- 1College of Safety and Ocean Engineering, China University of Petroleum, Beijing, China
- 2General Institute of Science and Technology Company of PipeChina, LangFang, China
- 3School of Mechanical Engineering, Xian Jiaotong University, Xian, China
It is not uncommon to see pipelines damaged by landslides. If the maximum strain that landslides exert on pipelines can be calculated from available survey data, then pipeline damage can be more accurately predicted and the safety of the pipeline can be improved. Furthermore, this information can assist targeted prevention and control measures, which should greatly reduce the risk that landslides pose to pipelines. However, although researchers have conducted considerable research on landslide-induced pipeline deformation, there is no model that can calculate the change in pipeline strain under the displacement of landslide material. Based on geological data collected from a buried crude oil pipeline damaged by a landslide near a city in southeast China, this work uses numerical simulations to obtain the deformation parameters of pipelines affected by landslides at different orientations and for different landslide and pipeline parameters. This work also summarizes the deformation characteristics of pipelines affected by landslides under these different variables. A model is then constructed and verified that simulates the change in pipeline strain with soil displacement while considering the characteristics of the landslide mass and the pipeline itself. The results show that the model can sufficiently reflect the influence of various factors that act on pipeline deformation and can accurately calculate the maximum strain that landslides exert on pipelines.
1 Introduction
In recent years, oil and gas pipeline leaks caused by landslides have been frequent. These events are influenced by geological activity, extreme weather, and human engineering activities, and the resulting pipeline damage has led to environmental pollution, many casualties, and economic loss. For example, in July 2013, nearly 1 ton of crude oil leaked from a pipeline ruptured by landslides in Xi’an, Shaanxi, China, which polluted local waters (Wang et al., 2015). In December 2015, the branch line of the West-East gas transmission project was damaged by a landslide that caused 94 casualties and direct economic losses of 881 million yuan in Shenzhen, China (Sun and Song, 2018). In July 2016, a landslide caused 250,000 L of crude oil to spill into the North Saskatchewan River in Canada, also leading to water pollution (Holliday et al., et al.). In July 2016, a landslide led to the fracture of a Sichuan gas pipeline, causing an explosion that killed two people and injured three in Cuijiaba, Enshi, China (Li, 2016). In December 2020, an oil pipeline broke and caught fire due to landslides in Salhoun, Iran. In-depth research on the deformation characteristics of pipelines subjected to landslides and improved models of how pipelines are deformed by landslides can inform predictions of pipeline safety during landslide events and aid in the development of landslide prevention and control measures.
The academic community has made some progress in the study of pipeline deformation characteristics under the action of landslides. For example, Feng et al. (2015) found that stress on pipelines is significantly concentrated in the middle and boundaries of a landslide. Niu et al. (2015) found that the location of maximum stress on a pipeline is related to the landslide stage and the burial condition of the pipeline. In recent years, numerical simulation techniques have been widely used in the study of landslide-induced pipeline deformation. For example, Xu et al. (2022) established a coupled model to examine how pipelines are deformed by landslides by combining smooth particle fluid dynamics with finite elements. The simulation found that the oil content of pipelines has a large effect on their displacement and deformation. Han and Fu (2020) used numerical simulations to analyze the relationship between pipeline deformation and landslides by changing the area of the landslide and the diameter of the pipeline. Zhang et al. (2018) developed a new finite element model with equal boundary springs and a new stiffness calculation method to effectively simulate the pipe-soil interaction in slope bodies. Scholars have also tried to construct computational models to more accurately simulate the deformation and damage characteristics of pipelines impacted by landslides,. For example, Zahid et al. (2020) proposed a mathematical model for calculating the ultimate axial strain of landslides on longitudinal gas pipelines by considering the effects of pipe-soil interaction, pipe pressure, weight, and temperature. Vasseghi et al. (2021) used the Winkler elastic foundation beam model to analyze the deformation and damage of natural gas pipelines impacted by landslides. Ma et al. (2022) established a physical model that couples oil pressure in the pipeline and soil to study the influence of oil pressure on pipeline deformation characteristics during landslide events. Wang et al. (2021) established a simplified mechanical model of the force deformation of buried pipelines under the action of lateral landslides based on the Winkler elastic foundation beam model and the lateral distribution model of landslide thrust. Zhang et al. (2022) analyzed the mechanical response of gas transmission pipelines to landslide thrusts by combining the Green-Ampt model and the Pasternak dual-reference model; they verified the accuracy of the analytical scheme. Han et al. (2022) established a static pipeline model based on consideration of the geotechnical properties at both ends of a pipeline.
These proposed models make it possible to predict the deformation characteristics of pipelines under landslide action; however, the following deficiencies remain:
1) Existing pipeline/landslide models can only determine the unidirectional deformation of buried pipelines. Additionally, most of these models focus on transversely buried pipelines.
2) Pipeline/landslide models do not consider the dynamic displacement process of the pipeline within the landslide soil and thus cannot determine the strain value of a pipeline during the landslide process.
Therefore, based on field-based analysis and numerical simulation, this study will investigate the effects of different slope shapes, buried pipeline directions, and pipeline properties on the deformation and damage characteristics of pipelines under landslide actions. Furthermore, it will construct a buried pipeline strain calculation model that considers landslide displacements and pipeline properties. The model considers pipelines that cross landslides laterally and longitudinally and which can provide the dynamic relationship between the maximum strain of the pipeline and landslide displacement, thereby laying the foundation for future predictions of pipeline safety during landslide events.
