- 1CNR-IGG, Consiglio Nazionale delle Ricerche, Istituto di Geoscienze e Georisorse, Via G. La Pira, 4, Firenze, Italy
- 2Dipartimento di Scienze della Terra, Università degli Studi di Firenze, Via G. La Pira, 4, Firenze, Italy
Since caldera collapse deformation is extremely difficult to study in real time - due to the high deformation rates that characterize this process and the difficult access to the caldera structures-analogue modeling has been widely used during past decades to integrate field data and, more recently, remote-sensing data (e.g., InSAR). However, the relationships between caldera collapse and inherited discontinuities, such as inherited crustal faults, remain poorly investigated. We therefore provide a new dataset of analogue models that aims to specifically address this issue and that can be potentially compared with literature and natural case studies worldwide. We present a dataset of 13 analogue models of caldera collapse investigating the interactions between caldera collapse processes and inherited crustal discontinuities. The dataset is composed of raw data and elaborations that can be used to qualitatively visualize and/or quantitatively analyze model deformation through the use of top-view photos, digital elevation models (DEM) and digital particle image velocimetry (DPIV).
1 Introduction
Caldera collapse is a geological process that is elusive to investigate, as its rapid occurrence makes it difficult to study, especially in case of explosive caldera eruptions. Addressing this issue has recently taken advantage of the improvement of remote-sensing techniques (e.g., InSAR), particularly for non-explosive caldera collapses (e.g., Sigmundsson, 2019). Nonetheless, intra-caldera structures are often masked by erosion, draping of post-caldera related volcanic deposits, and infilling of lacustrine sediments, whereby these features may be difficult to analyze (e.g., WoldeGabriel et al., 1990; WoldeGabriel et al., 1992; Le Turdu et al., 1999). For this reason, laboratory analogue experiments represent a powerful tool, and a variety of studies reproducing the collapse of calderas is available in literature (Acocella, 2007; Geyer and Martí, 2014 and reference therein). Many studies reproduced the collapse by applying overpressure mechanisms, which imply a pressure increase in the experimental magma chamber, with consequent superficial doming inducing subsequent collapse (e.g., Komuro.et al., 1984; Martì et al., 1994; Acocella et al., 2001a; Walter and Troll, 2001; Troll et al., 2002). Other models have applied the underpressure principle, supporting the idea of a pressure drop in the magma chamber and consequent collapse (e.g., Acocella et al., 2000; Roche et al., 2000; Acocella et al., 2001b; Kennedy et al., 2004; Lavallée et al., 2004; Geyer et al., 2006; Holohan et al., 2008a; Burchardt and Walter, 2010; Ruch et al., 2012; Coumans and Stix, 2016). Despite it is acknowledged that existing structures may play a significant role during caldera collapse, this process has been limitedly investigated with analogue models (e.g., Cailleau et al., 2003; Acocella et al., 2004; Holohan et al., 2005; Holohan et al., 2008b; Holohan et al., 2013; Bonini et al., 2021). In this Data Report, we present a database of analogue models of collapsed calderas, with specific setups testing the effect of inherited discontinuities.
Our models were carried out in the frame of the Horizon 2020 European GEMex project, which aimed to investigate for geothermal purposes two caldera collapse sites (Los Humeros and Acoculco caldera complexes in Mexico). The models aimed to provide new insights into the structural evolution of the two sites, highlighting the relationships between caldera collapse and inherited structures. The dataset presented in this Data Report contributes to the study of caldera collapse and its relationship with regional tectonics.
In addition, our models can be compared with previous analogue models as well as with natural examples worldwide. All the data available for this dataset (Maestrelli et al., 2020a) can be freely downloaded from the Open-Access Zenodo platform at the link http://doi.org/10.5281/zenodo.4084639.
2 Materials and Methods
The dataset includes 13 analogue models performed during the GEMex project from February 2018 to June 2019 at the Tectonic Modeling Laboratory of the Institute of Geosciences and Earth Resources (IGG-CNR) and the University of Florence.
