- 1Key Laboratory of Deep Oil and Gas, China University of Petroleum, Qingdao, China
- 2Dagang Oilfield Company of PetroChina, Tianjin, China
The significance of lacustrine shale oil has gradually become prominent. Lacustrine shale has complex lithologies, and their reservoir properties are quite various. The multi-scale pore structure of shale controls the law of shale oil enrichment. Typical lacustrine shale developed in the Member 2 of Kongdian Formation in Cangdong sag, Bohai Bay Basin. The lithofacies and multi-scale storage space of this lacustrine shale have been systematically studied. 1. The mineral composition is quite different, and the lithofacies can be summarized into siliceous, carbonate and mixed types. The rock structure can be summarized into laminated, layered, and massive types. 2. The pores are diverse and multi-scale. Interparticle pores contribute the main storage space, especially the interparticle pores of quartz and dolomite. 3. The physical properties of the massive shales is relatively inferior to those of layered and laminatedtypes, and it presents the characteristics of " laminated >layered > massive ". The developed laminae can significantly improve the space and seepage capacity of lacustrine shale. 4. Clay minerals provide the main nano-scale storage space, but they are often filled in pores and reduces the shale brittleness, which have destruction effects.
Introduction
There are big differences between lacustrine shale and marine shale. Compared with marine basins, continental lake basins have a smaller area and paleo-uplifts around the lake basins, always forming a topography with obvious height difference (Loucks et al., 2012; Lazar et al., 2015; Xu et al., 2019; Teng et al., 2021). In the lake basin, due to the sedimentary environment and diagenetic evolution, the shale system has strong heterogeneity and complex lithology, which always makes its reservoir characteristics different (Katz, 2001; Xu et al., 2017; Teng et al., 2021). The terrigenous clastics originating from the paleo-uplift experienced short-distance transport and deposition into the lake basin. Multiple terrestrial sources, rapid sedimentary facies change, low clastic roundness, and proximity to terrestrial sources are characteristics of lake basin sedimentation (Xu et al., 2017; Xu Q. et al., 2020).
The research on the reservoir characteristics of lacustrine mixed sediments is relatively weak, and the multi-scale pore characteristics of different lithologies have not been clarified, which severely restricts the study of shale oil enrichment rules and development (Feng et al., 2020; Wang et al., 2021). The Member 2 of Kongdian Formation in Cangdong sag, Bohai Bay Basin has shale oil development potential that cannot be ignored. It has many types of rocks, and its lithology changes quickly in vertical and horizontal directions. The types of reservoir spaces are diverse, and the reservoir control law is not yet clear (Chen et al., 2016; Zhao et al., 2017). After more than 50 years of exploration and development, the main body of the structural belt is highly proven, and the proven oil and gas area accounts for more than 80% of the main body of the structural belt (Zhao et al., 2017). How to change the thinking of exploration and development and find replacement resources under the guidance of new theories is an urgent problem to be solved. With the development of unconventional oil and gas theory, the exploration direction has begun to shift to deep shale oil, among which the Member 2 of Kongdian Formation an important replacement area (Zhao et al., 2017).
The shale strata in the central part of the lake basin in the study area are well-developed. However, due to its complex rock types, the production of shale oil wells is highly heterogeneous, and it is necessary to study the multiscale pore characteristics of different rock types. Previously, this research strata was thought as a source rock strata. Through the systematic coring analysis of Well G108-8, it is recognized that the shale strata have various rock types and frequent interbeds, with high-quality source rocks, many occluded hydrocarbons, and good oil content. Many wells such as KN9, Z1605, and G1608 have successively obtained industrial oil flow in different intervals, with the highest daily oil production of 47.1 t (Pu et al., 2016; Zhao et al., 2017).
Lithofacies gradually play a role in the study of shale heterogeneity, and it is gradually becoming a trend to use lithofacies as a link to predict shale oil sweet spots (Zhao et al., 2017; Wang et al., 2019; Yang et al., 2019). The research of shale facies mainly focuses on marine shale. Compared with marine shale, lacustrine shale is more complicated in terms of mineral composition, deposition, reservoir, and distribution of organic matter (Wang et al., 2018; Xu Q. et al., 2020). Lithofacies is a rock or rock combination formed in a certain depositional environment, which can reflect the depositional process and depositional environment, and can be better applied to the study of lacustrine shale reservoirs (Yang et al., 2015; Xu et al., 2020b).
With the deepening of shale oil and gas development, the research methods of reservoirs are also deepening. The research scale of reservoirs has gradually reached the micro-nano level, and refinement and quantification are the trends in reservoir geology research (Loucks et al., 2012; Lazar et al., 2015; Yang et al., 2019; Xu et al., 2020b). The difference in the size of the reservoir space is the essential difference between unconventional reservoirs and conventional reservoirs (Schieber, 2010; Lazar et al., 2015). Unconventional storage space can be micro-nano scale, which can reach more than 60% of the total storage space (Pang et al., 2018). In addition, the micro-nano-scale shale pores determine the oil and gas accumulation mode and seepage mechanism. The fluid in the pores of millimeter level migrates in a “pipe flow” mode, while the fluid in the pores of micrometer level is in a “seepage” mode. The oil and gas in nano-scale pores is adsorbed on the surface of minerals and kerogen in an adsorbed state, or adsorbed in the interior of organic matter in a diffused state (Yang et al., 2016, 2018). The micro-nano-scale pore structure obviously controls the migration of oil and gas, and the study of multi-scale pore characteristics of shale is very important (Yang et al., 2016; Pang et al., 2018; Yang et al., 2018; Xu Q. et al., 2020).
On the basis of lithofacies division, multiscale pore characteristics of different lacustrine shale types in the Member 2 of Kongdian Formation in Cangdong sag were studied systematically. Notably, we attempt to identify similarities and differences between the target lacustrine shale using quantitative methods, including high pressure mercury intrusion test, low temperature N2 adsorption/ desorption (LTNA) test, porosity and permeability. In addition, some qualitative methods, including the use of a field emission scanning electron microscope (FE-SEM) equipped with an energy dispersive spectroscopy (EDS) system, polarized light, and cathodoluminescence microscopes were also used to support our research.
Geological Background
Cangdong Sag is located in the southern part of Huanghua Depression in Bohai Bay Basin, bounded by Kongdian Uplift in the north, Dongguang Uplift in the south, Cangxian Uplift in the west, and Xuhei Uplift in the east (Figure 1). It is the second largest oil-rich sag in the Huanghua Depression, with a total exploration area of 4700 km2. It is a long and narrow Cenozoic rift basin developed on a regional extensional background (Pu et al., 2016; Zhao et al., 2017, 2018). During the sedimentary period of the second member of Kong, the activity of the Cangdong fault began to become the main controlling factor of the structural evolution, and the Nanpi, Cangdong and Changzhuang Sags have begun to form (Pu et al., 2016; Zhao et al., 2017, 2018).
