- 1School of Geoscience and Technology, Southwest Petroleum University, Chengdu, China
- 2Department of Railway Engineering, Sichuan College of Architectural Technology, Chengdu, China
Preservation conditions are the key factors that determine the effective accumulation of shale gas. The damage of faults formed by differential structures to the roof and floor and the shielding of lateral edges are the direct reasons for the difference in preservation conditions. Taking the organic-rich shale of the Wufeng–Longmaxi Formation in the south of the Sichuan Basin as an example, this paper reveals different types of shale gas-rich structures by using typical seismic profiles and puts forward the main controlling factors of different gas-rich structures and their influence on preservation. The results show that three kinds of gas-rich structures are developed in the Wufeng–Longmaxi Formation in southern Sichuan: positive type, negative type, and fault transformed slope type. The basin is dominated by a wide and gentle syncline, fault spreading fold, and low scope concealed anticlines. Wide and gentle anticline, arc anticline, and fault transformation slope are developed at the basin edge. Fault sealing is the main controlling factor for the preservation of shale gas in wide and gentle anticlines. The main controlling factors for the preservation of circular arc anticlines and hidden anticlines are anticline curvature and the distance between faults. The preservation of shale gas in a syncline is mainly controlled because it includes formation buried depth, foliation development degree, and formation dip angle. The preservation of fault transformed syncline is mainly affected by formation buried depth, dip angle, and fault sealing. Foliation and faults form a three-dimensional migration system, which jointly controls the intensity of gas escape. Positive structures such as wide and gentle anticline and circular arc anticline at the basin edge, and deep buried gentle syncline and low scope concealed anticline in the basin are favorable shale gas-rich structures.
Introduction
The contradiction between oil and gas demand growth and insufficient supply stimulates oil and gas exploration to enter deeper and wider fields (Jarvie et al., 2007; Ambrose et al., 2010; Fan et al., 2020; Zhang et al., 2020; Chen et al., 2021; He et al., 2021). As an important replacement area for unconventional oil and gas tools (Wang B. et al., 2021), commercial oil and gas reservoirs have been discovered in tight sandstone gas, super-heavy oil, coalbed methane, and other fields, which relieved the pressure on energy and adjusted the energy structure. The proportion of oil and gas production is increasing year by year (Pollastro, et al., 2007; Curtis et al., 2012). Organic-rich shale is considered as a special rock integrating the functions of “source, reservoir and cover” because of its characteristics of low porosity and low permeability (Jarvie et al., 2007; Yin et al., 2018; Xu et al., 2020). Hydrocarbon gas has the characteristics of “nearby adsorption and in-situ accumulation” in shale. The migration of shale gas is usually promoted by concentration diffusion and “saturated piston” (Borjigin et al., 2017), the preservation conditions determine the difference in gas-bearing properties of shale, and it is also a key indicator for optimizing favorable areas for shale exploration (Ge et al., 2021). The fault development and different structural styles caused by differential tectonic movement result in the division of favorable preservation areas, which restricts the exploration and development of shale gas. Therefore, it is indispensable to study the impact of structural deformation differences on shale gas preservation (Guo et al., 2017), The marine organic-rich shale of the Wufeng–Longmaxi Formation in the south of the Sichuan Basin has the characteristics of a wide distribution area, moderate burial, high effective thickness, high total organic carbon (TOC) content, high thermal evolution degree, high brittle mineral content, and high gas-bearing property. The “source-reservoir” condition is superior, and it is the key target area of shale gas exploration in the next stage (Ma and Xie, 2018; Shi et al., 2021). High yield shale gas flow has been obtained in Changning, Weiyuan area, but the gas-bearing properties and gas production of different single wells in the same block vary greatly (Chen et al., 2018), which is mainly due to the superposition and transformation of multistage tectonic movements such as the Indosinian movement, Yanshan movement, and Himalayan movement in southern Sichuan (Guo and Zhang, 2014).
In the process of shale gas in the Sichuan Basin, the predecessors pointed out that the difference in preservation conditions is the key factor for the formation of the “dual enrichment” of the Lower Paleozoic marine shale in the Sichuan Basin (Ren et al., 2016; Yuan et al., 2019). At the same time, it is pointed out that structural elements are the key factors restricting shale gas preservation. The difference of gas-bearing property between the DY2 well and DY1 well in the Dingshan area is mainly caused by the difference in fault development scale and sealing property (Fan et al., 2018). In the analysis of the paleotectonic stress field and preservation conditions in the Changning area, it is confirmed that the faults formed by the Yanshan movement and Himalayan movement are the main fracture forming period (Wang et al., 2017; Fan et al., 2020b). Fault sealing is the direct reason affecting the preservation conditions (Awdal et al., 2013; Gale et al., 2014; Li et al., 2019; Xie et al., 2019; Hou et al., 2020; Li et al., 2021a; Li, 2021; Qie et al., 2021; Wang and Wang, 2021); the distribution of faults and fractures is predicted by means of geophysical and stress field simulation. Combined with the analysis of shale gas-bearing property, it is pointed out that the main reasons for the difference of shale gas-bearing property are the buried depth of stratum, the development of faults, and the difference of structural style (Liu P. et al., 2018; Hu et al., 2018; Guo et al., 2021; Shan et al., 2021), Combined with the analysis of the Jiaoshiba area successfully developed in Southeast Sichuan, it is considered that under medium burial depth, the high points of the gentle anticline and arc anticline are favorable accumulation and direction areas of shale gas.
However, due to the difference in shale gas exploration degree and the lack of three-dimensional seismic resources, the evaluation of preservation conditions is often concentrated in the same block (Li et al., 2021b; Wang H. et al., 2021; Hadded et al., 2021; Hou et al., 2021). The differences in gas-rich structural types and preservation conditions in different structural areas outside the basin, at the edge of the basin, and in the basin still need to be further analyzed, which is the basis for the optimization of favorable areas for shale gas. With the deepening of shale gas exploration, in recent years, there are more detailed three-dimensional seismic data, which provide conditions for comparative analysis of structural style differences in different gas-producing areas and their impact on shale gas preservation. Therefore, based on the seismic profiles of typical wells in different structural areas, this paper compares and analyzes the typical shale gas-rich structural characteristics of shale gas-producing areas from basin edge to basin, combined with the differences of shale gas-bearing properties. This paper discusses the migration mode and preservation main controlling factors of shale gas under the control of different shale gas-rich structures.
Geological Setting
In terms of the regional structure, southern Sichuan crosses the low and steep structural belt in southern Sichuan and the low and steep structural belt in southwestern Sichuan (Figure 1). As a whole, it is sandwiched by the Huayingshan fault, Gulin fault, Qijiang fault, and Qiyueshan fault (Cheng et al., 2021; Liu et al., 2021). It is adjacent to the Loushan fault fold belt in the south and presents a “triangular structural belt” in the basin. The Yongchuan brush structural belt, Chishui–Luzhou superimposed structural belt, and Changning fault fold belt in the basin are developed from north to south in the study area, which is characterized by large north–south structural strength and weak deformation in the middle. The study area mainly develops northeast and northeast structures and develops east–west and northwest structures to the south, showing the characteristics of deformation differences (Liu et al., 2021).
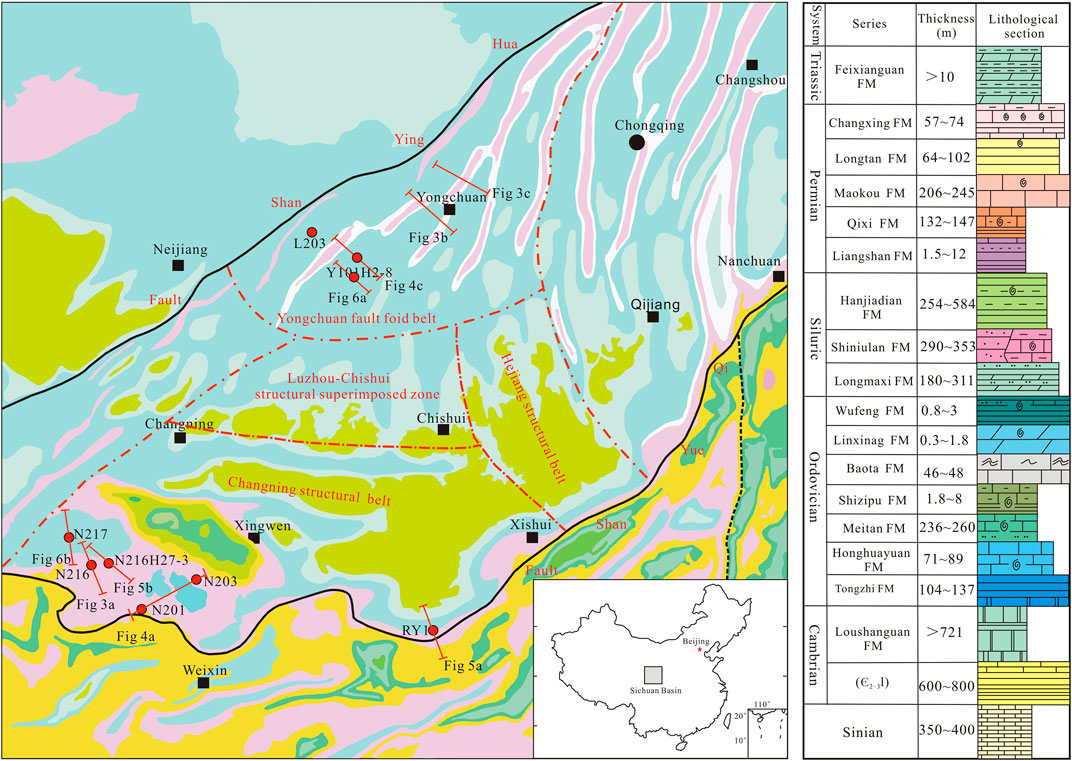
FIGURE 1. Regional structural location, stratum, and well location distribution in the Sichuan Basin.
