- 1College of Civil and Architectural Engineering, Guilin University of Technology, Guilin, China
- 2Guangxi Key Laboratory of Geomechanics and Geotechnical Engineering, Guilin, China
As a prominent component of the construction field of sponge cities, ecological concrete is an essential tool to reach the goals of green, low-carbon living and sustainable development. A quantitative summary of the preliminary research on ecological concrete infrastructure in sponge city architecture is needed. Therefore, CiteSpace and VOSviewer were applied to perform a comparative analysis of the number of papers, countries, institutions, core authors, literature co-citations, research hotspots, and future trends in ecological concrete in the sponge city construction industry. The results show that the number of papers on ecological concrete is increasing, the research collaboration between domestic and foreign authors is relatively single, and there is insufficient interdisciplinary integration between institutions and the phenomenon of “relatively independent research.” The number of papers published in the field of ecological concrete construction has been on the rise, reaching more than 100 in each of the last 10 years, with China and the United States contributing more to the scientific output of the field. To meet the needs of global environmental protection and resource conservation, the theme of “promoting comprehensive resource conservation and recycling” will continue in the future, making concrete a feature of green, low-carbon, sustainable development and other areas of environmental protection in the construction field.
1 Introduction
Due to the rapid development of science and technology, environmental pollution, ecological damage, resource shortage, and flooding caused by the exploitation of land resources and rapid urbanization have seriously affected the quality of the global living environment and the stability of the ecosystem (Ji, 2017; Cohen., 2011; Pandey., 2015; Sayne., 2011; Chourou., 2011; Upadhya and Persson, 2015; Li at el., 2022). Currently, the construction industry consumes the primary source of energy and mineral resources in the world (Shi and Liu, 2019). It is also a major cause of environmental pollution. The energy consumption of the construction industry accounts for about 30–40% of the world’s energy consumption (Abdallah at el., 2016). Faced with such a dilemma, the upgrading of the construction industry has been initiated, and a green, environmentally friendly and sustainable development path has been adopted. Just as an understanding of the mechanical properties of gas hydrate reservoirs is fundamental to the commercial exploitation of gas hydrate (Tang et al., 2022), we need to have a general understanding of the applications of the construction industry if we are to improve the construction industry.
Therefore, there is an urgent need to explore and build sustainable development models for the construction industry to change the status quo of high resource consumption and severe environmental pollution. As a result, the construction industry is facing a tough test in its development, with environmental pollution and natural damage evident in concrete, the most widely used construction material. China collects a total of 50×109 tonnes of raw materials such as sand and gravel, limestone and clay each year, most of which is used in the production of concrete or cement, according to the data. Such a large collection directly leads to changes in the natural form of the mountain and the original posture of the riverbed, resulting in deepening natural disasters such as landslides and soil erosion. In addition, cement production emits many polluting gases, harmful substances that further endanger our ecological environment. Therefore, the development of new green concrete will be our main task from the past to the long future.
The concept of “ecological concrete” has been proposed, but the concept of ecological concrete is unclear, and there is no clear normative definition. In this paper, we can summarize it as green concrete, sustainable concrete, and environmentally friendly concrete. It consists of cement, coarse aggregate, and admixture, and is made by a particular process of construction. It has a unique structure that coexists with plants, alleviates biocontainment, expands green space, reduces environmental load and is in harmony with the ecological environment. Ecological concrete has a variety of functions that ordinary concrete does not. In other words, it is how concrete production can reduce energy consumption and environmental pollution while using materials, provided humans can maintain a good ecological environment. At the same time, due to its unique structure, ecological concrete has excellent water permeability and purification performance, allowing rainwater to infiltrate into the ground to reduce the frequent and severe urban flooding in recent years, thus coping with natural disasters caused by rainfall (Chen et al., 2016; Mo et al., 2016). As a hydrophilic material, ecological concrete has received a lot of attention, not only in the concrete industry, but also in many fields such as ecology and environmental protection, with great potential application value. It ushered in a period of development opportunities in sponge city construction projects such as water and sewerage, ecological restoration and governance. Ecological concrete has become the “infrastructure” in the construction field of sponge cities”.
Due to rapid urbanization, land use changes, and rapid socio-economic development processes, surface water flooding is now considered the most severe water-related problem in many major cities in China. 2014 saw the introduction of “sponge cities” in the People’s Republic of China, which will address urban surface water floodings and related urban water management issues, such as purification of urban runoff and attenuation of peak runoff, and water conservation (Chan et al., 2018). It is envisaged that practices will enhance natural ecosystems, provide more aesthetically pleasing spaces for people to live and work in urban environments, and improve urban habitats for birds and other organisms through nature-based solutions. As a natural life support system, the sponge city is important to support ecological civilization construction (Saravanan et el., 2019). It allows cities to flexibly adapt to environmental changes and cope with natural disasters caused by rainwater (Cai et al., 2016; Chen et al., 2016; Li et al., 2017; Shen et al., 2020). In addition, it can adjust the urban ecological balance and achieve human-water harmony (Hu et al., 2016). Therefore, sponge cities’ urbanization construction depends on the “infrastructure.” The plasticity, spatial expressiveness, texture and shape of ecological concrete, which is richer than the artistic effects of stone, is an essential technical support for building sponge cities.
With the proposal and promotion of green ecological sustainable development, urban construction projects are increasingly focusing on environmental protection and conservation while ensuring material requirements. Ecological concrete consists of cement, coarse aggregates, and additives, and is made using specific construction techniques. It has a unique structure that co-exists with plants, alleviates biological isolation, expands green spaces, reduces environmental load, and harmonizes with the ecological environment, contributing to the construction and development of sponge cities.
Ecologic concrete has been developed in some European countries since the 1940s. Due to technical limitations, the scope of its application is exceptionally constrained. Ecological concrete was added to the road during paving in 1970 and worked well, but was damaged by freezing and thawing a decade later. Ecological concrete was also used in the United States in 1979 for the construction of parking lots, permeable sidewalks and plazas. The built-up car park met standards for use in terms of strength and water penetration, and was generally well received by nearby residents. Nearly half of all tennis courts in France are built of ecological concrete. Japan is a country with many applications of ecological concrete. In the 1990s, the Ecological Concrete Engineering Society (Okaka., 2000; Tanaka., 1998) began studying plant-based concrete. In 2001, Japan’s “Advanced Science and Technology Research Center” applied porous concrete to the river bank protection (Sun et al., 2021).
Nowadays, ecological concrete needs unique material selection and proportioner design. Nitendrea (Palankar et al., 2015) uses steel scrap residue from steel production to replace traditional aggregate, and it is proven to achieve good results in improving concrete strength. Yu et al. (2015) used slag powder, lime powder, and other parts to replace cement as cementitious materials and achieved good results in terms of strength and durability. Mizuriaev et al. (2015) discussed the composition of general porous concrete and suggested the replacement of ordinary silicates with alkali metal silicates and non-expanding clays. These all show the continuous exploration and innovation in constructing sponge cities abroad.
Since the late 1990s, China began to study ecological concrete. Wu (1998) first proposed high-performance concrete with good environmental coordination performance. Xu et al. (2002) used specific concrete and seed mixture formula to protect and afforest rock slopes and published the patented technology of China Three Gorges University—CBS Vegetation Biotechnical Slope. Wei et al. (2005) developed vegetation concrete highway slope protection technology that combines masonry protection and ecological protection. Lawn plants were planted on the porous concrete slopes to achieve the highway greening effect. In Shanghai Huangpu River Ecological Slope Protection Demonstration Project (Chen et al., 2007), ecological concrete is used to build ecological slope protection along the Huangpu River. The results showed that the plants grew vigorously, the water and soil conservation were excellent, and the bank slope was safe and stable. It can be seen that ecological bank protection has essential functions such as flood control, drainage, and water diversion and ecological, environmental protection, and leisure functions (Huang and Xie, 2017). Wu et al. (2018) discussed the application of ecological concrete in constructing sponge cities. They studied the impact of the water-to-cement and bone-to-cement ratios on the compressive strength, water permeability and frost resistance of ecological concrete, providing theoretical guidance for engineering applications. Zhang (2018) studied the performance of planted concrete slope protection, and the results show that the erosion resistance and stability of the slope after grown concrete slope protection are improved. Yu (2018) studied the closure reverse filtration characteristics of ecological concrete on topsoil and the effect of two slope protection methods on its adsorption and decontamination capacity through rainfall stimulation tests.
