a mesh viewer as well as a mesh processing application due to its capabilities. In preparation for 3DP, volumes can be cleaned of artifacts, sliced, and any holes in the mesh closed (Figure 2).
Overall, MeshLab has many more capabilities than ScanStudio HD Pro. Surface scans can be measured within the interface, change the scale of a scan, simplify, refine, and clean the meshes, among many other manipulations. Once post-processing is complete, the file can again be exported as an. stl,.obj file, or another file format compatible with the 3D printer.
At the FLMNH, surface scanners and open-source post-processing software are widely used to produce 3D replicas of the external features of a fossil for use in formal education and informal outreach. Specifically, the NextEngine laser scanner was instrumental equipment provided to K–12 teacher participants in the NSF-funded iDigFossils project. Access to surface scanners and 3D printers facilitated collaboration between UF researchers and educators to create multidisciplinary iDigFossils lesson plans that incorporated 3DP in geoscience curricula. For example, one K–12 lesson plan prompted students to print, measure, and interpret the 3D printed dentition of Carcharocles megalodon, to estimate the body size of this massive fossil shark (Mussetter et al., 2020). Three other K–12 iDigFossils lesson plans that utilize 3D data and prints of fossils are expanded upon in the Formal Education section of this paper.
Photogrammetry
Photogrammetry encompasses multiple methods to digitally reconstruct an object or scene as a 3D-rendering by compiling and analyzing a series 2D images with photogrammetric software (Luhmann et al., 2014). As a technique to produce 3D files for printing, photogrammetry is efficient in capturing surficial data of objects and only limited by the quantity, quality, and ability to process images. Photogrammetry also has the unique benefit of reproducing 3D models of objects or scenes at various scales. This scalability makes photogrammetry particularly useful for the geoscience because research objects often range in size from single fossil specimens only visible under a scanning electron microscope (Eilitz and Reiss, 2015) to entire geologic localities (Becker et al., 2018; Francioni et al., 2019). Advancements in complementary image capturing equipment (e.g., affordable digital cameras and drones) make image capturing of vast areas more efficient and the ability to generate photogrammetric 3D models of geologic outcrops possible. Overall, photogrammetry has emerged as a prominent tool in paleontological research since the beginning of the twenty-first century (Otero et al., 2020).
Compared to CT and laser surface scanning, photogrammetry often requires fewer materials (i.e., digital camera, lightbox or light source, and photogrammetric software) and less time to create digital 3D replicas (Westoby et al., 2012). The collection of overlapping images with a digital camera is the initial step crucial to the reconstruction and overall quality of a 3D model produced via photogrammetry (Figure 3). Due to the wide breadth of applications, there are a number of published works that outline best photogrammetric practices in detail, including those pertaining directly to paleontology (see Pruitt et al., 2017). In essence, capturing programmatic data is achieved by compiling a series of 2D images with a standard digital camera that overlap by about 50–66% from the previous photo for a large scene (e.g., fossil trackway, robust specimen, or geologic locality) or offset by 15° for a complete 360° around an isolated or mounted specimen (Falkingham, 2012).
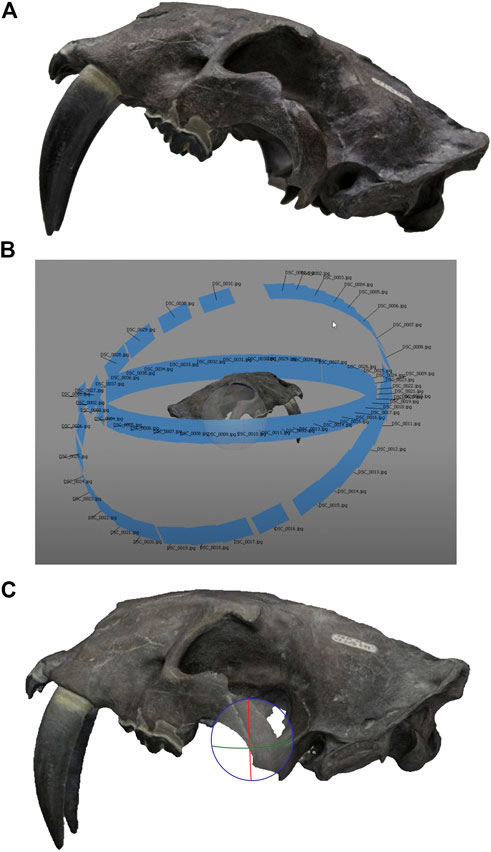
FIGURE 3. (A) Photo image of a Smilodon gracilis skull (UF-VP-206593) from the FLMNH (B)Agisoft Photoscan software displaying the orientation and number of digital photos taken around the fossil specimen after processing the raw 3D data (C) Photogrammetric model of the fossil specimen ready to be shared digitally or 3D printed.
Although photogrammetry is an efficient technique to produce high-fidelity 3D prints compared to CT or surface scans, it is not without its drawbacks. The ability to produce 3D data through photogrammetry, and its overall quality, is heavily dependent on controlling favorable light conditions. This can prove difficult when a specimen or locality is located in an open-air environment or geographically remote setting. Moreover, to preserve quality, images need to be captured and processed in an uncompressed or lossless compression file format like. tiff or, ideally,.raw, when possible because. jpeg compression degrades photogrammetric results (O’Connor et al., 2019). The ability to create a photogrammetric 3D model also is reliant on software to process the amassed images. In the last decade, there has been an influx of proprietary photogrammetric software packages available as open-source or commercial resources ranging in capability and expense and have been reviewed extensively across scientific disciplines (Cipriani et al., 2016; Alidoost and Arefi, 2017; Burns and Delparte, 2017; von Übel, 2020). These software programs include those that offer tiered subscriptions and require payment as compensation for licensed software (e.g., Agisoft Photoscan, Metashape) and those that are open-source and free to download (e.g., Regard3D, 3DF Zephyr). Depending on the software, open-source photogrammetric resources can be limited in function and ultimately affect the quality of the 3D model produced. In order to combat this, a mixed-methods approach can be implemented to retain higher resolution (see Falkingham, 2012 for detailed instructions).
Large-scale projects like the Digital Atlas of Ancient Life with the Paleontological Research Institution and the Idaho Virtualization Laboratory with the Idaho Museum of Natural History have utilized photogrammetry effectively to digitize museum vouchered invertebrate and vertebrate fossil specimens and make them available as open-source research and education materials (Holmer et al., 2014; Ross et al., 2019). A majority of the thousands of files associated with these projects are stored in the online repository, Sketchfab, because it allows the addition of texture and coloration to the 3D model. Similarly, the FLMNH utilizes the Sketchfab platform to make photogrammetric models associated with iDigFossils project lesson plans accessible to the educational, avocational, and research communities and will be discussed in subsequent sections.
3D Repositories
Open-source repositories are utilized to store 3D models created by the FLMNH (i.e., museum grade fossil specimens, geologic localities) and make them readily available for digital download. The advent of 3D data archived for research and other non-commercial use greatly expands the accessibility of replicas to be used for formal K–12 education (e.g., on MorphoSource; Boyer et al., 2016). It also places specimen replicas in the hands of educators and students, specimens that otherwise might be unavailable (e.g., holotypes). Now, K–12 students can hold, manipulate, and study, for example, hominin skulls and jaws on MorphoSource that were previously locked away in storage cabinets (MorphoSource, 2015), the cross section of an enlarged foraminifera microfossils on myFOSSIL (myFOSSIL, 2019), or the ichnofossils of North American dinosaurs on SketchFab (Paleoteach, 2018). Overall, these 3D repositories serve as inclusive educational tools essential to the storage, accessibility, and dissemination of quality 3D geoscience data. Here we will explore a few 3D repositories (e.g., MorphoSource, myFOSSIL, and Sketchfab) that store FLMNH paleontological data for scientific research, formal education, and informal outreach purposes.
MorphoSource
MorphoSource is an online 3D repository composed of digitally vouchered museum-grade biological and paleontological specimens for researchers, museum curators and the general public to virtually explore and download (MorphoSource, 2020). Although initially developed as a source to aggregate research-grade 3D scans, these resources are also frequently being used in K–12 education. User reports indicate that about one-quarter of all downloads of FLMNH specimens are intended for this purpose, which is an outcome likely not envisioned by the researcher who uploaded the fossil, but nevertheless adds value to these endeavors (MorphoSource, 2020). At the FLMNH, the iDigFossils project utilizes digital fossil specimens featured on MorphoSource to create STEAM-integrated K–12 lesson plans. At the time of writing (August 2020), the FLMNH had 8 MorphoSource projects and over 100 3D specimens associated with K–12 lesson plans. This amounted to more than 141,000 views and 8,400 downloads of FLMNH 3D data (Table 1).
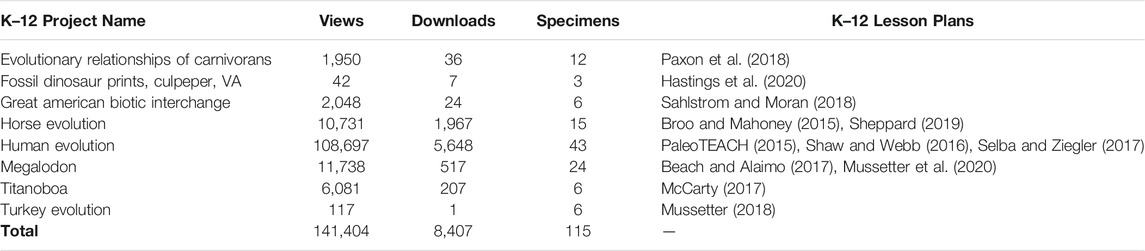
TABLE 1. Breakdown of 3D paleontological data on MorphoSource used in K–12 lesson plans through education and outreach projects at the FLMNH.
myFOSSIL
The FOSSIL project is a community-driven initiative to build a networked Community of Practice (CoP; Wenger et al., 2002) consisting of professional and amateur paleontologists. As of mid-2020, the project has more than 10,000 participants, including 2,000 on the myFOSSIL website and app with another 8,000 connecting via social media. Of relevance to this report, there is a module on the website (FOSSIL, 2020) in which 3D images can be uploaded into a virtual museum called the myFOSSIL eMuseum (mFeM). These images are available for study and can be freely downloaded (e.g., to be 3D printed for non-commercial uses).
Sketchfab
Using the Sketchfab viewer, researchers and citizen scientists can explore the morphology of modern and ancient specimens on their computer (Armit, 2019). Unlike MorphoSource, Sketchfab allows any individual with an account to upload 3D data and does not require specimens to be museum grade. Even so, Sketchfab is heavily used by researchers and educators alike because its platform facilitates interest in museums and cultural institutions by making digital models of artifacts widely available (Krasnodebska, 2015). Moreover, Sketchfab supports photogrammetric data, allowing users to upload 3D models with the original texture and color of an object which can enhance the educational value of and the experience with a digital artifact (Megale and Baione, 2017). Similarly, at the FLMNH, 3D visualizations of paleontological data are aggregated on Sketchfab under the username “paleoteach” and used in education and outreach events and activities through the iDigFossils project. These 3D models range from an isolated specimen like that of the saber-toothed cat, Smilodon gracilis (Paleoteach, 2017) to entire fossil localities (i.e., the recently discovered Florida Miocene-Pliocene locality in Florida called the Montbrook Fossil Site; Ziegler and Grant, 2017).
Applications of 3D Data
Scientific Research
Staff at the FLMNH have conducted and published paleontological research using 3D data derived from CT scans on a variety of different taxa, including primates (Morse et al., 2015; Harrington et al., 2016; Morse et al., 2019) and frogs (Xing et al., 2018; Blackburn et al., 2019a; Blackburn et al., 2019b; Blackburn et al., 2020). Paleontological research currently being conducted at the FLMNH using microCT scanning for non-destructive study of osteological and fossilized specimens includes analysis of density characteristics in lizard tooth enamel to infer diet (Riegler et al., 2019), digitization of porcupine specimens to determine degree of arboreality and taxonomic relationships (Vitek et al., 2020), and virtual extraction of anteater, armadillo, and sloth endocranial casts to infer locomotor strategy from brain shape (Narducci et al., 2019). Once scanned, FLMNH researchers have used the entire 3D object or specifically selected elements from it (e.g., humeri of a frog skeleton, cranial endocasts, crushed skulls) to answer research questions such as those surrounding burrowing ability (Keeffe and Blackburn, 2019) or inner ears of frogs (Singh et al., 2020). Additionally, 3D printed specimens have been used for outreach activities such as color-coded puzzles to compare skulls across vertebrate taxa (Singh et al., 2019).