2.1 Model Setup and Modeling Procedure
The models were built in a 33 cm × 38 cm × 15 cm Plexiglas box, at the base of which is placed an analogue magma chamber (Figure 1A). The latter is a plaster base specifically made by pouring liquid plaster into a silicon mold. The plaster base was designed with a cylindrical empty space (12 cm in diameter and 2 cm in height) filled with an analogue magma. The analogue magma chamber was overlaid by a 6 cm-thick sand mixture pack (defined as “brittle overburden” and composed of Quartz and K-Feldspar sands), mimicking the shallow upper crust. The sand was gently poured on one side of the box and flattened using a scraper to build a 1 cm-thick layer. Six layers were created to reach the total overburden thickness of 6 cm. Each layer was scraped alternatively from opposite directions to minimize particle iso-orientation. The latter may in fact generate weaknesses or induce residual stresses, which may potentially affect the development of caldera structures. Each layer was separated by sieving a very thin (∼1 mm) colored sand level (composed of pure K-feldspar sand), used during model analysis as passive marker of deformation. The collapse was induced by draining the analogue magma out of the analogue magma chamber (i.e., applying the underpressure mechanism) from a plastic tube (0.9 cm in diameter) connected to the base of the chamber by a circular hole (1 cm in diameter). Analogue magma draining was initiated by opening a valve placed at the end of the tube, and letting it flow by gravity. The same volume of analogue magma (65% of the initial amount) was drained out in all the models to ensure model comparison. This standard setup (Figure 1A, named “simple caldera collapse”, SCC) was then varied by introducing discontinuities at different locations that simulate inherited fabrics within the brittle overburden (“overburden discontinuities caldera collapse” ODCC; Figure 1B). The discontinuities were obtained by cutting the sand pile with a knife, which causes the re-orientation of sand particles. This operation induced a variation in grain compaction, as well as a decrease in the angle of internal friction and cohesion, creating millimetric weak zones that can be reactivated during model deformation (e.g., Bellahsen and Daniel, 2005; Maestrelli et al., 2020b).
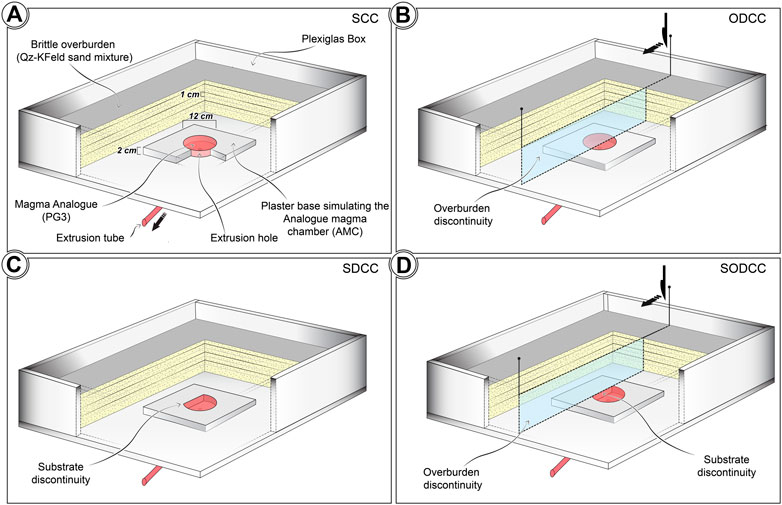
FIGURE 1. Sketch of the analogue model setup. (A) Basic caldera collapse (SCC setup), with a circular analogue magma chamber (AMC) and no inherited discontinuities. (B) Basic setup with an overburden discontinuity (ODCC setup). (C) Setup investigating the effect of a substrate discontinuity (SDCC setup). (D) Combination of ODCC and SDCC setups, investigating the effect of a substrate to overburden discontinuity (SODCC setup). Note that in “b-d” the setups show one sub-vertical discontinuity in the substrate and/or overburden, but models were also designed with two sub-vertical orthogonal discontinuities, or 60° inward/outward dipping discontinuities. The specific setup is shown for each model in the database.
Discontinuities were also introduced in correspondence of the analogue magma chamber, simulating in this way structures in the substratum that delimited the chamber. These discontinuities (“substrate discontinuities caldera collapse”, SDCC; Figure 1C) were simulated by designing the analogue magma chamber with one or two rectilinear sides (vertical or with a 60° inward/outward-dipping attitude). In a third variation of this setup, overburden and substrate discontinuities were combined to test the effect of one or two structures bounding the analogue magma chamber and crosscutting the overburden (“substrate to overburden caldera collapse”, SODCC; Figure 1D). A summary of model parameters is provided in Table 1. In each model, we changed the type/attitude of the inherited discontinuities, with the aim to specifically test its effect. All other parameters were kept constant. Once completed, models were saturated with water and frozen to be cross-sectioned and three-dimensionally analyzed.