The buried depth of the Wufeng–Longmaxi Formation shale in southern Sichuan is obviously different. The buried depth of the Wufeng–Longmaxi Formation shale in the Changning area is no more than 3,500 m, which is exposed on the surface in the Changning anticline area (Cheng et al., 2021; Wang Y. et al., 2021; Zhu et al., 2018; Zhu et al., 2022). The buried depth of shale in the Luzhou area is generally more than 3,500 m, the thickness of high-quality shale is between 20 and 80 m, the sedimentary environment is the deep-water shelf, and the lithology of high-quality shale is mainly organic siliceous shale and graptolite shale. The reservoir space includes primary matrix pores, hydrocarbon generation pores, and microfractures (Casini et al., 2016; Feng et al., 2020; Wang et al., 2020). The porosity of the high-quality shale section is between 2.35% and 7.84%, with developed foliation and good transverse permeability. Faults are developed in the study area, and there are obvious differences in fault longitudinal permeability and combination style. There are great differences in the productivity of single well shale gas wells in different structural parts (Wang, 2020). Among them, well N217 in the Changning area is located in the nose structure controlled by faults, with low shale gas content and single well test productivity of 11.12 × 104 m3; well N209 is located in a wide and gentle slope, shielded by faults, and high shale gas content. The test capacity of a single well is 62.02 × 104 m3.
Tectonic Zoning and Deformation Characteristics
There is a gradual contact relationship between the south of the Sichuan Basin and Dalou Mountains; there is no obvious basin mountain transition boundary; and the surface is characterized by vertical and horizontal geographical patterns of high mountains and deep valleys (Wang et al., 2017). From the basin edge to the basin, it can be divided into three structural areas: Changning strong reconstruction belt on the basin edge, Luzhou–Chishui structural superposition belt, and Yongchuan brush fault fold belt (He et al., 2016). There are obvious differences in stratigraphic deformation characteristics and structural styles in different structural areas.
Changningqiang Reconstruction Belt at the Basin Margin
Due to the northward wedging of the Dalou Mountains, the advancement of the Jiangnan–Xuefeng uplift, and the joint action of the Huaying Mountain strike-slip fault, the Changning area has strong structural deformation and is a strong transformation area. The main body includes the Changning anticline, Jianwu syncline, and Fujiang syncline. The main body of the Changning anticline is an asymmetric compound box anticline distributed in the NWW–SEE direction, which has the characteristics of a steep northeast wing and a gentle southwest wing. It dips in the Xuyong County in the southeast and ends in the Gaoxian County in the northwest. The structural trace deflection of the northwest wing of the anticline is distributed in the northeast direction, which is consistent with the structural axis of the Fujiang syncline, indicating that the current structural form of the Changning area is formed by the superposition and transformation of multistage tectonic movements, The oldest stratum exposed in the core of the anticline is the Cambrian Gaotai Formation, with secondary folds and reverse faults developed inside.
According to the surface tectonic trace and the relationship between fault cutting and restriction, the Changning tectonic deformation stage can be divided into three stages, and the stress action mechanism and influence range of different stages of tectonic movement are different. The main body of the Changning structural area was formed during the Yanshan movement and Himalayan movement. Before the Yanshan movement, the tectonic activity in the Changning area was weak, and the faults and folds were not developed. In the middle and late Yanshan movements, the central Guizhou block was strongly wedged in the NW direction of Daloushan along the Ziyun–Luodian fault and blocked by the rigid block in Central Sichuan, resulting in the nearly north–south compression of the Daloushan structural belt. It forms the prototype of the Changning anticline in the near east–west direction. From the end of the Yanshan period to the early Himalayan period, with the uplift of the Qinghai Tibet Plateau, the Sichuan Basin and its periphery were uplifted as a whole. Under the strong compression of NW–SE tectonic stress, the axial direction of the Changning anticline was twisted, and a large number of NE trending faults were formed. From the middle period of the Himalayan movement to now, under the combined action of the Jiangnan–Xuefeng uplift and central Sichuan uplift, the study area is affected by the dual stress from the southeast and north, respectively, develops NW trending faults, and cuts the early faults.
Luzhou–Chishui Structure Superposition Zone
The Luzhou–Chishui structural superposition zone includes the Xiangbichang syncline, Changyuanba anticline, Chishui nose anticline, and Shaxigou syncline. The structural form is characterized by narrow anticline and wide and gentle synclines. North–south and east–west structures are developed in the Chishui area. The north–south structure is located in the east of the superposition zone, and its formation is mainly affected by the Qiyueshan fault. The formation of the east–west structure is mainly affected by the northward wedge of the Dalou Mountains in the south. The oldest stratum exposed on the surface is Jurassic sandstone.
According to the surface structure trace and fault cutting characteristics, the east–west structure is formed earlier than the north–south structure, and the fault intersecting relationship is obvious. The north–south faults are mostly in the right-order echelon type, including the east and west directions, and the development degrees of faults with different tendencies are different. The fault layers inclined to the west are much more than those inclined to the east, and the fault distance is small and is limited by east–west faults. It shows that the formation time of the north–south structure is later than that of the east–west structure.
Combined with the analysis of structural evolution characteristics of the Sichuan Basin, the formation of the east–west structures in the Chishui area is superimposed and transformed by multistage tectonic movements. In the late Cretaceous, the uplift of the paleouplift in Central Guizhou led to the northward wedging of the Dalou Mountains, resulting in the north–south tectonic stress and forming the main body of the east–west structure. During the Himalayan movement, under the influence of the tectonic compression of the Jiangnan–Xufeng uplift and the regulation of the Sanjiang orogenic belt, the near east–west compressive stress was generated, and the north–south structure was formed.
Yongchuan Brush Fault Fold Belt
The Yongchuan brush fault fold belt is the product of the superposition of multiple structural systems. The fold structure converges to the north and diverges to the south, presenting a “brush shape” as a whole. The outer layer of the brush structure is a series of narrow anticlines with right oblique rows, and the inner anticline is arranged in an echelon, which is the product of a torsional structural stress field. In the north, the folds are strong, the stratum uplift is large, and the faults are relatively developed. The oldest stratum exposed in the core of the anticline is the Triassic Xujiahe Formation, which extends to the Luzhou area to the south. The fold strength is gradually weakened, there are a few faults, knee and hill structures are developed, and the Ziliujing Formation and Shaximiao Formation are exposed at the axis.
The main body of the Yongchuan brush fault fold belt includes the Xishan anticline, Gufushan anticline, Xindianzi anticline, Luoguanshan anticline, and other structures. The structural axis is NE, and the fault strike is mostly consistent with the anticline strike. Recoil faults occur along the steep wing of the anticline, controlling the anticline shape. Under the regulation of the Huayingshan strike-slip fault and the promotion of the Jiangnan–Xuefeng uplift and central Guizhou paleouplift, many rows of right-order en echelon folds are formed in the Yongchuan area, which are oblique to the Huayingshan fault. Due to the basin thrust of the Xuefeng orogenic belt, a southeast imbricate thrust fault is formed. At the same time, the thrust fault formed by the Middle Sichuan paleouplift converges downward in the lower Cambrian detachment layer, resulting in the difference between the upper and lower structural layers of the Longmaxi Formation. In the Cretaceous period, during the northward advancement of the paleouplift in Central Guizhou, the north–south tectonic stress was transmitted to the study area, causing the triangular area bounded by the Huayingshan fault zone and Qijiang fault zone to be affected by a certain north–south tectonic stress, resulting in the southward divergence of brush structure, and finally forming Yongchuan fault fold zone.
Deformation Strength and Typical Shale Gas-Rich Structural Types
Affected by the superposition and transformation of multistage tectonic movements, there are significant differences in deformation intensity in different structural areas, and there are great differences in shale burial depth, fault development, and gas-rich structural types, resulting in the discontinuous distribution of shale gas favorable areas.