Other formal research in vertebrate paleontology at the FLMNH is ongoing and researchers have used microCT scanning to answer questions based on internal structures via virtual extraction of endocranial casts from ∼60 million-year-old crown primate skulls to better understand primate brain evolution (Harrington et al., 2016). Here we present a case study on current FLMNH research involving 3D utility of endocranial casts derived from CT scanned skulls of extant and extinct xenarthrans (i.e., anteaters, armadillos, and sloths). Studies of fossil vertebrate endocrania are important to better understand questions surrounding the evolution of brain structures and size through time (Radinsky, 1968; Jerison, 1973; Edinger, 1975). Although, study of fossilized xenarthran endocrania has historically been achieved at the expense of destruction to physical specimens, via natural or artificial infilling which may peel away surrounding cranial bone or require sagittal slicing of skulls for removal (Jerison, 1973). With industrial microfocus X-ray technologies becoming more accessible to paleontological researchers, fossil endocranial study has become less destructive.
In our case study, xenarthrans are used because they are understudied and may be the earliest diverging group of placental mammals (O’Leary et al., 2013). Xenarthrans can also help contribute to our understanding of brain changes with incredible size differences, spanning from the extant tiny pink fairy armadillo, Chlamyphorus truncatus (0.12 kg; Vizcaíno and Milne, 2002), to the extinct giant ground sloth, Megatherium spp (∼4,500 kg; Fariña et al., 1998; Smith et al., 2003; Vizcaíno et al., 2006). The extinct xenarthran giants, including the giant ground sloths and armadillo-like glyptodonts (Figure 4), have no modern analogs, which provide an avenue to investigate several unanswered questions regarding brain size, structure, and function in such unique and massive animals. A diverse range of locomotor habits are exhibited by the unique xenarthran body forms, including fully subterranean, aquatic, graviportal, and suspensory, making them prime candidates for investigation of brain shape differences constrained by locomotion. To investigate questions surrounding the shape of xenarthran brains, the Phoenix V|tome|x M CT scanner at UF was used to scan fossil and osteological skulls from a broad sample of extant and extinct xenarthrans. With the resulting tomographs, 3D endocranial models were virtually extracted using VGSTUDIO MAX software. Biologically relevant and repeatable landmarks were specifically defined and placed on the xenarthran digital endocranial models using VGSTUDIO MAX. Pilot results using multivariate analyses hint at the similarity in patterns to those found in previous 3D geometric morphometric studies on mammalian virtual endocranial models (Ahrens 2014; Bertrand et al., 2019).
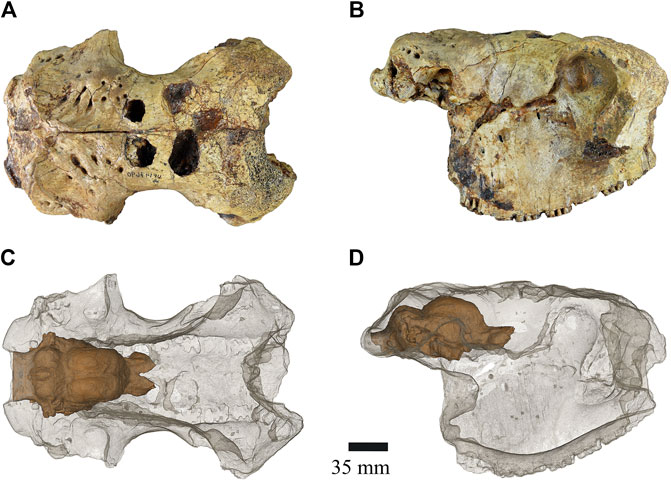
FIGURE 4. Physical (A,B) and virtual (C,D)Glyptodon sp. skull (UF-VP-147690) in dorsal (A,C) and right lateral (B,D) views, anterior to the right, with endocranial cast highlighted in orange. Scale = 35 mm.
Most of the fossilized xenarthran skulls sampled are one of a kind, so without the ability to nondestructively create 3D endocranial models, this study would not exist. Additionally, the rarity of the sampled xenarthran specimens’ limits access to researchers external to the FLMNH who may need to travel in order to study the specimens and for education and outreach initiatives. Since formal research with these virtual xenarthran skulls and endocrania has only just begun, plans for broad dissemination to researchers, educators, and enthusiasts are being formed. Following publication of the study results, all CT scan data and 3D models will be made publicly and freely available for download on MorphoSource and Sketchfab. Plans for incorporating these 3D models and associated results into education and outreach initiatives are also underway, involving 3DP for hands-on examination in a museum exhibit setting with the FLMNH. CT scanning, post-processing, and generating 3D printed models allows these rare xenarthran specimens to be widely distributed both virtually and physically to all interested audiences.
Formal Education
Advancements in 3D modeling techniques (e.g., CT, surface scanning, and photogrammetry) as well as the dissemination of 3D data through online repositories (e.g., MorphoSource and Sketchfab) have facilitated scientific research, formal and informal education, and public outreach. Here, we focus on three lesson plans created through the iDigFossils project as examples of how the FLMNH incorporates 3D paleontological data into formal K–12 education. These STEAM-integrated lesson plans are multidisciplinary, address large-scale concepts in geosciences, and are available for download on the iDigFossils website. As a collaborative project between UF researchers and K–12 educators, iDigFossils lesson plans align with Next Generation Science Standards (NGSS) and the 3D models featured in these lessons are downloadable from open-source repositories like those detailed in the previous section.
Lesson Plan: Dinosaur Trackways
The Luck Stone Quarry in Culpeper, Virginia is home to one of the largest dinosaur track sites in eastern North America (Weems, 1987; Weems, 1992). Two main stratigraphic layers of siltstone have been identified and each document hundreds of footprints; many of these footprints form tracks made by a single individual (Weems, 1992). The site has allowed for studies of body size and locomotion that would not have been possible with more isolated or fragmentary remains (e.g., Dalman and Weems 2013; Bishop et al., 2017). Geologic dating is difficult, requiring correlation of stratigraphic layers between rock outcrops and quarry exposures across the region, but the geologic age is Late Triassic, approximately 211 million years ago (Ma; Weems and Olsen, 1997). While the upper of the two layers has since been removed as part of quarry operations, the lower layer has been set aside while the quarry continues to operate. Due to the nature of the site, it is fully exposed to the elements, meaning wind, rain, and frost are slowly eroding these tracks. Moreover, because this is an active quarry, the public cannot access them except during a once-a-year outreach event which attracts hundreds of visitors each year. Digitization of these tracks allows not only for their widespread use in education, but also their conservation, preserving key morphology before it is lost to time.
As a way to preserve this phenomenal glimpse into the past, photogrammetry models of the dinosaur trackways were created (Figure 5) according to standardized techniques (Matthews et al., 2016). These 3D trackways serve as the core materials used in a K–12 lesson plan, hosted on the iDigFossils website (Hastings et al., 2020). This lesson focuses on the utilization of STEAM practices to measure, interpret, and reconstruct fossilized pathways from the 3D models. For example, after analyzing the geometric details of the fossilized footprints to identify what dinosaur the trackways belong to, students will calculate the height of the dinosaur. To do this, students use 3D data associated with a full-scale model of the dinosaur trackways, an ichnofossil identification guide, and open-source 3D software (e.g., MeshLab, 3DView) to describe, record, and measure the dinosaur trackway 3D models. Inquiry-based activities provide educators an extension to the core activity, prompting students to collaboratively calculate the velocity associated with Culpeper trackway makers and include Supplementary Materials to explain the stratigraphy of the fossil site.
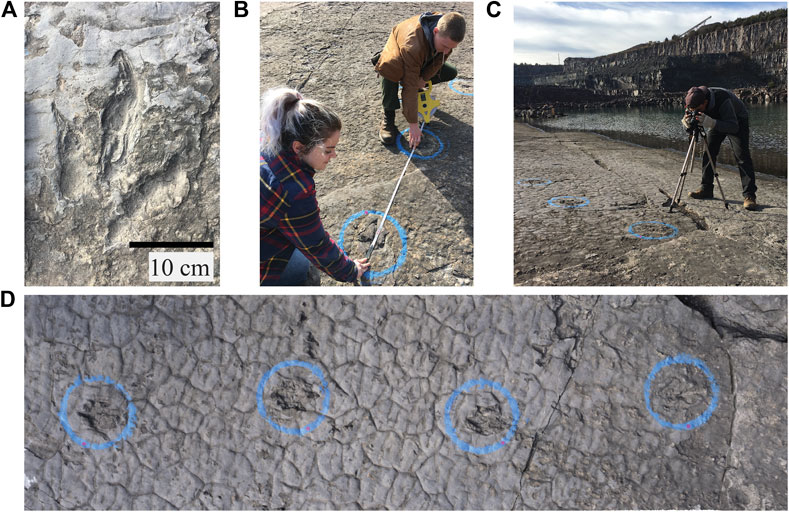
FIGURE 5. The photogrammetric process of capturing 3D digital surfaces of in situ fossil footprints (sourced from original publication; Ziegler et al., 2017) (A) Example of a footprint of the dinosaur ichnotaxon Kayentapus minor at the Luckstone Quarry, part of the K15 trackway (B), Measurements are needed to set the scale of the digital model and verify its accuracy. They also need to be easily found, so adding colorful stickers can make this simpler; Caroline Abbott (bottom); Mark Simonds (top) (C), About 70 well-focused, high-resolution photos need to be taken of each track in a full 360o circle, from multiple angles, including the connecting rock between them; Michael Ziegler (D) The images can then be compiled into a 3D mesh in the software Agisoft Photoscan, which can then be uploaded to Sketchfab and made freely available and used for K–12 education.
Lesson Plan: Great American Biotic Interchange
The Great American Biotic Interchange (GABI) provides what scientists have called a great natural experiment in intercontinental dispersal and faunal intermingling. After about 4‒5 Ma until the end of the Pleistocene, land-mammal evolution in the Americas is characterized by successive waves of dispersals north and south, primarily across the Panamanian land bridge (Woodburne, 2010). This also includes the charismatic megafauna that are gateways to learning about evolution in Deep Time. Starting with the Great American Biotic Interchange - Research Experience for Teachers (GABI-RET) project, teachers worked alongside paleontologists in Panama and then developed lesson plans about GABI immigrants. 3D printed specimens of the charismatic megafauna have been integral to learning about STEAM and building STEM identity (Vargas-Vergara, 2020). During these classroom activities, students printed out scanned images of GABI animals that were used in K–12 lesson plans (Sahlstrom and Moran, 2018). An added benefit of these activities is STEAM integration: whereas many students seemed to be intrinsically interested in the GABI and its megafauna, others were not. However, during a classroom visit, we noted that a subset of the students were relatively disinterested in science, but once the class needed assistance with the 3D technology, the tech-interested students jumped in to help. Thus, the incorporation of 3D data in K–12 lesson plans can create STEAM entry points for students and help facilitate engagement.
Lesson Plan: Horse Tooth Evolution
The horse, or equid, lineage has long been viewed as a model in understanding evolutionary patterns in Deep Time, so much so that many museums have exhibits devoted to depicting apparent morphological change (e.g., increase in tooth crown height, decrease in number of digits) in equids through geologic time (MacFadden, 2005; Janis, 2007; Mihlbachler et al., 2011). Despite this prevalence, the majority of these exhibits are scientifically misinformative and lead to public misconceptions of the fossil record (MacFadden et al., 2012). This is unfortunate as the abundant fossil record and general familiarity of these organisms allows for the development of robust educational material with relatively little effort necessary to delimit biases or scaffold understanding.
With these factors in mind, members of the FLMNH’s vertebrate paleontology division collaborated with high school biology teachers and UF’s Center for Precollegiate Education (CPET). We developed a comprehensive curriculum, aimed originally at high school level biology students and later adapted for nearly all of K-16 education, that uses equids as a model to teach concepts relating to evolution, paleoclimatic change, and geologic time (Bokor et al., 2016; McLaughlin et al., 2016). In the first of three units, students are guided through environmental and paleoenvironmental changes of the Cenozoic of North America, highlighting the general cooling trend from the Paleocene–Eocene Boundary to today and the expansion of grasslands in the late Neogene (Zachos et al., 2008; Strömberg and McInerney, 2011). Once the learners understand these underlying changes over the 55 Myr of horse evolution in North America, their group is provided with 15 3D prints of upper molars that represent a different species of North American horse, from the first known equid, Sifrhippus sandrae, to one of the last surviving species of pre–Columbian equids, Equus fraternus. The surface files of these specimens are archived on MorphoSource (MorphoSource, 2020).
