The Disaster Taxon Lystrosaurus: A Paleontological Myth
- Department of Biology, Cape Breton University, Sydney, NS, Canada
The term “disaster species” was a term originally conceived to describe marine microfossils that exhibited profound abundances in the wake of a biological crisis. The term was expanded in the 1990s to describe (as “disaster taxa”) opportunistic taxa that dominated their biota numerically (“bloomed”) during the survival interval of a mass extinction event. The Permo-Triassic tetrapod genus Lystrosaurus has been cited regularly as a “disaster taxon” of the end-Permian mass extinction. A review of the definitions that have been developed for disaster taxa, and data from recent biostratigraphic and phylogenetic studies that include species of Lystrosaurus, leads to the conclusion that the genus is not a “disaster taxon”. Further, the known biostratigraphy and tree topologies of species of Lystrosaurus do not satisfy more recent definitions that attribute diversification to disaster species. At most, species of Lystrosaurus that form the informal “Lystrosaurus abundant zone” in the lower Katberg Formation, Lower Triassic of South Africa, could be described as opportunistic species.
EPIGRAPH
“There is an extinction, which is a disaster, and this taxon is abundant, so it must be a disaster taxon”—Webb and Leighton (2011, p. 202).
Introduction
Five mass extinctions have punctuated the evolutionary history of metazoans. Broad-scale studies of mass extinctions have yielded in rich nomenclature with which to identify and to describe the fates and/or the roles of biota that have been subject to a mass extinction (Kauffman and Erwin, 1995; Harries et al., 1996; Kauffman and Harries, 1996; Hallam and Wignall, 1997). Accordingly, evolutionary biologists (Harries et al., 1996; Hallam and Wignall, 1997) have theorized of such biological entities as “disaster species,” “disaster taxa,” “opportunistic taxa,” and “Lazarus taxa,” with respect to successive “extinction,” “survival,” and “recovery” phases of an extinction event (Figure 1).
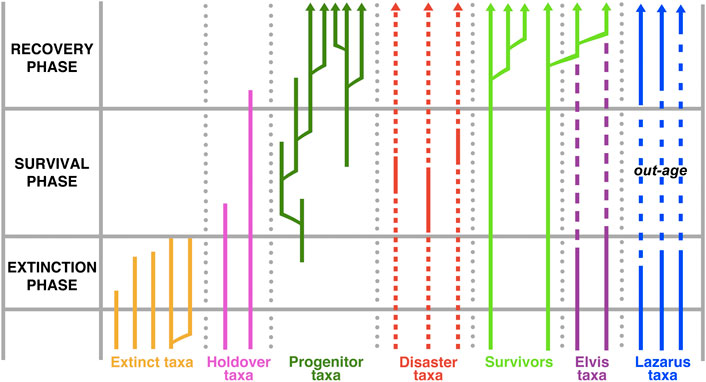
FIGURE 1. Hallam and Wignall (1997) generalized model showing the range of responses of species to mass extinction.
The end-Permian mass extinction (EPME) of ca. 252 Ma is widely regarded to be the most severe of the “Big Five” extinctions. The diversities of late Permian marine and terrestrial organisms were greatly impacted, with many groups becoming extinct (e.g., trilobites, eurypterids, gorgonopsian synapsids, and pareiasaurian parareptiles), followed by a multi-million-year-long hiatus in coal deposition and reef formation (Erwin, 1993, 2006; Jin et al., 2000; Benton, 2003; Benton and Twitchett, 2003; Wignall, 2007). A major focus of research programs of the EPME has been to determine whether the marine and terrestrial extinctions were synchronous (e.g. Twitchett et al., 2001; Ward et al., 2005; Shen et al., 2011; Gastaldo et al., 2020). Pursuant to that goal, Smith and Botha (2005) began a long-term collecting program in the Karoo Basin of South Africa to produce a species-level resolution of the biostratigraphic ranges of terrestrial vertebrates spanning the Permo-Triassic boundary (PTB). Smith and Botha (2005) identified “extinction,” “survivor,” and “recovery” faunas for PTB Karoo tetrapods, which were recognized in successive publications (Botha and Smith, 2006; Botha et al., 2007; Modesto and Botha-Brink, 2010), and refined in their more recent work (Smith and Botha-Brink, 2014).
A taxon that has played a chief role in research of tetrapod survivorship of the EPME is the anomodont synapsid genus Lystrosaurus. Species of this genus have been described from Asia (China and India), Europe (Russia), Africa (South Africa and Zambia), and Antarctica (e.g. Young, 1946; Cluver, 1971; Colbert, 1974; King, 1991; King and Jenkins, 1997; Ray, 2005; Surkov et al., 2005). Lystrosaurus fossils are the most commonly encountered vertebrate remains in lowermost Triassic rocks of the Karoo Basin, South Africa; Groenewald and Kitching (1995) estimated that 95% of the fossils from the Lystrosaurus declivis Assemblage Zone of the Karoo Basin are attributable to Lystrosaurus. As a consequence of this remarkable abundance and co-occurrence with the EPME, the genus Lystrosaurus is commonly described a “disaster taxon” (Wignall, 2007; Adams, 2008; Sahney and Benton, 2008; Benton, 2011; Kammerer et al., 2013; Botha-Brink et al., 2016; Brocklehurst et al., 2018).