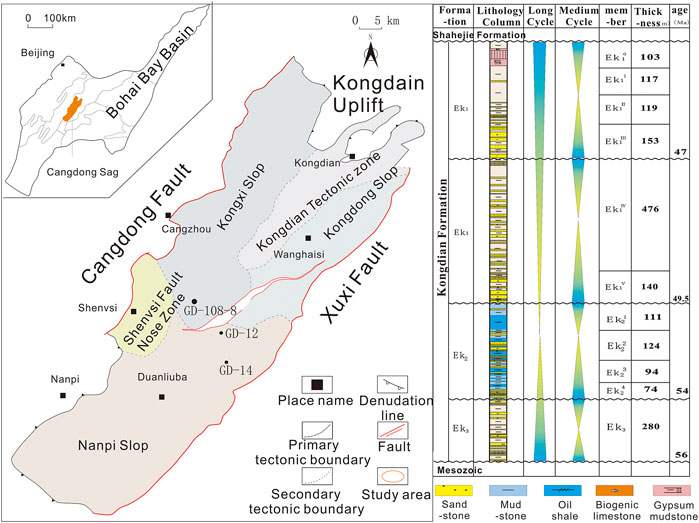
FIGURE 1. Geological map and general stratigraphy of the Member 2 of Kongdian Formation in Cangdong sag, Bohai Bay Basin, China.
The Ek2 in Cangdong sag belongs to a closed depression-type lake basin with stable structural evolution and extensive waters. The lithology is mainly dark gray shale, with a small amount of light gray silt sandstone, dolomite and other local developments. The gray-brown argillaceous dolomite section is gradually developed in the upward strata, with a total thickness of 400–600 m. The Ek2 section can be divided into four subsections from bottom to top, namely Ek24, Ek23, Ek22, and Ek21 (Figure 1).
Samples and Experimental Methods
The lithofacies types are systematically divided. The bulk mineralogy of 56 samples was determined using X-ray diffraction (XRD) methods. Through polarized light microscopy, XRF scanning, FE-SEM, electron probe, the micro-mineral types, pore types and sedimentary structure types were analyzed, and lithofacies types were classified (Figures 2–4; Table 1).
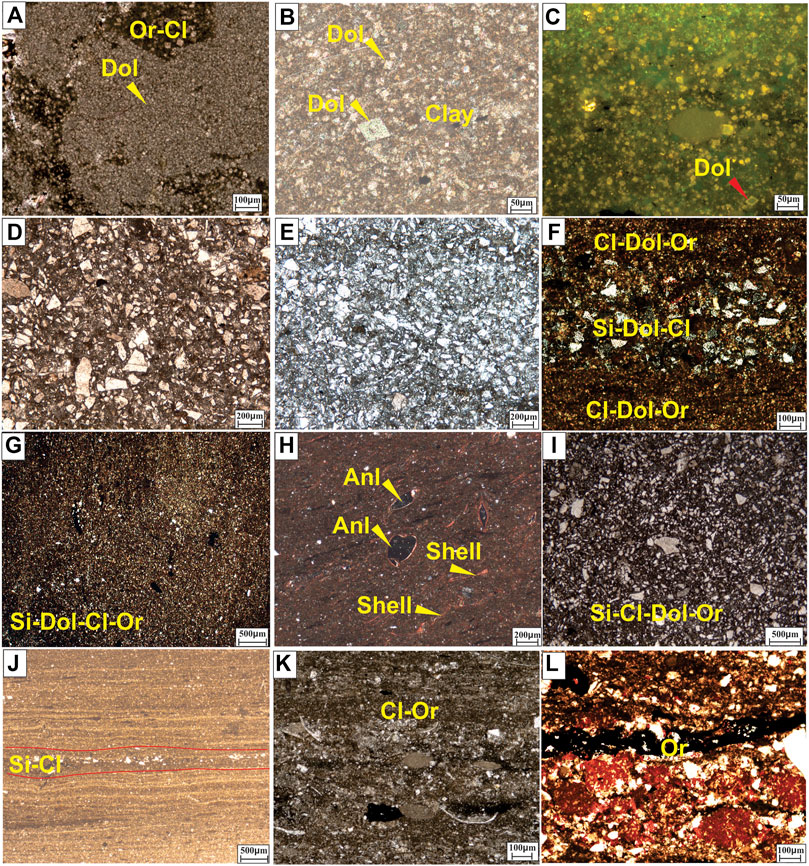
FIGURE 2. The characteristics of fine-grained mixed sedimentary rock of Ek2 lacustrine shale in the Congdong Sag (A). Fine-crystalline dolomite, with mixed organic matter and clay minerals. (B). Fine-crystal-medium-crystal dolomite is mixed with clay minerals (C). Fine-grained-medium-crystalline dolomite is mixed with clay minerals, and a good oil-bearing display can be seen under fluorescence (D). Shale with a large amount of quartz, feldspar, clay minerals, mixed deposits. (E) shale with a lot of quartz and feldspar (F). Layered structure, medium-coarse feldspar-quartz particles are layered. Clay minerals and fine-grained dolomite layers (G). Mixed shale, mixed deposition (H). Mixed shale, mixed deposition. Visible biological shells. Shells are filled with analcime (I). Mixed shale, mixed deposition (J). Fine-grained felsic and clay laminae, with a coarse-grained felsic layer in the middle (K). Fine-grained mixed sedimentation, with clay minerals-organic matter layer, shell and felsic in disorderly distribution. l: Calcite laminae-organic matter laminae, showing the messy distribution of felsic-dolomite. Ca-carbonate minerals; Si- felsic minerals; Cl-clay minerals; Anl- analcime; Or-organic matter.
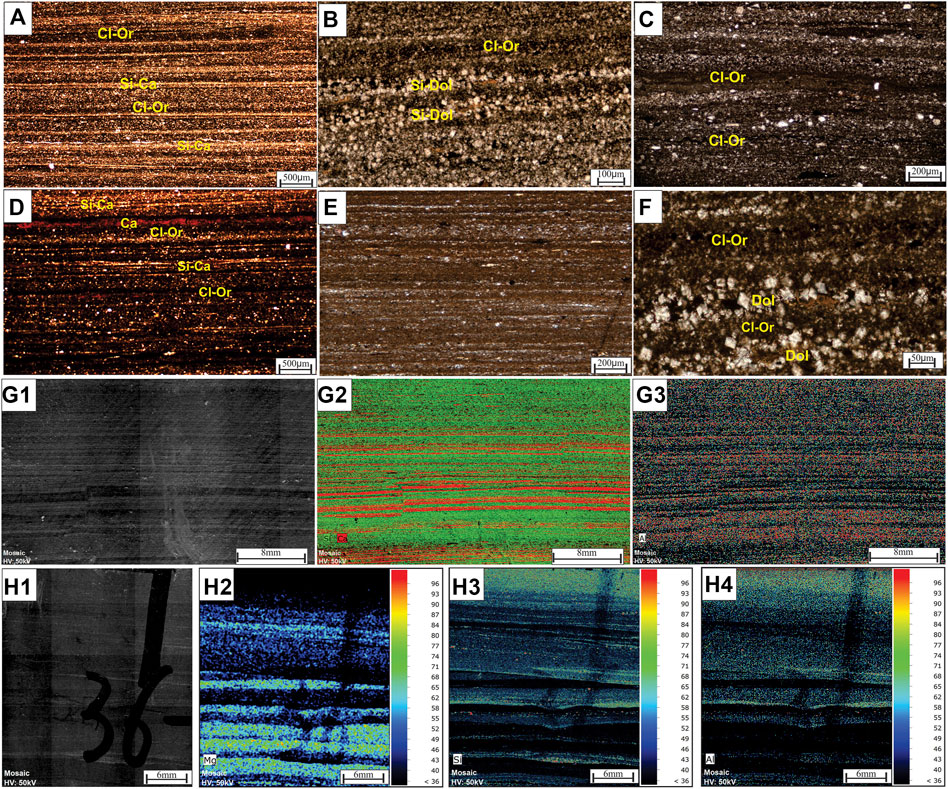
FIGURE 3. Various laminated types developed in the mixed sedimentary rocks [(A–F): single polarized light; (G,H): XRF scan].