The students are immersed in scientific practice by using calipers to measure several dimensions of tooth size on the 3D printed horse teeth. They then use these measurements to calculate the hypsodonty indices (i.e., a measure of tooth crown height) of all 15 horse species and plot these measurements through geologic time. Through this practice, and open discussion, the learners draw connections between the increase in hypsodonty in horses, as well as other characters such as the loss of toe digits, and paleoenvironmental shifts to grassland-dominated ecosystems and the related transition of horses from browsers to grazers (Wang et al., 1994). The last two units of the curriculum, though less reliant on 3D representation, expand on the foundation set in the first by focusing on microevolutionary mechanisms and the resultant complex phylogenetic tree. Preliminary analyses showed the curriculum fostered meaningful discussion and inquiry and led to a marked increase in understanding of concepts presented in the lesson (McLaughlin et al., 2016).
iDigFossils Empirical Data
As an emerging technology in K–12 education, 3DP has gained much attention from educators and researchers (Bull et al., 2013). However, meaningful 3DP integration in K–12 science curricula is still scarce, and little is known about how 3DP in science classrooms may influence student learning outcomes such as motivation and self-efficacy to learn science. A recent study examined the influence of 3DP and STEM integration in science classrooms on students’ STEM motivation, which is essential for students’ academic experiences and future careers (Cheng et al., 2020). The study sample included 26 K–12 science teachers across six states in the United States and 1,501 students who engaged with STEM learning using 3DP in the context of paleontology. Teachers’ lesson plans were analyzed to examine 3DP and STEM integration levels. Teachers’ beliefs and students’ STEM motivation were assessed with previously validated scales (Unfried et al., 2015). Multilevel modeling analyses indicated that while teachers’ beliefs and 3DP integration levels did not significantly predict students’ STEM motivation, teachers’ STEM integration levels facilitated by the use of 3DP technology positively predicted students’ math motivation (Cheng et al., 2020). This study also found that teachers’ STEM integration levels positively interacted with student gender for students’ science motivation (ɣ = 0.09, p = 0.01), indicating that female students’ motivation for science was impacted to a higher extent as a result of teachers’ STEM integration. This finding is interesting as research suggests that male students are typically more interested and motivated to learn STEM than female students (Wang and Degol, 2013).
Another recent study followed a 6th grade science teacher who designed and implemented a science learning activity focusing on the structure and function of shark teeth integrating 3D scanning and printing practices that are used by paleontologists to identify and study fossils (Antonenko et al., 2018). The NGSS-aligned lesson was designed using the Biological Sciences Curriculum Study 5E model, spanned five 50-minute class periods and addressed the following guiding question: “What biotic and abiotic factors have changed shark’s teeth over time?” The lesson included four main activities: a) sorting shark teeth to bring out students’ initial conceptions regarding classification, b) re-sorting shark teeth by shape and inferring their corresponding function and diet, c) using shark teeth as index fossils to determine geologic age and inferring climate change in functions through time, and d) scanning and printing lamniform shark teeth (e.g., Otodus obliquus, Carcharocles megalodon, Carcharodon hastalis, Carcharodon carcharias, Isurus oxyrinchus). Qualitative and quantitative measures were used to obtain a comprehensive view of how students and the teacher perceived the integrative STEM activity and the effects on their learning, self-efficacy, development of twenty-first century skills, and career interest. The data sources included student and teacher interviews, classroom observations using the Practices of Science Observation Protocol (Forbes et al., 2013) and the ISTE Classroom Observation Tool (Elmendorf and Song, 2015), student and teacher artifacts, and the S-STEM survey (Unfried et al., 2015).
Qualitatively, the study found that while only half of the interviewees were interested in science careers, all students showed increased interest in 3D scanning and printing and the careers that used these practices. All students spent much longer on 3D scanning than printing. Half of the students were able to recall 90% of the relevant science concepts from prior units. Quantitatively, students also exhibited significantly better attitudes and self-efficacy toward science learning and competency in twenty-first century skills. Students improved in their interest towards three STEM careers: Biology, Computer Science, and Medical Science. Girls demonstrated significantly higher science attitude scores than boys on the posttest of science attitudes that was conducted after they engaged in this multi-day STEM activity (F = 7.64, p = 0.007, ŋ2 = 0.08). A factorial ANOVA was conducted with the eight science attitudes scale items that reflected higher scores for girls compared to boys. The overall model produced notable differences (F9,84 = 2.24, p = 0.027, ŋ2 = 0.19) between girls and boys on 4 of 8 items (Table 2).
Another educational study funded by the iDigFossils project, was designed to explore if and how STEM identity develops after six months of implementing integrated STEM activities using 3D scanning and printing, through the lens of paleontology (Vargas-Vergara, 2020). This study was conducted with a group of minoritized high school students in California who were left behind by the traditional school system resulting in different learning struggles, among other issues that impact student’s motivation (Fine, 1991) in STEM learning. Over a period of six months, student participants were introduced to the use of NextEngine scanners and LulzBot 3D printers while 3DP and reconstructing the skeleton of the extinct snake Titanoboa. During this time, students 3D scanned a variety of fossils in order to learn the scanner settings. They also learned about topics of societal impact such as climate change (Head et al., 2009), and understood the connections between paleontology, geological sciences, biology, and mathematics. Engagement was high due to the novelty in technology and the use of a charismatic animal such as Titanoboa. But, how can we guide students from engagement to development of their STEM identity?
According to Vargas-Vergara (2020), findings through data analysis of Identity Maps (Futch and Fine, 2014), semi-structured interviews, and Pre- and Post-Survey, revealed that recognition, competence, performance, and perceptions by others are important needs related to STEM identity development. The transition from engagement to intrinsic motivation and ultimately STEM identity development is possible if students have freedom of speech and expression, which is made possible through a variety of assignments rather than just a standardized testing and memorization, as well as a combination of justice, fairness and honesty provided by teachers and schools. Fostering autonomy, competence and relatedness (Deci and Ryan, 2000) are crucial to understand how students’ goals operate and fluctuate between different contexts like their science classroom and home, for example. Further findings showed that, teacher and school support, in addition to instructional strategies were the most prominent determinants of student engagement and STEM identity development (Vargas-Vergara, 2020). These findings reflect principles of Human Motivation Theory (Maslow, 1943) and the use of universal Design Learning framework (UDL; CAST, 2020) for curriculum design. The design of Titanoboa activities using UDL principles was extremely valuable as it removes barriers and maximizes learning (CAST, 2020). This was evident when students interested in pursuing an art career witnessed how 3D technologies support not just scientific research, but also visual arts. Consequently, fueling interest and engagement in STEM by bridging artistic and creative identities through the use of 3D technology is more inclusive.
Informal Outreach
Museum Activities and Events
The FLMNH engages the public through exhibits and community outreach programs, which can broadly be referred to as informal education. The reach of the FLMNH informal education efforts expands from the physical institution to the local Gainesville community and beyond, outside of the state of Florida. The content for these informal education experiences is derived from the research and formal education practices employed by the museum. To make this content accessible to broad audiences, museum educators translate the processes and outcomes into digestible narratives. Given the utility of 3D technology in research and education described above, it should come as no surprise that 3D technology is inherently nestled within the FLMNH informal education efforts as well. 3D models offer low-cost materials for exhibits and serve as tangible objects for visitors to interact with.
In 2017, the FLMNH celebrated its 100-years anniversary with a temporary exhibit, entitled Rare, Beautiful and Fascinating: 100 years @FloridaMuseum, highlighting the diverse collections and practices associated with the museum. Among these remarkable natural history objects and specimens, a 3D printer was constantly at work printing models of various objects on display. The use of 3D models has become so ubiquitous across natural history disciplines, that it reflects one of the major practices associated with the museum. This simple act of producing a 3D model captivated museum visitors and provided them experience with the applications of cutting-edge technology in research and education. The presence of 3D models was even included in the advertisement for this event, “Hands-on stations will allow guests to examine museum artifacts, specimens and 3-D printed replicas.” (Gardiner, 2017).
During each week of the 100-years anniversary, a different range of the museum occupied a portion of the exhibit space to interact directly with the public (Figure 6A). The vertebrate paleontology range of the museum offered two hands-on activities that related directly to applications of 3D technology. The first activity asked museum visitors to compare the different information captured in a 2D photograph versus a 3D printed model of a fossil. This activity allowed visitors to conceptualize the benefits and limitations of different types of models, which serve as representations of the actual fossil specimen. In a high-resolution 2D photograph, color and fine details are observable, whereas, in a 3D printed model the overall shape and size are more apparent. Neither model accurately represents the chemistry or density of the actual specimen. Thus, neither model is necessarily better than the other, rather which model used is dependent on the intended purpose.
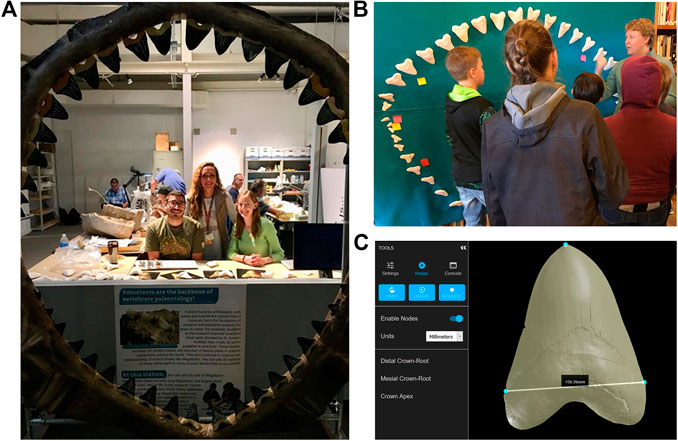
FIGURE 6. (A) FLMNH vertebrate paleontology public exhibit for the museum’s 100-years anniversary, showcasing the use of 3D printing in research and education (B) FLMNH graduate student, Sean Moran, leads a lesson on estimating the body size of C. megalodon at the El Rito Library in New Mexico (sourced from original publication; MacFadden, 2019) (C) A digital interface on MorphoSource to place points and record linear measurements.
In the second activity, museum visitors estimated the body length of the extinct shark, C. megalodon, by measuring tooth dimensions from 3D printed models of an extremely rare associated dentition. Without 3D technology, this rare fossil specimen would not be accessible to the public to physically interact with. Visitors engaged in authentic scientific research by testing a published method for estimating body size of C. megalodon (Shimada, 2002) and collectively disproving it. They did so by first comparing the isolated 3D printed teeth to a reconstructed model of the entire associated dentition, taking measurements, and then applying some basic linear algebra. This activity was derived from a formal K–12 lesson described in Grant et al. (2016), showcasing both the scientific research and education efforts conducted at the FLMNH. This same lesson has been repeatedly adapted and implemented for different venues and audiences. The light weight and low cost of printing the 3D C. megalodon dentition make it ideal for traveling exhibits.
In November 2017, the vertebrate paleontology range of the FLMNH partnered with several libraries in Rio Arriba County, New Mexico to provide inquiry-based education and outreach to the local communities. At one of these events, local elementary-aged children were guided through the C. megalodon body length lesson, using the 3D printed dentition. In a novel application of the curriculum, the children attending the event used Velcro affixed to the back of the 3D printed teeth to reconstruct the dentition of the shark on felt (Figure 6B). In 2018, the FLMNH setup a pop-up museum exhibit for the opening weekend of the movie The Meg (Turteltaub, 2018) outside a local theatre in Gainesville, FL. Visitors to the pop-up museum were again able to interact with the 3D printed dentition of C. megalodon to estimate its body size. This gave visitors a scientific perspective on the actual body size of this extinct macropredator before seeing the grossly overestimated depiction in the movie. These FLMNH pop-up museums often only allot for brief interaction times with visitors (Perez et al., 2020). To account for the brevity of the interactions, visitors entered their measurements into an RShiny application (Wood, 2016), to calculate body length rather than doing the linear algebra themselves. At both events, the experience culminated with the participants reconstructing the C. megalodon dentition.
Individuals gain a visual perspective for the immense size of C. megalodon by reconstructing the dentition. This visual representation provides room for artistic freedom in how the dentition is displayed. Further, many formal educators have taken the concept of reconstruction further than 3DP teeth on felt by recreating the jaws using materials like paper mâché, plaster, pool noodles, and paint. In fact, the dentition shown on display at the 100-year anniversary in Figure 6A was created by a group of middle school students and their teacher, Megan Hendrickson, at the Academy of the Holy Names in Tampa, Florida. The act of designing and building the dentition incorporates engineering practices, which round out this lesson as a fully STEAM integrated experience.
During the global COVID-19 pandemic, formal classroom education was converted almost entirely to virtual informal education. Consequently, educators of all kinds required digital resources to supplement their typical teaching materials. The digital files for the associated dentition utilized in the C. megalodon body length lesson are reposited on MorphoSource. To facilitate a fully digital adaptation of this lesson, the web developers at MorphoSource created an interface to take linear measurements within their digital repository (Figure 6C). In July 2020, K–12 teachers participating in a virtual professional development workshop hosted by the UF Thompson Earth Systems Institute were able to test and contribute design suggestions for this digital interface. Moreover, all other iDigFossils lesson plans featured in this paper are ready for download and prepared to be implemented in an online learning environment. This truly speaks to the versatility of 3D data as educational resources in diverse settings.
