- 1King Abdulaziz City for Science and Technology, Riyadh, Saudia Arabia
- 2School of Earth Atmosphere and Environment, Monash University, Clayton, VIC, Australia
Mantle convection and the interaction of buoyant plumes with the lithosphere have been a significant influence on plate tectonics. Plume-lithosphere interactions have been regarded as a major driver of continental rifting, and have been linked to triple junction development and major supercontinent break-up events. There are also many extensional tectonic settings that lack evidence for a mantle plume and associated magmatism, indicating far-field plate stresses also drive plate fragmentation. The Arabian Plate is a spectacular active example where both a mantle plume and far-field plate stresses interact to drive continental break-up. Despite more than 80 years of geological research, there remains significant conjecture concerning the geodynamic processes responsible for the plate motion and the nature or onset of extension/deformation of the Arabian Plate. Complex structural patterns within the Arabian Plate have been interpreted in the context of tectonic plate movements and reorganization related to the subduction of the Tethys Oceanic plate, collision between Arabian and Eurasian plates, and the superposition of Afar plume. These interactions have accordingly resulted in different explanations or understanding of the geodynamic of the Afro-Arabian rift system. We assess the relative influence of plume vs. far field influences by reviewing the current views on the concept and models of these forces and highlighting their significance and implications on Arabia. Our synthesis shows that most of the geodynamical models proposed so far are not applicable to the entire Arabian Plate and its surrounding boundaries.
Introduction
Mantle plume represent perturbations influencing the lithosphere, which could cause a deformation and variations in surface topography related to thermal buoyancy of the lithosphere and reflects low Pn wave velocities and high seismicity (e.g. Burov and Cloetingh, 2009). This contrasts with the negative buoyancy associated with slab-pull tectonic forces, caused by subduction of relatively cold oceanic slabs at convergent plate margins (e.g., Schellart, 2004). The slab-pull force is significant and represents up to 95% of the net driving forces of tectonic plates (e.g., Lithgow-Bertelloni and Richards, (1995)), although plume force along with mantle convection also contribute to plate kinematics (e.g., Yuen et al., 2007).
Afro-Arabian Rift System is well known as the Earth’s largest active rift system which contains the only known incipient ridge-ridge-ridge type triple junction on Earth (e.g., Gerya and Burov 2018). Various studies have connected the tectonic operations within and around the Arabian Plate in the last 30 Ma with the Tethys Ocean closure and/or the Afar plume (e.g. Bellahsen et al., 2003; Bosworth et al., 2005). Several authors have considered the importance of both the Afar plume and the closure of the Tethys Ocean in the break-up of Africa (e.g. Jolivet and Faccenna, 2000). The interplay of slab-pull and plume processes is not fully resolved. Many reconstruction models of the Arabian Plate and the surrounding region (e.g, Sultan et al. (1992); Reilinger and McClusky, (2011)) provide a wide range of possible evolutionary theories. This paper reviews the historical and current views on the geodynamic setting of Arabia in light with the latest findings from the region and discusses possible implications of plume and far-field stress conditions in order to improve our understanding of the Arabian Plate geodynamic.
The Arabian Plate
The Arabian Plate (Figure 1) consists of Precambrian basement exposure (Arabian Shield) along with Cenozoic alkalic volcanic fields, and Phanerozoic sedimentary sequence (Arabian Platform) (e.g., Stern and Johnson, 2010). There are well known regional observable interior deformations within the plate. Some of these deformation domains are located at the western margin of the plate in the form of dikes, grabens, and volcanic eruptions (e.g., Sharland et al. (2001)), while other deformation domains are located within the Arabian platform as defined by the central and northern Arabian structural systems (Weijermars, 1998; Barrier, et al., 2014). The plate boundaries comprise: 1) the Bitlis, Zagros and Makran sutures bounding the plate from the north to the east, as a result of the closure of the Tethys Ocean; 2) the Gulf of Aden (GOA) at the south which represent one of the arms of the Afar rift system; 3) the Red Sea (RS) to the west that forms the second arm of the Afar rift system; and 4) the Dead Sea Transform (DST) to the northwest that connects both the RS and the Bitlis.
