- 1Université de Paris, Institut de physique du globe de Paris, CNRS, Paris, France
- 2UMR CARRTEL, INRAE & Université Savoie Mont Blanc, Annecy, France
- 3UMR 7245, MCAM, CNRS-MNHN, Muséum National d’Histoire Naturelle, Paris, France
- 4MARBEC, Université de Montpellier IRD CNRS Ifremer, Sète, France
Nitrogen isotope compositions (δ15N) in sedimentary rocks are extensively used to investigate the biogeochemical nitrogen cycle through geological times. This use relies on the observation that, in modern continental platforms and anoxic basins, surface sediments faithfully record the δ15N of primary producers, assuming that it was similar in the past. Over Earth’s history, however, surface environments experienced profound changes, including the transition of ammonium-dominated to nitrate-dominated waters and the transition from exclusively microbial ecosystems to ecosystems including multicellularity, which make modern environments significantly different compared to earlier ones, potentially invalidating the fundamental assumption that surface sediments faithfully record the δ15N of primary producers. In order to improve our understanding of the nitrogen isotopic information contained in the early Earth’s sedimentary rock record, we investigate here the nitrogen isotope systematics in a microbial, nitrate free and ammonium-rich modern system, the Dziani Dzaha Lake. In this modern system, the δ15N of the reduced dissolved inorganic nitrogen (i.e., NH4+ and NH3) in the water column is close to ∼7‰ . δ15N of suspended particulate matter (SPM) show a similar average value in surface waters (i.e., where SPM is massively composed of active primary producers), but increases up to 14‰ in the deeper part of the water column during periods when it is enriched in dissolved reduced species (i.e., CH4, H2S/HS− and NH4+/NH3). Surface sediments δ15N, with values comprised between 10 and 14 ‰, seem to preferentially record these positive isotopic signatures, rather than those of active primary producers. We propose here that the observed isotopic pattern is mainly linked to the assimilation of ammonium strongly enriched in 15N by isotope exchange with ammonia under basic conditions. Although ammonium assimilation seems here to be responsible for a significant isotopic enrichment due to the basic conditions, in neutral anoxic environments inhabited by similar microbial ecosystems, this process may also significantly impact the δ15N of primary producers towards more negative values. This would have strong implications for our interpretation of the Precambrian sedimentary record as this finding challenges one the fundamental hypotheses underlying the use of sedimentary δ15N in paleo-oceanographic reconstructions, i.e. that surface sediments faithfully record the δ15N of active primary producers in the photic zone.
Introduction
In continental margins and modern anoxic basins the nitrogen isotope compositions (δ15N) of surface sediments faithfully record the δ15N of the nitrogen species assimilated by primary producers in surface waters (e.g. Thunell et al., 2004; Sigman et al., 2009; Robinson et al., 2012). In these environments, water column or sediment diagenesis processes were shown to have a limited impact on the primary producer’s δ15N (Lehmann et al., 2002; Chen et al., 2008; Mobius et al., 2010; Mobius, 2013), while in the oxic deep oceans 15N enrichments compared to the primary producers have been reported in both surface sediments and particles sinking in the deep waters (i.e., 2–5‰ increase, Altabet and François, 1994; Mobius et al., 2010; Mobius et al., 2011; Robinson et al., 2012).
In the last decades, numerous studies have been conducted to improve our understanding of the biogeochemical nitrogen cycle and the diverse metabolic processes that regulate it (e.g., Sigman et al., 2009; Mobius, 2013; Bruner et al., 2013; Zhang et al., 2014). Overall, they show that nitrogen speciation and δ15N in modern systems, including the open ocean (NO3− and on average 5 ‰, respectively), are mainly controlled by the balance in the dominant nitrogen metabolic pathways in the ocean (i.e., nitrogen fixation and denitrification), which are closely tied to the nutrient supply and water column redox structure (e.g., Galbraith et al., 2008; Sigman et al., 2009; Quan et al., 2013; Bruner et al., 2013; Mobius, 2013). It is based on these observations in modern systems that the sedimentary δ15N record has been used to infer the evolution of both the biogeochemical nitrogen cycle and water column redox structures through geological times (e.g., see reviews in Ader et al., 2016; Stueken et al., 2016).
Yet, in contrast to modern environments where the main nitrogen species is nitrate, early Earth’s environments must have been mainly dominated by ammonium (NH4+), at least before the ocean oxygenation during late Archean/early Proterozoic times (e.g., Beaumont and Robert, 1999; Canfield et al., 2010; Luo et al., 2018; Mettam et al., 2019; Yang et al., 2019). This has several implications for our understanding of the nitrogen isotope record of these time periods. First, ammonium assimilation is a well-known process associated with a large isotopic fractionation that may generate strongly 15N-depleted organic matter (Pennock et al., 1996; Sigman et al., 2009; Vo et al., 2013). Although it was proposed to explain negative δ15N values for some Cretaceous anoxic events (Higgins et al., 2012) or Archean and Proterozoic successions (Papineau et al., 2009; Mettam et al., 2019), there is still no known modern analogue to validate this scenario because their dissolved ammonium content is often limited, quantitatively assimilated or beyond reach of primary producers (e.g., Fuchsman et al., 2008). Second, the sediments of modern or past basic lacustrine environments often have strongly positive isotopic signatures (≥10 ‰) that have been interpreted as resulting from the NH4+/NH3 chemical and isotope equilibrium and volatilization of the 15N-depleted NH3 (e.g., Talbot and Johannessen, 1992; Menzel et al., 2013; McLauchlan et al., 2013; Stueken et al., 2015). However, in-depth studies of the nitrogen isotope systematics in such modern systems are still lacking, preventing a comprehensive documentation of the processes controlling the elevated δ15N values of sediments, including those related to the quality of preservation in the sedimentary record of the primary producer’s signature. In order to explore this question further, we investigate here the nitrogen isotope systematics in a newly identified, and so far unique, microbial dominated, nitrate free and ammonium-rich modern lacustrine system, the Dziani Dzaha Lake.
Materials and Methods
Study Site
The Dziani Dzaha Lake is a shallow tropical volcanic crater lake located on the Petite Terre Island of Mayotte (Comoros Archipelago, Indian Ocean, Figure 1). Its surface area is close to 2.36 × 105 m2 and it is separated from the nearby seashore by a 220-m thick crater wall. The volcanic crater is approximately 1 km in diameter and 50–100 m high, resulting in a very restricted lake watershed. The average depth of the Dziani Dzaha water column is about 3 m with a narrow depression reaching 18 m depth, probably related to the phreatomagmatic eruption at the origin of this lake (i.e., between 9 and 4 ka, Zinke et al., 2003).
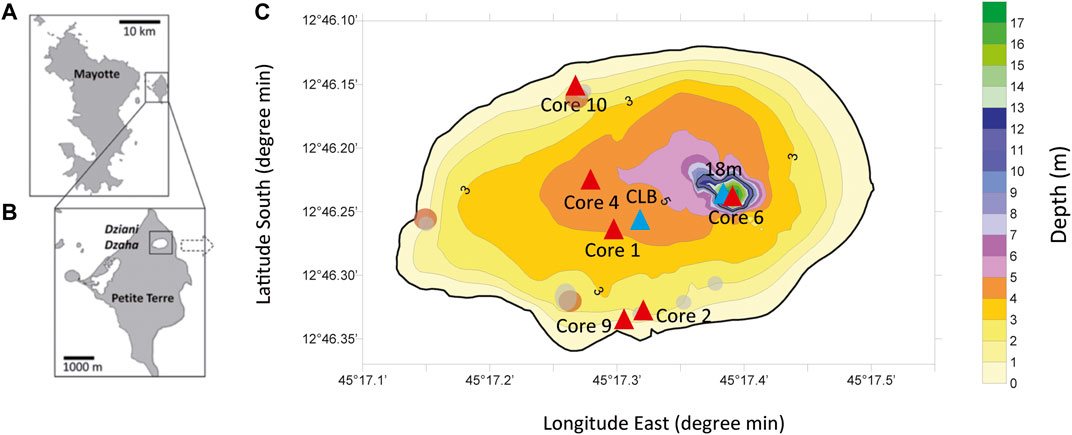
FIGURE 1. Location (A,B) and bathymetric map (C) of the Dziani Dzaha. Water column (blue triangles) and sediment cores (red triangles) sampling locations.
The Dziani Dzaha water column has a combination of physical, chemical and biological features that are atypical for a modern lacustrine system, which have been previously recently documented and are summarized here. Its waters probably originated from seawater and are now characterized by high salinity (i.e., ranging from 34 to 71 psu), strong alkalinity (i.e., ranging from 0.1 to 0.2 M), elevated pH value (i.e., comprised between 9 and 9.5), and very high primary productivity (i.e., 8 gC m−2 day−1 (Leboulanger et al., 2017; Sarazin et al., 2020). The lake ecosystem is dominated by microorganisms with a dense and perennial bloom of two photosynthetic species [i.e., Picocystis salinarum (Chlorophyta) and Arthrospira fusiformis (Cyanobacteria), Bernard et al., 2019]. The water column is permanently anoxic below ca. 1.5 m depth, with the anoxic water being periodically euxinic (i.e., presence of H2S and HS−) (e.g., Sarazin et al., 2020). The SO42− content is relatively low compared to seawater (<3 mM, Sarazin et al., 2020). Organic matter in the anoxic waters is remineralized through both sulfate reduction, as evidenced by the periodic total consumption of SO42−, when the lake becomes euxinic, and methanogenesis, as indicated by the high concentration of CH4 dissolved in the water column that degasses into the atmosphere (Cadeau et al., 2020). Finally, the water column is nitrate-free while the reduced dissolved nitrogen species (i.e., NH4+/NH3) accumulate in the deeper part of the Dziani Dzaha lake (Bernard et al., 2019).