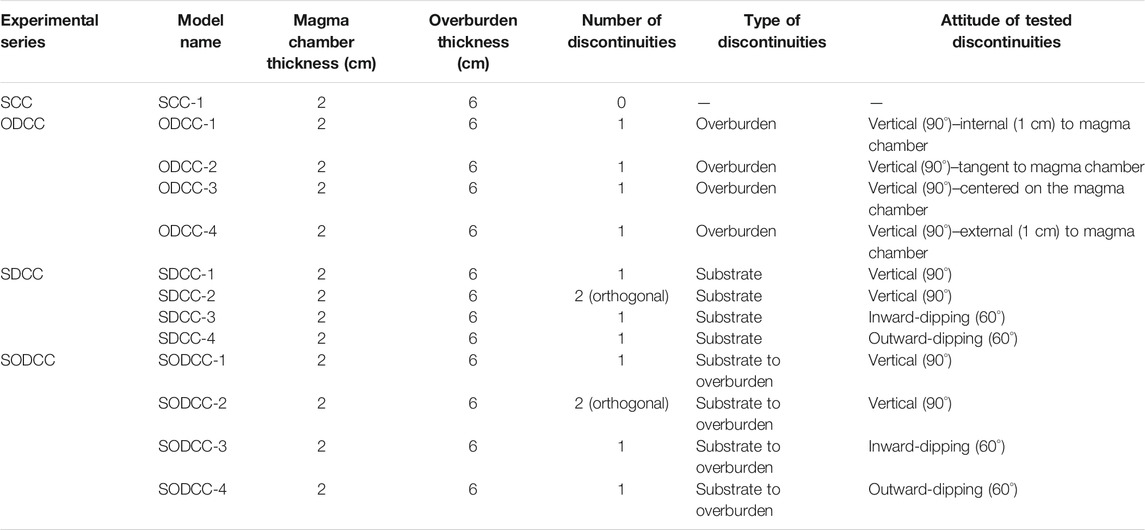
TABLE 1. Summary of adopted model parameters. Each model aimed to test specific characteristics of the inherited discontinuities.
2.2 Analogue Materials
The brittle overburden was simulated with Quartz sand (Fontainebleau sand, provided by Sibelco Italia S.p.A.) and Potassic-feldspar sand (K-feldspar superfine sand FS-900-SF produced by the AKW Kaolin) mixed with a 70:30 proportion in weight. Fontainebleau Quartz sand has an average grain diameter of ∼200 μm and is primarily composed of siliceous and sub-rounded grains. Angular grains of K-feldspar sand have diameters mostly (90%) in the range of 20–100 μm (Montanari et al., 2017a). The resulting 70:30 sand mixture has therefore a density of 1,408 kg m−3 and a cohesion of ∼10 Pa (Table 2). Colored sand layers were obtained using pure K-feldspar superfine sand. The analogue magma was simulated with pure vegetable Polyglycerine-3 (PG3, produced by Spiga Nord S.p.A.). Its viscosity (17 Pa s) is temperature dependent, therefore the laboratory temperature was constantly kept at 25°C before and during model deformation. The parameters of these materials correctly reproduce the properties of rocks of the upper crust and some common magma compositions, respectively (see Section 2.3). A detailed description of their characteristics can be found in Montanari et al. (2017a), Montanari et al. (2017b), Montanari et al. (2020), and Del Ventisette et al. (2019).
2.3 Model Scaling
Scaling of analogue models to nature is necessary for obtaining reliable and quantitative results (Ramberg, 1981). Our models were scaled according to the procedure described in Montanari et al. (2017a). Specifically, we set a length scaling ratio l* = lm/ln = of 10−5, where lm is the length in the model and ln in nature. This scaling ratio implies that 1 cm in the model equals 1 km in nature. The sand mixture density (ρm) of ∼1,550 kg m−3 implies a scaling ratio for density ρ* of ∼0.5, considering a natural rock density (ρn) of ∼2,800 kg m−3. Models were run in natural gravity, so that the g* = 1. From length and density scaling ratios we obtain a stress scaling ratio (σ* = ρ* g* l*) of ∼5 × 10–6. Following Weijermars et al. (1993), a prototype cohesion of zero is suggested for rocks shallower than 10 km, while a cohesion of 60 MPa is assumed for prototype depths higher than 11 km. Our models simulate depths shallower than 10 km, and therefore overburden cohesion of models is low enough (∼10 Pa) to ensure that models are dynamically scaled. The density scaling ratio for the brittle material/natural rock is similar to the scaling ratio calculated for the analogue/natural magma, PG3 density being ρm ∼ 1,280 kg m−3, and the density ρn of an andesitic to rhyolitic magma at ∼1000°C is ∼2,500–2,200 kg m−3 (Murase and McBirney, 1973). Despite the presence of a viscous material to simulate the natural magma, we analyze the brittle deformation of our models, which is strain-rate independent. Therefore, the velocity of magma extrusion does not require scaling. However, the used Polyglycerine-3 (PG3) may reproduce a wide range of values for both the time of deformation and magma viscosity (Montanari et al., 2020). A summary of the scaling and material parameters is reported in Table 2.
