- Pontificia Universidad Católica de Chile, Facultad de Educación and Research Center for Integrated Disaster Risk Management, Santiago, Chile
Population growth and spread have increased human exposure to natural hazards and potential disasters affecting people’s quality of life. This situation is especially manifested in marginalized or vulnerable areas. Moreover, within such vulnerable areas, children are especially affected, and are, at the same time, considered to be agents of change. However, children’s voices have been scarcely considered for disaster risk reduction planning, and science education has not widely addressed these ideas. This study explores the understanding of earthquakes and tsunamis by children living in high-risk areas of Chile during a learning unit and according to their geographical zone. The study was part of a context-based science education learning unit. One hundred and two students from four schools used explanations to draw and write the causes of the risk situation, revealing their understanding of each phenomenon. The results show most students attributed earthquakes to the Tectonic Plates Theory while holding ancient scientific ideas about tsunami causes, for example, some explanations were based on air pressures. More sophisticated reasoning was found at the end of the learning unit, advancing their understanding of hazards promoted by the science education of the learning unit. The relevance of context-based science education for disaster risk reduction is discussed, especially for the cases of children with animistic - transferring human needs and attributes to non-animated entities- or fatalist understandings of the phenomena. The scientific understanding perhaps promotes empowerment and action-based choices for safety. Furthermore, the argument for policy curriculum design in primary science education for mitigating disasters is discussed.
Introduction
Population growth and spread has increased human exposure to natural hazards. Although there are new technologies to support the information about hazards, the impact of disasters continues to advance around the globe with accelerating consequences (Delicado et al., 2017). Disasters affect people’s quality of life, especially within marginalized or vulnerable groups, such as women or children (Ronan et al., 2015; García, 2016; Delicado et al., 2017). Indeed, children suffer the devastating consequences of disasters because of the loss of daily routines, delayed academic progress, missed social opportunities in a critical development period, and increased exposure to various life stressors due to their partial or total dependence on adults (Peek, 2008). The Sendai framework for disaster risk reduction focuses on all community participation for this objective, which led to a child-centered disaster risk reduction approach to promote their agency in building individual and community resilience (Wachtendorf et al., 2008; Aitsi-Selmi et al., 2015). There is a need to shift from disaster recovery to prevention, focusing on peoples’ education for the primary goal of disaster reduction; the development of safety culture (Izadkhah, 2005). Reducing the risk of hazardous events can be achieved by helping children and families to learn exposure and vulnerability-risk-reduction strategies (Ronan et al., 2015). Educational programs can decrease children’s vulnerability and promote personal, family, and community resilience (Finnis et al., 2004; Wachtendorf et al., 2008; Muttarak et al., 2013). Nonetheless, children’s voices have been scarcely considered for disaster risk reduction planning (Freeman et al., 2015; Vásquez et al., 2018). Usually, they have been portrayed as passive, helpless victims or vulnerable aid recipients (Tanner 2010). Still they can play an active role in recovery and rebuilding; thus, recognizing their strengths and listening to them is crucial (Freeman et al., 2015).
There is evidence that understanding the natural phenomena that might become a local disaster is crucial for disaster risk management, empowering communities, and educating people to prevent possibly resultant negative impacts (de Guzman, 2003; Wesely, 2021). Acquiring knowledge on natural phenomena and its application in emergencies is the most effective way for preventing disasters or mitigating their effects (Izadkhah, 2005; Torani et al., 2019).
Studying children’s understanding of phenomena that might lead to disasters is an increasing area of research interest (i.e., Aydin, 2011; Leather, 1987; Torani et al., 2019; Tsai, 2001). Although, review reports (Ronan et al., 2015; Torani et al., 2019; Cabello et al., 2021) have shown that few studies have successfully connected curriculum-based formal science education with disaster risk reduction regarding understanding of those phenomena (e.g., Cahapay and Ramirez, 2020; Shoji et al., 2020; Tyas & Pujianto, 2020). Science curriculums can be transformative tools for students to view themselves as active in their role within current socio-political structures (Guerrero & Torres-Olave, 2021). Educational disaster-reduction initiatives often have dual orientations; to motivate children and families to act to be prepared to face disasters or to teach students about the science of natural phenomena (Izadkhah, 2005). Our initiative studies the second of these choices, considering that promoting educational programs must support teachers in the program implementation, because by only creating resources for voluntary use without guidance and assessment it is unlikely to lead to high uptake levels (Ronan et al., 2015).
Children need to understand natural hazards first to be prepared to face risk events (Finnis et al., 2004); scientific literacy is requisite but not guaranteed for disaster preparedness (Cahapay and Ramirez, 2020). Their understanding and reasoning capacities need to be explored because the ideas about causes and consequences of disasters are linked to preventing consequences via action-based safety choices (McDonald et al., 2019). However, no study has explicitly focused on cognitive aspects such as science literacy to explain student’s disaster preparedness variability (Cahapay and Ramirez, 2020). The studies of curriculum materials on natural hazards have shown to be oriented to lower cognitive skills such as recall of knowledge, not on higher-order abilities (Cahapay and Ramirez, 2020), such as the act of phenomena explanation. It is a matter of interest because children’s understanding of the phenomena, its causes, and local consequences, can build intergenerational capabilities for risk management and personified vectors of hazard education to those around them (Finnis et al., 2004; Izadkhah, 2005; Muzenda-Mudavanhu, 2016; Torani et al., 2019).
Understanding and Knowledge Construction
Understanding the geological aspects of Earth science is a complex process because of the multi-dimensional and hierarchical nature of the subject (Blake, 2005). Contextual factors matter in the understanding of phenomena. For instance, Bakopoulou et al. (2021) assume that the density and distribution of geodynamic phenomena in the time and place where people live can determine the form of ideas they hold. Learning about theories collectively is one of the components of developing scientific reasoning, defined as intentional knowledge-seeking and coordination of theory and evidence (Kuhn, 2002).
Scientific reasoning is a cumulative and cyclical process (Zimmerman, 2007). Usually, children’s reasoning starts from intuitive knowledge construction to progressively create precursor models of why the phenomena occur. Precursor models are “cognitive schemata compatible with scientifically appropriate knowledge since they are constructed based on certain elements pertinent to scientific models, which have a limited range of application, and which prepare children’s thinking for the construction of scientifically appropriate models” (Ravanis et al., 2013 p. 2259). Students often use mental structures as intermediate stages in science education to understand and interpret a phenomenon, progressively showing their knowledge acquisition (Vosniadou & Brewer, 1992; McDonald et al., 2019). In this knowledge construction, children’s ideas operate as synthetic alternative inputs representing the integration of new information into their initial ideas, advancing into more complex explanations of concepts and models (Libarkin, 2003).