The label of “disaster taxon” applied to Lystrosaurus—a genus of herbivorous tetrapods—is quite remarkable given the traditional application of this term to life of the past. The designation “disaster taxa” (nb. plural) was introduced by Copper (1994) to describe microbes, foraminiferans, and bryozoans of varying ranks (e.g. genus to ordinal) that became prolific in the wake of a biotic crisis. Hallam and Wignall (1997, p. 13) described disaster taxa as “usually long-ranged species of opportunists, whose presence in swarm abundances is a sure sign of elevated environmental stresses”. In the same work, they regarded the Lystrosaurus Assemblage Zone of South Africa (sensu Groenewald and Kitching 1995) as a “disaster-taxon assemblage” (Hallam and Wignall, 1997, p. 111). That description is the first to intimate, but not state specifically, that Lystrosaurus is a disaster taxon. Hallam and Wignall (1997) stated that the stratigraphic range of the genus Lystrosaurus is relatively short, especially if compared with that of the Capitanian–Anisian bivalve genus Claraia, but the range of the former genus is similar to that estimated for dinosaur genera (Dodson, 1990) and so may be considered unremarkable for a tetrapod genus. Accordingly, the recent branding of Lystrosaurus as a disaster taxon appears to be based largely upon its reported abundance and global distribution, and not the stratigraphic/longevity perspective attached to the concept (Hallam and Wignall, 1997; Rodland and Bottjer, 2001). However, the abundance of the genus Lystrosaurus varies in the basins where it has been found, and it is not known from every basin that preserves an Early Triassic tetrapod fauna (e.g. Dias-da-Silva et al., 2007; Dias-da-Silva et al., 2017). A further complication for statements that Lystrosaurus is a disaster taxon is that those works that have done so have neither provided a definition for “disaster taxon” nor cited a previously published definition; where such definitions appear in the literature, they vary from work to work.
In this paper I examine the basis, if any, for statements that the genus Lystrosaurus is a disaster taxon. Recent advances in the biostratigraphy of terrestrial vertebrates of the Karoo Basin of South Africa, which yields the most abundant fossils of Lystrosaurus (Botha and Smith, 2006; Botha and Smith, 2007; Botha and Smith, 2020; Viglietti et al., 2015), allow an assessment of the claim that Lystrosaurus is a disaster taxon.
Discussion
Lystrosaurus as a “Disaster Taxon”
The term “disaster taxon” evolved from the earlier term “disaster species”. The latter was first used by Percival and Fischer (1977) to describe the calcareous nannoplankton that appeared in bloom-like abundances in the earliest Tertiary seas. The term was adopted by Kauffman (1984) and Harries and Kauffman (1990) in their reviews of marine-life survivorship of the end-Cretaceous mass extinction (ECME). Schubert and Bottjer (1992) introduced the term “disaster form” for Early Triassic stromatolites (nb. the term “disaster form” reflects the nature of fossil stromatolites as ichnotaxa). Finally, Copper (1994:3) described “…‘simpler’, stress resistant disaster taxa at the genus to ordinal (or even phylum) level” for marine invertebrates that bloomed in the wake of reef collapse.
Subsequent considerations of “disaster taxa” concept evolved in the context of anatomizing a mass extinction event into extinction, survival, and recovery intervals, and restricting the term “disaster taxon” to low-level taxa (i.e. genus and/or species). Whereas Schubert and Bottjer (1992) and Schubert and Bottjer (1995) regarded “disaster forms” as “long-ranging opportunistic generalists that briefly proliferate in the aftermath of mass extinctions,” Kauffman and Erwin (1995), Kauffman and Harries (1996), and Harries et al. (1996) distinguished “disaster species” (disaster taxa) from “opportunistic species.” These categories of survivors were proposed to bloom successively in the survival interval of a mass extinction event (Figure 1); Kauffman and Harries (1996) suggested that disaster species were probably “r-strategists,” i.e. short-lived species that produce abundant offspring. Subsequently, Rodland and Bottjer (2001) conflated these survivor concepts and defined “disaster taxa” as “a subgroup of opportunistic taxa, characterised by long evolutionary histories, that invade vacant ecospace during the survival interval but which are forced into marginal settings during later phases of the recovery.” This concept has been embraced by many researchers (e.g., Petsios and Bottjer, 2016; Song et al., 2016; Lucas, 2017).