2 Field investigation of the threat landslides pose to pipelines
A site survey was carried out to obtain detailed information on and about the impact of landslide disasters on pipelines. The site investigated in this study is a buried crude oil pipeline located outside a city in southeast China, with six landslide disaster points: Cases 1 to 6 (see Table 1 for landslide parameters and Table 2 for pipeline information). The section of pipeline in Case 4 is located in the middle of the landslide, the section in Case 6 is located at the leading edge of the landslide, and the other four sections are located at the landslide's trailing edge. The pipeline was exposed but not significantly deformed after the landslide in Cases 1 and 3. In Cases 2, 5, and 6, the pipeline was deformed (e.g., bent and stretched) but did not break. In Case 4, the broken pipeline led to the leakage of crude oil and land pollution (Figure 1). The survey results show that small scale landslides are unlikely to cause a threat to the pipeline, and that the width of a landslide surface that can lead to pipeline deformation must exceed 30 m.
3 Numerical simulation of how landslides threaten pipelines
3.1 Simulation conditions and parameters
According to the investigation’s results, the width, length, thickness, and gradient of a landslide are the main factors affecting pipeline deformation. To clarify the specific effects of these factors, this work calculates the impacts of landslides with different widths, lengths, thicknesses, and gradients on a pipeline. There are in fact three ways for a pipeline to cross a landslide. A pipeline can intersect with a landslide horizontally (i.e., longitudinally), vertically (i.e., latitudinally), or obliquely. The condition of pipelines that obliquely cross landslides can be revealed by analyzing those that intersect landslides vertically and horizontally. Therefore, these latter two orientations are considered in this study (Figure 2). Landslide mass is divided into three areas: the soil mass at the edge of a landslide consists of gravely soil, at its bottom is silty clay, and at the surface of a landslide is plain fill. The pipe is a hollow round pipe with an outer diameter of 600 mm and a wall thickness of 8 mm; it is made of L360 steel. A uniform pressure of 2.3 MPa was applied to the inner wall of the pipe. The friction coefficient between the pipeline and the soil was 0.7, and the buried depth of the pipeline was 1.5 m. The length of the landslide was set at 60 m, its thickness at 6.0 m, its slope was 30°, and its width was 30 m.
According to the survey results, there are three different rock and soil materials in the landslide body. The parameters of the rock and soil mass obtained by the experimental results are shown in Table 3. The parameters of the L360 steel pipeline used in the exploration area are in accordance with the technical requirements for oil transportation steel pipelines and the standard “Steel Pipelines for Oil and Gas Industry Pipeline Conveying System” (GB/T9711-2011). For further detail, see Table 3.
3.2 Soil displacement and pipeline deformation characteristics
3.2.1 Pipeline influence on soil displacement
On the whole, due to the large scale of landslides, pipelines have little impact on the volume and location of soil displaced. Furthermore, a pipeline has little impact on the initial location of the landslide, the soil displacement during the landslide, and the area of soil deformation; it does, however, have a certain impact on the landslide’s final accumulated form (Figure 3).
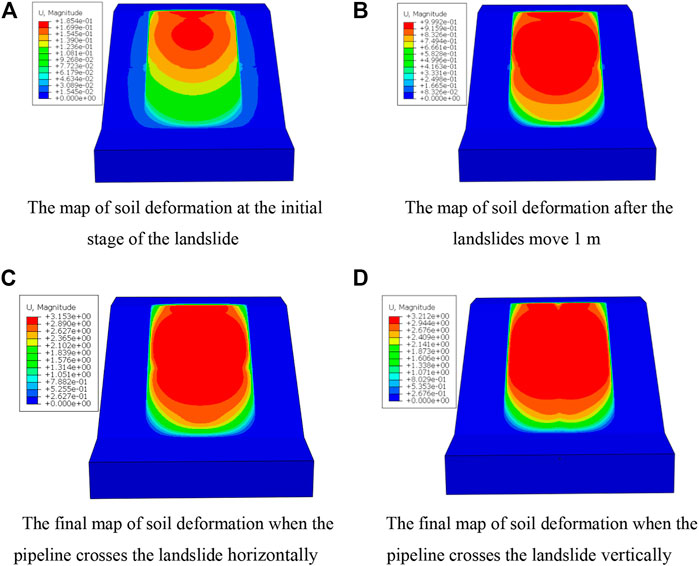
FIGURE 3. Landslide soil displacement and deformation map. (A) Map of soil deformation at the initial stage of the landslide. (B) Map of soil deformation after the landslides move 1 m. (C) Final map of soil deformation when the pipeline crosses the landslide horizontally. (D) Final map of soil deformation when the pipeline crosses the landslide vertically.
The stability of a soil mass is itself an important indicator of a slope’s stability. The reduction coefficients for pipelines oriented horizontally and vertically to the landslide are extracted, as shown in Figures 4, 5. The changing values of the reduction coefficient are 1.0154 and 1.0153 for the horizontally and vertically oriented pipelines, respectively, which are similar. It can be seen that the way in which the pipeline crosses the landslide has not affected the soil’s stability. It can be further inferred that, for large landslides, the presence of a pipeline has little impact on the slope itself.
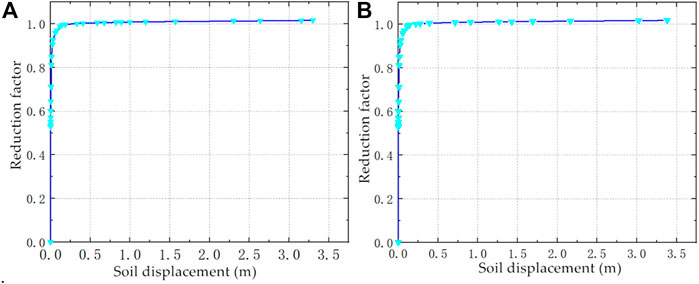
FIGURE 4. Variation diagram of the landslide reduction coefficient for pipelines passing through the landslide at different orientations: (A) horizontal, (B) vertical.