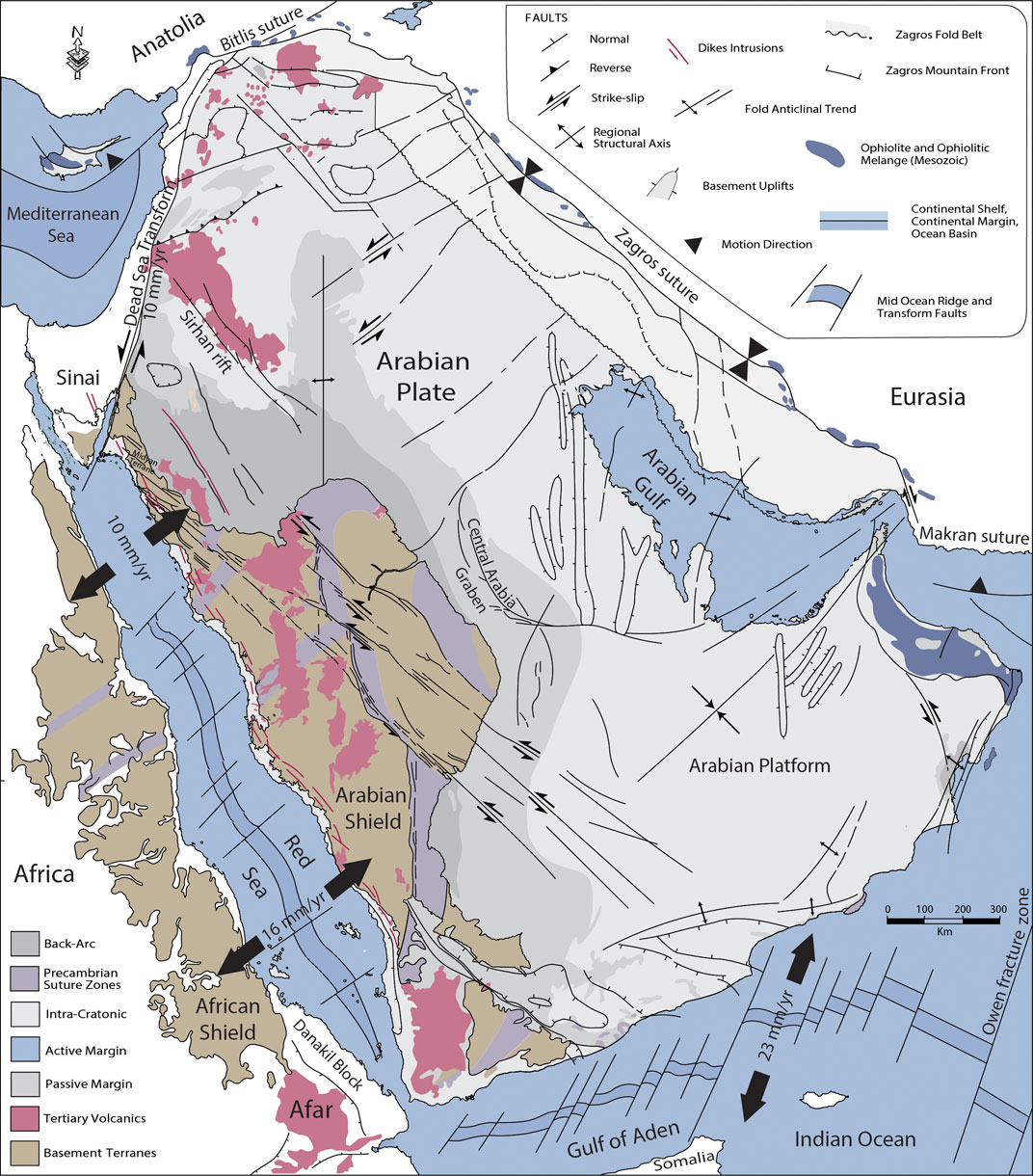
FIGURE 1. Geological and tectonic elements map of the Arabian Plate and surrounding region modified after Sharland et al. (2001). Geodetic observations estimate extensional rates along the RS between 7 to 15 mm/yr from north to south, and between 15 to 19 mm/yr from west to east at the GOA, left lateral slip rates along the southern Dead Sea Transform in the range of 4.5–4.7 mm/yr, and right lateral slip rates along the Owen fracture zone ranging from 3.2 to 2.5 mm/yr (e.g., ArRajehi et al., 2010). The remnant of Neo-Tethys (Makran) is subducting at 19.5 mm/yr beneath Eurasia (Vernant et al., 2004). Counterclockwise rotation of Arabia relative to Eurasia was reported Reilinger et al. (2006) in the range of 20–30 mm/yr, leading to left lateral strike slip (East Anatolian Fault) along the northern boundary of the Arabian Plate with GPS slip rates in the range of 17 –24 mm/yr from east to west Turkey. The slip rates increase eastward along the Zagros mountain belts from 18 mm/yr to 25 mm/yr (Walpersdorf et al., 2006).
Geodynamic Aspects
It is widely accepted that an initial collision between Eurasia and Africa plates occurred at ca 40 Ma (e.g., Hempton, 1987). Apatite fission track data suggest that collision took place at ca 20 Ma along the Bitlis-Zagros zone Okay et al. (2010), though collision at ca 27 Pirouz et al. (2017) or before 26 Ma Koshnaw et al. (2019) has also been proposed. Closure of the Tethys Ocean was interpreted to have been completed in the Miocene (e.g., Beydoun, (1999)), though no later than ca 10 Ma (e.g., McQuarrie et al., 2003). While the closure of Tethys Ocean was taking place at the eastern boundary of the plate, the proto-Arabian Plate had a counterclockwise rotation which has been attributed to either the diachronous Arabia-Eurasia collision (e.g., McQuarrie et al. (2003)), the activation of DST Ben-Avraham et al. (2008), or to a regional mantle flow (Faccenna et al., 2013). At the northern boundary of the Arabian Plate, the boundary forces seems to have changed from compression to strike slip style, due to the oblique collision between Anatolian and Arabian plates (e.g., Beydoun (1999)), which led to lateral escape tectonics of the Anatolian Plate Figure 1 (Reilinger et al., 2006). This may have occurred simultaneously with the crustal extension in the GOA and/or the RS (e.g., Bosworth et al., 2005). Following the collision of Arabia and Eurasia and the RS initiation, the strength contrast between Afro-Arabia continent and the oceanic lithosphere of the Mediterranean Steckler and Uri, (1986) may have initiated movement along the DST at ca 20 Ma Nuriel et al. (2017), soon after a dike intrusion event along the western margin of the Arabian Plate (e.g., Sebai et al., 1991). This ultimately resulted in the development of the Sinai micro-plate (e.g. Beydoun, 1999).
Beneath the Africa-Arabia lithosphere (Figure 2), it was suggested that the arrival of the Afar plume at ca 30 Ma (e.g., Bosworth et al. (2005)) or the onset of extension at the RS occurred during the same period with the onset of collision or convergent between Arabia and Eurasia (e.g., Bellahsen et al. (2003)), with possible interaction between plume and ridge that could extend the influence of the plume up to the eastern GOA (e.g., Basuyau et al., 2010). Plate reconstructions along with geodetic data suggest that African motion relative to Eurasia has been steady since ca 13 Ma but 70% slower than the rate that occurred between ca 30 and 13 Ma (e.g., McClusky et al., 2010). Thermal weakening of the Africa-Arabia lithosphere may have been enhanced because of the slowing of the northward motion of the Arabian Plate after collision (e.g., Almalki et al., 2015). It has been suggested that plume interactions concentrate crustal stretching and dike injection between proto-Arabia and Africa during the late Oligocene (e.g., Cochran, (1983)) while the Indian ridge at the southern boundary of the Arabian Plate was propagating westward above the Afar plume (e.g., Jolivet and Faccenna, 2000).