The Rodland and Bottjer (2001) disaster-taxon concept does not apply to the genus Lystrosaurus for several reasons. As mentioned in the Introduction, Lystrosaurus does not exhibit a remarkably long stratigraphic range compared to those known for other tetrapod genera. The lowermost occurrence for the genus is L. maccaigi at the boundary between the Dicynodon-Theriognathus (lower subzone) and Lystrosaurus maccaigi-Moschorhinus (upper subzone) vertebrate subzones, respectively, of Viglietti (2020) of the Daptocephalus Assemblage Zone (uppermost Balfour Formation of South Africa; Changhsingian; Viglietti et al., 2017; Viglietti, 2020), and the uppermost occurrence is L. declivis in the upper Lystrosaurus declivis Assemblage Zone (uppermost Katberg Formation of South Africa; Olenekian; Botha and Smith, 2007; Botha and Smith, 2020). As the Lystrosaurus maccaigi-Moschorhinus vertebrate subzone of the Daptocephalus Assemblage Zone and the Lystrosaurus declivis Assemblage Zone are contained entirely within the Changhsingian through to the Olenekian stages, which totals 6.94 Ma according to the International Committee for Stratigraphy (2020), this indicates that the genus ranged less than 7 Ma. To my knowledge, no one has gauged the longevity of non-mammalian synapsid genera, but Dodson (1990) estimated that dinosaur genera ranged 5–10.5 Ma, and estimated that 7.7 Ma was a likely average. Accordingly, the longevity of the genus Lystrosaurus seems quite comparable. In addition, there is no evidence that the youngest known representatives of the genus, L. declivis and L. murrayi, were forced into marginal (ecologically suboptimal) settings during later phases of the recovery: no herbivorous tetrapods co-occur with L. declivis and L. murrayi in the upper parts of their stratigraphic ranges in the Katberg Formation; the highest occurrences of these species lie below the contact between the Katberg Formation and the overlying Burgersdorp Formation, the upper portion of which preserves the herbivorous/omnivorous cynognathian Diademodon tetragonus (Hancox et al., 1995; Botha and Smith, 2007). Accordingly, there is a brief hiatus in the ranges of herbivorous tetrapods in the Karoo Basin of South Africa, so it is not possible to state that L. declivis and L. murrayi were forced into marginal settings later during the later recovery phase.
The primary impetus for regarding Lystrosaurus as a disaster taxon is its renowned abundance. However, Lystrosaurus fossils are not remarkably abundant outside of the Karoo Basin of South Africa, where they were estimated by Groenewald and Kitching (1995) to comprise 19 out of every 20 fossils found in the Lystrosaurus declivis Assemblage Zone. This estimate, of course, is drawn from recollection of those researchers’ traditional field collecting at Lystrosaurus AZ localities, which predated the precise logging of later field workers (Ward et al., 2005; Smith and Botha, 2005; Botha and Smith, 2006; Botha and Smith, 2007; Botha-Brink et al., 2014; Botha et al., 2019). More recent field and collection assessments indicate that the Groenewald and Kitching (1995) figure is inflated, and that Lystrosaurus specimens actually comprise ca. 73% of the vertebrate fossils known from the Lystrosaurus declivis AZ (Smith et al., 2012). Smith and Botha (2005) suggested that the “over abundance” of Lystrosaurus specimens (implied by Groenewald and Kitching, 1995), particularly “monospecific bonebeds” in the lower Katberg Formation, was a taphonomic bias resulting from the concentration of these dicynodonts at lowland floodplain “waterholes,” which dried up periodically. They subsequently recognized a “Lystrosaurus abundant zone” in the lower Katberg Formation, which looks appreciably like a post-extinction “bloom” (Botha and Smith, 2006; Figure 7). However, this “Lystrosaurus abundant zone” lies ca. 50 m above the Permian-Triassic boundary and falls within Smith and Botha (2005) “recovery fauna,” a stratigraphic observation at odds with the general concept that disaster taxa bloom in the immediate aftermath of a mass extinction, a.k.a. the survivor interval (Kauffman and Erwin, 1995; Harries et al., 1996; Kauffman and Harries, 1996; Rodland and Bottjer, 2001). Accordingly, even though recent biostratigraphic work suggests that Lystrosaurus fossils form an anomalously abundant interval, this interval does not coincide with the expected survival interval of the EPME.
To conclude this section, the genus Lystrosaurus fails to meet all of the criteria that have been established for a disaster taxon (sensu Harries et al., 1996; Rodland and Bottjer, 2001). I suspect that any attempt to apply the term “disaster taxon” to any taxon above the species level, particularly a speciose group of organisms such as Lystrosaurus, is problematic, because there is always the possibility of attributing qualities of a subset of species to the entire group.
Does the Genus Lystrosaurus Include Any Disaster Species?
As noted above, Smith and Botha (2005) informally recognized a “Lystrosaurus abundant zone” for L. declivis and L. murrayi in the lower Katberg Formation of South Africa. This putative “Lystrosaurus abundant zone” could be equated to the blooms that characterize the distribution profiles of disaster and opportunistic species of Kauffman and Harries (1996, Figure 1) and Harries et al. (1996, Figures 5, 6). However, the Smith and Botha (2005) identification of “extinction,” “survivor,” and “recovery” faunas among the Permo-Triassic tetrapods of the Karoo Basin is not compatible with the “extinction,” “survivor,” and “recovery” intervals of mass extinctions that were reconstructed in the 1990s (Kauffman and Erwin, 1995; Harries et al., 1996; Kauffman and Harries, 1996), in that the tetrapod faunas of Smith and Botha (2005) overlap in time whereas the mass-extinction intervals of Kauffman and his colleagues are discrete time units. The “extinction,” “survivor,” and “recovery” faunas of Smith and Botha (2005) suggest that the “extinction,” “survivor,” and “recovery” intervals theorized in the 1990s for mass extinctions are not a realistic reconstruction of biotic responses during and immediately after a mass extinction event. The Smith and Botha (2005) recognition of overlapping “extinction,” “survivor,” and “recovery” faunas for PTB tetrapods are echoed in the conclusions of Lindstrom and McLoughlin (2007) and Hochuli et al. (2010) for PTB plants in eastern Pangaea and north-central Laurasia, respectively.