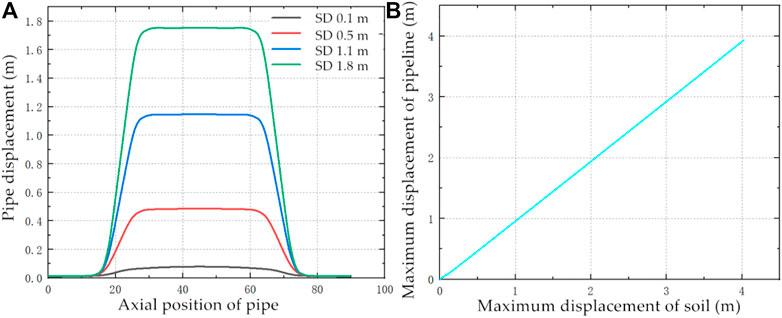
FIGURE 5. Relationship between pipeline displacement and landslide soil displacement (SD): (A) displacement at different positions along the pipeline, (B) maximum displacement of the pipeline.
3.2.2 Deformation characteristics of pipelines impacted by landslides
3.2.2.1 Pipeline crossing the landslide in a lateral orientation
3.2.2.1.1 Displacement of the pipeline
When a pipeline crosses a landslide with a lateral orientation, the displacement of the pipeline changes with the displacement of the landslide mass. In the initial stage of a landslide, the displacement of a pipeline is small, and the overall deformation is arc shaped. As the displacement of the soil increases, the pipeline’s displacement increases significantly. The geometry of the displacement gradually changes from an arc shape to a “symmetric three-segment” pattern with a stepped distribution (Figure 5A). The pipeline located in the middle section of the landslide has the largest displacement and exhibits a linear distribution. The maximum displacement of the pipeline and the landslide body during the entire landslide deformation process was extracted for analysis. As shown in Figure 5B, it was found that the maximum displacement of the pipeline was linearly correlated with the maximum displacement of the soil body.
3.2.2.1.2 Stress of the pipeline
The stress acting on the pipeline is symmetrical to the axis of the landslide. With the increase in the displacement of the landslide, the stress on the pipeline increases gradually. The stress of the pipeline section near the edges of both sides of the landslide is the largest and shows a “hump” shape. The peak point of stress along the pipeline is located at the junction of the landslide mass and the stable soil mass. The pipeline stress in the middle section of the landslide body is small and relatively fixed; it does not increase with increasing landslide soil displacement (Figure 6A). The maximum stress of the pipeline is extracted, and the maximum displacement of the landslide soil is analyzed. In the initial stage of the landslide, the maximum stress on the pipeline changes rapidly with the displacement of the landslide soil, which shows a sharp linear change. However, when the soil displacement reaches approximately 0.3 m, the stress of the pipeline increases slowly, and the pipeline enters the yield stage. When the soil displacement reaches 1.6 m, the stress of the pipeline does not change much and only gradually reaches the maximum stress on the pipeline. At this point, the pipeline can be considered damaged (Figure 6B).
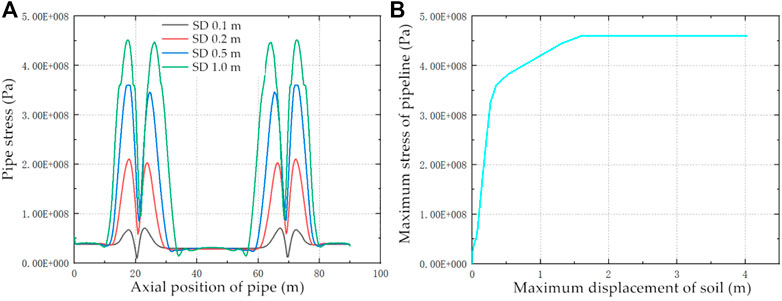
FIGURE 6. Relationship between pipeline stress and landslide soil displacement (SD): (A) stress at different positions of the pipeline, (B) maximum stress of the pipeline.
3.2.2.1.3 Strain of the pipeline
The strain on the pipeline is also distributed symmetrically on both sides of the landslide's central axis. The strain on the pipeline increases with the increase in the soil displacement of the landslide. The maximum strain point on the pipeline is located at the edge of the landslide body. The pipeline strain in the middle section of the landslide body is small and does not change with increasing landslide body displacement (Figure 7A). The maximum strain on the pipeline and the maximum displacement of the landslide soil are extracted, as shown in Figure 7B. The maximum strain on the pipeline increases with the displacement of the landslide soil. In the initial stage, the pipeline strain increases slowly with the displacement of the landslide soil and then increases rapidly. When the landslide soil displacement reaches 1.3 m, the pipeline strain further increases with the change rate of the landslide soil.
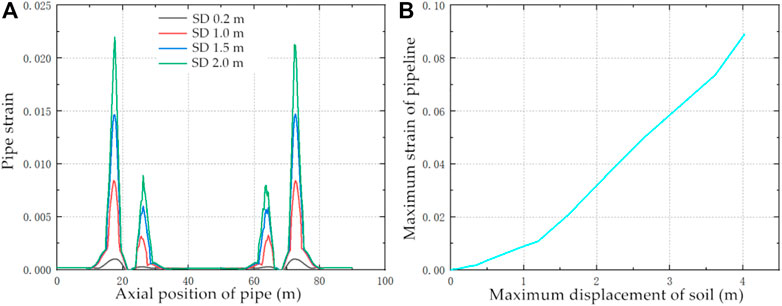
FIGURE 7. Relationship between pipeline strain and landslide soil displacement (SD): (A) strain at different positions along the pipeline, (B) maximum strain on the pipeline.