Children’s Ideas About Earthquakes and Tsunami
Children’s ideas about earthquakes have been explored in diverse geographies, although tsunami ideas are less researched so far. Regarding earthquakes, in the United States, the students know the phenomena before formal instruction, but most do not know its cause. Misconceptions were persistent even with formal science instruction. Particularly, weather conditions as causes of earthquakes or defining volcanoes and earthquakes as the same or similar phenomena revealed that classroom instruction did not guarantee understanding the topic (Ross and Shuell, 1993). Similarly, in France, Allain (1995) showed that students connect causally, and more often earthquakes to a volcanic activity, the internal structure of the planet, atmosphere, and less frequently to tectonic causes or faults; furthermore, causal connects were made with Earths’ rotational movement, human causes, and extra-Earth ideas such as meteorites. Singaporean students related earthquake phenomena mainly to magma activity (Wang et al., 2009), while in Puerto Rico, Mejías & Morcillo (2006) found that six percent of secondary students related earthquakes to Tectonic Plates Theory (TPT), with some confusion between the concepts of “layers” and “plates.” The complex interaction between personal beliefs, including religious, ancestral, or family beliefs; and scientific knowledge is generally known (Berkes, 2008). In Mexico, primary students hold causal ideas related to Aristotle’s ancient views as “Earth accommodation” or Neptunian as “products of volcano eruptions’ (Rodriguez-Pineda & Faustinos, 2017), which are considered nowadays for some authors as misconceptions (Francek, 2013) or alternative ideas (Licona et al., 2013; Rodriguez-Pineda & Faustinos, 2017).
Regarding tsunamis, Taylor and Peace (2015) found that Indonesian children’s religious beliefs shaped their worldviews and were used to explain causes of floods; God, to fate (as something that God would determine) or human recklessness, demonstrating a worldview in which the spiritual world does affect the physical world. Indeed, the participants viewed prayer as an action to prevent flooding and reduce its risk. Adyoso and Kanegae (2012) show that without curricular education, 40% of children attributed tsunami to Gods’ wrath, 36% to man-made activities, and 6% view it as a natural phenomenon, while with education, the percentages were 38% natural phenomenon, 31% God’s wrath and 22% man-made activities.
Concerning the change of ideas, students combine scientific and mythological ideas, using three cognitive strategies to solve perceived incongruencies; to accept the scientific theories and reject their world vision, to accept the scientific ideas but keep their world vision or keep their ideas and ignoring the scientific ones (Tsai, 2001). Likely then, by embracing indigenous explanations one might facilitate understanding of scientific models of the phenomena when complementarities are established, I.e., by respecting other ways of knowledge creation apart from science, such as oral or ancestral traditions. Indeed, there is no need to replace one system of beliefs, but rather to enrich each other. It also reinforces the right for indigenous people to explain using their own words (King & Goff, 2010).
Additionally, students’ attitudes towards learning science are positively associated with risk perception, perceived coping ability, knowledge about disaster mechanisms and response, and propensity to respond appropriately. Still, although years of schooling on disaster preparedness are positively associated with survival rate, Shoji et al. (2020) point out that the school curriculum determines disaster preparedness and response because it is more important what people learn and not how long they spend learning it. Thus, they suggest science education would reduce the mortality risk of children by influencing their knowledge, perception, and behaviors about disasters.
Regarding what is observed of children’s ideas, two alternative trends are seen: students lacking scientific knowledge or considering their ideas as sources for building models. For instance, a study conducted with 12–13-year-old Greek students showed more than half of them attribute earthquakes to two plates within or underneath Earth, and 24.2% added information about “collision.” The authors indicated their result depicts students’ insufficiency, ignorance, or unsuitable training to (a) correlate surface phenomena such as earthquakes to the inner Earth, (b) tackle with process orientated thinking, and (c) search for the cause of the phenomena (Bakopoulou et al., 2021). The imprecise ideas of the students can also be seen as a precursor model, based on some elements of a scientific model, but still holding room for improvement through learning (Ravanis et al., 2013). For instance, about the forementioned conclusion, Vergara-Díaz et al. (2021) found improved students’ ideas about Earth Sciences after model- and inquiry-based science instruction.
However, a difficulty arises with scientific education when many students use scientific terms such as magma, mantle, core, lithosphere, plates, or plate tectonics, which they cannot explain, meaning that incorporation of scientific terminology into an explanation does not necessarily imply understanding. Thus, although high school students are exposed to geoscience concepts and terminology, most of them demonstrate an incomplete understanding of the phenomena (Libarkin et al., 2005). Research in the effects of education for disaster risk reduction in children’s understanding of the phenomena lacks follow-up of adaptive coping following hazard events. It relies mainly on surveys, interviews, self-reports (Ronan et al., 2015).
To address and investigate further the problem described above, we have merged distinct learning products; we explored student’s understanding of tsunami and earthquakes, going beyond incorporating new vocabulary in order to interpret their comprehension of the phenomena through their expressed reasoning. Thus, the present study–through context-based science education - focuses on constructing precursor models using drawings and written explanations of earthquakes and tsunami in schools which are exposed to natural hazards. This approach helps students make sense of abstract phenomena through their conceptualization of causes and awareness of their consequences (Bennett et al., 2007), contributing to disaster risk reduction aided by science education. The research questions were: RQ1. Which are the initial explanations of 4th graders about earthquakes and tsunami? RQ2. Are there differences between the geographical zones? RQ3. To what extent do 4th graders’ scientific reasoning progress during a pedagogical unit? The findings of this study might be relevant to scholars, curriculum developers, researchers, and teachers.
Methods
This study considered the school’s exposure to natural hazards as a starting point to exploring fourth grader’s explanations about the causality and consequences of earthquakes and tsunami in two formats: drawn and written. The aim was to characterize children’s understanding of phenomena - earthquakes and tsunamis - that might become a disaster in their territories, their understanding of specific causes; then analyzing the scientific reasoning expressed in precursor models in context-based science education.
A participatory and pragmatic paradigm was followed (Yvonne Feilzer, 2010). The study involved schoolteachers in the decisions and aimed to help the educational school communities at risk to be aware of the hazardous situation in which they live. Moreover, the study pursued empowering children to be active in disaster risk reduction through raising their ideas about the phenomena and opening opportunities to express and further sophisticate their understanding.
The schools were selected because of their proximity to a disaster-risk zone, near the San Ramon’s active geographical fault in the capital of Chile, Santiago - which exposes the community to a disaster caused by earthquakes. Other schools were located near the tsunami flooding risk area in the Valparaíso coastal region of Chile.
The sampling was purposive (Patton, 2001), selected typical cases in the two extreme geographic conditions. The participants (n = 102), that completed at least one task, were aged between 8 and 9 years and had the permission of their parents and their consent to join the study. They belonged to four classes, two in the coastal zone and two in the capital. As the class sizes were not equivalent, we grouped them according to the zone criteria to run the quantitative analysis; coastal zone (n = 39), capital zone (n = 63). Moreover, as the students completed the learning tasks voluntarily, the numbers were not equivalent within the classes. Descriptive results were expressed in percentages to have a standardized approach. The Ethical Board at Pontificia Universidad Católica de Chile approved the research procedures.