3 Description of the Dataset
We describe below the database files, particularly:
1.sketch of each model setup;
2.movies of model deformation;
3.grey-scale input top-view photos for the digital particle image velocimetry (DPIV) analysis;
4.3D fully navigable pdf files showing virtual rendering of analogue models;
5.Digital Elevation Models (DEM) of final model surface to be used for quantitative analysis.
3.1 Sketch of Model Setup
These figures (.jpg) illustrate the setup, as well as the geometry and the specific position of the inherited discontinuities for each model and may serve as a reference for model understanding and their interpretation.
3.2 Movies of Model Deformation
During model deformation, high-resolution (10 MP) top-view photos were acquired (with 120 s time intervals) using a Canon EOS 1100D reflex camera mounted vertically above the model surface. The top-view photos (Figure 2A) were then mounted (with no filters, optical rectification or post editing) as “movies” of deformation, which are provided as. mp4 files that visualize the complete evolution of model deformation throughout time. We also provide original high-resolution top view photos (see section 3).
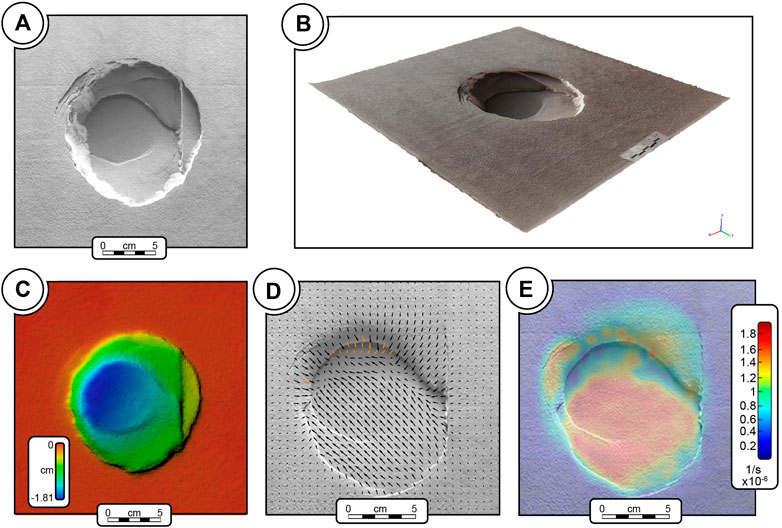
FIGURE 2. Example of data provided and possible outputs derived from the dataset (Model ODCC-1). (A) Top view image acquired during model deformation. These images are provided as separate. jpg files as input for DPIV analysis or mounted in a movie to show the complete model deformation. (B) 3D perspective view of a virtual model, fully navigable to investigate model deformation. (C) Digital Elevation Model. (D,E) Examples of DPIV analysis obtained with the PIVlab tool, particularly (D) velocity vectors and (E) velocity magnitude. These outputs can be obtained from the analysis of the provided input files.
3.3 3D Virtual Models and Digital Elevation Models of Final Deformation
We obtained virtual three-dimensional rendering of caldera collapse using the photogrammetric technique (e.g., Donnadieu et al., 2003; Schonberger and Frahm, 2016) implemented in Agisoft Photoscan® software. Each 3D virtual model (Figure 2B) was obtained by interpolating a minimum of 13 and a maximum of 58 high-resolution (18 MP) perspective photos, acquired with a Canon EOS 1300D reflex camera.