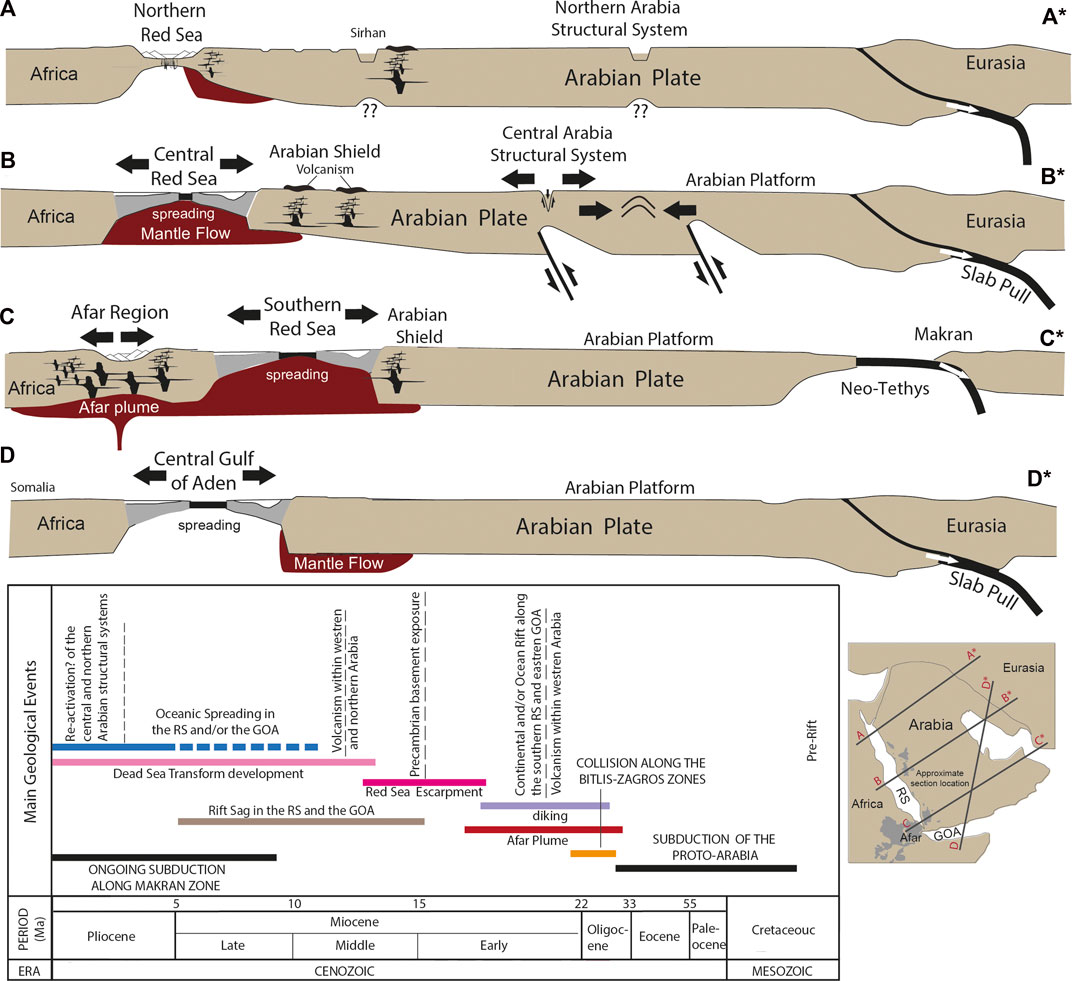
FIGURE 2. Simplified cross-sections along the Arabian Plate and its boundaries illustrating possible sub-crustal relationships, structural architectures and the extent of the regional forces that have been developed since ca 30 Ma. Inset shows the approximate section’s location. Cross section (A) highlights the wide rifting in the northern Red Sea, northern Arabia structural system Litak, et al. (1998), and the slab-pull in northwestern Zagros (Mouthereau, et al., 2012). Cross section (B) shows the central Red Sea along with western Arabia volcanic province Bosworth and Stockli, (2016), central Arabia structural system Weijermars, (1998), and slab-pull forces along central Zagros (Mouthereau, et al., 2012). Cross section (C) presents Afar region, southern Red Sea, and slab-pull forces in Makran (Penney, et al., 2017). Cross section (D) links the central Gulf of Aden with the central segment of Zagros (Mouthereau, et al., 2012). Refer to Figure 1 for structural features locations. The time-line table shows major geological events occurred from 30 Ma to present. The time span extent of these events are not unique, but it is based on our evaluation of the geologic reasonableness of the historical and current observations in an attempt to provide better understanding. The addition of more data in the future will allow for better constraint to arrive at the most plausible geological scenario.
The Arabian Plate became an independent plate with the formation of the RS and the GOA, after an extended period of time of regional extensional deformation (e.g., Szymanski et al., 2016). The RS is formed in response to separation of the African and Arabian plates and represents an example of an orthogonal to rotational extension system (e.g., Molnar et al., 2018). The adjacent GOA also records the separation of the African and Arabian plates but has evolved as an oblique ocean system (e.g., Leroy et al., 2013). An incipient plate boundary has also been introduced to the region as a micro-plate at Danakil area; north of Afar (e.g., Eagles et al., 2002).
Discussion
Despite extensive body of research, there remains conjecture about the timing of collision between the Arabian and Eurasian plates (e.g., Pirouz et al. (2017)), the onset of extension between the Arabian and African plates (e.g., Ligi et al. (2015)), and the extent to which the plume and slab-pull forces played in initiating and/or driving extension (e.g., Koptev et al., 2018). Lack of data has also provided gaps and inconsistencies in plate motion and interior deformation estimates (e.g., Tesauro et al., 2018). The interior deformation architecture of the plate may represent the interaction between far-field forces with the pre-existing zones of weakness, which casts additional uncertainty on linking the observed deformation to a defined set of regional forces.
There is still uncertainty about whether the RS and the GOA formed as a whole-sale tearing of the continental crust or as an episodic or diachronic extension (e.g., Almalki et al., 2016; Nonn et al., 2019). Various models have been proposed for the opening of the RS and the GOA including asymmetric, symmetrical extension and pull-apart models (e.g., Sultan et al., 1992; d’Acremont et al., 2005). The mechanism of opening may involve intrusive magmatic activity as a major role in thinning the continental lithosphere prior to the inception of spreading [e.g., Bohannon and Eittreim, (1991)), a mechanical stretching of the lithosphere (e.g., Martinez and Cochran, (1988)], or simply a localization of the ocean crust formation (e.g., Makris and Rihm, 1991). Consequently, geodynamic models for the divergent boundaries between the Arabian and African plates have fallen into two main categories. Several authors have suggested active rift models with the arrival of the Afar plume (e.g., Leroy et al. (2013); De Gouveia et al. (2018)), whereas other authors have linked extension with passive rifting processes driven by the onset of collision or convergent between Arabia and Eurasia imbricated on a lithospheric weakness zone (e.g., Brune and Autin 2013; Almalki and Betts, 2021). These are end model interpretations and hybrid processes have also been considered (e.g., Ebinger and Belachew, 2010).
Early interpretations of the Afro-Arabian rift System suggested the major driver of lithospheric extension was the arrival of the Afar plume based on geological, geophysical and geochemical observations (e.g., White and McKenzie, 1989; Zeyen et al., 1997; Rogers, 2006). These observations include 1) flood basalts that illustrate a major plume reservoir initiated in the Oligocene and declined during the Miocene (e.g., Rooney et al., 2013); 2) crustal velocity models that suggest thinning of the Arabian Plate from ∼40 km thick crust to about 10 km towards the RS (e.g, Mechie et al., 2013); 3) regional topographic patterns, which have been interpreted to be caused by thermal buoyancy associated with arrival of the Afar plume (e.g., Gvirtzman et al., 2016); 4) seismic tomography data that reveal shear wave splitting azimuths Faccenna et al. (2013) where basal tractions suggest northward mantle flow emanating from the Afar region and slightly oblique to the RS rift axis (e.g., Chang et al., 2011); 5) isotopic data which suggest that the mantle source can be separated into Pan-African continental lithosphere, mantle plume and depleted asthenosphere (Schilling et al., 1992); and 6) results from the volcanic suites sited on the stable shield which compared with other results from the Arabian Plate to conclude that it did not rotate significantly following closure of the Bitlis Suture until Late Miocene–Early Pliocene times since when it has rotated anticlockwise at a rate of ∼1.0 /Myr (Gürsoy et al., 2009).