The selected learning unit was part of the compulsory national curricula for fourth grade. The study was conducted between 2019 and 2021. Three classes participated at the end of 2019, while during 2020, the schools selected were closed because of the pandemic. The fourth class -needed to complete the sample-, was included in 2021 when the school reopened. The teachers taught “The internal dynamics of Earth” learning unit in 18 h of work distributed throughout 4 weeks approximately. The contents included by the mandatory curricula were; Earth structure models, Tectonic Plates Theory, dynamics of earthquakes, tsunami and volcano eruptions, security, and evacuation planning. The teachers received the pedagogical materials and support for designing context-based science education. This approach includes “using concepts and process skills in real-life contexts that are relevant to students from diverse backgrounds” (Koballa et al., 2005, p. 75). The teachers chose the lesson type and resources among; textbooks, manipulative material such as clay, Tectonic Plates puzzle, videos about the phenomena in their territories, simulations, augmented reality, posters, maps of hazards, and the school security plan to adapt or create. However, they had to authorize the researchers’ interviews leading to drawings and written worksheets of the project as learning samples, at the beginning and end of the learning unit to collect and compare the datasets, and allow at least one lesson be recorded.
A mixed-methods approach was used, combining qualitative and quantitative methodologies. The methods to generate the datasets were based on: (i) drawing representations, (ii) written worksheets, (iii) interviews, and (vi) lesson recording. This article reports findings from drawings and written explanations because they directly relate to the participant’s conceptual understanding of the phenomena. The specific prompts for eliciting drawn explanations were: “Represent or draw in this sheet your explanation about “Why does the ground move? What do you think this is due to? Why do big sea waves reach the town? What do you think this is due to?” (in Spanish: Representa o dibuja en esta hoja tu explicación sobre ¿por qué se mueve el suelo? ¿A qué crees tú que se debe esto? ¿Por qué hay grandes olas que llegan a la ciudad? ¿A qué crees tú que se debe esto?). Similarly, for eliciting written explanations, the prompts were “please explain: Why does the ground move? What do you think this is due to? Why do big sea waves reach the town? What do you think this is due to?” (in Spanish: Explica a continuación: ¿por qué se mueve el suelo? ¿Por qué hay grandes olas que llegan a la ciudad? ¿A qué crees tú que se debe esto?). None of the questions included the phenomena name “earthquakes” or “tsunami” because the first step of the learning progression assessment in explaining is the students’ identification of the phenomena by its name, according to Yao and Guo (2018). The initial measurements were applied during the first week of the learning unit. The final ones were when the teacher ended the unit, usually in the fourth week, following the regular science lesson plan. The drawings were coded using a rubric conceptually validated to analyze student’s understanding expressed in explanations based on a scientific theory. It has three advancing levels - and a level 0 that considered missing data- (Cabello et al., 2021). The rubric is described as follows:
- Level 1: Sensory level of understanding. The reasoning operates based on elements within the student’s perception of their senses. The phenomena are represented by their consequences, usually beneath the surface or the sea surface (tsunami case), not above.
- Level 2: Causal understanding includes drawing of elements beyond the sensory experience, usually connected with tectonic plates theory but not representing the dynamic mechanisms.
- Level 3: Causal understanding with dynamic mechanisms of tectonic plates theory. The drawing of representations includes non-visible theories or non-perceived elements to explain processes.
The written explanations were analyzed with Yao and Guo’s Scientific Explanation Progression Assessment (SEPA) (2018), with five inclusive stages described as:
- Stage 1: The student identifies the phenomenon, it has a single or a few variables, but their relationship is simple; the changing pattern conforms to everyday experience.
- Stage 2: The student additionally adds support for their explanation.
- Stage 3: The student additionally uses the critical variables as the clue for choosing scientific concepts, laws, theories, and or principles; makes a primary logical connection between idea, data, and phenomenon, through generalization, induction, or simple causal reasoning but with incorrect components.
- Stage 4: The student performs as in stage 3 but without incorrect or incomplete elements.
- Stage 5: The student additionally develops a causal chain or clarifies the mechanism that connects phenomenon, evidence, and theory through scientific reasoning, including isolation and control of variables, correlational reasoning, probabilistic reasoning, etc.
The written answers also were analyzed using qualitative content analysis to systematically categorize textual data (Miles & Huberman, 1994), using the causal categories by Mejías and Morcillo (2006).
- 1. Ancient science, such as Aristotle’s conceptualization of “Earth accommodation” or Seneca’s ideas about “trapped gases moving between internal Earth cavities,” which are present across cultures because of their sensory origin (Pozo & Gómez, 2001).
- 2. Tectonic plate theory’s current scientific knowledge is related to geological fault or tectonic plates.
- 3. Volcanic eruption as the cause of earthquakes and tsunamis.
- 4. Anthropogenic actions include earthquakes and tsunami caused by human deforestation, contamination, and extraction.
- 5. Religious beliefs, such as earthquakes or tsunamis as God’s punishment, faith or prayer can stop or prevent an earthquake.
- 6. Animistic; transfers human needs and attributes such as intentions, willingness, decision-making, emotions such as anger, revenge, etc., to Earth. “Animism” is an instinctive, primary mechanism of thinking development based on analogical transference (Piaget, 1933).
- 7. Astronomy, such as meteorites, Earth-Sun-Moon alignment.
- 8. Atmospheric or climatic factors, such as the change from a sunny day to a rainy.
Three research assistants coded the drawings and written answers independently from each other. They received training on the instruments by the main researcher and started applying to a small sub-sample data set. Several discussions followed, until reaching a consensus. Once the inter-rater reliability was over 90%, the team analyzed the rest of the material as blind peers. Thus, at least two raters analyzed each drawing and written explanation to enhance reliability. Weekly meetings allowed calibration of the group, and the four members solved the few divergences found. Thus, the final score was equal between two coders or determined in group agreement. The quantitative analysis explored the possible differences in children’s explanations and models at the learning unit’s beginning and end, and according to their geographical zone.
Results
RQ1. Which are the Initial Explanations of 4th Graders About Earthquakes and Tsunamis?
Written Explanations
In written explanations we used the categories by Mejías and Morcillo (2006). Table 1 shows examples of the answers categorized in each one, with a code formed by the ID number, the phenomenon (Earthquake: EQ, Tsunami: T) and the moment (Initial: I, Final: F).