Therefore, the inadequacy of ecological concrete compensation applied to sponge cities is discussed by reviewing ecological concrete research in the sponge city domain in a timely manner. This paper quantifies research in the ecological concrete area from 2000 to 2021. It analyzed the literature results retrieved by Web of Science (WoS) by combining CiteSpace, VOSviewer, and other visualization software. Then, the final aim is to comprehensively elucidate the essential background, research status, hot spots, and trends of global sponge city ecological concrete in terms of annual publications, significant countries, research institutions, scholars, and co-cited journals, to provide a reference for future research and practice of sponge city ecological concrete construction.
2 Data sources and research methods
In this paper, the analysis of the prevalence trends, domain distribution, and keyword factors of ecological concrete through core inclusion in the WoS database based on bibliometric analysis methods and information technology such as literature analysis software was carried out. WoS database is a subscription system that provides citation search services. Users have access to multiple databases that refer to interdisciplinary studies and can explore specialized subfields in depth (Wang et al., 2014; Yi et al., 2016).
2.1 Data sources
To obtain a comprehensive overview of the latest advances and trends in ecological concrete research in the field of the sponge city construction industry, the search steps were as follows: the search time was set from 1999-12-31 to 2021-12-31, with “ecological concrete” as the topic and TS (subject) = (“Ecological concrete OR Eco-concrete OR Green concrete OR pervious concrete OR Environmental friendly concrete OR Sustainable concrete”) as search criteria for advanced search, a total of 25353 documents were obtained, the research direction was selected as Construction Building Technology, and the article type was set as Article or Review, a total of 5300 articles were accepted.
2.2 Research methods
Currently, commonly used knowledge graph analysis software including VOSviewer, CiteSpace, etc. Are used to clearly and accurately analyze development trends, research fronts, research hotspots, and other related issues in a particular knowledge domain. CiteSpace uses the hot word analysis function to draw the knowledge map in the scientific area, and intuitively displays the research hotspots and development trends in a particular stage (Chen and Chen, 2005; Zhao et al., 2012; Chen et al., 2015; Li and Chen, 2016; Chen, 2017). VOSviewer is a software developed by Van Eck and Waltman at Leiden University in the Netherlands for constructing and visualizing econometric networks. It can build networks for journals, researchers, keywords, and publications based on co-citation, coupling, and co-authorship relationships (Waltman et al., 2010). This paper uses VOSviewer and CiteSpace software to analyze the number of published articles, publishing institutions, literature sources, core author groups, research hotspots, and future trends. Table 1 is the inclusion and exclusion criteria for the selected literature.
3 Results and analysis
3.1 Overview of research characteristics in the field of ecological concrete construction
In this paper, we analyze the number of papers published in the field of ecological concrete construction and the major source journals.
3.1.1 Analysis of the number of papers published in the field of ecological concrete construction
Statistics on the number of publications over the years provide insight into the development of the field. These data statistics assess an essential indicator of the popularity of academic research over time. Table 2 shows that the number of papers on ecological concrete is on the rise, with more than 100 papers published in the construction field since 2011. The average annual production of academic papers shows a rapid growth trend, indicating that research on green building projects is gaining importance globally, and sustainability goals are driving the green building movement. Green building projects originating from the green building movement are achieving unprecedented success because it provides a quantifiable measure of people’s efforts towards sustainable development. The development of ecological concrete is an emerging field, that is, keeping up with construction efforts while significantly contributing to green (Zhang and Wen, 2008). Advances in global environmental protection have driven the development of ecological concrete, which has gradually become the focus of the current construction industry, and related literature studies have been increasing. This area will remain a research hotspot in the future as the demand for ecological concrete increases.
3.1.2 Analysis of signficant source journals for research in the field of ecological concrete construction
Statistics on the distribution of high-frequency journals of ecological concrete application literature in various countries are useful to understand the activities of ecological concrete research institutions and to grasp the distribution of journals in the field of ecological concrete. After analyzing the distribution characteristics of high-frequency journals, Figure 1 shows the top ten journals that publish research articles related to this field, and it can be found that “Construction and Building Materials”, “Cement and Concrete Composites”, “Journal of Materials in Civil Engineering”, “Journal of Building Engineering”, “Materials and Structures”, “Journal of Sustainable Cement-based Materials”, “Case Studies in Construction Materials”, “ACI Materials Journal”, “Energy and Buildings” have paid much attention to the research on ecological concrete buildings, which has supported the field. Most primary source journals are concentrated in top journals in JSR I. Thus, it can be seen that although ecological concrete started late in the construction field, the attention of scholars and professional, authoritative journals from all walks of life has gradually increased with the keen global hope for the construction industry. From Table 3, the distribution of the aforementioned journals shows that research on ecological concrete is mainly focused on energy, environment, and ecology, and the discipline is exceptionally comprehensive.
3.2 Analysis of major research forces and collaborations in the ecological concrete construction industry
Analyzing the relationship between the number of papers published and the research results in the field of ecological concrete can provide research direction in this field and reflect the theoretical basis and practical value of cooperation in the research field to a certain extent, which can better highlight the Frontiers of research in the area (Xie et al., 2020). Interaction among scientists has long been the essence of scientific practice (Melin and Persson, 1996). Most stages of the research process involve a great deal of communication: scientists talk to each other; In short, they communicate and collaborate.
Models of Sino-foreign cooperation have emerged in a number of fields, including education and tourism. Similarly, in ecological city construction, Sino-foreign cooperation has been successful. De et al. (2013) identified the conditions for Sino-foreign cooperation in ecological cities, studied the organization of Sino-foreign cooperation, and constructed a typology of Sino-foreign cooperation in ecological knowledge cities. Their development has a critical impact on improving a region’s competitiveness in globalization (Ovalle et al., 2004; OECD, 2005). It requires robust organizational capacity to build such a foundation with broad participation from public and private players, academia and the wider community.The influx of high-tech knowledge usually comes from abroad, and promoting science and technology is also a key factor in the success of eco-towns.Hence, success is most likely to be achieved through collaboration within a solid consultation framework and exchange between individual scientists and institutions.
Collaborative analysis can be done specifically in terms of regions, institutions, and authors. When ecological concrete forms a systematic study in the construction industry, it facilitates the development of ecological concrete and forms a unique model and framework for ecological concrete research.
3.2.1 Analysis of major issuing countries and regions
From Figure 2, it can be seen that the Peoples of China, the United States, Australia, India, Canada, England, Malaysia, Spain, France, and Germany are the top 10 countries with the highest number of published works on ecological concrete. The top 10 high-profile countries for eco-concrete are spread across Asia, Europe and North America. Of these, China ranked first with 1,308 publications, followed by the United States with 808 and Australia with 414, while the remaining countries did not have more than 400 publications, with China, the United States and Australia accounting for 47.7 percent of the total. China and the United States have a large number of scientific achievements in the field of ecological concrete and are the leading publishers and strong promoters of ecological concrete research in construction (Hu and Milner, 2020).
To compare the changes in the number of articles issued by each country over time, Table 4 was created according to three time phases. As seen in Table 4, the Top 10 high-issuing countries of ecological concrete in the three phases were distributed in Europe, Asia, and North America. In phases 1 and 2, Canada and the United States are in the first place in terms of the number of publications, respectively.