However, comprehensive investigation of regional and local-scale geologic, geochronological and geophysical data over the whole collisional plate boundary suggest slab-pull force dominated at least until 12 Ma (Mouthereau et al., 2012). Observations that do not support an active rift model or limit the influence of the plume include the absence of high surface heat flow of the uplifted shield due to the temperature increase in the mantle upwelling McGuire and Bohannon, (1989) along with the lack of sign of an increase in geothermal gradient prior to rift initiation (Szymanski et al., 2016). Stretching and lithospheric thinning of the Arabian margin along the RS is asymmetrical (Figure 2) Yao et al. (2017), which are inconsistent with plume models. Further, topographic relief along the southern RS margin is a lithospheric flexural response related to a combination of sediment loading and magmatic underplating at the edge of the margin (e.g., Watts, 2001). A more comprehensive data set for shear wave splitting shows a uniform regional pattern within Arabia in agreement with the earlier reported azimuths Qaysi et al. (2018), suggesting potential regional mantle convection flow. Geodetic and plate tectonic observations affirm the pre-existing argument that attribute the rotation to the subduction of the Tethys oceanic lithosphere beneath the Eurasian Plate (Reilinger and McClusky, 2011). Recent crustal-thickness and Vp/Vs ratios estimates across the Arabian P late suggest a uniform extension (Blanchette et al., 2020). The propagation of the GOA toward Afar and the absence of oceanic crust at the Afar triple-junction zone Almalki and Betts, (2021) along with mantle flow divergent from the extension axis (Figure 2) Petrunin et al. (2020) add additional uncertainty about the extent and influence of the plume.
The RS opening is interpreted to have initiated at ca 24 ± 2 Ma, whereas the GOA is interpreted to have opened at ∼ 17 Ma (e.g., Fournier et al. (2010)), with 14.2–18.6 mm/yr motion between Arabia and Eurasia plates (e.g., ArRajehi et al., 2010). However, motion rate estimate at ∼1 Myr intervals between Africa-Somalia plates since 20 Ma DeMets and Merkouriev, (2016) is inconsistent with previous open estimates along the GOA, which highlight the idea of either a coincide formation along both divergent margins (e.g., Bellahsen et al. (2013)) or later timing for the opening of the GOA. The history of the RS extension may have been preserved in dike intrusions that manifested mainly along the Arabian margin (Figure 2) (e.g., Roobol and Stewart, 2019). These dike intrusions potentially resulted from regional stress rotation, though this regional change is still debatable (Bosworth and Durocher, 2017). The slowing of the convergence or the onset of collision between Arabia and Eurasia may be reflected by a stepping of the locus of extension from the spreading axis to off-axis dikes along the western Arabian margin between 24 and 22 Ma (Almalki et al., 2015). Similarly, the change in Africa-Eurasia motion at 13 Ma is temporarily correlated with the onset of volcanism within the western Arabian margin (Figure 2) Camp and Roobol (1992) and resumption of the Sirhan rift volcanism (Figure 1) (Bosworth and Stockli, 2016). This seems to be synchronized with the recently suggested RS spreading initiation age at 13 Ma (Augustin et al., 2021). The combination of asymmetrical closure of the Tethys Ocean, and escape tectonics of the Anatolian Plate may have contributed to the rifting and rupturing of the RS and the GOA. However, this correlation assumes that the Arabian Plate is rigid as it transits the regional stresses, which is an assumption that needs to be validated.
Regardless of the tectonic drivers, several studies have suggested that separation of the Arabian and African plates was focussed on zones of lithospheric weakness (e.g., Molnar et al. (2020)), which could include heterogeneities in the lithosphere caused by a mantle plume (e.g. Molnar et al., 2017). Other modelling results require unreasonable scale of the plume to generate the architecture of the African-Arabian rift systems (Koptev et al. (2018)) or show that the lithospheric weakening caused by a circular plume is insufficient to drive extension alone (Khalil et al., 2020). These studies support interpretation that the plume may only weaken the lithosphere and so may play a localized force and that extension was mainly influenced by slab-pull forces that were transferred for a large distance into the interior of the Arabian Plate (e.g., Bellahsen et al., 2003). Determining the deformation architecture of the plate interior may provide insights into the role of far-field forces and how they are superimposed on zones of pre-existing weakness (e.g., ancient sutures zones and mantle heterogeneities such as the Afar plume).
Interior Deformation Implication
Although the interior deformation of the Arabian Plate is well documented, there is still uncertainty about their driving forces. Tesauro et al. (2018) suggested that most of the Arabian Plate is a long-term stable tectonic feature, though regional sequences of graben and anticlines systems were reported in Arabia (Barrier, et al., 2014; Bamousa et al., 2020). These systems have an oblique angle with the regional plate motion direction (Figure 1). Recent study shows spatial variability of plate velocities suggesting an active plate interior deformation (Aldaajani et al., 2021). The conjugate systems of extensional and compressional structures formed at Cenozoic would cast doubts on the influence of far-field or plume forces on the interior deformation within Arabia.
The role of basal tractions, linked with the Afar plume, on the western Arabia volcanism (Figure 2) is argued in many studies (e.g, Murcia et al., 2013). However, geochemical observations show that the plume did not play a significant role (Konrad et al., 2016; Altherr et al., 2019). It has been suggested that some of the volcanic fields are typical of lithospheric and/or asthenospheric sources (Sgualdo et al., 2015). The absence of elevated rifting axis along the RS and GOA suggests a negligible force on the interior deformation within the plate (Figure 2). On the opposite margin, slab tear migration along Zagros westward and southeastward until ca 10–5 Ma (Omrani et al., 2008; Agard et al., 2011) may trigger toroidal mantle flow and promote slab steepening and associated slab pull forces (i.e. collision) that could be driving structural architecture within central Arabia and its eastern margin (Bamousa et al., 2020).
Interpretation of width variations of the Arabian extensional margins (e.g., Stockli and Bosworth, (2019)) have not included the reported strain in the plate interior (e.g., Weijermars, 1998). This raises the potential for localised lithospheric body forces, such as those documented in the East African Rift system Stamps et al. (2015), as the driving mechanism for the observed deformation. These forces are driven by the horizontal gradient of the gravitational potential energy (GPE) that is associated with the lithospheric strength/thickness variations across the continent (Ghosh and Holt, 2012). There is no published GPE model for the Arabian Plate, thus limiting assessment of the effect of local vs. mantle forces on the Arabian Plate interior.
Consequently, acting tectonic forces on the Arabian Plate can be fallen into three origins: 1) Edge forces (i.e. plate boundaries such as the RS or GOA and far-field stresses), 2) Lithospheric body forces (i.e. driven by the horizontal gradient of GPE), and 3) Mantle-related tractions (i.e. Afar plume or other mantle flow) (Figure 2) (Warners-Ruckstuhl et al., 2010). However, thermal variability across the Arabian Plate Rolandone et al. (2013) may reflect in the strength variations of the Arabian lithosphere, which is assumed to control the tectonic processes at plate boundaries Steckler and Uri, (1986) and in the plate interior. This emphasizes the challenge of considering multiple driving forces with spatial lithospheric strength variability.