The procedure adopted for 3D virtual model elaboration is summarized below:
1.Perspective photos acquisition
2.Photos upload, alignment and genesis of the sparse cloud (tie points)
3.Genesis of the interpolated dense cloud
4.Interpolation of the model mesh from the dense cloud
5.Genesis and projection of the model texture on the model mesh
Software parameters for this procedure were kept as set by default, with the exception of step 2), for which we increased the Key point limit to 250,000 and the Tie point limit to 40,000.
3D virtual models can be used to three-dimensionally visualize the final stage of the caldera collapse models, and are provided as fully navigable 3D pdf files.
A further step to this procedure allows for DEM reconstruction (Figure 2C). DEMs of final model surface were interpolated from the sparse cloud. The use of markers placed at fixed, and locally geo-referenced positions on the model setup allowed an equal scaling of DEMs. DEM resolution ranges from a minimum of 0.358 mm/pix (Model SDCC-1) to a maximum of 0.246 mm/pix (Model SODCC-3), with an average resolution of 0.309 mm/pix. DEMs are provided as .tiff (geotiff) files and can be used for quantification of the 3D deformation in any GIS software. When opened in a GIS software, DEMs are shown with meters (m) as unit of measurement.
3.4 Digital Particle Image Velocimetry Data
Digital Particle Image Velocimetry (DPIV) analysis was performed on models to evaluate the horizontal displacement by correlating couples of top view images (Figures 2D,E). The analysis was performed using the PIVlab software (Thielicke and Stamhuis, 2014), which can be freely downloaded at https://www.mathworks.com/matlabcentral/fileexchange/27659-pivlab-particle-image-velocimetry-piv-tool. The analysis made use of the high-resolution top-view photos acquired during model deformation, converted into grey-scale (8 bit). For our purposes, we used a 360 s frame time step, applying the FFT window deformation algorithm with a linear window deformation interpolator and “Gauss 2 × 3 point” sub-pixel estimator. The search window was set with three passes: Pass 1 was set with a 64 pixel interrogation area and 32 pixel step; Pass 2 had an interrogation area of 32 pixels and 16 pixels step, while Pass 3 was set with 16 pixels interrogation area and 8 pixels steps. The resulting field of displacement vectors was then calibrated extracting the reference distance from the scale visible in each photo and applying the adopted time step (i.e., 360 s). Obtained vectors where then validated during post-processing to filter out invalid values by defining velocity limits. The resulting post-processed interpolated vectors are shown in orange color by the software, to differentiate them from valid vectors. These settings are not unique, and the dataset user may run the analysis also with different parameters. We therefore provide all the original 8-bit input photos (time step between each photo is 60 s).
4 Brief Description of Analogue Models and Derived Data
Our models show the evolution of the caldera collapse process in the presence of inherited discontinuities. Depending on the model setup, the interaction between structures classically obtained during caldera collapse (see Acocella, 2007) and the inherited discontinuities can be different, highlighting the role exerted by the latter on the process. The presence of an inherited discontinuity placed at a specific location (e.g., internally or externally to the analogue magma chamber), and/or into the overlying overburden may affect the caldera collapse evolution. These aspects are better investigated using DPIV analysis, which provides 2D horizontal vector displacements, which can be used to quantify model deformation. DEMs can be also used for a three-dimensional characterization of the strain resulting from the interaction between inherited discontinuities and the caldera collapse process.
5 Model Limitations
The main limitations of our laboratory experiments are related to the simplifications necessarily introduced in the model setup, which simplifies a portion of the upper crust affected by inherited discontinuities interacting with a magma chamber. Analogue models of caldera collapse cannot simulate temperature/chemical effects, or the role of volatiles during the natural eruptions. It is worth noting that in our setup we did not simulate magma chamber pre-inflation, which is often associated with -and predates-the collapse of silicic calderas. Therefore, the effects of structures associated with pre-caldera bulging is not considered. Furthermore, due to the extremely rapid collapse, especially in natural silicic calderas, analogue models are difficult to be scaled in terms of velocities, something that may affect the quantification of extrusion and collapse rates. Nonetheless, bearing in mind these simplifications, analogue models of caldera collapse have been proven to be highly effective in studying this process (e.g., Acocella, 2007, and reference therein).
6 Possible Applications and Final Remarks
Our models were designed to investigate the caldera collapse process and its interactions with inherited structures. They can therefore provide information on this general issue, prompting the investigation of such a complex geological process. In particular, they can help to build the structural model of specific caldera systems. Furthermore, due to their scaling properties, our models can be compared with the parameters of many caldera systems worldwide, eventually providing some new hints in the evolution of such systems.