Almost 80% of the children attributed earthquakes to current scientific ideas connected with tectonic plate theory (category 2), at the early moments of formal education, and there was almost 90% attribution when the learning unit was finished. Some examples are “Because the plates collide, it causes an earthquake, and Chile is also next to a plate. The plates collide and form a quake or earthquake” (341, EQ, I) and “The plates move, and the plate moves … causing damage” (318, EQ, F). In comparison, 8.4% of the participants related the causes with elements of ancient science which reduced to 1.3% at the end of the learning unit, for instance, “Because of air pressure, a gas comes out and causes the earthquake” (220, EQ, I). About 5% of the students transferred human intentions to the Earth as causes that persisted until the end of the learning unit, as shown in these excerpts “Because the tectonic plates are accommodating because they are uncomfortable” (307, EQ, F), or “Because there is an earthquake because something itches the world” (310, EQ, I).
The initial ideas about tsunamis were more diverse; 52.2% of the students connected tsunamis with elements of tectonic plate theory. For example, “Because there is a tsunami that moves the sea. It is due to the plates moving and making quakes in the sea and generating tsunamis” (208, T, I).
Almost 26% attributed tsunami to ancient science ideas, as shown in these quotations “Due to earthquakes vibrations of the Earth, air pressures accumulate completely” (220, T, I), and “Because the wind creates the waves and there was a lot of wind at the same time … a lot of waves came together” (217, T, I). Around 4% held animistic beliefs, and another 4% indicated causes related to astronomy, for instance, “Because when there is a full moon there are high waves … to the Earth’s rotation (203, T, I), and “Because of the Solar System” (516, T, I). Important changes were found at the end of the learning unit; almost 90% of the students included elements associated with tectonic plate theory, and the category “ancient science” and “animistic ideas” were absent, while nearly 5% kept the causal reasons connected with astronomical factors, such as “Because when there is full moon the high waves can make a tsunami, and people will have to evacuate. There is a lot of movement and high waves are made” (203, T, F).
Table 2 presents the categories found percentage wise in the written explanations for both phenomena.
Drawn Explanations
In this task we used a rubric to analyze student’s reasoning expressed in explanations (Cabello et al., 2021). The initial students’ understanding of why earthquakes and tsunamis occur was not mainly connected with a scientific cause. Some examples are presented in Figure 1, categories 0 and 1, which represent the Earth Planet, or consequences of the earthquake such as a broken building (i. e. 209, EQ; 213, EQ). However, almost 41% of them expressed a reason (levels 2 or 3) in earthquake drawings and 33% in the tsunami. These elements were usually represented beneath the ground or sea surface, with arrows, words, or phrases to identify tectonic plate theory elements (i. e., 284, EQ).
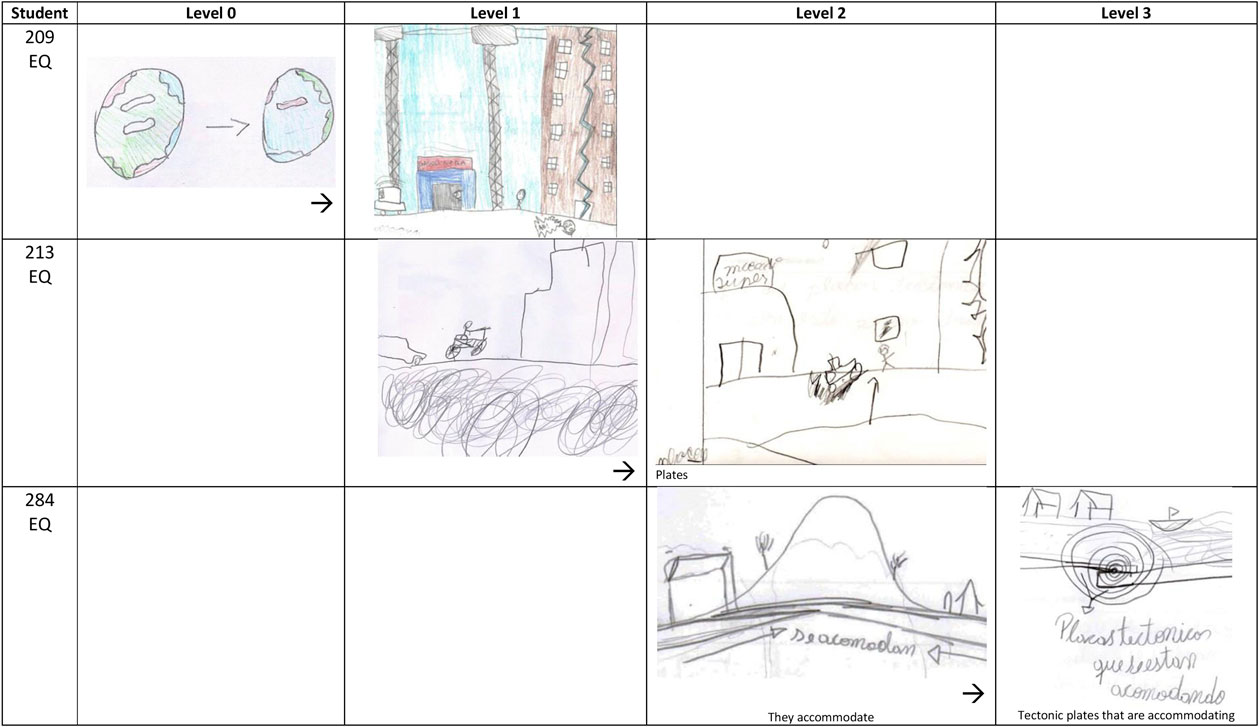
FIGURE 1. Examples of student’s drawing and levels, earthquake (EQ) task. The arrow points to the advance; initial moment → final moment.
After the instructional process, the percentages for levels 2–3 raised to 66% for the earthquakes and 64% for tsunamis. Most participants could represent scientific causal reasoning in the earthquake phenomenon, but an even higher increase was found in the tsunamis. The process of children’s reasoning expressed progression from the perceptible and imperceptible to more abstract ways of connecting tectonic plate theory and the phenomena under study. For instance, Figure 2 shows the advance from level 1 to level 2 (226, T) incorporating the representation of the Nazca and South American plates, and from level 2 to 3 replacing the cause related to “air accumulation” by the plates’ movement (220, T).
More sophisticated reasoning regarding the scientific theory underpinning earthquakes and tsunamis was found at the end of the learning unit. Most students connected the causes and consequences with the phenomena, advancing their understanding of hazards in the context of science education.