Lack of Data
Most of the previous studies have not always interpreted the data with the whole area in mind. It is quite clear that our firm comprehension of the plate margins evolution and extent along with the interior deformations would improve our understanding of the extent and impact of the regional forces. To that end, there is a need for more and higher-resolution geodetic, geophysical, and geochemical data in order to provide a coherent image about the geodynamical aspects of the Arabian Plate.
Author Contributions
All authors listed have made a substantial, direct, and intellectual contribution to the work and approved it for publication.
Conflict of Interest
The authors declare that the research was conducted in the absence of any commercial or financial relationships that could be construed as a potential conflict of interest.
Acknowledgments
TA and KA are supported by the King Abdulaziz City for Science and Technology (KACST). PB is supported by the Monash University. NA, EN, MO, and reviewers provided constructive comments that greatly improved the paper.
References
Agard, P., Omrani, J., Jolivet, L., Whitechurch, H., Vrielynck, B., Spakman, W., et al. (2011). Zagros Orogeny: A Subduction-Dominated Process. Geol. Mag. 148 (5–6), 692–725.
Aldaajani, T., Furlong, K., and Malservisi, R. (2021). The Rigidity of the Western Arabian Margin: Extensional Strain Rate Field from GPS Networks. Arabian J. Geosciences 14 (5), 1–11. doi:10.1007/s12517-021-06751-x
Almalki, K. A., Betts, P. G., and Ailleres, L. (2016). Incipient Seafloor Spreading Segments: Insights from the Red Sea. Geophys. Res. Lett. 43 (6), 2709–2715. doi:10.1002/2016gl068069
Almalki, K. A., Betts, P. G., and Ailleres, L. (2015). The Red Sea - 50 Years of Geological and Geophysical Research. Earth-Science Rev. 147, 109–140. doi:10.1016/j.earscirev.2015.05.002
Almalki, K. A., and Betts, P. G. (2021). Gulf of Aden Spreading Does Not Conform to Triple-junction Formation. Geology 49, 672–676. doi:10.1130/G48529.1
Altherr, R., Mertz-Kraus, R., Volker, F., Kreuzer, H., Henjes-Kunst, F., and Lange, U. (2019). Geodynamic Setting of Upper Miocene to Quaternary Alkaline Basalts from Harrat Al 'Uwayrid (NW Saudi Arabia): Constraints from K Ar Dating, Chemical and Sr-Nd-Pb Isotope Compositions, and Petrological Modeling. Lithos 330-331, 120–138. doi:10.1016/j.lithos.2019.02.007
ArRajehi, A., McClusky, S., Reilinger, R., Daoud, M., Alchalbi, A., Ergintav, S., et al. (2010). Geodetic Constraints on Present‐day Motion of the Arabian Plate: Implications for Red Sea and Gulf of Aden Rifting. Tectonics 29 (3). doi:10.1029/2009tc002482
Augustin, N., van der Zwan, F. M., Devey, C. W., and Brandsdóttir, B. (2021). 13 Million Years of Seafloor Spreading throughout the Red Sea Basin. Nat. Commun. 12, 2427. doi:10.1038/s41467-021-22586-2
Bamousa, A. O., Daoudi, M., and Hachemi, K. (2020). Tectono-Geomorphic Development of an Active Transtensional Depression: A Case Study of Mountain Tuwaiq Plateau, South Riyadh City, Saudi Arabia. Carb. Evap. 35 (4), 1–11.
Barrier, E., Machhour, L., and Blaizot, M. (2014). Petroleum Systems of Syria. AAPG Memoir. Pet. Syst. Tethyan region, 106, 335–378. doi:10.1036/13431862M1063612
Basuyau, C., Tiberi, C., Leroy, G., Stuart, A.A.-L., Al-Toubi, K., and Ebinger, C. (2010). Evidence of Partial Melting Beneath a Continental Margin: Case of Dhofar, in the Northeast Gulf of Aden (Sultanate of Oman). Geophys. J. Internat. 180 (2), 520–534.
Bellahsen, N., Faccenna, C., Funiciello, F., Daniel, J. M., and Jolivet, L. (2003). Why Did Arabia Separate from Africa? Insights from 3-D Laboratory Experiments. Earth Planet. Sci. Lett. 216, 365–381. doi:10.1016/s0012-821x(03)00516-8
Bellahsen, N., Husson, L., Autin, J., Leroy, S., and d'Acremont, E. (2013). The Effect of thermal Weakening and Buoyancy Forces on Rift Localization: Field Evidences from the Gulf of Aden Oblique Rifting. Tectonophysics 607, 80–97. doi:10.1016/j.tecto.2013.05.042
Ben-Avraham, Z., Garfunkel, Z., and Lazar, M. (2008). Geology and Evolution of the Southern Dead Sea Fault with Emphasis on Subsurface Structure. Annu. Rev. Earth Planet. Sci. 36, 357–387. doi:10.1146/annurev.earth.36.031207.124201
Beydoun, Z. R. (1999). Evolution and Development of the Levant (Dead Sea Rift) Transform System: a Historical-Chronological Review of a Structural Controversy. Geol. Soc. Lond. Spec. Publications 164 (1), 239–255. doi:10.1144/gsl.sp.1999.164.01.12
Blanchette, A., Klemperer, S., Mooney, W., and Zahran, H. (2020). Thickness of the Saudi Arabian Crust. EarthArXiv 1–43. doi:10.31223/X5CP47
Bohannon, R. G., and Eittreim, S. L. (1991). Tectonic Development of Passive continental Margins of the Southern and central Red Sea with a Comparison to Wilkes Land, Antarctica. Tectonophysics 198 (2-4), 129–154. doi:10.1016/0040-1951(91)90148-l
Bosworth, W., Huchon, P., and McClay, K. (2005). The Red Sea and Gulf of Aden Basins. J. Afr. Earth Sci. 43, 334–378. doi:10.1016/j.jafrearsci.2005.07.020
Bosworth, W., and Stockli, D. F. (2016). Early Magmatism in the Greater Red Sea Rift: Timing and Significance. Can. J. Earth Sci. 53 (11), 1158–1176. doi:10.1139/cjes-2016-0019
Bosworth, W., and Durocher, S. (2017). Present-Day Stress Fields of the Gulf of Suez (Egypt) Based on Exploratory Well Data: Non-Uniform Regional Extension and Its Relation to Inherited Structures and Local Plate Motion. J. Afr. Earth Sci. 136, 136–147.
