- 1Istituto Nazionale di Geofisica e Vulcanologia, Sezione di Bologna, Bologna, Italy
- 2School of Agriculture and Environment, Massey University, Palmerston North, New Zealand
- 3Institute of Earth Physics and Space Sciences, Sopron, Hungary
- 4Dipartimento di Scienze della Terra e del Mare (DiSTeM), Università degli Studi di Palermo, Palermo, Italy
- 5Istituto Nazionale di Geofisica e Vulcanologia, Sezione di Palermo, Palermo, Italy
- 6Cameroun-Banque Mondiale, Projet de Lutte Contre les Inondations (PULCI), Yagoua, Cameroon
Volcanic lakes pose specific hazards inherent to the presence of water: phreatic and phreatomagmatic eruptions, lahars, limnic gas bursts and dispersion of brines in the hydrological network. Here we introduce the updated, interactive and open-access database for African volcanic lakes, country by country. The previous database VOLADA (VOlcanic LAke DAta Base, Rouwet et al., Journal of Volcanology and Geothermal Research, 2014, 272, 78–97) reported 96 volcanic lakes for Africa. This number is now revised and established at 220, converting VOLADA_Africa 2.0 in the most comprehensive resource for African volcanic lakes: 81 in Uganda, 37 in Kenya, 33 in Cameroon, 28 in Madagascar, 19 in Ethiopia, 6 in Tanzania, 2 in Rwanda, 2 in Sudan, 2 in D.R. Congo, 1 in Libya, and 9 on the minor islands around Africa. We present the current state-of-the-art of arguably all the African volcanic lakes that the global experts and regional research teams are aware of, and provide hints for future research directions, with a special focus on the volcanic hazard assessment. All lakes in the updated database are classified for their genetic origin and their physical and chemical characteristics, and level of study. The predominant rift-related volcanism in Africa favors basaltic eruptive products, leading to volcanoes with highly permeable edifices, and hence less-developed hydrothermal systems. Basal aquifers accumulate under large volcanoes and in rift depressions providing a potential scenario for phreatomagmatic volcanism. This hypothesis, based on a morphometric analysis and volcanological research from literature, conveys the predominance of maar lakes in large monogenetic fields in Africa (e.g. Uganda, Cameroon, Ethiopia), and the absence of peak-activity crater lakes, generally found at polygenetic arc-volcanoes. Considering the large number of maar lakes in Africa (172), within similar geotectonic settings and meteoric conditions as in Cameroon, it is somewhat surprising that “only” from Lake Monoun and Lake Nyos fatal CO2 bursts have been recorded. Explaining why other maars did not experience limnic gas bursts is a question that can only be answered by enhancing insights into physical limnology and fluid geochemistry of the so far poorly studied lakes. From a hazard perspective, there is an urgent need to tackle this task as a community.
Introduction
The Cameroonian “killer lakes” Nyos and Monoun (Western Africa, Figure 1) are reputable for having induced the boom in volcanic lake studies since the late 1980s (e.g. Kling et al., 1989; Giggenbach, 1990; Giggenbach et al., 1991; Freeth, 1992; Evans et al., 1993, 1994; Freeth, 1994; Martini et al., 1994; Zhang, 1996, 1998; Viollier et al., 1995, 1997; Aeschbach-Hertig et al., 1996, 1999), to such a degree to have introduced a “Nyos bias” – for the good and the bad – on how to cope with lakes in volcanic craters in terms of hazard assessment and risk mitigation strategies (Rouwet et al., 2015a, 2019; Rouwet, 2021). A major question that arose from most of these Nyos-biased studies was whether any other lakes were capable of bursting CO2 in a sudden manner as Lakes Monoun and Nyos did in 1984 and 1986, respectively. Despite three decades of post-Nyos research, this question still remains unanswered for too many lakes (Rouwet, 2021).
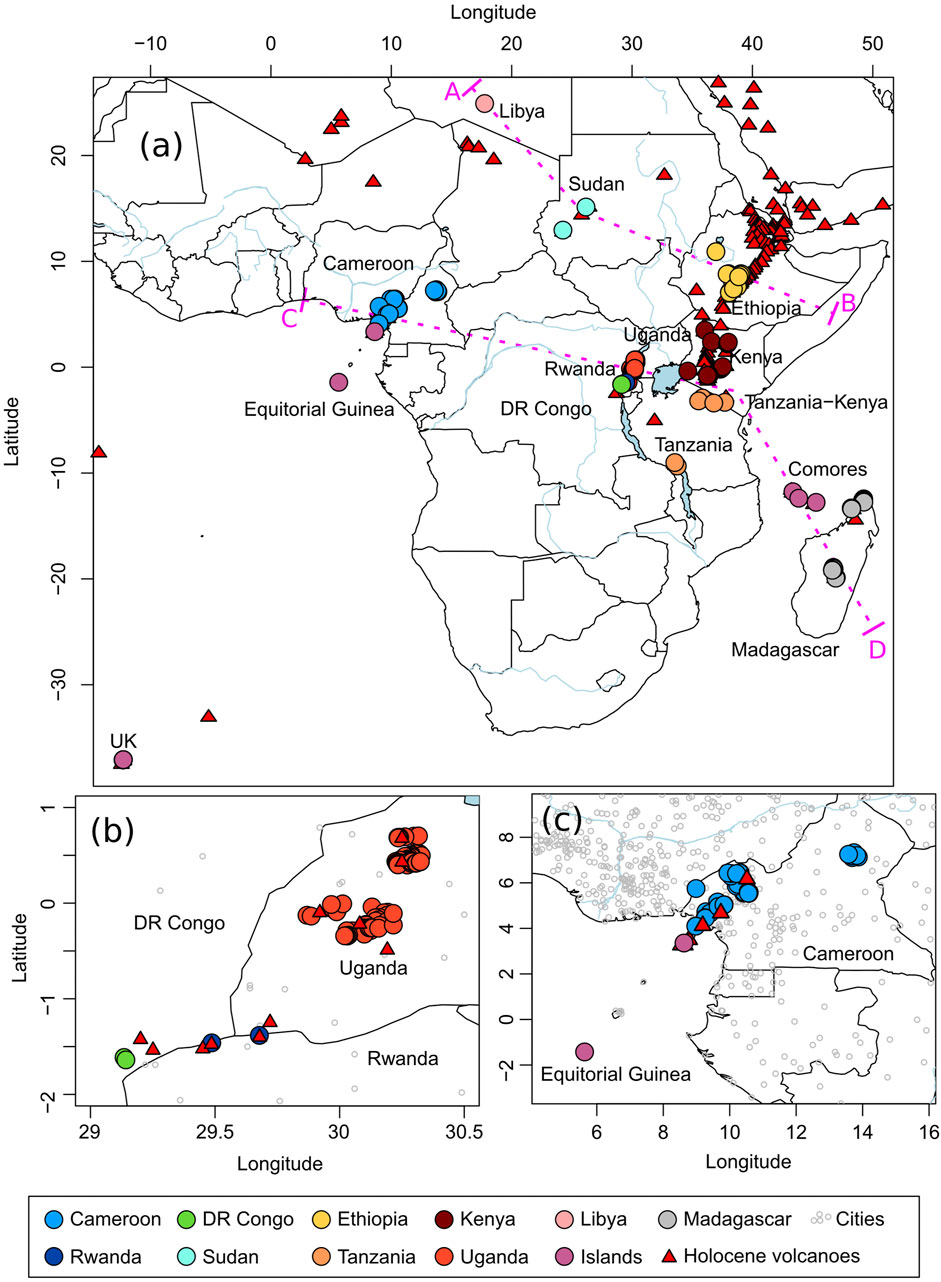
FIGURE 1. (A) Location map of the African volcanic lakes, color-coded per country. The zoomed area mainly shows the lakes in Bunyaruguru, Ndali-Kasenda and Fort Portal volcanic fields in Uganda (B), and the Cameroon Volcanic Line (C). Holocene active volcanoes are exported from the Global Volcanism Program-Smithsonian database (https://volcano.si.edu/).
The Nyos bias expresses an ambiguity. On the one hand, dynamics at other lakes, in Africa and other continents, are often over-interpreted as if they should be Nyos-type lakes (i.e. volcanic lakes affected by cyclical, explosive gas release due to gas pressure build up in deep waters) when CO2 degassing occurs in those lake areas; this view might be most prudent, in case of doubt, although it turns out to be unrealistic in some cases (e.g. Rouwet et al., 2019). On the other hand, the only way to discover whether CO2, or other gas species, are accumulated up to critical pressure conditions in deep lake strata is to lower Conductivity-Temperature-Depth/Pressure (CTD) probes, sample lake water and measure dissolved gases along vertical profiles. This direct investigation has been applied only to a few lakes in order to have a complete picture of how volcanic lake degassing works. Nevertheless, it is a fact that, especially, the 1986 Lake Nyos gas burst has increased the general awareness of the potential danger this type of volcanic lakes poses (e.g. Giggenbach et al., 1991; Martini et al., 1994; Aeschbach-Hertig et al., 1996, 1999; Caliro et al., 2008; Carapezza et al., 2008; Chiodini et al., 2012; Cabassi et al., 2013, 2014; Rouwet et al., 2019, 2020; Rouwet, 2021).
Rouwet et al. (2014) introduced the first, incomplete version of the community-based, interactive, and open-source (https://vhub.org/resources/2437) VOlcanic LAkes DAta base (VOLADA). Out of respect for Lake Nyos, “the mother of volcanic lakes,” here we first provide an update on the post-Pliocene (i.e. contemporaneous) volcanic lakes located on the African continent, Madagascar and minor islands (Annobon, Bioko, Tristan da Cunha, Karthala, Mohéli Island and Mayotte, Figure 1), presented as VOLADA_Africa 2.0. This version aims to 1) supply an updated list of the geo-referenced volcanic lakes, 2) shed light on the type of each lake, following classical (Pasternack and Varekamp, 1997; Varekamp et al., 2000) and novel (Christenson et al., 2015) classification schemes, 3) show a realistic picture on the level of study of each volcanic lake in Africa, regarding volcanological research sensu lato, and 4) provide a hazard assessment related to volcanic lakes in Africa. As volcanological literature revealed the maar nature of many of the catalogued lakes, an analysis of morphometric parameters for maar craters (Graettinger, 2018) for the entire database leads to a conceptual model that corroborates why African volcanic lakes actually are predominantly maar lakes. Consequently, we suggest strategies for future research and monitoring setups for those lakes we deem as potentially hazardous, or as peculiar for other reasons from a volcanological point of view.
The Volcanic Lake Catalogue for Africa
VOLADA “1.0”
VOLADA was introduced by Rouwet et al. (2014) with the aim to review and update the number of volcanic lakes reported in earlier studies (e.g. Delmelle and Bernard, 2000; Pérez et al., 2011; Lockwood and Kusakabe, 2018) and to better locate them on the globe. VOLADA aimed at being an interactive and open-access tool, perennially open for discussion, additions and corrections by the entire scientific community. In 2014, VOLADA listed 474 volcanic lakes, with 86 lakes in Europe (30 in the Azores), 97 in Africa (20 in Cameroon), 51 in North America (21 in Mexico), 58 in Central America (27 in Costa Rica), 28 in South America (18 in Chile-Argentina), 111 in Asia (33 in Indonesia), and 43 in Oceania (27 in New Zealand). If sufficient information was available, the lakes were classified following the physical (a scale from 1 to 10, based on the state of activity of the hosting volcano; Pasternack and Varekamp, 1997), and chemical properties of the lake water (“gas-dominated” versus “rock-dominated” lake waters, G versus R, Varekamp et al., 2000). The level of study of each lake was reported as a numerical scale from 1 to 5, from “well studied” (1) to “poorly or not studied” (5).
VOLADA_Africa 2.0: Revised Methodology
Subsequently, Christenson et al. (2015) proposed (by KN) an alternative classification scheme, based on the genetic process behind the lake basin formation, regardless of the current state of activity of the lakes, or the chemical-physical properties of the lake water. The latter information appeared to be often unavailable, as a consequence of the general poor level of study of most lakes.
The genetic classification scheme by Christenson et al. (2015) follows some basic rules, and results in alphanumerical codes (G0-1_R0-1_T0-1_L0-1), as:
(1) The geotectonic assessment in which the lake is located (G) can be related to monogenetic (0), or polygenetic (1) volcanism;
(2) The relationship between the volcanism and the eventual lake formation (R) is weak (0), or strong (1);
(3) The timing of lake formation in relation with the volcanism (T) can be long (0) or shortly (1) after the volcanic activity;
(4) The location of the volcanic lake in relation to the volcanic centre (L) can be off (0) or over (1) the vent.
A major accomplishment of this genetic classification scheme is to eliminate the ambiguity that existed in naming “volcanic lakes,” as each alphanumerical code points to a specific type of lake: crater lake (G1R1T1L1), caldera lake (G1R1T0L1), maar-diatreme lake (G0R1T1L1), geothermal lake (G0-1R1T1L0-1), lake in a volcanic environment (G1R0T0L0), lake dammed by volcanic deposits (G0-1R0-1T0-1L0), volcanic lake after snow melting (G0-1R1T1L1), and the generic “volcanic lake” covering the entire range of lake types (G0-1R0-1T0-1L0-1). This “grassroots” classification scheme, especially adapted to shed light on the poorly studied lakes, will now be applied to VOLADA, starting with an update for the African lakes (VOLADA_Africa 2.0) (Table 1).
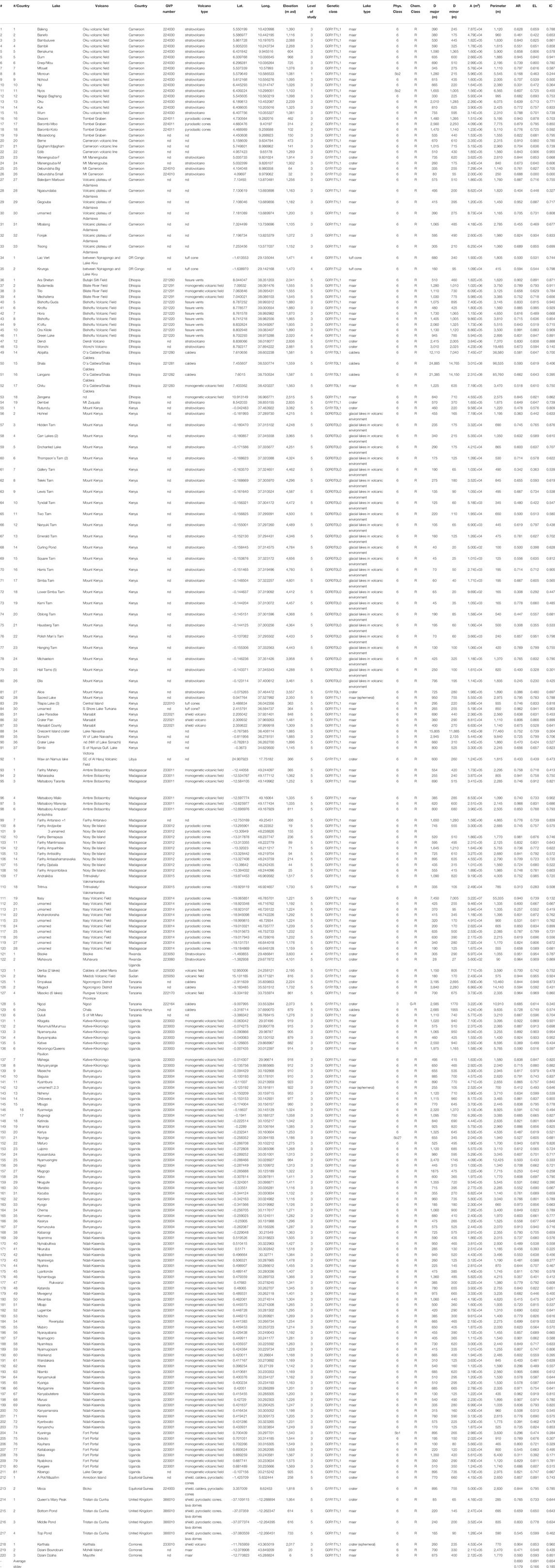
TABLE 1. The VOLADA_Africa 2.0 database, mentioning the lake number (#), the number of lakes in the same country (#/country), the lake name, the volcano the depends on, the country, GVP number (Global Volcanism Program number https://volcano.si.edu/search_volcano.cfm), volcano type, the latitude and longitude (Lat. and Long. in decimal coordinates), the elevation above sea level (m a.s.l.), level of study (1–5, see text for details), genetic class (see text for details), lake type, physical and chemical classification, length of major and minor lake axes (Dmajor and Dminor, respectively, in m), lake surface area (A, in m2), lake perimeter (in meter), Aspect Ratio (AR, Eq. 1), Elongation (EL, Eq. 2), Isoperimetric Circularity (IC, Eq. 3).
The chemical classification system (G-versus R-dominated, Varekamp et al., 2000) was maintained here, despite the poor knowledge on lake water chemistry of the African lakes. Gas-dominated lakes (G) are generally found in craters of (highly) active volcanoes, with low-pH and heated water bodies that result from gas and vapor input from the underlying magmatic-hydrothermal system. Rock-dominated lakes (R) host water with a purely meteoric origin that attained equilibrium through water-rock interaction with the host rock that composes the lake basin. As the gas-dominated lakes are often easily recognized by their color (turquoise, green, blue-green, white, grey, or even yellow; Christenson et al., 2015), or the presence of evaporation plumes coming off their surface, in the absence of this characteristic feature the lakes were, arguably correctly, classified as R-dominated. Apparently, Africa does not host gas-dominated, “erupting,” peak-, high- and medium-activity lakes, hence, all lakes are R-dominated (Table 1). The physical classification system by Rouwet et al. (2014) (simplified from Pasternack and Varekamp, 1997) is adopted here: 1) erupting (i.e. hot, hyper-saline and ultra-acidic (pH near 0) lakes breached periodically by phreatic or phreatomagmatic eruptions), 2) peak-activity (i.e. heated, saline and acidic (pH < 2 lakes topping actively degassing magmatic systems), 3) high activity (a and b, for higher and lower solutes contents, respectively) (i.e. saline and acidic (pH < 2) lakes showing evidence of heating and passively degassing hydrothermal systems; e.g. steam heated SO4-rich lakes), 4) medium activity (a and b, for higher and lower solutes contents, respectively) (i.e. lakes with low heating and input from an underlying hydrothermal system, but not composed of purely meteoric water), 5) low activity (a and b1/b2, for higher and lower solutes contents, respectively, i.e. non-acidic lakes generally with evidence of input of CO2-rich fluids), 6) no activity (i.e. lakes composed of purely meteoric water without any evidence of a degassing hydrothermal system). Noteworthy, lake class “5b2” points to the potentially hazardous “Nyos-type” lakes.