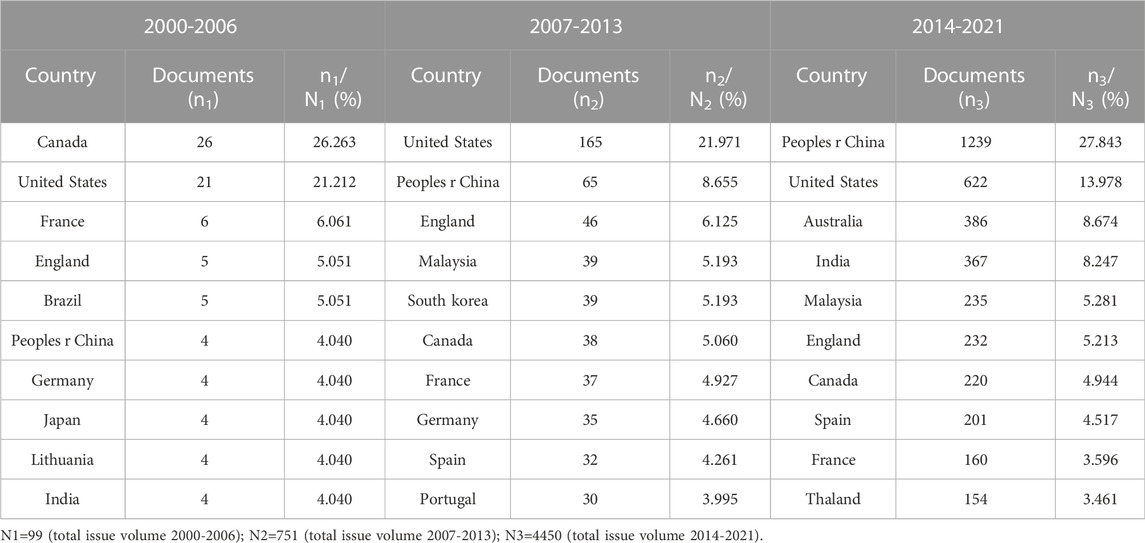
TABLE 4. Distribution of top 10 countries with high publication volume of ecological concrete in 3 phases.
In stage 3, China jumped to No. 1, which is related to the “sponge city” strategy proposed and implemented in China. The “sponge city” concept was first proposed in the “2012 Low Carbon City and Regional Development Science and Technology Forum” in 2012. Sponge City” concept in 2013, General Secretary Xi Jinping, in the “Central Urbanization Work Conference” speech, stressed the “construction of natural storage, natural infiltration, natural purification of sponge cities” in 2015, the introduction of the “guidance on promoting the construction of sponge cities” policy on China constructive guidance on the construction of sponge cities (Liu et al., 2017). The large-scale structure of concrete ecological projects and the large amount of state funding for scientific research have resulted in the output of a large number of scientific papers. Forming an ecological concrete system requires a lot of practice and time, and consuming human, material and financial resources is also a big concern. Therefore, it can be obtained that ecological concrete is mostly developed in highly productive and relatively developed areas. On the one hand, this is due to that the research of ecological concrete was first proposed and carried out by European countries. On the other hand, it’s due to the limited level of industrial development and weak scientific research in Africa, South Asia, and Southeast Asia, which do not pay much attention to this field.
Figure 3 shows the map of countries where ecological concrete is issued in the construction field. The more lines there are between countries, the more cooperation there is between countries. The thicker the lines are, the closer the cooperation is. The map clearly shows that the countries with more issuances cooperate more closely with other countries. Peoples r China, United States, Australia, India, Canada, England, Malaysia, Spain, France, and Germany play a linking role in the field of ecological concrete construction and have more cooperation with each other. However, many subject groups in other countries lack horizontal communication and cooperation, and they work independently with great limitations, which is not conducive to knowledge sharing in the field of ecologic concrete and limits the quantity and quality of research to a certain extent, which is not conducive to the formation of a unique global research model and framework of ecological concrete.
3.2.2 Analysis of major issuing scientific institutions
The analysis of global research institutions is an indispensable part to grasp the research priorities and trends of ecological concrete. Figure 4 shows that, in terms of issuing institutions, Wuhan Univ, Southeast Univ, Arizona State Univ, Swinburne Univ Technol, Khon Kaen Univ, Hong Kong Polytech Univ, Wuhan Univ Technol, Xian Univ Architecture & Technol, China Bldg Mat Acad, and Univ Ghent are the top 10 major research institutions. Considering that the application of ecological concrete needs industrialized technology revolution and government policy support, the future development needs more extensive participation of other institutions to realize the innovation of the whole chain of the industry, academia, and research. Individuals and institutions need to form more core groups, indicating that research groups mostly lack horizontal communication and cooperation, which is not conducive to knowledge flow and sharing in ecological context. The lack of a core group has led to limitations in the quality and level of research table 5.
3.2.3 Analysis of major high-yielding authors
In bibliometric research, quantitative analysis of authors can not only discover and analyze the foremost representative scholars in the field of ecologically specific research, but also reflect the academic contributions of authors in this field. The journals published by key authors play a fundamental role in this area of research and are an essential basis for measuring the formation of research communities. According to Price’s square root law, the number of publications of the least productive author should be equal to 0.749 times the square root of the number of publications of the highest influential authors (Li et al., 2013). The core author with the highest publication frequency is Caijun Shi, who has published 102 papers. As shown in formula (1):
Where M is the minimum number of papers to identify the core authors; Nmax is the number of documents published by the author with the most significant number of articles.
The integer calculation can identify authors with a publication volume greater than or equal to 8 as core authors in the field. According to Lockart’s law, when the proportion of authors with eight publications is about 60% of the total number of authors, it can be determined that a core group of authors in the field has been created (Wang and Wang, 2007). The core group of ecological concrete authors is listed in Table 6. It can be seen that 72 authors have published more than eight papers (including eight documents), and the total number of articles issued is 888. Authors who have published more than eight papers account for 8.11% of the total number of authors, which indicates that the core authors of ecological concrete in the construction domain are not yet formed.
Author collaboration network mapping was generated by VOSviewer software for core author group analysis. As in Figure 5, the size of the circles indicates the number of posts, and the thickness of the lines indicates the connections between authors who work closely together.
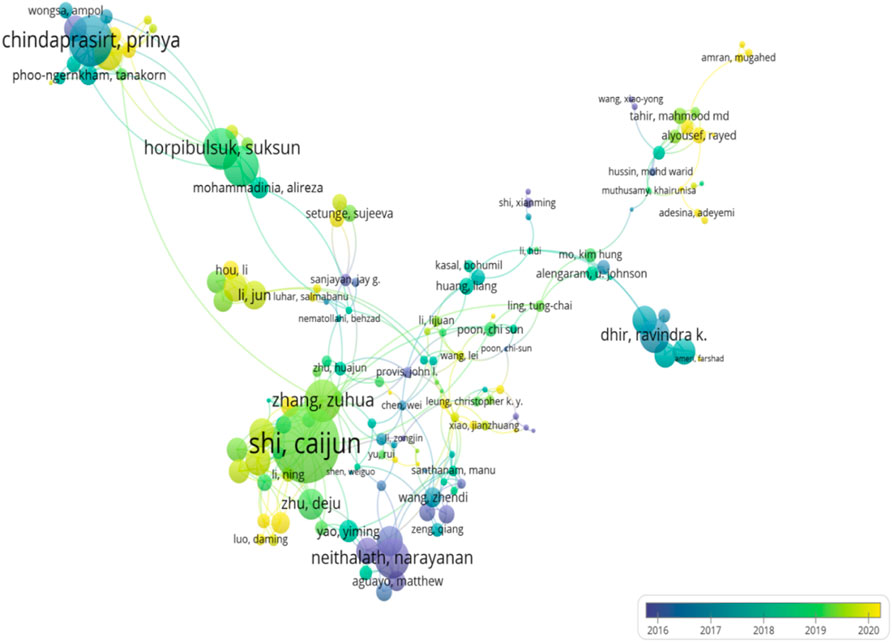
FIGURE 5. Collaborative network mapping of research authors in the field of ecological concrete construction.
