- 1School of Civil Engineering, Northeast Forestry University, Harbin, China
- 2Institute of Cold Regions Science and Engineering, Northeast Forestry University, Harbin, China
- 3State Key Laboratory of Frozen Soils Engineering, Northwest Institute of Eco-Environment and Engineering, Chinese Academy of Sciences, Lanzhou, China
- 4School of Forestry, Northeast Forestry University, Harbin, China
- 5School of Water Resources and Environment, China University of Geosciences, Beijing, China
- 6Institute for Alpine Environment, Eurac Research, Bozen-Bolzano, Italy
- 7School of Civil Engineering, Lanzhou Jiaotong University, Lanzhou, China
Under a persistent warming climate and increasing human activities, permafrost in the Source Area of the Yellow River (SAYR) has been degrading regionally, resulting in many eco-environmental problems. This paper reviews the changes in air temperature and precipitation over the past 60 years and presents the distribution and degradation of alpine permafrost in the SAYR. The review is focused on the permafrost degradation–induced changes in hydrology, wetlands, thermokarst lakes, ponds, and vegetation. Mean annual air temperatures have been rising at an average rate of 0.4°C/10a over the past 60 years, while precipitation has increased only slightly (16 mm/10a). Borehole temperature monitoring at the depth of 15 m shows the permafrost warming rates of 0.01–0.21°C/10a in the Headwater Aera of the Yellow River. As a result of permafrost thaw, the amount of surface waters has declined while groundwater storage has increased. Due to permafrost degradation, the supra-permafrost water table lowers gradually, resulting in a reduction in areal extents of wetlands and lakes in the SAYR. We further renamed the concept of the burial depth of the ecologically-safe supra-permafrost water table, the minimum depth of the groundwater table for sustaining the normal growth of alpine grassland vegetation, for the SAYR to describe the relationship between the lowering permafrost table and succeeding alpine vegetation. Furthermore, we recommended more studies focusing on snow cover and carbon stock and emissions related to permafrost degradation under a warming climate. We also advised to timely establish the long-term monitoring networks for the rapidly changing mountain cryosphere, alpine ecology, alpine hydrology, eco-hydrology, cryo-hydrogeology, and carbon fluxes. Moreover, process-based models should be developed and improved to better simulate and predict the responses of alpine ecosystem changes to the interacting cryospheric and other environmental variables and their ecological and ecohydrological impacts in the SAYR and downstream Yellow River basins. This study can help better manage the ecological and hydrological environments in the Upper Yellow River that are sensitive to changes in the alpine climate and cryosphere.
Introduction
Observations from meteorological stations and results from simulations have demonstrated that the climate is warming and this warming will continue (Intergovernmental Panel of Climate Change (IPCC), 2014), with enlarged amplitudes at higher elevations (Pepin et al., 2015). The climate warming has produced changes to the cryosphere, including alpine permafrost (Haeberli, 2013; Zhao et al., 2020). The Qinghai-Tibet Plateau, with an average elevation of more than 4,000 m, has 70% of the world’s elevational permafrost, which is warm and sensitive to climate warming (Jin et al., 2000; Yang et al., 2010; Bockheim 2015). Permafrost thawing on the Qinghai-Tibet Plateau has produced changes to the alpine environment, including the dynamics of hydrological and ecological conditions and other physical, chemical, and biogeochemical processes (Yang et al., 2010; Latif et al., 2019). Understanding the ecological impacts of the climate-induced permafrost degradation is essential for predicting changes in ecosystem, as well as for the development and implementation of management action plans for alpine ecosystems (Wrona et al., 2016; Zhao et al., 2020).
The Source Area of the Yellow River (SAYR) above the Tanag (35°30′N, 100°09′E; 2,691 m a. s. l), with a catchment area of 123,690 km2 in Qinghai Province, China, is on the northeastern Qinghai-Tibet Plateau (Figure 1). As an important part of China’s Water Towers, the SAYR provides approximately 38% of the total annual runoff of the Yellow River (Wu et al., 2018). It is characterized by the world’s richest biodiversity of alpine grasslands and is one of the core areas of the recently established Sanjiangyuan (The Source Area of the Three Rivers: Yellow, Yangtze and Lancang-Mekong) National Park as well (He et al., 2021). However, the alpine ecosystem here is sensitive and fragile to external disturbances associated with climate changes and human activities (Wang et al., 2001; Yuan et al., 2021).
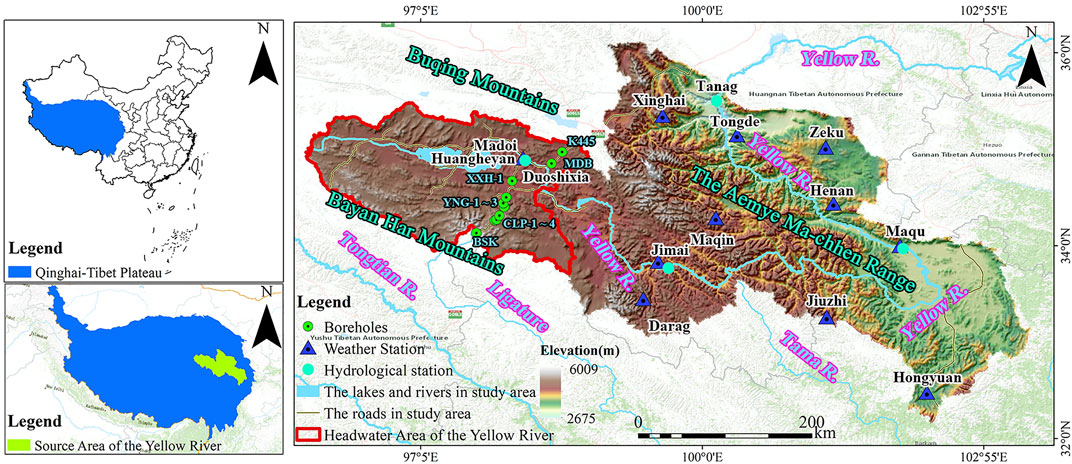
FIGURE 1. Location, mountains and water systems of the Source Area of the Yellow River on the northeastern Qinghai-Tibet Plateau in Southwest China.
Since the 1950s, the SAYR has undergone significant warming and this warming will continue in coupling with more and frequent extreme weather events (Wang et al., 2021). Permafrost in the SAYR is warm (≥−1°C) and discontinuous (<90% in areal extent of permafrost), and occupies an areal extent of 25,000 km2, or 85%, of the areal extent of the SAYR above the Tanag hydrological station, Qinghai Province, China (Li J. et al., 2016; Sheng et al., 2020; Cao H. et al., 2021). Since the 1990s, under the dual influence of a warming climate and anthropogenic activities, permafrost has been degrading, as typically evidenced by decreases in permafrost extent, increases in the active layer thickness (ALT), warming of the ground, and melting of ground ice (Jin et al., 2000; Jin et al., 2009; Luo et al., 2020a; Sheng et al., 2020). These changes have induced a series of ecological and environmental problems, such as the lowered water table, blackened soil spots, and reduced near-surface water resources (Jin et al., 2009). Consequently, the ecological successions from alpine paludal meadows to alpine steppes and deserts are always coupled with grassland degradation and desertification, shrinkage of wetlands and lakes, and drying up of some rivers (Wang et al., 2011; Yuan et al., 2021).