Finally, the database here presented may be of large interest for both the volcanological and analogue modeling research communities. Last but not least, the results derived from the analysis of the database may be of large interest for geothermal researches, caldera structures being often the target of geothermal exploration and exploitation.
Data Availability Statement
The datasets generated for this study can be found in the Zenodo Open Acces data repository as Maestrelli et al., 2020a. Caldera collapse vs inherited structures: a database of laboratory experiments [Data set]. Zendo. http://doi.org/10.5281/zenodo.4084639.
Author Contributions
DaM performed the laboratory experiments and elaborated the derived data. Model setup was designed by DaM, MB, DoM, and GC. DaM prepared the dataset and wrote the paper. All the authors contributed to the modeling discussion and to edit the paper. MB obtained funding and supervised the work with DoM.
Funding
The accomplishment of this database has received funding from the European Union (EU)’s Horizon 2020 Program under grant agreement No. 727550 (Project GEMex).
Conflict of Interest
The authors declare that the research was conducted in the absence of any commercial or financial relationships that could be construed as a potential conflict of interest.
Acknowledgments
This work was performed in the frame of the GEMex project. We kindly acknowledge the CFE (Comisión Federal de Electricidad; Mexico) for supporting the GEMex project and for sharing data with the GEMex partners. We are grateful to three anonymous Reviewers for their constructive comments that greatly improved our work.
References
Acocella, V., Cifelli, F., and Funiciello, R. (2000). Analogue models of collapse calderas and resurgent domes. J. Volcanol. Geoth. Res. 104 (1-4), 81–96. doi:10.1016/S0377-0273(00)00201-8
Acocella, V., Cifelli, F., and Funiciello, R. (2001a). The control of overburden thickness on resurgent domes: insights from analogue models. J. Volcanol. Geoth. Res. 111, 137–153. doi:10.1016/S0377-0273(01)00224-4
Acocella, V., Cifelli, F., and Funiciello, R. (2001b). Formation of nonintersecting nested calderas: insights from analogue models. Terra. Nova 13 (1), 58–63. doi:10.1046/j.1365-3121.2001.00317.x
Acocella, V., Funiciello, R., Marotta, E., Orsi, G., and De Vita, S. (2004). The role of extensional structures on experimental calderas and resurgence. J. Volcanol. Geoth. Res. 129 (1-3), 199–217. doi:10.1016/S0377-0273(03)00240-3
Acocella, V. (2007). Understanding caldera structure and development: an overview of analogue models compared to natural calderas. Earth Sci. Rev. 85 (3-4), 125–160. doi:10.1016/j.earscirev.2007.08.004
Bellahsen, N., and Daniel, J. M. (2005). Fault reactivation control on normal fault growth: an experimental study. J. Struct. Geol. 27 (4), 769–780. doi:10.1016/j.jsg.2004.12.003
Bonini, M., Maestrelli, D., Corti, G., Del Ventisette, C., Moratti, G., Carrasco-Núñez, G., et al. (2021). Modelling intra-caldera resurgence settings: Laboratory experiments with application to the Los Humeros Volcanic Complex (Mexico). J. Geophys. Res. Solid Earth, 126, e2020JB020438. doi:10.1029/2020JB020438
Burchardt, S., and Walter, T. R. (2010). Propagation, linkage, and interaction of caldera ring-faults: comparison between analogue experiments and caldera collapse at Miyakejima, Japan, in 2000. Bull. Volcanol. 72 (3), 297–308. doi:10.1007/s00445-009-0321-7
Cailleau, B., Walter, T. R., Janle, P., and Hauber, E. (2003). Modeling volcanic deformation in a regional stress field: Implications for the formation of graben structures on Alba Patera, Mars, J. Geophys. Res., 108, 5141. doi:10.1029/2003JE002135
Coumans, J. P., and Stix, J. (2016). Caldera collapse at near-ridge seamounts: an experimental investigation. Bull. Volcanol. 78 (10), 70. doi:10.1007/s00445-016-1065-9