3.2.2.2 Pipeline crossing the landslide in a longitudinal orientation
3.2.2.2.1 Displacement of the pipeline
Figure 8A shows the displacement data for the pipeline at different positions under different landslide soil displacement distances. When the pipeline crosses the landslide longitudinally, the displacement of the pipeline increases with increasing landslide displacement. In the initial stage of the landslide, the displacement of the pipeline can be roughly considered as occurring in three stages. The pipeline outside the landslide has almost no displacement. The displacement of the pipeline inside the landslide increases gradually from the bottom to the top of the slope. There are obvious displacement points at the bottom and top of the slope. With the increase in the displacement of the landslide, the accumulation of soil at the bottom of the slope leads to a rapid increase in the displacement of the pipeline approximately 10 m from the foot of the slope and gradually becomes the maximum point of the pipeline displacement. The maximum soil displacement of the landslide body and the maximum displacement of the entire pipeline are extracted (Figure 8B). Initially, the maximum displacement of the pipeline increases significantly with the maximum displacement of the landslide. Then, the displacement of the pipeline slows down. Finally, the maximum displacement of the pipeline and the maximum displacement of the landslide body have an approximately linear increase. The displacement of the entire pipeline does not change strongly with the displacement of the landslide, and the linear relationship between the maximum displacement of the pipeline and the maximum displacement of the landslide is less than 0.2 from the overall analysis.
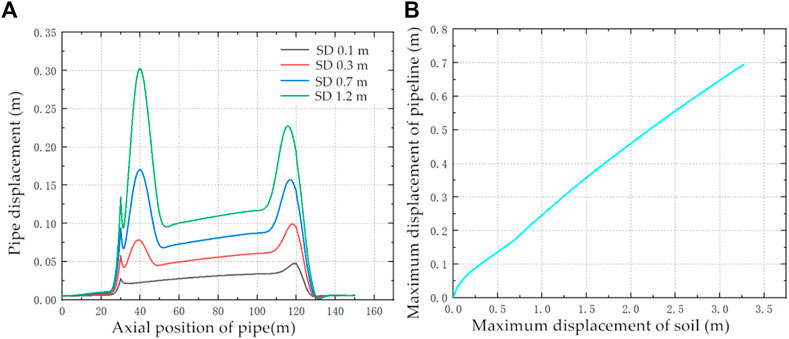
FIGURE 8. Relationship between pipeline displacement and landslide soil displacement (SD): (A) displacement at different positions of the pipeline, (B) maximum displacement of the pipeline.
3.2.2.2.2 Stress of the pipeline
Stress data along different positions of the pipeline under different landslide soil displacement distances are shown in Figure 9A. The stress on the pipeline increases with increasing landslide displacement. In the initial stage of the landslide, the pipeline stress at the top of the slope is significantly greater than that at the bottom of the slope. As the displacement of the landslide increases, the stress of the pipeline at the bottom of the slope increases rapidly and then exceeds the stress of the pipeline at the top of the slope. The section of pipeline approximately 10 m from the bottom of the slope also increases sharply with the increase in the displacement of the landslide, eventually exceeding the stress on the pipeline section at the top of the slope.
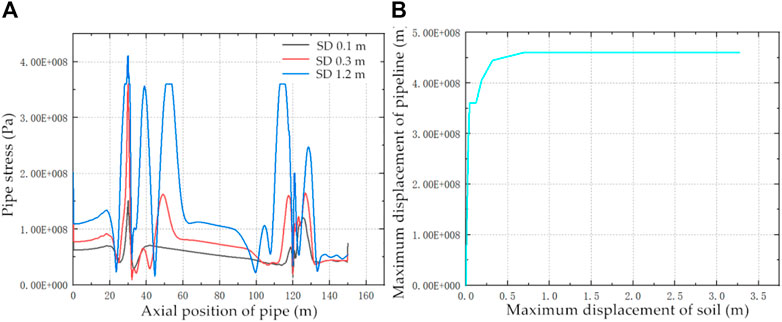
FIGURE 9. Relationship between pipeline stress and landslide soil displacement (SD): (A) stress at different positions of the pipeline, (B) maximum stress of the pipeline.
The maximum stress of the pipeline and the maximum displacement of the soil are shown in Figure 9B. When the pipeline crosses the landslide longitudinally, the maximum stress on the pipeline and the maximum displacement of the landslide have a three-stage relationship. In the initial stage of the landslide, the stress of the pipeline increases rapidly with the increase in the displacement of the landslide. With a further increase in the landslide displacement, the stress on the pipeline gradually increases until it reaches the strength limit of the pipeline.
3.2.2.2.3 Strain of the pipeline
Figure 10A shows the axial strain data at different positions of the pipeline under different landslide soil displacement distances. It is evident that the variable pattern of the strain along the whole pipeline is similar to the stress pattern of the pipeline observed for the longitudinal orientation. In the early stage of the landslide, the pipelines at the top and bottom of the landslide have the largest strain. Moreover, the strain of the pipeline at the top of the landslide, which is the maximum strain area of the entire pipeline, is greater than that at the bottom of the landslide. As the displacement of the landslide increases, the strain on the pipeline at the foot of the landslide exceeds the strain on the pipeline at the top of the landslide, thus becoming the largest strain area along the entire pipeline. Moreover, the strain on the pipeline at approximately 10 m from the bottom of the slope increases rapidly, which exceeds the strain on the pipeline at the top of the slope, becoming the second largest strain area of the pipeline. The pipeline at the top of the landslide is the third largest strain area of the pipeline. The strain on the other sections of the pipeline is small. In other pipeline sections, the strain changes are not large, despite increasing landslide displacement. The relationship of the maximum strain on the pipeline and the maximum displacement of the landslide soil are shown in Figure 10B. The pipeline strain increases with increasing landslide soil displacement. There is a linear relationship between pipeline strain and landslide soil displacement, with a correlation coefficient of 0.25.