Deformation Strength Difference
Early continuous burial controlled the pyrolysis and hydrocarbon generation of shale gas, and late structural uplift controlled the escape of shale gas. The preservation conditions are directly affected by the strength of the structure. The earlier the stratum uplift, the larger the uplift and the scale of the fault. The larger the value, the stronger the damage to the original state, and the longer the shale gas escape, which is not conducive to the preservation of shale gas. The large-scale uplift time and the uplift range of stratum uplift in different structural areas are quite different. According to the analysis of the burial history of typical wells, it can be seen that since the Cenozoic, the Changning area has started to uplift on a large scale at about 134 Ma, including the second moderate velocity and the first rapid uplift, and the stratum uplifted in the range of about 2,500–3,000 m (Figure 2A). The Yanshan structure formed the main body of the Changning structure with large-scale faults. The faults disappeared upward in the Permian detachment zone. During the Himalayan movement, strong tectonic compression caused the strata to occur. In a large-scale structural uplift, the Silurian strata are exposed on the surface of the Changning anticline, undergoing weathering and denudation, and forming a denudation zone. At the same time, strong tectonic movements formed large-scale faults that penetrated the Longmaxi Formation and the surface and destroyed the preservation conditions of shale.
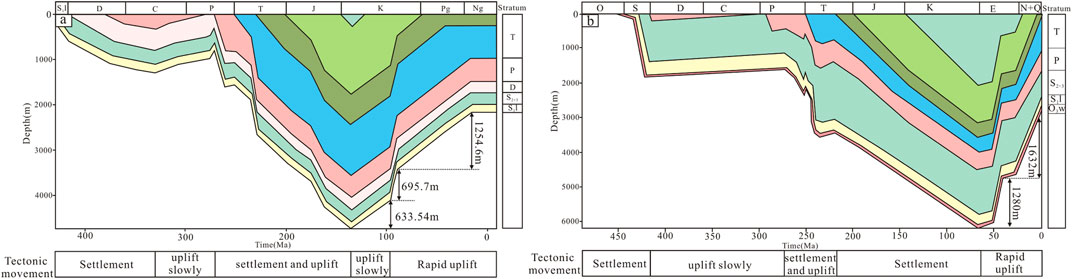
FIGURE 2. Burial history of Longmaxi Formation shale in southern Sichuan. (A) Burial history in Changning area and (B) burial history in Luzhou area.
The Wufeng–Longmaxi Formation in the Luzhou–Yongchuan area experienced two mid-range structural uplifts from the end of the Late Cretaceous to the early Cenozoic (74–40 Ma) and since the Late Cenozoic (20–0 Ma). The stratigraphic uplift was between 2,000 and 2,500 m (Figure 2B), and the stratigraphic uplift in the syncline area is below 2,000 m. During the Indosinian Movement, the Luzhou–Yongchuan area was formed, and the Yanshan movement and the Himalayas were intensified. Structural deformation was concentrated along the upper detachment zone. The Wufeng–Longmaxi Formation was always deeply buried, and large-scale faults developed in the anticline area. The fault distance is more than 40 m, and the fault scale in the syncline is small.
Typical Shale Gas-Rich Structure
There are obvious differences in fault combination characteristics, shale gas-rich structural types, and spatial distribution in different structural areas. As a whole, it can be divided into “anticline type” gas-rich structure, “syncline type” gas-rich structure, and “fault transformation slope type” gas-rich structure.
“Anticline Type” Shale Gas-Rich Structure
According to the curvature of fold turning end, the “anticline type” gas-rich structure in the study area can be divided into an arc-shaped anticline and inclined box anticlines (the two wings are relatively steep and asymmetrical, and the turn ends are flat and broad anticlines), and arc-shaped anticline can be divided into a concealed arc-shaped anticline and exposed arc-shaped anticline. The structural shape is affected by the reverse fault, forming fault spreading folds. The anticline shape is irregular, and the two wings are asymmetric. Generally, the dip angle of the front wing is greater than that of the rear wing, and the rear wing is usually controlled by the reverse fault with opposite inclination, forming a typical thrust structure.
The buried depth of the stratum is shallow in the Changning area, the fault development scale of the target layer is large, the anticline is wide and gentle, and an inclined box anticline is developed. The two wings and turning ends of the anticline are mostly transformed by the fault. Taking the section passing well N216 as an example, the seismic section shows that the anticline is asymmetric, the northwest wing of the anticline is steep, the core and turning ends are gentle, the fault transformation is strong, and the core and two wings are complicated by the fault transformation (Figure 3A).
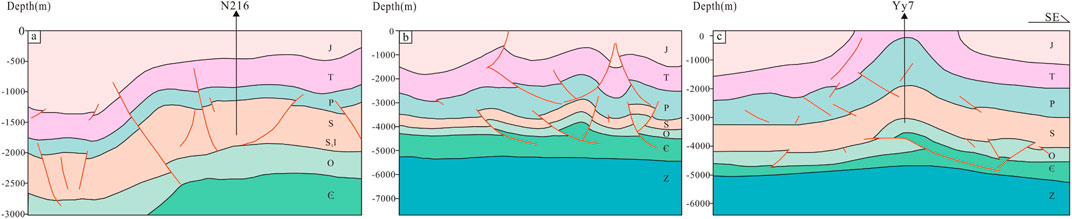
FIGURE 3. Typical “anticline type” shale gas-rich structure. (A) Cross N216 well profile in Changning area. (B) Rongchang structure profile in Luzhou area. (C) Cross Yy7 well profile in Luzhou area.
The shale of the Wufeng–Longmaxi Formation in the Luzhou–Yongchuan brush structural belt in southern Sichuan is deeply buried. Under the joint influence of the upper and lower sets of detachment layers of the Cambrian Gaotai Formation and Triassic Jialingjiang Formation, concealed arc anticlines are formed during the formation of reverse faults, which are mainly distributed in syncline structures. The stratum is deeply buried, and several parallel reverse faults in the syncline form fault spreading folds during the propagation process (Figure 3B). The main fault is a reverse fault arranged in parallel in the northeast direction, with the characteristics of steep up and slow down. The fault converges downward in the Cambrian gypsum salt layer and terminates upward in the Permian system. Secondary thrust faults are developed in the hanging wall of the thrust fault, which jointly control the shape and scale of the concealed arc anticline.
“Slope flat type” reverse fault is developed in the Yongchuan area. The fault converges downward, disappears in the Cambrian detachment layer, and disappears upward in the Silurian system. It has the characteristics of small upper and lower dip angles and large middle dip angles (Figure 3C). During the formation and propagation of the “slope flat type” reverse fault, the lower wall of the fault is stacked on the lower wall to form a typical fault bend fold. Under the condition of deep burial “slope flat type,” the reverse fault has strong sealing, and the fold core has become the high point of shale gas accumulation.
“Syncline Type” Shale Gas-Rich Structure
Affected by the Yanshan–Himalayan orogeny in different areas of southern Sichuan, the Wufeng–Longmaxi Formation in basin margin area is denuded to varying degrees, a residual syncline is developed, and the shape of the basin syncline is complete. According to the difference of axial plane shape, it can be divided into noninverted synclines and fault transformation synclines. Both Changning structures on the basin edge are developed, and the shape of the Luzhou syncline is complete. Affected by structural differences, the dip angles of the two wings of the Syncline in the Changning area are obviously different, mainly asymmetric synclines. Taking the sections passing through well N201 and well N203 in the Jianwu syncline as an example, the buried depth of shale in the core is about 2,200 m, of which the stratum in the northeast wing is steep with an inclination of about 45°, and the southwest wing is relatively flat with an inclination of about 30° (Figure 4A). The Changning area in the basin margin is superimposed and transformed by multistage tectonic movement, and multistage reverse faults are developed. Under the influence of upward and downward delamination, large faults passing through the target layer to the surface are concentrated in the core of the anticline, and there are few faults in the syncline. Combined with the seismic profile, small-scale faults are developed in the syncline, such as the section passing through well N216H11, the syncline is broad, the stratum dip angle is below 20°, and the shale layer of the Wufeng–Longmaxi Formation along the core of the syncline is reverse fault dislocation. A fault transformation syncline with asymmetric two wings is formed, and the two wings of the syncline are medium high-angle reverse fault transformations (Figure 4B). The syncline in the Luzhou area is wide and gentle, the stratum is deeply buried, the dip angle of the core stratum is below 10°, and the dip angle of the two wings is gradually increasing. The two wings of the syncline are limited by high-angle thrust faults, forming an opposite thrust (Figure 4C).

FIGURE 4. Typical “syncline type” shale gas-rich structure. (A) Cross N201–N203 well profile in Changning area. (B) Cross N216H11 well profile in Changning area. (C) Cross Y101H2-8 well profile in Luzhou area.