The physical and chemical structure of the Dziani Dzaha water column as well as the biological organism’s distribution within it (mostly dominated by cyanobacteria), are closely related to the seasonal variations (Hugoni et al., 2018; Bernard et al., 2019; Sarazin et al., 2020). The water column is periodically stratified with a halocline at about 2 m depth due a decrease in surface water salinity driven by rainy season precipitation (Figure 2). A permanent chemocline was present at ca. 14 m depth in the depression. During the stratified period (Figures 2, 3), salinity above the halocline was within 35–45 psu, alkalinity was close to 0.1 M, pH was close to 9.5, SO42− was close to 3 mM, and only traces of NH4+/NH3 were observed (<0.03 mM). Below the halocline the salinity and alkalinity increased up to 70 psu and 0.2 M, respectively, pH decreased to a value close to 9, no sulfates were observed, and reduced species accumulated. It is worth noting here while that HS−/H2S concentrations and CH4 concentrations increased sharply at 2 m depth, i.e., across the halocline, up to 6 and 2 mM, respectively, and remained relatively constant down to the bottom, NH4+/NH3 concentration increased in two steps, one up to 0.4 mM at 3 m depth (i.e., 1 m below the halocline) and another one up to 6 mM below the deep chemocline. Above the 2 m-depth halocline, the diversity was dominated by photosynthetic microorganisms, while below it, it was dominated by a dense and diverse population of archaea and heterotrophic bacteria (Hugoni et al., 2018). During the non-stratified period most of the physical, chemical and biological parameters were constant with depth down to the deep chemocline at 14 m depth, except for the dissolved oxygen that was only present down to a maximum of about 1 m depth (Figures 2, 3). The salinity was close to 65 psu, the alkalinity close to 0.14 M, the pH close to 9.2, the SO42− content close to 3 mM, NH4+/NH3 content was lower than 0.03 mM, and the planktonic biomass was dominated by photosynthetic microorganisms. The biological and physico-chemical parameters of the waters underlying the deep chemocline during non-stratified periods resembled strongly to stratified periods, albeit with even higher concentrations of reduced species (Sarazin et al., 2020).
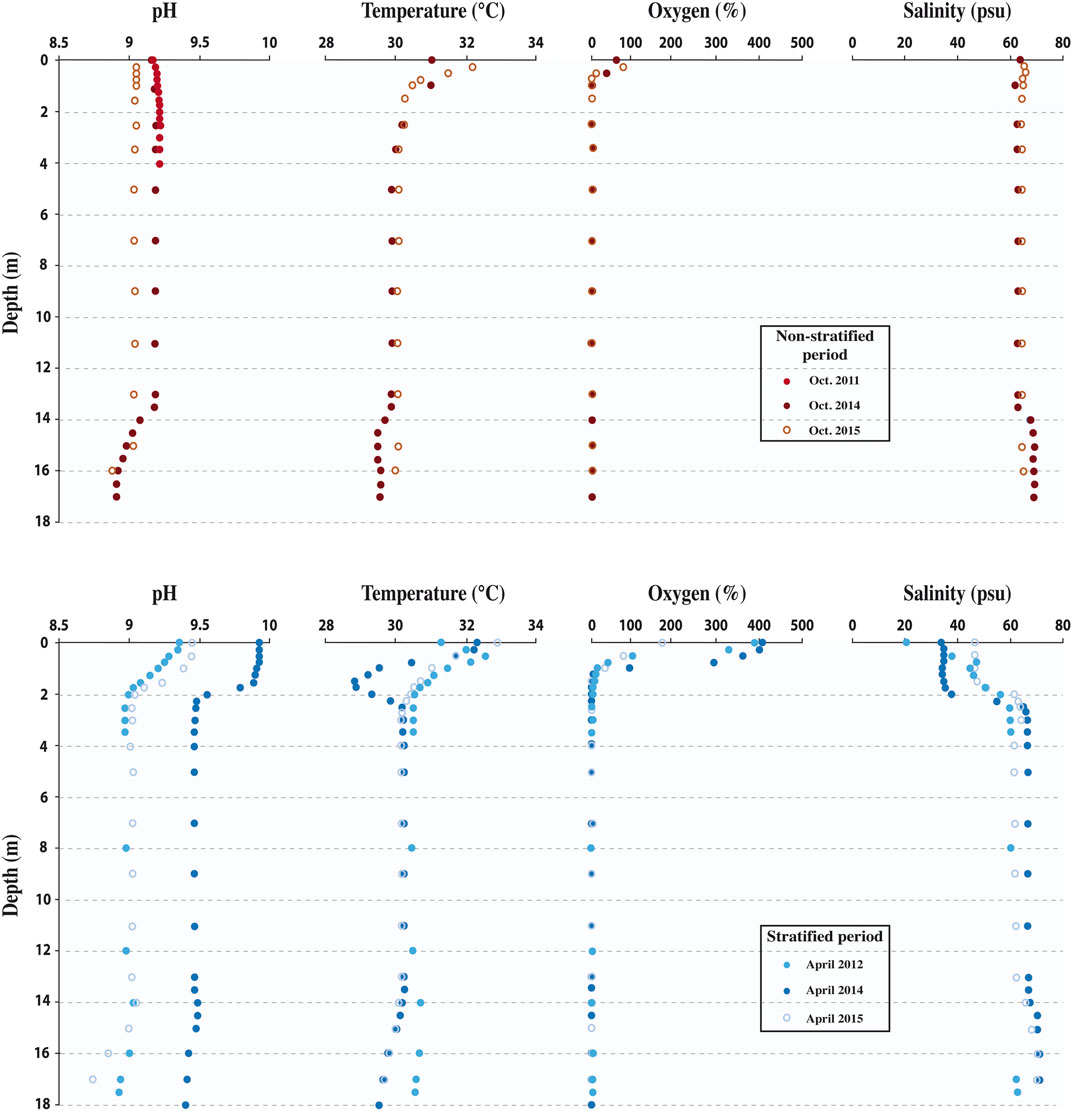
FIGURE 2. Vertical profiles of physical and chemical parameters in the Dziani Dzaha water column. pH, temperature (°C), O2 (%) and Salinity (psu) profiles during the non-stratified (2011, 2014 and 2015 in top panel) and stratified (2012, 2014 and 2015 in bottom panel) period (data from Sarazin et al., 2020).
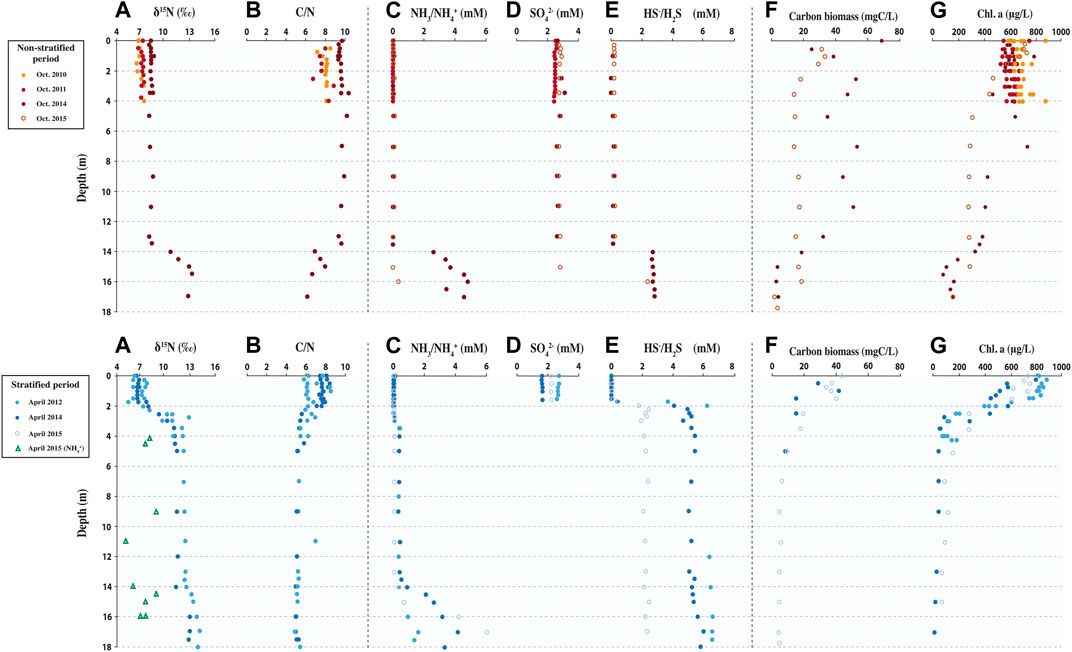
FIGURE 3. Compilation of biogeochemical data in the Dziani Dzaha water column, including δ15NSPM and δ15N of NH4+ (A), C/N ratio (B), (NH4+/NH3) (data from Sarazin et al., 2020) (C), (SO42−) and (HS−/H2S) (D,E) (data from Sarazin et al., 2020), carbon biomass of the two main photosynthetic microorganisms (data from Bernard et al., 2019) (F), and chlorophyll a (data this study and Leboulanger et al., 2017) (G), during the non-stratified (2011, 2014 and 2015 in top panel) and stratified (2012, 2014 and 2015 in bottom panel) period.
Methods
As shown by the above site description, many physical, chemical and biological parameters have been published previously [pH, T, Salinity, O2, NH4+/NH3 in Sarazin et al., 2020; SO42−, H2S/HS− in Sarazin et al., 2020; Chlorophyll a before 2012 in Leboulanger et al., 2017; carbon biomass associated to photosynthetic cell abundance in Bernard et al., 2019]. In this section we will thus describe only the sampling and the analytical methods used to acquire the new data presented here; chlorophyll a (for samples taken since 2012) and C/N and nitrogen isotope compositions.