There is a growing recognition that environmental changes associated with permafrost degradation may have profound implications for alpine grasslands (Wang et al., 2001; Jin et al., 2009; Wang et al., 2019; Jin et al., 2020; Jin X.-Y. et al., 2021). With the accelerated degradation of alpine permafrost in the SAYR, its eco-environment degradation and the causation have been given much attention and have been lively discussed (e.g., Feng et al., 2006; Qin et al., 2017; Cao H. et al., 2021). However, responses and feedbacks of alpine ecosystems to a warming climate and subsequently degrading permafrost remain poorly understood in the SAYR. Systematic data are needed to understand the responses of hydrological conditions and alpine grasslands to climate change, permafrost degradation, and human intervention in order to better interpret and predict the feedback between climate warming and alpine ecosystems (Cao et al., 2003; Li et al., 2008; Jin et al., 2009; Jin et al., 2020; Jin X.-Y. et al., 2021).
The multi-year average of mean annual air temperature ranges from −3.5°C at Madoi meteorological station to 1.8°C at Maqu meteorological station, and it decreases with the rising elevation. In our study, meteorological data were obtained from the National Meteorological Information Centre (http://data.cma.cn/). Air temperature in the SAYR has been rising during the last 6 decades. During 1961–2020, the mean annual air temperature over the SAYR increased at a rate of 0.4°C/10a, and the increasing rate did not show a clear elevational trend (Table 1). In addition, mean annual maximum and minimum air temperatures rose in a fluctuating way at a rate of 0.3 and 0.4°C/10a, respectively (Liu et al., 2021). The rise in the mean annual minimum temperature was the most prominent in winter, resulting in a rising mean annual air temperature (Hu et al., 2012; Luo et al., 2016). The patterns of air temperature rise in the SAYR display strong spatial heterogeneity. The overall rising in air temperature was generally slower in the west than that in the east (Iqbal et al., 2018; Liu et al., 2021).
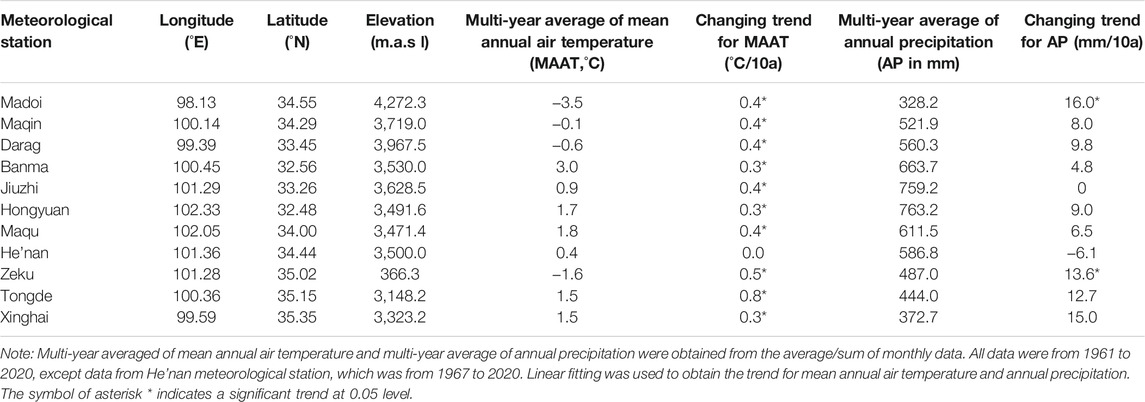
TABLE 1. Climate changes at the meteorological stations scale in the Source Area of the Yellow River on the northeastern Qinghai-Tibet Plateau in Southwest China from 1961 to 2020.
Unlike the rapidly-rising air temperature, regional average of annual precipitation in the SAYR was 554.4 mm (Table 1). Annual precipitation in the SAYR was increasing, but only that at Madio and Zeku slightly increased at a rate of 16 and 13.6 mm/10a, respectively. Seasonally, precipitation increased markedly in spring (47 mm/10a), summer (15 mm/10a), and winter (12 mm/10a), while it declined slightly in autumn (−0.5 mm/10a) (Li Q. et al., 2016; Liu et al., 2021). Spatially, precipitation increased significantly in the northwestern SAYR (Tian et al., 2015). In summary, the climate in the SAYR has exhibited a warming-wetting trend and the extreme weather events have become more frequent (Han et al., 2018; Jiang et al., 2019).
Our research groups have conducted many field surveys and investigations, mapping, and long-term observations of permafrost ecology and hydrology in the SAYR. Here, based on our findings and other research results, we have systemically reviewed the impacts of permafrost degradation on hydrology and plant ecology in the SAYR. We have summarized the changes in climate and permafrost, and we have focused on the alterations and interactions in hydrological regimes and vegetation succession. Finally, research priorities are identified. This study helps provide scientific support for the land, water, ecological management, and establishment of the Sanjiangyuan National Park.
Distribution and Degradation of Permafrost
The distribution of permafrost in the SAYR is mainly controlled by elevation, as well as local environmental variables, such as topography, surface vegetation, water bodies, soil texture, and snow-cover regime (Li J. et al., 2016; Luo et al., 2018). Simulations have shown that permafrost zones, seasonal frost zone, and other land types (rivers, reservoirs, and others) comprise approximately 85.1% (2.53 × 104 km2), 9.7% (0.3 × 104 km2), and 5.2% of the Headwater Area of the Yellow River (HAYR), and upstream of Duoshixia (Stony Gorge), respectively, (Li J. et al., 2016). The central HAYR around the Sisters’ Lakes (Gyaring and Ngöring lakes) (4,250–4,350 m. a. s. l) is found in the vicinity of the lower limit of alpine permafrost, and;alpine permafrost there is on the verge of thawing (Jin et al., 2009; Sheng et al., 2020). Above 4,250 m. a. s. l on north-facing slopes, permafrost generally develops better while there is generally no permafrost on south-facing slopes (Li J. et al., 2016). Permafrost begins to occur on all slopes above 4,350–4,500 m. a. s. l. (Jin et al., 2009). The lower limit of alpine permafrost on south-facing slopes is at least 100 m higher than that on north-facing slopes.
According to the data of ground temperatures measured in boreholes, the thickness of permafrost in the HAYR is generally less than 40 m, and the thickest measured permafrost is 74 m, as observed in the Chalaping boreholes near the National Highway G214 on the north-facing slope of the Bayan Har Mountains. Ground temperatures at the depth of zero annual amplitude are generally higher than −2°C, and the regional average of the ALT in the HAYR is 2.3 m (Luo et al., 2014; Luo et al., 2018; Wang et al., 2019). The total storage of ground ice is 51.68 ± 18.81 km3 at depths of 3.0–10.0 m in permafrost based on the data of landform types, lithology, and soil moisture content (Wang S. et al., 2018). Generally, on the Qinghai-Tibet Plateau ground ice is stored at depths close to the permafrost table and declines downwards with increasing depth.