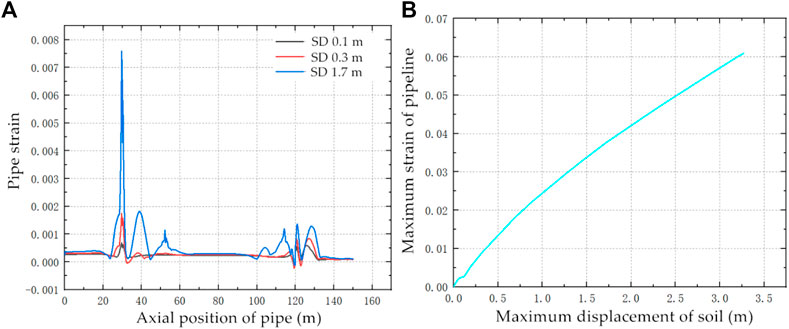
FIGURE 10. Relationship between pipeline strain and landslide soil displacement (SD): (A) strain at different positions of the pipeline, (B) maximum strain of the pipeline.
3.2.2.3 Mechanism of pipeline deformation
Where the pipeline crosses the landslide body laterally, it can be concluded from the stress, strain, and displacement data for the pipeline that the pipeline receives the largest force at the junction of the landslide body and the stable soil body, and it is most prone to damage. The mechanism driving pipeline damage when crossing the landslide laterally is mainly the combination of unstable and stable soil which acts on the pipeline. Consequently, the pipeline is subjected to excessive shear stress at the junction of the stable and unstable soil, causing pipeline damage.
When pipelines are oriented longitudinally to landslides, the effect of the landslide material on the pipeline is different at different locations along the pipeline. The pipeline section located at the top of the slope at the landslide trailing edge was mainly affected by the shear and tensile action of the soil, the pipeline at the foot of the landslide was mainly affected by the pressure of the soil, and the rest of the pipeline was affected by the friction and push of the soil. According to the data, with the development of landslide damage due to the accumulation of the landslide’s mass, the stress near the slope toe of the landslide increases rapidly, making that section of pipeline most likely to incur damage. Therefore, when the pipeline traverses the landslide vertically, the damage of the pipeline comes from the compression forces from the landslide body acting on the pipeline.
3.3 Influence of landslide geometry on pipeline deformation
3.3.1 Length of landslide
To conduct detailed analysis of the influence of landslide length on pipeline deformation and damage, landslide lengths of 30, 60, and 90 m were selected. Then, the maximum displacement of the landslide mass and maximum strain on the pipeline in the horizontal and vertical pipeline-crossing-landslide modes were extracted and analyzed (Figure 11) by numerically simulating the interaction between the pipeline and landslide.
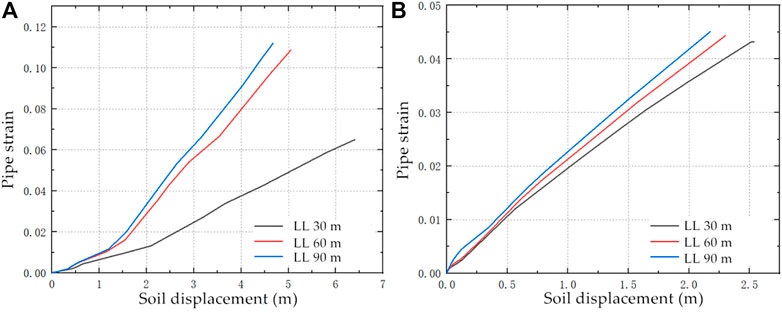
FIGURE 11. Influence of landslide length (LL) on the relationship between pipeline strain and landslide soil displacement: (A) pipeline crosses the landslide horizontally, (B) pipeline crosses the landslide vertically.
When the pipeline crossed the landslide horizontally, the maximum strain on the pipeline generally increased with the maximum displacement of the landslide soil mass. Two inflection points in this relationship were affected by the characteristics of the pipeline material. The first was in the initial stage of the landslide. At first, the landslide caused the pipeline to deform only slightly. However, the change rate of the maximum strain on the pipeline increased with the increase in the maximum displacement of the landslide. The second inflection point was observed after the landslide had travelled a certain distance, when the maximum strain on the pipeline increased with the maximum displacement of the landslide soil. The variation in landslide length did not change the mechanical modes acting on the pipeline or the relationship between the maximum strain on the pipeline and the maximum displacement of the landslide soil mass; however, the increase in landslide length did increase the maximum strain on the pipeline when subjected to the same landslide soil mass displacement. When the landslide length was 30 m, the soil displacement at the second point was 2.1 m; when the landslide length was 60 m, the soil displacement at the second point was 1.5 m; and when the landslide length was 90 m, the soil displacement at the second point was 1.2 m. It is evident that the lengthening of the landslide will cause the second inflection point between the maximum strain on the pipeline and the maximum displacement of the landslide soil mass to decrease. Comparing the correlation curves between the maximum pipeline strain and the maximum landslide soil displacement of different landslide lengths, we found that the larger the landslide length is, the less influence it has on the relationship between soil displacement and maximum pipeline strain.
When the pipeline crossed the landslide vertically, the overall pipeline strain had an approximately linear relationship with the maximum displacement of the landslide soil mass. Under the same soil displacement, the length of the landslide increased, and the strain of the pipeline increased; however, larger landslide lengths did not have smaller influences on the relationship between the displacement of the landslide soil and the maximum strain of the pipeline.
3.3.2 Landslide thickness
In order to conduct detailed analysis of the influence of landslide thickness on pipeline deformation and damage, the landslide thickness was set at 30, 60, and 90 m. The maximum displacement of the landslide mass and maximum strain on the pipeline in the horizontal and vertical modes were extracted and analyzed (Figure 12) by numerically simulating the interaction between the pipeline and landslide.
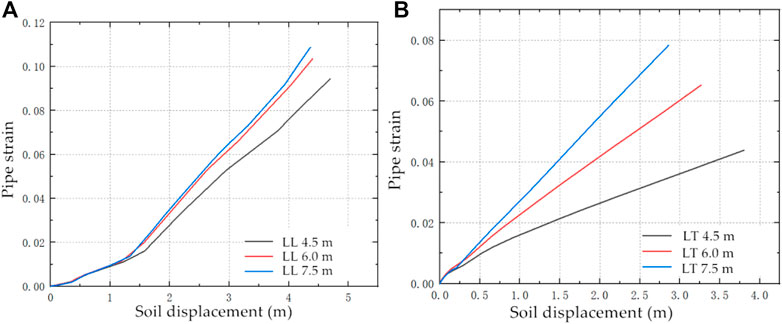
FIGURE 12. Influence of landslide thickness (LT) on the relationship between pipeline strain and landslide soil displacement: (A) pipeline crosses the landslide horizontally, (B) pipeline crosses the landslide vertically.