Sampling were performed at the end of the rainy season (April) in 2012, 2014 and 2015, and at the end of the dry season (October-November) in 2011, 2014 and 2015. The “CLB” and “18 m” names refer to the water column stations where samples were collected, throughout 4.5 m depth and 18 m depth respectively, as shown on the Figure 1. Water samples were collected using a horizontal 1.2 L Niskin bottles along a vertical profile at the CLB and 18 m stations (Figure 1) every 0.25–0.50 m depth for the first 4.5 m of water column and either every 50 cm across the deep chemocline (between 13 and 15 m depth), or every 1–2 m between 5 and 13 m depth where no significant variation was observed on the profiles from the multiparameter probe. An aliquot of sampled water for each depth was filtered onto precombusted (450°C–4 h) 47 mm Whatman GF/F glass fibre filters (0.7 µm porosity) to recover suspended particulate matter for chlorophyll a, C/N and δ15N analyses. Several sediment cores serially numbered from the first survey were collected at different depths (Figure 1), freeze-dried, rinsed with deionized water, centrifuged three times, and finally freeze-dried and crushed down to <80 μm.
The concentration of chlorophyll a was analyzed after a two-steps extraction, the extract being then filtered and the filtrate analyzed spectrophotometrically at 400–750 nm (Leboulanger et al., 2017). The concentration of chlorophyll a was calculated according to Camacho et al. (2009). Nitrogen isotope and C/N measurements were performed on the same samples as the carbon isotope analysis reported in Cadeau et al. (2020) (i.e., suspended particles and sediments), but during a different run dedicated to the δ15N analyses and on bulk samples to avoid alterations of δ15N signal during the acid decarbonatation required for δ13Corg analyses (Brodie et al., 2011). Nitrogen isotope measurements of NH4+/NH3 were performed using the ammonium diffusion method (Sebilo et al., 2004), which is based on the conversion of NH4+ to NH3 by pH adjustments and subsequent trapping of the released NH3 onto glass fibre filter. Nitrogen species retained on glass fibre filter (i.e., suspended particles, dissolved nitrogen trapped onto (NH4)2SO4 form) and sediment samples were analyzed using a Flash EA1112 elemental analyser coupled to a Thermo Finnigan Deltaplus XP mass spectrometer via a Conflo IV interface (Thermo Fisher Scientific, Waltham, MA, United States). Nitrogen isotope ratios were calibrated against four internal standards of organic-rich soil or sediment included in the sample sequence and previously calibrated against the certified IAEA-N1 and IAEA-N2 international standards. The nitrogen isotopic signatures are expressed as ‰ relative to air with a reproducibility of ±0.3‰ (1σ). Routine replicate measurements on standards had internal deviations of ±0.15‰ (2σ) for δ15N values, and less than 4% of the measured value for the nitrogen content.
Results
In the Dziani Dzaha water column, the δ15N values of suspended particulate matter (δ15NSPM) ranged from 5.6 to 14.1‰, with an average value of 9.1 ± 2.6 ‰ (Figure 3A). When the lake was stratified, the δ15NSPM showed an average value of 6.9 ± 0.7‰ above the halocline that increased sharply across the halocline to an average value of 12.2 ± 1.1‰ below 2.5 m depth (Figure 3A). When the lake was non-stratified, a similar pattern was observed, but with a δ15NSPM average value of 7.4 ± 0.6‰ from the surface to the permanent deep chemocline at 14 m depth (Figure 3A) and an average value of 12.2 ± 1.1 ‰ below it. Sediment δ15N values ranged from 11.0 to 12.9‰ with an average value of 12.1 ± 0.6‰ for the sediment core C6 (collected at 18 m depth, Figure 1); from 8.3 to 12.5‰ with an average value of 10.9 ± 1.0‰ for the cores C1 and C4 (collected at 4 m depth, Figure 1); and from 4.3 to 12.1‰ with an average value of 8.9 ± 2.0‰ for the cores C2, C9 and C10 (collected at 1 m depth, Figures 1, 4). For all cores, sediment δ15N values were similar to the high δ15NSPM observed below the halocline or deep chemocline depending on the water column stratification, than those observed in NH4+/NH3 or surface water suspended particles (Figure 3A). The C/N ratio of suspended particulate matter seemed to be correlated to the δ15NSPM (Figure 5), with an average value of 5.5 ± 0.8 when the δ15NSPM was close to 12‰ , and an average value of 7.8 ± 1.3 when the δ15NSPM was close to 7‰ , in both stratified or non-stratified periods (Figures 3B, 5). Finally, during the stratified period in 2015, the δ15N of dissolved NH4+/NH3 showed an average value of 7.7 ± 0.9 ‰ (Figure 3A). It is worth noting here that the depth of variations in δ15N and C/N values are more closely tied the depth of the halocline and chemocline than to those of the variations in NH4+/NH3 concentration (Figure 3C).
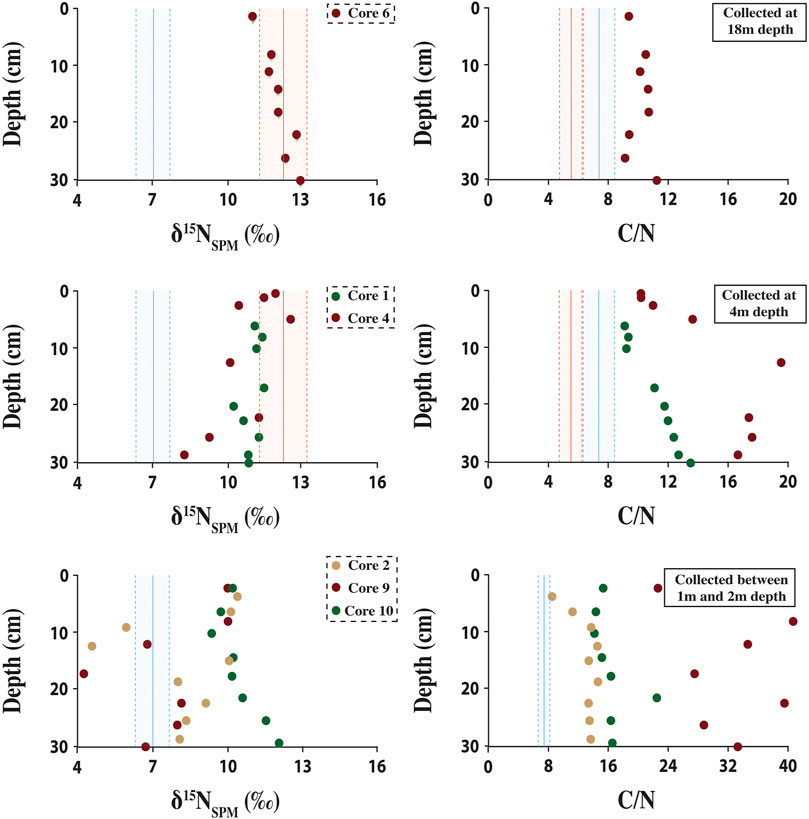
FIGURE 4. Nitrogen isotope compositions and C/N ratio in the Dziani Dzaha surface sediment. The dashed blue areas represent the δ15NSPM and C/N average values observed in the water column above 2 or 14 m depth during stratified or non-stratified period, respectively. The dashed red areas represent the δ15NSPM and C/N average values observed in the water column below the mentioned depths.
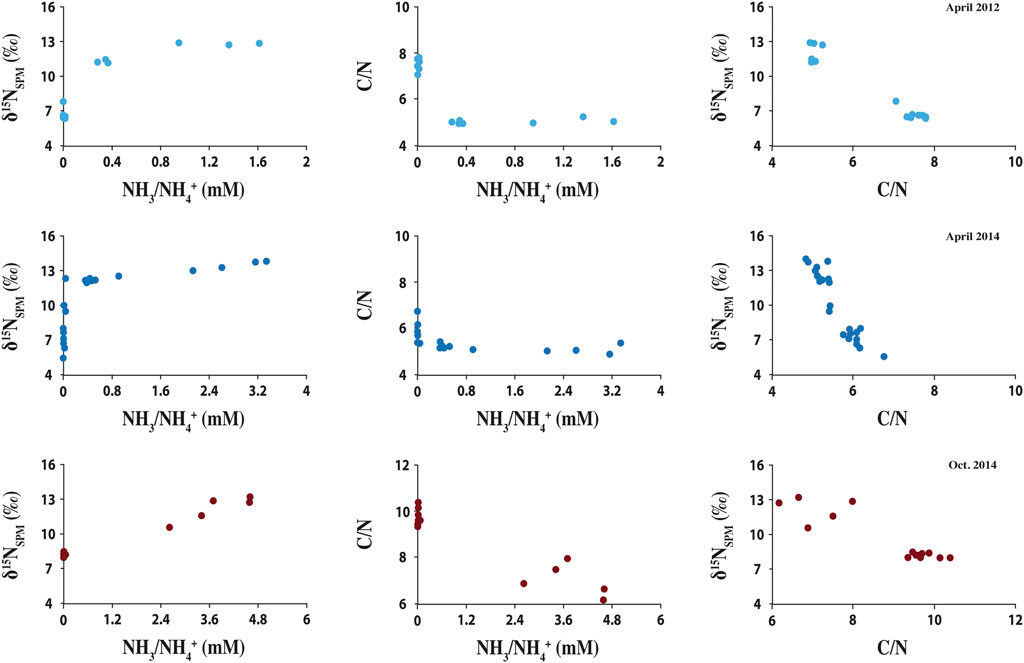
FIGURE 5. Cross-plots of δ15NSPM vs. (NH4+/NH3), C/N ratio vs. (NH4+/NH3), and δ15NSPM vs. C/N ratio in the Dziani Dzaha water column during the stratified (2012 and 2014) and non-stratified (2014) periods at the 18 m water column station.