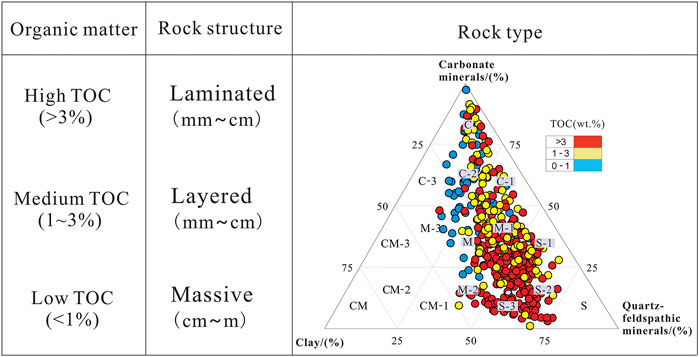
FIGURE 4. Lithofacies classification standard of the lacustrine shale system in the study area. C: Low organic matter (OM)—massive—carbonate shales. C1: Medium to high OM—laminated—siliceous carbonate shales. C2: Low to medium OM—layered—mixed carbonate shales. S1: Medium to high OM—laminated—carbonate siliceous shales. S2: Medium to high OM—massive—mixed siliceous shales. S3: Medium to high OM—laminated—argillaceous siliceous shale. M: Low to medium OM—massive -mixed shale. M1: Medium to high OM—laminated—mixed shale.
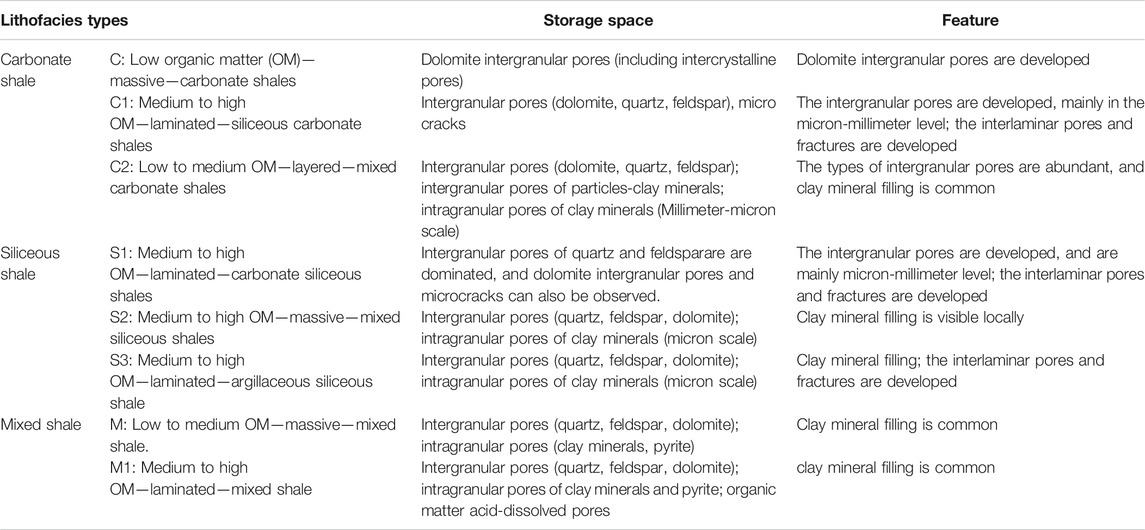
TABLE 1. Spatial characteristics and classification of EK2 lacustrine shale reservoirs in the study area.
Based on the classification of lithofacies, 34 samples of different lithofacies types were tested for the high-pressure mercury intrusion test at China University of Geosciences (Wuhan) with the experimental reference standard SY/T 5346-2005 (Table 2). In addition, in order to characterize the nanoscale pores, nitrogen adsorption tests were used to characterize the samples’ nanoscale pores with an ASAP 2020M device at the China University of Petroleum (East China).
Results and Discussion
Differences in Petrology
The lacustrine shale strata are highly heterogeneous under the influence of sedimentation, diagenesis, and tectonic processes. They are characterized by complex mineral composition and diverse rock structure types. Minerals mainly composed of felsic minerals, carbonate minerals and clay, in addition to an amount of analcime, pyrite, siderite, etc. The type of rock structure is also quite different, with laminated (visible felsic, carbonate, gray, clay, organic, and other laminae), layered, massive, etc. (Figures 2–4).
The particle size of felsic minerals varies greatly and the sedimentary structures are diverse. Generally, mud-silt felsic minerals are mostly layered, and often form interlayers with argillaceous layers; while medium-coarse sandy felsic minerals generally appear as scattered particles in massive dolomite, argillaceous dolomite or dolomitic mudstone (Figures 2, 3).
Carbonate minerals are mainly dolomite. Dolomite is mostly micrite-fine powder crystal, and crystal grains with better crystal shape are often seen. The intergranular pores of dolomite are developed. The production status of calcite is relatively diverse, generally in the form of scattered spots or crack filling. (Figures 2, 3).
The content of organic matter is generally high, but there are also big differences. The TOC content of the vast majority of tested samples range from 1.0 to 11.3% with avg. 4.5%. Rock types with higher felsic content have relatively higher organic matter, while the organic matter in the rock types with higher carbonate content are relatively lower.
The shale in the study area has low maturity, mainly in the low-mature stage. The Ro values are mainly in the range of 0.66–0.81%, and the average value is 0.72% (Jiao, 2017). Due to the low degree of organic matter maturity in this area, the organic matter pores are underdeveloped.
It can be seen that the rock types in the study area are complex and changeable, dominated by mixed sediments, and lithofacies can be used as links for further research on lacustrine shale reservoirs. Taking “clay minerals-carbonate minerals-felsic minerals” as the three terminal elements, with 50% of their respective content as the boundary, the shale samples are divided into four categories. The transitional rock types with no more than 50% ternary component are named mixed shale facies (Figure 4).