Many studies have shown a degrading permafrost in the SAYR, which has been accelerating since the 1990s (e.g., Jin et al., 2000; Jin et al., 2009). The simulation results have shown a reduction in the areal extent of permafrost from 2.4 × 104 km2 in 1980 to 2.2 × 104 km2 in 2016 (Luo et al., 2014). Model reconstruction results have shown a decline in permafrost extent by 1,056 km2 from 1972 to 2012 and will decline by 2,224, 2,347, and 2,559 km2 (accounting for 7.5%, 7.9%, and 8.6% of the current total permafrost extent in the SAYR) under the climate warming scenarios of RCP2.6, RCP6.0, and RCP8.5, respectively, by 2050 (Sheng et al., 2020).
Ground temperatures, including mean ground surface temperature and mean annual ground temperature (MAGT), are rising and the active layer is thickening in the SAYR (Luo et al., 2018; Qin et al., 2020). According to the data measured at meteorological stations and observational sites from 2010 to 2016, the regional average of mean annual ground surface temperature in the SAYR increased by 1.1°C/10a, and that of MAGT by 0.01–0.21°C/10a (Table 2) (Luo et al., 2018; Luo et al., 2020b). Temperature of warm permafrost (MAGT≥ −1°C) increased significantly, accompanied by the recent disappearance of permafrost with the temperature of 0.5°C, while the warming trends were relatively high for permafrost located in the open terrains with dense vegetation (Luo et al., 2018). In addition, model simulation results of the temperature at the top of permafrost (TTOP) based on the Stefan equation showed that the ALT in the HAYR increased at an average rate of 34.7 cm/10a from 1980 to 2018, while the maximum depth of seasonal frost penetration decreased at an average rate of 9.3 cm/10a (Wang R. et al., 2020). More recently, the maximum seasonal frost depth decreased at a rate of 3.1–7.0 cm/10a from 2003 to 2015 (Gao B. et al., 2020) and the most significant declines occurred in the transitional zone between permafrost and seasonal frost (Wang et al., 2019).
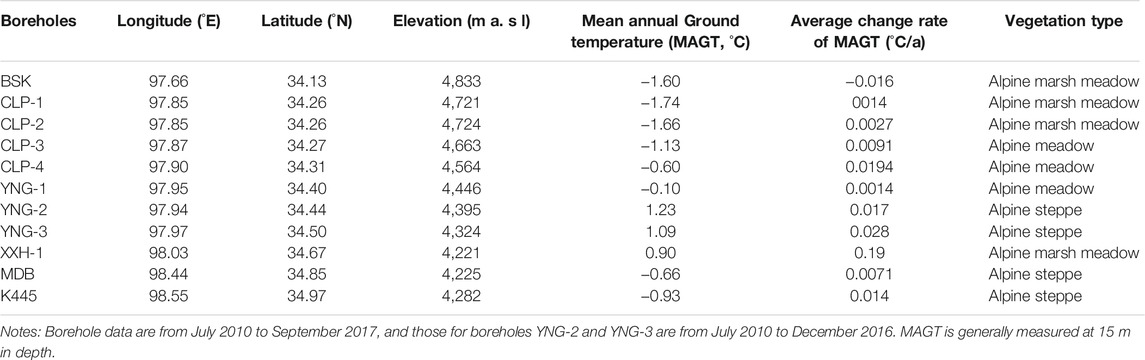
TABLE 2. Frozen ground temperatures in boreholes along the National Highway G214 in the Headwater Area of the Yellow River on the northeastern Qinghai-Tibet Plateau in Southwest China during 2010–2016 (modified from Luo et al., 2018).
Ecological Impacts of Permafrost Degradation
Hydrological Regimes
As an aquitard, permafrost weakens the downward infiltration from precipitation, glacier, and snow-cover melt-water and raises the supra-permafrost water table, influencing the streamflow and soil water content in the active layer. Surface waters and near-surface soil water availability are important for the growth and survival of alpine vegetation (Li et al., 2020a; Cao W. et al., 2021). Meanwhile, the melting of ground ice could release more water to soil, impacting the groundwater and the water cycle at a local scale, and even at a regional and global scale (Walvoord and Kurylyk, 2016; Schuur and Mack, 2018; Yang et al., 2019). Permafrost degradation has significant implications on alpine ecosystems through alterations in soil hydrological regimes (Wrona et al., 2016; Zhao et al., 2020). Coupled with lowering permafrost table and thickening active layer, regional permafrost degradation may result in lowering supra-permafrost water table and declining water-holding capacity of near-surface soils in the aeration zone (Jin X.-Y. et al., 2021; Jin H.-J. et al., 2021; Lv et al., 2022). As a result, the capillary water cannot move upward to the shallower soil layer where the roots of herbaceous and aquatic plants concentrate. Thus, near-surface soil could salinize by the enhanced evaporation, thereby restricting the growth of most vegetation (Wang et al., 2006; Qin et al., 2017; Sun et al., 2020).
Water resources in the SAYR are mainly recharged by precipitation, snow- and ice-melt, and groundwater of various types. Under a warming climate with permafrost degradation, mean annual evaporation increased and surface runoff have decreased (Lan et al., 2013; Wu et al., 2018; Yan et al., 2020). The mean annual runoff volume was approximately 2.02 × 1010 m3 and decreased by 5.5 × 109 m3 from 1960 to 2007 as recorded at the Tanag and/or Jimai hydrological stations, mainly due to the reduced runoff from mid-June to late October, i.e., the growth season (Wang T. et al., 2018; Wu et al., 2018). However, for the entire SAYR, the annual runoff exhibited a decreasing trend (6.51 mm/10a), while the annual precipitation increased (6.48 mm/10a), suggesting that other factors may influence the change trend of runoff, such as the potential evapotranspiration and more importantly permafrost degradation (Wang T. et al., 2018). The analysis of stable isotopes (δ18O and δD) in a representative watershed of the HAYR show a contribution ratio of precipitation of the surface runoff at 52.2–53.5% and that of ground ice melting at 13.2–16.7% (Ma et al., 2019; Yang et al., 2019). Water from melted ground ice directly contributes approximately 4.8% to the annual runoff of the Yellow River in of the SAYR, whereas the recharge from melted ground ice cannot completely offset the increase in land surface evapotranspiration under a persistently warming climate, hence resulting in a decrease in surface runoff, but otherwise an increase in groundwater storage (11.4 ± 13.9 × 108 m3/a from 2003 to 2009) (Xiang et al., 2016; Lin et al., 2019). However, little is known about the moving trajectories of water from ice melting, total volume water from the melting ground ice, and the magnitudes of melt-water of this ground ice to contribute to surface and subsurface water.