Chlorophyll a content ranged from 6 to 875 μg/L within the water column (Figure 3G). When the lake was stratified, chlorophyll a content showed an average value of 704 ± 158 μg/L above the halocline, and decreased strongly to an average value of 115 ± 91 μg/L below 2 m-depth. When the lake was non-stratified, a similar pattern was observed with an average value of chlorophyll a content of 635 ± 75 μg/L from the surface to 4 m-depth, a decrease between 4 and 14 m-depth to an average value of 334 ± 53 μg/L, and a another below 14 m-depth to an average value of 158 ± 77 μg/L.
Discussion
The three main results of this study are that, 1) the δ15N of primary producers (given by the δ15NSPM from surface water during both stratified and non-stratified period) is on average of 7 ‰, 2) the δ15NSPM values are on average 5‰ higher in the euxinic (i.e., H2S/HS−-rich) waters (i.e., below 2 m or 14 m depths during stratified periods or non-stratified periods, respectively), and 3) the sedimentary δ15N values are closer to the δ15NSPM of euxinic bottom waters than to those of primary producers.
δ15N values as high as 7‰ for dissolved inorganic nitrogen and primary producers in lakes have most often been interpreted as resulting from denitrification, anammox and/or NH3 volatilization (e.g., Talbot and Johannessen, 1992; Lehmann et al., 2004; Bratkic et al., 2012; Menzel et al., 2013; Wenk et al., 2013; Wenk et al., 2014). Given that the Dziani Dzaha is both redox-stratified and alkaline, any combination these processes could account for the δ15N average value of 7‰ in primary producers and NH4+/NH3 (only measured in April 2015 for dissolved inorganic nitrogen). Addressing this question more thoroughly would require additional data, such as NH3 fluxes at the water/air interface, or denitrification and anammox activities and is beyond the scope of this study. We will thus focus here on the possible processes that could account for the marked increase in δ15NSPM values in the euxinic waters and their transfer to the sediment.
Several processes are typically considered as being involved in modifying the δ15N signature of primary producers during particulate matter sinking and/or deposition in surface sediments. They encompass 1) contribution of external sources of nitrogen to the particulate matter or sediment, 2) alteration of the δ15N of primary producers during remineralization and 3) contribution of organic matter from non-photosynthetic organisms living in the water column or sediment, such as heterotrophs or chemolithotrophs. As discussed below, we conclude that none of these processes seem to account for the N-isotope pattern observed in the Dziani Dzaha. Instead, we propose that it results from NH4+ assimilation by the main organisms that compose the biomass in euxinic waters, i.e., cyanobacteria, together with a bias in the preservation of these N-enriched cyanobacteria.
Contribution of External Sources of 15N-Enriched Nitrogen to the Euxinic Waters
Two types of external N-sources can be envisaged: an influx of dissolved nitrogen-rich fluids and/or a detrital input of terrestrial organic matter. An influx of dissolved nitrogen-rich fluid (from seawater or hydrothermal fluids) is unlikely based on physical and chemical measurements in the water column, no on-going water infiltrations were identified and, from a water balance point of view, this lake seems to be endoreic (Sarazin et al., 2020). Moreover, seawater entrance would only marginally affect the dissolved nitrogen budget and its δ15N because the concentration of dissolved nitrate in the Indian Ocean (i.e., 5–15 μM up to 200 m depth, NOAA, 2017) are quite low compared to the concentration of dissolved reduced nitrogen species in euxinic waters of the Dziani Dzaha (i.e., mM range Figure 2) and the nitrate δ15N in the Indian Ocean (∼+6‰) is very close to that of the lake (∼+7 ‰). For an external dissolved nitrogen source to contribute significantly to the lake NH4+/NH3 budget, it would thus need to be sourced from NH4+/NH3-rich hydrothermal fluids. However, that would still not explain the fact that in the euxinic and NH4+/NH3-rich waters the δ15N value of suspended particles and sediments are more positive than the dissolved NH4+/NH3.
The supply of detrital organic matter from the surrounding catchment is also very unlikely to be responsible for the higher δ15NSPM values of the euxinic waters and δ15Nsed values. The carbon and nitrogen contents and isotopic compositions of seven kinds of higher plants and soil sampled in the lake watershed (Table 1) exhibited δ15N values that were lower than the lake primary producers (<7‰), except for the rush sample with a δ15N value close to 9 ‰. This high value is best explained by the fact that rushes grow in the shallow waters of the lakeshore and are probably assimilating 15N-enriched nitrogen from the lake sediment pore waters. In addition, the measured plants δ13C and C/N values are significantly lower and higher, respectively, than those of suspended particulate matter and surface sediments (Figures 3, 4 and Table 1). A contribution of detrital organic matter to the suspended particulate matter or sediment would thus decrease their δ15N, rather than increase it. It would also decrease their δ13Corg and increase their C/N, as observed in some samples of the shallow cores (i.e., C2 and C9, Figure 4), which we accordingly explain by a contribution of detrital organic matter.
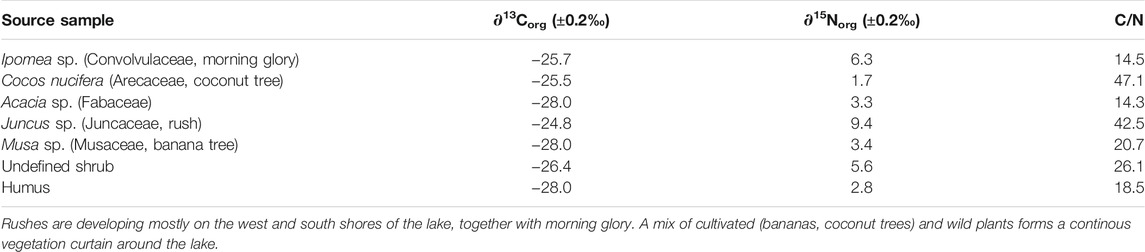
TABLE 1. δ13C, δ15N and C/N of organic terrestrial sources (Angiosperms leafs and soil humus) closely surrounding the Dziani Dzaha Lake.
Alteration of Particulate Matter δ15N During Their Mineralisation
Isotopic alteration due to organic matter mineralisation in modern settings was relatively well investigated (e.g., Lehmann et al., 2002; Robinson et al., 2012; Tesdal et al., 2013; Katsev and Crowe, 2015). The extent of organic matter degradation as well as the early diagenesis processes, and subsequently the extent of isotopic alteration of the primary nitrogen isotope signature, appear to depend on the oxygen exposure time, water depth and organic matter availability (e.g., Lehmann et al., 2002; Thunell et al., 2004; Robinson et al., 2012). So far, increases in both δ15NSPM and δ15Nsed of the order of a few per mil have only been reported in sinking particles in the deep ocean and have been interpreted as resulting from extensive organic matter remineralisation under oxic conditions (e.g., Lehmann et al., 2002; Gaye et al., 2009; Mobius et al., 2010; Mobius et al., 2011). It seems unlikely however that this process is applicable to the shallow and anoxic Dziani Dzaha for two reasons. First, 15N enrichment in sinking particles and in the surface sediments through organic matter mineralisation is usually associated with an increase in C/N ratio, as nitrogen is preferentially lost over carbon (Lehmann et al., 2002; Fenchel et al., 2012). In the Dziani Dzaha, as shown in Figure 4, the increase in δ15NSPM is not associated with an increase in the C/N ratio but rather decreased with depth from approximately 7 to 5. Second, to our knowledge, such an isotopic increase with depth has never been reported so far in lacustrine systems (e.g., Lehmann et al., 2004; Menzel et al., 2013; McLauchlan et al., 2013) or in modern anoxic marine environments (e.g., Thunell et al., 2004; Fulton et al., 2012). In these systems, when δ15N-depths profiles were available, increases in the δ15N of dissolved N species or suspended particles have been reported but they are either spatially limited to the vicinity of the chemocline (e.g., Cariaco Basin, Thunell et al., 2004; Black Sea, Fry et al., 1991; Lugano Lake, Wenk et al., 2014) or temporally limited to water column mixing events (e.g., Kinneret Lake, Hadas et al., 2009). They have been interpreted as resulting from denitrification and/or anammox together with assimilation of the enriched NO3− and/or NH4+ by chemoautotrophic organisms (Deutsch et al., 2001; Voss et al., 2001; Sigman et al., 2003; Thunell et al., 2004). These isotope signatures are however not transferred to the sinking particulate matter or the surface sediments, which both record the primary producer δ15N values (Thunell et al., 2004).