Classification and Differences in Pore Types
For shale reservoirs, the micro-nano scale is an important part. In order to obtain larger magnifications and clearer photos, the observation of the microscopic space is mainly through the FE-SEM, and the samples were subjected to Ar-iron milling before observation. In this study area, the reservoir space of the research area is summarized as: 1. interparticle pores, 2. intraparticle pores, 3. micro-cracks, 4. organic matter pores (Figures 2, 3, 5; Table 1). There are many types of interparticle pores, mainly mineral aggregate pores and mineral grain inner pores. These interparticle pores contribute the main reservoir space and are the most important pore type. The interparticle pores are dominated by the pores between particles, crystals and clay minerals. Micro-fractures are relatively developed in carbonate shale, dolomite and felsic shale sections, mainly caused by tectonic and diagenesis, and have good connectivity. Due to the relatively low maturity, the scale and contribution of organic matter pores are limited (Figures 2, 3, 5).
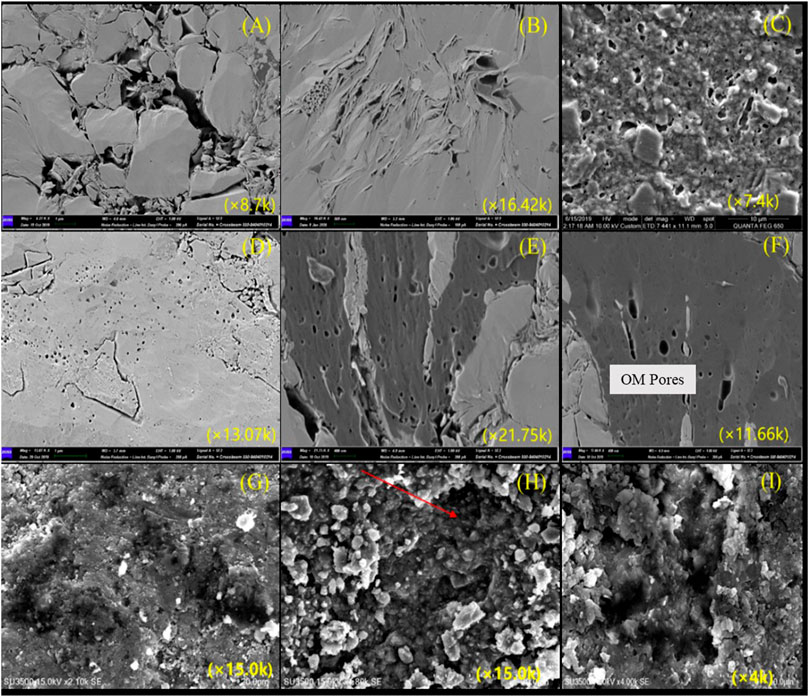
FIGURE 5. Characteristics of shale micro-pore by SEM observation. (A): G108-8, 3,040.6m, developed interparticle pores. (B): GD14, 4,115.6m, developed intercrystalline and interparticle pores of clay minerals. (C): GD14, 4,121.9m, massive dolomitic shale facies with developed dissolution pores. (D): G108-8, 3,040.63m, medium-organic laminar layered felsic dolomitic shale facies with well-developed pores. (E): G108-8, 3,040.6m, organic matter pores. (F): G108-8, 3,040.6m, organic matter pores. (G): GD12, 3,880.9m, developed interparticle pores with oil. (H): GD14, 4,135.5m, developed interparticle pores. (I): GD 12, 3,880.9m, developed interparticle pores.
Compared with marine shale, lacustrine shale in the study area has the following characteristics:
1) The rock types and their reservoir types are diverse. There are felsic, dolomitic, mixed shale reservoir types, and some dolomite interbeds and felsic interbeds.
2) Although some lithologies are relatively tight (mixed shale facies), developed nano-scale reservoir spaces can still be observed under the observation of SEM (Figure 5).
3) The micro-reservoir space is dominated by interparticle pores and intraparticle pores. The interparticle pores are relatively large with millimeter-micron macropores, and the intraparticle pores are mainly nano-micron scale (Figures 2, 5).
4) The organic matter content is high, but due to low maturity, the scale of organic matter pore development is limited (Figure 5).
5) The brittle minerals are high, and various levels of cracks can be observed, but there are certain filling phenomena of calcite and clay minerals (Figures 2, 5).
Differences in Multi-Scale Pore Structure
The physical properties of shale reservoirs mainly depend on the characteristics of multi-scale pore structure, including pore throat size, pore geometry, and pore connectivity (Gou et al., 2019; Xu et al., 2020c). Carrying out multi-scale pore structure studies of different lithofacies types can not only clarify the storage performance of the reservoir itself, but also reveal the occurrence status and enrichment rules of shale oil (Yang et al., 2019).
The samples in the study area have the characteristics of multi-scale spaces, and the curves show that the pore size distribution has the characteristics of high left and low right peaks (Figure 6). When the pore size is less than 100 nm, the mercury intake increases significantly, and nano-scale pores provide a large amount of storage space. But some samples also have developed right peaks indicating the rich large-scale storage spaces (Figure 6).
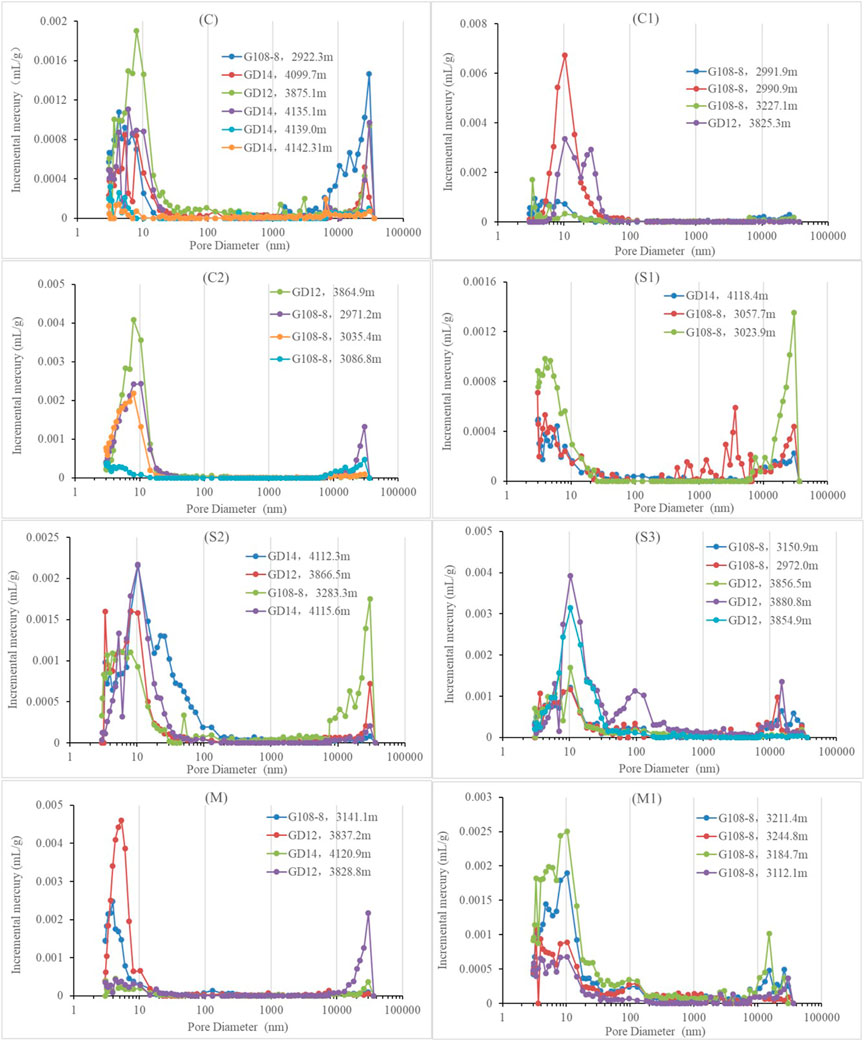
FIGURE 6. Distributions of pore-throat diameter of different lithofacies. C: Low organic matter (OM)—massive—carbonate shales. C1: Medium to high OM—laminated—siliceous carbonate shales. C2: Low to medium OM—layered—mixed carbonate shales. S1: Medium to high OM—laminated—carbonate siliceous shales. S2: Medium to high OM—massive—mixed siliceous shales. S3: Medium to high OM—laminated—argillaceous siliceous shale. M: Low to medium OM—massive -mixed shale. M1: Medium to high OM—laminated—mixed shale.