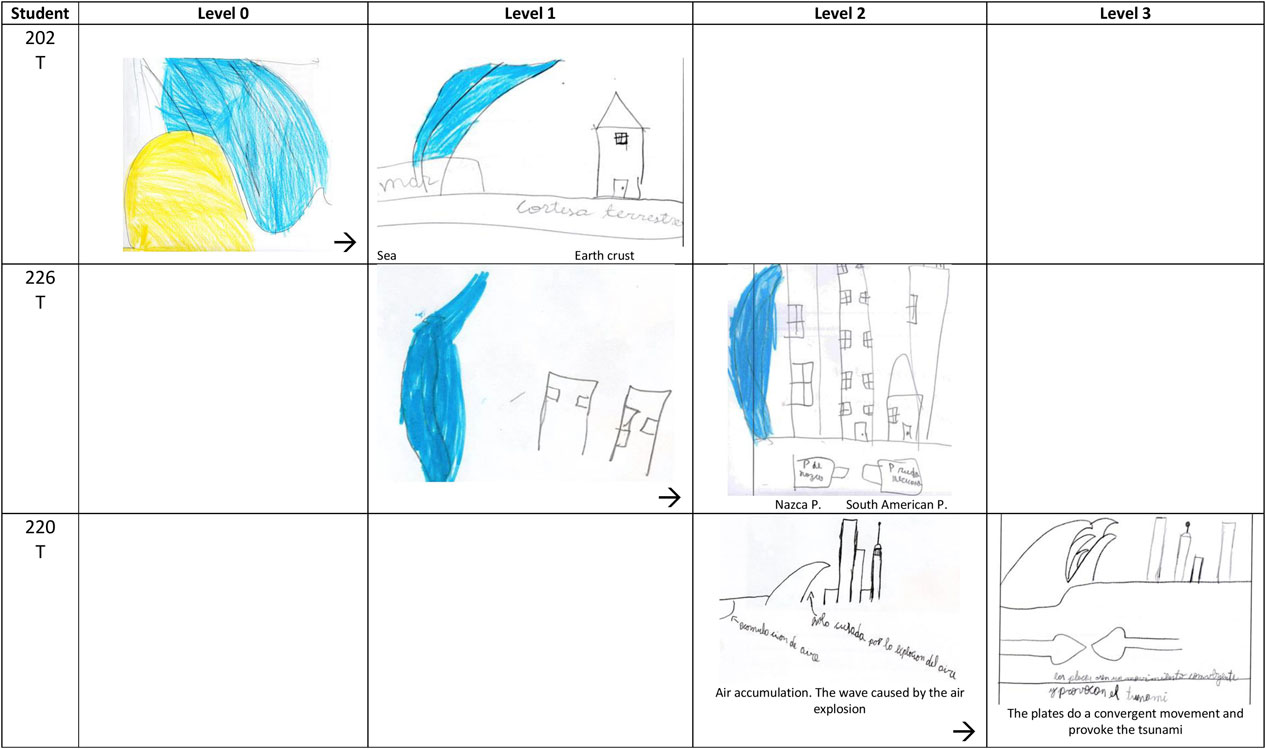
FIGURE 2. Examples of student’s drawing and levels, tsunami (T) task. The arrow points to the advance; initial moment → final moment.
RQ2. Are there Differences Between the Geographical Zones?
Intending to compare the initial explanations of 4th graders about earthquakes and tsunamis by their geographical zones, we used the Kruskal-Wallis test to assess differences in students’ initial scores (Wilcox, 2017). We have 66 students completing the drawing task on earthquakes (coastal = 24, capital = 42), and 86 students completing the writing task on earthquakes (coastal = 26, capital = 60). Regarding tsunamis tasks the sample sizes were not similar and were applied only by the teachers in the coast. Thus, we report scores comparison for the earthquakes drawing and writing tasks. Scores for drawing and writing tasks corresponded to ordinal responses and do not conform to a normal distribution. As such, we deemed it more appropriate to use a parametric test based on rank order to compare initial scores between students.
Among students who completed the drawing tasks (n = 66), we found no evidence of differences in their initial scores corresponding to geographical zones (H = 0.00 df = 1, p = 0.95, Kruskal–Wallis). In contrast, when we compared the students regarding their scores on the writing task (n = 86), we found higher scores among students from the capital zone exposed to the risk of disaster because of earthquakes than students in the coastal zone at-risk of a tsunami (H = 9.02, df = 1, p < 0.001, Kruskal–Wallis).
RQ3. To what extent do 4th Graders’ Scientific Reasoning Progress During a Pedagogical Unit?
The analysis of written explanations using Yao and Guo’s Scientific Explanation Progression Assessment (SEPA) (2018) showed that 49% of the participants gave answers in the levels 3–4 at the initial moment for earthquakes, while only 26% did it for the tsunami. After the learning module was finished, only 33.7% of the participants explained the levels 3–4 for earthquakes, lower than at the beginning. However, 50% of them attained levels 3–4 for the tsunami phenomenon after the learning module, representing an improvement.
Moreover, to assess the progression of the participant reasoning during a learning unit, we address the main effect between scores and explore if the geographical zone acts to condition these results. We use a mixed ordinal logit model to assess if student scores for the drawing and writing tasks improved after the learning unit. Because scores are ordinal responses, we opt for an ordinal logit model with a cumulative logit link. Indeed, student scores are sample dependents. Each student has a score before and after the learning unit; their scores are not independent observations. Therefore, we recur to a random intercept model to account for score dependencies. We include as “time” a dummy coded variable (0 = before the learning unit; 1 = after the learning unit), and include zone coded in similar fashion (0 = coastal, 1 = capital). Finally, we include an interaction term between these two factors. The present model specification is like an analysis of variance within and between-subject design, adapted for an ordinal dependent variable.
The estimates of these models are logits, or natural log-odds of the proportion of responses above a category and can be interpreted in a linear way (see Table 3). Thus, if the time factor results in a positive coefficient, it means students receive higher scores in their task after the learning unit. Similarly, if the zone factor presents positive coefficients, it implies students from the capital received higher scores overall. Finally, if the coefficient β3 is positive, it means students from the capital reached higher gains than those in the coastal zone. Conversely, if β3 is negative, then the students from the coastal area have higher gains than their capital peers. All estimates were produced using the R library “ordinal” (Christensen, 2019a; 2019b).
Student scores in the drawing task improved after the learning unit (β1 = 1.90, SE = 0.58, p < 0.01). Student odds of obtaining higher category scores are about six times larger after the learning unit than before (OR = 6.69). We see no substantial differences regarding zone nor for the interaction between factors.
Student scores in the writing task improved after the learning unit (β1 = 1.26, SE = 0.57, p < 0.05). Student odds of obtaining higher category scores are about three times larger after the learning unit than before the learning unit (OR = 3.53). In general, students from the capital obtained higher scores than their peers from the coastal area (β2 = 1.81, SE = 0.55, p < 0.001). The previous results are moderated. Additionally, we assessed if the scores were similar between groups with an interaction term. Students from the coastal zone presented higher gains after the learning unit compared to their peers from the capital (β3 = --2.23, SE = 0.70, p < 0.01).
Discussion
The current study explored children’s understanding of earthquakes and tsunami in risk areas of Chile in a context-based science education learning unit, using drawings and written explanations as a vehicle of children’s voice. The fourth graders represented the causes and the origin of the risk situation, expressing their ideas of the earthquakes and tsunami, which was relevant as a first step to advancing their understanding of hazards promoted by science education. Particularly, student scores in the drawing task improved after the learning unit, but we see no substantial differences regarding zone nor for the interaction between factors. Although student scores in the writing task also improved after the learning unit, the participants from the capital obtained higher scores than their peers from the coastal area. Students from the coastal zone showed higher gains in scores after the learning unit in comparison to their peers from the capital.