The sparse vertebrate paleontology literature that touches upon disaster species has eschewed the association of disaster species with a particular substage of a mass extinction. Benton (2003, p. 303), for example, defined disaster species as “forms that are able to radiate soon after a crisis.” More recently, Benton et al. (2014) define disaster species as “a species that survives and diversifies in post-extinction conditions, but disappears without giving rise to major components of the longer-term ecosystem.” A fundamental problem with both of these definitions is that the terms “diversifies” and “radiate” imply that a disaster species undergoes additive speciation in “post-extinction conditions.” Neither definition applies to any of the species of Lystrosaurus because stratigraphic calibration of the currently accepted tree topologies for the genus (Figure 2) demonstrate clearly that speciation events in this genus occurred before the PTB; there is no compelling evidence for any post-extinction speciation within the genus Lystrosaurus.
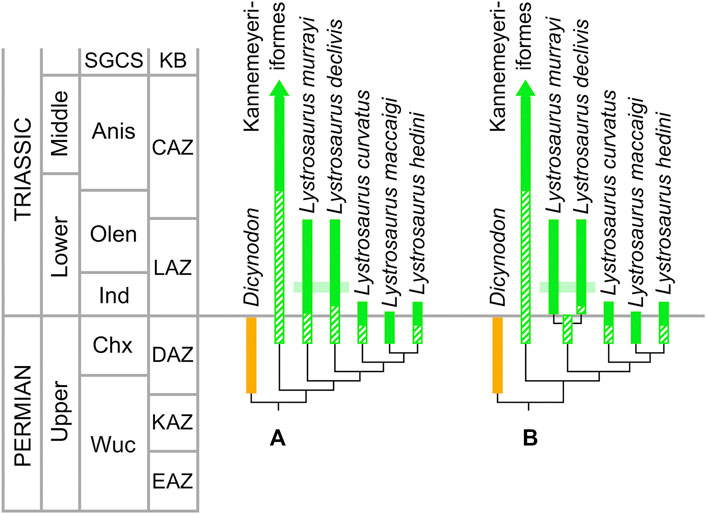
FIGURE 2. Lystrosaurid diversity across the Permo-Triassic boundary with stratigraphic data from Botha and Smith (2006), Smith and Botha-Brink (2014), Botha et al. (2019), Smith et al. (2020), and Viglietti et al. (2020), based on the leading tree topologies for the genus Lystrosaurus. (A) Topology 1 in which Lystrosaurus murrayi and L. declivus form a grade (e.g. Kammerer et al., 2013; Kammerer and Smith 2017; Cox and Angielczyk, 2015). (B) Topology 2 in which L. murrayi and L. declivus are sister taxa (Kammerer, 2019a; Kammerer, 2019b; Kammerer et al., 2019; Olroyd et al., 2017). Dicynodon and Kannemeyeriiformes are outgroups. Stratigraphic ranges of survivor/recovery taxa in medium green and extinction taxa in orange. Thick bars are known stratigraphic ranges; ghost lineages and ghost taxa are indicated by diagonal hatching; relationships indicated by thin black branches. Light green field represents “Lystrosaurus abundant zone” of Botha and Smith (2006). Lystrosaurid tree topologies from Angielczyk et al. (2013), Kammerer and Smith (2017), Kammerer (2019a), Kammerer (2019b), and Kammerer et al. (2019). Abbreviations: Anis, Anisian; CAZ, Cynognathus Assemblage Zone; Chx, Changhsingian; DAZ, Daptocephalus Assemblage Zone; EAZ, Endothiodon Assemblage Zone; Ind, Induan; KAZ, Cistecephalus Assemblage Zone; KB, Karoo biozones; LAZ, Lystrosaurus declivis Assemblage Zone; Olen, Olenekian; Wuc, Wuchiapingian.
To conclude this section, none of the species of Lystrosaurus meet the recent definitions for disaster species (Benton, 2003; Benton et al., 2014). Previously published definitions that associate disaster species with a critical time period (viz. “survival phase”) are called into question by recent studies that reconstruct overlapping extinction, survivor, and recovery biotas, and their utility is now doubtful, at least for continental biotas. Tetrapods such as L. declivis and L. murrayi that exhibit anomalously high post-extinction numbers could be identified, at most, as opportunistic species.