The comparison of the efficiency of mercury withdrawal and porosity in different rock structures presents the characteristics of " laminated > layered > massive" (Figure 7). The efficiency of mercury withdrawal of laminated facies is 35.86%, and the porosity is 3.83%. The efficiency of mercury withdrawal of layered shale facies is 25.13%, and the porosity is 3.59%. They are higher than those of the massive shale facies (Figure 7).
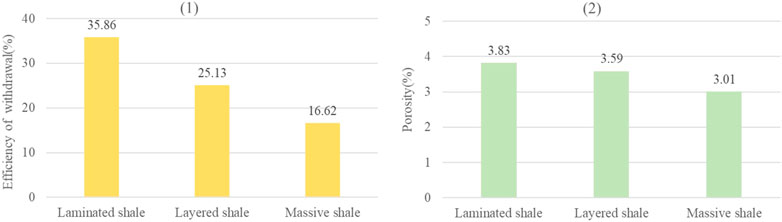
FIGURE 7. Comparison of the efficiency of mercury withdrawal and porosity in different rock structures.
The comparison of the efficiency of mercury withdrawal and porosity for the carbonate and siliceous shale facies are basically same and slightly lower than those of the mixed facies (Figure 8). The efficiency of mercury withdrawal of carbonate facies is 23.21%, and the porosity is 3.07%. The efficiency of mercury withdrawal of siliceous shale facies is 23.46%, and the porosity is 3.69%. The efficiency of mercury withdrawal of mixed facies is 37.57%, and the porosity is 3.81% (Figure 8).
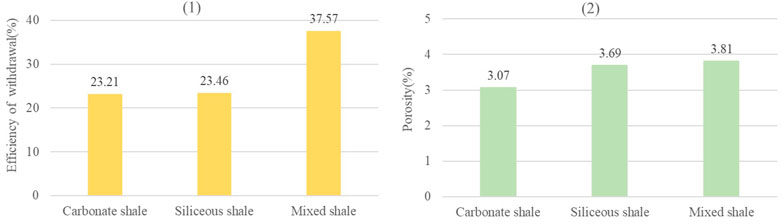
FIGURE 8. Comparison of the efficiency of mercury withdrawal and porosity in different mineral composition.
The C1, S1 and M1 types with developed laminae have relatively high efficiency of mercury withdrawal and porosity, showing better features of storage space and pore structure (Figure 9). The low temperature N2 adsorption/ desorption (LTNA) method can further accurately determine the storage space size and structural characteristics (2–100 nm) of the mesopores, which can make up for the shortcomings of high-pressure mercury intrusion in nano-scale pore characterization. The characteristics of the samples in the study area can be judged that the adsorption isotherm is mainly П type, and the adsorption loop is mainly H3 type, but also has H4 type characteristics (Figure 10); the overall loudness of the nano-scale pore structure is high-quality. Unlike other reservoirs, when the relative pressure P/P0 ranges from 0.9 to 1.0, the amount of N2 adsorption increases sharply without capillary condensation (Figure 10). When P/P0 is close to the saturated vapor pressure, the saturated adsorption phenomenon does not appear, indicating that the sample also contains relatively large pores that cannot be measured by LTNA (Ravikovitch and Neimark, 2002; Nie et al., 2015) (Figure 10).
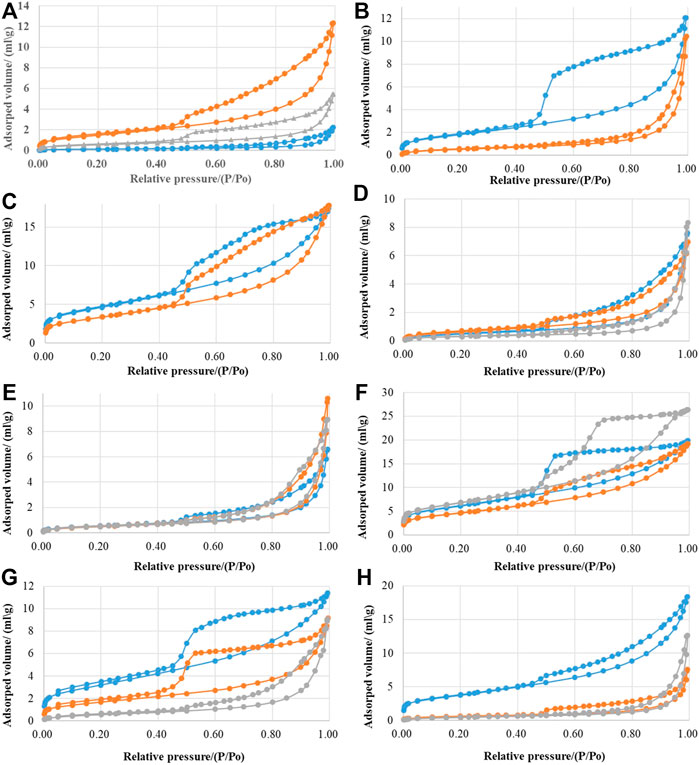
FIGURE 10. Comparison of characteristics of nitrogen adsorption lines for samples of different lithofacies. (A): Low organic matter (OM)—massive—carbonate shales. (B): Medium to high OM—laminated—siliceous carbonate shales. (C): Low to medium OM—layered—mixed carbonate shales. (D): Medium to high OM—laminated—carbonate siliceous shales. (E): Medium to high OM—massive—mixed siliceous shales. (F): Medium to high OM—laminated—argillaceous siliceous shale. (G): Low to medium OM—massive -mixed shale. (H): Medium to high OM—laminated—mixed shale.