The level of study (a numerical scale from 1 to 5) of volcanic lakes is revised here, following the new criteria: (1) monitored, (2) well studied in the scientific literature, (3) few scientific publications available, (4) no publications, but web-sourced information available, (5) no information available, at all.
African volcanic lakes are well studied in terms of micro- and macrobiology of the water, and palynology, microfacies analyses (climate studies), dating, stable isotopic composition, and non-terrestrial biological records of cores from lake sediments, among other topics (e.g. Gasse and Van Campo, 1998, 2001; Williamson et al., 1999; Barker et al., 2000; Rumes et al., 2005; Eggermont et al., 2006; Delalande et al., 2008; Kebede et al., 2009; Lemma, 2009; Russell et al., 2009; Cocquyt et al., 2010; Giresse and Makaya-Mvoubou, 2010; Ndebele-Murisa et al., 2010; Ryves et al., 2011; Garcin et al., 2012, 2014; Lebamba et al., 2016). These numerous studies sometimes revealed useful details, but their goals are outside the scope of the present review that aims to provide a better database on the volcanic lakes’s physical limnology and volcanology, to convert VOLADA_Africa 2.0 into a useful tool to better assess future volcanic hazards related to the African volcanic lakes (Aka et al., 2017). The physical volcanology and petrogenetic aspects of some lake-hosting areas, however, have largely increased our insights in single cases (Chapman et al., 1998; Barker et al., 2000; Freeth and Rex, 2000; Anema and Fesselet 2003; Haileab et al., 2004; Deruelle et al., 2007; Aka et al., 2008, 2018; Ngwa et al., 2010; Bruhn et al., 2011; Nkouandou and Temdjim, 2011; Ngos and Giresse, 2012; Temdjim, 2012; Aka and Yokoyama, 2013; Tchamabe et al., 2013; Rufer et al., 2014; Asaah et al., 2015; Delalande-Le Mouëllic et al., 2015; Balashova et al., 2016; Pouclet et al., 2016; Jolie, 2019; Venturi et al., 2019). Nevertheless, the general number of these studies remains limited in many areas.
The African volcanic lakes were pin-pointed by decimal coordinates by scanning the Earth’s surface through GoogleEarthPro (https://www.google.it/intl/it/earth/index.html) and OpenStreetMap (https://www.openstreetmap.org/) web sources (Figure 1), resulting in an interactive VOLADA-Africa 2.0 spreadsheet (Table 1), free available through VHub (https://vhub.org/groups/iavceicvl/resources). Lakes were ordered by country. Where available, the GVP number (http://volcano.si.edu/search_volcano.cfm) (Global Volcanism Program), its elevation above sea level, and the volcano and type of volcano the lake belongs to, are reported. Holocene volcanoes are exported on the map in Figure 1 from the Global Volcanism Program; it is apparent that not all volcanic lakes coincide with Holocene active volcanoes, which implies that 1) not all the lakes belong to Holocene active volcanoes, or 2) the date of the last eruptions from the reported lakes is unknown.
A recent study by Graettinger (2018) deduced dimensionless morphometric parameters (ratios) to better describe maar crater morphology. The use of ratios provides the benefit to represent lake shape, regardless of the absolute dimensions of the craters. We here apply the same approach for the lake morphology of the 220 volcanic lakes in Africa, through the “ruler” and “polyogone” tools in GoogleEarthPro (Figure 2), with an accuracy of ± 5 m (Table 1; Figure 2). The Aspect Ratio (AR) is the ratio between the lake’s minor (Dmin) and major (Dmax) diameter, with the minor diameter perpendicular to the major diameter:
An AR closer to 1 implies an equant distance from the center of the lake.
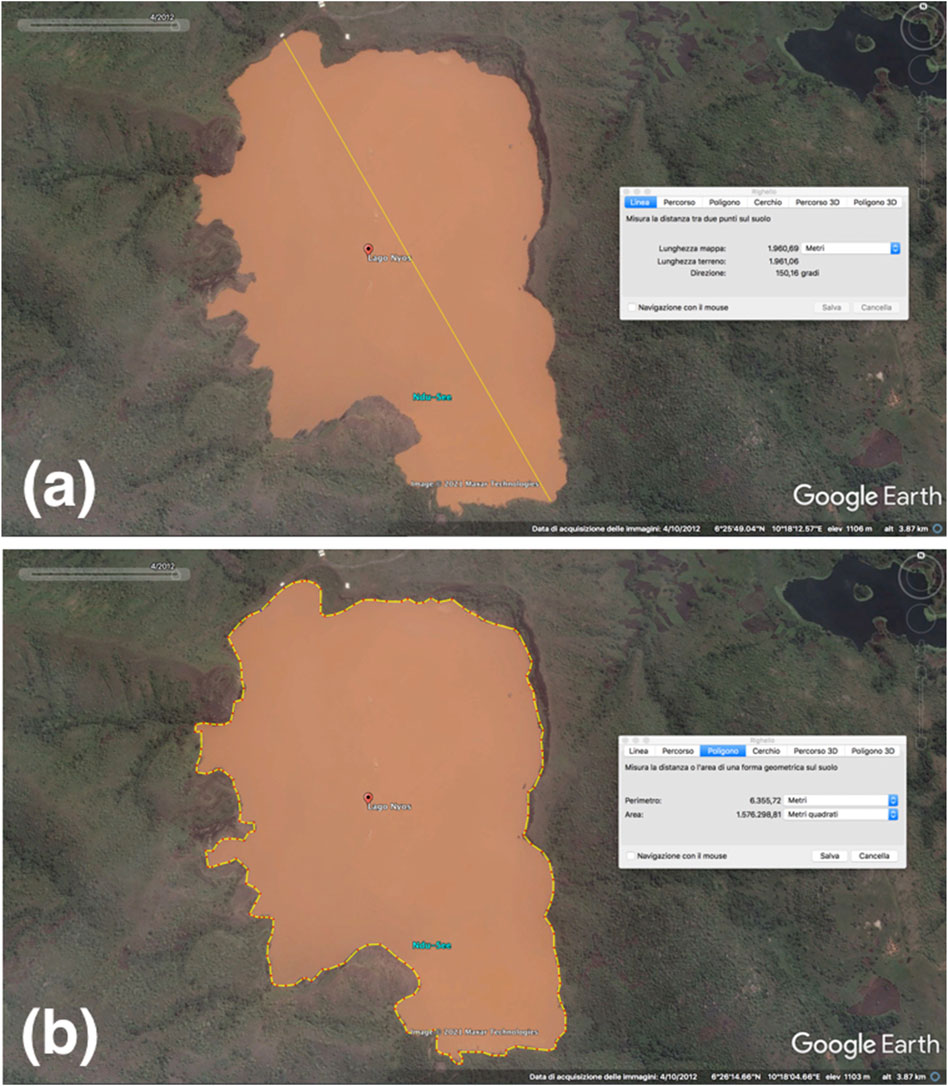
FIGURE 2. (A) Ruler option to measure distances in GoogleEarth, (B) Polygone option to measure circumferences and surface areas in GoogleEarth (image© Maxar Technologies).
The Elongation (EL) instead relates the area of a circle with the measured major diameter to the surface area of the lake (A). EL better describes asymmetrical morphologies compared to AR.
The Isoperimetric Circularity (IC) compares the lake surface area with the area (A) of a circle with the same perimeter (p):
Perfectly circu ar lake shapes have IC values near 1 or equal to 1, whereas lake shapes with a varying angle of curvature along their perimeter deviate further from 1.
A simple statistical approach (histograms) of the morphometric data for the African volcanic lakes is applied and compared to the data provided by Graettinger (2018) for maar craters in the world.
VOLADA_Africa 2.0: Country by Country
The updated database VOLADA_Africa 2.0 (Table 1) counts a significantly higher number (220) of volcanic lakes in Africa, compared to the 97 lakes in the original version of VOLADA (Rouwet et al., 2014). This large difference results mainly from the inclusion of numerous lakes in Uganda, Kenya, and Madagascar, previously not counted. Volcanic lakes in Africa are often located in remote and/or low population density regions (Figure 1). The country with most volcanic lakes is Uganda (81), followed by Kenya (37), Cameroon (33), Madagascar (28), Ethiopia (19), Tanzania (6), the minor islands (i.e. Annobon, Bioko and Tristan da Cunha, West of the continent; and Karthala, Mohéli and Mayotte, Southeast of the continent, (9), Rwanda (2), Sudan (2), D.R. Congo (2), and Libya (1).
Cameroon
The pioneering study by Kling (1988) on the limnology of 39 Cameroonian lakes, of which 33 are of volcanic origin, forms the basis of the revised catalogue for Cameroon. Recent insights on the chemical and stable isotopic composition of 17 of the 33 Cameroonian volcanic lakes came from Issa et al. (2014), which followed the cataloguing philosophy adopted by Kling (1988), and our current study. Needless to say, Lakes Nyos (Figure 3) and Monoun are arguably two of the most studied volcanic lakes on Earth. Well studied aspects for Lakes Nyos and Monoun are:
(5) the degassing dynamics of the 1984 and 1986 gas bursts (Freeth and Kay, 1987; Kling et al., 1987; Sigurdsson et al., 1987; Barberi et al., 1989; Kanari, 1989; Tazieff, 1989; Freeth, 1990; Freeth, 1992; Evans et al., 1993; Freeth, 1994);
(6) the hazard assessment mainly based on the chemistry of lake water and dissolved gases (Sano et al., 1987, 1990; Tuttle et al., 1987; Kling et al., 1989; Kusakabe et al., 1989, 2000, 2008; Lockwood and Rubin, 1989; Giggenbach, 1990; Nojiri et al., 1990, 1993; Faivre Pierret et al., 1992; Kusakabe and Sano, 1992; Tietze, 1992; Evans et al., 1993, 1994; Kantha and Freeth, 1996; Tanyileke et al., 1996; Nagao et al., 2010; Yoshida et al., 2010; Issa et al., 2014; Tassi and Rouwet, 2014; Anazawa et al., 2019; Kusakabe et al., 2019);
(7) the risk mitigation through artificial degassing (since 2001, intensified since 2011 and ongoing; Halbwachs and Sabroux, 2001; Halbwachs et al., 1993, 2004, 2020; McCord and Schladow, 1998; Schmid et al., 2003, 2004, 2006; Kling et al., 2005; Ohba et al., 2017; Saiki et al., 2017; Yoshida et al., 2017);
(8) the dam stability and its recent (2014–2015) reinforcement (Lockwood et al., 1988; Freeth and Rex, 2000; Aka et al., 2008; Aka and Yokoyama, 2013; Fantong et al., 2015; Tanyileke et al., 2019);
(9) the topic of numerous projects (e.g. NyMo degassing, France-Cameroon; SATREPS, Japan-Cameroon), studies and review papers (Aka, 2015; Kling et al., 2015; Kusakabe, 2015, 2017; Tanyileke et al., 2019).
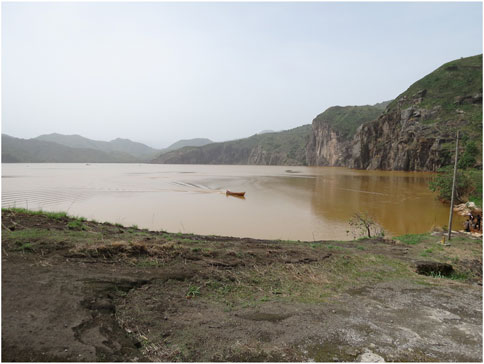
FIGURE 3. Panoramic view of Lake Nyos, Cameroon, showing the system of the three artificial degassing pipes near the horizon (picture by DR).
Besides Lake Nyos and Lake Monoun, the Oku Volcanic Field in NW Cameroon (Figure 1C) hosts 13 other maar lakes (Table 1). Lake Wum (Kusakabe et al., 1989), near the homonymous city (>80,000 inhabitants), and Lake Bambuluwe (Freeth, 1990) were subjected to a vertical sampling soon after the Lake Nyos gas burst, and resulted gas-free and not-heated from below. The remaining 12 lakes of the Oku Volcanic Field (i.e. Baleng, Banefo, Bambili, Benakuma, Elum – Figure 4A, Enep – Figure 4B, Mfouet, Nchout, Nyi, Negop Baghang, Oku – Figure 4C, and Kuk) are less studied (Table 1; Issa et al., 2014).
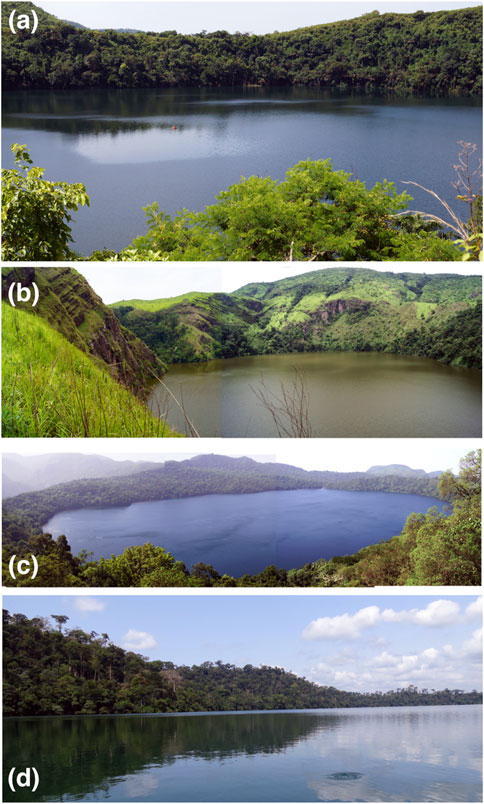
FIGURE 4. Views of the Cameroonian maar lakes (A) Elum, (B) Enep, (C) Oku and (D) Barombi Mbo (pictures (A–C) by Issa, picture (D) by DR).
During the 9th Workshop of the IAVCEI Commission on Volcanic Lakes (March 2016), Barombi Mbo (Maley et al., 1990; Kling et al., 1991; Tchamabe et al., 2013; Figure 4D), the maar lake with the largest surface area in Cameroon (Table 1), was subjected to a pioneering physical limnological and fluid geochemical survey. Within the philosophy of the “Nyos bias,” lowering CTD probes and water and dissolved gas sampling along the vertical profile of the 110 m deep lake, revealed that the gas stored in deep lake strata is far from reaching near-threshold pressures to cause a limnic gas burst. This “non-result” does have strong implications on the hazard assessment for the inhabited shores of Barombi Mbo, and the nearby city of Kumba (>125,000 inhabitants). The three remaining maar lakes in the Tombel Graben (Dissoni, the only 5 m deep Barombi Koto and Mboandang) are less studied (Kling, 1988; Issa et al., 2014).
Mt Manengouba, a 2,411 m high shield volcano overlain by a stratovolcano located in the Northern sector of the Tombel Graben, is probably the second most famous volcano in Cameroon after the active Mt Cameroon (Pouclet et al., 2014). The 3 km wide caldera hosts two “twin crater lakes,” Manengouba Male and Manengouba Female (Figure 5). The dark colored Manengouba Female is the second deepest volcanic lake in Cameroon (168 m), after Lake Nyos, while Manengouba Male is 90 m deep and green colored inside a steep-walled crater basin. Both lakes are inactive and fed by meteoric water, hence the color difference is explained by different microbial activity (Issa et al., 2014) reported, however, a weak degassing activity of Mt Manengouba, stressing the need for a monitoring setup for these summit crater lakes.

FIGURE 5. The Manengouba Female (left – black) and Manengouba Male (right – green) crater lakes of Mt Manengouba (Cameroon; picture by P. Hernández).
The Debunscha lakes (big and small, 7.27 × 104 m2 and 3.51 × 103 m2, respectively) are small maars located a few hundred meters from the Atlantic Ocean at the foot of Mt Cameroon. The massive amounts of rainfall in the coastal area (12,000 mm of rain/year; Issa et al., 2014) and strong winds change the isotopic composition of the lake water, despite being in mass balance equilibrium with the meteoric rain input (13.5 m deep, Debunscha big). Their nearness to the ocean (<50 m), and at the center of the most active sector of the Cameroon Volcanic Line (CVL hereafter; Aka et al., 2001, 2004) between Mt Cameroon and the island of Bioko, suggests that the Debunscha maar lakes were formed by phreatomagmatic eruptions from satellite vents of Mt Cameroon interacting with seawater inside the coastal aquifers (Ngwa et al., 2010, 2017).
To the Northeastern extent of the CVL seven maar lakes are located near the city of Ngaounderé (>260,000 inhabitants in 2005) in the Volcanic Plateau of Adamawa (Aka et al., 2018; and references therein). Kling (1988) put them on the map by studying their basic physical limnological characteristics, while recently Issa et al., 2014 tackled them for their stable isotopic composition of the water. Given its unique location between the Sahel and the sub-equatorial rainforest, paleoclimate during the Pleistocene-Holocene was reconstructed in recent studies (Ngos and Giresse, 2012; N’nanga et al., 2019), based on the geochemistry and mineralogy of sediments from Lake Fonjak, Mbalang and Tizong. Issa et al. (this topic collection) demonstrated a high similarity to Lakes Nyos and Monoun, and suggest that these seven lakes should become a priority in future monitoring efforts.