Conclusion
The dicynodont genus Lystrosaurus is commonly described as a disaster taxon in the recent literature on the end-Permian extinction event. However, those works that specifically describe Lystrosaurus as a disaster taxon neither refer to, nor provide, a definition of the term, and this problem is exacerbated by the numerous definitions that have appeared in the literature. The current phylogenetic and stratigraphic information available for species of Lystrosaurus fails to satisfy any of the published definitions for the term “disaster taxon,” leading to the conclusion that the term, at present, is too subjective and thus has questionable utility for studies of tetrapod survivorship of mass extinctions.
The concept of disaster species appears to be valid for many marine forms (e.g., Song et al., 2016), but those identified so far are suspension feeders (foraminifera, brachiopods, and bivalves). Their benthic marine ecology is very different from that of late Permian and Early Triassic Karoo tetrapods, which formed terrestrial vertebrate ecosystems of modern aspect (Sues and Reisz, 1998), where large numbers of herbivores support a relatively smaller number of carnivores (i.e. primary consumer biomass dwarfs that of secondary and higher consumers). In the case of the Lystrosaurus declivis Assemblage Zone fauna, L. murrayi and L. declivis comprised the “large number of herbivores” of the Karoo terrestrial vertebrate ecosystem, in lieu of that formed by a greater taxonomic diversity of herbivorous tetrapods recorded for the preceding late Permian Daptocephalus Assemblage Zone.
Data Availability Statement
The original contributions presented in the study are included in the article/Supplementary Material, further inquiries can be directed to the corresponding author.
Author Contributions
The author confirms being the sole contributor of this work and has approved it for publication.
Funding
This work was supported by a New Opportunities Fund award from the Canadian Foundation for Innovation and a grant from the Nova Scotia Research and Innovation Trust.
Conflict of Interest
The author declares that the research was conducted in the absence of any commercial or financial relationships that could be construed as a potential conflict of interest.
Acknowledgments
I thank J. Neveling and A. G. Martinelli for their constructive criticisms and SL for his editorial acumen. Jennifer Botha and Darla K. Zelenitsky are thanked for comments on an earlier version of the manuscript.
References
Adams, J. (2008). Species richness: patterns in the diversity of life. Dordrecht, Netherlands: Springer.
Angielczyk, K. D., Steyer, J.-S., Sidor, C. A., Smith, R. M. H., Whatley, R., and Tolan, S. (2013). “Permian and Triassic dicynodont (Therapsida: Anomodontia) faunas of the Luangwa Basin, Zambia: taxonomic update and implications for dicynodont biogeography and biostratigraphy,” in The early evolutionary history of synapsida. Editors C. F. Kammerer, K. D. Angielczyk, and J. Fröbisch (Dordrecht, Netherlands: Springer), 93–138.
Benton, M. J. (2011). Mass extinctions: the terrible two. New Sci. 209, iv–v. doi:10.1016/S0262-4079(11)60498-3
Benton, M. J. (2003). When life nearly died: the greatest mass extinction of all time. London: Thames and Hudson.
Benton, M. J., and Twitchett, R. (2003). How to kill (almost) all life: the end-Permian extinction event. Tr. Ecol. Evol. 18, 358–365. doi:10.1016/S0169-5347(03)00093-4
Benton, M. J., Forth, J., and Langer, M. C. (2014). Models for the rise of the dinosaurs. Current Biol. 24, R87–R95. doi:10.1016/j.cub.2013.11.063
Botha, J., and Smith, R. M. H. (2020). Biostratigraphy of the Lystrosaurus declivis Assemblage Zone (Beaufort group, Karoo supergroup), South Africa. S. Afr. J. Geol. 123, 207–216. doi:10.25131/sajg.123.0015
Botha, J., and Smith, R. M. H. (2007). Lystrosaurus species composition across the Permo–triassic boundary in the Karoo Basin of South Africa. Lethaia 40, 125–137. doi:10.1111/j.1502-3931.2007.00011.x
Botha, J., and Smith, R. M. H. (2006). Rapid vertebrate recuperation in the Karoo Basin of South Africa following the end-Permian extinction. J. Afr. Earth Sci. 45, 502–514. doi:10.1016/j.jafrearsci.2006.04.006
Botha, J., Huttenlocker, A., Smith, R., Prevec, R., Viglietti, P., and Modesto, S. P. (2019). New geochemical and palaeontological data from the Permo-Triassic boundary in the South African Karoo Basin test the synchronicity of terrestrial and marine extinctions. Palaeogeo. Palaeoclimatol. Palaeoecol. 540, 109467. doi:10.1016/j.palaeo.2019.109467
Botha, J., Modesto, S. P., and Smith, R. M. H. (2007). Extended procolophonid reptile survivorship after the end-Permian extinction. S. Afr. J. Sci. 103, 54–56.
Botha-Brink, J., Codron, D., Huttenlocker, A. K., Angielczyk, K. D., and Ruta, M. (2016). Breeding young as a strategy during Earth’s greatest mass extinction. Sci. Rep. 6:e24053. doi:10.1038/srep24053
Botha-Brink, J., Huttenlocker, A. K., and Modesto, S. P. (2014). “Vertebrate paleontology of Nooitgedacht 68: a Lystrosaurus maccaigi-rich Permo-Triassic boundary locality in South Africa,” in The early evolutionary history of synapsida. Editors C. F. Kammerer, K. D. Angielczyk, and J. Fröbisch (Dordrecht, Netherlands: Springer), 289–304.