It will be seen that the field of ecological concrete in architecture has only recently started to form several core groups of authors, which are the leading groups in the study of ecological concrete structures. Still, a large-scale author collaboration network has yet to form. In addition, most of the author collaborations are between domestic and foreign authors, and between foreign and domestic authors. It can be seen that with the global attention and promotion of ecological concrete, although its development is highly valued, the cooperation between authors is still weak. In the future research and development process, cooperation and mutual assistance between authors in different countries should be emphasized.
3.2.4 Co-citation analysis of literature on ecological concrete construction industry
To better explore the knowledge base and evolution of research in the field of ecological concrete buildings, this paper conducts a co-citation analysis of the literature related to the area of ecological concrete structures (Li and Chen, 2016), and uses CiteSpace to conduct co-citation analysis of the 5300 acquired documents, derives the top five papers in terms of co-citation frequency, and obtains a co-citation analysis table of ecological concrete literature to find its highly cited literature and understand. Critical results in this area have been obtained. The basic information of the top five positively mentioned literature in this area is shown in Table 7.
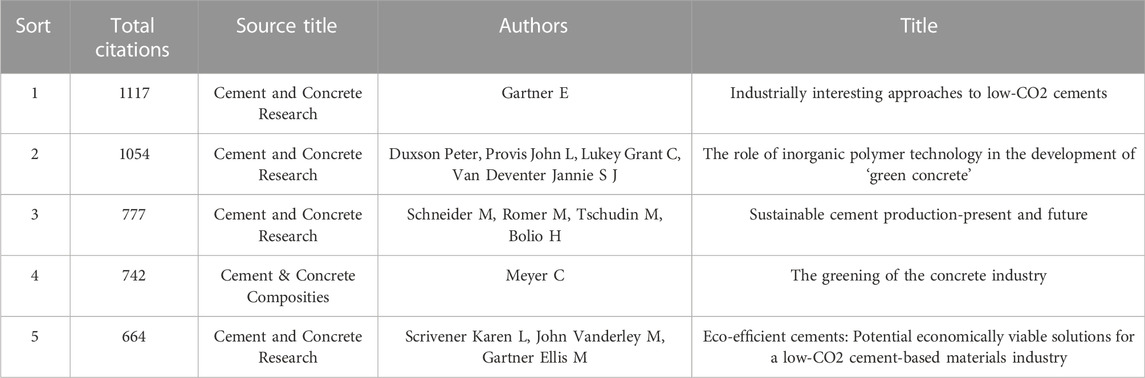
TABLE 7. Top five highly cited literature for research in the field of ecological concrete construction.
Gartner (2004) discusses the practicality of replacing silicate cement with industrial byproducts such as blast furnace slag and fly ash, and identifies the durability of concrete made from such cement. Duxson et al. (2007) discusses current and future developments in sustainable cement production. Meyer (2009) introduced some of the cementitious materials that replace Portland cement and found that significant progress has been made worldwide in replacing aggregates with renewable materials, the most important of which are recyclable aggregates, including scrapped glass, tires, plastics, and by-products from paper and other industries, and summarized recent progress in improving the situation where concrete, in general, is not particularly environmentally friendly or does not meet sustainability requirements. Environment et al. (2018) presents eco-efficient cement as an industrially viable solution.
4 Research hotspots and frontier analysis of ecological concrete
The analysis of frequency keywords is more limited as a hotspot for identification research. In this paper, the clustering analysis of keywords can deepen the keyword co-occurrence relationship and calculate a set of keywords with a closer connection to form a topic cluster containing multiple groups of words and further study the topic structure in the field of ecological concrete construction (Callon et al., 1991; Law and Whittaker, 1992).
4.1 Research hot topic analysis
To further explore the analysis of research hotspots in ecological concrete construction, this paper analyzes keyword clusters as well as keywords through Figure 6 and Table 8. The 14 groups are divided into two parts.
The first category of hot topics includes clusters such as #1 life cycle assessment, #12 sustainable design model, and #14 multi-scale computational model, which can be summarized as some methods, performance models, and evaluation systems based on the research of ecological concrete. “Construction”, “Design”, “Model”, “Pore structure ", “Pore structure”, and “Life cycle assessment” are the keywords of this section.
In China, based on research on recycled concrete technology, life-cycle assessment has been used to establish a whole-life-cycle environmental and economic performance assessment system for recycled concrete, analyzing, adjusting and setting key processes (Tian, 2012). A sustainable design model is a design that can be used on material structures to optimize the mix of building materials and reduce CO2 emissions. Choi et al. (2016) eveloped a sustainable design model for SRC composite structures, which was used to comprehensively analyze changes in CO2 emissions during construction of the building. Multiscale computational models are a method for multiscale modeling of interfacial connections in concrete structures. Gong et al., 2018 proposed a multi-scale simulation of visible mechanical damage to concrete caused by mixed microporous pressure. The combination of new models provides many new ideas for the study of ecological concrete (James et al., 2009; Jahn et al., 2012; Abson et al., 2017), and the integration of different models, disciplines, and fields is instructive for the development of ecological concrete in the construction field. Models can predict performance, and accurate models can reduce the number of experiments, shorten the experimental cycle, save time, cost, and materials, contribute to environmental protection, and enable new research topics and hot issues in the field to emerge and develop towards industrialization.
The second category of themes can be summarized as specific directions for the development of ecological concrete with the clusters #2 ultra- High-performance concrete、#3 recycled concrete aggregate、 #4 pervious concrete、#5 thermal properties、#6 3 days printing、#7 foamed concrete、#8 Glass cullet、#9 sodium chloride、#10 bfrp bar (Basalt fiber reinforced polymer (BFRP) bars)、#11 steel corrosion、#13 plastic waste these.
Due to the construction and development of railroads, highways, mines, and water conservancy projects (Andrew, 1990; Bennett, 1991; Cohen-Fernández and Naeth, 2013; Shao at el., 2014), a large number of exposed rock slopes and fragile banks slopes have been formed (Bengtsson at el., 2000; Zeng et al., 2020; Walia et al., 2017; Zeng et al., 2019). Affected by natural climates such as rainfall, weathering, and dry-wet cycles, the slopes are prone to runoff, resulting in a large amount of soil erosion (Caro et al., 2014; Handa et al., 2019). In this case, ecological concrete can meet the primary responsibilities of concrete and ecological conservation of biodiversity. Compressive strength, porosity, PH value, and vegetation properties affect its mechanical properties because when porosity is too high, good permeability promotes the decomposition of hydrates and reduces strength, while admixtures will inevitably affect durability (Wu et al., 2005; Wang et al., 2022). With the increasing demand for environmental protection and conservation, ecological concrete has attracted more and more attention (Zhang and Wen, 2008; Xiang et al., 2020). Previously, ecological concrete was mostly used in slope protection where the alkaline environment restricted the growth of plants (Shi et al., 2011; Wang et al., 2019; Wasim et al., 2022), so fly ash, silica fume, and rice hull ash are applied in vegetation concrete to replace cement and Portland cement to reduce the alkalinity of the internal environment while maintaining strength.
An essential technological breakthrough in ecological concrete is the reuse of waste concrete (Yu and Yu, 2009), and recycled concrete is a joint research topic in developed countries. So far, this has been done using recycled aggregates and waste materials such as corn cobs, shells, glass fragments, fabric and plastic waste, rather than ordinary aggregates made from recycled concrete. Pervious concrete has been increasingly used to reduce water runoff and improve water quality near sidewalks and parking lots, with high performance requirements, often with external admixtures such as silica fumigants and high-efficiency water dewatering agents to improve its performance. Foam concrete has high strength-to-weight ratio and low density, among other things. Its use reduces static loads on structures and foundations, which is beneficial for energy savings and reduces labor costs during construction. Amran et al., 2015 examines the composition, fabrication techniques and properties of foam concrete, providing a comprehensive insight into possible applications of foam concrete in today’s construction industry.