Contribution of Organic Matter From Non-photosynthetic Organisms Living in the Euxinic Waters
The decrease in C/N ratio within euxinic waters coincides with a strong change in biological diversity (Hugoni et al., 2018). In terms of abundance, the biological communities are dominated by two primary producers (the picoeukaryote Picocystis salinarum and the cyanobacterium Arthrospira fusiformis) above the halocline at 2 m depth during stratified periods, or above the deep chemocline at 14 m depth during non-stratified periods, and by bacteria and archaea in euxinic waters below the halocline or deep chemocline (Leboulanger et al., 2017; Hugoni et al., 2018). The C/N value close to 7 in surface waters is consistent with the predominantly cyanobacterial biomass (Godfrey and Glass, 2011). Indeed, previous work on continuous cultures of Arthrospira fusiformis from Lake Chitu (Ethiopia) reported that the C/N ratio was stable in this species, around 6.25 (Kebede and Ahlgren 1996). The generally lower C/N values in the euxinic waters, sometimes approaching 5, are consistent with a predominantly bacterial or archaeal biomass (Muller, 1977; They et al., 2017). From there, it is tempting to imagine that both the decrease in C/N and the increase in δ15NSPM observed in the euxinic waters could be linked to a higher proportion of bacterial and archaea biomass, which would be characterized by a more positive δ15NSPM values than the cyanobacterial biomass. However, even if in euxinic waters bacteria and archaea are predominant in terms of number of individuals compared to cyanobacteria (Hugoni et al., 2018), biomass estimations from cells abundance measurements and biovolumes show that cyanobacteria remain by far the main constituent of the biomass (Figure 3F) and are responsible of most of the primary production in the lake (Leboulanger et al., 2017). Therefore, in spite of their coincidence with a strong biodiversity change, the combined C/N decrease and δ15NSPM increase in euxinic waters are not directly related to it.
Ammonium Assimilation by Cyanobacteria in the Euxinic and Aphotic Bottom Waters
In this section, we investigate the possibility that the combined C/N decrease and δ15NSPM increase in the euxinic waters reflect NH4+ assimilation and storage by cyanobacteria (Figure 3). As previously shown in modern alkaline anoxic settings (e.g., Lonar Lake, Menzel et al., 2013), pH controls the dissolved reduced nitrogen speciation through the reaction of NH4+ dissociation to NH3 (i.e., Eq. 1 below, Li et al., 2012):
At isotope equilibrium the isotope exchange between NH4+ and NH3 is associated with a strong isotopic fractionation (i.e., of 45 ‰ at 23°C, Li et al., 2012), forming 15N-depleted NH3 and 15N-enriched NH4+. Considering an average temperature of 30°C in the NH4+-rich part of the Dziani Dzaha water column (Sarazin et al., 2020; Figure 2), and based on the equations proposed in Li et al. (2012), the pKa of acid-base couple (Eq. 1) is of 9.09 in the Dziani Dzaha conditions. Considering this and the minimum and maximum pH values observed in the NH4+-rich and euxinic waters of the Dziani Dzaha (i.e., close to 9 in April 2012 and 9.5 in April 2014), between 45 and 72% of the NH4+ is dissociated in NH3, enriching NH4+ in 15N by about 20‰ and 32‰ , respectively at pH 9 and 9.5, compared to the total dissolved inorganic nitrogen. These values represent a qualitative estimation of the dissolved NH4+ isotopic signatures range in equilibrium with ammonia, which strongly depends on variations of both pH values and total dissolved inorganic nitrogen δ15N.
Arthrospira fusiformis, the main cyanobacteria species that constitute most of the biomass in the Dziani Dzaha, is known to harbour aerotopes (gas vacuoles) that enhance cell buoyancy, and cyanophycin-rich granules increasing nitrogen intracellular storage capacity (Cellamare et al., 2018). These characteristics are consistent with a vertical migration process where cells increase in density during photosynthesis in lit layer, which makes them sink in the NH4+/NH3-rich water where they store nitrogen as cyanophycin or in phycobilisomes. When they reach aphotic waters, photosynthesis stops and respiration reduces their cell density, allowing them to move upward (Carey et al., 2012). However, when the lake is stratified and its deep waters are euxinic, this process could eventually lead to a death trap due the combination of the sulfide toxicity. The fact that the 15N-enrichment and C/N decrease of suspended particles is limited to the euxinic waters at times of stratification could thus reflect the assimilation of this 15N-enriched NH4+ by cyanobacteria, and their entrapment below the halocline. This would also be compatible with the delayed increase in NH3/NH4+ concentration in the first meter below the halocline (compared to CH4 and H2S+/H S−) where cyanobacteria might still be active enough to assimilate NH4+ efficiently. In addition, δ15NSPM in the euxinic and NH3/NH4+-rich waters shows an average value close to 12 ‰ (Figure 3), which represents an isotopic enrichment of only about 5 ‰ compared to the δ15NSPM observed in surface water despite the estimated δ15N values of dissolved NH4+ in the NH3/NH4+-rich waters comprised between 20‰ and 32 ‰. This is most likely related to the fact that NH4+ assimilation favours 14N uptake (Pennock et al., 1996; Vo et al., 2013) and that only up to approximately 29% of the total nitrogen in the 15N-enriched cyanobacterial biomass originated from assimilation of 15N-enriched NH4+ according to the change in C/N ratio. Finally, δ15NSPM values in the euxinic waters vary by only 1 ‰between 2012 and 2014 in spite of significant the variations of pH and NH4+/NH3 dissolved concentrations which impact of the δ15N of NH4+ may have balanced each other. Unfortunately, this cannot be assessed further here as in the absence of measurement of the total dissolved inorganic nitrogen δ15N in 2012 and 2014.
Preservation Bias Towards 15N-Enriched Cyanobacteria
Ammonium assimilation and storage by cyanobacteria, beyond accounting for the δ15NSPM increase in the euxinic waters, also explains the fact that the sediment δ15N is biased towards the δ15N of 15N-enriched cyanobacteria, even at shallow depths where the water column remains oxic. The high buoyancy of cyanobacteria that allows them to move vertically in the water column when they are alive and active, also prevents them from sinking quickly to the surface sediment when they are dead. This is consistent with the fact that although the Dziani Dzaha is a highly productive and shallow lacustrine system exhibiting highly favourable conditions for the preservation of organic matter (e.g., Leboulanger et al., 2017), the proportion of the net primary production preserved in the sediments is only of 2.9% (Cadeau et al., 2020), e.g., only slightly higher than the maximum value estimated in the stratified and euxinic Black Sea basin (i.e., 0.5–1.8%, Karl and Knauer., 1991).
As shown by the chlorophyll a and biomass data (Figures 3F,G), when the water column is sulfide-free (i.e., up to 2 or 14 m depth according to the stratified or non-stratified period, respectively), most cyanobacteria are active and the amount of biomass is stable with depth. Their high buoyancy, together with the wind agitation of the surface waters that propagates down to the lake bottom when it is non-stratified (as shown by the similarity between surface and bottom water temperatures and their high amplitude temporal changes, Sarazin et al., 2020), probably limits the export of organic matter to the sediment. Preferential organic matter export would thus probably occur from the bottom waters when the lake is density stratified and the mixing dynamics of bottom waters strongly reduced (as shown by the very dissimilar surface and bottom water temperatures with constant temperatures in bottom waters unaffected by the high frequency changes in surface temperatures, Sarazin et al., 2020), allowing the dead or weakened cyanobacteria to sink in the water column and accumulate in the sediment.
In the shallow parts of the lake (i.e., up to 2 m depth), cyanobacteria remain active all year long, even when they settle down onto the water/sediment interface as suggested by observation of a green surface layer in the flocs resting on the top of the shallow sediment cores. Since the water column is never euxinic and NH4+-rich at shallow depth, we would not expect shallow surface sediments to be 15N-enriched, and yet they are. It is most probable that the interstitial waters of the colloidal floc and surface sediments are probably anoxic and NH3/NH4+-rich and that 15N-enriched NH4+ is assimilated by cyanobacteria when they reach the floc, explaining the increase observed in the sedimentary δ15N values even at shallow sites.
Finally, NH4+ incorporation into clay minerals could also contribute to modifying the sediment δ15N towards more positive values (Muller, 1977). Indeed, the shallowest sediment cores (e.g., C10) contain half the amount of organic matter than those collected at 4 and 18 m depths (i.e., C4 and C6 respectively) (Milesi et al., 2019), and a higher clay mineral (e.g., saponite) content (Milesi et al., 2019). Pore waters pH being basic within the first 30 cm studied here (Milesi et al., 2019; Milesi et al., 2020), NH4+ is also 15N-enriched by isotope equilibrium with NH3. An incorporation of 15N-enriched ammonium into clay minerals by substituting for K+ could make the sediments isotopically heavier in all sediment cores (Muller, 1977; Stueken et al., 2019), especially into the core C10 presenting a higher clay mineral content (Milesi et al., 2019). Still, although this process cannot be ignored, it is probably not significant compared to the nitrogen input from primary producers (at the surface or deep in the water column), because organic matter content in all sediment cores remains significant and the proportion of ammonium potentially incorporated into the clay mineral fraction would represent only a small fraction of the overall clay mineral content.
Nitrogen isotopic signatures recorded in the Dziani Dzaha surface sediments would then result from a combination of 1) the cyanobacterial initial biomass with its original δ15N acquired from surface waters, 2) their nitrogen storage compartments with more positive δ15N values acquired through 15N-enriched ammonium assimilation in the euxinic part of the water column, in the floc or in the sediments interstitial waters, and possibly in a lesser extent 3) some abiotic incorporation of 15N-enriched ammonium into authigenic silicates.