Brocklehurst, N., Day, M. O., and Fröbisch, J. (2018). Accounting for differences in species frequency distributions when calculating beta diversity in the fossil record. Methods Ecol. Evol. 7, 1409–1420. doi:10.1111/2041-210X.13007
Cluver, M. A. (1971). The cranial morphology of the dicynodont genus Lystrosaurus. Ann. S. Afr. Mus. 56, 155–274.
Copper, P. (1994). Ancient reef ecosystem expansion and collapse. Coral Reefs 13, 3–11. doi:10.1007/bf00426428
Cox, C. B., and Angielczyk, K. D. (2015). A new endothiodont dicynodont (Therapsida, Anomodontia) from the Permian Ruhuhu Formation (Songea group) of Tanzania and its feeding system. From the permian ruhuhu formation (Songea group) of Tanzania and its feeding system J. Vertebr. Paleontol. 35:e935388. doi:10.1080/02724634.2014.935388
Dias-da-Silva S., , , Modesto, S. P., and Schultz, C. L. (2007). New material of procolophon (Parareptilia: Procolophonoidea) from the Lower Triassic of Brazil, with remarks on the ages of the Sanga do Cabral and Buene Vista formations of South America. Can. J. Earth Sci. 43, 1685–1693. doi:10.1139/e06-043
Dias-da-Silva, S., Pinheiro, F., Da-Rosa, Á. A. S., Martinelli, A. G., Schultz, C. L., Silva-Neves, E., et al. . (2017). Biostratigraphic reappraisal of the Lower Triassic Sanga do Cabral Supersequence from South America, with a description of new material attributable to the parareptile genus Procolophon. J. South Amer. Earth Sci 79, 281–296. doi:10.1016/j.jsames.2017.07.012
Dodson, P. (1990). Counting dinosaurs: how many kinds were there? Proc. Natl. Acad. Sci. 87, 7608–7612. doi:10.1073/pnas.87.19.7608
Erwin, D. H. (2006). Extinction: how life on earth nearly ended 250 million years ago. New Jersey: Princeton University Press.
Erwin, D. H. (1993). The great Paleozoic crisis: life and death in the Permian. New York: Columbia University Press.
Gastaldo, R. A., Kamo, S. L., Neveling, J., Geissman, J. W., Looy, C. V., and Martini, A. M. (2020). The base of the Lystrosaurus Assemblage Zone, Karoo Basin, predates the end-Permian marine extinction. Nat. Commun. 11, 1428. doi:10.1038/s41467-020-15243-7
Groenewald, G. H., and Kitching, J. W. (1995). “Biostratigraphy of the Lystrosaurus Assemblage Zone,” in Biostratigraphy the Beaufort Group (Karoo Supergroup). Editor B. S. Rubidge (Pretoria: South African Committee for Stratigraphy), 35–39.
Hallam, A., and Wignall, P. B. (1997). Mass extinctions and their aftermath. Oxford: Oxford University Press.
Hancox, P. J., Shishkin, M. A., Rubidge, B. S., and Kitching, J. W. (1995). A threefold subdivision of the Cynognathus Assemblage Zone (Beaufort group, South Africa) and its palaeogeographical implications. S. Afr. J. Sci. 91, 143–144.
Harries, P. J., and Kauffman, E. G. (1990). “Patterns of survival and recovery following the Cenomanian-Turonian (Late Cretaceous) mass extinction in the western Interior Basin, United States,” in Extinction events in earth history. Lecture notes in earth sciences. Editors E. G. Kauffman, and O. H. Walliser (Berlin: Springer), Vol. 30, 277–298.
Harries, P. J., Kauffman, E. G., and Hansen, T. A. (1996). “Models for biotic survival following mass extinction,” in Biotic recovery from mass extinction events. Editor H. B. Hart (Bath: Geological Society Special Publication), Vol. 102, 41–60.
Hochuli, P., Hermann, E., Vigran, J. O., Bucher, H., and Weissert, H. (2010). Rapid demise and recovery of plant ecosystems across the end-Permian extinction event. Global Planet. Change 74, 144–155. doi:10.1016/j.gloplacha.2010.10.004
International Committee for Stratigraphy (2020). International chronostratigraphic chart. Available at: https://stratigraphy.org (Accessed October 31, 2020).
Jin, Y.-G., Wang, Y., Wang, W., Shang, Q.-H., Cao, C.-Q., and Erwin, D. H. (2000). Pattern of marine mass extinction near the Permian-Triassic boundary in south China. Science 289, 432–436. doi:10.1126/science.289.5478.432
Kammerer, C. F. (2019a). A new dicynodont (Anomodontia: Emydopoidea) from the terminal Permian of KwaZulu-Natal, South Africa. Palaeontol. Afr. 53, 179–191.