According to the observations in the past 30 years, the local groundwater table in the Yellow River valley plain near the Madoi county town continuously lowered by 0.8–1.5 m, with a maximum lowering decreased depth of 1.8 m, with an average annual lowering rate of approximately 0.5 m/10a (Cheng and Jin, 2013). In the meantime, this lowering groundwater table may also be associated with good soil drainage due to a high percentage of sandy and gravely constituents of soil texture (Gao et al., 2018; Lv et al., 2022). According to the model results of the percentage reduction of the single-spring runoff modulus, the recharge amount of the supra-permafrost water in the SAYR decreased by 15 × 108 m3 during the 14 years from 1989 to 2003, with an average annual decrease rate of 1.1 × 107 m3/a (Jin et al., 2009; Cheng and Jin, 2013). Together with a slight wetting and an enhanced evapotranspiration, the sharp decrease in recharge to the supra-permafrost layer resulted in a lower level of the supra-permafrost water table, thus causing water tables in the HAYR to continuously decline (Wang T. et al., 2018; Wu et al., 2018).
Although the melting of ground ice may replenish water resources and increase the total groundwater storage—though probably at a very limited magnitude or rate— warming climate, increasing evaporation, and changing land surface processes have reduced runoff generation, resulting in a lower groundwater table (Wu et al., 2018; Lin et al., 2019; Sun et al., 2020). The main source of soil water is precipitation and with the continuous temperature rise, the evaporation of soil water increases, while the annual precipitation has been fluctuating with a minor increase trend of 16 mm/10a (Qin et al., 2017; Xie et al., 2021). As a result, near-surface soil moisture, the key to maintaining the long-term growth of herbaceous plants, is depleting continuously and is on the decline. As a result, the regional water table lowers as well. In arid regions on the Qinghai-Tibet Plateau, this kind of groundwater table for sustaining the long-term growth of herbaceous plants is called the “the burial-depth of ecologically safe groundwater table” (Liang et al., 2007; Wang et al., 2012). In permafrost regions, we can thus rename it as the “burial-depth of ecologically safe supra-permafrost table” (BESST).
Field surveys have found that the deepening permafrost table leads to a lowering BESST (Wang et al., 2006; Yang et al., 2013). When the groundwater table is less than 2 m, soil water content may suffice plant growth and accordingly maintain high vegetation cover (Wang et al., 2012; Jin et al., 2020). When the burial depth of groundwater table is lower than 2 m, most of alpine plants with a shallow root system suffer the drought stress, and only a few types of drought-tolerant plants with well-developed root systems may access the limited available soil water for survival (Jin et al., 2020). Therefore, the vegetation coverage decreases. When the burial depth of the groundwater table is greater than 5 m, herbaceous plants often wither and eventually die from drought and only shrubs with deep roots can be occasionally observed (Wang et al., 2006). This indicates that changes or variations in the permafrost table control the BESST and the unfrozen water content of near-surface soils, thereby affecting the growth of plants (Wang et al., 2012). In cold and arid regions, a burial depth of 2 m of the groundwater table can thus be regarded as the threshold of the ecologically safe groundwater table (Liang et al., 2007; Wang et al., 2012), or the BESST. At a BESST deeper than 2 m and with slow replenishment by surface water infiltration, the capillary water cannot rise to the shallow soil layer, where the roots of most plants are concentratively distributed, so most plants are subjected to long-term drought stress from the growth environment, leading adverse successions and perpetual degradation of alpine grasslands (Liang et al., 2007; Li et al., 2020a; Li et al., 2020b; Jin X.-Y. et al., 2021, Jin H.-J. et al., 2021). However, the mechanisms for this depth control of the alpine vegetation growth remains poorly understood.
Shrinking of Wetlands and Lakes
As an aquitard, permafrost help enrich soil water above the bottom of the active layer or above the permafrost table in the presence of the supra-permafrost subaerial talik, which increases evaporation and runoff, but reduces downward infiltration, thus favoring the formation of thermokarst lakes and ponds, shallow rivers, and wetlands in lowlands (Walvoord and Kurylyk, 2016; Schuur and Mack, 2018). There are numerous rivers and lakes in the HAYR and wetlands are widely distributed. There are more than 5,300 lakes in the SAYR with a total area of more than 1,270.77 km2 (Luo et al., 2020c). These lakes, which are characterized as small and densely distributed, are close to the main streams along the Yellow River, and low-lying flat marshes. According to the remote sensing data, the area and number of lakes greater than 0.01 km2 both experienced four stages from 1986 to 2019: reduction (1986–2004), increase (2004–2012), reduction (2012–2017), and increase again (2017–2019) (Luo et al., 2020c). The number of lakes greater than six hectares (>0.06 km2) decreased from 405 to 261 in Madoi County from 1990 to 2000 (Dong et al., 2009). Small lakes were extremely unstable because of their small area, shallow water depths, and high susceptibility to disturbances (Watts et al., 2014; Luo et al., 2020c; Șerban et al., 2020; Șerban et al., 2021). Since the 21st century, especially after 2004, the area and number of lakes have begun to increase, possibly due to the increased water resources replenished by ground ice meltwater (Li et al., 2008). To date, there is an urgent need to develop and improve the methods for mapping the distribution and changes of medium and small water bodies using the remote sensing in permafrost regions. Furthermore, we also need to have in-depth understanding of influences of permafrost degradation on water-heat dynamics of thermokarst lakes (Șerban et al., 2020; Șerban et al., 2021).
The main types of alpine wetlands are lacustrine wetlands, alpine peat bogs, and marshlands. The areal extent of wetlands is about 2,126.33 km2, accounting for approximately 10% of the total catchment area of the HAYR (Niu, 2020). The lacustrine wetlands in this region, as represented by those in the peripheries of the Gyaring and Ngöring lakes, are mainly distributed in lowlands, while the marshes are mostly close to rivers and lakes and are on the low-lying shadowy slopes (Li et al., 2016c; Luo et al., 2020a). Under the influence of climate warming, strong evaporation, and permafrost degradation, wetlands are shrinking (Gao et al., 2013; Dong et al., 2009). From 1990 to 2004, wetlands were continuously shrinking at a rate of 2,864.48 km2/a, resulting in a total areal reduction of 40,102.65 km2 during this 14-year period, including a decrease of 23,298.09 km2 in the first 10 years and a decrease of 16,804.56 km2 years in the last 4 years (Li et al., 2009). After 2004, the wetland shrinkage and degradation began to slow down (Li et al., 2009). The area of wetlands near Madoi town decreased by 570 km2 from 1990 to 2013, including a decrease of 1,481 km2 from 1990 to 2001, and an increase of 911 km2 from 2002 to 2013 (Li et al., 2016c). At present, these wetlands are very unstable, with a high rate of conversion from wetlands to other land types (Dong et al., 2009; Wang J. et al., 2020).
Under a warming climate, annual precipitation in the SAYR has fluctuated and increased slightly. Rising temperature has led to an increase in the ALT and land surface evaporation. Every 1 cm increase in the ALT may result in a 1.3 mm decrease in annual runoff and a 0.9 mm increase in annual evapotranspiration in the SAYR (Wang Y. et al., 2018). In addition, the thawing of permafrost resulted in an increase in the downward permeability of soil water, further reducing the surface runoff (Wang Y. et al., 2018; Wu et al., 2018). Under a warming climate, precipitation in the SAYR has fluctuated and increased only slightly. If this situation continues, the water balance in the SAYR will negatively feed back to the warming climate, eventually leading to the shrinkage and degradation of marshes.