“Fault Shielding Slope Type” Shale Gas-Rich Structure
The south edge of the Sichuan Basin is jointly transformed by multistage tectonic movement, and the Wufeng–Longmaxi Formation shale is exposed on the surface. Faults are developed. In the southeast edge of the basin, under the action of the Qiyueshan main fault nappe and secondary fault regulation, the buried depth of the Wufeng–Longmaxi Formation gradually increases from the basin edge to the basin, and low-angle faults are developed to block the slope, such as the profile of well RY1 (Figure 5A). In the eastern part of the southern margin, due to the action of the Daloushan wedge and Huayingshan fault, as well as the influence of the Gulin fault and secondary reverse fault, along the Changning anticlinal basin, the burial depth of the Longmaxi Formation increases rapidly, and the dip angle of the footwall of the fault is large, which is shown as a high-angle slope. At the same time, due to the action of multiple secondary faults, the target layer of the slope is divided into high dip fault blocks. For example, the Changning structure passes through well N216H27-3 profile (Figures 5B,C).
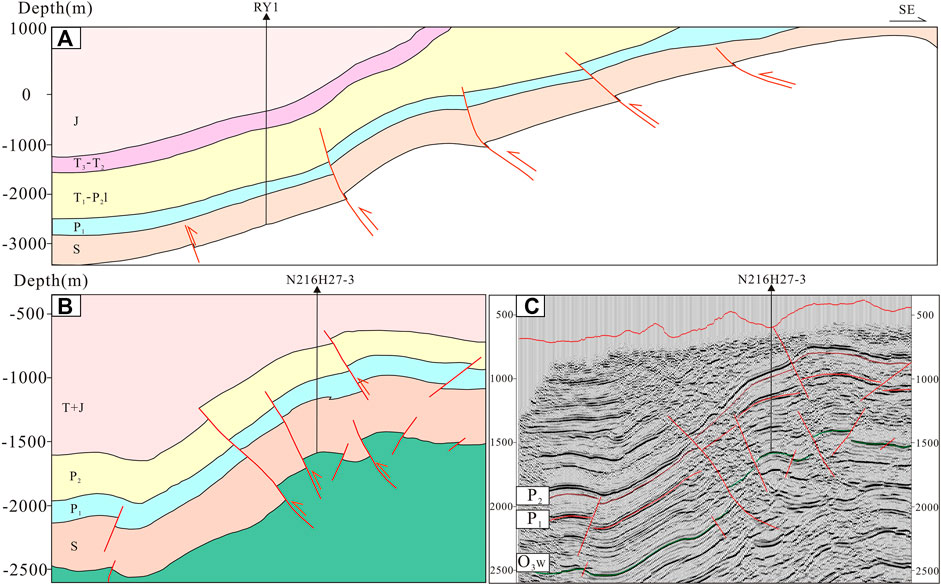
FIGURE 5. Basin margin fault blocks slope gas-rich structure. (A) Cross RY1 well profile in Renhuai area. (B) Cross N216H27-3 well profile in Changning area. (C) Cross N216H27-3 well seismic profile in Changning area.
Influence of Differential Structure on Preservation
Main Factors Controlling the Preservation of Different Shale Gas Structures
“Three dimensional effective sealing” is the key to the formation of shale gas reservoirs. In the process of shale gas formation, affected by hydrocarbon generation and pressurization, horizontal and vertical migration and diffusion will occur along the “depressurization channel.” Foliation, faults, and high-permeability strata may become the migration channel of shale gas (Wang et al., 2016; Chen et al., 2020; Cheng et al., 2020; Nie et al., 2020). The distribution of shale roofs and floors in southern Sichuan is stable. The failure of roof and floor and the shielding effect of lateral edge caused by faults formed in later structures determine the vertical capping and lateral migration. There are also some differences in fault combination styles, burial conditions, and main enrichment controlling factors of different gas-rich structures.
Main Controlling Factors for Preservation of Positive Gas-Rich Structures
In the forward structure, when the lamellation is not closed, shale gas migrates laterally to the structural high point to accumulate. The migration channels are mainly lamellation and bedding detachment fractures, and the shale gas mainly escapes through the vertical direction. The development of faults and fractures is the main controlling factor for the preservation conditions of shale gas. However, during the formation of the positive structure, the development of different types of forward structure faults and derived fractures is different. Therefore, the main control of preservation conditions is also different (Li et al., 2012; Wang et al., 2014; Wang et al., 2018; Li et al., 2020).
The turning end of the box-shaped anticline is wide and gentle, and local stress concentration occurs at the transition between the wing and the turning end. The formation is twisted at a large angle under the action of longitudinal bending and folds, secondary faults and derivative fractures are concentratedly developed, and the core of the anticline is gentle. The burial is shallow, and the lamellation is mostly in an open state. The shale gas migrates laterally along the lamellation surface to the core and accumulates. During the migration to the turning end, it escapes vertically through fractures and open fractures. Therefore, the main constraints on the storage conditions of the box-shaped anticline are the fracture, the development of cracks, and the sealing of the junction between the turning end and the wing.
In the process of squeezing formation to form folds, derived tensile stress occurs in the high part of the anticline, and tension fractures develop. When there are no faults in the anticline, these derived tension fractures become the main channels for the longitudinal migration of shale gas. The degree of development of derived extensional fractures is directly related to the curvature of the anticline formation. Generally speaking, the larger the scale of the fault, the greater the dip angle; and the closer the distance, the greater the curvature of the arc-shaped anticline, and the more developed the extensional fractures. Therefore, the main controlling factors for the preservation of the arc-shaped anticline are the scale, distance, and inclination of the fracture.
Main Controlling Factors of Negative Gas-Rich Structure Preservation
There are some differences in the negative structures developed in the Changning and Yongchuan areas in southern Sichuan. One side of the Syncline in the Changning area is exposed on the surface, and shale gas usually migrates along the syngenetic strata. When small reverse faults and microfractures are developed on both wings of the syncline, shale gas escapes vertically along the faults and fractures, and the opening of foliation directly affects the escape intensity of shale gas; it is the main factor controlling the preservation conditions of negative structures. When the syncline is transformed by faults, the sealing of faults determines the longitudinal escape strength.
According to the difference of buried depth, a negative shale gas system can be divided into foliation closed area, transitional foliation closed area, and foliation open area. The shale gas in the foliation closed area and transitional closed area is mainly ordinary diffusion, the shale gas in the foliation open area mostly escapes along with the stratum, and the migration intensity increases gradually (Gudmundsson et al., 2010; Ameen, 2016; Ashraf et al., 2020; Huang et al., 2021; Yu et al., 2021). The sealing of foliation depends on the positive pressure on the foliation surface. During the later tectonic movement, the strata on both wings develop different degrees of bending, the buried depth and dip angle of the strata change obviously, and the overlying strata pressure on different structural parts is different. When the positive stress of the overlying formation pressure acting on the foliation surface is greater than the foliation sealing pressure, the foliation is closed, which inhibits the escape of shale gas and is conducive to the preservation of shale gas. Therefore, the formation dip angle, buried depth, and fault sealing are the main control factors determining the enrichment of shale gas in negative structures.
Asymmetric synclines are developed in the Changning area, with different dip angles of the two wings. Most internal faults of the syncline are developed in the layer, and shale gas mainly escapes laterally through foliation. Taking the N201–N203 section as an example, well N201 and well N203 are located in the southwest wing and northeast wing of the Jianwu syncline, respectively. Well N201 in the southwest wing has a small dip angle, the buried depth of shale is about 2,100 m, and the gas production of a single well is 15 × 104 m3/day. Well N203 located in the northeast wing has a large formation dip angle, the buried depth of shale is about 1,800 m, and the gas production of a single well is 1.29 × 104 m3/day, showing the obvious difference between the two preservation conditions. For well Y101H2-8 in the deeply buried syncline area of the Yanggaosi area, Luzhou, the buried depth of the stratum is more than 3,000 m, the fault is less developed, the foliation is closed under the pressure of the overlying rock, the shale has high gas content, and the test differential energy of a single well is 50.7 × 104 m3/day.
Main Controlling Factors of Gas-Rich Structure Preservation in Sheltered Slope
One end of the slope structure is often exposed on the surface, with great differences in stratum buried depth. Most slopes are divided by faults. Shale foliation is relatively developed in the Changning and Luzhou–Yongchuan areas in southern Sichuan. Shale gas migrates along reverse faults and foliations. Foliation and faults control the horizontal and vertical migration of shale gas, respectively (Tuo et al., 2016; Liu Y. et al., 2018; Li et al., 2019; Ma et al., 2021; Yu et al., 2022). They jointly construct a “three-dimensional stepped migration system” and jointly control the escape of shale gas. Therefore, the sealing of faults and foliation is the main factor controlling the preservation conditions of slope gas-rich structures.
