- 1State Key Laboratory of Simulation and Regulation of Water Cycle in River Basin, China Institute of Water Resources and Hydropower Research, Beijing, China
- 2Key Laboratory of the Loess Plateau Soil Erosion and Water Loss Process and Control of Ministry of Water Resources, Yellow River Institute of Hydraulic Research, Zhengzhou, China
- 3College of Engineering, San Jose State University, San Jose, CA, United States
- 4School of Conservancy and Hydroelectric Power, Hebei University of Engineering, Handan, China
Water and soil losses from sloping farmlands potentially contribute to water eutrophication and land degradation. However, few studies explored the combined effects of ridge direction and ridge width on surface runoff and soil losses of sloping farmlands. Twenty-seven experimental plots (8 m long and 4 m wide) with nine treatments (three ridge direction: cross ridge, longitudinal ridge, and oblique ridge; and three ridge width: 40, 60, and 80 cm) were adopted under natural rainfall conditions for two years in the Luanhe River Basin of China. Results indicated that ridge direction had significant effects on runoff and sediment yield (p < 0.05). The ridge width had no significant effect on runoff and sediment yield. No significant interaction effect was found between ridge direction and width on runoff and sediment yield of the sloping farmland based on statistical analyses. Compared with cross-ridge (CR) tillage and oblique-ridge (OR) tillage, longitudinal-ridge (LR) tillage significantly decreased runoff by 78.9% and 64.9% and soil losses by 88.2 and 83.5%, respectively (p < 0.05). The effects of ridge directions on runoff and sediment yield were related to rainfall grade. When the rainfall grade reached rainstorm, the runoff yield under CR, LR, and SR had significant differences (p < 0.05). The runoff under LR and OR treatment was 5.16 and 3.3 times, respectively, of that under CR. When the rainfall level was heavy rain or rainstorm, the sediment yield under LR was significantly greater than that under CR. The sediment yield was 13.45 times of that under CR. Cross-ridge tillage with a ridge width of 40 cm is an optimally effective measure of soil and water conservation on sloping farmland in arid and semiarid regions of China.
Introduction
As a valuable land resource, sloping farmland occupies an important position in hilly agricultural areas. Nonetheless, soil erosion on sloping farmland caused by unreasonable farming methods is frequently reported as a threat to water quality and land degradation (Pimentel, 2006; Zuazo and Pleguezuelo, 2008; Zhao et al., 2015; Fang et al., 2017; Yu et al., 2020). Ridge tillage can affect roughness of soil surface: change flow velocity, water infiltration time, the infiltration process, and the runoff process. Thereby, the surface runoff and sediment yield are influenced by ridge tillage. The key parameters of ridge tillage are ridge direction and ridge geometry indices (ridge height and ride width). The influence of ridge direction on surface runoff and sediment yield has been studied a lot. However, the combined influence of ridge direction and ridge width on surface runoff and sediment yield has not been deeply studied.
Ground surface characteristics are a key factor influencing the magnitude of runoff and soil erosion on sloping farmland (Wei et al., 2014; Zhou et al., 2016). Ridge tillage is an agricultural practice which is widely used globally due to low cost and input. Ridge direction and ridge geometry indices (ridge height and ridge width) are important parameters which influence the reduction effect of water and sediment (Wischmeier and Smith, 1978; Renard et al., 1997; USDA-ARS, 2008). The studies of the influence of the ridge direction on runoff and sediment were almost focused on the comparison among cross-ridge tillage, longitudinal-ridge tillage, and conservation tillage. Cross-ridge tillage that plants crops on the contour has many advantages in reducing runoff and sediment yield (Hu et al., 2013). 49 % of runoff and 97% of sediment were lessened by cross-ridge tillage under heavy rainfall compared with the control group based on field experiment in southern China (Dai et al., 2018). Grum et al. (2017) showed that runoff in tied cross-ridge tillage was significantly reduced by 65% compared with normal agricultural practices in northern Ethiopia. Xia et al. (2015) also pointed out that cross-ridge tillage could reduce runoff and sediment yield by 30.1 and 27.6%, respectively, in the Three Gorges area of China. In eastern Scotland, modified sediment fence pinned to a contour fence near the base of a potato field was found to be effective to retain sediment (Vinten et al., 2013). Contour ridge decreased erosion by slowing down runoff flow velocity (Guo et al., 2019). Crop leaves can intercept rainfall, roots can consolidate the cross-ridge, and cross-ridge can retain rainfall by establishing contour lines on sloping cropland (Yang et al., 2013; Liu et al., 2018). In addition, cross-ridge tillage in South Korea, England, and Italy was superior to traditional farming and longitudinal-ridge tillage in reducing runoff and sediment (Chisci and Boschi, 1988; Stevens et al., 2009; Arnhold et al., 2013). At present, few studies investigated the effect of ridge width on runoff and sediment yield in sloping farmland. The indoor control experiments showed that the ridge width had a significant effect on runoff and sediment yield in cross-ridge tillage (Liu et al., 2014a). There is a lack of research on the combined effects of ridge direction and ridge width on runoff and sediment yield in field experiments.
According to the second national land survey, there are approximately 30% of the agricultural croplands with a slope above 6°, which need soil management measures to prevent soil erosion. Previous studies evaluating tillage impact on runoff and sediment yield were mostly focused on south China (Guo et al., 2019). Few studies have paid attention on the semiarid and semi-humid areas of North China. In this study, the Luanhe River Basin was selected as the research area. Three ridge directions and ridge widths were set up for field experiments to analyze the effects of ridge direction and width on runoff and sediment yield. The purposes of this study are as follows: 1) analyzing the influence of ridge direction and ridge width on runoff and sediment yield and 2) analyzing the relationship among precipitation, runoff, and sediment yield under different ridge layouts and rain grades, so as to provide technical support for water and soil conservation of sloping farmland in arid and semiarid areas of China.
Materials and Methods
Study Area
The Luanhe River Basin is a first-degree tributary of the Haihe River Basin. The basin is located within 115°34′E–119°50′E and 39°02′N–42°43′N, which also lies in the northeastern part of North China (Figure 1). This area typically has a temperate semi-humid and semiarid continental monsoon climate, which is rainy and hot in summer and cold and dry in winter (Wang et al., 2015). The mean annual air temperature is 7.8 °C. The annual average precipitation is 538.5 mm. As the main land use form in the Luanhe River Basin, the area of dry land is 26.27% of the total area of the basin. Sloping farmland with a slope between 3 and 15° accounted for 89% of dry land. Spring maize and potato are important crops in slope farmland of the Luanhe River Basin (Hebei Province. 2018). Water and soil erosion of sloping farmland that happens during June–September is an urgent problem, which causes nonpoint source pollution and soil quality decline.
Experimental Design
Based on spatial processing of DEM data and field investigation, the slope of the sloping farmland in the Luanhe River Basin is between 5° and 15°. The types of ridge tillage are mainly longitudinal-ridge tillage (LR) and cross-ridge tillage (CR). The ridge height is between 8 and 15 cm, and the ridge width is usually 60 cm. Based on the above, our study selected a typical sloping farmland with a slope of 10° to carry out in situ observation experiments. Three ridge directions (CR, LR, and oblique-ridge (OR) tillage) and three ridge widths (40, 60, and 80 cm) were analyzed in this study. Three × three treatments were set up with three replications. Twenty-seven field plots separated by aluminum composite plates were set up. We found that the soil depth of sloping farmland in the Luanhe River Basin is about 100 cm (Liu et al., 2021). The aluminum plastic plate was buried vertically 100 cm beneath the ground and 20 cm above the soil surface of each side of the plots to avoid water exchange. The size of each experimental plot was 32 m2 (8 m × 4 m) according to the local field size. To collect the surface runoff and sediments under rainfall events, a tray was constructed at the bottom of each plot. Two tanks were set up in the trend for each plot. A water level gauge was installed in two 80-L tanks used to measure the volume of runoff for each rainfall event of each plot.