Brune, S., and Autin, J. (2013). The Rift to Break-Up Evolution of the Gulf of Aden: Insights from 3D Numerical Lithospheric-Scale Modelling. Tectonophysics 607, 65–79. doi:10.1016/j.tecto.2013.06.029
Burov, E., and Cloetingh, S. (2009). Controls of Mantle Plumes and Lithospheric Folding on Modes of Intraplate continental Tectonics: Differences and Similarities. Geophys. J. Int. 178, 1691–1722. doi:10.1111/j.1365-246x.2009.04238.x
Camp, V. E., and Roobol, M. J. (1992). Upwelling Asthenosphere Beneath Western Arabia and Its Regional Implications. J. Geophys. Res. 97 (B11), 15255–15271.
Chang, S.-J., Merino, M., Van der Lee, S., Stein, S., and Stein, C. A. (2011). Mantle Flow beneath Arabia Offset from the Opening Red Sea. Geophys. Res. Lett. 38, 1–5. doi:10.1029/2010gl045852
Cochran, J. R. (1983). A Model for Development of Red Sea. Aapg Bull. 67 (1), 41–69. doi:10.1306/03b5acbe-16d1-11d7-8645000102c1865d
d'Acremont, E., Leroy, S., Beslier, M.-O., Bellahsen, N., Fournier, M., Robin, C., et al. (2005). Structure and Evolution of the Eastern Gulf of Aden Conjugate Margins from Seismic Reflection Data. Geophys. J. Int. 160 (3), 869–890. doi:10.1111/j.1365-246x.2005.02524.x
de Gouveia, S. V., Besse, J., de Lamotte, D. F., Greff-Lefftz, M., Lescanne, M., Gueydan, F., et al. (2018). Evidence of Hotspot Paths below Arabia and the Horn of Africa and Consequences on the Red Sea Opening. Earth Planet. Sci. Lett. 487, 210–220. doi:10.1016/j.epsl.2018.01.030
DeMets, C., and Merkouriev, S. (2016). High-resolution Estimates of Nubia-Somalia Plate Motion since 20 Ma from Reconstructions of the Southwest Indian Ridge, Red Sea and Gulf of Aden. Geophys. J. Int. 207 (1), 317–332. doi:10.1093/gji/ggw276
Eagles, G., Gloaguen, R., and Ebinger, C. (2002). Kinematics of the Danakil Microplate. Earth Planet. Sci. Lett. 203 (2), 607–620. doi:10.1016/s0012-821x(02)00916-0
Ebinger, C., and Belachew, M. (2010). Active Passive Margins. Nat. Geosci 3 (10), 670–671. doi:10.1038/ngeo972
Faccenna, C., Becker, T. W., Jolivet, L., and Keskin, M. (2013). Mantle Convection in the Middle East: Reconciling Afar Upwelling, Arabia Indentation and Aegean Trench Rollback. Earth Planet. Sci. Lett. 375, 254–269. doi:10.1016/j.epsl.2013.05.043
Fournier, M., Chamot-Rooke, N., Petit, C., Huchon, P., Al-Kathiri, A., Audin, L., et al. (2010). Arabia-Somalia Plate Kinematics, Evolution of the Aden-Owen-Carlsberg Triple junction, and Opening of the Gulf of Aden. J. Geophys. Res. 115, B04102. doi:10.1029/2008JB006257
Gerya, T., and Burov, E. (2018). Nucleation and Evolution of ridge-ridge-ridge Triple Junctions: Thermomechanical Model and Geometrical Theory. Tectonophysics 746, 83–105. doi:10.1016/j.tecto.2017.10.020
Ghosh, A., and Holt, W. E. (2012). Plate Motions and Stresses from Global Dynamic Models. Science 335, 6070–6843. doi:10.1126/science.1214209
Gürsoy, H., Tatar, O. R. H. A. N., Piper, J. D. A., Heimann, A., Kocbulut, F. İ. K. R. E. T., and Mesci, B. L. (2009). Palaeomagnetic Study of Tertiary Volcanic Domains in Southern Turkey and Neogene Anticlockwise Rotation of the Arabian Plate. Tectonophysics 465 (1-4), 114–127. doi:10.1016/j.tecto.2008.11.001
Gvirtzman, Z., Faccenna, C., and Becker, T. W. (2016). Isostasy, Flexure, and Dynamic Topography. Tectonophysics 683, 255–271. doi:10.1016/j.tecto.2016.05.041
Hempton, M. R. (1987). Constraints on Arabian Plate Motion and Extensional History of the Red Sea. Tectonics 6, 687–705. doi:10.1029/tc006i006p00687
Jolivet, L., and Faccenna, C. (2000). Mediterranean Extension and the Africa-Eurasia Collision. Tectonics 19, 1095–1106. doi:10.1029/2000tc900018
Khalil, H. M., Capitanio, F. A., Betts, P. G., and Cruden, A. R. (2020). 3‐D Analog Modeling Constraints on Rifting in the Afar Region. Tectonics 39 (10), e2020TC006339. doi:10.1029/2020tc006339
Konrad, K., Graham, D. W., Thornber, C. R., Duncan, R. A., Kent, A. J. R., and Al-Amri, A. M. (2016). Asthenosphere-lithosphere Interactions in Western Saudi Arabia: Inferences from 3He/4He in Xenoliths and Lava Flows from Harrat Hutaymah. Lithos 248-251, 339–352. doi:10.1016/j.lithos.2016.01.031
Koptev, A., Gerya, T., Calais, E., Leroy, S., and Burov, E. (2018). Afar Triple junction Triggered by Plume-Assisted Bi-directional continental Break-Up. Scientific Rep. 8 (1), 1–7. doi:10.1038/s41598-018-33117-3
Koshnaw, R. I., Stockli, D. F., and Schlunegger, F. (2019). Timing of the Arabia-Eurasia continental Collision-Evidence from Detrital Zircon U-Pb Geochronology of the Red Bed Series Strata of the Northwest Zagros Hinterland, Kurdistan Region of Iraq. Geology 47 (1), 47–50. doi:10.1130/g45499.1
Leroy, S., Razin, P., Autin, J., Bache, F., d’Acremont, E., Watremez, L., et al. (2013). “From Rifting to Oceanic Spreading in the Gulf of Aden: A Synthesis,” in Lithosphere Dynamics and Sedimentary Basins: The Arabian Plate and Analogues. Editor K. Al Hosani (Berlin, Heidelberg: Springer), 385–427. doi:10.1007/978-3-642-30609-9_20
Ligi, M., Bonatti, E., and Rasul, N. M. A. (2015). Seafloor Spreading Initiation: Geophysical and Geochemical Constraints from the Thetis and Nereus Deeps, central Red Sea. The Red Sea. Berlin, Heidelberg: Springer, 79–98. doi:10.1007/978-3-662-45201-1_4
Litak, R. K., Barazangi, M., Graham, B., Sawaf, T., Al-Imam, A., and Al-Youssef, W. (1998). Structure and Evolution of the Petroliferous Euphrates Graben System, Southeast Syria. AAPG Bull. 82 (6), 1173–1190. doi:10.1306/1d9bca2f-172d-11d7-8645000102c1865d