Virtual Field Trips
Another innovative application to visualize and share 3D geoscience data is through a virtual field trip (VFT). At its core, VFTs utilize 3D models to provide an experience similar to an in-person field trip where the individual can view and interact with digital renderings of physical features and feel what it might be like to visit a location (Nix, 1999; Maskall and Stokes, 2008; Mead et al., 2019). In K–12 education, VFT environments have been used as extensions to field experiences for educators as part of professional development (Granshaw, 2011) as well as an alternative to in-person field trips for students. As supplemental educational materials in K–12 and college classrooms, VFTs can promote collaboration between educational institutions, multidisciplinary learning, and increased access to international areas of interest (Tuthill and Barbara, 2002; Jacobson et al., 2009). Puhek et al. (2012) reported that K–12 students tend to have a positive opinion about VFTs in preparation for an in-person field trip, but a majority of students do not want VFTs to completely replace the in-person experiences. Even so, sometimes education and outreach field experiences cannot take place in-person and must be replaced with VFTs (Chan et al., 2002). This is especially true when studying remote locations, working with students with physical disabilities, or by the complexity of real-world environmental settings (e.g., COVID-19 worldwide pandemic).
VFTs have been used as a twenty-first century tool in paleontology classrooms and research labs to equip a new generation of researchers and educators with high-fidelity 3D models of geologic localities and fossiliferous sites and enhance open-source science (Yacobucci and Lockwood, 2012; Nesbit et al., 2020). The Eastern Pacific Invertebrate Communities of the Cenozoic (EPICC) initiative through the University of California Museum of Paleontology is an exemplary project aimed at creating immersive open-source virtual fieldwork experiences. Online, EPICC provides various modules to explore the processes of science as well as specific fossils, sediments, and regional geology of research localities (EPICC, 2020). As 3D techniques evolve, the capabilities of VFTs expand and are able to incorporate interactive features that reflect in-person field trips and authentic fieldwork experiences (Figure 7).
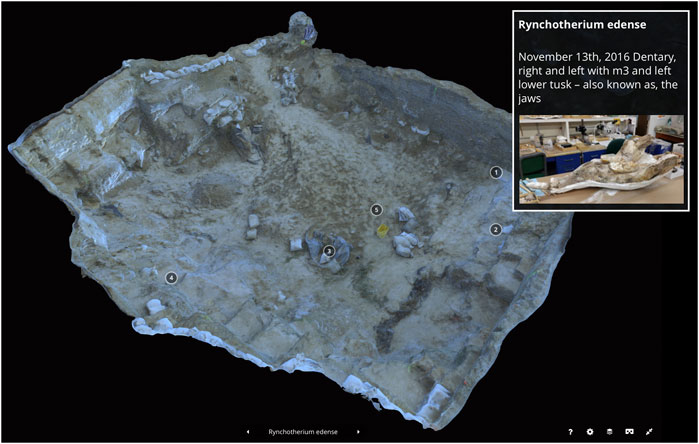
FIGURE 7. 3D Sketchfab model of a prolific fossil site in Florida after the 2016 field season. Additional data is superimposed onto the 3D model and obtainable by clicking on the numbered plot within the locality. An example of this expandable window features the dentary of the North and Central American proboscidea, Rhynchotherium edense.
At the FLMNH, the FOSSIL project reports that field trips and fieldwork is one of the most prominent shared interests between amateur and professional paleontologists amongst their online community (Crippen et al., 2015b). An authentic paleontological field experience hosted by the FLMNH permitted 15 local K–12 educators to excavate for extinct fauna at the Montbrook Fossil Site in Florida and proved to be an impactful professional development with rewarding learning experiences (MacFadden et al., 2020). Similarly, creating quality VFTs is an effective way to foster amateurs’, students’, and the public’s interest(s) in geoscience research. At UF’s environmental virtual field laboratory in the soil and water department is doing just that. They have created a 3D soil-landscape model and complementary VFT to help students learn about the spatial distribution of soils and their properties (Ramasundaram et al., 2005). The 3D modeling of field observations and creation of VFTs are particularly conducive to this type of geoscience research because the subsurface nature of their studies can be limiting. As a means to increase interactivity, VFTs often feature an outcrop with clickable high-resolution images of in situ fossils or geologic features superimposed onto the 3D model (Ross et al., 2007; Mead et al., 2019). Once selected, data (e.g., text, images, video, or hyperlinks) can be viewed to identify, explain, or pose a question about the isolated section (Figure 7). For example, photogrammetric models of the FLMNH fossil locality, Montbrook, have clickable features that elucidate significant fossil discoveries found during the 2016 field season by zooming in on a particular area (Ziegler and Grant, 2017).
Problems and Challenges
Although there are many potential benefits of using 3D technology in outreach, formal, and informal education, there is still much to learn about the effectiveness of these types of interventions with a variety of learning styles and abilities. One major consideration for the utilization of 3DP both in and out of the classroom is the impact that varying levels of spatial reasoning have on the success of these types of lessons. Some researchers have begun evaluating the implementation of lesson plans that use 3D-printed resources to determine which students benefit from the inclusion of such models into curricula. Huk (2006) found that only students with high spatial ability benefitted from inclusion of the 3D models into the curriculum and suggests that students with low spatial ability might become cognitively overloaded when using the models. Despite what this study suggests, other researchers have found conflicting evidence. Some studies have found that the direct manipulation, as opposed to passive viewing, offered through the use of 3D prints positively impacts student learning outcomes, and that manipulation benefits students with low spatial ability more than students with high spatial ability (Lee and Wong 2014; Jang et al., 2016). Haptic and tactile learning materials have been found to benefit a variety of students with various learning styles (Jones et al., 2005; Martinez et al., 2016; Pierce, 2016; Backhouse et al., 2018). Despite contrasting evidence on the effectiveness of these types of lessons, educators are incorporating 3D technology, both digital 3D models as well as 3D prints, more and more into their curricula in order to address morphologically complex topics in a way that they have been unable to do before.
Another concern of working with 3D prints in the lab and in the classroom is the fidelity of 3D prints and scans. Especially in a research context, reliable and high-fidelity prints are important for lessons, labs, or experiments where measurements are being taken. The highly processed nature of the digitization and fabrication process can impact resolution and introduce errors, including issues with overall scale, distortion of the surface, artifacts of 3DP, among others (Mallison, 2011; Lautenschlager and Rücklin, 2014). Mallison (2011) warns that if the digital files are reduced in size at any point in the process, it can cause digital erosion or lose sharp edges and surface texture. Furthermore, the “output is only as good as the input” (McMenamin et al., 2014), so even without a reduction in size, a print can be poor if the original 3D file is of low quality. Although the quality of the prints and associated artifacts of the 3DP process will also vary significantly based on the type of printer, printing medium, as well as a variety of external factors. Early research indicates that it is now possible to achieve high-fidelity prints with desktop 3D printers. Peterson and Krippner (2019) compared fidelity in the digitization and 3DP of vertebrate fossils and found that, while differences in digitization methods and 3D printed models existed, they were virtually indistinguishable. The main differences between the 3D prints and the original fossils were slight distortions in flat-shaped and tabular objects. Overall, the prints were still extremely successful for use as teaching and outreach aids.
Additionally, the 3D printed versions of various paleontological specimens differ in many of the physical properties inherent to the original fossils, including weight, density, color, and internal structure (Mallison, 2011). Some aspects of the fossils, such as scale, can be purposely modified to allow tiny specimens to be seen with the naked eye (Rahman et al., 2012). However, this can sometimes be confusing in education/outreach scenarios, where a trained volunteer or docent would need to inform audiences about the adjustments of the scale. The size of the print is also limited by the size of the printer bed, making it difficult to print large fossils to scale. One potential solution to this problem is to crop the 3D file into sections using a program like MeshLab or MeshMixer, or in a file slicing program like Simplify3D or Cura and print each of the sections individually. Once all of the sections are printed, they can then be re-assembled and glued together to achieve a larger specimen that is printed to the appropriate scale (see Hegna and Johnson, 2016 for an example protocol). However, the gluing together of pieces post-printing can cause additional raised ridges and discolorations of the surface. Any of these adjustments has the potential to confuse or misinform whoever is looking at the 3D print and can result in misperceptions of the morphology of the specimen.
The cost of the technology needed to use 3D files or utilize rapid prototyping is another potentially prohibitive aspect of using 3D data. To manipulate, view, and post-process data such as CT scans, a computer with ample memory and RAM is needed. The need for this type of expensive technology can limit the accessibility of 3D data and can exacerbate the inequities caused by the digital divide (Cullen, 2002; van Dijk, 2006). Additionally, limited access to 3D printers and 3DP materials due to expense (Mallison, 2011; Lautenschlager and Rücklin, 2014) can contribute to the digital divide and opportunity gap. Consumer-grade 3D printers can range in price from a few hundred dollars to several thousand, with professional printers costing more than $250,000 in some cases (Walker and Humphries, 2019). However, as printers have become more and more popular and the technology has continued to improve, 3D printers are now more affordable than ever (Hasiuk, 2014). What was once a technology only available to a select few who could afford the high price point is now able to be used in classrooms, museums, and labs around the world.
In regard to formal education, a significant challenge faced is that geosciences do not enjoy the same attention attributed to biology, physical sciences, and math within K–12 curricula (McNeal, 2010; Pickering et al., 2012). This is attributed to applied sciences not being considered “high stakes” on assessments, leading many administrators to not focus personnel and financial support (McNeal, 2010). Additionally, geosciences incorporate challenging emotional and political topics like evolution and climate change, which to some K–12 educators these topics can present difficulties if they lack thorough professional development (Drewes et al., 2018). It has been shown that through professional developments on geosciences topics, participating K–12 teachers improve their competence, reflecting directly in their students’ quality of learning (Hestness et al., 2014; Drewes et al., 2018). Still, professional development opportunities are not always readily available to educators. Similarly, limited exposure to quality geoscience education in K–12 classrooms may contribute to a lack of interest in geosciences as a career due, in part, to it being considered low-prestige and low-paying occupation amongst students (Lyon et al., 2019). Even so, more concerted efforts to provide geoscience projects to high school students in both formal and informal settings report an increase in awareness and knowledge about the geosciences as a whole, as well as an increased interest in geosciences as a career goal (Schmidt, 2013; Houser et al., 2015).
Benefits and Future Directions
Democratizing science and access to research specimens is the process through which scientific knowledge and resources are made freely available to the general community without marginalizing any population of people (McCormick, 2007). Democratizing science does not stop at the community level, but encompasses the rest of the world, including developing nations (Hughes et al., 2015). Distributions of scientific advances often occur via press release or accessing scientific papers online. Access to these resources require suitable telecommunications infrastructure and technology, and although much has changed in the last decade, there is still insufficient access in rural and developing communities (Cullen, 2002; Kaliisa et al., 2019). A quick solution to this problem is to provide virtual workshops at public spaces (i.e., local libraries and schools) equipped with sufficient telecommunications services and technologies.
One way to make geosciences more accessible to K–12 educators and students is through 3D technology. 3D modeling of terrain has a long history in the geosciences, from the first relief globes of the Earth in 1752 (Destombes, 1978), to the use of lunar models for the Apollo landing in the 1960s (Horowitz and Schultz, 2014). However, it has not been until the last decade that a wider introduction of 3DP into geoscience classrooms has occurred, covering topics ranging from topography, to tectonics and paleontology (Reyes et al., 2008; Grant et al., 2016; Koehler, 2017; Kyriakopoulos, 2019). This portability allows for paleontological projects to enter K–12 classrooms (iDigFossil, 2020). Several researchers advocate incorporating more 3DP in geoscience related lessons (Hasiuk, 2014; Horowitz and Schultz, 2014; Casas and Estop, 2015; Ford and Minshall, 2016; Ishutov et al., 2017; Buckley et al., 2019; Murrey, 2019), but there is little published on whether these lesson plans influence students’ interests. In this paper, we describe how Grant et al. (2016) used 3D printed C. megalodon teeth to create a lesson on body length. The researchers saw an increase in student engagement and recommend using 3D printed fossils as a way to develop future STEAM-inclusive content knowledge in difficult topics like evolution and climate change. As a tactile component to geoscience lesson plans, 3D printed specimens can open up educational avenues for differently abled audiences to participate (Buehler et al., 2015). For example, Teshima et al. (2010) used 3D printed scaled-up skeleton models of radiolarians and foraminifera and presented them to visually impaired high school students. Most of the students agreed that the models were useful in understanding the shapes of radiolarians and foraminifera. Similarly, Koehler (2017) examined how visually impaired middle school students understood the concept of plate tectonics with the aid of 3D printed models. Although there was no difference either positively or negatively regarding the students’ understanding, they did learn additional information from the models based on how they were able to interact with them.