In this regard, it is known that for disaster risk management, promoting informed actions when events occur is as important as the prior understanding of the causes of the phenomena and the extent to which they can be related to human decisions. It is crucial to empower communities and educate people to prevent the negative impacts of hazards (de Guzman, 2003; Wesely, 2021). Indeed, we agree that the placing of primary education as a core element for raising hazard awareness in the public sphere is possible and urgent (Izadkhah, 2005).
In this study we found most of the participants attributed earthquakes to current scientific ideas connected with tectonic plate theory without formal education, but less than the half of them did it for tsunami. However, with the help of a context-based science education learning unit, the students obtained similar results for both phenomena. Mejías & Morcillo (2006) found that six percent of secondary students related earthquakes to Tectonic Plate Theory (TPT). However, in our study, almost 90% of the children reached this milestone after the learning unit for the earthquakes, which was already high when the learning unit started (80%). This finding might be associated with the context of risk on which the study was conducted, considering that contextual factors matter in understanding phenomena (Bakopoulou et al., 2021). Ancient scientific ideas in other studies were known as myths or religious beliefs (e.g., Tsai, 2001). Nonetheless, in this study, we named them as ancient science, recognizing the role of the history of science in the configuration of knowledge integration and construction of precursor models by children (Ravanis et al., 2013). Moreover, we assume these types of understanding relate to local stories, insights and are culturally relevant. Thus, they are valuable for knowledge integration, complementary towards disaster risk reduction, anchored in local wisdom and mutual enrichment (King & Goff, 2010).
Regarding the students’ reasoning, we found no evidence of differences in their initial drawings corresponding to geographical zones, however, we found higher scores among students from the capital zone exposed to the risk of disaster because of earthquakes than students in the coastal zone at-risk of a tsunami. Moreover, we observed a high percentage of children attributed tectonic plates theory as causes of earthquakes and tsunami in the drawing task after the learning unit. More sophisticated reasoning was found at the end of the learning unit. Most children connected the causes and consequences with the phenomena, significantly advancing their understanding of tsunamis as hazards, in context-based science education. The context-based science education for disaster risk reduction has extensive possibilities for research and curriculum design, especially in the cases where children have an animistic understanding of the phenomena. We concur with prior research showing these ideas persist and are difficult to change even with formal instruction (Ross and Shuell, 1993; Mejías & Morcillo, 2006). In the current study, attribution to human intentions to Earth persisted, for example, considering that the Earth is uncomfortable, and it moves with an earthquake. The students who attributed the causes of the phenomena as a punishment or unavoidable hazards might not be empowered to choose safe behavioral alternatives, which is critical for teachers, parents, and policymakers in child-oriented disaster management. Shoji et al. (2020) suggested that these views might impact the children’s perception of knowledge but not the perceived response to disasters, making this point exciting for further research.
Some limitations of the study included the different learning sampling sizes that reduced the obtainable quantitative analysis. Additionally, as the study has no control group, it cannot be inferred that the learning unit or the context of risk were causally associated with the results. Indeed, it might be other reasons associated to teaching factors, specific conditions in the classrooms, etc. Although some conditions in this study were quite similar, such as the school socio-economic level or class resources, it is known that the capacity to support innovation can make a difference in the curriculum implementation, especially in developing countries (Rogan & Gryson, 2003). Nonetheless, the descriptive findings of the present work are relevant for raising the explanations of school children about phenomena that might affect their lives, according to their zones, and illustrating the connection between formal science education and aids to risk management which might be accounted for in primary school curriculum design. Here, the mediating role of the curriculum-based material is crucial to targeting high-order thinking skills and not only the recall of knowledge (Cahapay and Ramirez, 2020). We need to consider that many disaster-mitigation and preparedness programs have put disaster topics in the school curriculum because children are supposed to be more receptive than adults (Izadkhah, 2005). However, if we consider children as vectors of hazard education (Finnis et al., 2004), the multiplicative effect of preschool and primary education interventions should be of interest to curriculum developers and policymakers. Indeed, science curriculum can be a cultural tool for student’ agency triggering, by giving students a protagonist role in their in-site knowledge construction (Guerrero & Torres-Olave, 2021).
Therefore, policy curriculum design in primary education for mitigating disasters is necessary, considering that learning about the phenomena includes a critical approach to socio-natural disasters and the ways to act with responsibility if they occur. It is particularly relevant in vulnerable zones, due to, for instance, unresponsive education to disasters might increase this vulnerability. We suggest that science educators offer learning opportunities connecting hazards as socio-scientific issues with aspects of understanding the causes and consequences of phenomena. Thus, formal education might enhance a child-centered disaster risk reduction approach (Aitsi-Selmi et al., 2015), promoting student’ agency in building personal and collective explanations that can be shared and be valuable for making decisions. Explanations are scientific practices and epistemic tools that support individual and perhaps community resilience. It could mean, for instance, integrating children’s ideas as a source of information to construct school evacuation plans/mitigation strategies (e.g., Vásquez et al., 2018) can transfer positive resultant effects from the school to the community (Wachtendorf et al., 2008), informed by a situated knowledge or a socio-cognitive approach as identified in the present study.
Data Availability Statement
The datasets presented in this article are not completely available because the qualitative information contain details that could potentially identify the participants. Requests to access should be directed to the corresponding author.
Ethics Statement
This study involved human participants. The procedures were reviewed and approved by the Comité ético científico de Ciencias Sociales, Artes y Humanidades, Pontificia Universidad Católica de Chile, number 180514006. Written informed consent to participate in this study was provided by the participants’ legal guardian/next of kin.
Author Contributions
The author confirms being the sole contributor of this work and has approved it for publication.
Funding
CONICYT/FONDECYT/11181050, currently Agencia Nacional de Investigación y Desarrollo ANID/FONDECYT/ 11181050. Research Center for Integrated Disaster Risk Management (CIGIDEN), ANID/FONDAP/15110017.
Conflict of Interest
The author declares that the research was conducted in the absence of any commercial or financial relationships that could be construed as a potential conflict of interest.
Publisher’s Note
All claims expressed in this article are solely those of the authors and do not necessarily represent those of their affiliated organizations, or those of the publisher, the editors and the reviewers. Any product that may be evaluated in this article, or claim that may be made by its manufacturer, is not guaranteed or endorsed by the publisher.
References
Adiyoso, W., and Kanegae, H. (2012). The Effect of Different Disaster Education Programs on Tsunami Preparedness Among Schoolchildren in Aceh, Indonesia. Disaster Mitigation Cult. Heritage Historic Cities 6 (1), 165–172.