Lithgow-Bertelloni, C., and Richards, M. A. (1995). Cenozoic Plate Driving Forces. Geophys. Res. Lett. 22, 1317–1320. doi:10.1029/95gl01325
Makris, J., and Rihm, R. (1991). Shear-controlled Evolution of the Red Sea: Pull Apart Model. Tectonophysics 198 (2-4), 441–466. doi:10.1016/0040-1951(91)90166-p
Martinez, F., and Cochran, J. R. (1988). Structure and Tectonics of the Northern Red Sea: Catching a continental Margin between Rifting and Drifting. Tectonophysics 150 (1-2), 1–31. doi:10.1016/0040-1951(88)90293-4
McClusky, S., Reilinger, R., Ogubazghi, G., Amleson, A., Healeb, B., Vernant, P., et al. (2010). Kinematics of the Southern Red Sea–Afar Triple Junction and Implications for Plate Dynamics. Geophys. Res. Lett. 37 (5). doi:10.1029/2009gl041127
McGuire, A. V., and Bohannon, R. G. (1989). Timing of Mantle Upwelling: Evidence for a Passive Origin for the Red Sea Rift. J. Geophys. Res. 94 (B2), 1677–1682. doi:10.1029/jb094ib02p01677
McQuarrie, N., Stock, J. M., Verdel, C., and Wernicke, B. P. (2003). Cenozoic Evolution of Neotethys and Implications for the Causes of Plate Motions. Geophys. Res. Lett. 30, 2036. doi:10.1029/2003GL017992
Mechie, J., Ben-Avraham, Z., Weber, M. H., Götze, H.-J., Koulakov, I., Mohsen, A., et al. (2013). The Distribution of Moho Depths beneath the Arabian Plate and Margins. Tectonophysics 609, 234–249. doi:10.1016/j.tecto.2012.11.015
Molnar, N., Cruden, A., and Betts, P. (2020). The Role of Inherited Crustal and Lithospheric Architecture during the Evolution of the Red Sea: Insights from Three Dimensional Analogue Experiments. Earth Planet. Sci. Lett. 544, 116377. doi:10.1016/j.epsl.2020.116377
Molnar, N. E., Cruden, A. R., and Betts, P. G. (2017). Interactions between Propagating Rotational Rifts and Linear Rheological Heterogeneities: Insights from Three‐dimensional Laboratory Experiments. Tectonics 36 (3), 420–443. doi:10.1002/2016tc004447
Molnar, N. E., Cruden, A. R., and Betts, P. G. (2018). Unzipping Continents and the Birth of Microcontinents. Geology 46 (5), 451–454. doi:10.1130/g40021.1
Mouthereau, F., Lacombe, O., and Vergés, J. (2012). Building the Zagros Collisional Orogen: Timing, Strain Distribution and the Dynamics of Arabia/Eurasia Plate Convergence. Tectonophysics 532-535, 27–60. doi:10.1016/j.tecto.2012.01.022
Murcia, H., Lindsay, Jan. M., Niedermann, S., Cronin, S. J., Smith, I. E., Nabil Nasr El-Masry, , et al. (2013). The Potential Use of Cosmogenic Nuclides for Dating in Harrat Rahat. VORISA Scientific Meeting, Jeddah:King Abdulaziz University 17, 39–43. doi:10.13140/RG.2.1.3789.4484
Nonn, C., Leroy, S., Lescanne, M., and Castilla, R. (2019). Central Gulf of Aden Conjugate Margins (Yemen-Somalia): Tectono-Sedimentary and Magmatism Evolution in Hybrid-type Margins. Mar. Pet. Geology. 105, 100–123. doi:10.1016/j.marpetgeo.2018.11.053
Nuriel, P., Weinberger, R., Kylander-Clark, A. R. C., Hacker, B. R., and Craddock, J. P. (2017). The Onset of the Dead Sea Transform Based on Calcite Age-Strain Analyses. Geology 45 (7), 587–590. doi:10.1130/g38903.1
Okay, A. I., Zattin, M., and Cavazza, W. (2010). Apatite Fission-Track Data for the Miocene Arabia-Eurasia Collision. Geology 38 (1), 35–38. doi:10.1130/g30234.1
Omrani, J., Agard, P., Whitechurch, H., Benoit, M., Prouteau, G., and Jolivet, L. (2008). Arc-magmatism and Subduction History beneath the Zagros Mountains, Iran: a New Report of Adakites and Geodynamic Consequences. Lithos 106 (3-4), 380–398. doi:10.1016/j.lithos.2008.09.008
Penney, C., Tavakoli, F., Saadat, A., Nankali, H. R., Sedighi, M., Khorrami, F., et al. (2017). Megathrust and Accretionary Wedge Properties and Behaviour in the Makran Subduction Zone. Geophys. J. Int. 209 (3), 1800–1830. doi:10.1093/gji/ggx126
Petrunin, A. G., Kaban, M. K., El Khrepy, S., and Al-Arifi, N. (2020). Mantle Convection Patterns Reveal the Mechanism of the Red Sea Rifting. Tectonics 39 (2), e2019TC005829.
Pirouz, M., Avouac, J.-P., Hassanzadeh, J., Kirschvink, J. L., and Bahroudi, A. (2017). Early Neogene Foreland of the Zagros, Implications for the Initial Closure of the Neo-Tethys and Kinematics of Crustal Shortening. Earth Planet. Sci. Lett. 477, 168–182. doi:10.1016/j.epsl.2017.07.046
Qaysi, S., Liu, K. H., and Gao, S. S. (2018). A Database of Shear‐Wave Splitting Measurements for the Arabian Plate. Seismological Res. Lett. 89 (6), 2294–2298. doi:10.1785/0220180144
Reilinger, R., and McClusky, S. (2011). Nubia-Arabia-Eurasia Plate Motions and the Dynamics of Mediterranean and Middle East Tectonics. Geophys. J. Int. 186 (3), 971–979. doi:10.1111/j.1365-246x.2011.05133.x
Reilinger, R., McClusky, S., Vernant, P., Lawrence, S., Ergintav, S., Cakmak, R., et al. (2006). GPS Constraints on continental Deformation in the Africa‐Arabia‐Eurasia continental Collision Zone and Implications for the Dynamics of Plate Interactions. J. Geophys. Res. Solid Earth 111 (B5). doi:10.1029/2005jb004051
Rogers, N. W. (2006). Basaltic Magmatism and the Geodynamics of the East African Rift System. Geol. Soc. Lond. Spec. Publications 259 (1), 77–93. doi:10.1144/gsl.sp.2006.259.01.08
Roobol, M. J., and Stewart, I. C. F. (2019). “Cenozoic Faults and Seismicity in Northwest Saudi Arabia and the Gulf of Aqaba region” in Geological Setting, Palaeoenvironment and Archaeology of the Red Sea. Cham:Springer, pp. 275–305.