Due to the rapid development of ecological concrete, it has become a trend to combine technical and common concrete. 3D printed concrete combines 3D printing and concrete, and there are still many challenges in the level of technology and processing. 3D printed concrete is a new type of digitally manufactured concrete, with the addition of technology to increase shape complexity and reduce the use of materials in concrete, which is more environmentally friendly but lacks rheological and hardening properties. Numerical simulation and machine learning, an emerging method combined with ecological concrete construction, is an inevitable trend in concrete development (Jiang et al., 2018; Chang et al., 2020; Huang et al., 2020).
To promote the mass use of ecological concrete for the sustainable development of the world (Kim and Park., 2016; Gao et al., 2020; Faiz et al., 2022; Kong et al., 2022), researchers need to continue their efforts to realize the industrial production of ecological concrete table 8.
4.2 Analysis of research knowledge evolution
Emergent words, which can provide researchers with the latest evolution and direction of the discipline, refer to a sudden increase in the citation frequency of a particular document within a certain period. A larger number of citations indicates that many related studies have appeared. Thus, the recent emergent nature represents a research Frontier. Using the mutation detection algorithm of CiteSpace V, information on emergent words is obtained, as shown in Figure 7. After analysis, this period can be divided into three points in time. By tracing these emergent words, we can find that they are closely related to ecology and that the protection of the global environment and sustainable development have been the primary goals in the world since the last century, even now and in the future (Jacobs, 1999).
Since 2000, concrete has been required not only to meet the requirements of being a structural material, but also to minimize load and negative impact on the global environment and contribute to global sustainability. Gupta et al., 2021 is focused on developing green self-compacting concrete using industrial by-products such as foundry waste sand, coal bed ash, waste tire rubber, copper slag and waste glass as acceptable aggregates substitutes.These industrial by-products have great potential for use in self-compacting concrete, leading to sustainable development. The reuse of waste concrete (Yu and Yu, 2009) is an essential technological breakthrough in the field of ecological concrete. There are many studies on the effect of waste concrete as recycled aggregate on the performance of recycled concrete (Chen et al., 2013; Zhang et al., 2018). Zou and Cao (2020) used waste concrete with different strengths to make regenerate aggregates and study their properties. In addition, attention has been paid to the resource utilization of industrial waste through innovative disposal methods, one of which is its use as a filling material or volcanic ash for concrete production. Ji et al. (2021) and Liu et al. (2021) prepared corn cob aggregate ecological concrete by mixing broken corn cob particles into recycled mortar. Xie et al. (2021) and Liu et al. (2020) prepared ecological concrete using volcanic stone as coarse aggregate with different porosity. Murugan et al. (2020) used shells as a substitute for granite aggregate. Olofinnade et al. (2018) used waste glass in concrete to achieve sustainable benefits of reducing solid waste and greenhouse gas emissions. Javali et al. (2017) proposed using aluminum slag and granular iron slag as partial replacement materials for cement and natural sand, respectively, to develop ecological concrete. The research on recycled aggregates has important implications for energy saving and emission reduction, as well as a new innovative direction for ecological concrete.
Since 2011, the concept of “life cycle” has been emerging. The basic idea of a life-cycle assessment is that all environmental burdens associated with a product or service must be assessed, from the raw material all the way through to waste disposal. When the need for raw concrete materials shifted to the study of recycled materials, it entered the “life cycle” category. The Life Cycle Assessment (LCA) is the only environmental assessment tool that avoids a positive rating that only includes the measurement of the transfer burden. Life-cycle assessment can provide recommendations for the optimal design of ecological concrete processes, suggesting strategies and policies for industrialization of recycled concrete that are consistent with national conditions, taking into account the issues that need to be addressed in the promotion of ecological concrete. After 2016, it can be concluded from the emergent words such as lightweight aggregate concrete, high strength, and permeable pavement that the development of ecological concrete tends to mature with the improvement of the economy and technology, and there are higher requirements for other properties other than the basic performance, such as light aggregate concrete and high strength permeable pavement. Lightweight aggregate concrete is characterized by its light weight, high strength, thermal insulation, fire resistance and good seismic performance, as well as its good deformability, low modulus elasticity and low shrinkage creep. It can be used in industrial and civil construction, among other projects, and has the advantage of reducing the weight of structures and saving material consumption. Permeable pavement makes porous concrete in cold climates to solve freeze-thaw and crack resistance problems. It can be seen that the development of ecological concrete in the construction sector is getting better, and the transition from ordinary ecological concrete to high-performance ecological concrete is just around the corner.
5 Conclusion
In this paper, we use the Web of Science core database as a data source and perform a statistical and visual analysis of the ecological concrete related literature in the field of sponge city construction from 31 December 1999 to 31 December 2021 with the help of CiteSpace and VOSviewer software. The study was conducted in a variety of aspects, such as number of publications, discipline type, core country, institution, authors, etc. The combination of clustering, keyword, and emergence analysis is used to effectively reflect the current status, hot spots, and frontiers of ecological concrete research in construction with high research reliability. However, sampling also lacks representability due to many imperfections in the literature. Thus, the literature mainly reflects information collected from dominant groups, and these issues still have to be overcome to obtain the most accurate data possible.
1) The research of ecological concrete is mostly concentrated in relatively developed regions, while relatively backward regions are in the state of “relatively independent research”, without core groups between individuals and institutions, which indicates that most research groups lack horizontal communication and cooperation, which is not conducive to the flow and sharing of knowledge in the field of ecological concrete. Strengthening cooperation among different subjects and directions is the key to interdisciplinary integration, and strengthening research cooperation is the key to the development of ecology.
2) Combining emerging methods such as methodological models, numerical simulations, and machine learning with ecological concrete to establish certain ecological concrete assessment systems, integrating different models, subjects, and different fields is the future trend that can increase the application of ecological concrete in construction and make it develop towards industrialization and popularization.
3) Different types of waste are used in place of cement or aggregates to make ecological concrete. In the future, there will be more diversity in alternative cement materials, innovation of more environmentally friendly ecological concrete technologies based on waste-resolved and recycled aggregates, and promotion of recycling of global construction waste in ecological concrete. Australia’s water resources: from use to management, 2007, Abdallah et al., 2016, Bengtsson et al., 2000, Chourou, Cohen, 2011, De Oliveira Andrade et al., 2018, Environmental Change Disasters and Security, 2011, Harris, 2008, Li et al., 2022, Liu et al., 2000, Owaid et al., 2013, Pandey, 2015, Saravanan et al., 2019, Sayne, 2011, Sergey et al., 2015, Shao et al., 2014, Tanaka, 1998, Vieira et al., 2016.
Author contributions
All authors listed have made a substantial, direct, and intellectual contribution to the work and approved it for publication.
Funding
This research was funded by the National Natural Science Foundation of China (41867039), Guangxi Key Laboratory of Geomechanics and Geotechnical Engineering (20-Y-XT-03), and Foundation of Technical Innovation Center of Mine Geological Environmental in Southern Area (CXZX2020002).
Conflict of interest
The authors declare that the research was conducted in the absence of any commercial or financial relationships that could be construed as a potential conflict of interest.
Publisher’s note
All claims expressed in this article are solely those of the authors and do not necessarily represent those of their affiliated organizations, or those of the publisher, the editors and the reviewers. Any product that may be evaluated in this article, or claim that may be made by its manufacturer, is not guaranteed or endorsed by the publisher.