Changes in precipitation, runoff, and permafrost degradation are the main influencing factors for wetland hydrothermal dynamics in the SAYR (Wang et al., 2001; Wu et al., 2018; Wu et al., 2021). Persistent climate warming has enhanced land surface evaporation, lowered the burial depth of the permafrost table, and has deepened the active layer and the aeration/vadose zone, resulting in a deeper groundwater table, causing the continuously distributed wetlands to become fragmented or even completely disappear (drained) (Li et al., 2016c; Li G. et al., 2020c). With shrinking wetlands, alpine vegetation coverage declined, in turn, resulting in the bare patches of ground surface (blackened soil spots) in grasslands (Yi et al., 2011). In addition, the increased rodent activity and wind erosion reduced the area of marsh meadows and further increased the area of bare ground patches, leading to the degradation of moist and paludal alpine meadows dominated by Artemisia wellbyi into alpine steppes dominated by constructive species Artemisia absinthium and Stipa capillata. Furthermore, the water conservation capacity may have declined considerably (Wang et al., 2001; Feng et al., 2006; Qin et al., 2017). These hydrothermal processes are interactive, interdependent, and produce extensive and profound eco-hydrological impacts.
There is a recognition that permafrost degradation (melt of ground ice or presence of taliks) may have important implications for changes in wetlands and lakes (Jin et al., 2009; Wang Y. et al., 2018; Shi et al., 2020; Cao W. et al., 2021). These changes, in turn, are accelerating the permafrost thaw by transferring more thermal energy via heat convection and advection (Sjöberg et al., 2016; Luo et al., 2018). An electrical resistivity tomography study shows that sbu- permafrost groundwater moves towards to talik zones and this brings the heat to the surrounding permafrost as well, and hence, a further thaw of permafrost (Gao et al., 2019).
Impacts of Permafrost Degradation on Alpine Vegetation Succession
The plant ecosystem in the SAYR is fragile due to its simple structure. Main vegetation types here include alpine paludal meadows, alpine meadows, and alpine steppes. Borehole temperatures and other data have revealed a good correlation between vegetation distribution and permafrost development (Figure 2). Positions of these boreholes are shown in Figure 2A and their corresponding ground temperature curves are shown in Figure 2B. Borehole A is located at the edge of a degrading alpine meadow with positive temperature at depths of 0–12 m. The zero curtain phenomenon has been observed at depths of 12–24 m. Stable positive temperatures are recorded at depths greater than 24 m. Thus, this borehole site is underlain by degrading permafrost on the margin of thawing. Borehole B is located in a paludal meadow underlain by 18-m-thick, very warm (−0.5°C) permafrost. Borehole C is set in an alpine desert steppe and ground temperature here is always positive, indicating a site of talik.
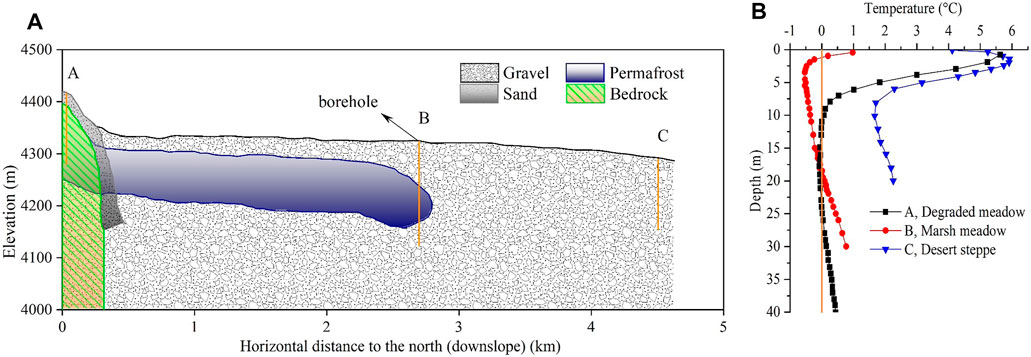
FIGURE 2. Borehole locations and vegetation types (A) and the corresponding soil temperature (B). Ground temperatures in Panel 2B were measured on 30 September 2016.
In the SAYR, as illustrated in Figure 2, paludal and alpine meadows are generally areas where permafrost develops and desert steppes are generally underlain by seasonal frost or talik. With permafrost degradation, the zone of alpine meadows retreated while alpine desert steppes expanded into the former ground of alpine meadows. Additionally, alpine desert steppes are dry and covered by a thin layer of steppe soil (crust), under which, are glaciofluvial sand, pebbles, and gravel. In the severely cold, dry, and windy environment, the sparse and cushion-like vegetation is often disrupted, so land desertification processes are prone to occur, resulting in land desertification as evidenced by mobilizing sand dunes or drifting sand (Hu et al., 2013; Li et al., 2016d).
Climate warming and permafrost degradation have changed the distributive features of surface waters and deepened and elongated the flow paths of groundwater, resulting in a series of vegetation successions from alpine marsh meadows to alpine meadows, and further to alpine steppes, resulting in the blackened soil spots, or even alpine deserts (Figure 3) (Mu et al., 2018; Jin et al., 2020; Jin X.-Y. et al., 2021). Permafrost, as an aquitard, blocks the downward movement of water from infiltration and stores water at the bottom of the active layer, generally at depths of about 1–2 m, which could be utilized by plants (Gao Z. et al., 2020). With a thickening of the active layer and the deepening of the permafrost table, water moves further downward, and the surface layer of soil becomes drier (Wang et al., 2006; Jin et al., 2020). This may accelerate the grassland degradation, especially in low-lying valley zones underlain by the isolated patches of alpine permafrost, where the soil water infiltration is dominated by lateral flow and downward movements (Sun et al., 2019; Jin et al., 2020; Cao H. et al., 2021). These land surface changes, furthermore, have resulted in the reduction of vegetation coverage.
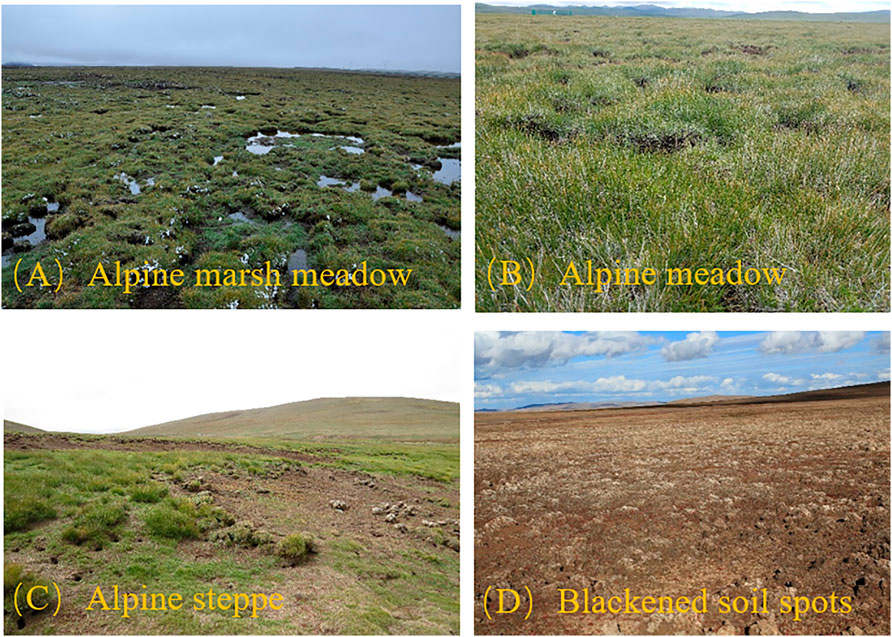
FIGURE 3. Degradation of alpine meadows into blackened soil spots in the Headwater Area of Yellow River on the northeastern Qinghai-Tibet Plateau, Southwest China. (A) Alpine marsh meadow with high sedge cover and saturated soil, (B) alpine meadow with high sedge cover but less soil water content, (C) Alpine steppe with a lower sedge cover and weeds, and (D) blackened soil spots without sedge and with dry soil.