Implication for δ15N Interpretations
As previously suggested, albeit for a different reason, these results confirm that basic pH conditions have a strong control on the sedimentary nitrogen isotopic signature, which hence represents an useful tool to track such geological settings over times (e.g., Stueken et al., 2019). However to date, the mechanisms envisioned to explain the overall 15N enrichment of sediment in modern or past basic lakes are NH4+ isotope equilibrium with NH3 coupled to NH3 volatilization into the atmosphere and/or denitrification or anammox (e.g., Menzel et al., 2013; Stueken et al., 2015; Stueken et al., 2019; Stueken et al., 2020). Our results demonstrate that additional processes to those commonly used to explain these high values can contribute to increase the sedimentary δ15N. Indeed, in the Dziani Dzaha Lake a significant isotopic 15N enrichment is observed at depths within the water column and/or directly within surface sediments. We propose here that it is mainly due to 15N-enriched NH4+ assimilation by cyanobacteria either in the deep-water column or in surface sediments. The most important aspect of this result is that the primary producer δ15N values observed in the surface water of the Dziani Dzaha Lake are not well recorded in surface sediments. This represents a strong contradiction with one of the main assumptions underlying the use of nitrogen isotope compositions as a tool for the reconstruction of the past nitrogen cycle: i.e., that sediment δ15N values record those acquired by primary producers, themselves recording the δ15N value of the nitrogen species assimilated in the photic zone (e.g., Ader et al., 2016). This hypothesis is well established for all previously studied modern continental platforms or anoxic basins, which are usually characterised by high export production, low oxygen content and high organic matter preservation (e.g., Pride et al., 1999; Altabet et al., 1999; Emmer and Thunell, 2000; Thunell and Kepple, 2004; Thunell et al., 2004). But our results show that it may not apply to ammonium replete environments or paleo-environments of the Precambrian, which are thought to have been inhabited by dominantly microbial ecosystems (e.g., Butterfield., 2015), in which cyanobacteria were responsible for the bulk of the primary productivity.
Depending on the water pH, the ability of cyanobacteria to assimilate ammonium when sinking in the water column may have various impacts on their initial δ15N. Although under basic settings, the large isotopic fractionation associated with NH4+ dissociation into NH3 leads to 15N enrichment through the assimilation of 15N-enriched NH4+, NH4+ assimilation is associated with an isotopic fractionation that favours 14N and is proportional to the NH4+ concentration (Pennock et al., 1996; Vo et al., 2013). Hence, under the neutral condition (i.e., without NH4+ dissociation into NH3), NH4+ assimilation could modify the cyanobacteria δ15N towards more negative isotopic values, leading, if ignored, to an underestimation of the assimilated nitrogen δ15N in such settings. For instance, in the Dziani Dzaha Lake the total dissolved nitrogen δ15N in the water column (i.e., NH4+ and NH3) exhibits an isotopic value close to 7‰ . If the pH values in the Dziani Dzaha were neutral, NH4+ assimilation could have modified the δ15N of primary producers towards negative values. These findings require further consideration about the reliability of paleoenvironmental evidences provided by the δ15N in such stratified and NH4+-rich settings.
Conclusion
The Dziani Dzaha is an atypical modern lacustrine system in which specific biological (e.g., predominantly cyanobacterial biomass) and chemical (e.g., low O2, high NH3/NH4+, euxinia and high pH) features appear to significantly modify both the C/N ratio and the nitrogen isotopic signature of primary producers during their export to the sediments.
Here we propose that this pattern is due to the assimilation and storage of 15N-enriched NH4+ by cyanobacteria as they sink through the euxinic part of the water column and settle in the surface sediments. This observation challenges the basic assumption that systematically underlies the use of this isotopic tool in paleoenvironmental reconstructions: i.e., that sediment δ15N values record those that the primary producers have acquired in the photic zone. Although atypical compared to modern environments, the Dziani Dzaha features are likely representative of the past, especially before the advent of multicellular organisms and the oxygenation of the atmosphere and the ocean during the Proterozoic (Logan et al., 1995; Lenton et al., 2014; Lyons et al., 2014; Butterfield, 2015). While in the Dziani Dzaha case the basic pH conditions are responsible for an isotopic enrichment of the nitrogen isotopic composition of organic matter, the same process in a neutral environment would lead to a 15N-depletion. Hence, more generally, NH4+ assimilation by cyanobacteria while they sink through an anoxic and NH4+-rich water column may shift the isotopic signatures of the sinking particulate matter to more positive or negative isotopic values than those initially acquired in surface waters, depending on the pH of the environment considered. This could have significant implications for the interpretation of δ15N in the sedimentary record and hence for our understanding of the evolution of the nitrogen cycle over geological time.
Data Availability Statement
The original contributions presented in the study are included in the article/Supplementary Material, further inquiries can be directed to the corresponding author.
Author Contributions
MA and CL designed the study and supervised PC PhD thesis. DJ, CC, and MA performed ammonium isotopic analyses. PC performed all other isotopic analyses and took the lead in the interpretation and writing the original draft. All authors contributed to at least one fieldtrip mission and provided critical feedback in shaping both the research results and the manuscript.
Conflict of Interest
The authors declare that the research was conducted in the absence of any commercial or financial relationships that could be construed as a potential conflict of interest.
Publisher’s Note
All claims expressed in this article are solely those of the authors and do not necessarily represent those of their affiliated organizations, or those of the publisher, the editors and the reviewers. Any product that may be evaluated in this article, or claim that may be made by its manufacturer, is not guaranteed or endorsed by the publisher.
References
Ader, M., Thomazo, C., Sansjofre, P., Busigny, V., Papineau, D., Laffont, R., et al. (2016). Interpretation of the Nitrogen Isotopic Composition of Precambrian Sedimentary Rocks: Assumptions and Perspectives. Chem. Geology. 429, 93–110. doi:10.1016/j.chemgeo.2016.02.010
Altabet, M. A., and Francois, R. (1994). Sedimentary Nitrogen Isotopic Ratio as a Recorder for Surface Ocean Nitrate Utilization. Glob. Biogeochem. Cycles 8 (1), 103–116. doi:10.1029/93gb03396
Altabet, M. A., Pilskaln, C., Thunell, R., Pride, C., Sigman, D., Chavez, F., et al. (1999). The Nitrogen Isotope Biogeochemistry of Sinking Particles from the Margin of the Eastern North Pacific. Deep Sea Res. Oceanographic Res. Pap. 46 (4), 655–679. doi:10.1016/s0967-0637(98)00084-3
Beaumont, V., and Robert, F. (1999). Nitrogen Isotope Ratios of Kerogens in Precambrian Cherts: a Record of the Evolution of Atmosphere Chemistry? Precambrian Res. 96 (1-2), 63–82. doi:10.1016/s0301-9268(99)00005-4
Bernard, C., Escalas, A., Villeriot, N., Agogué, H., Hugoni, M., Duval, C., et al. (2019). Very Low Phytoplankton Diversity in a Tropical saline-alkaline lake, with Co-dominance of Arthrospira Fusiformis (Cyanobacteria) and Picocystis Salinarum (Chlorophyta). Microb. Ecol. 78 (3), 603–617. doi:10.1007/s00248-019-01332-8
Bratkič, A., Šturm, M., Faganeli, J., and Ogrinc, N. (2012). Semi-annual Carbon and Nitrogen Isotope Variations in the Water Column of Lake Bled, NW Slovenia. Biogeosciences 9 (1), 1–11. doi:10.5194/bg-9-1-2012
Brodie, C. R., Casford, J. S., Lloyd, J. M., Leng, M. J., Heaton, T. H., Kendrick, C. P., et al. (2011). Evidence for Bias in C/N, δ13C and δ15N Values of Bulk Organic Matter, and on Environmental Interpretation, from a lake Sedimentary Sequence by Pre-analysis Acid Treatment Methods. Quat. Sci. Rev. 30 (21-22), 3076–3087. doi:10.1016/j.quascirev.2011.07.003
Brunner, B., Contreras, S., Lehmann, M. F., Matantseva, O., Rollog, M., Kalvelage, T., et al. (2013). Nitrogen Isotope Effects Induced by Anammox Bacteria. Proc. Natl. Acad. Sci. 110 (47), 18994–18999. doi:10.1073/pnas.1310488110
Butterfield, N. J. (2015). Early Evolution of the Eukaryota. Palaeontology 58 (1), 5–17. doi:10.1111/pala.12139
Cadeau, P., Jézéquel, D., Leboulanger, C., Fouilland, E., Le Floc'h, E., Chaduteau, C., et al. (2020). Carbon Isotope Evidence for Large Methane Emissions to the Proterozoic Atmosphere. Sci. Rep. 10 (1), 18186. doi:10.1038/s41598-020-75100-x
Camacho, C., Coulouris, G., Avagyan, V., Ma, N., Papadopoulos, J., Bealer, K., et al. (2009). BLAST+: Architecture and Applications. BMC bioinformatics 10 (1), 421–429. doi:10.1186/1471-2105-10-421
Canfield, D. E., Glazer, A. N., and Falkowski, P. G. (2010). The Evolution and Future of Earth's Nitrogen Cycle. science 330 (6001), 192–196. doi:10.1126/science.1186120
Carey, C. C., Ibelings, B. W., Hoffmann, E. P., Hamilton, D. P., and Brookes, J. D. (2012). Eco-physiological Adaptations that Favour Freshwater Cyanobacteria in a Changing Climate. Water Res. 46 (5), 1394–1407. doi:10.1016/j.watres.2011.12.016
Cellamare, M., Duval, C., Drelin, Y., Djediat, C., Touibi, N., Agogué, H., et al. (2018). Characterization of Phototrophic Microorganisms and Description of New Cyanobacteria Isolated from the saline-alkaline Crater-lake Dziani Dzaha (Mayotte, Indian Ocean). FEMS Microbiol. Ecol. 94 (8), fiy108. doi:10.1093/femsec/fiy108
Chen, F., Zhang, L., Yang, Y., and Zhang, D. (2008). Chemical and Isotopic Alteration of Organic Matter during Early Diagenesis: Evidence from the Coastal Area Off-Shore the Pearl River Estuary, south China. J. Mar. Syst. 74 (1-2), 372–380. doi:10.1016/j.jmarsys.2008.02.004
Deutsch, C., Gruber, N., Key, R. M., Sarmiento, J. L., and Ganachaud, A. (2001). Denitrification and N2fixation in the Pacific Ocean. Glob. Biogeochem. Cycles 15 (2), 483–506. doi:10.1029/2000gb001291
Emmer, E., and Thunell, R. C. (2000). Nitrogen Isotope Variations in Santa Barbara Basin Sediments: Implications for Denitrification in the Eastern Tropical North Pacific during the Last 50,000 Years. Paleoceanography 15 (4), 377–387. doi:10.1029/1999pa000417
Fenchel, T., Blackburn, H., King, G. M., and Blackburn, T. H. (2012). Bacterial Biogeochemistry: The Ecophysiology of mineral Cycling. Cambridge: Academic Press.