When the pipeline crosses the landslide horizontally, the landslide thickness does not change the rule that the maximum strain on the pipeline changes with the maximum displacement of the landslide soil. Under the same soil displacement conditions, the greater the landslide thickness is, the greater the maximum strain on the pipeline, and the lower the impact of that strain has on the pipeline. The greater the landslide thickness is, the greater the force acting on the pipeline will be, which will lead to a greater strain on the pipeline. As the depth of the pipeline is fixed, while increasing the thickness of the landslide will lead to an increase in the overall force of the soil, the deeper the thickness of the landslide is, the farther away the soil from the pipeline will be and the smaller the effect it has on the pipeline. Therefore, the deeper the landslide thickness is, the less influence it will have on the pipeline strain growth rate.
When the pipeline crossed the landslide vertically, the maximum strain of the pipeline was linearly related to the maximum displacement of the landslide soil. Under the same landslide displacement conditions, the greater the landslide thickness, the greater the pipeline strain. In addition, compared with the horizontal orientation, the strain on the pipeline was more significantly affected by the thickness of the landslide when the pipeline crossed the landslide vertically. Based on the above, it can be concluded that the maximum strain on the pipeline is located at the foot of the slope, which is compressed by the accumulation of mass. The increase in landslide thickness can directly increase the landslide mass, thus increasing the strain on the pipeline.
3.3.3 Slope of landslide
Generally, the larger the slope of the landslide, the more likely the landslide will occur and the faster its rate of deformation. Additionally, different slopes will affect the degree of deformation and damage of pipelines impacted by landslides. According to the survey data, the slopes of six typical pipeline landslides were between 15° and 40°, most being approximately 30°. Therefore, landslide slopes of 20°, 30°, and 40° were set to analyze the influence of the landslide slope on the law of deformation and damage of the pipeline (Figure 13).
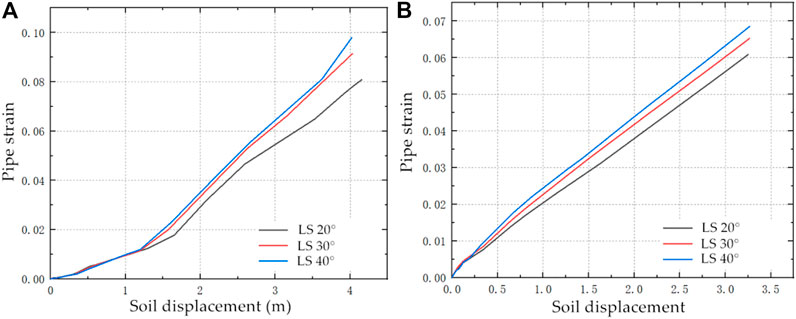
FIGURE 13. Influence of landslide slope on the relationship between pipeline displacement strain and landslide soil (LS): (A) pipeline crosses the landslide horizontally, (B) pipeline crosses the landslide vertically.
When the pipeline crosses the landslide horizontally, the overall trend of the relationship between the maximum strain of the pipeline and the maximum displacement of the landslide soil mass under different landslide slopes was consistent with the above two conditions. At the initial stage of the landslide, the relationship between the maximum strain on the pipeline with different slopes and the maximum displacement of the soil mass was the same. With the increase in soil displacement, the maximum strain on the pipeline increased with the increase in landslide slope under the same conditions, but the impact of the landslide slope on the pipeline decreased.
When the pipeline crossed the landslide vertically, the landslide slope did not affect the relationship between the maximum strain on the pipeline and the maximum displacement of the soil. When the displacement of the soil was the same, the maximum strain on the pipeline increased with an increasing slope. Therefore, when the slope was larger, the displacement of the pipeline was smaller.
3.3.4 Width of landslides
The increase in the width of the landslide will lead to a larger area of deformation and failure length for pipelines that cross landslides horizontally. Furthermore, the deformation and failure degree of the pipeline will be different with the different landslide widths. The pipeline was placed in the middle of the landslide, and the change in the landslide width did not influence the consistency of the relationship between the maximum strain of the pipeline and the maximum displacement of the soil. Therefore, this study only analyzed the influence of landslide width on the deformation and failure law of pipelines that cross landslides horizontally.
The maximum strain on the pipeline and the maximum displacement of the landslide soil mass were extracted and analyzed (Figure 14) from the horizontal pipeline model. The change in landslide width did not change the relationship between the maximum strain on the pipeline and the maximum displacement of the landslide soil, but when the distance of the soil displacement was the same, the maximum strain on the pipeline decreased with increasing landslide width. As the maximum strain of the pipeline was caused by the pipeline bending at the landslide boundary, when the landslide displacement was kept constant, smaller landslide widths induced larger bends in the pipeline at the landslide boundary; thus, the maximum strain of the pipeline was larger. In contrast, when the width of the landslide increased, the bending of the pipeline at the landslide boundary decreased, and the maximum strain on the pipeline decreased.
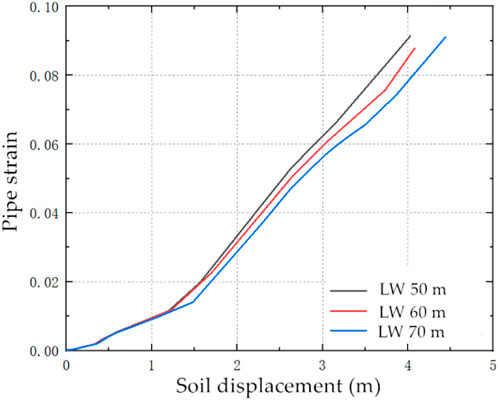
FIGURE 14. Influence of landslide width (LW) on the relationship between pipeline strain and landslide soil displacement.