Before ridge pillaging, the plots were prepared to ensure that the slope of each plot is consistent. After that, ridges were arranged from bottom of the plots according to the designed ridge layout. Spring maize (Zea mays L) was evenly planted on the ridge at 25 cm intervals. According to the ridge width, the maize planting density was 10 plants/m2 (40 cm ridge width), 6.7 plants/m2 (60 cm ridge width), and 5 plants/m2 (80 cm ridge width). The design details for different experimental plots are presented in Figure 2. All the tests were conducted in the growing season of spring maize. The experiment was conducted from April to October in 2019 and 2020.
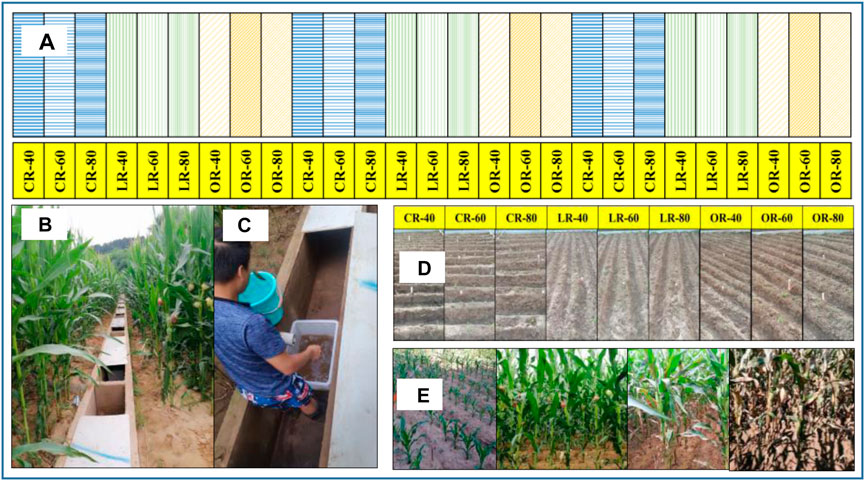
FIGURE 2. The experimental plots and corresponding devices. (A) Experimental plots for measurement of runoff and sediment yield in different ridge tillage systems, (B) runoff and sediment collecting tray, (C) runoff and sediment collect tank, (D) ridge direction and ridge width, and (E) growth process of spring maize.
Rainfall, Runoff, and Sediment Yield Measurement
An SM-1 rain gauge with a diameter of 20 cm was used to observe precipitation, which was installed at the four corners and the center of the experimental site. Precipitation was continuously recorded every hour, and the average value of five gauges was taken as the precipitation of this period until the end of rainfall. The water level of the tank was multiplied by the bottom area to obtain the runoff volume of the rainfall events. The supernatant was removed, and the residual water and sediments were dried in the oven at 105 °C for 48 h to obtain the sediment amount in each experimental plot (Guo et al., 2019).
Runoff depth, runoff sediment concentration, and sediment yield were used to reflect the influence of ridge tillage practice on runoff and sediment yield. The calculation methods of runoff depth, runoff sediment concentration, and sediment yield were as follows. Runoff depth = runoff volume/plot area, with a unit of mm. Runoff sediment concentration = sediment yield/runoff volume with a unit of g/L. Sediment yield = sediment amount/plot area with a unit of kg/hm2.
Data Analysis
Data were analyzed in IBM SPSS Statistics 18.0 and OriginPro 2019. Analysis of variance (one-way ANOVA) was used to test the significance of difference between treatment means for ridge direction and ridge width by SPSS 18.0 software (SPSS Inc., Chicago, United States) (Li and Wang, 2016). When the F value in the ANOVA analysis was statistically significant, a least significant difference test (p = 0.05) was used for the separation of means. The effects of ridge direction and ridge width on the runoff sediment concentration, yield of runoff, sediment for all experimental treatments, and interactions between these variables were tested by a multivariate analysis. Tukey’s test was used to identify the significant differences among the treatments at a significance level of p < 0.05. Linear regression analyses were used to evaluate the relationships among rainfall depth, runoff depth, and sediment yield (Dai et al., 2018).
Results
Rainfall Events
Ninety-six rainfalls were observed during 2019 and 2020. The total rainfall amount was 591 mm. There was no surface runoff in the rainfall of less than 10 mm. There were 16 recorded rainfall events that produced surface runoff. In accordance with the classification standard of precipitation (GBT 28592-2012, 2012), six heavy rainfall events, seven large rainfall events, and three moderate rainfall events were recorded (Table 1). The rainfall depth under heavy rainfalls was 347.8 mm, which was 59% of the total rainfalls. The rainfall depth of large and moderate rainfall events contributed to 41% of the total rainfalls.
Runoff Depth, Sediment Concentration, and Sediment Yield Under Different Treatments
The runoff depth was significantly affected by ridge direction (p < 0.05) (Figure 3). The average runoff depth in the CR treatment was lower than that of LR and OR by 78.7 and 65.5%, respectively. The difference of the runoff depth between CR and LR treatment was significant according to the one-way ANOVA test (p < 0.05). Ridge width had no significant effect on the runoff depth (Figure 3). The average runoff depth in the 60 cm ridge width treatment was lower than that of 40 and 80 cm by 9.1 and 17.5%, respectively. When the ridge width was 40 cm, the runoff depth in the CR treatment was lower than that of LR and OR by 89 and 84%, respectively. When the ridge width was 60 cm, the runoff depth in the CR treatment was lower than that of LR and OR by 86 and 74.8%, respectively. The differences of runoff depth between CR and LR treatment were significant under 40 and 60 cm ridge width, respectively (Figure 4). Significant difference was not found among CR, LR, and OR under the 80 cm ridge width. The runoff depth of CR was lower than that of LR and OR by 61.2 and 36.4%, respectively. There was no between-subjects effect of ridge direction and ridge width on the runoff depth.
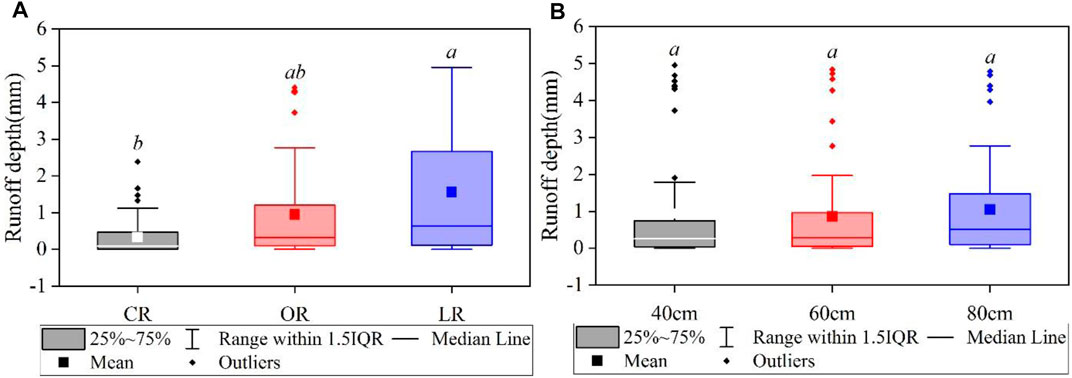
FIGURE 3. Runoff depth under different ridge directions (A) and ridge widths (B), and boxes labeled with different letters indicate significant differences at p < 0.05. CR, OR, and LR represent cross-ridge tillage, oblique-ridge tillage, and longitudinal-ridge tillage, respectively. 40, 60, and 80 cm represent 40 cm ridge width, 60 cm ridge width, and 80 cm ridge width, respectively.