Kammerer, C. F. (2019b). Revision of the Tanzanian dicynodont Dicynodon huenei (Therapsida: Anomodontia) from the Permian Usili formation. PeerJ 7, e7420. doi:10.7717/peerj.7420
Kammerer, C. F., and Smith, R. M. H. (2017). An early geikiid dicynodont from the Tropidostoma Assemblage Zone (late Permian) of South Africa. PeerJ 5:e2913. doi:10.7717/peerj.2913
Kammerer, C. F., Fröbisch, J., and Angielczyk, K. D. (2013). On the validity and phylogenetic position of Eubrachiosaurus browni, a kannemeyeriiform dicynodont (Anomodontia) from Triassic North America. PLoS ONE 8, e64203. doi:10.1371/journal.pone.0064203
Kammerer, C. F., Viglietti, P. A., Hancox, P. J., Butler, R. J., and Choiniere, J. N. (2019). A new kannemeyeriiform dicynodont (Ufudocyclops mukanelai, gen. et sp. nov.) from subzone C of the Cynognathus Assemblage Zone, Triassic of South Africa, with implications for biostratigraphic correlation with other African Triassic faunas. J. Vertebr. Paleont. 39, e1596921. doi:10.1080/02724634.2019.1596921
Kauffman, E. G. (1984). “The fabric of Cretaceous marine extinctions,” in Catastrophes in earth history. Editors W. A. Bergren and J. A. Van Couvering (Princeton: Princeton University Press), 151–246.
Kauffman, E. G., and Harries, P. J. (1996). “The importance of crisis progenitors in recovery from mass extinction,” in Biotic recovery from mass extinction events. Editor M. B. Hart (Bath: Geological Society), 15–39.
King, G. M. (1991). The aquatic Lystrosaurus: a palaeontological myth. Hist. Biol. 4, 285–321. doi:10.1080/08912969009386547
King, G. M., and Jenkins, I. (1997). The dicynodont Lystrosaurus from the upper Permian of Zambia: evolutionary and stratigraphical implications. Palaeontology 40, 149–156.
Lindström, S., and McLoughlin, S. (2007). Synchronous palynofloristic extinction and recovery after the end-Permian event in the Prince Charles Mountains, Antarctica: implications for palynofloristic turnover across Gondwana. Rev. Palaeobot. Palynol. 145, 89–122. doi:10.1016/j.revpalbo.2006.09.002
Lucas, S. G. (2017). Permian tetrapod extinction events. Earth Sci. Rev. 170, 31–60. doi:10.1016/j.earscirev.2017.04.008
Modesto, S. P., and Botha-Brink, J. (2010). Problems of correlation of South African and South American tetrapod faunas across the Permian–Triassic boundary. J. Afr. Earth Sci. 57, 242–248. doi:10.1016/j.jafrearsci.2009.08.004
Olroyd, S. L., Sidor, C. A., and Angielczyk, K. D. (2017). New materials of the enigmatic dicynodont Abajudon kaayai (Therapsida, Anomodontia) from the lower Madumabisa Mudstone Formation, middle Permian of Zambia. J. Vertebr. Paleontol. 37:e1403442. doi:10.1080/02724634.2017.1403442
Percival, S. F., and Fischer, A. G. (1977). Changes in calcareous nannoplankton in the Cretaceous-Tertiary biotic crisis at Zumaya Spain. Evol. Theory 2, 1–35.
Petsios, E., and Bottjer, D. J. (2016). Quantitative analysis of the ecological dominance of benthic disaster taxa in the aftermath of the end-Permian mass extinction. Paleobiology 42, 380–393. doi:10.1017/pab.2015.47
Ray, S. (2005). Lystrosaurus (Therapsida, Dicynodontia) from India: taxonomy, relative growth, and cranial dimorphism. J. Syst. Palaeontol. 3, 203–221. doi:10.1017/S1477201905001574
Rodland, D. L., and Bottjer, D. J. (2001). Biotic recovery from the end-Permian mass extinction: Behavior of the inarticulate brachiopod Lingula as a disaster taxon. Palaios 16, 95–101. doi:10.1669/0883-1351(2001)016<0095:BRFTEP>2.0.CO;2
Sahney, S., and Benton, M. J. (2008). Recovery from the most profound mass extinction of all time. Proc. B Roy. Soc. 275, 759–765. doi:10.1098/rspb.2007.1370
Schubert, J. K., and Bottjer, D. J. (1995). Aftermath of the Permo-Triassic mass extinction event: paleoecology of Lower Triassic carbonates in the western USA. Palaeogeogr. Palaeoclimatol. Palaeoecol. 116, 1–39. doi:10.1016/0031-0182(94)00093-N
Schubert, J. K., and Bottjer, D. J. (1992). Early Triassic stromatolites as post-mass extinction disaster forms. Geology 20, 883–886. doi:10.1130/0091-7613(1992)020<0883:ETSAPM>2.3.CO;2
Shen, S.-Z., Crowley, J. L., Wang, Y., Bowring, S. A., Erwin, D. H., Sadler, P. M., et al. . (2011). Calibrating the end-Permian mass extinction. Science 334, 1367–1372. doi:10.1126/science.1213454
Smith, R. M. H., and Botha, J. (2005). The recovery of terrestrial vertebrate diversity in the South African Karoo Basin after the end-Permian extinction. Comptes Rendus Palevol 4, 555–568. doi:10.1016/j.crpv.2005.07.005
Smith, R. M. H., and Botha-Brink, J. (2014). Anatomy of a mass extinction: Sedimentological and taphonomic evidence for drought-induced die-offs at the Permo-Triassic boundary in the main Karoo Basin, South Africa. Palaeogeogr. Palaeoclimatol. Palaeoecol. 396, 99–118. doi:10.1016/j.palaeo.2014.01.002
Smith, R. M. H., Rubidge, B. S., Day, M. O., and Botha, J. (2020). Introduction to the tetrapod biozonation of the Karoo Supergroup. S. Afr. J. Geol. 123, 131–140. doi:10.25131/sajg.123.0009
Smith, R. M. H., Rubidge, B. S., and van der Walt, M. (2012). “Therapsid biodiversity patterns and paleoenvironments of the Karoo Basin, South Africa,” in Forerunners of mammals: radiation, histology, biology. Editor A. Chinsamy-Turan (Bloomington: Indiana University Press), 223–246.