3.3.5 The degree of influence
Different values of landslide geometric parameters (landslide length, thickness, slope, and width) will affect the deformation and failure law of pipelines within the landslide mass, but the influence of different parameters of landslide geometry on the deformation and failure law of pipelines is not clear. Understanding the strength of their influence can provide a reference and basis for disaster prevention and mitigation in terms of pipeline engineering. Therefore, it is necessary to carry out sensitivity analysis on the influence of these geometric parameters on the deformation and failure law of pipelines.
There are many methods for analyzing the sensitivity of multiple factors to a certain index, among which orthogonal experiments is a common and effective method.
When the pipeline crosses the landslide horizontally, the geometric parameters of the landslide that affect the deformation and failure of the pipeline in the landslide mass include the length, width, thickness, and slope. To compare the influence of the four factors on the deformation and failure of the pipeline, orthogonal experiments were used to set three levels for the four factors; nine groups of simulation tests were conducted for the analysis. The strain index was extracted for the pipeline when the displacement of the landslide soil mass was 1.6 m for each working condition in the simulation results (Table 4), where Rj was the comparative value of the influence degree of each factor calculated by the intuitive analysis method. The length of the landslide has the greatest impact on the deformation and failure of the pipeline, followed by the width, thickness, and slope.
When the pipeline crosses the landslide vertically, because the pipeline was laid on the central axis of the sliding direction of the landslide mass, the width of the landslide did not affect the deformation or damage law of the pipeline; therefore, only three geometric landslide characteristics—length, slope, and thickness—needed to be analyzed. The orthogonal experiment was used to set up three levels for the three factors, and a total of nine groups of experiments were used to compare the influence of the three factors on the deformation and damage law of the pipeline subjected to landslides. Similarly, the strain on the pipeline was extracted when the displacement of the landslide soil mass under each working condition was 1.6 m, and the comparative value Rj of the influence degree of each factor was calculated (Table 5). We found that the thickness of the landslide had the greatest impact on the deformation and damage law of the pipeline, followed by the length of the landslide and, finally, the slope of the landslide.
4 Model and evaluation of pipeline strain
The geometric landslide characteristics (length, width, thickness, and slope) and displacement are important parameters to assess the degree of pipeline deformation. Analyzing the relationship between the geometric characteristics and displacement of the landslide and the pipeline strain is key to establishing the pipeline strain calculation model.
The dimensional analysis method was used to analyze the relationship between the landslide and pipeline strain and to establish a calculation model. The length, thickness, slope, width, and displacement of the landslide are represented by L, D, A, W, and U, respectively. According to the dimensional analysis equation, the dimensions on the left and right sides are equal. Pipeline strain (ε) and landslide slope (A) are non-dimensional values. The dimensions of landslide length (L), thickness (D), width (W), and displacement (U) are, therefore, the dimensional values. However, these can be represented as the non-dimensional parameters L/d, D/d, W/d, and U/d, where d is the diameter of the pipeline.
When the pipeline is perpendicular to the direction of the landslide, the length, thickness, slope, width, and displacement of the landslide are the main factors affecting the deformation of the pipeline. The calculation model of the relationship between the pipeline strain and the geometric characteristics and displacement of the landslide is as follows:
where
This model was used to calculate the maximum strain value of the pipeline under various working conditions. The results of the model and the numerical simulation are compared, as shown in Figure 15A. The results show that the model results and the numerical simulation results are linearly correlated, and the linear slope is 0.975. Therefore, the calculation results of the model are reasonable.
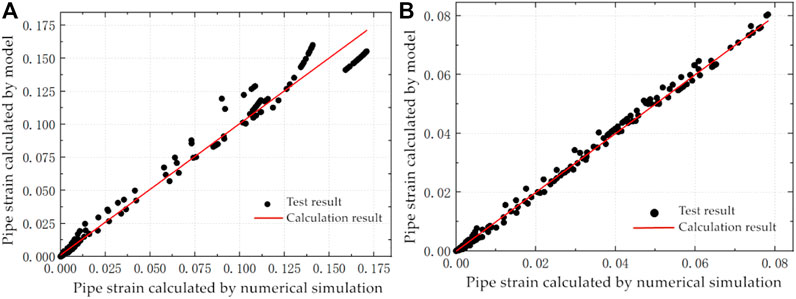
FIGURE 15. Comparison of strain model calculation results and numerical simulation results: (A) pipeline transversely crosses the landslide, (B) pipeline longitudinally crosses landslide.
When the pipeline is parallel to the sliding direction of the landslide, the soil mass of the landslide is evenly distributed on both sides of the pipeline, and the width of the landslide has no effect on the deformation of the pipeline. Therefore, the calculation model of the relationship between the pipeline strain and the geometric characteristics and displacement of the landslide is as follows:
where
This model was used to calculate the maximum strain on the pipeline under various working conditions. The results of the model and the numerical simulation are compared, as shown in Figure 15B. The results show that the model results and the numerical simulation results are linearly correlated, and the linear slope is 1.007. Therefore, the calculation results of the model are reasonable.
5 Conclusion
In this study, the strain on a pipeline oriented horizontally and vertically to a landslide is analyzed. Based on the research results, a calculation model of pipeline strain is established, and the conclusions are as follows:
(1) The deformation and damage mechanisms are different for pipelines with different orientations to landslides. When the pipeline crosses the landslide laterally, it is affected by the combined action of stable and unstable soil. The pipeline is subjected to considerable shear stress at the interface between these soils, which results in pipeline damage. When the pipeline crosses the landslide longitudinally, the pipeline is compressed by the landslide at the bottom of the slope, which results in pipeline damage.