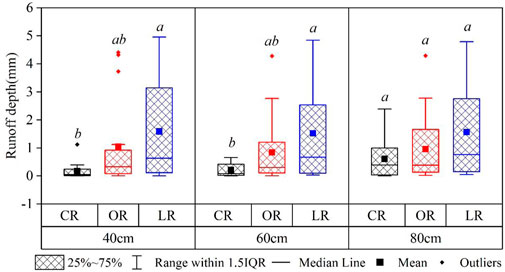
FIGURE 4. Runoff depth under CR, LR, and OR and three ridge widths, and boxes labeled with different letters within groups indicate significant differences at p < 0.05. CR, OR, and LR represent cross-ridge tillage, oblique-ridge tillage, and longitudinal-ridge tillage. 40, 60, and 80 cm represent 40 cm ridge width, 60 cm ridge width, and 80 cm ridge width, respectively.
Ridge direction also had significant effects on sediment concentration in runoff (p < 0.05) (Figure 5). The average volume-weighted sediment concentration was the lowest under CR treatment, with a value of 8.2 g/L. The average volume-weighted sediment concentration under LR treatment was significantly higher than that of CR, with a value of 15.7 g/L. The average volume-weighted sediment concentration under OR treatment was 12.6 g/L. The average volume-weighted sediment concentration of LR under 60 cm ridge width increased significantly by 3.6 times than that of CR under 60 cm ridge width. The average volume-weighted sediment concentration of OR under 60 cm ridge width was higher than that of CR by 2.7 times under 60 cm ridge width. No significant differences were found in average volume-weighted sediment concentration among CR, LR, and OR under 40 cm or 80 cm ridge width. However, the average volume-weighted sediment concentration in runoff of LR and OR was still 1.05–1.75 times of the CR treatment (Figure 6). Ridge width had no significant effects on sediment concentration in runoff (Figure 4). 40 cm ridge width had the lowest sediment concentration, with a value of 10.8 g/L.
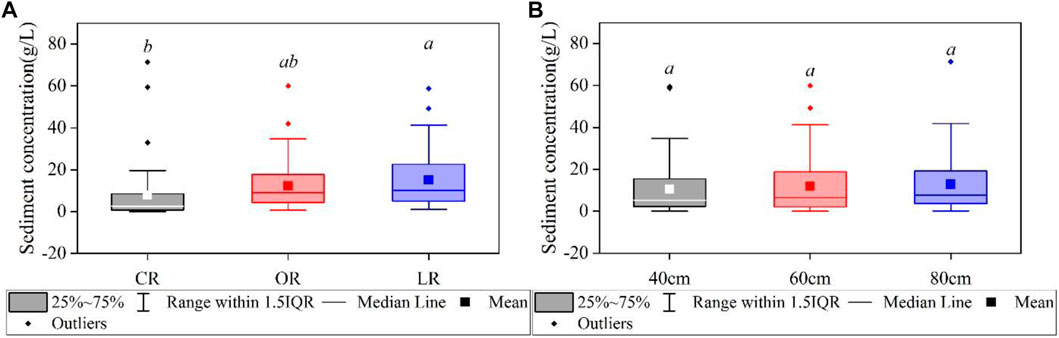
FIGURE 5. Runoff sediment concentration under different ridge directions (A) and ridge widths (B), and boxes labeled with different letters indicate significant differences at p < 0.05. CR, OR, and LR represent cross-ridge tillage, oblique-ridge tillage, and longitudinal-ridge tillage, respectively. 40, 60, and 80 cm represent 40 cm ridge width, 60 cm ridge width, and 80 cm ridge width, respectively.
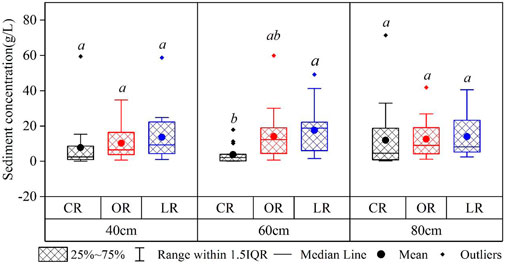
FIGURE 6. Runoff sediment concentration under CR, LR, and OR and three ridge widths, boxes labeled with different letters within groups indicate significant differences at p < 0.05. CR, OR, and LR represent cross-ridge tillage, oblique-ridge tillage, and longitudinal-ridge tillage, respectively. 40, 60, and 80 cm represent 40 cm ridge width, 60 cm ridge width, and 80 cm ridge width, respectively.
Ridge direction had significant effects on sediment yield (p < 0.05) (Figure 7). Compared with CR treatment, sediment yield increased by 11.3 and 5.4 times than that under LR and OR treatment, respectively. The difference of sediment yield was significant between CR and LR treatments (p < 0.05). When the ridge width was 60 cm, sediment yield was greatly affected by ridge direction. The sediment yield under LR and OR treatments was higher than that of CR by 35 and 13.8 times, respectively. No significant difference was found in sediment yield among CR, LR, and OR under 40 and 60 cm ridge width (Figure 8). The sediment yield of LR and OR was 5.7–12 times that of CR treatment. The sediment yield under 40 cm ridge width was the smallest, followed by 80 cm, and that under 60 cm ridge width was the largest. There was no significant difference in sediment yield under different ridge widths (Figure 7).
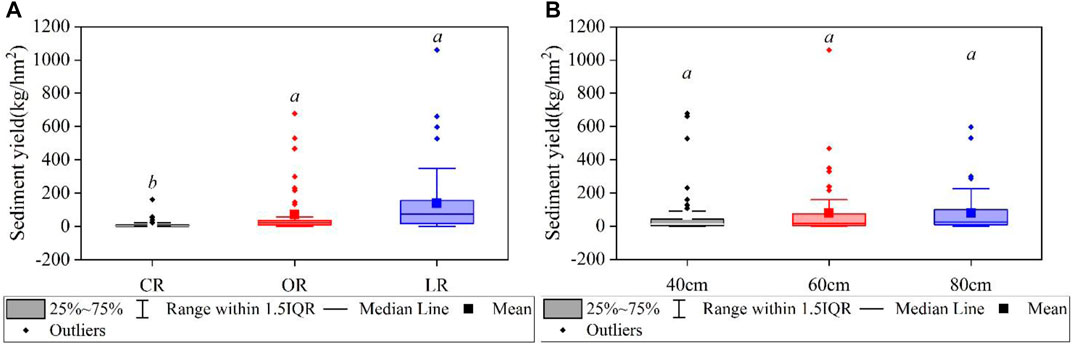
FIGURE 7. Sediment yield under different ridge directions (A) and ridge widths (B), and boxes labeled with different letters indicate significant differences at p < 0.05. CR, OR, and LR represent cross-ridge tillage, oblique-ridge tillage, and longitudinal-ridge tillage, respectively. 40, 60, and 80 cm represent 40 cm ridge width, 60 cm ridge width, and 80 cm ridge width, respectively.
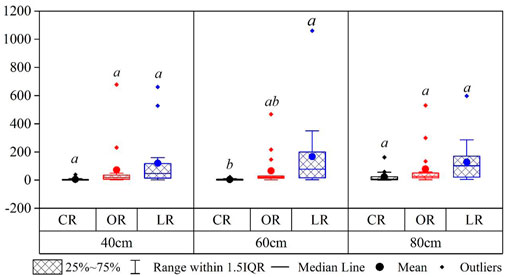
FIGURE 8. Sediment yield under CR, LR, and OR and three ridge widths, and boxes labeled with different letters within groups indicate significant differences at p < 0.05. CR, OR, and LR represent cross-ridge tillage, oblique-ridge tillage, and longitudinal-ridge tillage, respectively. 40, 60, and 80 cm represent 40 cm ridge width, 60 cm ridge width, and 80 cm ridge width, respectively.