Del Ventisette, C., Bonini, M., Agostini, A., Corti, G., Maestrelli, D., and Montanari, D. (2019). Using different grain-size granular mixtures (quartz and K-feldspar sand) in analogue extensional models. J. Struct. Geol. 129, 103888. doi:10.1016/j.jsg.2019.103888
Donnadieu, F., Kelfoun, K., de Vries, B. V. W., Cecchi, E., and Merle, O. (2003). Digital photogrammetry as a tool in analogue modelling: applications to volcano instability. J. Volcanol. Geoth. Res. 123 (1-2), 161–180. doi:10.1016/S0377-0273(03)00034-9
Geyer, A., Folch, A., and Martí, J. (2006). Relationship between caldera collapse and magma chamber withdrawal: an experimental approach. J. Volcanol. Geoth. Res. 157 (4), 375–386. doi:10.1016/j.jvolgeores.2006.05.001
Geyer, A., and Martí, J. (2014). A short review of our current understanding of the development of ring faults during collapse caldera formation. Front. Earth Sci. 2, 22. doi:10.3389/feart.2014.00022
Holohan, E. P., Troll, V. R., van Wyk de Vries, B., Walsh, J. J., and Walter, T. R. (2008a). Unzipping long valley: an explanation for vent migration patterns during an elliptical ring fracture eruption. Geology 36 (4), 323–326. doi:10.1130/G24329A.1
Holohan, E. P., de Vries, B. V. W., and Troll, V. R. (2008b). Analogue models of caldera collapse in strike-slip tectonic regimes. Bull. Volcanol. 70 (7), 773–796. doi:10.1007/s00445-007-0166-x
Holohan, E. P., Troll, V. R., Walter, T. R., Münn, S., McDonnell, S., and Shipton, Z. K. (2005). Elliptical calderas in active tectonic settings: an experimental approach. J. Volcanol. Geoth. Res. 144 (1-4), 119–136. doi:10.1016/j.jvolgeores.2004.11.020
Holohan, E. P., Walter, T. R., Schöpfer, M. P., Walsh, J. J., De Vries, B. V. W., and Troll, V. R. (2013). Origins of oblique‐slip faulting during caldera subsidence. J. Geophys. Res.: Solid Earth 118 (4), 1778–1794. doi:10.1002/jgrb.50057
Kennedy, B., Stix, J., Vallance, J. W., Lavallée, Y., and Longpré, M. A. (2004). Controls on caldera structure: results from analogue sandbox modeling. Geol. Soc. Am. Bull. 116 (5-6), 515–524. doi:10.1130/B25228.1
Komuro, H., Fujita, Y., and Kodama, K. (1984). Numerical and experimental models on the formation mechanism of collapse basins during the green tuff orogenesis of Japan. Bull. Volcanol. 47, 649–666. doi:10.1007/BF01961233
Lavallée, Y., Stix, J., Kennedy, B., Richer, M., and Longpré, M. A. (2004). Caldera subsidence in areas of variable topographic relief: results from analogue modeling. J. Volcanol. Geoth. Res. 129 (1-3), 219–236. doi:10.1016/S0377-0273(03)00241-5
Le Turdu, C., Tiercelin, J.-J., Gibert, E., Travi, Y., Lezzar, K.-E., Richert, J.-P., et al. (1999). The Ziway–Shala lake basin system, main ethiopian rift: influence of volcanism, tectonics, and climatic forcing on basin formation and sedimentation. Palaeogeogr. Palaeoclimatol. Palaeoecol. 150, 135–177. doi:10.1016/S0031-0182(98)00220-X
Maestrelli, D., Bonini, M., Corti, G., Del Ventisette, C., Moratti, G., and Montanari, D. (2020a). Caldera collapse vs inherited structures: a database of laboratory experiments [Data set]. Zenodo. doi:10.5281/zenodo.4084639
Maestrelli, D., Montanari, D., Corti, G., Del Ventisette, C., Moratti, G., and Bonini, M. (2020b). Exploring the interactions between rift propagation and inherited crustal fabrics through experimental modelling. Tectonics 39. e2020TC006211. doi:10.1029/2020TC006211
Marti, J., Ablay, G. J., Redshaw, L. T., and Sparks, R. S. J. (1994). Experimental studies of collapse calderas. J. Geol. Soc. 151 (6), 919–929. doi:10.1144/gsjgs.151.6.0919
Montanari, D., Bonini, M., Corti, G., Agostini, A., and Del Ventisette, C. (2017a). Forced folding above shallow magma intrusions: insights on supercritical fluid flow from analogue modelling. J. Volcanol. Geoth. Res. 345, 67–80. doi:10.1016/j.jvolgeores.2017.07.022