(2) When the pipeline crosses the landslide laterally, the influence of landslide parameters on the pipeline from greatest to least are length, width, thickness, and slope of the landslide. When the pipeline crosses the landslide longitudinally, the influence of landslide parameters on the pipeline from greatest to least are thickness, length, and slope of the landslide.
(3) The pipeline strain calculation model constructed from the dimensionless analysis method can well reflect the influence of the various factors that influence pipeline strain and can accurately calculate the maximum strain on the pipeline.
Data availability statement
The datasets presented in this study can be found in online repositories. The names of the repository/repositories and accession number(s) can be found in the article/supplementary material.
Author contributions
NS: conceptualization, methodology, software, validation, formal analysis, investigation, and resources. L-lL: validation, formal analysis, investigation, and resources. Y-bM: software, validation, and formal analysis. Q-jL: investigation. L-yB: resources.
Conflict of interest
NS, L-lL, Y-bM, Q-jL, and L-yB were employed by General Institute of Science and Technology Company of Pipeline China.
Publisher’s note
All claims expressed in this article are solely those of the authors and do not necessarily represent those of their affiliated organizations, or those of the publisher, the editors and the reviewers. Any product that may be evaluated in this article, or claim that may be made by its manufacturer, is not guaranteed or endorsed by the publisher.
References
Feng, W. K., Huang, R. A., Liu, J. A., Xu, X., and Luo, M. (2015). Large-scale field trial to explore landslide and pipeline interaction. Soils Found. 55 (6), 1466–1473. doi:10.1016/j.sandf.2015.10.011
Han, B., and Fu, Q. (2020). Study on the pipeline fragility appraisal indicators affected by landslide based on numerical simulation. J. Phys. Conf. Ser. 1549 (4), 042123–123. doi:10.1088/1742-6596/1549/4/042123
Han, J. J., Huang, D. W., and Wang, H. C., (2022). Research on failure mechanism of a fuel gas pipeline transversely crossing landslide. Yangtze river 53 (1), 209–215.
Holliday, C., Young, A., and Funk, T., The north Sasktchewan river valley landslide- slope and pipeline condition monitoring. ASME13th Int. Pipeline Conf. (IPC). 19, 12, 10.1115/IPC2020-9532,
Li, Y. (2016). Gas supply resumed on July 30 after the Sinopec Sichuan-East gas pipeline explosion and combustion accident in Enshi, which took 10d. Gas Heat 36 (09), 22.
Ma, H. Q., He, B. X., and Cai, W. H., (2022). Prediction model of deformation distribution of buried natural gas pipeline under longitudinal action of landslide debris flow. J. Harbin Inst. Technol. 12, 1–9.
Niu, W. Q., Zheng, J., and Wu, H. G., (2015). Experimental study on effect of orthogonal landslide on pipeline by model simulation. Railw. Eng. 12 (06), 117–120.
Sun, Y. J., and Song, E. (2018). Dynamic simulation of “12·20” Shenzhen landslide. Chin. J. Geotechnical Eng. 40 (03), 441–448.
Vasseghi, A., Haghshenas, E., Soroushian, A., and Rakhshandeh, M. (2021). Failure analysis of a natural gas pipeline subjected to landslide. Eng. Fail. Anal. 119, 105009. doi:10.1016/j.engfailanal.2020.105009
Wang, G. L., Li, T. L., Xing, X. L., and Zou, Y. (2015). Research on loess flow-slides induced by rainfall in July 2013 in Yan’an, NW China. Environ. Earth Sci. 73 (12), 7933–7944. doi:10.1007/s12665-014-3951-9
Wang, R. Y., Zhang, J. M., and Wang, Cong (2021). Comparative analysis of statics and numerical simulation of buried pipeline under the action of lateral landslide. Sci. Technol. Eng. 21 (1), 326–333.
Xu, T. L., Ma, X. L., Lan, X. B., Jiang, H., and Wang, J. (2022). Research on treatment scheme of soil landslide in aviation oil pipeline based on oil-pipeline-soil multi-physical field coupling and anti-sliding simulation. Int. J. Press. Vessels Pip. 200, 104780–104814. doi:10.1016/j.ijpvp.2022.104780
Zahid, U., Godio, A., and Mauro, S. (2020). An analytical procedure for modelling pipeline-landslide interaction in gas pipelines. J. Nat. Gas Sci. Eng. 81, 103474. doi:10.1016/j.jngse.2020.103474
Zhang, L. S., Fang, M. L., Pang, X., Yan, X., and Cao, Y. (2018). Mechanical behavior of pipelines subjecting to horizontal landslides using a new finite element model with equivalent boundary springs. Thin-Walled Struct. 124 (5), 501–513. doi:10.1016/j.tws.2017.12.019
Keywords: deformation characteristics, strain calculation, pipeline, landslide, numerical simulation
Citation: Shi N, Li L-l, Ma Y-b, Li Q-j and Bai L-y (2023) Study of deformation characteristics and a strain calculation model for pipelines impacted by landslides. Front. Earth Sci. 10:1049740. doi: 10.3389/feart.2022.1049740
Received: 21 September 2022; Accepted: 02 December 2022;
Published: 04 January 2023.
Edited by:
Jie Dou, China University of Geosciences Wuhan, ChinaCopyright © 2023 Shi, Li, Ma, Li and Bai. This is an open-access article distributed under the terms of the Creative Commons Attribution License (CC BY). The use, distribution or reproduction in other forums is permitted, provided the original author(s) and the copyright owner(s) are credited and that the original publication in this journal is cited, in accordance with accepted academic practice. No use, distribution or reproduction is permitted which does not comply with these terms.
*Correspondence: Ning Shi, NTY0NzcwMzU5QHFxLmNvbQ==; Liang-liang Li, eWFudHUwNTIwNDEwOEAxNjMuY29t