Song, H., Tong, J., Wignall, P. B., Luo, M., Tian, L., Song, H., et al. . (2016). Early Triassic disaster and opportunistic foraminifers in South China. Geol. Mag. 153, 298–315. doi:10.1017/S0016756815000497
Sues, H.-D., and Reisz, R. R. (1998). Origins and early evolution of herbivory in tetrapods. Tr. Ecol. Evol. 13, 141–145. doi:10.1016/S0169-5347(97)01257-3
Surkov, M. V., Kalandadze, N. N., and Benton, M. J. (2005). Lystrosaurus georgi, a dicynodont from the Lower Triassic of Russia. J. Vertebr. Paleontol. 25, 402–413. doi:10.1671/0272-4634(2005)025[0402:LGADFT]2.0.CO;2
Twitchett, R. J., Looy, C. V., Morante, R., Visscher, H., and Wignall, P. B. (2001). Rapid and synchronous collapse of marine and terrestrial ecosystems during the end-Permian biotic crisis. Geology 29, 351–354. 10.1130/0091-7613(2001)029<0351:RASCOM>2.0.CO;2
Viglietti, P. A. (2020). Biostratigraphy of the Daptocephalus Assemblage Zone (Beaufort Group, Karoo Supergroup), South Africa. S. Afr. J. Geol. 123, 191–206. doi:10.25131/sajg.123.0014
Viglietti, P. A., Smith, R. M. H., Angielczyk, K. D., Kammerer, C. F., Fröbisch, J., and Rubidge, B. S. (2015). The Daptocephalus Assemblage Zone (Lopingian), South Africa: a proposed biostratigraphy based on a new compilation of stratigraphic ranges. J. Afr. Earth Sci. 113, 153–164. doi:10.1016/j.jafrearsci.2015.10.011
Viglietti, P. A., Smith, R. M. H., and Rubidge, B. S. (2017). Changing palaeoenvironments and tetrapod populations in the Daptocephalus Assemblage Zone (Karoo Basin, South Africa) indicate early onset of the Permo-Triassic mass extinction. J. Afr. Earth Sci. 138, 102–111. doi:10.1016/j.jafrearsci.2017.11.010
Ward, P. D., Botha, J., Buick, R., De Cock, M. O., Erwin, D. H., Garrison, G. H., et al. . (2005). Abrupt and gradual extinction among Late Permian land vertebrates in the Karoo Basin, South Africa. Science 307, 709–714. doi:10.1126/science.1107068
Webb, A. E., and Leighton, L. R. (2011). “Exploring the ecological dynamics of extinction,” in Quantifying the evolution of early life. Editors M. Laflamme, J. D. Schiffbauer, and S. Q. Dornbob (Dordrecht, Netherlands: Springer), 186–220.
Wignall, P. B. (2007). The end-Permian mass extinction–how bad did it get? Geobiology 5, 303–309. doi:10.1111/j.1472-4669.2007.00130.x
Keywords: Triassic, Permian, extinction, disaster, Lystrosaurus
Citation: Modesto SP (2020) The Disaster Taxon Lystrosaurus: A Paleontological Myth. Front. Earth Sci. 8:610463. doi: 10.3389/feart.2020.610463
Received: 25 September 2020; Accepted: 16 November 2020;
Published: 16 December 2020.
Edited by:
Spencer G. Lucas, New Mexico Museum of Natural History and Science, United StatesReviewed by:
Johann Neveling, Council for Geoscience, South AfricaAgustin G. Martinelli, Federal University of Rio Grande do Sul, Brazil
Copyright © 2020 Modesto. This is an open-access article distributed under the terms of the Creative Commons Attribution License (CC BY). The use, distribution or reproduction in other forums is permitted, provided the original author(s) and the copyright owner(s) are credited and that the original publication in this journal is cited, in accordance with accepted academic practice. No use, distribution or reproduction is permitted which does not comply with these terms.
*Correspondence: Sean P. Modesto, sean_modesto@cbu.ca