The lithofacies types (S-3, M, M-1) developed by clay minerals have relatively developed nanoscale storage spaces (<100 nm). But the nanoscale storage spaces of the felsic and dolomitic shales are not developed (Figure 11A,B). The content of clay minerals and nano-scale storage space parameters (specific surface area and total pore volume) show a good positive correlation. It can be seen that the nanoscale storage space (<100 nm) (specific surface area and total pore volume) can be mainly provided by clay minerals (Figure 11C,D).
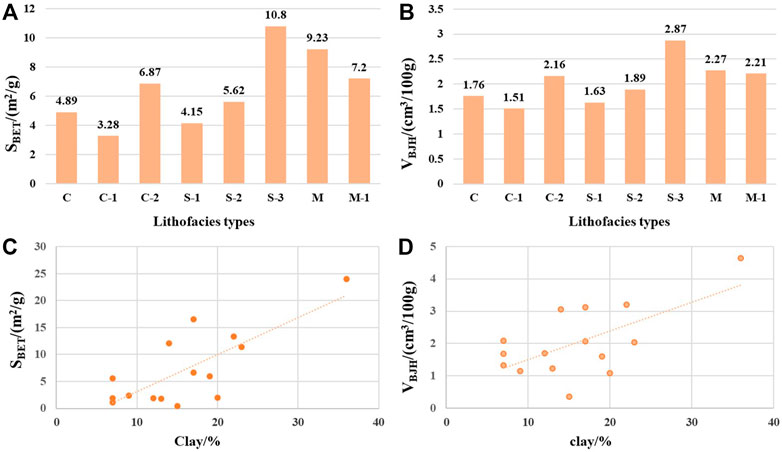
FIGURE 11. Comparison of nano-scale pore characteristics of different lithofacies types. (A) and (B) refer to the values of S and V of different lithofacies. (C) and (D) refer to the correlation between clay minerals and nano-scale reservoir space (S, V). C: Low organic matter (OM)—massive - carbonate shales. C1: Medium to high OM—laminated—siliceous carbonate shales. C2: Low to medium OM—layered—mixed carbonate shales. S1: Medium to high OM—laminated—carbonate siliceous shales. S2: Medium to high OM—massive—mixed siliceous shales. S3: Medium to high OM—laminated—argillaceous siliceous shale. M: Low to medium OM—massive -mixed shale. M1: Medium to high OM—laminated—mixed shale.
Control Mechanism of Lacustrine Shale Oil Reservoir
The combined application of multi-scale qualitative-quantitative analysis methods can deeply compare the differences of different lithofacies and point out the important role of laminae in the reservoir-migration system. On one hand, these laminated shales including the C1 type (medium to high OM - laminated - siliceous carbonate shales), S1type (medium to high OM - laminated - carbonate siliceous shales) and M1 type (medium to high OM - laminated - mixed shale) show better storage performance and connectivity. One the other hand, the pore structure characteristics of mixed shale types are better than those of the other two types.
In the experimental test, the appearance of cracks will greatly change the physical characteristics of the sample (Xu et al., 2019; Xu et al., 2020b). The clay mineral content in the mixed shales is relatively high, and the shale with high clay mineral content is prone to undercompaction during the diagenetic evolution process (Liu et al., 2019; Panja et al., 2019; Teng et al., 2021). The cementation between layers of clay minerals is relatively weak, with cracks visible (Figures 12A,B). Clay minerals can provide rich nano-scale storage space, but they are often filled in big pores and cracks, which have destruction effects.
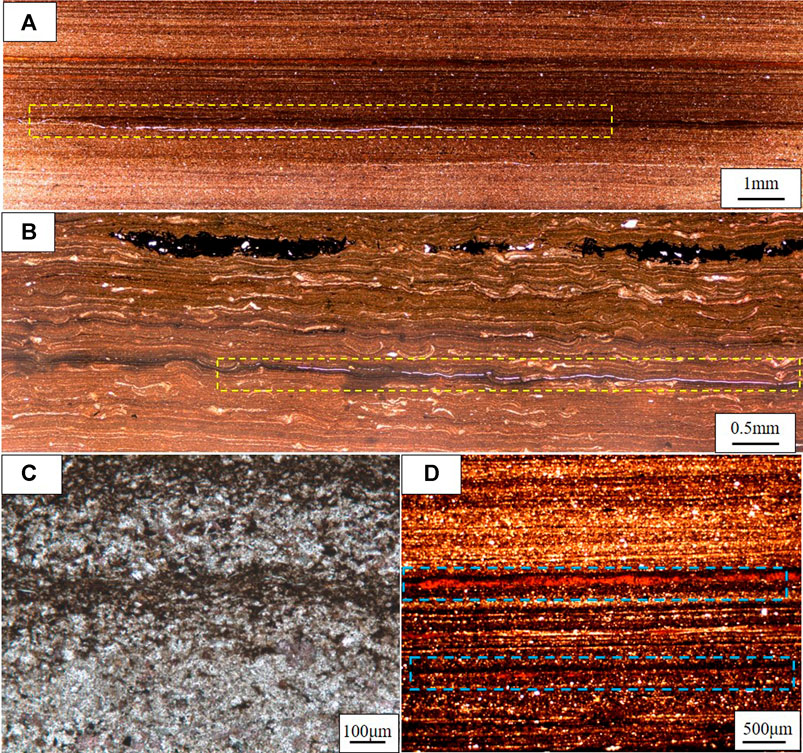
FIGURE 12. Microcracks can develop between clay minerals (A,B), and cementation of calcite mineral make the rock more tight (C,D). (A): GD12, 3,880.9m; (B): G108-8, 3,040.6m; (C): G108-8, 3,043.5m; (D): GD14, 4,135.5 m.
Calcite in carbonate shales is usually a cementitious material (Figures 12C,D). Siliceous carbonate shales can also be cemented with silicon. This is also the reason why the carbonate shales and siliceous shales can have poor physical parameters, and these samples with poor parameters can change the comparison results.
Due to theundercompaction and weak cementation of clay minerals in M1 type, this type can show good physical properties and pore structure characteristics. It should be noted that this is only for test samples. For shale oil exploration, fracturing should also be considered. Lithography with high clay mineral content cannot be used as a favorable reservoir type. C1 and S1 types have good pore structure and storage space, and have good fracturing properties, so they should be the best lithofacies types.
Conclusion
1. The rock types of lacustrine fine-grained mixed sedimentary rocks in the study area are quite different. The minerals mainly composed of felsic minerals, carbonate, clay and analcime, and the rock structure mainly include lamellar, layered, massive types. Based on the method of organic matter- rock structure-rock type, the main lithofacies can be summarized into eight types.