Fry, B., Jannasch, H. W., Molyneaux, S. J., Wirsen, C. O., Muramoto, J. A., and King, S. (1991). Stable Isotope Studies of the Carbon, Nitrogen and Sulfur Cycles in the Black Sea and the Cariaco Trench. Deep Sea Research Part A. Deep Sea Res. A. Oceanographic Res. Pap. 38, S1003–S1019. doi:10.1016/s0198-0149(10)80021-4
Fuchsman, C. A., Murray, J. W., and Konovalov, S. K. (2008). Concentration and Natural Stable Isotope Profiles of Nitrogen Species in theBlack Sea. Mar. Chem. 111 (1-2), 90–105. doi:10.1016/j.marchem.2008.04.009
Fulton, J. M., Arthur, M. A., and Freeman, K. H. (2012). Black Sea Nitrogen Cycling and the Preservation of Phytoplankton δ15N Signals during the Holocene. Glob. Biogeochem. Cycles 26 (2), 1–15. doi:10.1029/2011gb004196
Galbraith, E. D., Sigman, D. M., Robinson, R. S., and Pedersen, T. F. (2008). Nitrogen in Past marine Environments. Nitrogen Mar. Environ. 2, 1497–1535. doi:10.1016/b978-0-12-372522-6.00034-7
Gaye, B., Wiesner, M. G., and Lahajnar, N. (2009). Nitrogen Sources in the South China Sea, as Discerned from Stable Nitrogen Isotopic Ratios in Rivers, Sinking Particles, and Sediments. Mar. Chem. 114 (3-4), 72–85. doi:10.1016/j.marchem.2009.04.003
Godfrey, L. V., and Glass, J. B. (2011). The Geochemical Record of the Ancient Nitrogen Cycle, Nitrogen Isotopes, and Metal Cofactors. Methods Enzymol. 486, 483–506. doi:10.1016/b978-0-12-381294-0.00022-5
Hadas, O., Altabet, M. A., and Agnihotri, R. (2009). Seasonally Varying Nitrogen Isotope Biogeochemistry of Particulate Organic Matter in Lake Kinneret, Israel. Limnol. Oceanogr. 54 (1), 75–85. doi:10.4319/lo.2009.54.1.0075
Higgins, M. B., Robinson, R. S., Husson, J. M., Carter, S. J., and Pearson, A. (2012). Dominant Eukaryotic export Production during Ocean Anoxic Events Reflects the Importance of Recycled NH4+. Proc. Natl. Acad. Sci. 109 (7), 2269–2274. doi:10.1073/pnas.1104313109
Hugoni, M., Escalas, A., Bernard, C., Nicolas, S., Jézéquel, D., Vazzoler, F., et al. (2018). Spatiotemporal Variations in Microbial Diversity across the Three Domains of Life in a Tropical Thalassohaline lake (Dziani Dzaha, Mayotte Island). Mol. Ecol. 27 (23), 4775–4786. doi:10.1111/mec.14901
Karl, D. M., and Knauer, G. A. (1991). Microbial Production and Particle Flux in the Upper 350 M of the Black Sea. Deep Sea Research Part A. Deep Sea Res. Part A. Oceanographic Res. Pap. 38, S921–S942. doi:10.1016/s0198-0149(10)80017-2
Katsev, S., and Crowe, S. A. (2015). Organic Carbon Burial Efficiencies in Sediments: The Power Law of Mineralization Revisited. Geology 43 (7), 607–610. doi:10.1130/g36626.1
Kebede, E., and Ahlgren, G. (1996). Optimum Growth Conditions and Light Utilization Efficiency of Spirulina Platensis (= Arthrospira Fusiformis) (Cyanophyta) from Lake Chitu, Ethiopia. Hydrobiologia 332 (2), 99–109. doi:10.1007/bf00016689
Leboulanger, C., Agogué, H., Bernard, C., Bouvy, M., Carré, C., Cellamare, M., et al. (2017). Microbial Diversity and Cyanobacterial Production in Dziani Dzaha Crater lake, a Unique Tropical Thalassohaline Environment. PLoS One 12 (1), e0168879. doi:10.1371/journal.pone.0168879
Lehmann, M. F., Bernasconi, S. M., Barbieri, A., and McKenzie, J. A. (2002). Preservation of Organic Matter and Alteration of its Carbon and Nitrogen Isotope Composition during Simulated and In Situ Early Sedimentary Diagenesis. Geochimica et Cosmochimica Acta 66 (20), 3573–3584. doi:10.1016/s0016-7037(02)00968-7
Lehmann, M. F., Sigman, D. M., and Berelson, W. M. (2004). Coupling the 15N/14N and 18O/16O of Nitrate as a Constraint on Benthic Nitrogen Cycling. Mar. Chem. 88 (1-2), 1–20. doi:10.1016/j.marchem.2004.02.001
Lenton, T. M., Boyle, R. A., Poulton, S. W., Shields-Zhou, G. A., and Butterfield, N. J. (2014). Co-evolution of Eukaryotes and Ocean Oxygenation in the Neoproterozoic Era. Nat. Geosci 7 (4), 257–265. doi:10.1038/ngeo2108
Li, L., Lollar, B. S., Li, H., Wortmann, U. G., and Lacrampe-Couloume, G. (2012). Ammonium Stability and Nitrogen Isotope Fractionations for -NH3(aq)-NH3(gas) Systems at 20-70°C and pH of 2-13: Applications to Habitability and Nitrogen Cycling in Low-Temperature Hydrothermal Systems. Geochimica et Cosmochimica Acta 84, 280–296. doi:10.1016/j.gca.2012.01.040
Logan, G. A., Hayes, J. M., Hieshima, G. B., and Summons, R. E. (1995). Terminal Proterozoic Reorganization of Biogeochemical Cycles. Nature 376 (6535), 53–56. doi:10.1038/376053a0
Luo, G., Junium, C. K., Izon, G., Ono, S., Beukes, N. J., Algeo, T. J., Cui, Y., Xie, S., and Summons, R. E. (2018). Nitrogen Fixation Sustained Productivity in the Wake of the Palaeoproterozoic Great Oxygenation Event. Nat. Commun. 9 (1), 978–979. doi:10.1038/s41467-018-03361-2
Lyons, T. W., Reinhard, C. T., and Planavsky, N. J. (2014). The Rise of Oxygen in Earth's Early Ocean and Atmosphere. Nature 506 (7488), 307–315. doi:10.1038/nature13068
McLauchlan, K. K., Williams, J. J., Craine, J. M., and Jeffers, E. S. (2013). Changes in Global Nitrogen Cycling during the Holocene Epoch. Nature 495 (7441), 352–355. doi:10.1038/nature11916
Menzel, P., Gaye, B., Wiesner, M. G., Prasad, S., Stebich, M., Das, B. K., et al. (2013). Influence of Bottom Water Anoxia on Nitrogen Isotopic Ratios and Amino Acid Contributions of Recent Sediments from Small Eutrophic Lonar Lake, central India. Limnol. Oceanogr. 58 (3), 1061–1074. doi:10.4319/lo.2013.58.3.1061
Mettam, C., Zerkle, A. L., Claire, M. W., Prave, A. R., Poulton, S. W., and Junium, C. K. (2019). Anaerobic Nitrogen Cycling on a Neoarchaean Ocean Margin. Earth Planet. Sci. Lett. 527, 115800. doi:10.1016/j.epsl.2019.115800
Milesi, V. P., Debure, M., Marty, N. C. M., Capano, M., Jézéquel, D., Steefel, C., et al. (2020). Early Diagenesis of Lacustrine Carbonates in Volcanic Settings: the Role of Magmatic CO2 (Lake Dziani Dzaha, Mayotte, Indian Ocean). ACS Earth Space Chem. 4 (3), 363–378. doi:10.1021/acsearthspacechem.9b00279
Milesi, V. P., Jézéquel, D., Debure, M., Cadeau, P., Guyot, F., Sarazin, G., et al. (2019). Formation of Magnesium‐smectite during Lacustrine Carbonates Early Diagenesis: Study Case of the Volcanic Crater lake Dziani Dzaha (Mayotte–Indian Ocean). Sedimentology 66 (3), 983–1001. doi:10.1111/sed.12531
Möbius, J., Gaye, B., Lahajnar, N., Bahlmann, E., and Emeis, K. C. (2011). Influence of Diagenesis on Sedimentary δ15N in the Arabian Sea over the Last 130 Kyr. Mar. Geology. 284 (1-4), 127–138. doi:10.1016/j.margeo.2011.03.013
Möbius, J. (2013). Isotope Fractionation during Nitrogen Remineralization (Ammonification): Implications for Nitrogen Isotope Biogeochemistry. Geochimica et Cosmochimica Acta 105, 422–432. doi:10.1016/j.gca.2012.11.048
Möbius, J., Lahajnar, N., and Emeis, K. C. (2010). Diagenetic Control of Nitrogen Isotope Ratios in Holocene Sapropels and Recent Sediments from the Eastern Mediterranean Sea. Biogeosciences 7 (11), 3901–3914. doi:10.5194/bg-7-3901-2010
Müller, P. J. (1977). CN Ratios in Pacific Deep-Sea Sediments: Effect of Inorganic Ammonium and Organic Nitrogen Compounds Sorbed by Clays. Geochimica et Cosmochimica Acta 41 (6), 765–776.