Montanari, D., Agostini, A., Bonini, M., Corti, G., and Ventisette, C. D. (2017b). The use of empirical methods for testing granular materials in analogue modelling. Materials 10 (6), 635. doi:10.3390/ma10060635
Montanari, D., Del Ventisette, C., and Bonini, M. (2020). Lateral magma migration through interconnected sills: evidence from analogue modeling. Earth Planet Sci. Lett. 551, 1–2020. doi:10.1016/j.epsl.2020.116568
Murase, T., and McBirney, A. R. (1973). Properties of some common igneous rocks and their melts at high temperatures. Geol. Soc. Am. Bull. 84 (11), 3563–3592. doi:10.1130/0016-7606(1973)84<3563:POSCIR>2.0.CO
Roche, O., Druitt, T. H., and Merle, O. (2000). Experimental study of caldera formation. J. Geophys. Res. Solid Earth 105 (B1), 395–416. doi:10.1029/1999JB900298
Ruch, J., Acocella, V., Geshi, N., Nobile, A., and Corbi, F. (2012). Kinematic analysis of vertical collapse on volcanoes using experimental models time series. J. Geophys. Res., 117, B07301. doi:10.1029/2012JB009229
Schonberger, J. L., and Frahm, J. M. (2016). “Structure-from-motion revisited,” in Proceedings of the IEEE conference on computer vision and pattern recognition, 17-19 June 1997; San Juan, Puerto Rico, USA 4104–4113.
Sigmundsson, F. (2019). Calderas collapse as magma flows into rifts. Science 366 (6470), 1200–1201. doi:10.1126/science.aaz7126
Thielicke, W., and Stamhuis, E. J. (2014). PIVlab–towards user-friendly, affordable and accurate digital particle image velocimetry in MATLAB. J. Open Res. Software, 2: e30. 10.5334/jors.bl
Troll, V. R., Walter, T. R., and Schmincke, H. U. (2002). Cyclic caldera collapse: piston or piecemeal subsidence? field and experimental evidence. Geology 30 (2), 135–138. doi:10.1130/0091-7613(2002)030<0135:CCCPOP>2.0.CO
Walter, T. R., and Troll, V. R. (2001). Formation of caldera periphery faults: an experimental study. Bull. Volcanol. 63 (2-3), 191. doi:10.1007/s004450100135
Weijermars, R., Jackson, M. P. A., and Vendeville, B. (1993). Rheological and tectonic modeling of salt provinces. Tectonophysics 217, 143–174. doi:10.1016/0040-1951(93)90208-2
WoldeGabriel, G., Aronson, J. L., and Walter, R. C. (1990). Geology, geochronology, and rift basin development in the central sector of the main ethiopia rift. Geol. Soc. Am. Bull. 102, 439–458. doi:10.1130/0016-7606(1990)102<0439:ggarbd>2.3.co;2
Keywords: caldera collapse, analogue modeling, inherited structures, crustal discontinuities, fault propagation, fault reactivation, particle image velocimetry, digital elevation model
Citation: Maestrelli D, Bonini M, Corti G, Del Ventisette C, Moratti G and Montanari D (2021) A Database of Laboratory Analogue Models of Caldera Collapse Testing the Role of Inherited Structures. Front. Earth Sci. 9:618258. doi: 10.3389/feart.2021.618258
Received: 16 October 2020; Accepted: 12 January 2021;
Published: 16 February 2021.
Edited by:
Adelina Geyer, Instituto De Ciencias De La Tierra Jaume Almera (ICTJA), SpainReviewed by:
Alvaro Marquez, Rey Juan Carlos University, SpainOlivier Galland, University of Oslo, Norway
Joan Marti, Consejo Superior De Investigaciones Científicas (CSIC), Spain
Copyright © 2021 Maestrelli, Bonini, Corti, Del Ventisette, Moratti and Montanari. This is an open-access article distributed under the terms of the Creative Commons Attribution License (CC BY). The use, distribution or reproduction in other forums is permitted, provided the original author(s) and the copyright owner(s) are credited and that the original publication in this journal is cited, in accordance with accepted academic practice. No use, distribution or reproduction is permitted which does not comply with these terms.
*Correspondence: Daniele Maestrelli, ZGFuaWVsZS5tYWVzdHJlbGxpQGdtYWlsLmNvbQ==; Domenico Montanari, ZG9tZW5pY28ubW9udGFuYXJpQGlnZy5jbnIuaXQ=