References
Abdallah, M., El-Rayes, K., and Liu, L. (2016). Optimizing the selection of sustainability measures to minimize life-cycle cost of existing buildings. Can. J. Civ. Eng. 43 (2), 151–163. doi:10.1139/cjce-2015-0179
Abson, D. J., Fischer, J., Leventon, J., Newig, J., Schomerus, T., Vilsmaier, U., et al. (2017). Leverage points for sustainability transformation. Ambio 46 (1), 30–39. doi:10.1007/s13280-016-0800-y
Amran, Y. H. M., Farzadnia, N., and Ali, A. A. A. (2015). Properties and applications of foamed concrete; a review. Constr. Build. Mater. 101, 990–1005. doi:10.1016/j.conbuildmat.2015.10.112
Andrew, A. (1990). Fragmentation of habitat by roads and utility corridors: A review. Aust. Zool. 26, 130–141. doi:10.7882/az.1990.005
Bengtsson, J., Nilsson, S. G., Franc, A., and Menozzi, P. (2000). Biodiversity, disturbances, ecosystem function and management of European forests. For. Ecol. Manage. 132, 39–50. doi:10.1016/s0378-1127(00)00378-9
Bennett, A. F. (1991). “Roads, roadsides and wildlife conservation: A review,” in Nature conservation 2: The role of corridors. Editors D. A. Saunders, and R. J. Hobbs (Australia: Surrey Beatty & Sons), 99–117.
Cai, B., Wang, J., He, J., and Geng, Y. (2016). Evaluating CO2 emission performance in China’s cement industry: An enterprise perspective. Appl. energy 166, 191–200. doi:10.1016/j.apenergy.2015.11.006
Callon, M., Courtial, J. P., and Laville, F. (1991). Co-word analysis as a tool for describing the network of interactions between basic and technological research: The case of polymer chemsitry. Scientometrics 22, 155–205. doi:10.1007/bf02019280
Caro, T., Dobson, A., Marshall, A. J., and Peres, C. A. (2014). Compromise solutions between conservation and road building in the tropics. Curr. Biol. 24, 722–725. doi:10.1016/j.cub.2014.07.007
Chan, F. K. S., Griffiths, J. A., Higgitt, D., Xu, S., Zhu, F., Tang, Y. T., et al. (2018). Sponge City” in China-a breakthrough of planning and flood risk management in the urban context. Land use policy 76, 772–778. doi:10.1016/j.landusepol.2018.03.005
Chang, Z., Du, Z., Zhang, F., Huang, F*., Chen, J., Li, W., et al. (2020). Landslide susceptibility prediction based on remote sensing images and GIS: Comparisons of supervised and unsupervised machine learning models. Remote Sens. 12, 502. doi:10.3390/rs12030502
Chen, C., and Chen, Y. (2005). Searching for clinical evidence in CiteSpace. AMIA Annu. Symp. Proc. 2005, 121–125.
Chen, C. (2017). Science mapping: A systematic review of the literature. J. Data Inf. Sci. 2 (2), 1–40. doi:10.1515/jdis-2017-0006
Chen, P., Zhang, J., Sun, Y., and Liu, X. (2016). Wargame simulation theory and evaluation method for emergency evacuation of residents from urban waterlogging disaster area. Int. J. Environ. Res. Public Health 13 (12), 1260. doi:10.3390/ijerph13121260
Chen, Y., Chen, C. M., and Liu, Z. Y. (2015). Methodological function of CiteSpace knowledge map. Sci. Res. 33, 242–253.
Chen, Y. H., Wu, Y. F., and Lv, X. W. (2007). Application of ecological concrete in river slope protection. Soil water Conservation China (6), 42.
Chen, Z. P., Xu, J. J., Zheng, H. H., Su, Y. S., Xie, J. Y., and Li, J. T. (2013). Basic mechanical properties test and stress-strain constitutive relations of recycled coarse aggregate concrete. J. Build. Mater. 16 (1), 24–32.
Choi, S. W., Oh, B. K., Park, J. S., and Park, H. S. (2016). Sustainable design model to reduce environmental impact of building construction with composite structures. J. Clean. Prod. 137, 823–832. doi:10.1016/j.jclepro.2016.07.174
Cohen, S. (2011). Sustainability management: Lessons from and for New York city, America, and the planet. New York, United States, Columbia University Press.
Cohen-Fernandez, A. C., and Naeth, M. A. (2013). Erosion control blankets, organic amend-ments and site variability influenced the initial plant community at a limestonequarry in the Canadian Rocky Mountains. Biogeosciences 10, 5243–5253. doi:10.5194/bg-10-5243-2013
De Jong, M., Yu, C., Chen, X., Wang, D., and Weijnen, M. (2013). Developing robust organizational frameworks for sino-foreign eco-cities: Comparing Sino-Dutch shenzhen low carbon city with other initiatives. J. Clean. Prod. 57, 209–220. doi:10.1016/j.jclepro.2013.06.036
De Oliveira Andrade, J. J., Wenzel, M. C., da Rocha, G. H., and da Silva, S. R. (2018). Performance of rendering mortars containing sludge from water treatment plants as fine recycled aggregate. J. Clean. Prod. 192, 159–168. doi:10.1016/j.jclepro.2018.04.246
Duxson, P., Provis, J. L., Lukey, G. C., and Van Deventer, J. S. (2007). The role of inorganic polymer technology in the development of ‘green concrete. Cem. Concr. Res. 37 (12), 1590–1597. doi:10.1016/j.cemconres.2007.08.018
Environment, U. N., Scrivener, K. L., John, V. M., and Gartner, E. M. (2018). Eco-efficient cements: Potential economically viable solutions for a low-CO2 cement-based materials industry. Cem. Concr. Res. 114, 2–26. doi:10.1016/j.cemconres.2018.03.015
Faiz, H., Ng, S., and Rahman, M. (2022). A state-of-the-art review on the advancement of sustainable vegetation concrete in slope stability. Constr. Build. Mater. 326, 126502. doi:10.1016/j.conbuildmat.2022.126502
Gao, J., Liu, D., Xu, Y., Chen, J., Yang, Y., Xia, D., et al. (2020). Effects of two types of activated carbon on the properties of vegetation concrete and Cynodon dactylon growth. Sci. Rep. 10 (1), 14483–14512. doi:10.1038/s41598-020-71440-w
Gartner, E. (2004). Industrially interesting approaches to “low-CO2” cements. Cem. Concr. Res. 34 (9), 1489–1498. doi:10.1016/j.cemconres.2004.01.021
Gong, F., Takahashi, Y., and Maekawa, K. (2018). Multi-scale computational modeling for concrete damage by mixed pore pressures-case of coupled alkali-silica reaction and cyclic freeze/thaw. Engineering Computations, 35, 4, doi:10.1108/EC-11-2017-0431
Gupta, N., Siddique, R., and Belarbi, R. (2021). Sustainable and greener self-compacting concrete incorporating industrial by-products: A review. J. Clean. Prod. 284, 124803. doi:10.1016/j.jclepro.2020.124803
Handa, S., Aggaral, R. K., and Bhardwaj, S. K. (2019). A critical review on environmental impact assessment of highway development in Himalayan region. IJCS 7, 28–35.
Harris, P. G. (2008). Green or Brown? Environmental attitudes and governance in greater China. Nat. Cult. 3 (2), 151–182. doi:10.3167/nc.2008.030202
Hu, M., and Milner, D. (2020). Visualizing the research of embodied energy and environmental impact research in the building and construction field: A bibliometric analysis. Dev. Built Environ. 3, 100010. doi:10.1016/j.dibe.2020.100010
Hu, Q. F., Wang, Y. T., Xu, H. B., Liu, Y., and Cui, T. T. (2016). Five technical considerations for sponge city construction. Water Resour. Prot. 32 (05), 152–153.
Huang, F. M., Cao, Z. S., Jiang, S. H., Zhou, C. B., and Guo, Z. Z. (2020). Landslide susceptibility prediction based on a semi-supervised multiple-layer perceptron model. Landslides 17, 2919–2930. doi:10.1007/s10346-020-01473-9
Huang, X. P., and Xie, J. (2017). Types of ecological revetments in river regulation. Port Waterw. Eng. 10, 2, 109–113.
Jacobs, M. (1999). Sustainable development as a contested concept. Fairness Futur. Essays Environ. Sustain. Soc. justice 1, 21–46.