In addition to printing objects for K–12 classrooms, museums also utilize 3D technology to digitize natural history collections. The adoption of 3D technology by museums is not just in the realm of printing objects, but in digitizing their collections. By preserving physical collections in the form of 3D data, and making them available via open-source repositories, the public now has access to a tremendous amount of scientific 3D material (Mitsopoulou et al., 2015; Short, 2015; Boyer et al., 2016). However, having available 3D resources and technology will not solve all of the problems. 3D data and Supplementary Materials need to be made accessible by researchers (Lewis, 2019) with detailed guides in multiple languages for non-English speakers (Dong et al., 2009; Taylor et al., 2018). Creating detailed guides can be time consuming, but they can promote a sustained interest in the material and help build transferable skills. Still, democratizing science and providing access to data is every scientist’s duty. By providing material that is accessible and easily understood by broad audiences, we can effectively communicate research and generate more positive sentiments towards science (MacFadden, 2019).
Conclusion
Advances in 3D techniques (e.g., CT, surface scans, photogrammetry), in concert with supportive 3D technologies (e.g., hardware, software, equipment) during the twenty-first century, have facilitated the creation of quality 3D geoscience data. The application of 3D data at the FLMNH promotes advances in paleontological research and complements formal education and informal outreach. In this case study, innovative research on xenarthran endocrania at the FLMNH was made possible through non-destructive CT scanning. Other 3D models created via surface scanning and photogrammetry enable virtual experiences with fossil localities and museum specimens. 3DP these high-fidelity replicas places fossil specimens in the hands of educators and students, many of which would be otherwise inaccessible (e.g., delicate fossils, holotypes, remote localities). Moreover, utilizing open-source repositories (e.g., MorphoSource, myFOSSIL, Sketchfab) aggregate 3D paleontological data from the FLMNH and provide an inclusive avenue for the digital dissemination of research-grade 3D models worldwide. This helps to reduce the opportunity gap and digital divide prevalent in K–12 education. Specifically, lesson plans and 3D data of paleontological specimens created through the iDigFossils project has been instrumental in encouraging the next generation of scientists, democratizing authentic K–12 geoscience curricula, and engaging with the public through outreach.
Author Contributions
MZ: Co-author contributed to the Introduction, 3D Techniques: Photogrammetry, 3D Repositories: MorphoSource, 3D Repositories: Sketchfab, Virtual Field Trips, and Conclusion sections. Co-author composed Figure 3, Figure 7 and Table 1. VP: Co-author contributed to the Introduction and Informal Outreach sections. Co-author composed Figure 6. JP: Co-author contributed to the 3D Techniques: Surface Scan and Benefits & Future Directions sections. Co-author composed Figure 2. RN: Co-author contributed research data and to the 3D Techniques: CT Scans and Application of 3D Data: Scientific Research sections. Composed Figure 1 and Figure 4. SM: Co-author contributed to the Formal Education: GABI and Formal Education: Horse Tooth Evolution sections. MS: Co-author contributed to the Problems & Challenges section. AH: Co-author contributed to the Formal Education: Dinosaur Trackways section. Co-author composed Figure 5. CV-V: Co-author contributed research data and to the Empirical Data section. PA: Co-author contributed research data and to the Empirical Data section. Co-author composed Table 2. BM: Co-author contributed to the Statement of Purpose, 3D Repositories: myFOSSIL, and Formal Education: GABI sections
Funding
This paper describes activities and outcomes that were directly or indirectly supported by several NSF awards, including: DRL 1322725 (FOSSIL), EAR 1358919 (GABI-RET); DRL 151040 (iDigFossils); DRL 1748347 (FOSSIL Supplement), DGE 1315138, DGE 1842473 (GRFP).
Conflicts of Interest
The authors declare that the research was conducted in the absence of any commercial or financial relationships that could be construed as a potential conflict of interest
Acknowledgments
This is University of Florida Contribution to Paleobiology (No. 878). Material is based upon work supported by the National Science Foundation Grants: DRL 1322725 (FOSSIL), EAR 1358919 (GABI-RET); DRL 151040 (iDigFossils); DRL 1748347 (FOSSIL Supplement), DGE 1315138, DGE 1842473 (GRFP). Any opinions, findings, and conclusions or recommendations expressed in this material are those of the author(s) and do not necessarily reflect the views of the National Science Foundation.
Supplementary Material
The Supplementary Material for this article can be found online at: https://www.frontiersin.org/articles/10.3389/feart.2020.600696/full#supplementary-material.
References
Adams, T. L., Strganac, C., Polcyn, M. J., and Jacobs, L. L. (2010). High resolution three-dimensional laser-scanning of the type specimen of Eubrontes (?) Glenrosensis shuler, 1935, from the comanchean (lower cretaceous) of Texas: implications for digital archiving and preservation. Palaeontol. Electron. 13, 3.
Ahrens, H. E. (2014). Morphometric study of phylogenetic and ecologic signals in procyonid (mammalia: carnivora) endocasts. Anat. Rec. 297, 12. doi:10.1002/ar.22996
Alidoost, F., and Arefi, H. (2017). Comparison of UAS-based photogrammetry software for 3D point cloud generation: a survey over a historical site. ISPRS Ann. Photogramm., Remote Sens. Spat. Inform. Sci. W4, 55–61. doi:10.5194/isprs-annals-IV-4-W4-55-2017
Antonenko, P., Cordero, M., Grant, C., Luo, W., MacFadden, B., Perez, V., et al. (2018). “Shark teeth and 3D technologies: learning science using the authentic practices of Paleontologists,” in Proceedings of the conference of the American educational research association symposium “supporting learning of science and technology through novel applications of technology, New York, NY, April 13–April 17, 2018.
Armit, C. (2019). Lost worlds: via Sketchfab a 3D fossil offers a glimpse of prehistoric marine Life. Available at: http://gigasciencejournal.com/blog/sketchfab-3d-fossil-seabed/ (Accessed July 1, 2020).
Artec, 3D. (2018). Artec studio professional. Available at: https://www.artec3d.com/3d-software/artec-studio (Accessed March 20, 2020).
Backhouse, S., Taylor, D., and Armitage, J. A. (2018). Is this mine to keep? Three-dimensional printing enables active, personalized learning in anatomy. Anat. Sci. Educ. 12, 1–11. doi:10.1002/ase.1840
Beach, L., and Alaimo, G. (2017). A comparison of Carcharodon carcharias (great white shark) to Carcharocles megalodon. Available at: http://www.idigfossils.org/a-comparison-of-carcharodon-carcharias-white-shark-to-carcharocles-megalodon/ (Accessed August 1, 2020).
Becker, R. E., Galayda, L. J., and MacLaughlin, M. M. (2018). Digital photogrammetry software comparison for rock mass characterization. Alexandria, VI: American Rock Mechanics Association. Vol. 18, 1211.
Bertrand, O. C., San Martin-Flores, G., and Silcox, M. T. (2019). Endocranial shape variation in the squirrel-related clade and their fossil relatives using 3D geometric morphometrics: contributions of locomotion and phylogeny to brain shape. J. Zool. 308, 197–211. doi:10.1111/jzo.12665
Bishop, P. J., Clemente, C. J., Weems, R. E., Graham, D. F., Lamas, L. P., Hutchinson, J. R., et al. (2017). Using step width to compare locomotor biomechanics between extinct, non-avian theropod dinosaurs and modern obligate bipeds. J. R. Soc. Interface 14, 132. doi:10.1098/rsif.2017.0276
Blackburn, D. C., Keeffe, R. M., Vallejo-Pareja, M. C., and Vélez-Juarbe, J. (2020). The earliest record of caribbean frogs: a fossil coquí from Puerto Rico. Biol. Lett. 16, 4. doi:10.1098/rsbl.2019.0947
Blackburn, D. C., Paluh, D. J., Krone, I., Roberts, E. M., Stanley, E. L., and Stevens, N. J. (2019a). The earliest fossil of the african clawed frog (genus Xenopus) from sub-saharan africa. J. Herpetol. 53 (2), 125–130. doi:10.1670/18-139
Blackburn, D. C., Roberts, L., Vallejo-Pareja, M. C., and Stanley, E. L. (2019b). First record of the Anuran family rhinophrynidae from the oligocene of eastern North America. J. Herpetol. 53, 316–323. doi:10.1670/19-044
Bokor, J., Broo, J., and Mahoney, J. (2016). Using fossil teeth to study the evolution of horses in response to a changing climate. Am. Biol. Teach. 78 (2), 166–169. doi:10.1525/abt.2016.78.2.166
Boyer, D. M., Gunnell, G. F., Kaufman, S., and McGeary, T. M. (2016). MorphoSource: archiving and sharing 3-D digital specimen data. Paleontol. Soc. Pap. 22, 157–181. doi:10.1017/scs.2017.13
Broo, J., and Mahoney, J. (2015). Chewing on change: exploring the evolution of horses in response to climate change. Available at: https://www.cpet.ufl.edu/media/cpetufledu/pdfs/curriculum/cpet-curriculum/evolution-and-climate-change/HorseEvolution2015_LoRes-Full-Curriculum.pdf (Accessed August 1, 2020).
Buckley, S. J., Ringdal, K., Naumann, N., Dolva, B., Kurz, T. H., Howell, J. A., et al. (2019). LIME: software for 3-D visualization, interpretation, and communication of virtual geoscience models. Geosphere 15, 222–235. doi:10.1130/GES02002.1
Buehler, E., Easley, W., McDonald, S., Comrie, N., and Hurst, A. (2015). “Inclusion and education: 3D printing for integrated classrooms,” in Proceedings of the 17th international ACM SIGACCESS conference on computers & accessibility, New York, NY. Association for Computing Machinery, 281–290. doi:10.1145/2700648.2809844
Bull, G., Chiu, J. L., Berry, R. Q., and Lipson, H. (2013). “Advancing children’s engineering through desktop manufacturing,” in Handbook of research on educational communications and technology. 4th Edn, Editors J. Spector, M. Merrill, J. Elen, and M. J. Bishop (New York, NY: Springer-Verlag), 675–688.
Burns, J. H. R., and Delparte, D. (2017). Comparison of commercial structure-from-motion photogrammetry software used for underwater three-dimensional modeling of coral reef environments. ISPRS Ann. Photogramm., Remote Sens. Spat. Inform. Sci. W3, 127–131. doi:10.5194/isprs-archives-XLII-2-W3-127-2017
Casas, L., and Estop, E. (2015). Virtual and printed 3D models for teaching crystal symmetry and point groups. J. Chem. Educ. 92, 8. doi:10.1021/acs.jchemed.5b00147
CAST (2020). The UDL guidelines. Available at: http://udlguidelines.cast.org/ (Accessed July 29, 2020).
Chan, B. K. K., Hodgkiss, I. J., and Chan, R. Y. P. (2002). A distributd learning model for freshwater ecology practical classes. J. Comput. Assist. Learn. 18, 3. doi:10.1046/j.0266-4909.2002.00242.x
Cheng, L., Antonenko, P., Ritzhaupt, A. D., Dawson, K., Miller, D., MacFadden, B. J., et al. (2020). Exploring the influence of teachers’ beliefs and 3D printing integrated STEM instruction on students’ STEM motivation. Comput. Educ. 158, 103983. doi:10.1016/j.compedu.2020.103983
Cignoni, P., Callieri, M., Corsini, M., Dellepiane, M., Ganovelli, F., and Ranzuglia, G. (2008). “Meshlab: an open-source mesh processing tool,” in In eurographics Italian chapter conference. Editors V. Scarano, R. De Chiara, and U. Erra (Geneve, Switzerland: The Eurographics Association), 129–136.
Cipriani, A., Citton, P., Romano, M., and Fabbi, S. (2016). Testing two open-source photogrammetry software as a tool to digitally preserve and objectively communicate significant geological data: the agolla case study (Umbria-Marche apennines). Italian J. Geosci. 135 (2), 199209. doi:10.3301/IJG.2015.21
Crippen, K., Brown, J. C., Apraiz, K., Busi, R., and Evran, D. (2015). A process model of the U.S. Federal perspective on STEM. J. STEM Teach. Educ. 50, 19–47. doi:10.30707/JSTE50.1Crippen
Crippen, K., Dunckel, B., MacFadden, B., Ellis, S., Lundgren, L., Ho, C., et al. (2015). “A framework for social paleontology via an online community space,” in Proceedings of E-learn: world conference on E-learning in corporate, government, healthcare, and higher education. Kona, HI: Association for the Advancement of Computing in Education, 305–311.