The migration intensity of shale gas is affected by the development degree of foliation, formation dip angle, burial depth, and other factors. Generally speaking, the greater the burial depth, the smaller the dip angle of the stratum, the greater the normal stress of the overlying stratum acting on the foliation surface, and the stronger the sealing of the foliation. The greater the dip angle and the more developed the foliation, the greater the escape intensity of shale gas, which is not conducive to the preservation of shale gas. Generally speaking, the preservation condition of a low-angle slope is better than that of a high-angle slope. When the slope is transformed by a reverse fault to form a certain curve, a local shale gas enrichment area will be formed, which is conducive to the preservation of shale gas.
Effect of Fracture Combination Style on Preservation
The roof and floor of the Wufeng–Longmaxi Formation in southern Sichuan are characterized by large continuous thickness and low porosity, the strata are under overpressure, and the integrity of the caprock is affected by fault development. There are obvious differences in fault development characteristics and combination styles in different structural areas. According to the difference of fault combination, it can be divided into three types: hedge thrust fault combination, back thrust fault combination, and high-angle compression fault block, which control the development of different shale gas-rich structures.
Back Thrust Reverse Fault Combination
The structure in southern Sichuan is strong, dominated by the development of reverse faults, which controls the development of different forward structures, among which the thrust structure is the most common. According to the occurrence and vertical combination characteristics of reverse faults, it can be divided into high-angle reverse fault combination and “detachment reverse fault combination,” which respectively control the development of exposed arc anticlines and concealed anticlines. Anticline curvature and fault sealing are the main factors restricting the preservation conditions of thrust structures. The distance and dip angle between thrust faults jointly determine the scale of gas-rich structures and anticline curvature and affect the preservation conditions of shale gas.
Taking Yanggaosi structure in the Yongchuan area as an example, the structure of well Y101 is a low scope concealed anticline with wide and gentle anticline (Figure 6A). The main control fault is buried in the shale of the Niutitang Formation downward, with the characteristics of steep up and slow down. The dip angle of the secondary fault is about 45° and terminates at the main control fault downward, in a “Y-shape” longitudinally. The anticline formed under the control of the two is large in scope. The top surface curvature of the Wufeng–Longmaxi Formation is small. Shale gas mainly escapes through reverse faults on both sides and derived tensile fractures on the top surface. The top surface curvature of the anticline is small, the development degree of derived tensile fractures is low, deep burial is strong, and the sealing is strong under the compression environment, which is conducive to the enrichment of shale gas. Combined with the test productivity of shale gas in a single well, well Y101H1-2 is 46.89 × 104 m3/day.
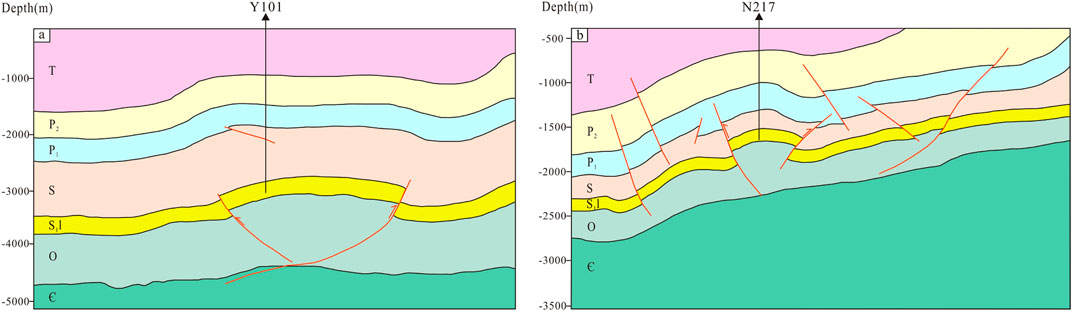
FIGURE 6. Back thrust reverse fault assembly. (A) Cross Y101 well profile in Luzhou area. (B) Cross N217 well profile in Changning area.
Ramp Reverse Fault Combination
The ramp reverse fault controls the development of negative structures. There are certain differences in the development of hedge structures in the Changning and Yongchuan areas in southern Sichuan. The shale in the Changning area is buried shallow; the hedge fault is close, is large in scale, and has many cutting horizons; and the controlled syncline is small in scale. The section passing through well YS107 in the Changning area is controlled by two large reverse faults in the Longmaxi Formation (Figure 7A), and the formed syncline is small in scale. Both sides of the fault are strongly squeezed, resulting in many derived fractures. Although well YS107 is located in the core of the syncline, due to the close distance between faults, the escape intensity of shale gas along the fault is large, resulting in low shale gas content and single well productivity.
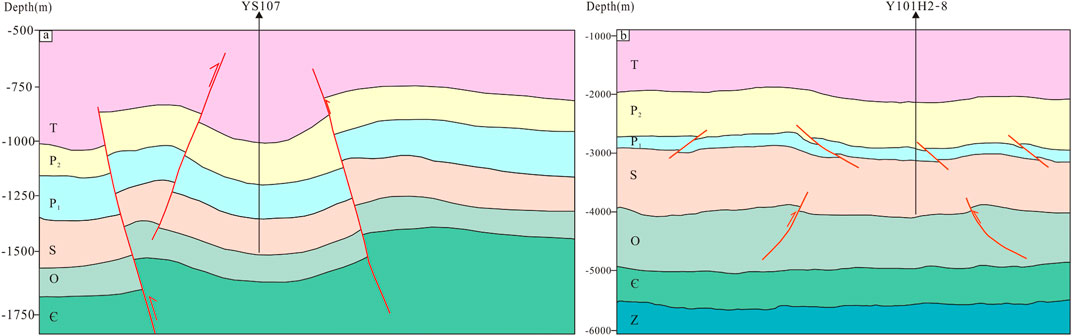
FIGURE 7. Hedging reverse fault combination. (A) Cross YS107 well profile in Changning area. (B) Cross Y101H2-8 well profile in Luzhou area.
The syncline of the Yongchuan brush fault fold belt is wide and gentle, the stratum is deeply buried, the development scale of a thrust syncline is large, and the fault space combination is in a large inverted “V” shape. According to the migration mechanism of shale gas and the difference of fracture development degree in different structural parts, the core of the syncline of the reverse fault on both sides of the opposite fault is the concentrated development area of fractures, and derived shear fractures are developed near the faults on both sides. The fault is in a compressed state and has strong tightness; the deformation process of the syncline core is low, mainly interlayer fractures and small microfractures, and has good preservation conditions. Well Y101H2-8 in the Yanggaosi area of the Luzhou block is located in the core of the Desheng syncline (Figure 7B). The formation pressure coefficient is 2.0, and both sides are limited by the thrust structure. A large number of small structural fractures and high-pressure fractures are developed in the core formation, which is conducive to the enrichment of shale gas. The shale gas content is 5.8 m3/t, and the single well productivity is 50.83 × 104 m3/day, showing good storage conditions.
High-Angle Compressional Fault Combination
High-angle compressional fault blocks are developed in the wide and gentle syncline in the Yongchuan Luzhou area. On the plane, they are shown as a series of parallel reverse faults with the same fault tendency. Most of the faults are grade III and grade IV faults, which disappear longitudinally inside the Longmaxi Formation without damaging the integrity of the roof and floor. Under the influence of multistage tectonic compression, propagation folds are mostly developed in the hanging wall of the reverse fault in the process of nappe. Under the environment of deep burial and strong compression, the reverse fault has strong tightness. In the process of reverse fault propagation, the high part of the propagation fold is affected by the derived tensile stress. A large number of tensile fractures and interlayer fractures are formed in the layer, which becomes the migration channel of shale gas (Zeng et al., 2016; Guo et al., 2017; Hooker et al., 2018; Nie et al., 2020; Song et al., 2021). Shale gas migrates along with the foliation and interlayer fractures to the high point of the propagation fold, forming a local shale gas enrichment area. Well L208 and well L209 in the Fuji Syncline in the Luzhou area are located at the high point of propagation fold (Figure 8), with a formation stress coefficient of about 2.2 and single well production of more than 20.00 × 104 m3, indicating good preservation conditions.
Conclusion
1) The gradient structural system in southern Sichuan develops different shale gas-rich structures from the basin edge to the basin, including “anticline type” gas-rich structure, “syncline type” gas-rich structure, and “fault transformation slope type” gas-rich structure. The anticline type gas-rich structure includes inclined box anticlines, arc-shaped anticlines, and low-scope concealed anticlines. The syncline type gas-rich structure includes a noninverted steering slope and fault transformation syncline. At the same time, a high-angle fault reconstruction slope is developed.
2) The preservation conditions of the complex structural area in southern Sichuan are mainly affected by foliation and fault sealing. The shale gas of wide and gentle anticline and circular arc anticline mainly escapes longitudinally along faults and secondary fractures. The preservation is mainly controlled by anticline curvature and fault sealing, and the negative structure mainly escapes along foliation. The main preservation factors are stratum buried depth and dip angle. Foliation and faults together form a stepped “three-dimensional escape system” of the shielding slope. The main preservation controlling factors include fault sealing, stratum buried depth, and stratum dip angle.