Rainfall–Runoff–Sediment Relationship Under Different Ridge Directions
Significant correlation among rainfall, runoff depth, and sediment yield under the three ridge directions was found (Figure 9). The correlation coefficient between rainfall and runoff depth under cross-ridge tillage was the smallest and that between rainfall and runoff depth under longitudinal-ridge tillage was the largest. In the same rainfall event, the runoff depth and sediment yield under cross-ridge tillage was the smallest, that under longitudinal-ridge tillage was the largest, and that under oblique-ridge tillage was in the middle.
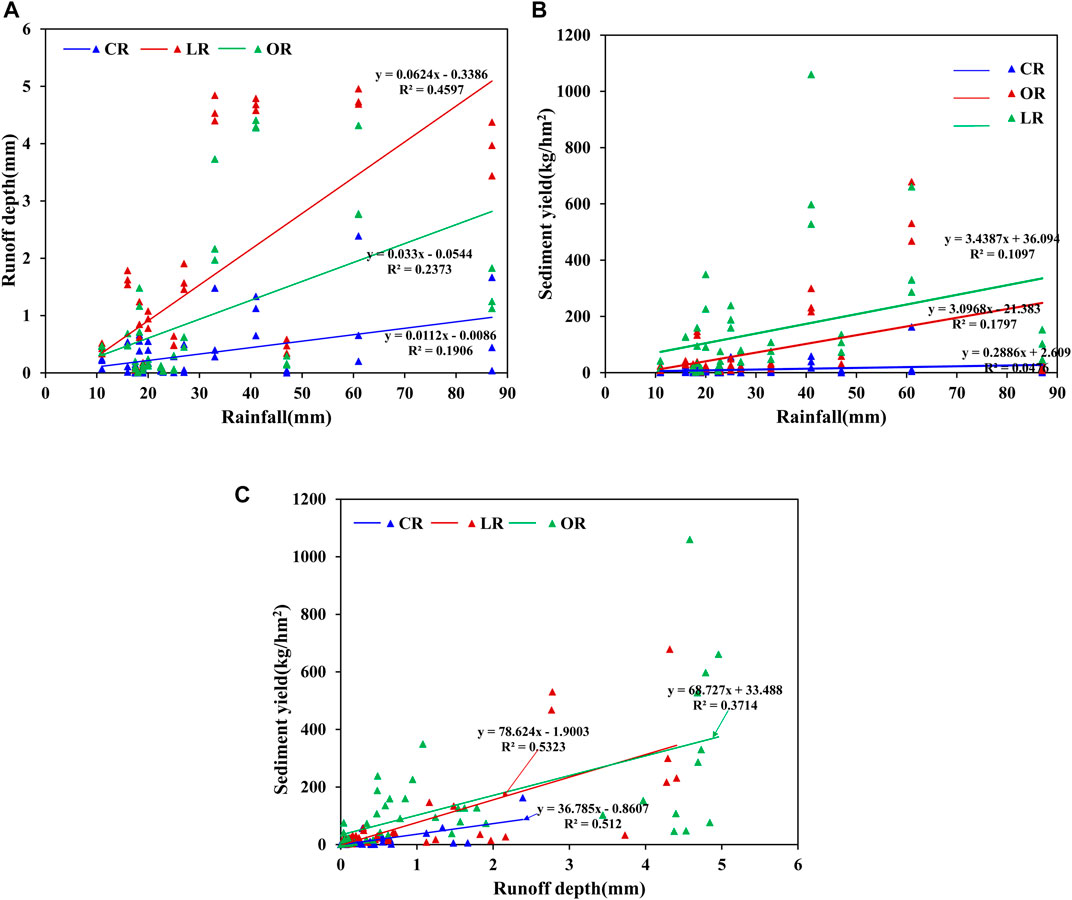
FIGURE 9. Linear regressions of rainfall, the runoff depth, and sediment yield under CR (cross-ridge tillage), OR (oblique-ridge tillage), and LR (longitudinal-ridge tillage). (A) Rainfall and the runoff depth, (B) rainfall and sediment yield, and (C) the runoff depth and sediment yield.
The Influence of Rainfall Pattern on Runoff Depth and Sediment Yield
Under different rainfall patterns, the influences of ridge direction on the runoff depth and sediment were different (Figures 10–12). When the rainfall pattern was moderate and large, the differences of the runoff depth and sediment yield among CR, LR, and OR were not significant. When the rainfall pattern was heavy, the runoff depth had significant differences among CR, LR, and OR. The runoff depth under LR and OR was 5.16 and 3.3 times of that under CR treatment, respectively, (Figure 10). The sediment yield of CR was significantly lower than that of LR when the rainfall pattern was heavy. The sediment yield under LR was 13.45 times of that under CR treatment (Figure 12).
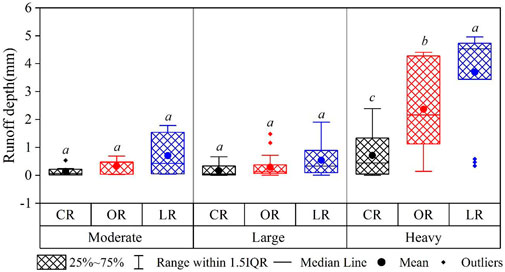
FIGURE 10. Runoff depth of CR, OR, and LR under different rainfall patterns, and boxes labeled with different letters within groups indicate significant differences at p < 0.05. CR, OR, and LR represent cross-ridge tillage, oblique-ridge tillage, and longitudinal-ridge tillage, respectively. Moderate, large, and heavy represent moderate, large, and heavy rainfall patterns, respectively.
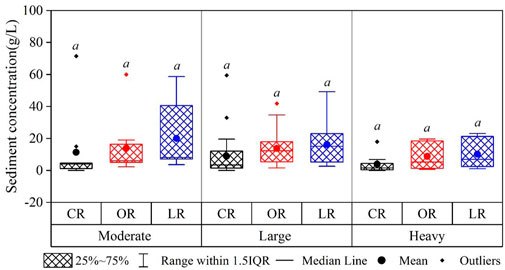
FIGURE 11. Sediment concentration of CR, OR, and LR under different rainfall patterns, and boxes labeled with different letters within groups indicate significant differences at p < 0.05. CR, OR, and LR represent cross-ridge tillage, oblique-ridge tillage, and longitudinal-ridge tillage, respectively. Moderate, large, and heavy represent moderate, large, and heavy rainfall patterns, respectively.
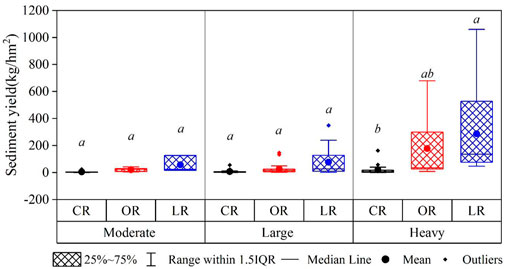
FIGURE 12. Sediment yield of CR, OR, and LR under different rainfall patterns, and boxes labeled with different letters within groups indicate significant differences at p < 0.05. CR, OR, and LR represent cross-ridge tillage, oblique-ridge tillage, and longitudinal-ridge tillage. Moderate, large, and heavy represent moderate, large, and heavy rainfall patterns, respectively.