2. Multi-scale storage spaces are generally developed in these shales, and interparticle pores support the main storage spaces. Pores can be summarized as interparticle pores (including intercrystalline pores), intraparticle pores, organic pores, and microcracks. Quartz and dolomite interparticle pores contributes the main spaces.
3. The physical properties of the massive shales is relatively inferior to those of layered and massive types, and it presents the characteristics of " laminated >layered > massive e". The development of laminated features can strongly improve storage space and permeability. Among these lithofacies types, C1 (Medium to high OM—laminated—siliceous carbonate shales) and S1 (Medium to high OM—laminated—carbonate siliceous shales) types have good pore structure, storage space and fracturing properties, and should be the best lithofacies types.
4. Clay minerals provide the main nano-scale storage space, but they are often filled in pores and reduces the shale brittleness, which have destruction effects. The lithofacies types (S-3, M, M-1) developed by clay minerals have relatively developed nanoscale spaces (<100 nm). The adsorption isotherms of nano-scale pores are mainly П type, and the adsorption loops are mainly H3 type. Clay lamina can produce cracks due to undercompaction and weak cementation. However, high clay content is not conducive to shale fracturing.
Data Availability Statement
The original contributions presented in the study are included in the article/Supplementary Material, further inquiries can be directed to the corresponding authors.
Author Contributions
QX: Methodology, Writing-original draft, Writing-review and editing. XZ: Data curation, Project administration, Funding acquisition. XP: Data curation, Project administration. WH and ZS: Data curation, Project administration, Funding acquisition. JT and BX: Data curation, Project administration, Writing-review and editing. BZ and PG: Formal analysis, Writing-review and editing.
Funding
Our study is supported by the National Natural Science Foundation of China (Grants 41821002 and 41902131). The authors also sincerely appreciate the support from the Dagang Oilfield Company of PetroChina.
Conflict of Interest
QX was employed by the Company Dagang Oilfield Company of PetroChina.
The remaining authors declare that the research was conducted in the absence of any commercial or financial relationships that could be construed as a potential conflict of interest.
Publisher’s Note
All claims expressed in this article are solely those of the authors and do not necessarily represent those of their affiliated organizations, or those of the publisher, the editors and the reviewers. Any product that may be evaluated in this article, or claim that may be made by its manufacturer, is not guaranteed or endorsed by the publisher.
Acknowledgments
The authors also sincerely appreciate the support from the Dagang Oilfield Company of PetroChina.
References
Chen, S., Gong, W., Zhang, S., Pu, X., Ma, H., Shi, Z., et al. (2016). Fracture Characteristics and Main Controlling Factors of Shales of the Second Member of Kongdian Formation in Cangdong Sag, Huanghua Depression. Geoscience 30 (1), 144–153.
Feng, Q., Xu, S., Xing, X., Zhang, W., and Wang, S. (2020). Advances and Challenges in Shale Oil Development: A Critical Review. Adv. Geo-energy Res. 4 (4), 406–418. doi:10.46690/ager.2020.04.06
Gou, Q., Xu, S., Hao, F., Yang, F., Zhang, B., Shu, Z., et al. (2019). Full-scale Pores and Micro-fractures Characterization Using FE-SEM, Gas Adsorption, Nano-CT and micro-CT: A Case Study of the Silurian Longmaxi Formation Shale in the Fuling Area, Sichuan Basin, China. Fuel 253, 167–179. doi:10.1016/j.fuel.2019.04.116
Jiao, Y. (2017). Sedimentary Characteristics and the Formation Conditions of fine-grained Mixed Sedimentary Rocks in the Second Member of Kongdian Formation in Cangdong Sag, Doctoral dissertation. Qingdao, China: China University of Petroleum. (East China).
Katz, B. J. (2001). Lacustrine basin Hydrocarbon Exploration–Current Thoughts. J. paleolimnology 26 (2), 161–179. doi:10.1023/a:1011173805661
Lazar, O. R., Bohacs, K. M., Macquaker, J. H. S., Schieber, J., and Demko, T. M. (2015). Capturing Key Attributes of fine-grained Sedimentary Rocks in Outcrops, Cores, and Thin Sections: Nomenclature and Description Guidelines. J. Sediment. Res. 85 (3), 230–246. doi:10.2110/jsr.2015.11
Liu, B., Schieber, J., Mastalerz, M., and Teng, J. (2019). Organic Matter Content and Type Variation in the Sequence Stratigraphic Context of the Upper Devonian New Albany Shale, Illinois Basin. Sediment. Geology. 383, 101–120. doi:10.1016/j.sedgeo.2019.02.004
Loucks, R. G., Reed, R. M., Ruppel, S. C., and Hammes, U. (2012). Spectrum of Pore Types and Networks in Mudrocks and a Descriptive Classification for Matrix-Related Mudrock Pores. Bulletin 96 (6), 1071–1098. doi:10.1306/08171111061
Nie, B., Liu, X., Yang, L., Meng, J., and Li, X. (2015). Pore Structure Characterization of Different Rank Coals Using Gas Adsorption and Scanning Electron Microscopy. Fuel 158, 908–917. doi:10.1016/j.fuel.2015.06.050
Pang, Z., Tao, S., Zhang, Q., yang, J., Zhang, T., Yang, X., et al. (2018). Reservoir Micro Structure of Da'anzhai Member of Jurassic and its Petroleum Significance in Central Sichuan Basin, SW China. Pet. Exploration Dev. 45 (1), 1–11. doi:10.1016/s1876-3804(18)30006-5
Panja, P., Pathak, M., and Deo, M. (2019). Productions of Volatile Oil and Gas-Condensate from Liquid Rich Shales. Adv. Geo-energy Res. 3 (1), 29–42. doi:10.26804/ager.2019.01.02
Pu, X., Zhou, L., Han, W., Zhou, J., Wang, W., Zhang, W., et al. (2016). Geologic Features of fine-grained Facies Sedimentation and Tight Oil Exploration: A Case from the Second Member of Paleogene Kongdian Formation of Cangdong Sag, Bohai Bay Basin. Pet. Exploration Dev. 43 (1), 24–34. doi:10.1016/s1876-3804(16)30003-9
Ravikovitch, P. I., and Neimark, A. V. (2002). Experimental Confirmation of Different Mechanisms of Evaporation from Ink-Bottle Type Pores: Equilibrium, Pore Blocking, and Cavitation. Langmuir 18 (25), 9830–9837. doi:10.1021/la026140z
Schieber, J. (2010), Common Themes in the Formation and Preservation of Intrinsic Porosity in Shales and Mudstones–Illustrated with Examples across the Phanerozoic. SPE Unconventional Gas Conference. OnePetro. February.
Teng, J., Deng, H., Liu, B., Chen, W., Fu, M., Xia, Y., et al. (2021). Insights of the Pore System of Lacustrine Shales from Immature to Late Mature with the Aid of Petrology, Mineralogy and Porosimetry: A Case Study of the Triassic Yanchang Formation of the Ordos Basin, North China. J. Pet. Sci. Eng. 196, 107631. doi:10.1016/j.petrol.2020.107631
Wang, R., Hu, Z., Dong, L., Gao, B., and Yin, S. (2021). Advancement and Trends of Shale Gas Reservoir Characterization and Evaluation. Oil Gas Geology. 42 (1), 54–65.