3) The back thrust fault combination controls the development of arc anticline and low scope concealed anticline. The high point of the anticline becomes the directional migration and accumulation area of shale gas. The core stratum of the hedge syncline formed under the control of the hedge fault is flat, and the foliation is closed, which is conducive to the migration and accumulation of shale gas. The high-angle reverse fault combination controls the distribution of fault extension folds, and the propagation folds in the hanging wall of the fault become the effective enrichment area of shale gas.
Data Availability Statement
The original contributions presented in the study are included in the article/supplementary material. Further inquiries can be directed to the corresponding author.
Author Contributions
SH contributed to writing, reviewing, and editing; data curation; and writing-original draft preparation. QQ and HL contributed to the formal analysis, validation, methodology, supervision, project administration, and funding acquisition. SW contributed to the visualization and investigation.
Funding
This research was supported by Open Fund (G5800-20-ZSKFZY002) of State Key Laboratory of Oil and Gas Enrichment Mechanisms and Effective Development and the National Science and Technology Major Project (2017ZX05036003-003) of the 13th Five-Year Plan Period.
Conflict of Interest
The authors declare that the research was conducted in the absence of any commercial or financial relationships that could be construed as a potential conflict of interest.
Publisher’s Note
All claims expressed in this article are solely those of the authors and do not necessarily represent those of their affiliated organizations, or those of the publisher, the editors, and the reviewers. Any product that may be evaluated in this article, or claim that may be made by its manufacturer, is not guaranteed or endorsed by the publisher.
References
Ambrose, R. J., Hartman, R. C., Diaz-Campos, M., Akkutlu, I. Y., and Sondergeld, C. H. (2012). Shale Gas-In-Place Calculations Part I: New Pore-Scale Considerations. Soc. Petrol. Eng. 17 (1), 219–229. doi:10.2118/131772-PA
Ameen, M. S. (2016). Fracture Modes in the Silurian Qusaiba Shale Play, Northern Saudi arabia and Their Geomechanical Implications. Mar. Pet. Geology. 78, 312–355. doi:10.1016/j.marpetgeo.2016.07.013
Ashraf, U., Zhang, H. C., Anees, A., Mangi, H. N., Ali, M., Ullah, H. Z., et al. (2020). Application of Unconventional Seismic Attributes and Unsupervised Machine Learning for the Identification of Fault and Fracture Network. Appl. Sci. 10, 2–25. doi:10.3390/app10113864
Awdal, A. H., Braathen, A., Wennberg, O. P., and Sherwani, G. H. (2013). The Characteristics of Fracture Networks in the Shiranish Formation of the Bina Bawi Anticline; Comparison with the Taq Taq Field, Zagros, Kurdistan, NE Iraq. Pet. Geosci. 19 (2), 139–155. doi:10.1144/petgeo2012-036
Borjigin, T., Shen, B., Yu, L., Yang, Y., Zhang, W., Tao, C., et al. (2017). Mechanisms of Shale Gas Generation and Accumulation in the Ordovician Wufeng-Longmaxi Formation, Sichuan Basin, SW China. Pet. Exploration Develop. 44 (1), 69–78. doi:10.1016/S1876-3804(17)30009-5
Casini, U., Hunt, D. W., Monsen, E., and Bounaim, A. (2016). Fracture Characterization and Modeling from Virtual Outcrops. AAPG Bull. 100 (1), 41–61. doi:10.1306/09141514228
Chen, J., Gao, J. K., Pu, Y. Y., Jiang, D. Y., Qi, Q. X., Wen, Z. J., et al. (2021). Machine Learning Method for Predicting and Warning of Rock Burst. J. Min. Strat. Control Eng. 3 (1), 013026. doi:10.13532/j.jmsce.cn10-1638/td.20200922.001
Chen, S. L., Huang, B. X., Li, D., Zhao, X. L., Xu, J., and Wang, C. W. (2020). Experiment Study on the Basic Law of High Pressure Abrasive Hydraulic Cutting for Coal-Rock Mass. J. Min. Strata Control. Eng. 2 (4), 047521. doi:10.13532/j.jmsce.cn10-1638/td.20200506.002
Chen, S., Zhao, W., Zeng, Q., Yang, Q., He, P., Gai, S., et al. (2018). Quantitative Prediction of Total Organic Carbon Content in Shale-Gas Reservoirs Using Seismic Data: A Case Study from the Lower Silurian Longmaxi Formation in the Chang Ning Gas Field of the Sichuan Basin, China. Interpretation 6 (4), SN153–SN168. doi:10.1190/INT-2018-0038.1
Cheng, G., Jiang, B., Li, M., Li, F., and Zhu, M. (2021). Structural Evolution of Southern Sichuan Basin (South China) and its Control Effects on Tectonic Fracture Distribution in Longmaxi Shale. J. Struct. Geology. 153, 104465. doi:10.1016/j.jsg.2021.104465
Cheng, J. W., Zhao, G., Sa, Z. Y., Zheng, W. C., Wang, Y. G., and Liu, J. (2020). Overlying Strata Movement and Deformation Calculation Prediction Models for Underground Coal Mines. J. Min. Strata Control. Eng. 2 (4), 043523. doi:10.13532/j.jmsce.cn10-1638/td.20200716.001
Curtis, M. E., Cardott, B. J., Sondergeld, C. H., and Rai, C. S. (2012). Development of Organic Porosity in the Woodford Shale with Increasing thermal Maturity. Int. J. Coal Geology. 103, 26–31. doi:10.1016/j.coal.2012.08.004
Fan, C. H., He, S., Zhang, Y., Qin, Q. R., and Zhong, C. (2018). Development Phases and Mechanisms of Tectonic Fractures in the Longmaxi Formation Shale of the Dingshan Area in Southeast Sichuan Basin, China. Acta Geol. Sin. Engl. 92 (6), 2351–2366. doi:10.1111/1755-6724.13732
Fan, C., Li, H., Qin, Q., He, S., and Zhong, C. (2020a). Geological Conditions and Exploration Potential of Shale Gas Reservoir in Wufeng and Longmaxi Formation of southeastern Sichuan Basin, China. J. Pet. Sci. Eng. 191, 107138. doi:10.1016/j.petrol.2020.107138
Fan, C., Li, H., Zhao, S., Qin, Q., Fan, Y., Wu, J., et al. (2020b). Formation Stages and Evolution Patterns of Structural Fractures in Marine Shale: Case Study of the Lower Silurian Longmaxi Formation in the Changning Area of the Southern Sichuan Basin, China. Energy Fuels 34 (8), 9524–9539. doi:10.1021/acs.energyfuels.0c01748
Feng, Z., HaoZhou, F. S. W., Zhou, S., Wu, W., Tian, J., Xie, C., et al. (2020). Pore Characteristics and Methane Adsorption Capacity of Different Lithofacies of the Wufeng Formation-Longmaxi Formation Shales, Southern Sichuan Basin. Energy Fuels 34 (7), 8046–8062. doi:10.1021/acs.energyfuels.0c00782
Gale, J. F. W., Laubach, S. E., Olson, J. E., Eichhuble, P., and Fall, A. (2014). Natural Fractures in Shale: a Review and New Observations. Bulletin 98 (11), 2165–2216. doi:10.1306/08121413151
Ge, X., Hu, W., Ma, Y., Li, M., Tang, J., and Zhao, P. (2021). Quantitative Evaluation of Geological Conditions for Shale Gas Preservation Based on Vertical and Lateral Constraints in the Songkan Area, Northern Guizhou, Southern China. Mar. Pet. Geology. 124, 104787. doi:10.1016/j.marpetgeo.2020.104787
Gudmundsson, A., Simmenes, T. H., Larsen, B., and Philipp, S. L. (2010). Effects of Internal Structure and Local Stresses on Fracture Propagation, Deflection, and Arrest in Fault Zonesflection, and Arrest in Fault Zones. J. Struct. Geology. 32, 1643–1655. doi:10.1016/j.jsg.2009.08.013
Guo, H. J., Ji, M., Sun, Z. G., and Zhou, Z. (2021). Energy Evolution Characteristics of Red sandstone under Cyclic Load. J. Min. Strata Control. Eng. 3 (4), 043019. doi:10.13532/j.jmsce.cn10-1638/td.20211008.001