Heavy rainfalls had the largest contribution to the runoff depth for all plots (Figure 13). In the CR tillage plots, the runoff depth under heavy rainfalls accounted for 58.72–70.72% of the total runoff depth during the experiment. In the OR tillage plots, heavy rainfalls contributed to 73.9–82.29% of the total runoff depth. The proportions of heavy rainfalls in the runoff depth were 73.42–74.83% under LR tillage. The sediment yields at OR and LR tillage plots were highest under heavy rainfalls, which accounted for 74.38–83.64% and 62.15–71.87% of the total sediment yield during the two years, respectively. Large rainfalls contributed to 48.46% of the sediment yield of the total sediment yield under CR tillage of 40 ridge width. The proportions of heavy rainfalls (46.64%) and large rainfalls (43.76%) in sediment yield were similar under CR tillage of 60 ridge width. The contributions of moderate rainfalls in the runoff depth and sediment yield were all below 10% for all plots.
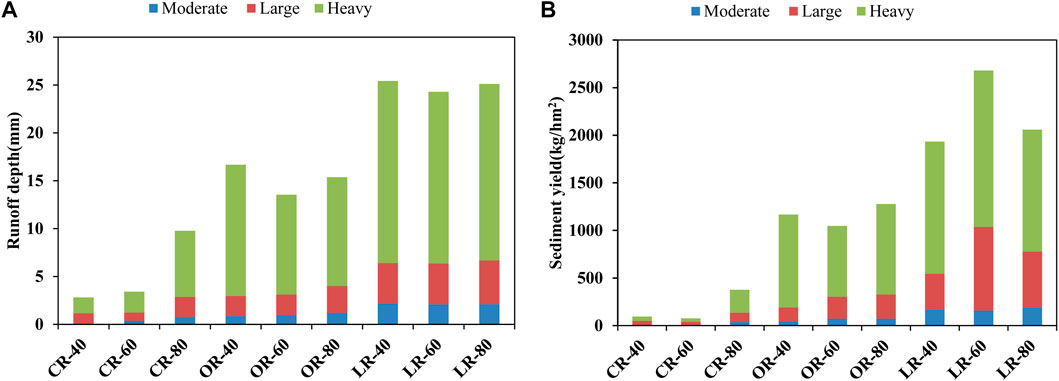
FIGURE 13. Runoff depth (A) and sediment yield (B) of different treatments under different rainfall patterns, and CR-40, CR-60, and CR-80 represent cross-ridge tillage with 40, 60, and 80 cm ridge width, respectively. OR-40, OR-60, and OR-80 represent oblique-ridge tillage with 40, 60, and 80 cm ridge widths, respectively. LR-40, LR-60, and LR-80 represent longitudinal-ridge tillage with 40, 60, and 80 cm ridge widths, respectively. Moderate, large, and heavy represent moderate, large, and heavy rainfall patterns, respectively.
Discussion
The Influence of Ridge Direction and Ridge Width on the Runoff Depth and Sediment Yield
Ridge direction affected the runoff depth significantly in sloping farmland. Compared with longitudinal-ridge tillage commonly used by the locals, cross-ridge tillage exhibited a runoff depth reduction of 78.9%. Compared with oblique-ridge tillage, cross-ridge tillage decreased the runoff depth by 64.9%. Field experiments showed that the runoff depth of cross-ridge tillage was significantly less than that of longitudinal-ridge tillage at four experiment sites in south hilly regions of China (Guo et al., 2019). The performances of cross-ridge tillage in reducing the runoff depth during heavy rainfalls in this study were similar with those of Guo’s result. Guo et al. (2019) pointed out that the ridges along contour lines form barriers to reduce the slope runoff depth from sloping farmland. Practices indicated that cross-ridge tillage could reduce the runoff depth and increase infiltration on sloping land compared with longitudinal-ridge tillage in north of China (Liu et al., 2010). By intercepting the runoff and decreasing the flow velocity, the water infiltration was increased, and then the surface runoff was reduced under cross-ridge tillage (Quinton and Catt, 2004; Yang et al., 2013). Crop roots also could consolidate the ridge to retain more water in the sloping farmland with the growth of crops (Liu et al., 2018).
Ridge direction also had a significant effect on sediment concentration and sediment yield (p < 0.05). The sediment yield of LR and OR was 12.3 and 6.4 times of that under CR tillage, respectively. Xu et al. (2018) reported that tillage systems with different direction ridges had different erosion processes. Under LR tillage, soil particles were gradually stripped from the top to the base of the ridge, and under the effect of runoff, small trench erosion was formed on the ridge (Liu et al., 2014a; Li et al., 2016; Liu et al., 2016; Shen et al., 2016). Under CR tillage, the “depression storage” in the furrows gradually increased (USDA-ARS, 2008; USDA-ARS, 2013; Liu et al., 2015). When the water volume crossed the ridge, runoff formed at the lowest point of the ridges, and destroyed the ridges and led to soil loss. If the ridge of CR tillage collapsed, a large amount of sediment was produced.
In this study, the observed rainfall and sediment yield data did not show the phenomenon of cross-ridge collapse. Therefore, the sediment yield of CR was much smaller than that of LR and OR. As the ridge of OR tillage was downward in the slope direction, the erosion effect of runoff flow on the ridges was similar to that of LR tillage. The sediment yield of OR tillage was in the range of CR—LR tillage. A heavy rainfall of 95 mm was observed on June 24, 2020. The rainfall flooded the road to the experiment site. No data about runoff and sediment were observed. During the process of clearing the sediment, it was found that the sediment of LR and OR is more than that of CR tillage although there was a certain damage for CR tillage plots. The results were consistent with the finding of Guo et al. (2019), Bu et al. (2008).
Few previous in situ experimental studies focused on the effects of ridge width on the runoff depth and sediment yield. As shown in this study, the ridge width has no significant effect on the runoff and sediment yield. Based on laboratory studies, Xu et al. (2018) found that when the rainfall depth was larger than 50 m/h, the microtopography and specification design of ridges are the key factors for cross-ridge tillage to reduce runoff and sediment loss. Under this circumstances, the geometric shape of the ridge plays a key role in rainfall and runoff generation. On June 24, 2020, the experiment site observed rainfall that could not measure the runoff and sediment, causing partial damage to the field ridges. The penetrating erosion ditches in the 40 cm width ridges were found, and no large erosion ditches were found in the 80 cm width ridges under CR tillage. Based on artificially simulated rainfall experiments, Liu et al. (2014b) demonstrated that there was a negative effect of ridge width on runoff and sediment yield under cross-ridge tillage. Different with Liu’s finding, 60 cm ridge width had the least sediment yield under CR tillage in our study. The difference of the results is due to less influence of indoor control experiment and more influence of in situ experiment. Considering that the in situ experiment is closer to the actual production active, the ridge width can be determined from the perspective of nutrient loss and crop yield (Wang et al., 2018; Liu et al., 2020). In this study, the runoff depth under CR tillage increased with the increase of ridge width. With the increase of the ridge width, the space between ridges, which is beneficial for storing water, decreased (Xu et al., 2018). The amount of stored water between ridges and the erosion prevention of the ridge reach a balance under 60 cm width ridge tillage.
Relationship Among Rainfall, Runoff Depth, and Sediment Yield
Many researchers have studied the relationships among rainfall, runoff depth, and sediment yield (Kothyari et al., 2004; Mathys et al., 2005; Wang et al., 2010; Liu et al., 2012; Dai et al., 2018). The relationships among them were mostly described by linear, exponential, and power functions. The results based on a field experiment by Liu et al. (2012) showed that there was a positive power function correlation between rainfall and the runoff depth. Field experiments conducted by Wang et al. (2010) found that the relationship between the runoff depth and sediment yield could be described by the exponential function. Linear correlation was found between the runoff depth and sediment loss by Kothyari’s study (Kothyari et al., 2004). In this study, correlation analysis showed a significant correlated relationship among rainfall, runoff depth, and sediment yield (p < 0.05). Figure 5 showed that rainfall was significantly positively correlated with the runoff depth and sediment yield. The runoff depth was significantly positively linearly correlated with sediment yield. The trend line showed that the runoff depth and sediment yield under the same rainfall increased when the ridge direction changed from CR to OR to LR.