Jahn, T., Bergmann, M., and Keil, F. (2012). Transdisciplinarity: Between mainstreaming and marginalization. Ecol. Econ. 79, 1–10. doi:10.1016/j.ecolecon.2012.04.017
James, P., Tzoulas, K., Adams, M. D., Barber, A., Box, J., Breuste, J., et al. (2009). Towards an integrated understanding of green space in the European built environment. Urban For. Urban Green. 8 (2), 65–75. doi:10.1016/j.ufug.2009.02.001
Javali, S., Chandrashekar, A. R., Naganna, S. R., Manu, D. S., Hiremath, P., Preethi, H. G., et al. (2017). Eco-concrete for sustainability: Utilizing aluminium dross and iron slag as partial replacement materials. Clean. Technol. Environ. Policy 19 (9), 2291–2304. doi:10.1007/s10098-017-1419-9
Ji, X. S., Li, Q. Y., and Liu, J. (2021). Preparation and properties of ecological concrete with maize-cob aggregate. Trans. Chin. Soc. Agric. Eng. 37 (06), 289–294.
Ji, Y. (2017). Status and treatment model of water resources pollution in the yellow river basin of China. Nat. Environ. Pollut. Technol. 16 (3).
Jiang, S. H., Huang, J., Huang, Faming*, Yang, Jianhua, Yao, Chi, and Zhou, Chuangbing (2018). Modelling of spatial variability of soil undrained shear strength by conditional random fields for slope reliability analysis. Appl. Math. Model. 63, 374–389. doi:10.1016/j.apm.2018.06.030
Kim, H. H., and Park, C. G. (2016). Performance evaluation and field application of porous vegetation concrete made with by-product materials for ecological restoration projects. Sustainability 8 (4), 294. doi:10.3390/su8040294
Kong, L., Wang, X., Guo, W., and Zhang, Y. (2022). Effects of municipal solid waste on planting properties and scouring resistance of vegetation concrete (wuhan, China). Int. J. Environ. Res. Public Health 19 (13), 8143. doi:10.3390/ijerph19138143
Law, J., and Whittaker, J. (1992). Mapping acidification research: A test of the co-word method. Scientometrics 3, 417–461. doi:10.1007/bf02029807
Li, H., Ding, L., Ren, M., Li, C., and Wang, H. (2017). Sponge city construction in China: A survey of the challenges and opportunities. Water 9 (9), 594. doi:10.3390/w9090594
Li, J., and Chen, C. M. (2016). CiteSpace: Scientifific text mining and visualization. Beijing, China: Capital University of Economics and Business Press, 78–89.
Li, X., Wang, Y., Hu, Y., Zhou, C., and Zhang, H. (2022). Numerical investigation on stratum and surface deformation in underground phosphorite mining under different mining methods. Front. Earth Sci. (Lausanne). 10. doi:10.3389/feart.2022.831856
Li, Y., Zhao, L. Z., and Li, H. X. (2013). Visual analysis of papers published in China safety science journal from 1991 to 2012. China Saf. Sci. J. 23 (9), 136–141.
Liu, A. Y., Li, R. J., Qin, X. L., and Gong, A. M. (2000). Research progress of ecological concrete application. ChinaOkaka W. The interaction between concrete and its environment: An overview.
Liu, F. P., Xie, F., Xu, J., Gong, A. M., and Chen, C. W. (2020). Exploration on the application of volcanic rock ecological concrete in ecological reservoir slope protection. Water Power 46 (11), 61–64.
Liu, H., Jia, Y., and Niu, C. (2017). Sponge city” concept helps solve China’s urban water problems. Environ. Earth Sci. 76 (14), 473–475. doi:10.1007/s12665-017-6652-3
Liu, J., Zhang, K., Li, Q. Y., and Yue, G. B. (2021). Experimental study on the performance of corn-cob aggregate ecological concrete. Concrete 11(9), 67–70+74.
Melin, G., and Persson, O. (1996). Studying research collaboration using co-authorships. Scientometrics 36 (3), 363–377. doi:10.1007/bf02129600
Meyer, C. (2009). The greening of the concrete industry. Cem. Concr. Compos. 31 (8), 601–605. doi:10.1016/j.cemconcomp.2008.12.010
Mo, K. H., Alengaram, U. J., Jumaat, M. Z., Yap, S. P., and Lee, S. C. (2016). Green concrete partially comprised of farming waste residues: A review. J. Clean. Prod. 117, 122–138. doi:10.1016/j.jclepro.2016.01.022
Murugan, S., Natarajan, M., Karthik, V., and Johnpaul, V. (2020). Withdrawn: Utilization of cockle shell aggregates in the production of eco-concrete. Mater. Today Proc. (republish). doi:10.1016/j.matpr.2020.10.511
Mizuriaev, S. A., Zhigulina, A. Y., and Solopova, G. S. (2015). Production technology of waterproof porous aggregates based on alkali silicate and non-bloating clay for concrete of general usage. Procedia Eng. 111, 540–544.
Oecd. Development (Paris), (2005). “Organisation de coopération et de développement économiques (Paris), Statistical Office of the European Communities, Development (OECD) Staff, Development,” in Oslo manual: Guidelines for collecting and interpreting innovation data, Paris, (Development Centre, Society for International DevelopmentOrg. for Economic Cooperation & Development).
Olofinnade, O. M., Ede, A. N., Ndambuki, J. M., Ngene, B. U., Akinwumi, I. I., and Ofuyatan, O. (2018). Strength and microstructure of eco-concrete produced using waste glass as partial and complete replacement for sand. Cogent Eng.Standardization 5 (114), 148386042–148386046. doi:10.1080/23311916.2018.1483860
Ovalle, M. R. G., Márquez, J. A. A., and Salomón, S. D. M. (2004). A compilation of resources on knowledge cities and knowledge-based development. J. Knowl. Manag. 8, 107–127. doi:10.1108/13673270410558819
Owaid, H. M., Hamid, R., Abdullah, S. R. S., Kofli, N. T., and Taha, M. R. (2013). Physical and mechanical properties of high performance concrete with alum sludge as partial cement replacement. J. Teknol. 65 (2). doi:10.11113/jt.v65.2198
Palankar, N., Shankar, A. U. R., and Mithun, B. M. (2015). Studies on eco-friendly concrete incorporating industrial waste as aggregates. Int. J. Sustain. built Environ. 4 (2), 378–390. doi:10.1016/j.ijsbe.2015.05.002
Pandey, C. L. (2015). Environmental security in the asia-pacific. New York: Palgrave Macmillan, 95–126.Climate change in South Asia: Green bridging between Nepal and India
Saravanan, S., Nagajothi, S., and Elavenil, S. (2019). Investigation OnCompressive strength development of geopolymer concrete using manufactured sand. Mater. Today Proc. 18, 114–124. doi:10.1016/j.matpr.2019.06.284
Sayne, A. (2011). Climate change adaptation and conflict in Nigeria. Washington, D.C., United States, US Institute of Peace.
Sergey, A. M., Anna Yu, Z., and Galina, S. S. (2015). Production technology of waterproof porous aggregates based on alkali silicate and non-bloating clay for concrete of general usage. Procedia Eng. 111, 540–544. doi:10.1016/j.proeng.2015.07.038
Shao, Q., Gu, W., Dai, Q., Makoto, S., and Liu, Y. (2014). Effectiveness of geotextile mulches for slope restoration in semi-arid northern China. Catena 116, 1–9. doi:10.1016/j.catena.2013.12.006
Shen, W., Liu, Y., Wu, M., Zhang, D., Du, X., Zhao, D., et al. (2020). Ecological carbonated steel slag pervious concrete prepared as a key material of sponge city. J. Clean. Prod. 256, 120244. doi:10.1016/j.jclepro.2020.120244
Shi, Y., and Liu, X. (2019). Research on the literature of green building based on the Web of science: A scientometricanalysis in CiteSpace (2002-2018). Sustainability 11 (13), 3716. doi:10.3390/su11133716
Sun, R., Wang, D., Cao, H., Wang, Y., Lu, Z., and Xia, J. (2021). Ecological pervious concrete in revetment and restoration of coastal wetlands: A review. Constr. Build. Mater. 303, 124590. doi:10.1016/j.conbuildmat.2021.124590
Tanaka, M. (1998). Reduction of environmental load by eco-concrete. Sound absorption for reducing noise burden; Kankyo fuka wo teigensuru eco-concrete. Kyuonsei ni yoru fuka teigen. Konkurito Kogaku Concr. J. 36.