NOAA (2017). National Oceanic and Atmospheric Administration. Available at: https://www.nodc.noaa.gov/.
Papineau, D., Purohit, R., Goldberg, T., Pi, D., Shields, G. A., Bhu, H., et al. (2009). High Primary Productivity and Nitrogen Cycling after the Paleoproterozoic Phosphogenic Event in the Aravalli Supergroup, India. Precambrian Res. 171 (1-4), 37–56. doi:10.1016/j.precamres.2009.03.005
Pennock, J. R., Velinsky, D. J., Ludlam, J. M., Sharp, J. H., and Fogel, M. L. (1996). Isotopic Fractionation of Ammonium and Nitrate during Uptake by Skeletonema Costatum : Implications for δ15N Dynamics under Bloom Conditions. Limnol. Oceanogr. 41 (3), 451–459. doi:10.4319/lo.1996.41.3.0451
Pride, C., Thunell, R., Sigman, D., Keigwin, L., Altabet, M., and Tappa, E. (1999). Nitrogen Isotopic Variations in the Gulf of California since the Last Deglaciation: Response to Global Climate Change. Paleoceanography 14 (3), 397–409. doi:10.1029/1999pa900004
Quan, T. M., Wright, J. D., and Falkowski, P. G. (2013). Co-variation of Nitrogen Isotopes and Redox States through Glacial-Interglacial Cycles in the Black Sea. Geochimica et Cosmochimica Acta 112, 305–320. doi:10.1016/j.gca.2013.02.029
Robinson, R. S., Kienast, M., Luiza Albuquerque, A., Altabet, M., Contreras, S., De Pol Holz, R., et al. (2012). A Review of Nitrogen Isotopic Alteration in marine Sediments. Paleoceanography 27 (4), 1–13. doi:10.1029/2012pa002321
Sarazin, G., Jezequel, D., Leboulanger, C., Fouilland, E., Le Floc’h, E., Bouvy, M., et al. (2020). Geochemistry of an Endorheic Thalassohaline Ecosystem: the Dziani Dzaha Crater lake (Mayotte Archipelago, Indian Ocean). Comptes Rendus. Géoscience 352 (8), 559–577. doi:10.5802/crgeos.43
Sebilo, M., Mayer, B., Grably, M., Billiou, D., and Mariotti, A. (2004). The Use of the 'Ammonium Diffusion' Method for δ15N-NH4+ and δ15N-NO3 - Measurements: Comparison with Other Techniques. Environ. Chem. 1 (2), 99–103. doi:10.1071/en04037
Sigman, D. M., Karsh, K. L., and Casciotti, K. L. (2009). “Ocean Process Tracers: Nitrogen Isotopes in the Ocean,” in Encyclopedia of Ocean Sciences. 2nd Edition (Cambridge: Academic Press), 40–54. doi:10.1016/b978-012374473-9.00632-9
Sigman, D. M., Robinson, R., Knapp, A. N., Van Geen, A., McCorkle, D. C., Brandes, J. A., et al. (2003). Distinguishing between Water Column and Sedimentary Denitrification in the Santa Barbara Basin Using the Stable Isotopes of Nitrate. Geochem. Geophys. Geosystems 4 (5), 1–20. doi:10.1029/2002gc000384
Stüeken, E. E., Tino, C., Arp, G., Jung, D., and Lyons, T. W. (2020). Nitrogen Isotope Ratios Trace High-pH Conditions in a Terrestrial Mars Analog Site. Sci. Adv. 6 (9), eaay3440. doi:10.1126/sciadv.aay3440
Stüeken, E. E., Buick, R., and Schauer, A. J. (2015). Nitrogen Isotope Evidence for Alkaline Lakes on Late Archean Continents. Earth Planet. Sci. Lett. 411, 1–10. doi:10.1016/j.epsl.2014.11.037
Stüeken, E. E., Kipp, M. A., Koehler, M. C., and Buick, R. (2016). The Evolution of Earth's Biogeochemical Nitrogen Cycle. Earth-Science Rev. 160, 220–239. doi:10.1016/j.earscirev.2016.07.007
Stüeken, E. E., Martinez, A., Love, G., Olsen, P. E., Bates, S., and Lyons, T. W. (2019). Effects of pH on Redox Proxies in a Jurassic Rift lake: Implications for Interpreting Environmental Records in Deep Time. Geochimica et Cosmochimica Acta 252, 240–267. doi:10.1016/j.gca.2019.03.014
Talbot, M. R., and Johannessen, T. (1992). A High Resolution Palaeoclimatic Record for the Last 27,500 Years in Tropical West Africa from the Carbon and Nitrogen Isotopic Composition of Lacustrine Organic Matter. Earth Planet. Sci. Lett. 110 (1-4), 23–37. doi:10.1016/0012-821x(92)90036-u
Tesdal, J.-E., Galbraith, E. D., and Kienast, M. (2013). Nitrogen Isotopes in Bulk marine Sediment: Linking Seafloor Observations with Subseafloor Records. Biogeosciences 10 (1), 101–118. doi:10.5194/bg-10-101-2013
They, N. H., Amado, A. M., and Cotner, J. B. (2017). Redfield Ratios in Inland Waters: Higher Biological Control of C:N:P Ratios in Tropical Semi-arid High Water Residence Time Lakes. Front. Microbiol. 8, 1505. doi:10.3389/fmicb.2017.01505
Thunell, R. C., and Kepple, A. B. (2004). Glacial‐Holocene δ15N Record from the Gulf of Tehuantepec, Mexico: Implications for Denitrification in the Eastern Equatorial Pacific and Changes in Atmospheric N2O. Glob. Biogeochem. Cycles 18 (1), 1–12. doi:10.1029/2002gb002028
Thunell, R. C., Sigman, D. M., Muller‐Karger, F., Astor, Y., and Varela, R. (2004). Nitrogen Isotope Dynamics of the Cariaco Basin, Venezuela. Glob. Biogeochem. Cycles 18 (3), 1–15. doi:10.1029/2003gb002185
Vo, J., Inwood, W., Hayes, J. M., and Kustu, S. (2013). Mechanism for Nitrogen Isotope Fractionation during Ammonium Assimilation by Escherichia coli K12. Proc. Natl. Acad. Sci. 110 (21), 8696–8701. doi:10.1073/pnas.1216683110
Voss, M., Dippner, J. W., and Montoya, J. P. (2001). Nitrogen Isotope Patterns in the Oxygen-Deficient Waters of the Eastern Tropical North Pacific Ocean. Deep Sea Res. Part Oceanographic Res. Pap. 48 (8), 1905–1921. doi:10.1016/s0967-0637(00)00110-2
Wenk, C. B., Blees, J., Zopfi, J., Veronesi, M., Bourbonnais, A., Schubert, C. J., et al. (2013). Anaerobic Ammonium Oxidation (Anammox) Bacteria and Sulfide-dependent Denitrifiers Coexist in the Water Column of a Meromictic South-alpine lake. Limnol. Oceanogr. 58 (1), 1–12. doi:10.4319/lo.2013.58.1.0001
Wenk, C. B., Zopfi, J., Blees, J., Veronesi, M., Niemann, H., and Lehmann, M. F. (2014). Community N and O Isotope Fractionation by Sulfide-dependent Denitrification and Anammox in a Stratified Lacustrine Water Column. Geochimica et cosmochimica acta 125, 551–563. doi:10.1016/j.gca.2013.10.034
Yang, J., Junium, C. K., Grassineau, N. V., Nisbet, E. G., Izon, G., Mettam, C., et al. (2019). Ammonium Availability in the Late Archaean Nitrogen Cycle. Nat. Geosci. 12 (7), 553–557. doi:10.1038/s41561-019-0371-1
Zhang, X., Sigman, D. M., Morel, F. M. M., and Kraepiel, A. M. L. (2014). Nitrogen Isotope Fractionation by Alternative Nitrogenases and Past Ocean Anoxia. Proc. Natl. Acad. Sci. USA 111 (13), 4782–4787. doi:10.1073/pnas.1402976111
Keywords: nitrogen isotopes, Dziani Dzaha, ammonium assimilation, pH, basic condition, alkaline lake, 15N enrichment
Citation: Cadeau P, Ader M, Jézéquel D, Chaduteau C, Sarazin G, Bernard C and Leboulanger C (2021) Nitrogen Isotope Discrepancy Between Primary Producers and Sediments in an Anoxic and Alkaline Lake. Front. Earth Sci. 9:787386. doi: 10.3389/feart.2021.787386
Received: 30 September 2021; Accepted: 26 November 2021;
Published: 23 December 2021.
Edited by:
Philippe Claeys, Vrije Universiteit Brussel, BelgiumReviewed by:
William Patrick Gilhooly III, Indiana University—Purdue University Indianapolis, United StatesIchiro Tayasu, Research Institute for Humanity and Nature, Japan
Copyright © 2021 Cadeau, Ader, Jézéquel, Chaduteau, Sarazin, Bernard and Leboulanger. This is an open-access article distributed under the terms of the Creative Commons Attribution License (CC BY). The use, distribution or reproduction in other forums is permitted, provided the original author(s) and the copyright owner(s) are credited and that the original publication in this journal is cited, in accordance with accepted academic practice. No use, distribution or reproduction is permitted which does not comply with these terms.
*Correspondence: Pierre Cadeau, cGllcnJlLmNhZGVhdUBnbWFpbC5jb20=