D.R. Congo
Lac Vert, less than 2 km north of the western sector of the Lake Kivu main basin (Figures 1A,B), fills up the central sector (695–385 m) of three nested craters, interpreted as a maar complex. Lake Kivu, a major rift lake of the Western branch of the East African Rift (EAR hereafter), creates an ideal hydrological and tectonic setting to create phreatomagmatic volcanism near its shores. Poppe et al. (2016) emphasized the importance of phreatomagmatic volcanism from a hazard point of view in the area between Lake Kivu and the famous, active volcanoes of the Virunga Volcanic Province: Nyamulagira and Nyiragongo. Moreover, Lac Vert is situated northeast of the Kabuno Basin, the northwestern sub-basin of Lake Kivu that is almost cut off entirely from Lake Kivu. The Kabuno Basin area is characterized by more magmatic gas signatures (3He/4He ratios of 5.4 Ra), and the inland degassing features tend to have similar degassing sources as Nyiragongo (3He/4He ratios of 8.7 Ra): Sake (west of Lac Vert, 3He/4He ratios of 7.7 Ra) and the CO2-rich degassing areas (Mazukos, 3He/4He ratios of 8.4 Ra; Tedesco et al., 2010; Vaselli et al., 2015) towards Lac Vert. The depth of Lac Vert is unknown; CTD measurements and water and gas sampling along the vertical profiles still lack.
A small lake partially occupies the Kirunga tuff ring, less than 100 m from the Lake Kivu shore. Kirunga is one of the 15 eruptive centers recognized along the northern shore of Lake Kivu, here occasionally filled by a lake (Poppe et al., 2016). Besides the direct gas hazard that could originate from the lakes (Lac Vert), the occurrence of renewed phreatomagmatic volcanism due to effective magma-water interaction with Lake Kivu is not excluded in the future for the densely inhabited Goma area, home to at least 750.000 people (note: at the moment of writing, the crisis of Nyiragongo volcano is ongoing, and includes this proposed scenario as one of the related hazardous outcomes).
Ethiopia
The maar lakes in the Bishoftu Volcanic Field in Central Ethiopia (Figure 1A) have been studied during the early 1960s for their physical limnology (Wood et al., 1976). They were recognised to turn over in winter (December), and do not show a stable stratification. A recent biolimnological study by Lemma (2009) on Lake Hora (35 m deep) and Lake Bishoftu Guda (55 m deep) show a seasonal decrease in temperature from 22–23°C at the surface to 20–21°C at depth. Anoxic environments set in below 5–10 m depth. These physical characteristics demonstrate that the “Bishoftu lakes” are unstably stratified, solar heated and highly sensitive to seasonal, and even diurnal variations. From the hazard point of view, this implies that these lakes are inefficient in storing CO2 in their bottom waters, regardless of the fact that this recharge effectively occurs, which does not seem to be the case. The city of Debre Zenit (approx. 100,000 inhabitants) surrounding the lakes is hence not exposed to possible Nyos-type hazards.
The lakes in the Butajiri Silti and Bilate River Fields are unreported in the scientific literature (level of study 5). A geodynamic model for the Butajiri Silti Field was proposed by Hunt et al. (2020), however, they did not enter in detail on the existence of volcanic lakes in the area (e.g. Ara Shetan, Table 1).
Mt Dendi is an inactive volcano peaking at 3,260 m a.s.l. with a 4 km-wide caldera like crater. Inside this crater, a peculiar dumbbell-shaped lake filled with meteoric water is hosted. Still, in the Central highlands of Ethiopia, 13.5 km Southwest of Mt Dendi a similarly shaped volcano, Mt Wonchi (3,450 m a.s.l.) houses an irregular-shaped crater lake that fills part of the 4.5 km wide summit caldera, also host to hot springs. Nevertheless, both large edifice volcanoes are inactive, and hence their crater lakes do not seem to be a topic of interest regarding natural hazard assessment. The extinct Mt Zuqualla volcano, 40 km south of Ethiopia’s capital Addis Abeba, hosts Dembel crater lake.
The strongly rift-related soda Lakes Shala, Langano, Chitu and Abijatta are arguably maar lakes (Le Turdu et al., 1999). The maar Lake Shala is approximately 87 m deep (max depth 266 m) and has a pH ranging from 9.8 to 10.2, depending on the season. No clear stratification appears, coinciding with the fact that “soda lakes” are often sensitive to external changes (Osato et al., 2016). Lake Zengena appears to be a small solitary maar lake (approx. 450 m diameter) towards the Lake Tana wetlands area in Northwestern Ethiopia.
Kenya
Of the 33 volcanic lakes in Kenya (Figure 1A), 27 are located on the flanks of the inactive (eruptive period between 3.1 and 2.6 Ma) large-edifice stratovolcano, Mt Kenya (5,199 m a.s.l.), the second highest mountain in Africa, after Kilimanjaro, in Tanzania. The volcano does not show a clear summit crater, and all lakes appear to be distributed in satellite vents and craters, or filled-up glacial depressions on the volcano flank (Loomis et al., 2012). No data are available on the chemical and physical properties of these lakes. Hydrothermal activity is not reported for Mt Kenya.
Three peculiar maar lakes (Tilapia lakes) are formed on the Central Island of Lake Turkana, a major rift lake in the EAR. These lakes are unstudied, but their setting and appearance suggest that they are formed by phreatomagmatic eruptions beneath Lake Turkana. Another unnamed maar-type lake is found near the southern shores of Lake Turkana.
The shield volcano Marsabit (1,707 m a.s.l.), towards Northern Kenya, is dotted by 22 maars, three of which are recognized to contain lakes. No further information is available on these maar lakes.
“Crater Lake” just East of the large freshwater (non-volcanic) Lake Naivasha fills in a third of a maar. Lake Naivasha is a large (150 km2), shallow (17 m) basin fed by extended aquifers (wetland) (Yihdego and Becht, 2013; Fazi et al., 2018), that arguably provided an aquifer through which phreatomagmatic eruptions breached, giving origin to the “Crater Lake,” or Lake Sonachi. The temperature and alkalinity increase with depth (max 7 m) at Lake Sonachi, which suggests sublacustrine input of alkaline springs. Despite its shallow depth, Lake Sonachi is permanently stratified (meromixis) at depth due to the high content of organic carbon, but mixes its upper water layers even in day-night cycles. The high pH (9.5) limits CO2 dissolution in bottom waters, where instead a CH4 environment reigns (Venturi et al., 2019). Lake Sonachi is an exotic, small “bio-activity” crater lake (Cabassi et al., 2014; Rouwet, 2021).
Libya
Miocene to recent intracontinental volcanic fields compose distinct landforms in Libya’s Sahara region. In these volcanic fields, monogenetic volcanic bodies, such as scoria and spatter cones, are the most dominant volcano types; however, broad craters with low rims are commonly interpreted as maar volcanoes in spite that most of them are dry, or host only shallow playa lakes. In Al Haruj al Abyad broad maar-like depressions are common (Németh, 2004; Martin and Németh, 2006; Cvetkovic et al., 2010; Bardintzeff et al., 2012).
The most recently active volcano in Southern Libya, Waw an Namus (Figure 1A), figures as a black dot of tephra in the Quaternary yellow sediments of the Sahara Desert (Bardintzeff et al., 2012). The volcano, a broad volcanic depression, is thought to be a satellite vent of the Al Haruj Volcanic Province to the North (Bardintzeff et al., 2012; Elshaafi and Gudmundsson, 2016, 107, 2018). Inside the 4 km-wide depression, inferred to be a maar volcano, a 150 m cone rises up to 547 m a.s.l. Three shallow, warm and saline lakes flank the base of the tephra cone. Although apparently young sulfur and (probably) alunite deposits are reported (Bardintzeff et al., 2012), there is no further mention of hydrothermal activity. We interpret the salinity and temperature of the three Waw an Namus crater (or intracaldera) lakes as resulting from solar heating and consequent steady evaporation, without further implications for volcanic hazard.
Madagascar
Considering their shapes, observed from satellite images, and their geology from rare studies (Rasamimanana et al., 1998; Bardintzeff et al., 2010; Rufer et al., 2014), the volcanic lakes in Madagascar appear to be maar lakes. From North to central Madagascar, maar lakes are located in several volcanic fields: Ambre Bobaomby (six lakes), Farihy Antanavo (one single lake), Nosy Be Island (NW Madagascar, nine lakes), Tritrivakely (two lakes; Sibree, 1891; Gasse et al., 1994; Gasse and Van Campo, 1998, 2001; Williamson et al., 1998), and Itasy (10 lakes) (Figure 1A). Lake Itasy is an irregularly shaped large lake (6 × 7 km approx.), clearly composed of multiple craters, ruling out its origin as a caldera basin. Lake Itasy is surrounded by many smaller (unnamed) maars. Lake Tritriva shows that maar lakes are not necessarily circular or ellipse-shaped, as recently conveyed by Graettinger (2018), pointing to a multiple-vent origin and/or erosional enlargement due to landslides along the inner crater wall. To the best of our knowledge, no data are available on water chemistry, or physical limnological characteristics of the maar lakes in Madagascar.
Rwanda
In the shadows of the infamous volcanoes Nyiragongo and Nyamulagira, Mt Bisoke stratovolcano (3,711 m a.s.l.) straddles the border between Rwanda and D.R. Congo (Barette et al., 2017) (Figures 1A,B). The summit contains a circular shaped crater lake (Figure 6), with a diameter of approximately 300 m. The lake was sampled in November 2013 and analyzed for its water chemistry (by SC and Giovanni Bruno Giuffrida), whereas its water temperature varied from 10° to 15°C, with a measured pH of 6.27. The chemistry did not show any evidence of hydrothermal activity, reflected by very low SO4 and Cl contents (<3 and <1 mg L-1, respectively), although a local guide reports on vague memories of the smell of sulfur close to the water surface. Despite this limited data set, we argue that Mt Bisoke is in a state of quiescence after it last erupted in 1957. If the volcano reawakens in the future, the physical and chemical properties of the crater lake are suspected to show variations, possibly detectable by satellite imagery (e.g. lake disappearance upon evaporation, color changes).
The tiny crater lake (27–28 m) at the >4,100 Muhavura stratovolcano in the Virunga Volcanic Province, at the border between Rwanda and Uganda, has well preserved its pollen-record for the Holocene (McGlynn et al., 2013), but does not show evidence of volcanic activity.
Sudan
The 3,042 m high Deriba Caldera is the most spectacular feature of the Jebel Marra Volcanic Field in the Darfur region of Western Sudan (Burton and Wickers, 1966; Hammerton, 1968; Vail, 1972; Davidson and Wilson, 1989; Franz et al., 1997) (Figure 1A). Inside the 5 km-wide steep-walled caldera, formed 3,500 yr B.P. during voluminous pumice fall eruptions, a 700 × 1000 m-wide crater lake fills a horseshoe-shaped crater. A second lake is formed to the Northeast, at the lower rim of the caldera, probably as an affluent basin of rain falling inside the caldera capture area. To the best of our knowledge, no limnological or geochemical information is available for the two crater lakes. Nevertheless, it is worth noting that fumarolic activity has been observed inside the caldera in relatively recent times (Burton and Wickers, 1966).
One of the approximately 700 vents of the Pliocene to Holocene Meidob Volcanic field in Western Sudan, 150 km Northeast of the Deriba Caldera (Figure 1A), hosts a 180 m-diameter maar lake, without manifestation of any activity. The small monogenetic Bayuda Volcanic Field (BVF; 480 km2) about 700 km NE from the Meidob Volcanic Field, comprises at least 53 cinder cones and 15 maar volcanoes in the Bayuda desert of northern Sudan of Quaternary age. The largest maar (800 m in diameter and 386 m deep) in the area is the Hosh Ea Dalam (32°35′38,32″E, 18°24′29,07″N). None of the 15 maars has a permanent lake, and are only shallow “salt pans” (Almond et al., 1969; Lenhardt et al., 2018).
Tanzania
Two renowned volcanic lakes are located in the Ngorongoro Crater Highlands in Northern Tanzania (Figure 1A): Lake Empakaai (or Lake Emakat, Figure 7) and Lake Magadi. Empakaai crater lake is 79 m-deep and well studied for its palynology and paleoclimate record from water and sediment cores (Muzuka et al., 2004; Ryner et al., 2006). The lake water chemistry reflects a high salinity (Total Dissolved Solid contents between 12,000 and 14,000 mg L-1), with Na and K as main cationic solutes (500–600 mg L-1 and 400–500 mg L-1, respectively; Muzuka et al., 2004). This composition probably originates from water-rock interaction with trachytic and phonolitic magmas (Fontijn et al., 2012), consequently enriched by enhanced evaporation by solar heating. No clear evidence of a hydrothermal system exists to hypothesize alternatively.
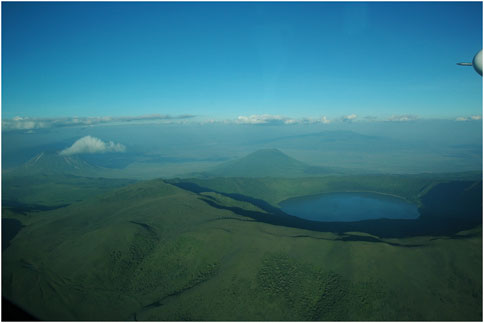
FIGURE 7. Lake Empakaai (seen from the southwest), Northern Tanzania (picture by B. Fontaine). Volcanoes in the back are Oldoinyo Lengai (left) and Kerimasi (right).
Northeast of Lake Empakaai (Figures 1A, 7), passing the famous natro-carbonatitic volcano Oldoinyo Lengai (Keller and Zaitsev, 2012), Lake Natron fills the rift depression towards the border with Kenya. Lake Natron is not a volcanic lake, but rather a “rift lake in a volcanic environment,” and we hence choose to not include it in VOLADA_Africa 2.0 despite its particular chemistry resulting from the water-rock interaction with carbonatitic country rocks: Na-HCO3-CO3 with a pH as high as 9.5 (seePecoraino et al., 2015, for a review,; Fazi et al., 2018). Lake Magadi, Southeast of Lake Empakaai, partially fills the almost 20 km-wide Ngorongoro crater and hosts a saline brine, similar to Lake Empakaai. The exotic water chemistry of the three lakes (Empakaai, Magadi and Natron) hence results from solute enrichment by evaporation from an endorheic basin after water-rock interaction with the exceptional host rocks of the Ngorongoro area.
Lake Chala is located on the lower Eastern flanks of Mt Kilimanjaro, Africa’s highest peak, at an elevation of 880 m a.s.l., and is shared with Kenya (Figure 1A). We here classify Lake Chala as a caldera lake. The lake is 94 m deep and has a nearly triangular shape with a main diameter of approx. 2 km. Lake Chala has been well studied in recent years in terms of palynology and limnology (Barker et al., 2013; Buckles et al., 2014, 2016; van Bree et al., 2018), although volcanology-focussed information lacks. The stratification of the lake is highly sensitive to seasonal variations (e.g. multiple rainy “seasons” per year; van Bree et al., 2018), which makes us suspect that long-term accumulation of gases in bottom waters is strongly inhibited–if gas input occurs in the first place.
The maar lakes in Southern Tanzania (Lake Masoko and five others in the Rungwe Volcanic Province; Fontijn et al., 2012), near the northern shores of Lake Malawi, are relatively well studied for their hydrology, (paleo)limnology, isotopy and palynology (Williamson et al., 1999; Barker et al., 2000; Delalande et al., 2008). The small maar lakes are sensitive to climate changes and hence ideal to reconstruct paleoclimate in the region, and to store ash records of past volcanic eruptions in the area (Fontijn et al., 2012). As the lakes in Northern Tanzania, the six “Mbaka lakes” are saline; suspicion exists on a possible hydrothermal input from beneath these lakes, which invites to future fluid geochemical surveys and hazard analyses for the area.
Lake Ngozi (83 m deep) tops one of the three major volcanoes, the 2,620 m-high homonymous volcano, in the Northwestern part of the Rungwe Volcanic Province (Fontijn et al., 2012) (Figure 1A). The irregular shape of the 3 km-wide collapse caldera and its crater lake is in agreement with the presumed young age of the crater and the latest magmatic eruption (<1 ka; Fontijn et al., 2010). A pioneering study on the water chemistry of Lake Ngozi (Delalande-Le Mouëllic et al., 2015) hypothesizes that the lake is affected by geothermal input (Na-Cl-rich, Mg-poor) and hydrothermal activity (pH 6.4–6.9, SO4-rich steam heated waters), similar to the caldera lakes in El Salvador (Cabassi et al., 2019; Rouwet, 2021). Dominant CO2 degassing (δ13C-DIC from +2.8 to +5.8‰, Delalande-Le Mouëllic et al., 2015) is probably of magmatic origin as also suggested by the high 3He/4He ratios (7–8.3 Ra, de Moor et al., 2013). The absence of any thermal and chemical stratification and the high pCO2 along the water column results in diffuse CO2 degassing at the lake surface (Jolie, 2019). This CO2 degassing is structurally controlled, along E-W trending faults at the southern parts of the caldera lake, near the presumably last eruptive vent (Fontijn et al., 2010). Funnel-shaped depressions along this fault reach lake bottom temperatures up to 89°C (i.e. near boiling at the elevation of the lake, 2,060 m a.s.l.) (Jolie, 2019). These findings suggest that Lake Ngozi is topping an active volcano that could escalate into unrest in the future. A similar, though more vigorous CO2 degassing dynamics was observed at Kelud crater lake, Indonesia, prior to the 2007 dome extrusion eruption (Caudron et al., 2012).
Moreover, we can mention that there are many maar-like dry craters in Tanzania. The genetic origin of these maars is currently under debate, as they arguably seem to be inexplicable by the general phreatomagmatic model (Mattson and Tripoli, 2011; Berghuijs and Mattson, 2013). This alternative model would imply that they could have been water filled during wet periods. Dry maars are, however, a recurrent feature in monogenetic volcanic fields, in Africa and elsewhere.