Guo, T., and Zhang, H. (2014). Formation and Enrichment Mode of Jiaoshiba Shale Gas Field, Sichuan Basin. Pet. Exploration Develop. 41 (1), 31–40. doi:10.1016/S1876-3804(14)60003-3
Guo, X. S., Hu, D. F., Li, Y. P., Wei, Z. H., Wei, X., and Liu, Z. (2017). Geological Factors Controlling Shale Gas Enrichment and High Production in Fuling Shale Gas Field. Petrol. Explor. Dev. 44 (4), 516–526. doi:10.1016/S1876-3804(17)30060-5
Hadded, A., Layeb, M., Mannai-Tayech, B., Milad, B., Saïdi, M., and Soussi, M. (2021). Subsurface Geochemical and Mineralogical Evaluation for Unconventional "shale" Oil Play of the Bahloul Formation (Cenomanian-Turonian) in the Sahel Basin, Eastern Tunisia. Arab. J. Geosci. 14 (17), 1701. doi:10.1007/s12517-021-07977-5
He, S., Li, H., Qin, Q., and Long, S. (2021). Influence of Mineral Compositions on Shale Pore Development of Longmaxi Formation in the Dingshan Area, Southeastern Sichuan Basin, China. Energy Fuels 35 (13), 10551–10561. doi:10.1021/acs.energyfuels.1c01026
He, Z. L., Nie, H. K., and Zhang, Y. Y. (2016). The Main Factors of Shale Gas Enrichment of Ordovician Wufeng Foumation - Silurian Longmaxi Formation in the Sichuan Basin and its Adjacent Areas. Earth Sci. Front. 23, 8–17. doi:10.13745/j.esf.2016.02.002
Hooker, J. N., Abu-Mahfouz, I. S., Meng, Q., and Cartwright, J. (2018). Fractures in Mudrocks: Advances in Constraining Timing and Understanding Mechanisms. J. Struct. Geol. 125, 166–173. doi:10.1016/j.jsg.2018.04.020
Hou, E. K., Cong, T., Xie, X. S., and Wei, J. B. (2020). Ground Surface Fracture Development Characteristics of Shallow Double Coal Seam Staggered Mining Based on Particle Flow. J. Min. Strata Control. Eng. 2 (1), 013521. doi:10.13532/j.jmsce.cn10-1638/td.2020.01.002
Hou, Y., Gao, J., Ren, K., Liu, Y., He, S., Tang, D., et al. (2021). Variations of Lacustrine Shale Reservoirs in Different Deformation Zones of Mohe Basin, Northeastern China: Insights into the Impact of Thrust Nappe Structure on Shale Gas Preservation. Mar. Pet. Geology. 133, 105272. doi:10.1016/j.marpetgeo.2021.105272
Hu, Z., Du, W., Sun, C., Wu, J., Zhu, T., Zhao, J., et al. (2018). Evolution and Migration of Shale Facies and Their Control on Shale Gas: A Case Study from the Wufeng-Longmaxi Formations in the Sichuan Basin and its Surroundings. Interpretation 6 (4), SN57–SN70. doi:10.1190/INT-2018-0015.1
Huang, F. R., Yan, S. X., Wang, X. L., Jiang, P. C., and Zhan, S. B. (2021). Experimental Study on Infrared Radiation Characteristics of Gneiss under Uniaxial Compression. J. Min. Strata Control. Eng. 3 (1), 013011. doi:10.13532/j.jmsce.cn10-1638/td.20200730.001
Jarvie, D. M., Hill, R. J., Ruble, T. E., and Pollastro, R. M. (2007). Unconventional Shale-Gas Systems: The Mississippian Barnett Shale of north-central Texas as One Model for Thermogenic Shale-Gas Assessment. Bulletin 91 (4), 475–499. doi:10.1306/12190606068
Li, H. (2021). Quantitative Prediction of Complex Tectonic Fractures in the Tight sandstone Reservoirs: A Fractal Method. Arab. J. Geosci. 14, 1986. doi:10.1007/s12517-021-08344-0
Li, H., Tang, H., Qin, Q., Zhou, J., Qin, Z., Fan, C., et al. (2019). Characteristics, Formation Periods and Genetic Mechanisms of Tectonic Fractures in the Tight Gas Sandstones Reservoir: A Case Study of Xujiahe Formation in YB Area, Sichuan Basin, China. J. Pet. Sci. Eng. 178, 723–735. doi:10.1016/j.petrol.2019.04.007
Li, H. T., Peng, R., Du, W. S., Li, X. P., and Zhang, N. B. (2021b). Experimental Study on Structural Sensitivity and Intervention Mechanism of Mechanical Behavior of Coal Samples. J. Min. Strata Control. Eng. 3 (4), 043012. 13532/j.jmsce.cn10-1638/td.20210820.001.
Li, H., Wang, Q., Qin, Q., and Ge, X. (2021a). Characteristics of Natural Fractures in an Ultradeep Marine Carbonate Gas Reservoir and Their Impact on the Reservoir: A Case Study of the Maokou Formation of the JLS Structure in the Sichuan Basin, China. Energy Fuels 35, 13098–13108. doi:10.1021/acs.energyfuels.1c01581
Li, L., Tan, J., Wood, D. A., Zhao, Z., Becker, D., Lyu, Q., et al. (2019). A Review of the Current Status of Induced Seismicity Monitoring for Hydraulic Fracturing in Unconventional Tight Oil and Gas Reservoirs. Fuel 242, 195–210. doi:10.1016/j.fuel.2019.01.026
Li, S., Li, Y., He, Z., Chen, K., Zhou, Y., and Yan, D. (2020). Differential Deformation on Two Sides of Qiyueshan Fault along the Eastern Margin of Sichuan Basin, China, and its Influence on Shale Gas Preservationfluence on Shale Gas Preservation. Mar. Pet. Geology. 121, 104602. doi:10.1016/j.marpetgeo.2020.104602
Li, S., Santosh, M., Zhao, G., Zhang, G., and Jin, C. (2012). Intracontinental Deformation in a Frontier of Super-convergence: a Perspective on the Tectonic Milieu of the South China Block. J. Asian Earth Sci. 49, 313–329. doi:10.1016/j.jseaes.2011.07.026
Liu, P., Wang, X., Meng, Q., Wang, X., Zhang, L., Liu, C., et al. (2018a). Simulation of Shale Gas Generation by Using Different Experimental Systems: A Case Study from Chang7 Shale in the Ordos Basin. J. Nat. Gas Sci. Eng. 49, 169–178. doi:10.1016/j.jngse.2017.10.017
Liu, W., Wu, J., Jiang, H., Zhou, Z., Luo, C., Wu, W., et al. (2021). Cenozoic Exhumation and Shale-Gas Enrichment of the Wufeng-Longmaxi Formation in the Southern Sichuan basin, Western China. Mar. Pet. Geology. 125, 104865. doi:10.1016/j.marpetgeo.2020.104865
Liu, Y., Tang, X., Zhang, J., Mo, X., Huang, H., and Liu, Z. (2018b). Geochemical Characteristics of the Extremely High thermal Maturity Transitional Shale Gas in the Southern North China Basin (SNCB) and its Differences with marine Shale Gas. Int. J. Coal Geology. 194, 33–44. doi:10.1016/j.coal.2018.05.005
Ma, X. H., and Xie, J. (2018). The Progress and Prospects of Shale Gas Exploration and Exploitation in Southern Sichuan Basin, SW China. Petrol. Explor. Dev. 45 (1), 161–169. doi:10.1016/S1876-3804(18)30018-1
Ma, Z., Tan, J., Zheng, L., Shen, B., Wang, Z., Shahzad, A., et al. (2021). Evaluating Gas Generation and Preservation of the Wufeng-Longmaxi Formation Shale in southeastern Sichuan Basin, China: Implications from Semiclosed Hydrous Pyrolysis. Mar. Pet. Geology. 129, 105102. doi:10.1016/j.marpetgeo.2021.105102
Nie, H., He, Z., Wang, R., Zhang, G., Chen, Q., Li, D., et al. (2020). Temperature and Origin of Fluid Inclusions in Shale Veins of Wufeng-Longmaxi Formations, Sichuan Basin, south China: Implications for Shale Gas Preservation and Enrichment. J. Pet. Sci. Eng. 193, 107329. doi:10.1016/j.petrol.2020.107329
Pollastro, R. M., Jarvie, D. M., Hill, R. J., and Adams, C. W. (2007). Geologic Framework of the Mississippian Barnett Shale, Barnett-Paleozoic Total Petroleum System, Bend Arch-Fort Worth Basin, Texas. Bulletin 91 (4), 405–436. doi:10.1306/10300606008
Qie, L., Shi, Y. N., and Liu, J. G. (2021). Experimental Study on Grouting Diffusion of Gangue Solid Filling Bulk Materials. J. Min. Strata Control. Eng. 3 (2), 023011. doi:10.13532/j.jmsce.cn10-1638/td.20201111.001
Ran, B., Liu, S., Jansa, L., Sun, W., Yang, D., Wang, S., et al. (2016). Reservoir Characteristics and Preservation Conditions of Longmaxi Shale in the Upper Yangtze Block, South China. Acta Geologica Sinica - English Edition 90 (6), 2182–2205. doi:10.1111/1755-6724.13030