Wang, R., Hu, Z., Long, S., Liu, G., Zhao, J., Dong, L., et al. (2019). Differential Characteristics of the Upper Ordovician‐Lower Silurian Wufeng‐Longmaxi Shale Reservoir and its Implications for Exploration and Development of Shale Gas In/around the Sichuan Basin. Acta Geologica Sinica ‐ English Edition 93 (3), 520–535. doi:10.1111/1755-6724.13875
Wang, R., Hu, Z., Sun, C., Liu, Z., Zhang, C., Gao, B., et al. (2018). Comparative Analysis of Shale Reservoir Characteristics in the Wufeng-Longmaxi (O3w-S1l) and Niutitang (Є1n) Formations: A Case Study of wells JY1 and TX1 in the southeastern Sichuan Basin and its Neighboring Areas, Southwestern China. Interpretation 6 (4), SN31–SN45. doi:10.1190/int-2018-0024.1
Xu, Q., Hao, F., Ma, Y., Liu, B., and Song, X. (2020a). Effects of the Matrix on the Oil Production of Supertight limestone in a Lacustrine Mixed Sedimentary Environment: The Case of the Jurassic Da'anzhai Member in the central Sichuan Basin, China. Mar. Pet. Geology. 121, 104583. doi:10.1016/j.marpetgeo.2020.104583
Xu, Q., Liu, B., Ma, Y., Song, X., Wang, Y., Xin, X., et al. (2017). Controlling Factors and Dynamical Formation Models of Lacustrine Organic Matter Accumulation for the Jurassic Da'anzhai Member in the central Sichuan Basin, Southwestern China. Mar. Pet. Geology. 86, 1391–1405. doi:10.1016/j.marpetgeo.2017.07.014
Xu, Q., Ma, Y., Liu, B., Song, X., Su, J., and Chen, Z. (2019). Characteristics and Control Mechanism of Nanoscale Pores in Lacustrine Tight Carbonates: Examples from the Jurassic Da'anzhai Member in the central Sichuan Basin, China. J. Asian Earth Sci. 178, 156–172. doi:10.1016/j.jseaes.2018.05.009
Xu, S., Gou, Q., Hao, F., Zhang, B., Shu, Z., and Zhang, Y. (2020b). Multiscale Faults and Fractures Characterization and Their Effects on Shale Gas Accumulation in the Jiaoshiba Area, Sichuan Basin, China. J. Pet. Sci. Eng. 189, 107026. doi:10.1016/j.petrol.2020.107026
Xu, S., Hao, F., Shu, Z., Zhang, A., and Yang, F. (2020c). Pore Structures of Different Types of Shales and Shale Gas Exploration of the Ordovician Wufeng and Silurian Longmaxi Successions in the Eastern Sichuan Basin, South China. J. Asian Earth Sci. 193, 104271. doi:10.1016/j.jseaes.2020.104271
Xu, S., Liu, R., Hao, F., Engelder, T., Yi, J., Zhang, B., et al. (2019). Complex Rotation of Maximum Horizontal Stress in the Wufeng-Longmaxi Shale on the Eastern Margin of the Sichuan Basin, China: Implications for Predicting Natural Fractures. Mar. Pet. Geology., 109, 519–529. doi:10.1016/j.marpetgeo.2019.06.008
Yang, F., Hu, B., Xu, S., Meng, Q., and Krooss, B. M. (2018). Thermodynamic Characteristic of Methane Sorption on Shales from Oil, Gas, and Condensate Windows. Mar. Pet. Geology. 32 (10), 10443–10456. doi:10.1016/j.marpetgeo.2019.06.008
Yang, F., Ning, Z., Wang, Q., Zhang, R., and Krooss, B. M. (2016). Pore Structure Characteristics of Lower Silurian Shales in the Southern Sichuan Basin, China: Insights to Pore Development and Gas Storage Mechanism. Int. J. Coal Geology. 156, 12–24. doi:10.1016/j.coal.2015.12.015
Yang, F., Xu, S., Hao, F., Hu, B., Zhang, B., Shu, Z., et al. (2019). Petrophysical Characteristics of Shales with Different Lithofacies in Jiaoshiba Area, Sichuan Basin, China: Implications for Shale Gas Accumulation Mechanism. Mar. Pet. Geology. 109, 394–407. doi:10.1016/j.marpetgeo.2019.06.028
Zhao, X., Pu, X., Han, W., Zhou, L., Shi, Z., Chen, S., et al. (2017). A New Method for Lithology Identification of fine Grained Deposits and Reservoir Sweet Spot Analysis: A Case Study of Kong 2 Member in Cangdong Sag, Bohai Bay Basin, China. Pet. Exploration Dev. 44 (4), 492–502. doi:10.1016/s1876-3804(17)30061-7
Zhao, X., Zhou, L., Pu, X., Jin, F., Han, W., Xiao, D., et al. (2018). Geological Characteristics of Shale Rock System and Shale Oil Exploration Breakthrough in a Lacustrine basin: A Case Study from the Paleogene 1st Sub-member of Kong 2 Member in Cangdong Sag, Bohai Bay Basin, China. Pet. Exploration Dev. 45 (3), 361–373. doi:10.1016/s1876-3804(18)30043-0
Keywords: lacustrine shale oil, multiscale pore, member 2 of kongdian formation, pore structure, shale lithofacie
Citation: Xu Q, Zhao X, Pu X, Han W, Shi Z, Tian J, Zhang B, Xin B and Guo P (2021) Characteristics and Control Mechanism of Lacustrine Shale Oil Reservoir in the Member 2 of Kongdian Formation in Cangdong Sag, Bohai Bay Basin, China. Front. Earth Sci. 9:783042. doi: 10.3389/feart.2021.783042
Received: 25 September 2021; Accepted: 08 November 2021;
Published: 24 November 2021.
Edited by:
Feng Yang, China University of Geosciences Wuhan, ChinaReviewed by:
Bei Liu, China University of Geosciences Wuhan, ChinaRuyue Wang, SINOPEC Petroleum Exploration and Production Research Institute, China
Juan Teng, Chengdu University of Technology, China
Copyright © 2021 Xu, Zhao, Pu, Han, Shi, Tian, Zhang, Xin and Guo. This is an open-access article distributed under the terms of the Creative Commons Attribution License (CC BY). The use, distribution or reproduction in other forums is permitted, provided the original author(s) and the copyright owner(s) are credited and that the original publication in this journal is cited, in accordance with accepted academic practice. No use, distribution or reproduction is permitted which does not comply with these terms.
*Correspondence: Qilu Xu, cWlsdV94dUBvdXRsb29rLmNvbQ==; Jinqiang Tian, dGlhbmpxQHVwYy5lZHUuY24=