Uganda
The 81 volcanic lakes in Uganda are distributed in four volcanic fields: the Bunyaruguru (30 lakes) and Katwe-Kikorongo (eight lakes) Volcanic Fields in the South, near the EAR rift lakes George and Edward, and Ndale-Kasenda (36 lakes) and Fort Portal (seven lakes) Volcanic Fields in the North (Rumes et al., 2005; Stoppa and Schiazza, 2013) (Figures 1A,B). All the 81 lakes are classified as maar lakes (Melack, 1978). An early study by Mungoma (1990) revealed that the lake water chemistry of eight lakes in the Katwe-Kikorongo (Kikorongo, Nyamunyuka, Katwe, Bunyampaka and Kitagata; Lowenstein and Russell, 2011) and Bunyaruguru (Maseshe, Bagusa, Mahega) are highly saline (Conductivity 16.3 to 455 mS cm-1) and alkaline (pH 9–10.5), caused by water-rock interaction and enhanced evaporation of the generally small and shallow maar lakes. Solar heating results in mesothermal stratification of the studied lakes, shown by inverted vertical temperature profiles. Three water types are distinguished: carbonate-chloride- and chloride-type lakes in the Katwe-Kikorongo, and carbonate-sulfate-type lakes in Bunyaruguru.
In their turn, the deeper lakes in Uganda (e.g. Lake Kyaninga, 220 m deep, the largest lake in the Fort Portal Volcanic Field) seem to be geothermally heated, as shown by heated bottom waters. Lake Kyaninga is arguably meromictic (i.e. permanently stratified) below 100 m depth (Cocquyt et al., 2010). The particular chemistry, with high Cl, HCO3 and SO4 concentrations (up to 149, 108 and 64 mg L-1, respectively), rises curiosity on generic processes behind their formation and the need for renewed hypotheses and conceptual models, regarding natural hazard assessment. A recent study by De Crop and Verschuren (2019) on 11 maar lakes in Uganda stressed that high-frequency monitoring of physical-chemical parameters along water columns in tropical lakes is a must to better understand water mixing at various time scales (days to decades). The limnological control on water mixing can have implications on hazard, especially when gas or heat enters lake bottoms.
African Minor Islands
Peculiarly, some of the minor islands around the African continent host volcanic lakes. The islands of Bioko and Annobon (Equitorial Guinea) are shield volcanoes along the ocean-ward side of the CVL (Aka et al., 2001, 2004) (Figures 1A,C), topped by crater lakes Moca and Pot, respectively. Bioko, together with Mt Cameroon, is considered the most active centre of the CVL (Aka et al., 2004).
In the summit crater of Queen’s Mary Peak (2,060 m a.s.l.), on the remote island of Tristan da Cunha (a British Overseas Territory, South Atlantic) (Figure 1A), a heart-shaped shallow lake filled with meteoric water is present (Figure 8A), whereas three aligned maar lakes (Figure 8B) –called “the ponds” by the locals (1, 2, 3 in Figure 8C)– are found on the Northeastern lower flank of the stratovolcano. The peculiar setting might teach us that maar volcanoes can form on top of composite polygenetic volcanoes, if the environment (e.g. ground-water and/or surface water availability) is favorable to produce explosive magma-water interaction (Kereszturi et al., 2011, 2014; Smith and Németh, 2017; Geshi et al., 2019). In the case of Tristan da Cunha, the maar lakes could have been formed by magma interacting with the incursion of marine water into the near-coastal aquifer (Németh and Cronin, 2009, 2011). The last eruptive activity that occurred in 1961–1962 did not originate from the summit crater, but on a coastal plateau near the northern shores of the island (Baker et al., 1964).
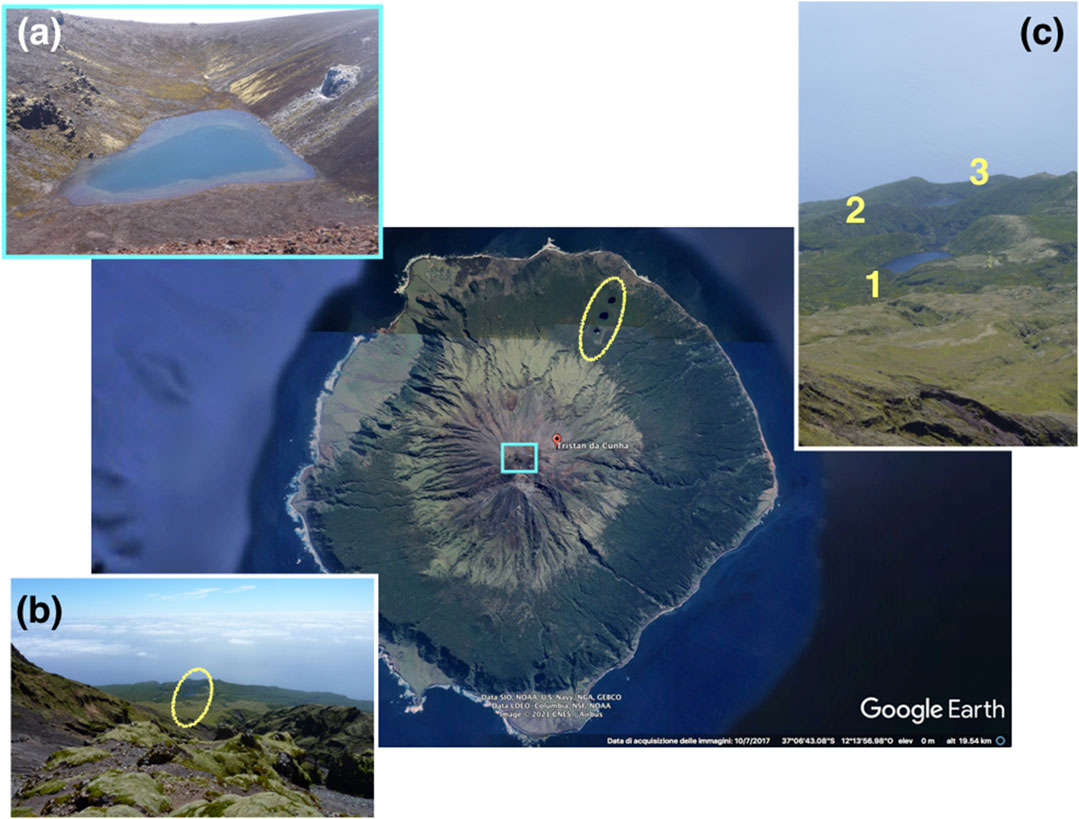
FIGURE 8. (A) The heart-shaped crater lake of the St. Mary’s Peak of Tristan da Cunha, Southern Atlantic Ocean (turquoise square in inset map); (B) location of the aligned maars at northern flank of Tristan da Cunha island (yellow ellipse in inset map); (C) the three maar lakes, called “the ponds.” Note: the yellow patches to the right of “pond 1” are lichens, not sulphur remnants of extinct fumaroles (pictures by A. Hicks).
Southeast of Africa, in the Comores Archipelago, the crater of the Karthala shield volcano was filled by a green lake prior to the 2005 eruption (Pons, 2006) (Figure 1A). The lake is ephemeral as the open-conduit volcano is often a heat emitter too high for the lake to sustain. The Dziani Boundouni lake on Mohéli Island (Comores, Figure 1A) is a maar lake with, arguably, at least two nested craters. Lake Dziani Dzaha, a shallow (5 m deep) maar lake on a minor island east of Mayotte (Comores, Figure 1A) is located only 240 m from the Indian Ocean, and its chemistry is hence affected by seawater. The lake is studied for its microbialites (i.e. sediment aggregates formed by microbial activity) and microbial productivity (Fouilland et al., 2014; Dupuy et al., 2016). Volcanic hazard assessment remains an untouched topic, despite the manifestations of minor CO2 degassing, probably of volcanic origin (https://www.youtube.com/watch?v=XsgoWl728hM).
All nine “off-shore” volcanic lakes in Africa are poorly studied, and probably mainly fed by meteoric water.
Morphometric Analysis
From the 220 volcanic lakes in Africa, 172 are classified as maar lakes, based on their volcanic setting (seesection 2) and morphological aspects (Table 1). Whereas approximately 54% of the maars (i.e. craters) in the MaarVLS database by Graettinger (2018) are located at elevations below 750 m a.s.l., about 86% of the African volcanic lakes are located above 800 m a.s.l., despite being predominantly maar lakes (Figure 9A). In fact, the maar lakes in Kenya, Tanzania and Uganda are located in volcanic fields in the highlands created by the horst-graben structure of the EAR, in Cameroon in the CVL, and in the central highlands of Madagascar. Crater lakes on the stratovolcanoes Dendi, Wonchi and Zuquala in Ethiopia, Mt Kenya in Kenya and Bisoke and Muhavura in Rwanda are hosted in craters or glacial depressions above 2,800 m a.s.l. Lakes at low elevations (0–300 m a.s.l.) are found on the islands (Nosy Be Island in Madagascar and off-continent islands), near the Atlantic Ocean in Cameroon, or in the Sahara Desert in Libya. Excluding the four caldera lakes and one composite maar (Lake Itasy, Madagascar), the distribution of the maximum diameter of African volcanic lakes peak between 400 and 800 m, similar as for maar lakes worldwide (Graettinger, 2018) (Figure 9B). In conclusion, the African volcanic lakes are hence merely maar lakes, but are clearly located at higher elevations, if compared with maars worldwide (Graettinger, 2018).
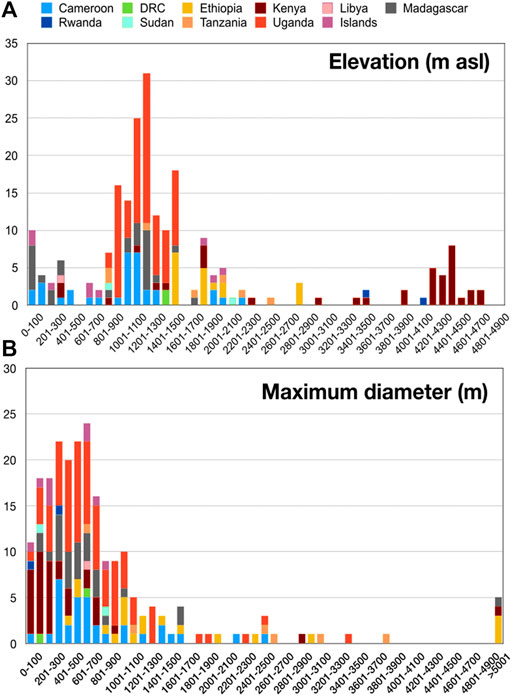
FIGURE 9. Histograms of parameters for the 220 African volcanic lakes: (A) elevation (in m a.s.l.), and (B) maximum diameter (in m).
The average Aspect Ratio (AR, i.e. a measure of the distance from the centre of the lake for minor and major axis) for the African volcanic lakes of 0.69 (±0.18 STDEV) is lower than for maar craters worldwide (0.81, Graettinger, 2018), also reflected in Figure 10A as a more smeared out distribution towards lower AR values. The AR peaks at 0.82–0.86, with a secondary peak at 0.74–0.80 (Figure 10A). Only 4% of the AR ratios are above 0.96, whereas 17% are below 0.5. The irregularly shaped Lake Monoun has the lowest AR (0.17), despite being a maar lake. Its river flow-through nature can explain its irregular shape, stressing a dynamic sedimentary regime for Lake Monoun. Indeed, Graettinger, 2018 suggested that maars with anomalous shapes should become a focus for future research to better understand the interaction between local hydrology and its volcanic creation; multiple overlapping maar craters corroborate polygenetic maar formations.
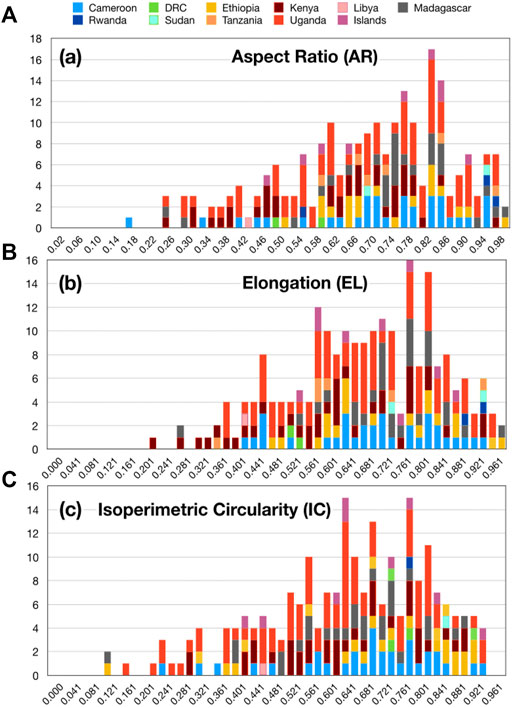
FIGURE 10. Histograms of morphometric parameters (from Graettinger, 2018) for the 220 African volcanic lakes: (A) Aspect Ratio (AR), (B) Elongation (EL), and (C) Isoperimetric circularity (IC).
The Elongation (EL, i.e. a measure of the asymmetry of the lake shapes) of African volcanic lakes averages 0.69 (±0.16 STDEV), again lower than the EL values for maar craters (0.80 ± 0.12; Graettinger, 2018). 93% of EL values are below 0.92 (versus 85% for maar craters); 16% are below 0.5 (versus 5% for maar craters; Graettinger, 2018) (Table 1). EL peaks at 0.76–0.82 (Figure 10B). Although the lakes on the flanks of Mt Kenya weigh in this distribution, caldera and crater lakes do not deviate more from El = 1 than maar lakes do, hence suggesting that maar lakes in Africa appear more elongated with respect to maar craters worldwide. This observation can be explained by a structural control on the morphology of maar lakes and volcanism of the East African Rift system (e.g. Hunt et al., 2020), or more in general, that the maars are located in an extensional rift axis where hydrogeology also plays a role as they are low, longitudinal valleys.
Figure 10C represents a similar distribution of Isoperimetric Circularity (IC, i.e. a measure of the circularity of the lake shape) as for AR and EL. The average IC for African volcanic lakes is 0.66 (±0.18 STDEV), clearly lower than for maar craters worldwide (0.9 ± 0.08; Graettinger, 2018). IC values of 0.62–0.64 and 0.76–0.78 are the most common (Figure 10C). Contrary to the statistical distribution for maar craters, only 6% of volcanic lakes in Africa have an IC value above 0.9 (versus 65% for maar craters worldwide), whereas 76% have an IC value below 0.8 (versus 9% for maar craters worldwide; Graettinger, 2018). For maar craters, IC values below 0.9 were explained to reflect a compound shape. Caution is needed to avoid over interpretation of the IC trends for African volcanic lakes, in the light of Graettinger, 2018 correct hypothesis for maar craters: 1) lakes do not necessarily fill up entire circular-shaped craters, but can present with a more exotic, less circular morphology, and 2) islands and peninsula in the larger lakes, considered in the calculation of A and p (Eq. 3) of the volcanic lakes, can drastically decrease the IC values. Nevertheless, the lower IC values resulting from (2) can be an additional argument in favor of polygenetic phreatomagmatic volcanism leading to irregular craters and lakes.
Conceptual Model: why Are Maar Lakes so Dominant in Africa?
Besides Lakes Nyos and Monoun, none of the African volcanic lakes are (well) studied regarding volcanic hazard assessment. Pioneering work on paleo-, bio- and physical limnology and hydroclimatology for lakes in Cameroon, Uganda, Kenya, Tanzania and Ethiopia (Kling, 1988; Eggermont et al., 2006; Kebede et al., 2009; Lemma, 2009; Russell et al., 2009; Giresse and Makaya-Mvoubou, 2010; Ryves et al., 2011; Garcin et al., 2012; Loomis et al., 2012; Garcin et al., 2014; Lebamba et al., 2016; De Crop and Verschuren, 2019) should guide volcanologists to shed light on the many poorly studied lakes in Africa (predominantly quoted “5” in VOLADA_Africa 2.0, Table 1) with the scope to better assess future natural hazards.
Besides the 26 glacial volcanic lakes of Mt Kenya (Loomis et al., 2012), about 89% (172) of volcanic lakes reported for Africa are maar lakes (Table 1). Africa does not host any peak activity lakes (i.e. acidic crater lakes overlying degassing magmatic-hydrothermal systems, often subjected to phreatic and phreatomagmatic eruptions; Rouwet, 2021), peculiar for such a large continent with active volcanoes (Global Volcanism Program, 2013). However, the continent does not manifest “classical” arc-type volcanism, but evidently hosts intraplate polygenetic volcanoes with a different chemical affinity than arc volcanoes. Hydrothermal activity is abundant, but is mainly related to the gradual break-up of the thick continental crust (e.g. EAR, Figure 11), and to mafic magmas. Stratovolcanoes or (dome) complex volcanoes with well-developed hydrothermal systems that provide the prototype settings to develop crater lakes on volcano summits (wet volcanoes; Caudron et al., 2015; Rouwet et al., 2015b), are relatively scarce in Africa. The EAR volcanism is MORB- or OIB-type leading to predominantly basaltic (Mt Cameroon; Mbassa et al., 2012; Kervyn et al., 2014; Adams et al., 2015; Nyamulagira, Nyiragongo; Pouclet et al., 2016; Karthala, outside the EAR; Class et al., 2009; Pelleter et al., 2014), or sporadically carbonatitic volcanism (Oldoinyo Lengai; Kervyn et al., 2014; Keller and Zaitsev, 2012; Weidendorfer et al., 2017). Despite the tropical climate and abundant rainfall in subsaharan Africa, insufficient constraints are met to sustain crater lake presence at the active volcanoes (Pasternack and Varekamp, 1997), for example: 1) a too high heat flux from open-conduit volcanoes (i.e., lava lakes instead of “water lakes” in most extreme cases, e.g. Nyiragonogo, Nyamulagira, and Erta Ale; Giggenbach and Le Guern, 1976), and 2) a too high permeability of the volcanic edifice, flushing out meteoric water from the summit areas of volcanoes. Instead, aquifers arguably accumulate at the base of volcanoes, or in rift depressions, hence creating an ideal hydrogeological architecture for magma-water interaction to take place at lower elevations (Figure 11), resulting in phreatomagmatic eruptions and consequent maar formation, a common phenomenon observed in monogenetic volcanic fields (e.g. Cas et al., 2017; Kereszturi et al., 2017). The many maar fields in Africa (e.g. Uganda, in the EAR, and Cameroon, in the CVL) probably reflect this large-scale and tectonically driven process. In fact, active seismicity occurs along the EAR (International Seismological Centre, 2020), as well as Holocene active volcanism does (Global Volcanism Program, 2013) (Figures 1, 11). Unsurprisingly, seismicity, volcanism and volcanic lakes occur where the Earth crusts thins due to rifting, as shown in the two transects A-B and C-D in Figures 11A,B, respectively. The CVL is often interpreted as resulting from failed rifting following continental break up starting 120 Ma ago (Fitton, 1983; Aka et al., 2004); volcanism in Cameroon and the southern islands hence occur along this aulacogen (Figure 11A).