The Influence of Rainfall Grade on the Runoff Depth and Sediment Yield
Rainfall is a main driving force for the runoff depth and sediment yield in rain-fed agriculture (de Lima and Singh, 2002; Wei et al., 2009; Sasal et al., 2010). Rainfall patterns had significant effects on the runoff depth and sediment yield (Zhang et al., 2016; Yang et al., 2018). The runoff depth and sediment yield of CR tillage were consistently less than those of OR and LR tillage under the same rainfall grade. The performance in reducing runoff and sediment yield of cross-ridge tillage was the best during heavy rainfall. As mentioned in 5.1, cross-ridge tillage formed barriers and decreased surface runoff from the plot. Moreover, Liu et al. (2010) also reported that cross-ridge tillage retain runoff compared with LR tillage. The cross-ridge could also effectively increase soil water infiltration and reduce runoff volume by intercepting the runoff and reducing the flow velocity (Quinton and Catt, 2004; Yang et al., 2013).
Comparing OR and LR tillage, the reduction effect of CR tillage on runoff and sediment yield was not significant under moderate and large rainfall. When the rainfall was heavy, the runoff depth and sediment yield under CR tillage were significantly less than those of OR and LR tillage. Most runoff depth and sediment yield for all plots except for CR at 40 cm ridge width occurred under heavy rainfall. The runoff proportion under heavy rainfalls varied from 58.73 to 82.29% for all plots. Except that large rainfall contributed 48.36% to sediment under CR at 40 ridge width, the contribution of heavy rainfall varied from 46.64 to 83.64% for other plots. The results were consistent with the previous study that heavy rainfall had the most effect on soil erosion (Sharma et al., 1993; van Dijk et al., 2002; Gao et al., 2005; Kinnell, 2005; Ran et al., 2012; Mohamadi and Kavian, 2015). The study of Wei et al. (2010) also pointed out that extreme rainfall causes severe soil erosion, for example, the rainfalls that happened on 15th July and 15th September caused 56% of the total soil loss. For the Luanhe River Basin, July and August are the months with the most frequent heavy rainfalls in the Luan River Basin. The government and farmers should pay more attention to this period when soil erosion is easy to occur.
The results of CR at 40 ridge width were different from those of other plots and previous studies, considering that the sediment yield of CR tillage was significantly lower than that of LR tillage under heavy rainfall. The reason may be that most of the heavy rain occurred during the jointing and heading stages of spring maize. At this time, spring maize roots were abundant, and there were a lot of aerial roots (Thidar et al., 2020), which could fix the soil of the ridge and reduce soil loss from the ridge.
At present, many studies are conducted on the runoff depth and sediment yield on sloping farmland in southern China (Dai et al., 2018; Guo et al., 2019). However, in the arid and semiarid areas of northern China, although the average annual rainfall is smaller than that in south China, the rainfall is concentrated from June to September, which can easily cause soil erosion on the sloping farmland. In this study, cross-ridge tillage was demonstrated to be an effective tillage method in reducing surface runoff and soil loss of sloping farmland in the northern regions of China.
Conclusion
Based on two-year in situ experiments, the effect of ridge direction and ridge width on the runoff depth and sediment yield was analyzed in sloping farmland of the Luan River Basin. The results showed the following:
1) Ridge direction had significant impacts on the runoff depth and sediment yield of sloping farmland (p < 0.05). The runoff depth and sediment under cross-ridge tillage (CR) were the least. Ridge width had no significant effect on the runoff depth and sediment yield. The runoff depth and sediment yield of 80 cm ridge width were the largest under CR and OR tillage. The runoff depth of 40 cm ridge width and the sediment yield of 60 cm ridge were the largest under LR tillage. There were no between-subjects effects of ridge direction and ridge width on the runoff depth and sediment yield.
2) When the rainfall pattern was heavy, ridge direction had a significant impact on the runoff depth and sediment yield (p < 0.05). The runoff depth of cross-ridge tillage was significantly less than that of longitudinal-ridge tillage and oblique-ridge tillage. When the rainfall pattern was moderate and large, ridge tillage had no significant effect on the runoff depth and sediment yield.
From the perspective of water conservation and soil conservation, cross-ridge tillage with 40 cm ridge width is the best tillage method for slope farming in the Luan River Basin. In order to study the effects of ridge direction and ridge width on the runoff depth and sediment yield at the watershed scale, a watershed-scale refined hydrological model should be built on the basis of this study.
Data Availability Statement
The original contributions presented in the study are included in the article/supplementary material; further inquiries can be directed to the corresponding authors.
Author Contributions
SL, TQ, and XL conceived the main idea of this manuscript. BD, JW, and CL designed and carried out the experiment. SL wrote the manuscript, and XS and BD put forward suggestions on language modification of the manuscript.
Funding
This research was supported by the National Key Research and Development Project (No. 2017YFA0605004), the National Science Fund for Distinguished Young Scholars (No. 51725905), and the National Key Research and Development Project (No. 2016YFA0601503).
Conflict of Interest
The authors declare that the research was conducted in the absence of any commercial or financial relationships that could be construed as a potential conflict of interest.
Acknowledgments
We thank the farmers who helped us during the experiment, and we thank the editors and reviewers for their suggestions and comments.