Cullen, R. (2002). Addressing the digital divide. Online Inf. Rev. 25, 5. doi:10.1108/14684520110410517
Dalman, S. G., and Weems, R. E. (2013). A new look at morphological variation in the ichnogenus Anomoepus, with special reference to material from the lower jurassic newark supergroup: implications for ichnotaxonomy and ichnodiversity. Bull. - Peabody Mus. Nat. Hist. 54, 67–124. doi:10.3374/014.054.0104
Deci, E. L., and Ryan, R. M. (2000). The “what” and “why” of goal pursuits: human needs and the self-determination of behavior. Psychol. Inq. 11 (4), 227–268. doi:10.1207/s15327965pli1104_01
Destombes, M. (1978). “Globes en relief du XVIIIe si`ecle,” in In Der globusfreund: wissenschaftliche zeitschrift Fur globographie und instrumentenkunde. Festschrift zum 25jahrigen Bestand des Coronelli-Weltbundes der Globusfreunde). Editors E. Bemiethner, and F. Aurada (Wien, Germany). S.225–S.231.
Dong, S., Xu, S., and Lu, X. (2009). Development of online instructional resources for Earth system science education: an example of current practice from China. Comput. Geosci. 35 (6), 1271–1279. doi:10.1016/j.cageo.2008.08.013
Drewes, A., Henderson, J., and Mouza, C. (2018). Professional development design considerations in climate change education: teacher enactment and student learning. Int. J. Sci. Educ. 40, 67–89. doi:10.1080/09500693.2017.1397798
du Plessis, A., Broeckhoven, C., Guelpa, A., and le Roux, S. G. (2017). Laboratory X-ray micro-computed tomography: a user guideline for biological samples. GigaScience 6, 6. doi:10.1093/gigascience/gix027
Dunn, K. N., Bybee, S., Stanger-Hall, K., and Branham, M. A. (2019). Nano-CT scanning the light organs of fireflies (Coleoptera: Lampyridae). 7. ToSCA North America, 29–30. Abstract retrieved from Abstracts in Tomography for Scientific Advancement website (Session No. 6).
Edinger, T. (1975). Paleoneurology 1804–1966: an annotated bibliography. Adv. Anat. Embryol. Cell Biol. 49, 1–258. doi:10.1007/978-3-642-66029-0
Eilitz, M., and Reiss, G. (2015). 3D reconstruction of SEM images by use of optical photogrammetry software. J. Struct. Biol. 191 (2), 190–196. doi:10.1016/j.jsb.2015.06.010
Elmendorf, D. C., and Song, L. (2015). Developing indicators for a classroom observation tool on pedagogy and technology integration: a delphi study. Comput. Sch. 32, 1–19. doi:10.1080/07380569.2014.967620
Epicc (2020). Eastern pacific invertebrate communities of the CenozoicEPICC virtual field experiencesEPICCAvailable at: https://epiccvfe.berkeley.edu/about/ (Accessed June 20, 2020).
Falkingham, P. L. (2012). Acquisition of high-resolution 3D models using free, open-source, photogrammetric software. Palaeontol. Electron. 15, 1. doi:10.26879/264
Fariña, R. A., Vizcaíno, S. F., and Bargo, M. S. (1998). Body mass estimations in lujanian (late pleistocene-early holocene of south America) mammal megafauna. Mastozool. Neotrop. 5, 87–108.
Fine, M. (1991). Framing dropouts: notes on the politics of an urban high school. New York, NY: SUNY Press.
Florida Museum of Natural History, (2020). Digital Discovery & dissemination (3D) lab: computed tomography applications for museum research. Gainesville, FL: Florida Museum of Natural HistoryAvailable at: https://www.floridamuseum.ufl.edu/digital-lab/ct/ (Accessed June 1, 2020).
Forbes, C. T., Biggers, M., and Zangori, L. (2013). Investigating essential characteristics of scientific practices in elementary science learning environments: the practices of science observation protocol (P-sop). Sch. Sci. Math. 113 (4), 180–190. doi:10.1111/ssm.12014
Ford, S., and Minshall, T. (2016). 3D printing in education: a literature review. ResearchGate [PrePrint]. Available at: https://www.researchgate.net/publication/320617391_Invited_review_article_Where_and_how_3D_printing_is_used_in_teaching_and_education (Accessed May 10, 2020).
Fossil, (2020). Fostering opportunities for synergistic STEM with informal learners. myFOSSILAvailable at: [PrePrint] https://www.myfossil.org/ (Accessed June 5, 2020).
Francioni, M., Simone, M., Stead, D., Sciarra, N., Mataloni, G., and Calamita, F. (2019). A new fast and low-cost photogrammetry method for the engineering characterization of rock slopes. Rem. Sens. 11, 1276. doi:10.3390/rs11111267
Fujiwara, D. (2012). 3D printing pivotal to forbidden city extreme makeover. Available at: http://3dprinterhub.com/3d-printer-news/3d-printing-pivotalto-forbidden-city-extreme-makeover/169/ (Accessed May 25, 2020).
Futch, V. A., and Fine, M. (2014). Mapping as a method: history and theoretical commitments. Qual. Res. Psychol. 11, 42–59. doi:10.1080/14780887.2012.719070
Gangjee, N., Lipson, H., and Owen, D. I. (2011). 3D printing of cuneiform tablets at cornell creative machines lab. Available at: https://www.creativemachineslab.com/cuneiforms.html (Accessed May 25, 2020).
Gardiner, K. (2017). Rare, beautiful, & fascinating 100 years at the Florida museum: new 100th anniversary exhibit to open saturday with free special activities. Available at: https://www.floridamuseum.ufl.edu/pressroom/2017/09/21/new-100th-anniversary-exhibit-to-open-saturday-with-free-special-activities/ (Accessed May 15, 2020).
Gokhare, V. G., Raut, D. N., and Shinde, D. K. (2017). A review paper on 3D-printing aspects and various processes used in the 3D printing. Int. J. Eng. Res. Technol. 6, 6953–6958. doi:10.17577/IJERTV6IS060409
Granshaw, F. D. (2011). Designing and using virtual field environments to enhance and extend field experience in professional development programs in geology for K–12 teachers. Dissertation. Portland (OR): Portland State University.
Grant, C., MacFadden, B. J., Antonenko, P., and Perez, V. (2016). 3-D fossils for K–12 education: a case example using the giant extinct shark Carcharocles megalodon. Paleontol. Soc. Pap. 22, 197–209. doi:10.1017/scs.2017.15
Harrington, A. R., Silcox, M. T., Yapuncich, G. S., Boyer, D. M., and Bloch, J. I. (2016). First virtual endocasts of adapiform primates. J. Hum. Evol. 99, 52–78. doi:10.1016/j.jhevol.2016.06.005
Hasiuk, F. (2014). Making things geological: 3-D printing in the geosciences. Geol. Soc. Am. 24, 8–28. doi:10.1130/GSATG211GW.1
Hastings, A. K., Vargas-Vergara, C., Corbin, S., and Ziegler, M. J. (2020). Tracking the evidence from fossil dinosaur footprints. Available at: http://www.idigfossils.org/Hastings_iDigFossils_Lesson.pdf (Accessed August 1, 2020).
Head, J. J., Bloch, J. I., Hastings, A. K., Bourque, J. R., Cadena, E. A., Herrera, F. A., et al. (2009). Giant boid snake from the Paleocene neotropics reveals hotter past equatorial temperatures. Nature 457, 7230. doi:10.1038/nature07671
Hegna, T. A., and Johnson, R. E. (2016). Preparation of fossil and osteological 3D-printable models from freely available CT-scan movies. J. Paleontol 16, 1–10.
Hestness, E., McDonald, R. C., Breslyn, W., McGinnis, J. R., and Mouza, C. (2014). Science teacher professional development in climate change education informed by the next generation science standards. J. Geosci. Educ. 62, 3319–3329. doi:10.5408/13-049.1
Holmer, N. A., Clement, N., Dehart, K., Maschner, H., Pruitt, J., Schlader, R., et al. (2014). The Idaho virtualization laboratory 3D pipeline. Virtual Archaeology Review 5, 10. doi:10.4995/var.2014.4208
Horowitz, S. S., and Schultz, P. H. (2014). Printing space: using 3D printing of digital terrain models in geosciences education and research. J. Geosci. Educ. 62, 1–138. doi:10.5408/13-031.1
Houser, C., Garcia, S., and Torres, J. (2015). Effectiveness of geosciences exploration summer program (GeoX) for increasing awareness and knowledge of geosciences. J. Geosci. Educ. 63 (2), 116–126. doi:10.5408/14-016.1
Hughes, N. C., Ghosh, P., and Bhattacharya, D. (2015). The monishar pathorer bon (monisha and the Stone forest) book project: novel educational outreach to children in rural communities, eastern Indian subcontinent. J. Geosci. Educ. 63, 1–18. doi:10.5408/14-014.1
Huk, T. (2006). Who benefits from learning with 3D models? The case of spatial ability. J. Comput. Assist. Learn. 22, 392–404. doi:10.1111/j.1365-2729.2006.00180.x
iDigFossils, (2020). iDigFossils. Available at: http://www.idigfossils.org/ (Accessed May 25, 2020).
Ishutov, S., Jobe, D., Zhang, S., Gonzalez, M., Agar, S. M., Hasiuk, F. J., et al. (2017). Three-dimensional printing for geoscience: fundamental research, education, and applications for the petroleum industry. Am. Assoc. Pet. Geol. Bull. 102 (1), 1–26. doi:10.1306/0329171621117056
Jacobson, A. R., Militello, R., and Baveye, P. C. (2009). Development of computer-assisted virtual field trips to support multidisciplinary learning. Comput. Educ. 52, 571–580. doi:10.1016/j.compedu.2008.11.007
Jang, S., Vitale, J. M., Jyung, R. W., and Black, J. B. (2016). Direct manipulation is better than passive viewing for learning anatomy in a three-dimensional virtual reality environment. Comput. Educ. 106, 150–165. doi:10.1016/j.compedu.2016.12.009
Janis, C. M. (2007). “The horse series,” in Icons of evolution. Editor B. Regal (Westport, CT: Greenwood Press), 257–280.
Jones, M. G., Minogue, J., Tretter, T. R., Negishi, A., and Taylor, R. (2005). Haptic augmentation of science instruction: does touch matter?. Sci. Educ. 90, 111–123. doi:10.1002/sce.20086
Kaliisa, R., Palmer, E., and Miller, J. (2019). Mobile learning in higher education: a comparative analysis of developed and developing country contexts. Br. J. Educ. Technol. 50 (2), 546–561. doi:10.1111/bjet.12583
Keeffe, R. M., and Blackburn, D. C. (2019). Comparative morphology of the forelimb and pectoral girdle in forward-burrowing frogs. Soci. Integrat. Comparat. Biol. 59, E120Abstract received from Abstracts in SICB database(Accession No. 116-4)
Kikinis, R., Pieper, S. D., and Vosburgh, K. G. (2014). “3D slicer: a platform for subject-specific image analysis, visualization, and clinical support,” in In intraoperative imaging and image-guided therapy. Editor F. Jolesz (New York, NY: Springer), 277–289.
Koehler, K. E. (2017). Examining the conceptual understandings of geoscience concepts of students with visual impairments: implications of 3-D printing. Doctoral Dissertation, Columbus, (OH): The Ohio State University.
Krasnodebska, N. (2015). Sketchfab supports museums and cultural institutions. Available at: https://sketchfab.com/blogs/community/sketchfab-supports-museums-and-cultural-institutions/ (Accessed July 1, 2020).
Kyriakopoulos, C. (2019). 3D printing: a remedy to common misconceptions about earthquakes. Seismol Res. Lett. 90, 4. doi:10.1785/0220190121
Land, M. H. (2013). Full STEAM Ahead: the benefits of integrating the arts into STEM. Proc. Compu. Sci. 20, 547–552. doi:10.1016/j.procs.2013.09.317
Lautenschlager, S., and Rücklin, M. (2014). Beyond the print: virtual paleontology in science publishing, outreach, and education. J. Paleontol. 88, 727–734. doi:10.1666/13-085
Lebrun, R., and Orliac, M. J. (2017). MorphoMuseum: an online platform for publication and storage of virtual specimens. Paleontol. Soc. Pap. 22, 183–195. doi:10.1017/scs.2017.14
Lee, E. A., and Wong, K. W. (2014). Learning with desktop virtual reality: low spatial ability learners are more positively affected. Comput. Educ. 79, 49–58. doi:10.1016/j.compedu.2014.07.010
Lee, J. J. (2013). 5 ways smithsonian uses 3-D scanning to open up history. Available at: https://www.nationalgeographic.com/news/2013/9/130904-3d-printing-smithsonian-whale-skeleton-technology-science/ (Accessed July 17, 2020).
Lee, S. G., Lee, J. Y., Park, K. E., Lee, J. H., and Ahn, S. C. (2015). Mathematics, art and 3D-printing in STEAM education. Commun. Mathemat. Educ. 29, 35–49. doi:10.7468/jksmee.2015.29.1.35
Leipner, A., Obertová, Z., Wermuth, M., Thali, M., Ottiker, T., and Sieberth, T. (2019). 3D mug shot: 3D head models from photogrammetry for forensic identification. Forensic Sci. Int. 300, 6–12. doi:10.1016/j.forsciint.2019.04.015
Lewis, D. (2019). The fight for Control over virtual fossils. Available at: https://www.nature.com/articles/d41586-019-00739-0 (Accessed August 23, 2020).