As the active layer deepens progressively, the plant species richness, vegetation coverage, and underground biomass in alpine paludal meadows and the coverage of Cyperaceae decreases, but the coverage of forbs species increases (Jin et al., 2020). With further degradation of alpine steppes, the similarity index among communities decreases, and aboveground biomass significantly decreases in grasses, while it first increases and then decreases in forbs (Wang et al., 2012). In addition to ALT, an acceleration of ground freeze–thaw cycles (at 5 cm in depth) under the changing climate, could reduce the activity of root cells of plants and even leads to the death of plant root and rhizospheric microorganisms, thereby lowering the nitrogen fixation ability (Man et al., 2019). Moreover, ground thaw at a depth of 5 cm has advanced at rates of 0.1–1.5 days/year (2003–2015), and the maximum depth of frost penetration has decreased at rates of 0.7–3.1 cm/year, resulting in an earlier start date of the vegetation growing season (Gao B. et al., 2020).
All these aspects have led to the stresses of alpine vegetation from physiological droughts, accelerating grassland degradation. If effective measures are not taken in time to mitigate further grassland degradation, the imbalance between the permafrost environment and alpine vegetation in this region will result in positive feedback between permafrost degradation and grassland degradation (Figure 4). The consequence will be the extensive, persistent, and intensifying deterioration of the ecological environment, such as the degradation of grasslands and land desertification. These changes will lead to an increase in surface albedo, a decrease in the convection effect of the near-surface air flows, and an increase in near-surface ground temperatures. In addition, the patterns of atmospheric circulations over the Qinghai-Tibet Plateau will change, resulting in extensive environmental impacts.
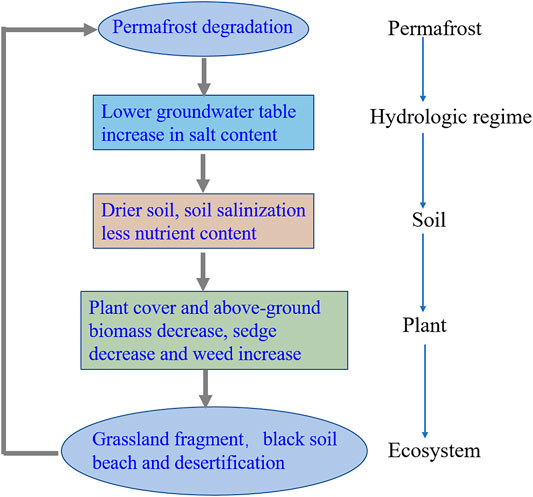
FIGURE 4. Interactions between permafrost degradation and vegetation degradation in the Headwater Area of Yellow River on northeastern Qinghai-Tibet Plateau, Southwest China.
Recent remote sensing studies have found increasing normalized difference vegetation index (NDVI) and plant productivity in the SAYR on the northeastern Qinghai-Tibet Plateau, Southwest China (Ge et al., 2018; Bai et al., 2020; Wang M. et al., 2020). Using the TTOP model simulations and remote sensing data, a recent study has showed that from 1980 to 2018, half of the areal extent of alpine permafrost in the SAYR (where permafrost accounts for 20.37% in areal extent) has been converted to seasonal frost, but the NDVI in the growing season has been increasing at an average rate of 0.002/10a, and; the increase in NDVI mainly occurred in the transitional zone between permafrost and seasonal frost (Wang R. et al., 2020). However, this does not necessarily signal the recovery of vegetation upon permafrost thaw in the HAYR. First, changes in vegetation species are difficult to determine based on the increase in NDVI, and the increased NDVI is likely due to the increase in weeds. In addition, the increase in NDVI is likely to be a short-term effect of permafrost degradation. In a long run, under a weak increase in precipitation, permafrost thaw will eventually lead to aridification of the environment, resulting in the degradation of alpine grasslands. However, the timely and proper implementation of ecological protection programs and establishment and prudent operation of national parks may have mitigated the vegetation degradation to some extents (e.g., Zhao et al., 2018).
Research Priorities
In the SAYR, undergoing changes in alpine vegetation are closely related to the complex interactions of climate warming, permafrost degradation, soil hydrological regimes, soil texture, and other environmental factors (Jin et al., 2020; Shi et al., 2020). However, more studies are needed on the moving trajectories of water from ice melting, total volume of the melt-water from the ground ice, and how the magnitudes of this melt-water contribute to surface water and groundwater. In addition, although it has been observed that snowfall accounts for 8.8% of annual precipitation, it has substantial implication for the occurrence of permafrost and the overlying alpine plants (Li et al., 2021). However, not much research, except for some studies from remote sensing, focus on the snow cover in this region, its changes, and its ecological, hydrological and engineering impact. This is mainly due to the area’s harsh environment and the challenge to establish and sustain longer term ground-based measurements and observation stations. More monitoring networks of snow cover, glaciers and frozen ground should be built and improved in the SAYR. In addition to the mountain cryosphere observational networks, long-term ecosystem and flow-system monitoring sites and networks should also be established and progressively improved in this region to study the vegetation succession trajectories coupled with the rapidly and extensively changing cryospheric components and their profound and extensive impacts. The impacts of permafrost degradation on carbon storage and stability, as well as greenhouse gases emissions and dissolved organic and inorganic carbon and nitrogen, in the SAYR, especially those in the extensively distributed alpine wetlands, meadows and peatlands and peat plateaus, are also critical for future climate change due to their positive feedback to climate warming through an enhanced carbon and nitrogen cycling.
All these environmental attributes have collectively structured the alpine plant ecosystem and changes in any individual driver can interact with other interwoven and interdependent variables. These complex and dynamically changing processes and mechanisms are the prompting challenges for the current scientific research and ecological management. Future research should build and improve the process-based model to better understand and predict how alpine vegetation may respond to permafrost/cryosphere-related hydrological and cryopedological changes, which is based on more systematic and integrated observational networks.