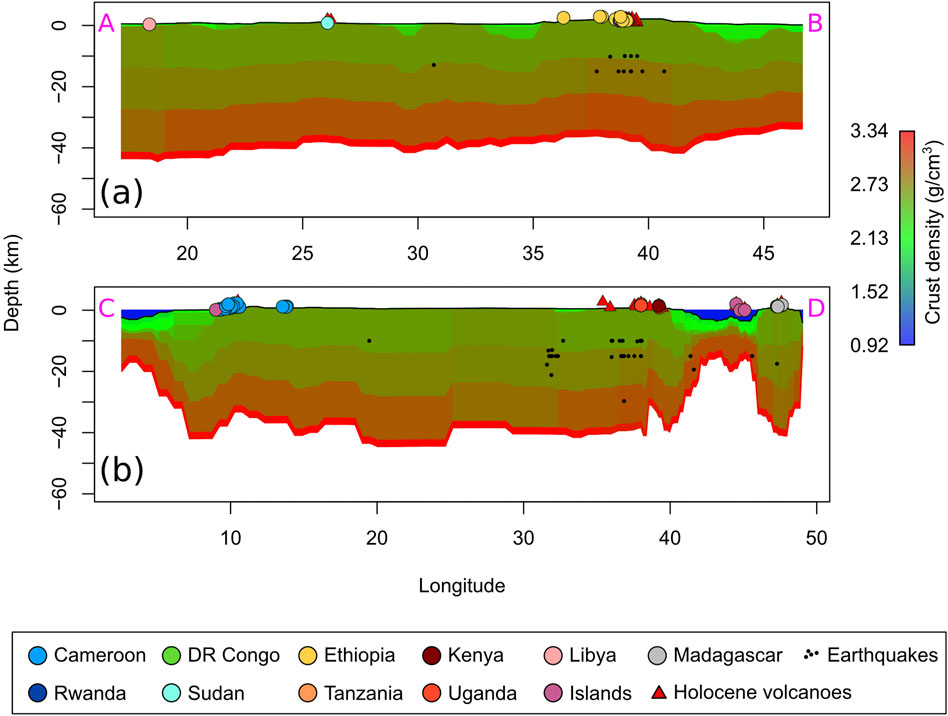
FIGURE 11. Conceptual model to explain the abundant maar volcanism of the EAR and CVL, through a vertical section of the first 40–50 km through the Earth’s crust (Laske et al., 2013). The transects A-B and C-D are traced in Figure 1. Holocene active volcanoes are exported from the Global Volcanism Program-Smithsonian database (https://volcano.si.edu/).
Within this maar-rich setting, combined with regional scale CO2-rich degassing along the EAR and CVL, the deepest lakes in Africa might be able to store dissolved CO2 in their bottom waters. The absence of a high gradient in yearly atmospheric temperature, and thus lake surface water temperature, favors meromixsy (e.g. Lake Nyos); instead, possible geothermal heating in deep rift lakes (e.g. Lake Kyaninga) inhibits a stable thermal stratification. The latter process provides a hypothesis for the observation that no other maar lake along the EAR or CVL has bursted in a fatal way like Lake Monoun and Lake Nyos did in 1984 and 1986, respectively. Moreover, possible diurnal cycles in the stratification, especially in the epilimnion of high-altitude lakes in Uganda, Kenya, Ethiopia, Tanzania and Cameroon, however, favors daily partial mixing and, hence, possible degassing if the lakes are fed by gas-rich fluids from below. Needless to say that further research in physical limnology, hydrogeology and fluid geochemistry of single lakes is highly recommendable in order to better assess future volcanic hazard for the 172 African maar lakes.
Final Remarks and Strategies for Future Research
VOLADA_Africa 2.0 compiles arguably the complete number of volcanic lakes on the African continent (220), Madagascar and the minor islands. Volcanic lakes are pin-pointed, country by country, through decimal coordinates, made available to the community (https://vhub.org/resources/). All lakes are classified for their genetic origin (Christenson et al., 2015), and physical and chemical characteristics (Rouwet et al., 2014, and references therein). The classification results as follows:
(1) 18 lakes are crater lakes (Manengouba Lakes, Cameroon; crater lakes of Zuquala, Dendi and Wonchi volcanoes, Ethiopia; Rutundu and Alice on Mt Kenya, Kenya; Waw an Namus in Libya; Mt Bisoke’s and Mt Muhavura crater lakes, Rwanda; Deriba crater lakes, Sudan; Empakaai, Magadi and Ngozi crater lakes in Tanzania; crater lakes on the islands of Annobon, Bioko, Tristan da Cunha and Karthala);
(2) 4 are caldera lakes (3 in the O’a Caldera, Ethiopia; Lake Chala in Tanzania);
(3) 26 are lakes filling glacial depressions on the flanks of Mt Kenya (Kenya);
(4) The remaining 172 volcanic lakes are maar/tuff cone lakes.
Africa does not host peak-activity or high-activity lakes in active volcano craters. The renowned “killer lakes” Nyos and Monoun are “5b2-type” lakes (i.e. gas storing Nyos-type lake); Lake Nyungu and Lake Kyaninga, two of the 81 maar lakes in Uganda, are suspected to be “Nyos-type lakes.” Arguably all lake waters have a purely meteoric origin, that attained a chemical equilibrium upon water-rock interaction with the host rock of the lake basin (i.e. rock-dominated lakes), and evaporation, in more or less extent. We proposed a conceptual model on why Africa provides the ideal tectonic and volcanic settings for maar lakes to form, and why peak activity crater lakes, “windows” into “wet volcanoes” are absent, in spite of the often ideal climatic conditions for such lakes to form. The dominance of intraplate volcanism and the absence of arc-type volcanism appear to be key factors.
The uniqueness to accomplish the necessary conditions for a gas burst to occur has been extensively studied during the past 30+ years. As we presumably know why the two fatal gas bursts did occur, the question for the remaining 172 African maar lakes seems to be “why do gas bursts not occur?” (by assuming they did not), as climatic, limnological and volcanic constraints are very similar than those for the Cameroonian “killer” lakes. Non-volcanological explanations that haze our insights into possible other “Nyos-type” events in the past lie in the facts that 1) written history is reported only since the late 19th century for most of the African continent, and 2) volcanic lakes are often located in remote and poorly inhabited regions. The recurrence time of geological phenomena is longer (e.g. 100–10,000’s of years for dyke intrusions, maar formation, and “Nyos-type” gas bursts), and hence not synchronized with written history. Nevertheless, clues on suspicious “Nyos-type” behavior could be found in the study of oral traditions and legends that trace further back in the past. Future historiographical research should hence aim at explaining if “only” two lethal gas bursts occurred (Monoun-1984, Nyos-1986), as reported in the documented history (Westerman, 2013). Moreover, the database on volcanic lakes in Africa presented here can be a useful resource to apply to other continents as a future aim of the International Association of Volcanology and Chemistry of the Earth’s Interior (IAVCEI) Commission on Volcanic Lakes.
Author Contributions
DR Development idea, MS writing, DB compilation, elaboration data KN Development idea, MS writing GT MS writing, DB compilation, elaboration data SC MS writing, field work, elaboration data I MS writing, field work.
Conflict of Interest
The authors declare that the research was conducted in the absence of any commercial or financial relationships that could be construed as a potential conflict of interest.
Publisher’s Note
All claims expressed in this article are solely those of the authors and do not necessarily represent those of their affiliated organizations, or those of the publisher, the editors and the reviewers. Any product that may be evaluated in this article, or claim that may be made by its manufacturer, is not guaranteed or endorsed by the publisher.
Acknowledgments
Research funding for DR during this research came from CALAPA-FISR 2016 (INGV, 2018). We thank Anna Hicks for providing comments on and the picture of the remote Tristan da Cunha crater lake. Pedro Hernández and Bernard Fontaine are grateful for sharing the pictures in Figures 5, 7, respectively.
References
Adams, A. N., Wiens, D. A., Nyblade, A. A., Euler, G. G., Shore, P. J., and Tibi, R. (2015). Lithospheric Instability and the Source of the Cameroon Volcanic Line: Evidence from Rayleigh Wave Phase Velocity Tomography. J. Geophys. Res. Solid Earth. 120 (3), 1708–1727. doi:10.1002/2014jb011580
Aeschbach-Hertig, W., Hofer, M., Kipfer, R., Imboden, D. M., and Wieler, R. (1999). Accumulation of Mantle Gases in a Permanently Stratified Volcanic lake (Lac Pavin, France). Geochim. Cosmochim. Acta. 63 (19/20), 3357–3372. doi:10.1016/s0016-7037(99)00257-4
Aeschbach-Hertig, W., Kipfer, R., Hofer, M., Imboden, D. M., Wieler, R., and Signer, P. (1996). Quantification of Gas Fluxes from the Subcontinental Mantle: The Example of Laacher See, a Maar lake in Germany. Geochimica et Cosmochimica Acta. 60, 31–41. doi:10.1016/0016-7037(95)00370-3
Aka, F. T., Buh, G. W., Fantong, W. Y., Issa, I. T., Zouh, S. L. B., Ghogomu, R. T., et al. (2017). Disaster Prevention, Disaster Preparedness and Local Community Resilience within the Context of Disaster Risk Management in Cameroon. Nat. Hazards. 86 (1), 57–88. doi:10.1007/s11069-016-2674-5
Aka, F. T. (2015). “Depth of Melt Segregation Below the Nyos Maar-Diatreme Volcano (Cameroon, West Africa): Major-Trace Element Evidence and Their Bearing on the Origin of CO2 in Lake Nyos,” in Volcanic Lakes. Editors D. Rouwet, B. Christenson, F. Tassi, and J. Vandemeulebrouck (Heidelberg: Springer), 467–488. doi:10.1007/978-3-642-36833-2_21
Aka, F. T., Hasegawa, T., Nche, L. A., Asaah, A. N. E., Mimba, M. E., Teitchou, I., et al. (2018). Upper Triassic Mafic Dykes of Lake Nyos, Cameroon (West Africa) I: K-Ar Age Evidence within the Context of Cameroon Line Magmatism, and the Tectonic Significance. J. Afr. Earth Sci. 141, 49–59. doi:10.1016/j.jafrearsci.2018.02.001
Aka, F. T., Kusakabe, M., Nagao, K., and Tanyileke, G. (2001). Noble Gas Isotopic Compositions and Water/gas Chemistry of soda springs from the Islands of Bioko, São Tomé and Annobon, along with Cameroon Volcanic Line, West Africa. Appl. Geochem. 16, 323–338. doi:10.1016/s0883-2927(00)00037-8
Aka, F. T., Nagao, K., Kusakabe, M., Sumino, H., Tanyileke, G., Ateba, B., et al. (2004). Symmetrical Helium Isotope Distribution on the Cameroon Volcanic Line, West Africa. Chem. Geology. 203, 205–223. doi:10.1016/j.chemgeo.2003.10.003
Aka, F. T., and Yokoyama, T. (2013). Current Status of the Debate about the Age of Lake Nyos Dam (Cameroon) and its Bearing on Potential Flood Hazards. Nat. Hazards. 65, 875–885. doi:10.1007/s11069-012-0401-4
Aka, F. T., Yokoyama, T., Kusakabe, M., Nakamura, E., Tanyileke, G., Ateba, B., et al. (2008). U-series Dating of Lake Nyos Maar Basalts, Cameroon (West Africa): Implications for Potential Hazards on the Lake Nyos Dam. J. Volcanology Geothermal Res. 176, 212–224. doi:10.1016/j.jvolgeores.2008.04.009
Alexandrine, N. n., Simon, N., and Gabriel, N. (2019). The Late Pleistocene - Holocene Paleoclimate Reconstruction in the Adamawa Plateau (Central Cameroon) Inferred from the Geochemistry and Mineralogy of the Lake Fonjak Sediments. J. Afr. Earth Sci. 150, 23–36. doi:10.1016/j.jafrearsci.2018.09.024
Almond, D. C., Ahmed, F., and Khalil, B. E. (1969). An Excursion to the Bayuda Volcanic Field of Northern Sudan. Bull. Volcanol. 33, 549–565. doi:10.1007/BF02596524
Anazawa, K., Fantong, W. Y., Ueda, A., Ozawa, A., Kusakabe, M., Yoshida, Y., et al. (2019). Environmental Modifications of Lake Nyos Surface Water by Artificial Degassing. J. Afr. Earth Sci. 152, 115–121. doi:10.1016/j.jafrearsci.2019.02.009
Anema, A., and Fesselet, J.-F. (2003). A Volcanic Issue - Lessons Learned in Goma. Waterlines. 21 (4), 9–11. doi:10.3362/0262-8104.2003.019
Asaah, A. N. E., Yokoyama, T., Aka, F. T., Usui, T., Kuritani, T., Wirmvem, M. J., et al. (2015). Geochemistry of Lavas from Maar-Bearing Volcanoes in the Oku Volcanic Group of the Cameroon Volcanic Line. Chem. Geology. 406, 55–69. doi:10.1016/j.chemgeo.2015.03.030
Baker, P. E., Gass, I. G., Harris, P. G., and le Maitre, R. W. (1964). The Volcanological Report of the Royal Society Expedition to Tristan da Cunha, 1962. Phil. Trans. R. Soc. Lond. A. 256, 439–575. doi:10.1098/rsta.1964.0011
Balashova, A., Mattsson, H. B., Hirt, A. M., and Almqvist, B. S. G. (2016). The Lake Natron Footprint Tuff (Northern Tanzania): Volcanic Source, Depositional Processes and Age Constraints from Field Relations. J. Quat. Sci. 31 (5), 526–537. doi:10.1002/jqs.2876
Barberi, F., Chelini, W., Marinelli, G., and Martini, M. (1989). The Gas Cloud of Lake Nyos (Cameroon, 1986): Results of the Italian Technical mission. J. Volcanology Geothermal Res. 39, 125–134. doi:10.1016/0377-0273(89)90053-x
Bardintzeff, J.-M., Deniel, C., Guillou, H., Platevoet, B., Télouk, P., and Oun, K. M. (2012). Miocene to Recent Alkaline Volcanism Between Al Haruj and Waw an Namous (Southern Libya). Int. J. Earth Sci. (Geol Rundsch). 101, 1047–1063. doi:10.1007/s00531-011-0708-5
Bardintzeff, J.-M., Liégeois, J.-P., Bonin, B., Bellon, H., and Rasamimanana, G. (2010). Madagascar Volcanic Provinces Linked to the Gondwana Break-Up: Geochemical and Isotopic Evidences for Contrasting Mantle Sources. Gondwana Res. 18, 295–314. doi:10.1016/j.gr.2009.11.010
Barette, F., Poppe, S., Smets, B., Benbakkar, M., and Kervyn, M. (2017). Spatial Variation of Volcanic Rock Geochemistry in the Virunga Volcanic Province: Statistical Analysis of an Integrated Database. J. Afr. Earth Sci. 134, 888–903. doi:10.1016/j.jafrearsci.2016.09.018
Barker, P. A., Hurrell, E. R., Leng, M. J., Plessen, B., Wolff, C., Conley, D. J., et al. (2013). Carbon Cycling within an East African lake Revealed by the Carbon Isotope Composition of Diatom Silica: A 25-ka Record from Lake Challa, Mt. Kilimanjaro. Quat. Sci. Rev. 66, 55–63. doi:10.1016/j.quascirev.2012.07.016
Barker, P., Telford, R., Merdaci, O., Williamson, D., Taieb, M., Vincens, A., et al. (2000). The Sensitivity of a Tanzanian Crater lake to Catastrophic Tephra Input and Four Millennia of Climate Change. The Holocene. 10 (3), 303–310. doi:10.1191/095968300672848582
Berghuijs, J. F., and Mattsson, H. B. (2013). Magma Ascent, Fragmentation and Depositional Characteristics of "Dry" Maar Volcanoes: Similarities with Vent-Facies Kimberlite Deposits. J. Volcanology Geothermal Res. 252, 53–72. doi:10.1016/j.jvolgeores.2012.11.005
Bruhn, R. L., Brown, F. H., Gathogo, P. N., and Haileab, B. (2011). Pliocene Volcano-Tectonics and Paleogeography of the Turkana Basin, Kenya and Ethiopia. J. Afric. Earth Sci. 59 (2-3), 295–312. doi:10.1016/j.jafrearsci.2010.12.002
Buckles, L. K., Verschuren, D., Weijers, J. W. H., Cocquyt, C., Blaauw, M., and Sinninghe Damsté, J. S. (2016). Interannual and (Multi-)decadal Variability in the Sedimentary BIT Index of Lake Challa, East Africa, Over the Past 2200 years: Assessment of the Precipitation Proxy. Clim. Past. 12, 1243–1262. doi:10.5194/cp-12-1243-2016
Buckles, L. K., Weijers, J. W. H., Verschuren, D., and Sinninghe Damsté, J. S. (2014). Sources of Core and Intact Branched Tetraether Membrane Lipids in the Lacustrine Environment: Anatomy of Lake Challa and its Catchment, Equatorial East Africa. Geochimica et Cosmochimica Acta. 140, 106–126. doi:10.1016/j.gca.2014.04.042
Burton, A. N., and Wickens, G. E. (1966). Jebel Marra Volcano, Sudan. Nature 210, 1146–1147. doi:10.1038/2101146a0
Cabassi, J., Capecchiacci, F., Magi, F., Vaselli, O., Tassi, F., Montalvo, F., et al. (2019). Water and Dissolved Gas Geochemistry at Coatepeque, Ilopango and Chanmico Volcanic Lakes (El Salvador, Central America). J. Volcanology Geothermal Res. 378, 1–15. doi:10.1016/j.jvolgeores.2019.04.009
Cabassi, J., Tassi, F., Mapelli, F., Borin, S., Calabrese, S., Rouwet, D., et al. (2014). Geosphere-Biosphere Interactions in Bio-Activity Volcanic Lakes: Evidences from Hule and Rìo Cuarto (Costa Rica). PLoS ONE. 9 (7), e102456. doi:10.1371/journal.pone.0102456
Cabassi, J., Tassi, F., Vaselli, O., Fiebig, J., Nocentini, M., Capecchiacci, F., et al. (2013). Biogeochemical Processes Involving Dissolved CO2 and CH4 at Albano, Averno, and Monticchio Meromictic Volcanic Lakes (Central-Southern Italy). Bull. Volcanol. 75, 683. doi:10.1007/s00445-012-0683-0
Caliro, S., Chiodini, G., Izzo, G., Minopoli, C., Signorini, A., Avino, R., et al. (2008). Geochemical and Biochemical Evidence of lake Overturn and Fish Kill at Lake Averno, Italy. J. Volcanology Geothermal Res. 178 (2), 305–316. doi:10.1016/j.jvolgeores.2008.06.023
Carapezza, M. L., Lelli, M., and Tarchini, L. (2008). Geochemistry of the Albano and Nemi Crater Lakes in the Volcanic District of Alban Hills (Rome, Italy). J. Volcanology Geothermal Res. 178, 297–304. doi:10.1016/j.jvolgeores.2008.06.031
Cas, R., van Otterloo, J. B. T., and van den Hove, J. (2017). “The Dynamics of a Very Large Intra-plate Continental Basaltic Volcanic Province, the Newer Volcanics Province, South-Eastern Australia, and Implications for Other Provinces,” in Monogenetic Volcanism. Editors K. Németh, G. Carrasco-Nuñez, J. J. Aranda-Gomez, and I. E. M. Smith (Bath, UK: The Geological Society Publishing House).