Lucas, S. G., and Harris, J. D. (2019). The “plastotype problem” in ichnological taxonomy. Ichnos 27, 107. doi:11010.1080/10420940.2019.1688802
Luhmann, T., Robson, S., Kyle, S., and Boehm, J. (2014). Close-range photogrammetry and 3D imaging. Berlin, Germany: De Gruyter.
Lyon, E., Freeman, R. L., Bathon, J., Fryar, A., McGlue, M., Erhardt, A. M., et al. (2019). Attitudinal impediments to geology major recruitment among ninth graders at a STEM high school. J. Geosci. Educ. 68 (3), 237–253. doi:10.1080/10899995.2019.1700593
MacFadden, B. J. (2005). Fossil horses: evidence for evolution. Science 307, 1728–1730. doi:10.1126/science.1105458
MacFadden, B. J. (2019). Broader impacts of science on society. Cambridge, UK: Cambridge University Press.
MacFadden, B. J., Oviedo, L. H., Seymour, G. M., and Ellis, S. (2012). Fossil horses, orthogenesis, and communicating evolution in museums. Evolu. Educ. Outreach 5 (1), 29–37. doi:10.1007/s12052-012-0394-1
MacFadden, B. J., Pirlo, J., Abramowitz, B., Killingsworth, S., and Ziegler, M. J. (2020). Authentic field experiences for STEM teachers: collecting Florida fossils. Available at: https://www.biorxiv.org/content/10.1101/2020.07.02.184077v1.full (Accessed August 23, 2020).
Mallison, H. (2011). “Digitizing methods for paleontology: applications, benefits and limitations,” in Computational paleontology. Editor A. T. Elewa (Berlin, Germany: Springer), 7–43. doi:10.1007/978-3-642-16271-8_2
Maloy, R., Kommers, S., Malinowski, A., and LaRoche, I. (2017). 3D modeling and printing in history/social studies classrooms: initial lessons and insights. Contemp. Issues Technol. Teach. Educ. 17, 229–249.
Martinez, M. O., Morimoto, T. K., Taylor, A. T., Barron, A. C., Pultorak, J. A., Wang, J., et al. (2016). “3-D printed haptic devices for educational applications,,” in IEEE haptics symposium 2016. Editors S. Choi, K. J. Kuchenbecker, and G. Gerling (Piscataway, NJ: Institute of Electrical and Electronics Engineers, Inc.), 126–133. doi:10.1109/HAPTICS.2016.7463166
Maskall, J., and Stokes, A. (2008). GEES subject centre learning and teaching guide: designing effective fieldwork for the environmental and natural sciences. Plymouth, England: University of Plymouth: The Higher Education Academy Subject Center for Geography, Earth and Environmental Sciences.
Matthews, N. A., Noble, T. A., and Breithaupt, B. H. (2016). “Close-range photogrammetry for 3D ichnology: the basics of photogrammetric ichnology,” in Dinosaur tracks: the next steps. Editors P. L. Falkingham, D. Marty, A. Richter, and Bloomington (Bloomington, IN: Indiana University Press), 29–55.
McCarty, S. (2017). Titanoboa: giant snake from earth’s past. Available at: http://www.idigfossils.org/wp-content/uploads/2019/03/McCarty_Titanoboa-Lesson_5th-6th-Grade.pdf (Accessed August 1, 2020).
McCormick, S. (2007). Democratizing science movements: a new framework for mobilization and contestation. Soc. Stud. Sci. 37 (4), 609–623. doi:10.1177/0306312707076598
McLaughlin, C. A., Broo, J., MacFadden, B. J., and Moran, S. (2016). Not looking a gift horse in the mouth: exploring the merits of a student–teacher–scientist partnership. J. Biol. Educ. 50, 174–184. doi:10.1080/00219266.2015.1028571
McMenamin, P. G., Quayle, M. R., McHenry, C. R., and Adams, J. W. (2014). The production of anatomical teaching resources using three-dimensional (3D) printing technology. Anat. Sci. Educ. 7, 6479–6486. doi:10.1002/ase.1475
McNeal, K. S. (2010). The geosciences gap in K–12 education. J. Geosci. Educ. 58 (4), 197. doi:10.5408/1.3534857
Mead, C., Buxner, S., Bruce, G., Taylor, W., Semken, S., and Anbar, A. D. (2019). Immersive, interactive virtual field trips promote science learning. J. Geosci. Educ. 67, 131–142. doi:10.1080/10899995.2019.1565285
Megale, C., and Baione, C. (2017). The virtual etruscan museum of populonia gasparri collection: enhancing the visitor’s experience. J. Public Archeol. 1, 67–77. doi:10.23821/2017_3d
Mihlbachler, M. C., Rivals, F., Solounias, N., and Semprebon, G. M. (2011). Dietary change and evolution of horses in North America. Science 331, 6021. doi:10.1126/science.1196166
Mitsopoulou, V., Michailidis, D., Theodorou, E., Isidorou, S., Roussiakis, S., Vasilopoulos, T., et al. (2015). Digitizing, modelling and 3D printing of skeletal digital models of Palaeoloxodon tiliensis (tilos, dodecanese, Greece). Quat. Int. 379, 4–13. doi:10.1016/j.quaint.2015.06.068
MorphoSource, (2015). K–12 human evolution project: hhomo habilis. Available at: https://www.morphosource.org/MyProjects/Specimens/form/specimen_id/1981 (Accessed August 9, 2020).
MorphoSource (2020). MorphoSource. Available at: https://www.morphosource.org/ (Accessed June 5, 2020).
Morse, P. E., Bloch, J. I., Yapuncich, G. S., Boyer, D. M., and Strait, S. G. (2015). Dental topography and dietary ecology of the first north American euprimates. Am. J. Phys. Anthropol. 156, 230.
Morse, P. E., Chester, S. G., Boyer, D. M., Smith, T., Smith, R., Gigase, P., et al. (2019). New fossils, systematics, and biogeography of the oldest known crown primate teilhardina from the earliest eocene of asia, europe, and north America. J. Hum. Evol. 128, 103–131. doi:10.1016/j.jhevol.2018.08.005
Murrey, M. (2019). Improving geoscience education with a public database of 3D-printable crystal models. OSR J. Stud. Res. 5, 279. Available at: https://scholarworks.lib.csusb.edu/osr/vol5/iss1/279. (Abstract received from CSUSB ScholarWorks database).
Mussetter, B., Hendrickson, M., and Perez, V. J. (2020). How big was the megalodon? Available at: http://www.idigfossils.org/wp-content/uploads/2019/03/Mussetter_Megalodon-Lesson-Plan_4th-Grade.pdf (Accessed August 9, 2020).
Mussetter, B. (2018). Sizing up turkeys: an exploration of proportional size. Available at: http://www.idigfossils.org/wp-content/uploads/2019/03/Mussetter_Sizing-up-Turkeys-Lesson_6th-Grade.pdf (Accessed August 9, 2020).
myFOSSIL (2019). Fusilinid on MyFOSSIL eMuseum. myFOSSILAvailable at: https://www.myfossil.org/ac-media/31392/ (Accessed July 20, 2020).
Narducci, R., DeLeon, V., Silcox, T., and Bloch, J. (2019). Endocranial shape variation in fossil and modern xenarthrans using 3D geometric morphometrics. Soc. Vertebr. Paleontol. Progr. Abstr. 79, 158Abstract Retrieved from Abstracts in Society of Vertebrate Paleontology Database.
Nesbit, P. R., Boulding, A. D., Hugenholtz, C. H., Durkin, P. R., and Hubbard, S. M. (2020). Visualization and sharing of 3D digital outcrop models to promote open. Science 30, 6. doi:10.1130/GSATG425A.1
Neumüller, M., Reichinger, A., Rist, F., and Kern, C. (2014). “3D printing for cultural heritage: preservation, accessibility, research and education,” in In 3D research challenges in cultural heritage. Editors M. Ioannides, and E. Quak (Berlin: Heidelberg: Springer), 119–134.
Nix, R. (1999). A critical evaluation of science-related virtual field trips available on the world wide web. Master’s Thesis. Perth, (Austria): Curtin University of Technology Western Australia.
Otero, A., Moreno, A. P., Falkingham, P. L., Cassini, G., Ruella, A., Militello, M., et al. (2020). Three-dimensional image surface acquisition in vertebrate paleontology: a review of principal techniques. Asociac. Paleontol. Argent. 20 (1), 1–14. doi:10.5710/PEAPA.04.04.2020.310
O’Connor, J., Smith, M., and James, M. R. (2019). Impact of image compression on structure from motion photogrammetry. Geophys. Res. Abstr. 21, 1Abstract retrieved from Abstracts in General Assemblies of the European Geosciences Union database(Accession No. 140482353).
O’Leary, M. A., Bloch, J. I., Flynn, J. J., Gaudin, T. J., Giallombardo, A., Giannini, N. P., et al. (2013). The placental mammal ancestor and the post-K-pg radiation of placentals. Science 339, 662–667. doi:10.1126/science.1229237
Pai, S., Gourish, B., Moger, P., and Mahale, P. (2018). Application of 3D printing in education. Int. J. Comput. Appl. Technol. Res. 7, 278–280.
PaleoTEACH (2015). K–12 project: human evolution. Available at: http://www.paleoteach.org/specimens/human-evolution/ (Accessed August 1, 2020).
Paleoteach (2017). Smilodon gracilis (saber tooth cat) on Sketchfab. Available at: https://sketchfab.com/3d-models/smilodon-gracilis-saber-toothed-tiger-63387169c26b402f8dbc87392f63e236 (Accessed August 9, 2020).
Paleoteach (2018). Phytosaur belly on Sketchfab. Available at: https://sketchfab.com/3d-models/phytosaur-belly-346ec4b6462249bfa00e75bd2bfbc3a5 (Accessed on August 7, 2020).
Paxon, M., Holte, S., Perez, V. J., and Lounders, A. (2018). What is in an arm: how does forelimb anatomy of carnivorans affect ecological niche and evolutionary advantages? Available at: http://www.idigfossils.org/high-school/how-forelimb-anatomy-of-carnivorans-affects-ecological-niche-and-evolutionary-advantages/ (Accessed on August 1, 2020).
Pcast, (2012). “President’s council of advisors on science and technology,” in Engage to excel: producing one million additional college graduates with degrees in science, technology, engineering, and mathematics. Washington, D.C.: The National Academy Press.
Perez, V. J., Moran, S. M., and Grant, C. (2020). A novel evaluation tool for characterizing audiences in informal education settings. J. Mus. Educ. 45, 3. doi:10.1080/10598650.2020.1774270
Peterson, J. E., and Krippner, M. L. (2019). Comparisons of fidelity in the digitization and 3D printing of vertebrate fossils. J. Paleontol 22, 1–9. doi:10.1130/abs/2017ne-290684
Pickering, J., Ague, J. J., Rath, K. A., Heiser, D. M., and Sirch, J. N. (2012). Museum-based teacher professional development: peabody fellows in Earth science. J. Geosci. Educ. 60, 337–349. doi:10.5408/11-241.1
Pierce, D. (2016). 3D technologies add another dimension to learning: new enhancements to 3D instruction create a more immersive, interactive experience. Technol. Horizons Educ. 43, 9–10.
Pruitt, J. B., Clement, N. G., and Tapanila, L. (2017). Laser and structured light scanning to acquire 3-D morphology. Paleontol. Soc. Pap. 22, 57–69. doi:10.1017/scs.2017.8
Puhek, M., Perše, M., and Šorgo, A. (2012). Comparison between a real field trip and a virtual field trip in a nature preserve: knowledge gained in biology and ecology. J. Baltic Sci. Educ. 11 (2), 164–174.
Pyenson, N. D., Gutstein, C. S., Parham, J. F., Le Roux, J. P., Chavarría, C. C., Little, H., et al. (2014). Repeated mass strandings of Miocene marine mammals from atacama region of Chile point to sudden death at sea. Proceed. Royal Soc. B. 281, 1781. doi:10.1098/rspb.2013.3316
Radinsky, L. B. (1968). Evolution of somatic sensory specialization in otter brains. J. Comp. Neurol. 134, 495–505. doi:10.1002/cne.901340408
Rahman, I. A., Adcock, K., and Garwood, R. J. (2012). Virtual fossils: a new resource for science communication in paleontology. Evolu.: Educ. Outreach 5, 4635–4641. doi:10.1007/s12052-012-0458-2
Ramasundaram, V., Grunwald, S., Mangeot, A., Comerford, N. B., and Bliss, C. M. (2005). Development of an environmental virtual field laboratory. Comput. Educ. 45, 21–34. doi:10.1016/j.compedu.2004.03.002
Reyes, R., Bellian, J. A., Dunlap, D. B., and Eustice, R. A. (2008). Cyber techniques used to produce physical geological models. 40. Geological Society of America Abstracts with Programs, 136Abstract retrieved from Abstracts in GSA database. (Accession No. 142-2).