Conclusion
Research based on historical and contemporary data and simulations showed that alpine ecosystems in the SAYR, one of the cores of the Asian Water Towers and niches and refuges, for alpine species diversity, were, are, and will be affected by many environmental factors related to climate warming and permafrost degradation. According to the observational data from meteorological stations during the last 60 years, regional average air temperature in the SAYR has been rising at a multi-year average rate of 0.4°C/10a, while annual precipitation has been fluctuating with a slight wetting trend. As a result, from 1980 to 2016, permafrost extent shrank by about 2,000 km2; the ground warmed at a rate of 0.01–0.21°C/10a and the ALT enlarged at a rate of 35 cm/10a. These changes in permafrost features have produced substantial changes in surface and subsurface hydrologic regimes and flow dynamics. Under a warming climate, mean annual evaporation increased, while the surface runoff decreased, possibly due to the imbalance of only slightly increased annual precipitation, much enhanced evaporation, and extensively degrading permafrost. Moreover, the regional groundwater table in the Yellow River valleys declines simultaneously with the lowering permafrost table. This cannot be offset by the appreciably added water from the melting of ground ice and/or slightly increased precipitation. Field surveys have found a close association of grassland expansion and the lowering of groundwater table. Alpine vegetation cover is generally high when the burial depth of the supra-permafrost water table is less than 2 m, which is a critical value for alpine plants, and thus dubbed as the burial-depth of the ecologically safe supra-permafrost water table (BESST).
Temperature rise and permafrost degradation have been linked to the shrinkage of wetlands and marsh/paludal meadows, a decline in water conservation capacity, driving the adverse successions of alpine grassland ecosystems in the SAYR, and resulting in severe degradation of grasslands and reduced grassland productivity, diversity, and vegetation coverage. Moreover, blackened soil spots and land desertification have arisen with severe grassland degradation, seriously threatening the ecological and water security of the SAYR and surrounding areas. The growth of short-rooted herbaceous plants will be restricted if the BESST becomes deeper than 2 m. The long-term effect of a deeper ecological water table will promote a change in vegetation species, regional evolution of plant life, the overall evolution from hygrophytes to xerophytes, as well as the degradation and even desertification of alpine grasslands.
Data Availability Statement
The original contributions presented in the study are included in the article/Supplementary Material, further inquiries can be directed to the corresponding author.
Author Contributions
XJ and HJ conceived the project and organized the draft. DL, YS, and JW collected the boreholes data and offered the pictures. WW, SH, YL, and QM helped plot the figures. SL, QW, RH, RDS, and SG helped improve the manuscript. HJ, QW, and YS provided the funding for research activities.
Funding
This research was funded by the Chinese Academy of Sciences (CAS) Strategic Priority Research Program “Changing permafrost hydrology in the Qilian Mountains and the Source Area of the Yellow River and its impacts on water supplies and security” (Grant No. XDA20100103), National Natural Science Foundation of China (Grant Nos U20A2082 and 41871052), and Consulting Project of Ministry of Natural Resources “Impacts of shrinking glaciers and degrading permafrost on ecosystems, Yellow River discharge and carbon sources and sinks in the Source Area of Yellow River on northeastern Qinghai-Tibet Plateau, China”.
Conflict of Interest
The authors declare that the research was conducted in the absence of any commercial or financial relationships that could be construed as a potential conflict of interest.
Publisher’s Note
All claims expressed in this article are solely those of the authors and do not necessarily represent those of their affiliated organizations, or those of the publisher, the editors and the reviewers. Any product that may be evaluated in this article, or claim that may be made by its manufacturer, is not guaranteed or endorsed by the publisher.
Acknowledgments
The authors thank the reviewers for their review work to improve this manuscript.
References
Bai, Y., Guo, C., Degen, A. A., Ahmad, A. A., Wang, W., Zhang, T., et al. (2020). Climate Warming Benefits alpine Vegetation Growth in Three-River Headwater Region, China. Sci. Total Environ. 742, 140574. doi:10.1016/j.scitotenv.2020.140574
Bockheim, J. G. (2015). Global Distribution of Cryosols with Mountain Permafrost: an Overview. Permafrost Periglac. Process. 26, 1–12. doi:10.1002/ppp.1830
Cao, W., Wan, L., Zhou, X., Hu, F., Li, Z., and Liang, S. (2003). A Study of the Geological Environmental of Suprapermafrost Water in the Headwater Area of the Yellow River. Hydrogeol. Eng. Geol. 6, 6–10. (In Chinese with English abstract). doi:10.16030/j.cnki.issn.1000-3665.2003.06.002
Cao, H., Gao, B., Gong, T., and Wang, B. (2021a). Analyzing Changes in Frozen Soil in the Source Region of the Yellow River Using the MODIS Land Surface Temperature Products. Remote Sensing 13 (2), 180. doi:10.3390/rs13020180
Cao, W., Sheng, Y., Wu, J., and Peng, E. (2021b). Differential Response to Rainfall of Soil Moisture Infiltration in Permafrost and Seasonally Frozen Ground in Kangqiong Small basin on the Qinghai-Tibet Plateau. Hydrological Sci. J. 66, 525–543. doi:10.1080/02626667.2021.1883619
Cheng, G., and Jin, H. (2013). Groundwater in the Permafrost Regions on the Qinghai-Tibet Plateau and it Changes. Hydrogeol. Eng. Geol. 40, 1–11. doi:10.16030/j.cnki.issn.1000-3665.2013.01.017
Dong, L., Wang, W., Ma, M., Kong, J., and Veroustraete, F. (2009). The Change of Land Cover and Land Use and its Impact Factors in Upriver Key Regions of the Yellow River. Int. J. Remote Sensing 30, 1251–1265. doi:10.1080/01431160802468248
Feng, J., Wang, T., and Xie, C. (2006). Eco-environmental Degradation in the Source Region of the Yellow River, Northeast Qinghai-Xizang Plateau. Environ. Monit. Assess. 122, 125–143. doi:10.1007/s10661-005-9169-2
Gao, J., Li, X.-l., Cheung, A., and Yang, Y.-w. (2013). Degradation of Wetlands on the Qinghai-Tibet Plateau: A Comparison of the Effectiveness of Three Indicators. J. Mt. Sci. 10, 658–667. doi:10.1007/s11629-013-2562-3