Caudron, C., Lecocq, T., Syahbana, D. K., McCausland, W., Watlet, A., Camelbeeck, T., et al. (2015). Stress and Mass Changes at a "Wet" Volcano: Example During the 2011-2012 Volcanic Unrest at Kawah Ijen Volcano (Indonesia). J. Geophys. Res. Solid Earth. 120, 5117–5134. doi:10.1002/2014JB011590
Caudron, C., Mazot, A., and Bernard, A. (2012). Carbon Dioxide Dynamics in Kelud Volcanic Lake. J. Geophys. Res. 117, B05102. doi:10.1029/2011JB008806
Chapman, L. J., Chapman, C. A., Crisman, T. L., and Nordlie, F. G. (1998). Dissolved Oxygen and Thermal Regimes of a Ugandan Crater lake. Hydrobiologia. 385, 201–211. doi:10.1023/a:1003527016384
Chiodini, G., Tassi, F., Caliro, S., Chiarabba, C., Vaselli, O., and Rouwet, D. (2012). Time-dependent CO2 Variations in Lake Albano Associated with Seismic Activity. Bull. Volcanol. 74, 861–871. doi:10.1007/s00445-011-0573-x
Christenson, B., Németh, K., Rouwet, D., Tassi, F., Vandemeulebrouck, J., and Varekamp, J. C. (2015). “Volcanic Lakes,” in Volcanic Lakes. Editors D. Rouwet, B. Christenson, F. Tassi, and J. Vandemeulebrouck (Heidelberg: Springer), 1–20. doi:10.1007/978-3-642-36833-2_1
Class, C., Goldstein, S. L., and Shirey, S. B. (2009). Osmium Isotopes in Grande Comore Lavas: A New Extreme Among a Spectrum of EM-type Mantle Endmembers. Earth Planet. Sci. Lett. 284 (1-2), 219–227. doi:10.1016/j.epsl.2009.04.031
Cocquyt, C., Plisnier, P.-D., Gelorini, V., Rumes, B., and Verschuren, D. (2010). Observations on the Limnology and Phytoplankton Community of Crater Lake Kyaninga (Uganda), with Special Attention to its Diatom flora. Plecevo. 143 (3), 365–377. doi:10.5091/plecevo.2010.420
Cvetkovic, V., Toljic, M., Ammar, N. A., Rundic, L., and Trish, K. B. (2010). Petrogenesis of the Eastern Part of the Al Haruj Basalts (Libya). J. Afric. Earth Sci. 58 (1), 37–50. doi:10.1016/j.jafrearsci.2010.01.006
Davidson, J. P., and Wilson, I. R. (1989). Evolution of an Alkali basalt-trachyte Suite from Jebel Marra Volcano, Sudan, Through Assimilation and Fractional Crystallization. Earth Planet. Sci. Lett. 95, 141–160. doi:10.1016/0012-821x(89)90173-8
De Crop, W., and Verschuren, D. (2019). Determining Patterns of Stratification and Mixing in Tropical Crater Lakes Through Intermittent Water-Column Profiling: A Case Study in Western Uganda. J. Afr. Earth Sci. 153, 17–30. doi:10.1016/j.jafrearsci.2019.02.019
Delalande, M., Bergonzini, L., Branchu, P., Filly, A., and Williamson, D. (2008). Hydroclimatic and Geothermal Controls on the Salinity of Mbaka Lakes (SW Tanzania): Limnological and Paleolimnological Implications. J. Hydrol. 359 (3-4), 274–286. doi:10.1016/j.jhydrol.2008.07.007
Delalande-Le Mouëllic, M., Gherardi, F., Williamson, D., Kajula, S., Kraml, M., Noret, A., et al. (2015). Hydrogeochemical Features of Lake Ngozi (SW Tanzania). J. Afr. Earth Sci. 103, 153–167. doi:10.1016/j.jafrearsci.2014.11.004
Delmelle, P., and Bernard, A. (2000). “Volcanic Lakes,” in Encyclopedia of Volcanoes. Editors H. Sigurdsson, B. Houghton, S. McNutt, H. Rymer, and J. Stix (San Diego, CA: Academic Press), 877–895.
de Moor, J. M., Fischer, T. P., King, P. L., Botcharnikov, R. E., Hervig, R. L., Hilton, D. R., et al. (2013). Volatile-Ricj Solicate Melts From Oldoinyo Lengai Volcano (Tanzania): Implications for Carbinatite Genesis and Eruptive Behavior. Earth Planet. Sci. Lett. 361, 379–390. doi:10.1016/j.epsl.2012.11.006
Déruelle, B., Ngounouno, I., and Demaiffe, D. (2007). The 'Cameroon Hot Line’ (CHL): A Unique Example of Active Alkaline Intraplate Structure in Both Oceanic and Continental Lithospheres. Comptes Rendus Geosci. 339 (9), 589–600. doi:10.1016/j.crte.2007.07.007
Dupuy, C., Pagano, M., Got, P., Domaizon, I., Chappuis, A., Marchessaux, G., et al. (2016). Trophic Relationships Between Metazooplankton Communities and Their Plankton Food Sources in the Iles Eparses (Western Indian Ocean). Mar. Environ. Res. 116, 18–31. doi:10.1016/j.marenvres.2016.02.011
Ebinger, C. J. (1989). Geometric and Kinematic Development of Border Faults and Accommodation Zones, Kivu-Rusizi Rift, Africa. Tectonics. 8 (1), 117–133. doi:10.1029/tc008i001p00117
Eggermont, H., Heiri, O., and Verschuren, D. (2006). Fossil Chironomidae (Insecta: Diptera) as Quantitative Indicators of Past Salinity in African Lakes. Quat. Sci. Rev. 25, 1966–1994. doi:10.1016/j.quascirev.2005.04.011
Elshaafi, A., and Gudmundsson, A. (2017). Distribution and Size of Lava Shields on the Al Haruj Al Aswad and the Al Haruj Al Abyad Volcanic Systems, Central Libya. J. Volcanology Geothermal Res. 338, 46–62. doi:10.1016/j.jvolgeores.2017.03.012
Elshaafi, A., and Gudmundsson, A. (2018). Mechanical Interaction Between Volcanic Systems in Libya. Tectonophysics. 722, 549–565. doi:10.1016/j.tecto.2017.11.031
Elshaafi, A., and Gudmundsson, A. (2016). Volcano-tectonics of the Al Haruj Volcanic Province, Central Libya. J. Volcanology Geothermal Res. 325, 189–202. doi:10.1016/j.jvolgeores.2016.06.025
Evans, W. C., Kling, G. W., Tuttle, M. L., Tanyileke, G., and White, L. D. (1993). Gas Buildup in Lake Nyos, Cameroon: The Recharge Process and its Consequences. Appl. Geochem. 8, 207–221. doi:10.1016/0883-2927(93)90036-g
Evans, W. C., White, L. D., Tuttle, M. L., Kling, G. W., Tanyileke, G., and Michel, R. L. (1994). Six Years of Change at Lake Nyos, Cameroon, Yield Clues to the Past and Cautions for the Future. Geochem. J. 28, 139–162. doi:10.2343/geochemj.28.139
Faivre Pierret, R. X., Berne, P., Roussel, C., and Le Guern, F. (1992). The Lake Nyos Disaster: Model Calculations for the Flow of Carbon Dioxide. J. Volcanology Geothermal Res. 51, 161–170. doi:10.1016/0377-0273(92)90066-m
Fantong, W. Y., Kamtchueng, B. T., Yamaguchi, K., Ueda, A., Issa, R., Wirmvem, M. J., et al. (2015). Characteristics of Chemical Weathering and Water-Rock Interaction in Lake Nyos Dam (Cameroon): Implications for Vulnerability to Failure and Re-enforcement. J. Afr. Earth Sci. 101, 42–55. doi:10.1016/j.jafrearsci.2014.08.011
Fazi, S., Butturini, A., Tassi, F., Amalfitano, S., Venturi, S., Vázquez, E., et al. (2018). Biogeochemistry and Biodiversity in a Network of Saline-alkaline Lakes: Implications of Ecohydrological Connectivity in the Kenyan Rift Valley. Ecohydrology & Hydrobiology. 18, 96–106. doi:10.1016/j.ecohyd.2017.09.003
Fontijn, K., Ernst, G. G. J., Elburg, M. A., Williamson, D., Abdallah, E., Kwelwa, S., et al. (2010). Holocene Explosive Eruptions in the Rungwe Volcanic Province, Tanzania. J. Volcanology Geothermal Res. 196, 91–110. doi:10.1016/j.jvolgeores.2010.07.021
Fontijn, K., Williamson, D., Mbede, E., and Ernst, G. G. J. (2012). The Rungwe Volcanic Province, Tanzania - A Volcanological Review. J. Afr. Earth Sci. 63, 12–31. doi:10.1016/j.jafrearsci.2011.11.005
Fouilland, E., Vasseur, C., Leboulanger, C., Le Floc'h, E., Carré, C., Marty, B., et al. (2014). Coupling Algal Biomass Production and Anaerobic Digestion: Production Assessment of Some Native Temperate and Tropical Microalgae. Biomass and Bioenergy. 70, 564–569. doi:10.1016/j.biombioe.2014.08.027
Franz, G., Breitkreuz, C., Coyle, D. A., El Hur, B., Heinrich, W., Paulick, H., et al. (1997). The Alkaline Meidob Volcanic Field (Late Cenozoic, Northwest Sudan). J. Afr. Earth Sci. 25 (2), 263–291. doi:10.1016/s0899-5362(97)00103-6
Freeth, S. J., and Kay, R. L. F. (1987). The Lake Nyos Gas Disaster. Nature. 325, 104–105. doi:10.1038/325104a0
Freeth, S. J., Kling, G. W., Kusakabe, M., Maley, J., Tchoua, F. M., and Tietze, K. (1990). Conclusions from Lake Nyos Disaster. Nature. 348, 201. doi:10.1038/348201a0
Freeth, S. J. (1994). Lake Nyos: Can Another Disaster Be Avoided? Geochem. J. 28, 163–172. doi:10.2343/geochemj.28.163
Freeth, S. J., and Rex, D. C. (2000). Constraints on the Age of Lake Nyos, Cameroon. J. Volcanol. Geotherm. Res. 97 (1-4), 261–269. doi:10.1016/s0377-0273(99)00172-9
Freeth, S. J. (1990). The Anecdotal Evidence, Did it Help or Hinder Investigation of the Lake Nyos Gas Disaster? J. Volcanology Geothermal Res. 42, 373–380. doi:10.1016/0377-0273(90)90033-c
Freeth, S. J. (1992). “The Lake Nyos Gas Disaster,” in Natural Hazards in West and Central Africa. Editors S. J. Freeth, C. O. Ofoegbu, and K. M. Onuoha (Braunschweig: Vieweg), 63–82. doi:10.1007/978-3-663-05239-5_8
Garcin, Y., Schefuß, E., Schwab, V. F., Garreta, V., Gleixner, G., Vincens, A., et al. (2014). Reconstructing C3 and C4 Vegetation Cover Using N -alkane Carbon Isotope Ratios in Recent Lake Sediments from Cameroon, Western Central Africa. Geochimica et Cosmochimica Acta. 142, 482–500. doi:10.1016/j.gca.2014.07.004
Garcin, Y., Schwab, V. F., Gleixner, G., Kahmen, A., Todou, G., Séné, O., et al. (2012). Hydrogen Isotope Ratios of Lacustrine Sedimentary N-Alkanes as Proxies of Tropical African Hydrology: Insights from a Calibration Transect across Cameroon. Geochimica et Cosmochimica Acta. 79, 106–126. doi:10.1016/j.gca.2011.11.039
Gasse, F., Cortijo, E., Disnar, J. R., Ferry, L., Gibert, E., Kissel, C., et al. (1994). A 36-ka Environmental Record in the Southern Tropics—Lake Tritrivakely (Madagascar). Comptes Rendus de L’academie des Sciences—Serie II. 318, 1513–1519.
Gasse, F., and Van Campo, E. (1998). A 40,000-yr Pollen and Diatom Record from Lake Tritrivakely, Madagascar, in the Southern Tropics. Quat. Res. 49, 299–311. doi:10.1006/qres.1998.1967
Gasse, F., and Van Campo, E. (2001). Late Quaternary Environmental Changes from a Pollen and Diatom Record in the Southern Tropics (Lake Tritrivakely, Madagascar). Palaeogeogr. Palaeoclimatol. Palaeoecol. 167, 287–308. doi:10.1016/s0031-0182(00)00242-x
Geshi, N., Németh, K., Noguchi, R., and Oikawa, T. (2019). Shift from Magmatic to Phreatomagmatic Explosions Controlled by the Lateral Evolution of a Feeder dike in the Suoana-Kazahaya Eruption, Miyakejima Volcano, Japan. Earth Planet. Sci. Lett. 511, 177–189. doi:10.1016/j.epsl.2019.01.038
Giggenbach, W. F., and Guern, F. L. (1976). The Chemistry of Magmatic Gases from Erta'Ale, Ethiopia. Geochimica et Cosmochimica Acta. 40, 25–30. doi:10.1016/0016-7037(76)90190-3
Giggenbach, W. F., Sano, Y., and Schmincke, H. U. (1991). CO2-rich Gases from Lakes Nyos and Monoun, Cameroon; Laacher See, Germany; Dieng, Indonesia, and Mt. Gambier, Australia-variations on a Common Theme. J. Volcanology Geothermal Res. 45, 311–323. doi:10.1016/0377-0273(91)90065-8
Giggenbach, W. F. (1990). Water and Gas Chemistry of Lake Nyos and its Bearing on the Eruptive Process. J. Volcanology Geothermal Res. 42, 337–362. doi:10.1016/0377-0273(90)90031-a
Giresse, P., and Makaya-Mvoubou, (2010). Sediment and Particulate Organic Carbon Fluxes in Various Lacustrine Basins of Tropical Africa and in the Gulf of Guinea. Glob. Planet. Change. 72, 341–355. doi:10.1016/j.gloplacha.2010.01.010
Global Volcanism Program (2013). “Volcanoes of the World (VOTW) Database Information,” in Volcanoes of the World. Editors E. Venzke (Washington, DC: Smithsonian Institution). doi:10.5479/si.GVP.VOTW4-2013
Godfrey Fitton, J. (1983). Active Versus Passive continental Rifting: Evidence from the West African Rift System. Tectonophysics. 94, 473–481. doi:10.1016/0040-1951(83)90030-6
Graettinger, A. H. (2018). Trends in Maar Crater Size and Shape Using the Global Maar Volcano Location and Shape (MaarVLS) Database. J. Volcanology Geothermal Res. 357, 1–13. doi:10.1016/j.jvolgeores.2018.04.002
Haileab, B., Brown, F. H., McDougall, I., and Gathogo, P. N. (2004). Gombe Group Basalts and Initiation of Pliocene Deposition in the Turkana Depression, Northern Kenya and Southern Ethiopia. Geol. Mag. 141 (1), 41–53. doi:10.1017/s001675680300815x
Halbwachs, M., and Sabroux, J. C. (2001). Removing CO2 from Lake Nyos in Cameroon. Science. 292, 438. doi:10.1126/science.292.5516.438a
Halbwachs, M., Grangeon, J., Sabroux, J. -C., and Villevielle, A. (1993). Purge par auto-siphon du gaz carbonique dissous dans le lac Monoun (Cameroun): premiers resultants experimentaux. C. R. Acad. Sci. Paris. 316, 483–489.
Halbwachs, M., Sabroux, J.-C., Grangeon, J., Kayser, G., Tochon-Danguy, J.-C., Felix, A., et al. (2004). Degassing the “Killer Lakes” Nyos and Monoun, Cameroon. Eos Trans. AGU. 85, 281–285. doi:10.1029/2004eo300001
Halbwachs, M., Sabroux, J.-C., and Kayser, G. (2020). Final Step of the 32-year Lake Nyos Degassing Adventure: Natural CO2 Recharge Is to Be Balanced by Discharge Through the Degassing Pipes. J. Afr. Earth Sci. 167, 103575. doi:10.1016/j.jafrearsci.2019.103575
Hammerton, D. (1968). “Recent Discoveries in the Caldera of Jebel Marra,” in Sudan Notes and Records (Khartoum: University of Khartoum), 136–148.