Aitsi-Selmi, A., Egawa, S., Sasaki, H., Wannous, C., and Murray, V. (2015). The Sendai Framework for Disaster Risk Reduction: Renewing the Global Commitment to People's Resilience, Health, and Well-Being. Int. J. Disaster Risk Sci. 6 (2), 164–176. doi:10.1007/s13753-015-0050-9
Allain, J. C. (1995). Séismes, éruptions volcaniques et intérieur de la Terre: Conceptions d’elèves de huit à dix ans. Aster 20, 43–60. doi:10.4267/2042/8625
Aydin, F. (2011). Gifted Opinions about Earthquake: A Qualitative Study. Int. J. Phys. Sci. 6, 1863–1867.
Bakopoulou, A., Antonarakou, A., and Zambetakis-Lekkas, A. (2021). Existing and Emerging Students’ Alternative Ideas on Geodynamic Phenomena: Development, Controlling Factors, Characteristics. Educ. Sci. 11 (10), 646. doi:10.3390/educsci11100646
Bennett, J., Lubben, F., and Hogarth, S. (2007). Bringing Science to Life: a Synthesis of the Research Evidence on the Effects of Context-Based and STS Approaches to Science Teaching. Sci. Ed. 91 (3), 347–370. doi:10.1002/sce.20186
Blake, A. (2005). Do young Children's Ideas about the Earth's Structure and Processes Reveal Underlying Patterns of Descriptive and Causal Understanding in Earth Science? Res. Sci. Technol. Educ. 23 (1), 59–74. doi:10.1080/02635140500068450
Cabello, V. M., Véliz, K. D., Moncada-Arce, A. M., Irarrázaval García-Huidobro, M., and Juillerat, F. (2021). Disaster Risk Reduction Education: Tensions and Connections with Sustainable Development Goals. Sustainability 13 (19), 10933. doi:10.3390/su131910933
Cahapay, M., and Ramirez, R. P. (2020). Relationship Between Science Literacy and Disaster Preparedness: The Possible Role of Curriculum in Behavior Theories. Asian J. Sci. Educ. 2 (2), 78–86. doi:10.24815/ajse.v2i2.16803
Christensen, R. H. B. (2019a). Cumulative Link Models for Ordinal Regression with the R Package Ordinal. Available at: http://cran.unimuenster.de/web/packages/ordinal/vignettes/clm_article.pdf.
Christensen, R. H. B. (2019b). Ordinal—Regression Models for Ordinal Data. R package version 2019.12-10. 54. Available at: https://cran.r-project.org/package=ordinal.
de Guzman, E. M. (2003). Towards Total Disaster Risk Management Approach. United National Office for the Coordination of Humanitarian Affairs, Asian Disaster Response Unit. Available at: https://www.adrc.asia/publications/Asian_Conference_2003/E)TDRM%20February%202003.pdf.
Delicado, A., Rowland, J., Fonseca, S., de Almeida, A. N., Schmidt, L., and Ribeiro, A. S. (2017). Children in Disaster Risk Reduction in Portugal: Policies, Education, and (Non) Participation. Int. J. Disaster Risk Sci. 8 (3), 246–257. doi:10.1007/s13753-017-0138-5
Finnis, K., Standring, S., Johnston, D., and Ronan, K. (2004). Children's Understanding of Natural Hazards in Christchurch, New Zealand. Aust. J. Emerg. Manage. 19 (2), 11–20.
Francek, M. (2013). A Compilation and Review of over 500 Geoscience Misconceptions. Int. J. Sci. Educ. 35 (1), 31–64. doi:10.1080/09500693.2012.736644
Freeman, C., Nairn, K., and Gollop, M. (2015). Disaster Impact and Recovery: What Children and Young People Can Tell Us. Kōtuitui: New Zealand J. Soc. Sci. Online 10 (2), 103–115. doi:10.1080/1177083X.2015.1066400
Garcia, D. M., and Sheehan, M. C. (2016). Extreme Weather-Driven Disasters and Children's Health. Int. J. Health Serv. 46 (1), 79–105. doi:10.1177/0020731415625254
Guerrero, G. R., and Torres‐Olave, B. (2021). Scientific Literacy and agency within the Chilean Science Curriculum: A Critical Discourse Analysis. Curriculum J. 00, 1–17. doi:10.1002/curj.141
Izadkhah, Y. O., and Hosseini, M. (2005). Towards Resilient Communities in Developing Countries through Education of Children for Disaster Preparedness. Int. J. Emerg. Manage. 2 (3), 138–148. doi:10.1504/ijem.2005.007355
King, D. N., and Goff, J. R. (2010). Benefitting from Differences in Knowledge, Practice and Belief: Māori Oral Traditions and Natural Hazards Science. Nat. Hazards Earth Syst. Sci. 10 (9), 1927–1940. doi:10.5194/nhess-10-1927-2010
Koballa, T. R., Glynn, S. M., and Upson, L. (2005). Conceptions of Teaching Science Held by Novice Teachers in an Alternative Certification Program. J. Sci. Teach. Educ. 16 (4), 287–308. doi:10.1007/s10972-005-0192-5
Kuhn, D. (2002). “What Is Scientific Thinking and How Does it Develop?,” in Blackwell Handbook of Childhood Cognitive Development. Editor U. Goswami (Oxford: Blackwell Publishing), 371e393.
Leather, A. (1987). Views of the Origin and Nature of Earthquakes and Oil Held by Eleven-To Seventeen-Year-Olds. Geol. Teach. 12, 102–108.
Libarkin, J., Beilfuss, M., and Kurdziel, J. P. (2003). Mental Models and Cognition in Education. J. Geosci. Educ. 51, 121–126.
Libarkin, J. C., Anderson, S. W., Science, J. D., Beilfuss, M., Boone, W., and Kurdziel, J. (2005). Qualitative Analysis of College Students' Ideas about the Earth: Interviews and Open-Ended Questionnaires. J. Geosci. Educ. 53, 17–26. doi:10.5408/1089-9995-53.1.17
Licona, P., McDonald, S., and Furman, T. (2013). Student Conceptions of the Cause and Locations of Earthquakes. Earth Sci. XXIX, 50–54.
McDonald, S., Bateman, K., Gall, H., Tanis-Ozcelik, A., Webb, A., and Furman, T. (2019). Mapping the Increasing Sophistication of Students' Understandings of Plate Tectonics: A Learning Progressions Approach. J. Geosci. Educ. 67, 83–96. doi:10.1080/10899995.2018.1550972
Mejías, N. E., and Morcillo, J. G. (2006). “Concepciones sobre el origen de los terremotos: estudio de un grupo de alumnos de 14 años de Puerto Rico,” in Enseñanza de las Ciencias: Revista de investigación y experiencias didácticas, 125–138.
Miles, M. B., and Huberman, A. M. (1994). Qualitative Data Analysis: An Expanded Sourcebook. 2nd ed. Thousand Oaks, CA: Sage Publications.