References
Bu, C.-F., Cai, Q.-G., Ng, S.-L., Chau, K.-C., and Ding, S.-W. (2008). Effects of Hedgerows on Sediment Erosion in Three Gorges Dam Area, China. Int. J. Sediment Res. 23, 119–129. doi:10.1016/S1001-6279(08)60011-6
Chisci, G., and Boschi, V. (1988). Runoff and Erosion Control with hill Farming in the Sub-coastal Apennines Climate. Soil Tillage Res. 12, 105–120. doi:10.1016/0167-1987(88)90035-9
Dai, C., Liu, Y., Wang, T., Li, Z., and Zhou, Y. (2018). Exploring Optimal Measures to Reduce Soil Erosion and Nutrient Losses in Southern China. Agric. Water Manag. 210, 41–48. doi:10.1016/j.agwat.2018.07.032
de Lima, J. L. M. P., and Singh, V. P. (2002). The Influence of the Pattern of Moving Rainstorms on Overland Flow. Adv. Water Resour. 25, 817–828. doi:10.1016/S0309-1708(02)00067-2
Fang, N. F., Wang, L., and Shi, Z. H. (2017). Runoff and Soil Erosion of Field Plots in a Subtropical Mountainous Region of China. J. Hydrol. 552, 387–395. doi:10.1016/j.jhydrol.2017.06.048
Gao, C., Zhu, J., Zhu, J., Hosen, Y., Zhou, J., Wang, D., et al. (2005). Effects of Extreme Rainfall on the export of Nutrients from Agricultural Land. Acta Geographica Sinica 60, 991–997. doi:10.11821/xb200506012(In Chinese with English abstract)
Grum, B., Woldearegay, K., Hessel, R., Baartman, J. E. M., Abdulkadir, M., Yazew, E., et al. (2017). Assessing the Effect of Water Harvesting Techniques on Event-Based Hydrological Responses and Sediment Yield at a Catchment Scale in Northern Ethiopia Using the Limburg Soil Erosion Model (LISEM). CATENA 159, 20–34. doi:10.1016/j.catena.2017.07.018
Guo, S., Zhai, L., Liu, J., Liu, H., Chen, A., Wang, H., et al. (2019). Cross-ridge Tillage Decreases Nitrogen and Phosphorus Losses from Sloping Farmlands in Southern Hilly Regions of China. Soil Tillage Res. 191, 48–56. doi:10.1016/j.still.2019.03.015
Hu, Z.-F., Gao, M., Xie, D.-T., and Wang, Z.-F. (2013). Phosphorus Loss from Dry Sloping Lands of Three Gorges Reservoir Area, China. Pedosphere 23, 385–394. doi:10.1016/S1002-0160(13)60030-2
Kinnell, P. I. A. (2005). Raindrop-impact-induced Erosion Processes and Prediction: a Review. Hydrol. Process. 19, 2815–2844. doi:10.1002/hyp.5788
Kothyari, B. P., Verma, P. K., Joshi, B. K., and Kothyari, U. C. (2004). Rainfall-runoff-soil and Nutrient Loss Relationships for Plot Size Areas of Bhetagad Watershed in Central Himalaya, India. J. Hydrol. 293, 137–150. doi:10.1016/j.jhydrol.2004.01.011
Li, F. H., and Wang, A. P. (2016). Interaction Effects of Polyacrylamide Application and Slope Gradient on Potassium and Nitrogen Losses under Simulated Rainfall. Catena 136, 162–174. doi:10.1016/j.catena.2015.05.008
Li, G., Zheng, F., Lu, J., Xu, X., Hu, W., and Han, Y. (2016). Inflow Rate Impact on Hillslope Erosion Processes and Flow Hydrodynamics. Soil Sci. Soc. America J. 80 (3), 711–719. doi:10.2136/sssaj2016.02.0025
Liu, H., Yao, L., Lin, C., Wang, X., Xu, W., and Wang, H. (2018). 18-year Grass Hedge Effect on Soil Water Loss and Soil Productivity on Sloping Cropland. Soil Tillage Res. 177, 12–18. doi:10.1016/j.still.2017.11.007
Liu, L., Liu, Q. J., and Yu, X. X. (2016). The Influences of Row Grade, ridge Height and Field Slope on the Seepage Hydraulics of Row Sideslopes in Contour ridge Systems. Catena 147, 686–694. doi:10.1016/j.catena.2016.08.025
Liu, Q. J., An, J., Wang, L. Z., Wu, Y. Z., and Zhang, H. Y. (2015). Influence of ridge Height, Row Grade, and Field Slope on Soil Erosion in Contour Ridging Systems under Seepage Conditions. Soil Tillage Res. 147, 50–59. doi:10.1016/j.still.2014.11.008
Liu, Q. J., Zhang, H. Y., An, J., and Wu, Y. Z. (2014a). Soil Erosion Processes on Row Sideslopes within Contour Ridging Systems. Catena 115, 11–18. doi:10.1016/j.catena.2013.11.013
Liu, Q. J., Shi, Z. H., Yu, X. X., and Zhang, H. Y. (2014b). Influence of Microtopography, ridge Geometry and Rainfall Intensity on Soil Erosion Induced by Contouring Failure. Soil Tillage Res. 136, 1–8. doi:10.1016/j.still.2013.09.006
Liu, S., Qin, T., Dong, B., Shi, X., Lv, Z., and Zhang, G. (2021). The Influence of Climate, Soil Properties and Vegetation on Soil Nitrogen in Sloping Farmland. Sustainability 13, 1480. doi:10.3390/su13031480
Liu, X. B., Zhang, X. Y., Wang, Y. X., Sui, Y. Y., Zhang, S. L., Herbert, S. J., et al. (2010). Soil Degradation: a Problem Threatening the Sustainable Development of Agriculture in Northeast China. Plant Soil Environ. 56 (2), 87–97. doi:10.17221/155/2009-pse
Liu, X., Wang, Y., Yan, X., Hou, H., Liu, P., Cai, T., et al. (2020). Appropriate ridge-furrow Ratio Can Enhance Crop Production and Resource Use Efficiency by Improving Soil Moisture and thermal Condition in a Semi-arid Region. Agric. Water Manag. 240, 106289. doi:10.1016/j.agwat.2020.106289
Liu, Y., Tao, Y., Wan, K. Y., Zhang, G. S., Liu, D. B., Xiong, G. Y., et al. (2012). Runoff and Nutrient Losses in Citrus Orchards on Sloping Land Subjected to Different Surface Mulching Practices in the Danjiangkou Reservoir Area of China. Agric. Water Manag. 110, 34–40. doi:10.1016/j.agwat.2012.03.011
Mathys, N., Klotz, S., Esteves, M., Descroix, L., and Lapetite, J. M. (2005). Runoff and Erosion in the Black Marls of the French Alps: Observations and Measurements at the Plot Scale. Catena 63 (2-3), 261–281. doi:10.1016/j.catena.2005.06.010
Mohamadi, M. A., and Kavian, A. (2015). Effects of Rainfall Patterns on Runoff and Soil Erosion in Field Plots. Int. Soil Water Conservation Res. 3, 273–281. doi:10.1016/j.iswcr.2015.10.001
Pimentel, D. (2006). Soil Erosion: a Food and Environmental Threat. Environ. Dev. Sustain. 8, 119–137. doi:10.1007/s10668-005-1262-8
Quinton, J. N., Quinton, J. N., and Catt, J. A. (2004). The Effects of Minimal Tillage and Contour Cultivation on Surface Runoff, Soil Loss and Crop Yield in the Long-Term Woburn Erosion Reference Experiment on sandy Soil at Woburn, England. soil use manage 20, 343–349. doi:10.1111/j.1475-2743.2004.tb00379.x10.1079/sum2004267
Ran, Q., Su, D., Li, P., and He, Z. (2012). Experimental Study of the Impact of Rainfall Characteristics on Runoff Generation and Soil Erosion. J. Hydrol. 424-425, 99–111. doi:10.1016/j.jhydrol.2011.12.035
Renard, K. G., Foster, G. R., Weesies, G. A., McCool, D. K., and Yoder, D. C. (1997). Predicting Soil Erosion by Water. A Guide to Conservation Planning with the Revised Universal Soil Loss Equation (RUSLE). in Agriculture Handbook 703. Washington, D.C: USDA. doi:10.1007/springerreference_77104
Sasal, M. C., Castiglioni, M. G., and Wilson, M. G. (2010). Effect of Crop Sequences on Soil Properties and Runoff on Natural-Rainfall Erosion Plots under No Tillage. Soil Tillage Res. 108, 24–29. doi:10.1016/j.still.2010.03.010
Sebastian Arnhold, S., Marianne Ruidisch, M., Svenja Bartsch, S., Christopher L. Shope, C. L., and Bernd Huwe, B. (2013). Simulation of Runoff Patterns and Soil Erosion on Mountainous farmland with and without Plastic-Covered ridge-furrow Cultivation in South Korea. Trans. ASABE 56 (2), 667–679. doi:10.13031/2013.42671
Sharma, P. P., Gupta, S. C., and Foster, G. R. (1993). Predicting Soil Detachment by Raindrops. Soil Sci. Soc. America J. 57, 674–680. doi:10.2136/sssaj1993.03615995005700030007x
Shen, H., Zheng, F., Wen, L., Han, Y., and Hu, W. (2016). Impacts of Rainfall Intensity and Slope Gradient on Rill Erosion Processes at Loessial Hillslope. Soil Tillage Res. 155, 429–436. doi:10.1016/j.still.2015.09.011
Stevens, C. J., Quinton, J. N., Bailey, A. P., Deasy, C., Silgram, M., and Jackson, D. R. (2009). The Effects of Minimal Tillage, Contour Cultivation and In-Field Vegetative Barriers on Soil Erosion and Phosphorus Loss. Soil Tillage Res. 106, 145–151. doi:10.1016/j.still.2009.04.009
Thidar, M., Gong, D., Mei, X., Gao, L., Li, H., Hao, W., et al. (2020). Mulching Improved Soil Water, Root Distribution and Yield of maize in the Loess Plateau of Northwest China. Agric. Water Manag. 241, 106340. doi:10.1016/j.agwat.2020.106340
Usda-ARS, (2008). Draft Science Documentation, Revised Universal Soil Loss Equation Version 2 Avaliable at: http.//www.ars.usda.gov/sp2UserFiles/Place/64080510/RUSLE/RUSLE2_Science_Doc.pdf.(accessed 09 01, 15).