Riegler, M., Stanley, E., and Bloch, J. (2019). Describing enamel in squamates: utilizing non-destructive CT scans to characterize enamel in extant and extinct lizard species. Soc. Vertebr. Paleontol. 79, 179–180. Abstract Retrieved from Abstracts in Society of Vertebrate Paleontology Database.
Ritschl, L. M., Wolff, K. D., Erben, P., and Grill, F. D. (2019). Simultaneous, radiation-free registration of the dentoalveolar position and the face by combining 3D photography with a portable scanner and impression-taking. Head Face Med. 15, 1–9. doi:10.1186/s13005-019-0212-x
Ross, R. M., Haas, D. A., Hendricks, J. R., and Allmon, W. D. (2019). “Paleontological research institution’s museum of the Earth and taughannock state park as teaching resources,” In the New York state geological association 91st annual meeting field trip guide, Vol. 1–31. Geneva, NY: New York State Association of Professional GeologistsHobart and William Smith Colleges.
Ross, R. M., McRoberts, C. A., and Duggan-Haas, D. (2007). “Creating a virtual fieldwork experience at a fossil–rich quarry,” in 79th annual meeting field trip guidebook Editor C. A. McRoberts (Alfred, NY: New York State Geological Association), 177–187.
Sahlstrom, S., and Moran, S. M. (2018). The great American biotic Interchange project. Available at: http://www.idigfossils.org/great-american-biotic-interchange-project/ (Accessed June 5, 2020).
Savini, A., and Savini, G. G. (2015). A Short history of 3D printing, a technological revolution just started In 2015 international history of high-technologies and their socio-cultural contexts conference (HISTELCON). Red Hook, NY: Institute of Electrical and Electronics EngineersCurran Associates, 1–8. doi:10.1109/histelcon.2015.7307314
Schmidt, R. W. (2013). Bridging the geoscientist workforce gap: advanced high school geoscience programs. (Dissertation), Philadelphia, (PA): Drexel University.
Selba, M. C., and Ziegler, M. J. (2017). A comparison of hominins using 3D printed fossil jaws. Available at: http://www.idigfossils.org/a-comparison-of-hominins-using-fossils-such-as-teeth-and-skulls/ (Accessed August 9, 2020).
Shaw, M., and Webb, L. (2016). Anatomical similarities and differences between modern and ancient humans using fossil evidence. Available at: http://www.idigfossils.org/anatomical-similarities-and-differences-between-modern-and-ancient-humans-using-fossil-evidence/ (Accessed on August 1, 2020).
Sheppard, T. (2019). Trends and changes: feeding evolution of horse teeth. Available at: http://www.idigfossils.org/wp-content/uploads/2019/03/Sheppard_Trends-Changes-Feeding-Evolution-of-Horse-Teeth-Lesson_6th-8th-Grade.pdf (Accessed August 1, 2020).
Shimada, K. (2002). The relationship between the tooth size and total body length in the white shark. J. Fossil Res. 35, 28–33.
Short, D. B. (2015). Use of 3D printing by museums: educational exhibits, artifact education, and artifact restoration. 3D Print. Addit. Manuf. 2 (4), 209–215. doi:10.1089/3dp.2015.0030
Singh, A. L., Keeffe, R. M., and Blackburn, D. C. (2019). Tips and fits: tricks to 3D-puzzle making. Soc. Integr. Comparat. Biol. 59, E409Abstract received from ResearchGate database.
Singh, A. L., Paluh, D. J., and Blackburn, D. C. (2020). University of Florida undergraduate virtual research symposium: variation in bony labyrinth (inner ear) of Anurans. Available at: https://cur.aa.ufl.edu/2020/04/14/singh-amber/ (Accessed July 5, 2020).
Slizewski, A., Friess, M., and Semal, P. (2010). Surface scanning of anthropological specimens: nominal-actual comparison with low cost laser scanner and high-end fringe light projection surface scanning systems. Quartar 57, 179–187.
Smith, F. A., Lyons, S. K., Ernest, S. K. M., Jones, K. E., Kaufman, D. M., Dayan, T., et al. (2003). Body mass of late quaternary mammals. Ecology 84 (12). 3403. doi:10.1890/02-9003
Strömberg, C., and McInerney, F. A. (2011). The Neogene transition from C3 to C4 grasslands in North America: assemblage analysis of fossil phytoliths. Paleobiology 37, 50–71. doi:10.2307/23014716
Sutton, M. (2008). Tomographic techniques for the study of exceptionally preserved fossils. Proceed. Royal Soc. B. 275, 1643. doi:10.1098/rspb.2008.0263
Sutton, M., Rahman, I., and Garwood, R. (2016). Virtual paleontology: an overview. Paleontol. Soc. Pap. 22, 1–20. doi:10.1017/scs.2017.5
Taylor, W. L., Högström, A., Ebbestad, J. O. R., Høyberget, M., Jensen, S., Meinhold, G., et al. (2018). Teaching with trilobites: E-learning resources to enhance student learning at the tromsø university museum. UiT Arctic University of Norway5th International Palaeontological Conference. 1077Abstract retrieved from Abstracts in Uppsala University Publications database. (Accession No. urn:nbn:se:uu:diva-371725.
Teshima, Y., Matsuoka, A., Fujiyoshi, M., Ikegami, Y., Kaneko, T., Oouchi, S., et al. (2010). “Enlarged skeleton models of plankton for tactile teaching,” in International conference on computers for handicapped persons. Berlin, Germany: Springer, 523–526.
Tokkari, N., Verdaasdonk, R. M., Liberton, N., Wolff, J., Den Heijer, M., Van Der Veen, A., et al. (2017). Comparison and use of 3D scanners to improve the quantification of medical images (surface structures and volumes) during follow up of clinical (surgical) procedures. Procee. SPIE 15, 10054. doi:10.1117/12.2253241
Trust, T., and Maloy, R. W. (2017). Why 3D print? The 21st-century skills students develop while engaging in 3D printing projects. Comput. Sch. 34, 4–253. doi:10.1080/07380569.2017.1384684
Tuthill, G., and Barbara, K. E. (2002). Virtual field trips: alternatives to actual field trips. Int. J. Instr. Media 29, 453–468.
Unfried, A., Faber, M., Stanhope, D., and Wiebe, E. (2015). The development and validation of a measure of student attitudes toward science, technology, engineering, and math (S-STEM). J. Psychoeduc. Assess. 33, 7–622. doi:10.1177/0734282915571160
University of Florida Research Services (2020). NANO-CT. University of Florida Research ServicesAvailable at: https://rsc.aux.eng.ufl.edu/ccb/resource.asp?id=79 (Accessed June 1, 2020).
van Dijk, J. A. (2006). Digital divide research, achievements and shortcomings. Poetics 34, 221–235. doi:10.1016/j.poetic.2006.05.004
Vargas-Vergara, C. (2020). STEM identity development in minoritized youth at a public alternative high school in California. (Dissertation), Gainesville (FL): University of Florida.
Vitek, N. S., Hoeflich, J., Magallanes, I., Moran, S. M., Narducci, R. E., Ocon, S. B., et al. (2020). Early Pleistocene porcupines in temperate north America retained characteristic tropical, arboreal morphology. Bethesda, MD: Society of Vertebrate Paleontology.
Vizcaíno, S. F., Bargo, M. S., and Cassini, G. H. (2006). Dental occlusal surface area in relation to body mass, food habits and other biological features in fossil xenarthrans. Ameghiniana 43, 11–26.
Vizcaíno, S. F., and Milne, N. (2002). Structure and function in armadillo limbs (mammalia: xenarthra: dasypodidae). J. Zool. 257, 117–127. doi:10.1017/S0952836902000717
von Übel, M. (2020). 2020 best photogrammetry software. Available at: https://all3dp.com/1/best-photogrammetry-software/ (Accessed June 5, 2020).
Walker, M., and Humphries, S. (2019). 3D printing: applications in evolution and ecology. Ecol. Evol. 9 (7), 4289–4301. doi:10.1002/ece3.5050
Wang, M. T., and Degol, J. (2013). Motivational pathways to STEM career choices: using expectancy-value perspective to understand individual and gender differences in STEM fields. Dev. Rev. 33 (4), 304. doi:10.1016/j.dr.2013.08.001
Wang, Y., Cerling, T. E., and MacFadden, B. J. (1994). Fossil horses and carbon isotopes: new evidence for cenozoic dietary, habitat, and ecosystem changes in North America. Palaeogeogr. Palaeoclimatol. Palaeoecol. 107, 269–279. doi:10.1016/0031-0182(94)90099-X
Weems, R. E. (1992). A re-evaluation of the taxonomy of the newark supergroup saurischian dinosaur tracks, using extensive statistical data from a recently exposed tracksite near Culpeper, Virginia. Virginia Div. Mineral Resou. Public. 119. 113–127.
Weems, R. E. (1987). a late triassic footprint fauna from the culpeper basin Northern Virginia (U.S.A.). Trans. Am. Phil. Soc. 77, 1–79. doi:10.2307/1006417
Weems, R. E., and Olsen, P. E. (1997). Synthesis and revision of groups within the newark supergroup. Eastern North America. Geol. Soc. Am. Bull. 109, 195–209. doi:10.1130/0016-7606(1997)109<0195:SAROGW>2.3.CO;2
Wenger, E., McDermott, R., and Snyder, W. M. (2002). Cultivating communities of practice: a guide to managing knowledge. Cambridge, Massachusetts: Harvard Business Press.
Westoby, M. J., Brasington, J., Glasser, N. F., Hambrey, M. J., and Reynolds, J. M. (2012). ‘Structure-from-motion’ photogrammetry: a low-cost, effective tool for geoscience applications. Geomorphology 179, 300–314. doi:10.1016/j.geomorph.2012.08.021
Wood, A. (2016). Megalodon body size estimates. Available at: https://wood-idigfossils.shinyapps.io/Megalodon/ (Accessed July 7, 2020).
Woodburne, M. O. (2010). The great american biotic interchange: dispersals, tectonics, climate, sea level and holding pens. J. Mamm. Evol. 17, 245–264. doi:10.1007/s10914-010-9144-8
Xing, L., Stanley, E. L., Bai, M., and Blackburn, D. C. (2018). The earliest direct evidence of frogs in wet tropical forests from cretaceous burmese amber. Sci. Rep. 8 (1), 8770. doi:10.1038/s41598-018-26848-w
Yacobucci, M. M., and Lockwood, R. (2012). The paleontological society special publications: teaching paleontology in the 21st century. New Haven, CT: Yale University Printing and Publishing Services.
Zachos, J., Dickens, G., and Zeebe, R. (2008). An early cenozoic perspective on greenhouse warming and carbon-cycle dynamics. Nature 451, 279–283. doi:10.1038/nature06588
Ziegler, M. J., and Grant, C. (2017). Montbrook site on Sketchfab. Available at: https://sketchfab.com/3d-models/montbrook-site-october-2017-500620c1a78349e29ede5eb8eb6efd31 (Accessed August 9, 2020).
Keywords: 3D printing and scanning, paleontology - general, science technology engineering mathematics, education and outreach, k–12 collaboration
Citation: Ziegler MJ, Perez VJ, Pirlo J, Narducci RE, Moran SM, Selba MC, Hastings AK, Vargas-Vergara C, Antonenko PD and MacFadden BJ (2020) Applications of 3D Paleontological Data at the Florida Museum of Natural History. Front. Earth Sci. 8:600696. doi: 10.3389/feart.2020.600696
Received: 30 August 2020; Accepted: 30 October 2020;
Published: 09 December 2020.
Edited by:
Christodoulos Kyriakopoulos, University of Memphis, United StatesReviewed by:
Nigel Hughes, University of California, Riverside, United StatesLindsay Zanno, North Carolina State University, United States
Copyright © 2020 Ziegler, Perez, Pirlo, Narducci, Moran, Selba, Hastings, Vargas-Vergara, Antonenko and MacFadden. This is an open-access article distributed under the terms of the Creative Commons Attribution License (CC BY). The use, distribution or reproduction in other forums is permitted, provided the original author(s) and the copyright owner(s) are credited and that the original publication in this journal is cited, in accordance with accepted academic practice. No use, distribution or reproduction is permitted which does not comply with these terms.
*Correspondence: Michael J. Ziegler, emllZ2xlci5qLm1pY2hhZWxAZ21haWwuY29t