Muttarak, R., and Pothisiri, W. (2013). The Role of Education on Disaster Preparedness: Case Study of 2012 Indian Ocean Earthquakes on Thailand’s Andaman Coast. Ecol. Soc. 18 (4), 451. doi:10.5751/es-06101-180451
Muzenda-Mudavanhu, C., Manyena, B., and Collins, A. E. (2016). Disaster Risk Reduction Knowledge Among Children in Muzarabani District, Zimbabwe. Nat. Hazards 84 (2), 911–931. doi:10.1007/s11069-016-2465-z
Patton, M. (2001). Qualitative Evaluation and Research Methods. 3rd ed. Newbury Park, CA: Sage Publications.
Peek, L. (2008). Children and Disasters: Understanding Vulnerability, Developing Capacities, and Promoting Resilience—An Introduction. Child. Youth Environments 18 (1), 1–29.
Ravanis, K., Christidou, V., and Hatzinikita, V. (2013). Enhancing Conceptual Change in Preschool Children's Representations of Light: A Sociocognitive Approach. Res. Sci. Educ. 43, 2257–2276. doi:10.1007/s11165-013-9356-z
Rodríguez-Pineda, A., and Faustinos, L. (2017). “Progresión de modelos sísmicos escolares: una estrategia didáctica para modelizar el origen de los terremotos en la educación primaria,” in Enseñanza de las Ciencias: Revista de Investigación y Experiencias Didácticas, Extra, 461–468.
Rogan, J. M., and Grayson, D. J. (2003). Towards a Theory of Curriculum Implementation with Particular Reference to Science Education in Developing Countries. Int. J. Sci. Educ. 25 (10), 1171–1204. doi:10.1080/09500690210145819
Ronan, K. R., Alisic, E., Towers, B., Johnson, V. A., and Johnston, D. M. (2015). Disaster Preparedness for Children and Families: a Critical Review. Curr. Psychiatry Rep. 17 (7), 58. doi:10.1007/s11920-015-0589-6
Ross, K. E. K., and Shuell, T. J. (1993). Children's Beliefs about Earthquakes. Sci. Ed. 77 (2), 191–205. doi:10.1002/sce.3730770207
Shoji, M., Takafuji, Y., and Harada, T. (2020). Behavioral Impact of Disaster Education: Evidence from a Dance-Based Program in Indonesia. Int. J. Disaster Risk reduction 45, 101489. doi:10.1016/j.ijdrr.2020.101489
Tanner, T. (2010). Shifting the Narrative: Child-Led Responses to Climate Change and Disasters in El Salvador and the Philippines. Child. Soc. 24 (4), 339–351. doi:10.1111/j.1099-0860.2010.00316.x
Taylor, H., and Peace, R. (2015). Children and Cultural Influences in a Natural Disaster: Flood Response in Surakarta, Indonesia. Int. J. Disaster Risk reduction 13, 76–84. doi:10.1016/j.ijdrr.2015.04.001
Torani, S., Majd, P. M., Maroufi, S. S., Dowlati, M., and Sheikhi, R. A. (2019). The Importance of Education on Disasters and Emergencies: A Review Article. J. Educ. Health Promot. 8, 85. doi:10.4103/jehp.jehp_262_18
Tsai, C.-C. (2001). Ideas about Earthquakes after Experiencing a Natural Disaster in Taiwan: An Analysis of Students' Worldviews. Int. J. Sci. Educ. 23 (10), 1007–1016. doi:10.1080/09500690010016085
Tyas, R. A., and Pujianto, S. (2020). Integrating Disaster Risk Reduction with Science Education to Student of Junior High School in Merapi Mountain Areas, Indonesia. Int. J. Eng. Res. Technol. 13 (12), 4551–4557.
Vásquez, A., Marinkovic, K., Bernales, M., León, J., González, J., and Castro, S. (2018). Children's Views on Evacuation Drills and School Preparedness: Mapping Experiences and Unfolding Perspectives. Int. J. Disaster Risk reduction 28, 165–175. doi:10.1016/j.ijdrr.2018.03.001
Vergara-Díaz, C., Bustamante, K., Pinto, L., and Cofré, H. (2020). Exploring Chilean Seventh Grade Students' Conceptions of Earth Dynamics before and after Model- and Inquiry-Based Instruction. J. Geosci. Educ. 68, 360–370. doi:10.1080/10899995.2020.1725406
Vosniadou, S., and Brewer, W. F. (1992). Mental Models of the Earth: A Study of Conceptual Change in Childhood. Cogn. Psychol. 24, 535–585. doi:10.1016/0010-0285(92)90018-w
Wachtendorf, T., Brown, B., and Nickle, M. C. (2008). Big Bird, Disaster Masters, and High School Students Taking Charge: The Social Capacities of Children in Disaster Education. Child. Youth Environments 18 (1), 456–469.
Wang, X., Kim, B., and Kim, M. S. (2009). “Idea of 'heat' and Understanding of Earth Phenomena,” in Proceedings of the International Science Education Conference, Singapore, 24–26 November 2009, 2208–2234.
Wesely, J. (2021). Towards Understanding Enabling Environments for Good Practices in Disaster Risk Management: An Analysis of Critical Junctures in Manizales. Colombia: Environment and Urbanization.
Wilcox, R. R. (2017). Understanding and Applying Basic Statistical Methods Using R. Hoboken, NJ, USA: John Wiley & Sons.
Yao, J.-X., and Guo, Y.-Y. (2018). Validity Evidence for a Learning Progression of Scientific Explanation. J. Res. Sci. Teach. 55 (2), 299–317. doi:10.1002/tea.21420
Yvonne Feilzer, M. (2010). Doing Mixed Methods Research Pragmatically: Implications for the Rediscovery of Pragmatism as a Research Paradigm. J. Mixed Methods Res. 4 (1), 6–16. doi:10.1177/1558689809349691
Keywords: explanation, earthquake, tsunami, science education, students-basic education, reasoning, children
Citation: Cabello VM (2022) Students Understanding of Earthquakes and Tsunamis in High Risk Areas. Front. Earth Sci. 10:841251. doi: 10.3389/feart.2022.841251
Received: 22 December 2021; Accepted: 08 February 2022;
Published: 25 April 2022.
Edited by:
Irasema Alcántara-Ayala, National Autonomous University of Mexico, MexicoReviewed by:
Glenn Fernandez, Sichuan University, ChinaBen Matthew Kennedy, University of Canterbury, New Zealand
Copyright © 2022 Cabello. This is an open-access article distributed under the terms of the Creative Commons Attribution License (CC BY). The use, distribution or reproduction in other forums is permitted, provided the original author(s) and the copyright owner(s) are credited and that the original publication in this journal is cited, in accordance with accepted academic practice. No use, distribution or reproduction is permitted which does not comply with these terms.
*Correspondence: Valeria M. Cabello, dm1jYWJlbGxvQHVjLmNs