Usda-ARS, (2013). Science documentation, Revised Universal Soil Loss Equation Version 2 Avaliable at: https://www.ars.usda.gov/ARSUserFiles/60600505/RUSLE/RUSLE2_Science_Doc.pdf.
van Dijk, A. I. J. M., Bruijnzeel, L. A., and Rosewell, C. J. (2002). Rainfall Intensity-Kinetic Energy Relationships: a Critical Literature Appraisal. J. Hydrol. 261, 1–23. doi:10.1016/S0022-1694(02)00020-3
Vinten, A. J., Loades, K., Addy, S., Richards, S., Stutter, M., Cook, Y., et al. (2014). Reprint of: Assessment of the Use of Sediment Fences for Control of Erosion and Sediment Phosphorus Loss after Potato Harvesting on Sloping Land. Sci. Total Environ. 468-469, 1234–1244. doi:10.1016/j.scitotenv.2013.10.050
Wang, K.-y., Li, Q.-f., Yang, Y., Zeng, M., Li, P.-c., and Zhang, J.-x. (2015). Analysis of Spatio-Temporal Evolution of Droughts in Luanhe River Basin Using Different Drought Indices. Water Sci. Eng. 8 (4), 282–290. doi:10.1016/j.wse.2015.11.004
Wang, L., Tang, L., Wang, X., and Chen, F. (2010). Effects of Alley Crop Planting on Soil and Nutrient Losses in the Citrus Orchards of the Three Gorges Region. Soil Tillage Res. 110 (2), 243–250. doi:10.1016/j.still.2010.08.012
Wang, Q., Li, F., Zhang, D., Liu, Q., Li, G., Liu, X., et al. (2018). Sediment Control and Fodder Yield Increase in Alfalfa (Medicago Sativa L) Production with tied-ridge-furrow Rainwater Harvesting on Sloping Land. Field Crops Res. 225, 55–63. doi:10.1016/j.fcr.2018.05.017
Wei, W., Chen, L., Fu, B., Lü, Y., and Gong, J. (2009). Responses of Water Erosion to Rainfall Extremes and Vegetation Types in a Loess Semiarid Hilly Area, NW China. Hydrol. Process. 23, 1780–1791. doi:10.1002/hyp.7294
Wei, W., Chen, L., Fu, B., Lü, Y., and Gong, J. (2010). Responses of Water Erosion to Rainfall Extremes and Vegetation Types in a Loess Semiarid Hilly Area, NW China. Hydrol. Process. 23, 1780–1791. doi:10.1002/hyp.7294
Wei, W., Chen, L., Zhang, H., Yang, L., Yu, Y., and Chen, J. (2014). Effects of Crop Rotation and Rainfall on Water Erosion on a Gentle Slope in the Hilly Loess Area, China. Catena 123, 205–214. doi:10.1016/j.catena.2014.08.002
Wischmeier, W. H., and Smith, D. D. (1978). Predicting Rainfall Erosion Losses A Guide to Conservation Planning. Agriculture Handbook, 537. Washington, D.C: USDA. doi:10.1016/s0341-8162(00)00089-8
Xia, L.-Z., Liu, G.-H., Wu, Y.-H., Ma, L., and Li, Y.-D. (2015). Protection Methods to Reduce Nitrogen and Phosphorus Losses from Sloping Citrus Land in the Three Gorges Area of China. Pedosphere 25, 478–488. doi:10.1016/S1002-0160(15)30015-1
Xu, X., Zheng, F., Wilson, G. V., He, C., Lu, J., and Bian, F. (2018). Comparison of Runoff and Soil Loss in Different Tillage Systems in the Mollisol Region of Northeast China. Soil Tillage Res. 177, 1–11. doi:10.1016/j.still.2017.10.005
Yang, J., Zheng, H., Chen, X., and Shen, L. (2013). Effects of Tillage Practices on Nutrient Loss and Soybean Growth in Red-Soil Slope farmland. Int. Soil Water Conserv. Res. 1, 49–55. doi:10.1016/S2095-6339(15)30030-7
Yang, S., Han, R., Xing, L., Liu, H., Wu, H., and Yang, Z. (2018). Effect of Slope farmland Soil and Water and Soil Nitrogen and Phosphorus Loss Based on Different Crop and Straw Applications and ridge Patterns in the basin of the Main Stream of the Songhua River. Acta Ecologica Sinica 38, 42–47. doi:10.1016/j.chnaes.2018.01.007
Yu, Y., Zhao, W., Martinez-Murillo, J. F., and Pereira, P. (2020). Loess Plateau: from Degradation to Restoration. Sci. Total Environ. 738, 140206. doi:10.1016/j.scitotenv.2020.140206
Zhang, Q., Liu, D., Cheng, S., and Huang, X. (2016). Combined Effects of Runoff and Soil Erodibility on Available Nitrogen Losses from Sloping farmland Affected by Agricultural Practices. Agric. Water Manag. 176, 1–8. doi:10.1016/j.agwat.2016.05.018
Zhao, P., Tang, X., Tang, J., and Zhu, B. (2015). The Nitrogen Loss flushing Mechanism in Sloping Farmlands of Shallow Entisol in Southwestern China: a Study of the Water Source Effect. Arab. J. Geosci. 8 (12), 10325–10337. doi:10.1007/s12517-015-1983-4
Zhou, P., Zhuang, W. H., Wen, A. B., and Shi, Z. L. (2016). Fractal Features of Soil Particle Redistribution along Sloping Landscapes with Hedge Berms in the Three Gorges Reservoir Region of China. Soil Use Manage 32, 594–602. doi:10.1111/sum.12307
Keywords: runoff, sediment, sloping farmland, ridge direction, ridge width
Citation: Liu S, Qin T, Lv X, Shi X, Dong B, Wang J and Liu C (2021) Experimental Study of Runoff and Sediment Yield Affected by Ridge Direction and Width of Sloping Farmland. Front. Earth Sci. 9:694773. doi: 10.3389/feart.2021.694773
Received: 13 April 2021; Accepted: 16 June 2021;
Published: 14 July 2021.
Edited by:
Ataollah Kavian, Sari Agricultural Sciences and Natural Resources University, IranReviewed by:
Jun Niu, China Agricultural University, ChinaMahboobeh Kiani-harchegani, Yazd University, Iran
Iman Saleh, Agricultural Research, Education and Extension Organization (AREEO), Iran
Copyright © 2021 Liu, Qin, Lv, Shi, Dong, Wang and Liu. This is an open-access article distributed under the terms of the Creative Commons Attribution License (CC BY). The use, distribution or reproduction in other forums is permitted, provided the original author(s) and the copyright owner(s) are credited and that the original publication in this journal is cited, in accordance with accepted academic practice. No use, distribution or reproduction is permitted which does not comply with these terms.
*Correspondence: Tianling Qin, dGlhbmxpbmc0MDZAMTI2LmNvbQ==; Xizhi Lv, bmlodWx2eGl6aGlAMTYzLmNvbQ==