Rolandone, F., Lucazeau, F., Leroy, S., Mareschal, J.-C., Jorand, R., Goutorbe, B., et al. (2013). New Heat Flow Measurements in Oman and the thermal State of the Arabian Shield and Platform. Tectonophysics 589, 77–89. doi:10.1016/j.tecto.2012.12.034
Rooney, T. O., Mohr, P., Dosso, L., and Hall, C. (2013). Geochemical Evidence of Mantle Reservoir Evolution during Progressive Rifting along the Western Afar Margin. Geochimica et Cosmochimica Acta 102, 65–88. doi:10.1016/j.gca.2012.08.019
Sebai, A., Zumbo, V., Féraud, G., Bertrand, H., Hussain, A. G., Giannerini, G., et al. (1991). 40Ar/39Ar Dating of Alkaline and Tholeiitic Magmatism of Saudi Arabia Related to the Early Red Sea Rifting. Earth Planet. Sci. Lett. 104 (2-4), 473–487. doi:10.1016/0012-821x(91)90223-5
Schellart, W. P. (2004). Quantifying the Net Slab Pull Force as a Driving Mechanism for Plate Tectonics Geophys. Res. Lett. 31 (7), L07611.
Sgualdo, P., Aviado, K., Beccaluva, L., Bianchini, G., Blichert-Toft, J., Bryce, J. G., et al. (2015). Lithospheric Mantle Evolution in the Afro-Arabian Domain: Insights from Bir Ali Mantle Xenoliths (Yemen). Tectonophysics 650, 3–17. doi:10.1016/j.tecto.2014.11.025
Sharland, P. R., Archer, R., Casey, D. M., Davies, R. B., Hall, S. H., Heward, A. P., et al. (2001). Arabian Plate Sequence Stratigraphy. Bahrain: GeoArabia Special Publication, 2. 371.
Stamps, D. S., Iaffaldano, G., and Calais, E. (2015). Role of Mantle Flow in Nubia-Somalia Plate Divergence. Geophys. Res. Lett. 42 (2), 290–296. doi:10.1002/2014gl062515
Steckler, M. S., and Uri, S. (1986). Lithospheric Strength Variations as a Control on New Plate Boundaries: Examples from the Northern Red Sea Region. Earth Planet. Sci. Lett. 79 (1-2), 120–132. doi:10.1016/0012-821x(86)90045-2
Stern, R. J., and Johnson, P. (2010). Continental Lithosphere of the Arabian Plate: a Geologic, Petrologic, and Geophysical Synthesis. Earth-Science Rev. 101 (1-2), 29–67. doi:10.1016/j.earscirev.2010.01.002
Stockli, D. F., and Bosworth., W. (2019). Iming of Extensional Faulting along the Magma-Poor Central and Northern Red Sea Rift Margin-Transition from Regional Extension to Necking along a Hyperextended Rifted Margin. Geological Setting, Palaeoenvironment and Archaeology of the Red Sea. Cham: Springer, 81–111. doi:10.1007/978-3-319-99408-6_5T
Sultan, M., Becker, R., Arvidson, R. E., Shore, P., Stern, R. J., El Alfy, Z., et al. (1992). Nature of the Red Sea Crust: A Controversy Revisited. Geol 20, 593–596. doi:10.1130/0091-7613(1992)020<0593:notrsc>2.3.co;2
Szymanski, E., Stockli, D. F., Johnson, P. R., and Hager, C. (2016). Thermochronometric Evidence for Diffuse Extension and Two-phase Rifting within the Central Arabian Margin of the Red Sea Rift. Tectonics 35 (12), 2863–2895. doi:10.1002/2016tc004336
Tesauro, M., Kaban, M. K., Petrunin, A. G., El Khrepy, S., and Al-Arifi, N. (2018). Strength and Elastic Thickness Variations in the Arabian Plate: A Combination of Temperature, Composition and Strain Rates of the Lithosphere. Tectonophysics 746, 398–411. doi:10.1016/j.tecto.2017.03.004
Vernant, P., Nilforoushan, F., Hatzfeld, D., Abbassi, M. R., Vigny, C., Masson, F., et al. (2004). Present-day Crustal Deformation and Plate Kinematics in the Middle East Constrained by GPS Measurements in Iran and Northern Oman. Geophys. J. Int. 157 (1), 381–398. doi:10.1111/j.1365-246x.2004.02222.x
Walpersdorf, A., Hatzfeld, D., Nankali, H., Tavakoli, F., Nilforoushan, F., Tatar, M., et al. (2006). Difference in the GPS Deformation Pattern of North and Central Zagros (Iran). Geophys. J. Int. 167 (3), 1077–1088. doi:10.1111/j.1365-246x.2006.03147.x
Warners‐Ruckstuhl, K. N., Th Meijer, P., Govers, R., and Wortel, M. J. R. (2010). A Lithosphere‐dynamics Constraint on Mantle Flow: Analysis of the Eurasian Plate. Geophys. Res. Lett. 37 (18), 1–6. doi:10.1029/2010GL044431
Weijermars, R. (1998). Plio-quaternary Movement of the East Arabian Block. GeoArabia 3 (4), 509–540.
White, R., and McKenzie, D. (1989). Magmatism at Rift Zones: the Generation of Volcanic continental Margins and Flood Basalts. J. Geophys. Res. 94 (B6), 7685–7729. doi:10.1029/jb094ib06p07685
Yao, Z., Mooney, W. D., Zahran, H. M., and Youssef, S. E.-H. (2017). Upper Mantle Velocity Structure beneath the Arabian Shield from Rayleigh Surface Wave Tomography and its Implications. J. Geophys. Res. Solid Earth 122 (8), 6552–6568. doi:10.1002/2016jb013805
D.A. Yuen, S.I. Karato, S. Maruyama, and B.F. Windley (2007). in Superplumes: Beyond Plate Tectonics (New York: Springer), 569.
Keywords: plume, far-field stresses, geodynamic, arabian plate, slab-pull
Citation: Aldaajani TZ, Almalki KA and Betts PG (2021) Plume Versus Slab-Pull: Example from the Arabian Plate. Front. Earth Sci. 9:700550. doi: 10.3389/feart.2021.700550
Received: 26 April 2021; Accepted: 07 June 2021;
Published: 17 June 2021.
Edited by:
Nico Augustin, GEOMAR Helmholtz Center for Ocean Research Kiel, GermanyReviewed by:
Emmanuel Njinju, Virginia Tech, United StatesMasaki Ogawa, The University of Tokyo, Japan
Copyright © 2021 Aldaajani, Almalki and Betts. This is an open-access article distributed under the terms of the Creative Commons Attribution License (CC BY). The use, distribution or reproduction in other forums is permitted, provided the original author(s) and the copyright owner(s) are credited and that the original publication in this journal is cited, in accordance with accepted academic practice. No use, distribution or reproduction is permitted which does not comply with these terms.
*Correspondence: Khalid A. Almalki, bW1hbGtpQGthY3N0LmVkdS5zYQ==