Hunt, J. A., Mather, T. A., and Pyle, D. M. (2020). Morphological Comparison of Distributed Volcanic fields in the Main Ethiopian Rift Using High-Resolution Digital Elevation Models. J. Volcanology Geothermal Res. 393, 106732. doi:10.1016/j.jvolgeores.2019.106732
Issa, , Aka Tongwa, F., Mouliom, A. G., Rouwet, D., Fantong, W. Y., Chako Tchamabé, B., et al. (2014). δ18O and δD Variations in Some Volcanic Lakes on the Cameroon Volcanic Line (West-Africa): Generating Isotopic Baseline Data for Volcano Monitoring and Surveillance in Cameroon. J. Limnol. 73 (1), 95–113. doi:10.4081/jlimnol.2014.966
International Seismological Centre (2020). ISC-GEM Earthquake Catalogue. Thatcham, United Kingdom: International Seismological Centre. doi:10.31905/d808b825
Jolie, E. (2019). Detecting Gas-Rich Hydrothermal Vents in Ngozi Crater Lake Using Integrated Exploration Tools. Sci. Rep. 9, 12164. doi:10.1038/s41598-019-48576-5
Kanari, S.-I. (1989). An Inference on the Process of Gas Outburst from Lake Nyos, Cameroon. J. Volcanology Geothermal Res. 39, 135–149. doi:10.1016/0377-0273(89)90054-1
Kantha, L. H., and Freeth, S. J. (1996). A Numerical Simulation of the Evolution of Temperature and CO2 stratification in Lake Nyos Since the 1986 Disaster. J. Geophys. Res. 101 (B4), 8187–8203. doi:10.1029/96jb00324
Kebede, S., Travi, Y., and Rozanski, K. (2009). The δ18O and δ2H Enrichment of Ethiopian Lakes. J. Hydrol. 365, 173–182. doi:10.1016/j.jhydrol.2008.11.027
Keller, J., and Zaitsev, A. N. (2012). Geochemistry and Petrogenetic Significance of Natrocarbonatites at Oldoinyo Lengai, Tanzania: Composition of Lavas from 1988 to 2007. Lithos. 148, 45–53. doi:10.1016/j.lithos.2012.05.022
Kereszturi, G., Bebbington, M., and Németh, K. (2017). Forecasting Transitions in Monogenetic Eruptions Using the Geologic Record. Geology. 45 (3), 283–286. doi:10.1130/g38596.1
Kereszturi, G., Németh, K., Cronin, S. J., Procter, J., and Agustín-Flores, J. (2014). Influences on the Variability of Eruption Sequences and Style Transitions in the Auckland Volcanic Field, New Zealand. J. Volcanology Geothermal Res. 286, 101–115. doi:10.1016/j.jvolgeores.2014.09.002
Kereszturi, G., Németh, K., Csillag, G., Balogh, K., and Kovacs, J. (2011). The Role of External Environmental Factors in Changing Eruption Styles of Monogenetic Volcanoes in a Mio/Pleistocene continental Volcanic Field in Western Hungary. J. Volcanol. Geotherm. Res. 201 (1-4), 227–240. doi:10.1016/j.jvolgeores.2010.08.018
Kervyn, M., van Wyk de Vries, B., Walter, T. R., Njome, M. S., Suh, C. E., and Ernst, G. G. J. (2014). Directional Flank Spreading at Mount Cameroon Volcano: Evidence from Analogue Modeling. J. Geophys. Res. Solid Earth. 119 (10), 7542–7563. doi:10.1002/2014jb011330
Kling, G. W., Clark, M. A., Wagner, G. N., Compton, H. R., Humphrey, A. M., Devine, J. D., et al. (1987). The 1986 lake Nyos Gas Disaster in Cameroon, West Africa. Science. 236, 169–175. doi:10.1126/science.236.4798.169
Kling, G. W., Clark, M. A., Wagner, G. N., Compton, H. R., Humphrey, A. M., Devine, J. D., et al. (1987). The 1986 Lake Nyos Gas Disaster in Cameroon, West Africa. Science. 236, 169–175. doi:10.1126/science.236.4798.169
Kling, G. W. (1988). Comparative Transparency, Depth of Mixing, and Stability of Stratification in Lakes of Cameroon, West Africa1. Limnol. Oceanogr. 33 (1), 27–40. doi:10.4319/lo.1988.33.1.0027
Kling, G. W., Evans, W. C., Tanyileke, G., Kusakabe, M., Ohba, T., Yoshida, Y., et al. (2005). From the Cover: Degassing Lakes Nyos and Monoun: Defusing Certain Disaster. Proc. Natl. Acad. Sci. 102 (40), 14185–14190. doi:10.1073/pnas.050337410210.1073/pnas.0502274102
Kling, G. W., Evans, W. C., and Tanyileke, G. Z. (2015). “The Comparative Limnology of Lakes Nyos and Monoun, Cameroon,” in Volcanic Lakes. Editors D. Rouwet, B. Christenson, F. Tassi, and J. Vandemeulebrouck (Heidelberg: Springer), 401–425. doi:10.1007/978-3-642-36833-2_18
Kling, G. W., Evans, W. C., and Tuttle, M. L. (1991). A Comparative View of Lakes Nyos and Monoun, Cameroon, West Africa. SIL Proc. 1922-2010. 24, 1102–1105. doi:10.1080/03680770.1989.11898921
Kling, G. W., Tuttle, M. L., and Evans, W. C. (1989). The Evolution of Thermal Structure and Water Chemistry in Lake Nyos. J. Volcanology Geothermal Res. 39, 151–165. doi:10.1016/0377-0273(89)90055-3
Kusakabe, M. (2015). “Evolution of CO2 Content in Lakes Nyos and Monoun, and Sub-lacustrine CO2-recharge System at Lake Nyos as Envisaged from CO2/3He Ratios and Noble Gas Signatures,” in Volcanic Lakes. Editors D. Rouwet, B. Christenson, F. Tassi, and J. Vandemeulebrouck (Heidelberg: Springer). 427–450. doi:10.1007/978-3-642-36833-2_19
Kusakabe, M. (2017). Lakes Nyos and Monoun Gas Disasters (Cameroon)-Limnic Eruptions Caused by Excessive Accumulation of Magmatic CO2 in Crater Lakes. Geochem. Monogr. Ser. 1 (1), 1–50. doi:10.5047/gems.2017.00101.0001
Kusakabe, M., Ohba, T., Issa, Y., Yoshida, H., Ohizumi, T., Evans, W. C., et al. (2008). Evolution of CO2 in Lakes Monoun and Nyos, Cameroon, Before and During Controlled Degassing. Geochem. J. 42, 93–118. doi:10.2343/geochemj.42.93
Kusakabe, M., Ohsumi, T., and Aramaki, S. (1989). The Lake Nyos Gas Disaster: Chemical and Isotopic Evidence in Waters and Dissolved Gases from Three Cameroonian Crater Lakes, Nyos, Monoun and Wum. J. Volcanology Geothermal Res. 39, 167–185. doi:10.1016/0377-0273(89)90056-5
Kusakabe, M., and Sano, Y. (1992). “The Origin of Gases in Lake Nyos, Cameroon,” in International Monograph Series On Interdisciplinary Earth Science Research And Applications. Editors S. J. Freeth, C. O. Ofoegb, and K. M. Onohua (Braunschweig, Wiesbaden: Friedrich Vieweg & SohnVerlag), 83–95. doi:10.1007/978-3-663-05239-5_9
Kusakabe, M., Tanyileke, G. Z., McCord, S. A., and Schladow, S. G. (2000). Recent pH and CO2 Profiles at Lakes Nyos and Monoun, Cameroon: Implications for the Degassing Strategy and its Numerical Simulation. J. Volcanology Geothermal Res. 97, 241–260. doi:10.1016/s0377-0273(99)00170-5
Kusakabe, M., Tiodjio, R. E., Christenson, B., Saiki, K., Ohba, T., and Yaguchi, M. (2019). Enrichment of Ferrous Iron in the Bottom Water of Lake Nyos. J. Afr. Earth Sci. 150, 37–46. doi:10.1016/j.jafrearsci.2018.10.014
Laske, G., Masters, G., Ma, Z., and Pasyanos, M. (2013). Update on CRUST1.0 - A 1-degree Global Model of Earth's Crust. Geophys. Res. Abstr. 15, 2013–2658.
Le Turdu, C., Tiercelin, J.-J., Gibert, E., Travi, Y., Lezzar, K.-E., Richert, J.-P., et al. (1999). The Ziway-Shala Lake Basin System, Main Ethiopian Rift: Influence of Volcanism, Tectonics, and Climatic Forcing on Basin Formation and Sedimentation. Palaeogeogr. Palaeoclimatol. Palaeoecol. 150, 135–177. doi:10.1016/s0031-0182(98)00220-x
Lebamba, J., Vincens, A., Lézine, A.-M., Marchant, R., and Buchet, G. (2016). Forest-savannah Dynamics on the Adamawa Plateau (Central Cameroon) during the "African Humid Period" Termination: A New High-Resolution Pollen Record from Lake Tizong. Rev. Palaeobotany Palynology. 235, 129–139. doi:10.1016/j.revpalbo.2016.10.001
Lemma, B. (2009). Observations on the Relations of Some Physico-Chemical Features and DVM of Paradiaptomus Africanus in Lakes Bishoftu-Guda and Hora-Arsedi, Bishoftu, Ethiopia. Limnologica. 39, 230–243. doi:10.1016/j.limno.2008.06.007
Lenhardt, N., Borah, S. B., Lenhardt, S. Z., Bumby, A. J., Ibinoof, M. A., and Salih, S. A. (2018). The Monogenetic Bayuda Volcanic Field, Sudan - New Insights into Geology and Volcanic Morphology. J. Volcanology Geothermal Res. 356, 211–224. doi:10.1016/j.jvolgeores.2018.03.010
Lockwood, J., and Kusakabe, M. (2018). IWGCL Newsletters. Available at: https://iavceicvl.files.wordpress.com/2018/07/iwgcl-cvl-newsletters.pdf.
Lockwood, J. P., Costa, J. E., Tuttle, M. L., Nni, J., and Tebor, S. G. (1988). The Potential for Catastrophic Dam Failure at Lake Nyos Maar, Cameroon. Bull. Volcanol. 50, 340–349. doi:10.1007/bf01073590
Lockwood, J. P., and Rubin, M. (1989). Origin and Age of the Lake Nyos Maar, Cameroon. J. Volcanology Geothermal Res. 39, 117–124. doi:10.1016/0377-0273(89)90052-8
Loomis, S. E., Russell, J. M., Ladd, B., Street-Perrott, F. A., and Sinninghe Damsté, J. S. (2012). Calibration and Application of the Branched GDGT Temperature Proxy on East African Lake Sediments. Earth Planet. Sci. Lett. 357-358, 277–288. doi:10.1016/j.epsl.2012.09.031
Lowenstein, T. K., and Russell, J. M. (2011). A Brine Evolution Model and Mineralogy of Chemical Sediments in a Volcanic Crater, Lake Kitagata, Uganda. Aquat. Geochem. 17 (2), 129–140. doi:10.1007/s10498-010-9108-x
Maley, J., Livingstone, D. A., Giresse, P., Thouveny, N., Brenac, P., Kelts, K., et al. (1990). Lithostratigraphy, Volcanism, Paleomagnetism and Palynology of Quaternary Lacustrine Deposits From Barombi Mbo (West Cameroon): Preliminary Results. J. Volcanol. Geotherm. Res. 42, 319–335. doi:10.1016/s0031-0182(98)00220-x
Martin, U., and Németh, K. (2006). How Strombolian Is a "Strombolian" Scoria Cone? Some Irregularities in Scoria Cone Architecture from the Transmexican Volcanic Belt, Near Volcan Ceboruco, (Mexico) and Al Haruj (Libya). J. Volcanol. Geotherm. Res. 155 (1-2), 104–118. doi:10.1016/j.jvolgeores.2006.02.012
Martini, M., Giannini, L., Prati, F., Tassi, F., Capaccioni, B., and Iozzelli, P. (1994). Chemical Characters of Crater Lakes in the Azores and Italy: The Anomaly of Lake Albano. Geochem. J. 28 (3), 173–184. doi:10.2343/geochemj.28.173
Mattson, H. B., and Tripoli, B. A. (2011). Depositional Characteristics and Volcanic Landforms in the Lake Natron-Engaruka Monogenetic Field, Northern Tanzania. J. Volcanol. Geotherm. Res. 203 (1-2), 23–34. doi:10.1016/j.jvolgeores.2011.04.010
Mbassa, B. J., Njonfang, E., Benoit, M., Kamgang, P., Gregoire, M., Duchene, S., et al. (2012). Mineralogy, Geochemistry and Petrogenesis of the Recent Magmatic Formations from Mbengwi, a Continental Sector of the Cameroon Volcanic Line (CVL), Central Africa. Mineralogy Pet. 106 (3-4), 217–242. doi:10.1007/s00710-012-0227-5
McCord, S. A., and Schladow, S. G. (1998). Numerical Simulations of Degassing Scenarios for CO2-rich Lake Nyos, Cameroon. J. Geophys. Res. 103, 12355–12364. doi:10.1029/98jb00393
McGlynn, G., Mooney, S., and Taylor, D. (2013). Palaeoecological Evidence for Holocene Environmental Change from the Virunga Volcanoes in the Albertine Rift, Central Africa. Quat. Sci. Rev. 61, 32–46. doi:10.1016/j.quascirev.2012.11.008
Mungoma, S. (1990). The Alkaline, Saline Lakes of Uganda – A Review. Hydrobiologia. 208 (1-2), 75–80. doi:10.1007/bf00008445
Muzuka, A. N. N., Ryner, M., and Holmgren, K. (2004). 12,000-Year, Preliminary Results of the Stable Nitrogen and Carbon Isotope Record from the Empakai Crater Lake Sediments, Northern Tanzania. J. Afr. Earth Sci. 40 (5), 293–303. doi:10.1016/j.jafrearsci.2004.12.005
Nagao, K., Kusakabe, M., Yoshida, Y., and Tanyileke, G. (2010). Noble Gases in Lakes Nyos and Monoun, Cameroon. Geochem. J. 44, 519–543. doi:10.2343/geochemj.1.0101
Ndebele-Murisa, M. R., Musil, C. F., and Raitt, L. (2010). A Review of Phytoplankton Dynamics in Tropical African Lakes. S. Afric. J. Sci. 106 (1-2), 13–18. doi:10.4102/sajs.v106i1/2.64
Németh, K., and Cronin, S. J. (2011). Drivers of Explosivity and Elevated Hazard in Basaltic Fissure Eruptions: The 1913 Eruption of Ambrym Volcano, Vanuatu (SW-Pacific). J. Volcanol. Geotherm. Res. 201 (1-4), 194–209. doi:10.1016/j.jvolgeores.2010.12.007
Németh, K., and Cronin, S. J. (2009). Phreatomagmatic Volcanic Hazards Where Rift-Systems Meet the Sea, a Study from Ambae Island, Vanuatu. J. Volcanol. Geotherm. Res. 180 (2-4), 246–258. doi:10.1016/j.jvolgeores.2008.08.011
Németh, K. (2004). The Morphology and Origin of Wide Craters at Al Haruj Al Abyad, Libya: Maars and Phreatomagmatism in a Large Intracontinental Flood Lava Field? zfg. 48 (4), 417–439. doi:10.1127/zfg/48/2004/417
Ngos, S., and Giresse, P. (2012). The Holocene Sedimentary and Pyroclastic Accumulations of Two Crater Lakes (Mbalang,Tizong) of the Volcanic Plateau of Adamawa (Cameroon): Palaeoenvironmental Reconstruction. The Holocene. 22 (1), 31–42. doi:10.1177/0959683611409779
Ngwa, C. N., Hansteen, T. H., Devey, C. W., van der Zwan, F. M., and Suh, C. E. (2017). Origin and Evolution of Primitive Melts from the Debunscha Maar, Cameroon: Consequences for Mantle Source Heterogeneity Within the Cameroon Volcanic Line. Lithos. 288-289, 326–337. doi:10.1016/j.lithos.2017.06.028
Ngwa, C. N., Suh, C. E., and Devey, C. W. (2010). Phreatomagmatic Deposits and Stratigraphic Reconstruction at Debunscha Maar (Mt Cameroon Volcano). J. Volcanol. Geotherm. Res. 192 (3-4), 201–211. doi:10.1016/j.jvolgeores.2010.02.012
Nkouandou, O. F., and Temdjim, R. (2011). Petrology of Spinel Lherzolite Xenoliths and Host Basaltic Lava from Ngao Voglar Volcano, Adamawa Massif (Cameroon Volcanic Line, West Africa): Equilibrium Conditions and Mantle Characteristics. J. Geosci. 56 (4), 375–387.