Shan, S. C., Wu, Y. Z., Fu, Y. K., and Zhou, P. H. (2021). Shear Mechanical Properties of Anchored Rock Mass under Impact Load. J. Min. Strata Control. Eng. 3 (4), 043034. doi:10.13532/j.jmsce.cn10-1638/td.20211014.001
Shi, X., Luo, C., Cao, G., He, Y., Li, Y., Zhong, K., et al. (2021). Differences of Main Enrichment Factors of S1l11-1 Sublayer Shale Gas in Southern Sichuan Basin. Energies 14 (17), 5472. doi:10.3390/en14175472
Song, J. F., Lu, C. P., Li, Z. W., Ou, Y. G. C., Cao, X. M., and Zhou, F. L. (2021). Characteristics of Stress Distribution and Microseismic Activity in Rock Parting Occurrence Area. J. Min. Strata Control. Eng. 3 (4), 043518. doi:10.13532/j.jmsce.cn10-1638/td.20210607.002
Tuo, J., Wu, C., and Zhang, M. (2016). Organic Matter Properties and Shale Gas Potential of Paleozoic Shales in Sichuan Basin, China. J. Nat. Gas Sci. Eng. 28, 434–446. doi:10.1016/j.jngse.2015.12.003
Wang, B., Lu, C. L., Huang, Z. K., and Hu, S. Y. (2021a). Experimental Study on Damage Evolution Characteristics of Rock under Triaxial Rheological Disturbance. J. Min. Strata Control. Eng. 3 (4), 043028. doi:10.13532/j.jmsce.cn10-1638/td.20210525.001
Wang, E., Meng, K., Su, Z., Meng, Q., Chu, J. J., Chen, Z., et al. (2014). Block Rotation: Tectonic Response of the Sichuan basin to the Southeastward Growth of the Tibetan Plateau along the Xianshuihe‐Xiaojiang Fault. Tectonics 33, 686–718. doi:10.1002/2013tc003337
Wang, G. (2020). Deformation of Organic Matter and its Effect on Pores in Mud Rocks. Bulletin 103, 21–36. doi:10.1306/04241918098
Wang, H., Shi, Z., and Sun, S. (2021b). Biostratigraphy and Reservoir Characteristics of the Ordovician Wufeng Formation-Silurian Longmaxi Formation Shale in the Sichuan Basin and its Surrounding Areas, China. Petrol. Explor. Dev. 43 (3), 1–7. doi:10.1016/S1876-3804(21)60088-5
Wang, J. Q., Gong, J. M., Zhang, L., Cheng, H.-Y., Liao, J., Chen Yang, J. -W. C. S., et al. (2018). Discussion on "sandwich" Structures and Preservation Conditions of Shale Gas in the South Yellow Sea Basin. China Geol. 1 (4), 485–492. doi:10.31035/cg2018064
Wang, J., and Wang, X. L. (2021). Seepage Characteristic and Fracture Development of Protected Seam Caused by Mining Protecting Strata. J. Min. Strata Control. Eng. 3 (3), 033511. doi:10.13532/j.jmsce.cn10-1638/td.20201215.001
Wang, X., Liu, L., Wang, Y., Sheng, Y., Zheng, S., Wu, W., et al. (2020). Comparison of the Pore Structures of Lower Silurian Longmaxi Formation Shales with Different Lithofacies in the Southern Sichuan Basin, China. J. Nat. Gas Sci. Eng. 81, 103419. doi:10.1016/j.jngse.2020.103419
Wang, Y., Cheng, H., Hu, Q., Liu, L., and Hao, L. (2021c). Diagenesis and Pore Evolution for Various Lithofacies of the Wufeng-Longmaxi Shale, Southern Sichuan Basin, China. Mar. Pet. Geology. 133, 105251. doi:10.1016/j.marpetgeo.2021.105251
Wang, Y., Wang, H., Zhang, C., Li, X., and Dong, D. (2017). Fracture Pore Evaluation of the Upper Ordovician Wufeng to Lower Silurian Longmaxi Formations in Southern Sichuan Basin, SW China. Pet. Exploration Develop. 44 (4), 563–572. doi:10.1016/S1876-3804(17)30065-4
Wang, Y., Zhang, J., Zhang, B., and Zhao, H. (2018). Cenozoic Exhumation History of South China: a Case Study from the Xuefeng Mt. Range. J. Asian Earth Sci. 151, 173–189. doi:10.1016/j.jseaes.2017.10.039
Xie, J., Qin, Q., Fan, C., Hu, D., and Li, H. (2019). The Quantitative Prediction of Tectonic Fractures of Longmaxi Formation in Dingshan Area, southeastern Sichuan Basin. Earth Sci. Res. J. 23 (2), 157–162. doi:10.15446/esrj.v23n2.79957
Xu, S., Gou, Q., Hao, F., Zhang, B., Shu, Z., Lu, Y., et al. (2020). Shale Pore Structure Characteristics of the High and Low Productivity wells, Jiaoshiba Shale Gas Field, Sichuan Basin, China: Dominated by Lithofacies or Preservation Condition? Mar. Pet. Geology. 114, 104211. doi:10.1016/j.marpetgeo.2019.104211
Yin, S., Lv, D., Jin, L., and Ding, W. (2018). Experimental Analysis and Application of the Effect of Stress on continental Shale Reservoir Brittleness. J. Geophys. Eng. 15 (2), 478–494. doi:10.1088/1742-2140/aaa5d2
Yu, X., Bian, J. Q., and Liu, C. Y. (2022). Determination of Energy Release Parameters of Hydraulic Fracturing Roof Near Goaf Based on Surrounding Rock Control of Dynamic Pressure Roadway. J. Min. Strata Control. Eng. 4 (1), 013016. doi:10.13532/j.jmsce.cn10-1638/td.20210908.001
Yu, X. Y., Wang, Z. S., Yang, Y., and Mao, X. W. (2021). Numerical Study on the Movement Rule of Overburden in Fully Mechanized Caving Mining with Thick Depth and High Mining Height. J. Min. Strata Control. Eng. 3 (1), 013533. doi:10.13532/j.jmsce.cn10-1638/td.20200715.001
Yuan, Y., Chen, J., Liang, J., Xu, M., Lei, B., Zhang, Y., et al. (2019). Hydrocarbon Geological Conditions and Exploration Potential of Mesozoic-Paleozoic Marine Strata in the South Yellow Sea Basin. J. Ocean Univ. China 18 (6), 1329–1343. doi:10.1007/s11802-019-3853-2
Zeng, L., Lyu, W., Li, J., Zhu, L., Weng, J., Yue, F., et al. (2016). Natural Fractures and Their Influence on Shale Gas Enrichment in Sichuan Basin, Chinafluence on Shale Gas Enrichment in Sichuan Basin, China. J. Nat. Gas Sci. Eng. 30, 1–9. doi:10.1016/j.jngse.2015.11.048
Zhang, K., Jia, C., Song, Y., Jiang, S., Jiang, Z., Wen, M., et al. (2020). Analysis of Lower Cambrian Shale Gas Composition, Source and Accumulation Pattern in Different Tectonic Backgrounds: A Case Study of Weiyuan Block in the Upper Yangtze Region and Xiuwu Basin in the Lower Yangtze Region. Fuel 263, 115978. doi:10.1016/j.fuel.2019.115978
Zhu, H., Ju, Y., Qi, Y., Huang, C., and Zhang, L. (2018). Impact of Tectonism on Pore Type and Pore Structure Evolution in Organic-Rich Shale: Implications for Gas Storage and Migration Pathways in Naturally Deformed Rocks. Fuel 228, 272–289. doi:10.1016/j.fuel.2018.04.137
Keywords: preservation conditions, construction style, differential deformation, complex structure area, Sichuan basin
Citation: He S, Qin Q, Li H and Wang S (2022) Deformation Differences in Complex Structural Areas in the Southern Sichuan Basin and Its Influence on Shale Gas Preservation: A Case Study of Changning and Luzhou Areas. Front. Earth Sci. 9:818534. doi: 10.3389/feart.2021.818534
Received: 19 November 2021; Accepted: 20 December 2021;
Published: 20 January 2022.
Edited by:
Jijun Li, China University of Petroleum, Huadong (UPC), ChinaReviewed by:
Zhu Baiyu, Yangtze University, ChinaShuai Yin, Xi’an Shiyou University, China
Meng Wang, Chongqing University of Science and Technology, China
Copyright © 2022 He, Qin, Li and Wang. This is an open-access article distributed under the terms of the Creative Commons Attribution License (CC BY). The use, distribution or reproduction in other forums is permitted, provided the original author(s) and the copyright owner(s) are credited and that the original publication in this journal is cited, in accordance with accepted academic practice. No use, distribution or reproduction is permitted which does not comply with these terms.
*Correspondence: Hu Li, bGlodTg2MDYyOEAxMjYuY29t