Tang, Q., Chen, Y., Jia, R., Guo, W., Chen, W., Li, X., et al. (2022). Effect of clay type and content on the mechanical properties of clayey silt hydrate sediments. J. Petroleum Sci. Eng. 220, 111203. doi:10.1016/j.petrol.2022.111203
Tian, Z. W. (2012). The research on assessment of environmentaland economic performance for recycled concrete based on LCA-LCC. Hangzhou, Zhejiang, China, Hangzhou University.
Upadhya, M., and Kandel, B. R. (2015). Environmental challenges and governance. Milton Park, Abingdon-on-Thames, Oxfordshire, England, UK, Routledge, 99–115.Understanding the state of environmental governance in Nepal
Vieira, D. R., Calmon, J. L., and Coelho, F. Z. (2016). Life cycle assessment (LCA) applied to the manufacturing of common and ecological concrete: A review. Constr. Build. Mater. 124, 656–666. doi:10.1016/j.conbuildmat.2016.07.125
Walia, K., Aggarwal, R. K., and Bhardwaj, S. K. (2017). Environment impact assessment of highway expansion–a review. Curr. World Environ. 12, 507–519. doi:10.12944/cwe.12.3.04
Waltman, L., Eck, N. J. V., and Noyons, E. C. M. (2010). A unifified approach to mapping and clustering of bibliometric networks. J. Informetr. 4, 629–635.
Wang, J. Q., Wu, J. S., Huang, K. J., and Wang, J. L. (2022). Planting performance and durability of eco-concrete for slope protection. J. Civ. Environ. Eng. 48, 1–12.
Wang, J., and Wang, H. X. (2007). [Standardized therapy and progression for patients with small cell lung cancer]. J. Intell. 30 (4), 94–97.
Wang, Y. X., Arora, R., Choi, Y., Chung, H. W., Egorov, V. I., Frahm, J., et al. (2014). Implications of Web of Science journal impact factor for scientific output evaluation in 16 institutions and investigators’opinion. Quant. Imaging Med. Surg. 4 (6), 453–461. doi:10.3978/j.issn.2223-4292.2014.11.16
Wasim, M., Abadel, A., Bakar, B. H. A., and Alshaikh, I. M. (2022). Future directions for the application of zero carbon concrete in civil engineering-A review. Case Stud. Constr. Mater, e01318.
Wei, T., Zhang, L. J., and Zhang, H. J. (2005). Application of vegetation concrete technology for highway slope protection and ecological observation of slope protection effect. Highw. traffic Technol. (5), 123–129.
Wu, Y. M., Guo, K. X., and Jia, H. F. (2018). Application of ecological concrete in sponge city construction and its characteristic experiment design. Concrete (7), 122–125.
Wu, Z. R., Lu, C. H., Liu, R. G., Wu, C. D., and Peng, Z. H. (2005). Performance index and durability of eco-concrete cast on site for rivage-protecting and plant- growing. J. Jiangsu Univ. Sci. Ed. (05), 380–383.
Xiang, X., Liu, C., Yang, M., and Zhao, X. (2020). Confession or justification: The effects of environmental disclosure on corporate green innovation in China. Corp. Soc. Responsib. Environ. Manag. 27 (6), 2735–2750. doi:10.1002/csr.1998
Xie, F., Liu, F. P., Gong, A. M., Mei, W., Luo, C. C., and Yin, Q. (2021). Effect of porosity on compressive strength and planting performance of ecological concrete. Fly. Ash Compr. Util. 35 (01), 57–60+65.
Xie, H., Zhang, Y., Zeng, X., and He, Y. (2020). Sustainable land use and management research: A scientometric review. Landsc. Ecol. 35 (11), 2381–2411. doi:10.1007/s10980-020-01002-y
Xu, W. N., Wang, T. Q., and Ye, J. J. (2002). Study on application of revegetation technology for rock slope protection. Water Resour. Hydropower Eng. (07), 35–36+40.
Yi, F., Yang, P., and Sheng, H. (2016). Tracing the scientific outputs in the field of Ebola research based on publications in the Web of Science. BMC Res. Notes 9 (1), 221. doi:10.1186/s13104-016-2026-2
Yu, P. F. (2018). Study on the characteristics of backwashing soil of vegetation concrete river slope protection. Water Sci. Eng. Technol. (6), 43–46.
Yu, R., Spiesz, P., and Brouwers, H. J. H. (2015). Development of an eco-friendly Ultra-High Performance Concrete (UHPC) with efficient cement and mineral admixtures uses. Cem. Concr. Compos. 55, 383–394. doi:10.1016/j.cemconcomp.2014.09.024
Yu, S. P., and Yu, S. C. (2009). The influence of cement concrete on ecological environment and countermeasures were discussed. Inn. Mong. Sci. Technol. Econ. (4), 54–58.
Zeng, L., Liu, J., Gao, Q. F., and Gao, Q. F. (2019b). Evolution characteristics of the cracks in the completely disintegrated carbonaceous mudstone subjected to cyclic wetting and drying. Adv. Civ. Eng. 2019, 1–10. doi:10.1155/2019/1279695
Zeng, L., Xiao, L. Y., Zhang, J. H., and Gao, Q. F. (2019a). Effect of the characteristics of surface cracks on the transient saturated zones in colluvial soil slopes during rainfall. Bull. Eng. Geol. Environ. 79 (2), 699–709. doi:10.1007/s10064-019-01584-1
Zhang, H. M. (2018). Test and application of planting concrete in highway side slope protection. North. Commun. (1), 62–66.
Zhang, K., and Wen, Z. (2008). Review and challenges of policies of environmental protection and sustainable development in China. J. Environ. Manag. 88 (4), 1249–1261. doi:10.1016/j.jenvman.2007.06.019
Zhang, X. G., Deng, D. P., Yang, J. H., Wang, F., and Fan, Y. H. (2018). Study on the stress-strain curve of recycled lightweight aggregate concrete. Bull. Chin. Ceram. Soc. 37 (10), 3166–3172.
Zhao, Y., Ao, H., Chen, L., Sottas, C. M., Ge, R. S., Li, L., et al. (2012). Mono-(2-ethylhexyl) phthalate affects the steroidogenesis in rat Leydig cells through provoking ROS perturbation. Toxicol. Vitro 26 (6), 950–955. doi:10.1016/j.tiv.2012.04.003
Keywords: ecological concrete, VOSviewer, CiteSpace, visual analysis, sponge city building field
Citation: Wang L, Liu Z, Yan Y, Li S and Xie Y (2023) Overview of the application of ecological concrete in sponge city construction. Front. Earth Sci. 10:1085419. doi: 10.3389/feart.2022.1085419
Received: 31 October 2022; Accepted: 28 November 2022;
Published: 18 January 2023.
Edited by:
Zizheng Guo, Hebei University of Technology, ChinaReviewed by:
Jan Bondaruk, Central Mining Institute, PolandMohammad K. Najjar, Federal University of Rio de Janeiro, Brazil
Copyright © 2023 Wang, Liu, Yan, Li and Xie. This is an open-access article distributed under the terms of the Creative Commons Attribution License (CC BY). The use, distribution or reproduction in other forums is permitted, provided the original author(s) and the copyright owner(s) are credited and that the original publication in this journal is cited, in accordance with accepted academic practice. No use, distribution or reproduction is permitted which does not comply with these terms.
*Correspondence: Zhikui Liu, MTk5ODAwOUBnbHV0LmVkdS5jbg==