Nojiri, Y., Kusakabe, M., Hirabatashi, J.-I., Sato, H., Sano, Y., Shinohara, H., et al. (1990). Gas Discharge at Lake Nyos. Nature. 346, 322–323. doi:10.1038/346322a0
Nojiri, Y., Kusakabe, M., Tietze, K., Hirabayashi, J.-i., Sato, H., Sano, Y., et al. (1993). An Estimate of CO2 Flux in Lake Nyos, Cameroon. Limnol. Oceanogr. 38, 739–752. doi:10.4319/lo.1993.38.4.0739
Ogato, T., Kifle, D., and Lemma, B. (2016). Spatio-temporal Variations in Underwater Light Climate, Thermal and Chemical Characteristics of the Tropical Soda Lake, Lake Shala, Ethiopia. Limnology. 17, 59–69. doi:10.1007/s10201-015-0462-7
Ohba, T., Ooki, S., Oginuma, Y., Kusakabe, M., Yoshida, Y., Ueda, A., et al. (2017). Decreasing Removal Rate of the Dissolved CO2 in Lake Nyos, Cameroon, After the Installation of Additional Degassing Pipes, Geol. Soc. Lond. Spec. Publications. 437, 177–184. doi:10.1144/SP437.6
Pasternack, G. B., and Varekamp, J. C. (1997). Volcanic lake Systematics I. Physical Constraints. Bull. Volcanology. 58, 528–538. doi:10.1007/s004450050160
Pecoraino, G., D’Alessandro, W., and Inguaggiato, S. (2015). “The Other Side of the Coin: Geochemistry of Alkaline Lakes in Volcanic Areas,” in Volcanic Lakes. Editors D. Rouwet, B. Christenson, F. Tassi, and J. Vandemeulebrouck (Heidelberg: Springer), 219–237. doi:10.1007/978-3-642-36833-2_9
Pelleter, A.-A., Caroff, M., Cordier, C., Bachelery, P., Nehlig, P., Debeuf, D., et al. (2014). Melilite-bearing Lavas in Mayotte (France): An Insight into the Mantle Source Below the Comores. Lithos. 208-209, 281–297. doi:10.1016/j.lithos.2014.09.012
Pérez, N. M., Hernández, P. A., Padilla, G., Nolasco, D., Barrancos, J., Melían, G., et al. (2011). Global CO2 Emission from Volcanic Lakes. Geology. 39, 235–238. doi:10.1130/g31586.1
Pons, E. (2006). Le Karthala, Un Volcan Méconnu? Scientific Documentary, DVD Footage. Le Centre Multimédia de l’Université de La Réunion.
Poppe, S., Smets, B., Fontijn, K., Rukeza, M. B., De Marie Fikiri Migabo, A., Milungu, A. K., et al. (2016). Holocene Phreatomagmatic Eruptions Alongside the Densely Populated Northern Shoreline of Lake Kivu, East African Rift: Timing and Hazard Implications. Bull. Volcanol. 78, 82. doi:10.1007/s00445-016-1074-8
Pouclet, A., Bellon, H., and Bram, K. (2016). The Cenozoic Volcanism in the Kivu Rift: Assessment of the Tectonic Setting, Geochemistry, and Geochronology of the Volcanic Activity in the South-Kivu and Virunga Regions. J. Afr. Earth Sci. 121, 219–246. doi:10.1016/j.jafrearsci.2016.05.026
Pouclet, A., Kagou Dongmo, A., Bardintzeff, J.-M., Wandji, P., Chakam Tagheu, P., Nkouathio, D., et al. (2014). The Mount Manengouba, A Complex Volcano of the Cameroon Line: Volcanic History, Petrological and Geochemical Features. J. Afr. Earth Sci. 97, 297–321. doi:10.1016/j.jafrearsci.2014.04.023
Rasamimanana, G., Bardintzeff, J.-M., Rasendrasoa, J., Bellon, H., Thouin, C., Gioan, P., et al. (1998). Les épisodes magmatiques du Sud-Ouest de Madagascar (bassin de Morondava), marqueurs des phénomènes de rifting crétacé et néogène. Comptes Rendus de l'Académie des Sci. - Ser. IIA - Earth Planet. Sci. 326, 685–691. doi:10.1016/s1251-8050(98)80179-1
Rouwet, D., Chiodini, G., Ciuccarelli, C., Comastri, A., and Costa, A. (2019). Lago Albano, The "Anti-nyos-type" lake: The Past as a Key for the Future. J. Afr. Earth Sci. 150, 425–440. doi:10.1016/j.jafrearsci.2018.09.019
Rouwet, D., Christenson, B., Tassi, F., and Vandemeulebrouck, J. (2015a). “Volcanic Lakes,” in Volcanic Lakes. Editors D. Rouwet, B. Christenson, F. Tassi, and J. Vandemeulebrouck (Heidelberg: Springer), 533.
Rouwet, D., Tamburello, G., Chiodini, G., Pecoraino, G., Procesi, M., Ricci, T., et al. (2021). New Insights into the Degassing Dynamics of Lago Albano (Colli Albani Volcano, Rome, Italy) During the Last Three Decades (1989-2019). Ijg. 140 (1), 29–41. doi:10.3301/IJG.2020.19
Rouwet, D., Taran, Y., and Inguaggiato, S. (2015b). Fluid Geochemistry of Tacaná Volcano-Hydrothermal System,” in Active Volcanoes of Chiapas (Mexico): El Chichón and Tacaná. Editors J. L. Macías, and T. Scolamacchia (Heidelberg: Springer), 139–154. doi:10.1007/978-3-642-25890-9_7
Rouwet, D., Tassi, F., Mora-Amador, R., Sandri, L., and Chiarini, V. (2014). Past, Present and Future of Volcanic lake Monitoring. J. Volcanology Geothermal Res. 272, 78–97. doi:10.1016/j.jvolgeores.2013.12.009
Rouwet, D. (2021). “Volcanic lake Dynamics and Related Hazards,” in Forecasting and Planning for Volcanic Hazards, Risks and Disasters. Editor P Papale, 439–471. doi:10.1016/B978-0-12-818082-210.1016/b978-0-12-818082-2.00011-1
Rufer, D., Preusser, F., Schreurs, G., Gnos, E., and Berger, A. (2014). Late Quaternary History of the Vakinankaratra Volcanic Field (Central Madagascar): Insights from Luminescence Dating of Phreatomagmatic Eruption Deposits. Bull. Volcanol. 76 (5), 817. doi:10.1007/s00445-014-0817-7
Rumes, B., Eggermont, H., and Verschuren, D. (2005). Representation of Aquatic Invertebrate Communities in Subfossil Death Assemblages Sampled Along a Salinity Gradient of Western Uganda Crater Lakes. Hydrobiologia. 542, 297–314. doi:10.1007/s10750-004-1873-3
Russell, J. M., McCoy, S. J., Verschuren, D., Bessems, I., and Huang, Y. (2009). Human Impacts, Climate Change, and Aquatic Ecosystem Response During the Past 2000 Yr at Lake Wandakara, Uganda. Quat. Res. 72, 315–324. doi:10.1016/j.yqres.2009.06.008
Ryner, M., Bonnefille, R., Holmgren, K., and Muzuka, A. (2006). Vegetation Changes in Empakaai Crater, Northern Tanzania, at 14,800-9300 Cal Yr BP. Rev. Palaeobot. Palynol. 140 (3-4), 163–174. doi:10.1016/j.revpalbo.2006.03.006
Ryves, D. B., Mills, K., Bennike, O., Brodersen, K. P., Lamb, A. L., Leng, M. J., et al. (2011). Environmental Change over the Last Millennium Recorded in Two Contrasting Crater Lakes in Western Uganda, Eastern Africa (Lakes Kasenda and Wandakara). Quat. Sci. Rev. 30, 555–569. doi:10.1016/j.quascirev.2010.11.011
Saiki, K., Kaneko, K., Ohba, T., Sanemasa, M., Kusakabe, M., Ntchantcho, R., et al. (2017). Vertical Distribution of Dissolved CO2 in Lakes Nyos and Monoun (Cameroon) as Estimated by Sound Speed in Water. Geol. Soc. Lond. Spec. Publications. 437, 185–192. doi:10.1144/SP437.10
Sano, Y., Kusakabe, M., Hirabayashi, J.-i., Nojiri, Y., Shinohara, H., Njine, T., et al. (1990). Helium and Carbon Fluxes in Lake Nyos, Cameroon: Constraint on Next Gas Burst. Earth Planet. Sci. Lett. 99, 303–314. doi:10.1016/0012-821x(90)90136-l
Sano, Y., Wakita, H., Ohsumi, T., and Kusakabe, M. (1987). Helium Isotope Evidence for Magmatic Gases in Lake Nyos, Cameroon. Geophys. Res. Lett. 14, 1039–1041. doi:10.1029/gl014i010p01039
Schmid, M., Halbwachs, M., and Wüest, A. (2006). Simulation of CO2 concentrations, Temperature, and Stratification in Lake Nyos for Different Degassing Scenarios. Geochem. Geophys. Geosyst. 7. doi:10.1029/2005GC001164
Schmid, M., Lorke, A., Dinkel, C., Tanyileke, G., and Wüest, A. (2004). Double-diffusive Convection in Lake Nyos, Cameroon. Deep Sea Res. Oceanographic Res. Pap. 51, 1097–1111. doi:10.1016/j.dsr.2004.02.010
Schmid, M., Lorke, A., Wuest, A., Halbwachs, M., and Tanyileke, G. (2003). Development and Sensitivity Analysis of a Model for Assessing Stratification and Safety of Lake Nyos During Artificial Degassing. Ocean Dyn. 53, 288–301. doi:10.1007/s10236-003-0032-0
Sibree, J. (1891). The Volcanic Lake of Tritriva, Central Madagascar. Proc. R. Geographical Soc. Monthly Rec. Geogr. 13, 477–483. doi:10.2307/1801202
Sigurdsson, H., Devine, J. D., Tchua, F. M., Presser, F. M., Pringle, M. K. W., and Evans, W. C. (1987). Origin of the Lethal Gas Burst from Lake Monoun, Cameroun. J. Volcanology Geothermal Res. 31, 1–16. doi:10.1016/0377-0273(87)90002-3
Smith, I. E. M., and Németh, K. (2017). “Source to Surface Model of Monogenetic Volcanism: A Critical Review,” in Monogenetic Volcanism. Editors K. Németh, G. Carrasco-Nuñez, J. J. Aranda-Gomez, and I. E. M. Smith (Bath, United Kingdom: The Geological Society Publishing House). doi:10.1144/sp446.14
Stoppa, F., and Schiazza, M. (2013). An Overview of Monogenetic Carbonatitic Magmatism from Uganda, Italy, China and Spain: Volcanologic and Geochemical Features. J. South Am. Earth Sci. 41, 140–159. doi:10.1016/j.jsames.2012.10.004
Tanyileke, G., Ntchantcho, R., Fantong, W. Y., Aka, F. T., and Hell, J. V. (2019). 30 Years of the Lakes Nyos and Monoun Gas Disasters: A Scientific, Technological, Institutional and Social Adventure. J. Afr. Earth Sci. 150, 415–424. doi:10.1016/j.jafrearsci.2018.11.022
Tanyileke, G. Z., Kusakabe, M., and Evans, W. C. (1996). Chemical and Isotopic Characteristics of Fluids Along the Cameroon Volcanic Line, Cameroon. J. Afr. Earth Sci. 22, 433–441. doi:10.1016/0899-5362(96)00025-5
Tassi, F., and Rouwet, D. (2014). An Overview of the Structure, Hazards, and Methods of Investigation of Nyos-type Lakes from the Geochemical Perspective. J. Limnol. 73 (1), 55–70. doi:10.4081/jlimnol.2014.836
Tazieff, H. (1989). Mechanisms of the Nyos Carbon Dioxide Disaster and of So-Called Phreatic Steam Eruptions. J. Volcanology Geothermal Res. 39, 109–116. doi:10.1016/0377-0273(89)90051-6
Tchamabe, B. C., Youmen, D., Owona, S., Issa, T., Németh, K., Ngapna, M. N., et al. (2013). Eruptive History of the Barombi Mbo Maar, Cameroon Volcanic Line, Central Africa: Constraints from Volcanic Facies Analysis. Cent. Europ. J. Geosci. 5 (4), 480–496. doi:10.2478/s13533-012-0147-2
Tedesco, D., Tassi, F., Vaselli, O., Poreda, R. J., Darrah, T., Cuoco, E., et al. (2010). Gas Isotopic Signatures (He, C, and Ar) in the Lake Kivu Region (Western Branch of the East African Rift System): Geodynamic and Volcanological Implications. J. Geophys. Res. 115, B01205. doi:10.1029/2008JB006227
Temdjim, R. (2012). Ultramafic Xenoliths from Lake Nyos Area, Cameroon Volcanic Line, West-central Africa: Petrography, mineral Chemistry, Equilibration Conditions and Metasomatic Features. Geochemistry. 72 (1), 39–60. doi:10.1016/j.chemer.2011.07.002
Tietze, K. (1992). “Cyclic Gas Bursts: Are They a 'usual' Feature of Lake Nyos and Other Gas-Bearing Lakes?,” in Natural Hazards in West and Central Africa. Editors S. J. Freeth, C. O. Ofoegbu, and K. M. Onuoha (Braunschweig: Vieweg), 97–107. doi:10.1007/978-3-663-05239-5_10
Vail, J. R. (1972). Jebel Marra, A Dormant Volcano in Darfur Province, Western Sudan. Bull. Volcanol. 36, 251–265. doi:10.1007/BF02596994
van Bree, L. G. J., Peterse, F., van der Meer, M. T. J., Middelburg, J. J., Negash, A. M. D., De Crop, W., et al. (2018). Seasonal Variability in the Abundance and Stable Carbon-Isotopic Composition of Lipid Biomarkers in Suspended Particulate Matter from a Stratified Equatorial Lake (Lake Chala, Kenya/Tanzania): Implications for the Sedimentary Record. Quat. Sci. Rev. 192, 208–224. doi:10.1016/j.quascirev.2018.05.023
Varekamp, J. C., Pasternack, G. B., and Rowe, G. L. (2000). Volcanic lake Systematics II. Chemical Constraints. J. Volcanology Geothermal Res. 97, 161–179. doi:10.1016/s0377-0273(99)00182-1
Vaselli, O., Tedesco, D., Cuoco, E., and Tassi, F. (2015). “Are Limnic Eruptions in the CO2-CH4-Rich Gas Reservoir of Lake Kivu (Democratic Republic of the Congo and Rwanda) Possible? Insights from Physico-Chemical and Isotopic Data,” in Volcanic Lakes. Editors D. Rouwet, B. Christenson, F. Tassi, and J. Vandemeulebrouck (Heidelberg: Springer), 489–505. doi:10.1007/978-3-642-36833-2_22
Venturi, S., Cabassi, J., Butturini, A., Vázquez, E., Pacini, N., Tassi, F., et al. (2019). Biogeochemical Processes in a Small Kenyan Rift Valley Soda lake (Lake Sonachi): Implications for Methane Emissions. Taupo, New Zealand, 45.
Viollier, E., Jézéquel, D., Michard, G., Pèpe, M., Sarazin, G., and Albéric, P. (1995). Geochernical Study of a Crater lake (Pavin Lake, France): Trace-Element Behaviour in the Monimolimnion. Chem. Geology. 125, 61–72. doi:10.1016/0009-2541(95)00059-u
Viollier, E., Michard, G., Jézéquel, D., Pèpe, M., and Sarazin, G. (1997). Geochemical study of a Crater lake: Lake Pavin, Puy de Dôme, France. Constraints Afforded by the Particulate Matter Distribution in the Element Cycling Within the Lake. Chem. Geology. 142, 225–241. doi:10.1016/s0009-2541(97)00093-4
Weidendorfer, D., Schmidt, M. W., and Mattsson, H. B. (2017). A Common Origin of Carbonatite Magmas. Geology. 45 (6), 507–510. doi:10.1130/g38801.1
Williamson, D., Jackson, M. J., Banerjee, S. K., Marvin, J., Merdaci, O., Thouveny, N., et al. (1999). Magnetic Signatures of Hydrological Change in a Tropical Maar-lake (Lake Massoko, Tanzania): Preliminary Results. Phys. Chem. Earth, A: Solid Earth Geodesy. 24 (9), 799–803. doi:10.1016/s1464-1895(99)00117-9
Williamson, D., Jelinowska, A., Kissel, C., Tucholka, P., Gibert, E., Gasse, F., et al. (1998). Mineral-magnetic Proxies of Erosion/oxidation Cycles in Tropical Maar-lake Sediments (Lake Tritrivakely, Madagascar): Paleoenvironmental Implications. Earth Planet. Sci. Lett. 155, 205–219. doi:10.1016/s0012-821x(97)00217-3
Wood, R. B., Prosser, M. V., and Baxter, R. M. (1976). The Seasonal Pattern of Thermal Characteristics of Four of the Bishoftu Crater Lakes, Ethiopia. Freshw. Biol. 6, 519–530. doi:10.1111/j.1365-2427.1976.tb01643.x
Yihdego, Y., and Becht, R. (2013). Simulation of Lake-aquifer Interaction at Lake Naivasha, Kenya Using a Three-Dimensional Flow Model with the High Conductivity Technique and a DEM with Bathymetry. J. Hydrol. 503, 111–122. doi:10.1016/j.jhydrol.2013.08.034
Yoshida, Y., Issa, M., Kusakabe, H., and Ohba, T. (2010). An Efficient Method for Measuring CO2 Concentration in Gassy Lakes: Application to Lakes Nyos and Monoun, Cameroon. Geochem. J. 44, 441–448. doi:10.2343/geochemj.1.0078
Yoshida, Y., Kusakabe, M., Issa, T., Ohba, G., and Hell, J. V. (2017). Decreasing Capability of the Degassing Systems at Lakes Nyos and Monoun (Cameroon): A New Gas Removal System Applied to Lake Monoun to Prevent a Future Limnic Eruption. Geol. Soc. Lond. Spec. Publications. 437, 205–212. doi:10.1144/SP437.3
Keywords: Africa, volcanic lakes, maar, Lake Nyos, database, hazard assessment
Citation: Rouwet D, Németh K, Tamburello G, Calabrese S and Issa (2021) Volcanic Lakes in Africa: The VOLADA_Africa 2.0 Database, and Implications for Volcanic Hazard. Front. Earth Sci. 9:717798. doi: 10.3389/feart.2021.717798
Received: 31 May 2021; Accepted: 09 September 2021;
Published: 28 September 2021.
Edited by:
Roberto Sulpizio, University of Bari Aldo Moro, ItalyReviewed by:
Dario Pedrazzi, Instituto de Ciencias de la Tierra Jaume Almera (ICTJA), SpainMark Thomas, University of Leeds, United Kingdom
Copyright © 2021 Rouwet, Németh, Tamburello, Calabrese and Issa. This is an open-access article distributed under the terms of the Creative Commons Attribution License (CC BY). The use, distribution or reproduction in other forums is permitted, provided the original author(s) and the copyright owner(s) are credited and that the original publication in this journal is cited, in accordance with accepted academic practice. No use, distribution or reproduction is permitted which does not comply with these terms.
*Correspondence: Dmitri Rouwet, ZG1pdHJpLnJvdXdldEBpbmd2Lml0