Gao, Z., Niu, F., Wang, Y., Lin, Z., Luo, J., and Liu, M. (2018). Root-induced Changes to Soil Water Retention in Permafrost Regions of the Qinghai-Tibet Plateau, China. J. Soils Sediments 18, 791–803. doi:10.1007/s11368-017-1815-0
Gao, S., Jin, H., Bense, V. F., Wang, X., and Chai, X. (2019). Application of Electrical Resistivity Tomography for Delineating Permafrost Hydrogeology in the Headwater Area of Yellow River on Qinghai-Tibet Plateau, SW China. Hydrogeol. J. 27 (5), 1725–1737. doi:10.1007/s10040-019-01942-z
Gao, B., Li, J., and Wang, X. (2020a). Impact of Frozen Soil Changes on Vegetation Phenology in the Source Region of the Yellow River from 2003 to 2015. Theor. Appl. Climatol. 141, 1219–1234. doi:10.1007/s00704-020-03266-5
Gao, Z., Lin, Z., Niu, F., and Luo, J. (2020b). Soil Water Dynamics in the Active Layers under Different Land-Cover Types in the Permafrost Regions of the Qinghai-Tibet Plateau, China. Geoderma 364, 114176. doi:10.1016/j.geoderma.2020.114176
Ge, J., Meng, B., Liang, T., Feng, Q., Gao, J., Yang, S., et al. (2018). Modeling alpine Grassland Cover Based on MODIS Data and Support Vector Machine Regression in the Headwater Region of the Huanghe River, China. Remote Sensing Environ. 218, 162–173. doi:10.1016/j.rse.2018.09.019
G., W., J., Q., G., C., and Y., L. (2001). Eco-Environmental Degradation and Causal Analysis in the Source Region of the Yellow River. Environ. Geology. 40, 884–890. doi:10.1007/s002540100248
Haeberli, W. (2013). Mountain Permafrost - Research Frontiers and a Special Long-Term challenge. Cold Regions Sci. Technol. 96, 71–76. doi:10.1016/j.coldregions.2013.02.004
Han, Y. P., Chen, Z. T., and Xiao, H. (2018). Projection in Extreme Climate Events and Uncertainty Analysis in the Source Area of the Yellow River for the Next Three Decades. IOP Conf. Ser. Earth Environ. Sci. 199, 022024. doi:10.1088/1755-1315/199/2/022024
He, X., Yu, Y., Cui, Z., and He, T. (2021). Climate Change and Ecological Projects Jointly Promote Vegetation Restoration in Three-River Source Region of China. Chin. Geogr. Sci. 31, 1108–1122. doi:10.1007/s11769-021-1245-1
Hu, Y., Maskey, S., and Uhlenbrook, S. (2012). Trends in Temperature and Rainfall Extremes in the Yellow River Source Region, China. Climatic Change 110, 403–429. doi:10.1007/s10584-011-0056-2
Hu, G., Jin, H., Dong, Z., Lu, J., and Yan, C. (2013). Driving Forces of Aeolian Desertification in the Source Region of the Yellow River: 1975-2005. Environ. Earth Sci. 70, 3245–3254. doi:10.1007/s12665-013-2389-9
Intergovernmental Panel of Climate Change (IPCC) (2014). Climate Change 2014: Synthesis Report. Contribution of Working Groups I, II and III to the Fifth Assessment Report of the Intergovernmental Panel on Climate Change. Geneva, Switzerland: IPCC, 151.
Iqbal, M., Wen, J., Wang, X., Lan, Y., Tian, H., Anjum, M. N., et al. (2018). Assessment of Air Temperature Trends in the Source Region of Yellow River and its Sub-Basins, China. Asia-Pacific J. Atmos. Sci. 54, 111–123. doi:10.1007/s13143-017-0064-x
Jiang, P., Yu, Z., Yuan, F., and Acharya, K. (2019). The Multi-Scale Temporal Variability of Extreme Precipitation in the Source Region of the Yellow River. Water 11, 92. doi:10.3390/w11010092
Jin, H., Li, S., Cheng, G., Shaoling, W., and Li, X. (2000). Permafrost and Climatic Change in China. Glob. Planet. Change 26, 387–404. doi:10.1016/S0921-8181(00)00051-5
Jin, H., He, R., Cheng, G., Wu, Q., Wang, S., Lü, L., et al. (2009). Changes in Frozen Ground in the Source Area of the Yellow River on the Qinghai-Tibet Plateau, China, and Their Eco-Environmental Impacts. Environ. Res. Lett. 4, 045206. doi:10.1088/1748-9326/4/4/045206
Jin, X., Jin, H., Wu, X., Luo, D., Yu, S., Li, X., et al. (2020). Permafrost Degradation Leads to Biomass and Species Richness Decreases on the Northeastern Qinghai-Tibet Plateau. Plants 9, 1453. doi:10.3390/plants9111453
Jin, X.-Y., Jin, H.-J., Iwahana, G., Marchenko, S. S., Luo, D.-L., Li, X.-Y., et al. (2021). Impacts of Climate-Induced Permafrost Degradation on Vegetation: A Review. Adv. Clim. Change Res. 12 (1), 29–47. doi:10.1016/j.accre.2020.07.002
Jin, H.-J., Wu, Q.-B., and Romanovsky, V. E. (2021). Degrading Permafrost and its Impacts. Adv. Clim. Change Res. 12 (1), 1–5. doi:10.1016/j.accre.2021.01.007
Lan, C., Zhang, Y., Gao, Y., Hao, Z., and Cairang, L. (2013). The Impacts of Climate Change and Land Cover/use Transition on the Hydrology in the Upper Yellow River Basin, China. J. Hydrol. 502, 37–52. doi:10.1016/j.jhydrol.2013.08.003
Latif, A., Ilyas, S., Zhang, Y., Xin, Y., Zhou, L., and Zhou, Q. (2019). Review on Global Change Status and its Impacts on the Tibetan Plateau Environment. J. Plant Ecol. 12, 917–930. doi:10.1093/jpe/rtz038
Li, L., Wu, S., Zhu, X., Chang, G., and Li, F. (2008). Response of the Plateau Lakes to Changes of Climate and Frozen Earth Environment in the Headwaters of the Yellow River since the 21st century. J. Nat. Resour. 23, 245–253. (In Chinese with English abstract). doi:10.11849/zrzyxb.2008.02.009
Li, F., Chang, G., Xiao, J., Zhou, B., and Fu, Y. (2009). Relationship between Wetland Changes and Climate Change in the Yellow River Source Region. J. Nat. Resour. 24, 683–690. (In Chinese with English abstract). doi:10.11849/zrzyxb.2009.04.014
Li, J., Sheng, Y., Wu, J., Feng, Z., Ning, Z., Hu, X., et al. (2016). Landform-related Permafrost Characteristics in the Source Area of the Yellow River, Eastern Qinghai-Tibet Plateau. Geomorphology 269, 104–111. doi:10.1016/j.geomorph.2016.06.024
Li, Q., Yang, M., Wan, G., and Wang, X. (2016). Spatial and Temporal Precipitation Variability in the Source Region of the Yellow River. Environ. Earth Sci. 75, 594. doi:10.1007/s12665-016-5583-8
Li, X., Xue, Z., and Gao, J. (2016c). Dynamic Changes of Plateau Wetlands in Madou County, the Yellow River Source Zone of China: 1990-2013. Wetlands 36, 299–310. doi:10.1007/s13157-016-0739-6
Li, X., Yao, Z., Dong, Z., and Xiao, J. (2016d). Causes and Processes of sandy Desertification in Guinan County, Qinghai-Tibet Plateau. Environ. Earth Sci. 75, 1–12. doi:10.1007/s12665-016-5481-0
Li, G., Li, X., Chen, W., Li, J., Zhu, H., Hu, X., et al. (2020c). Effects of Degradation Severity on the Physical, Chemical and Mechanical Properties of Topsoil in alpine Meadow on the Qinghai-Tibet Plateau, West China. Catena 187, 104370. doi:10.1016/j.catena.2019.104370
Li, Z., Ma, J., Song, L., Gui, J., Xue, J., Zhang, B., et al. (2020a). Investigation of Soil Water Hydrological Process in the Permafrost Active Layer Using Stable Isotopes. Hydrological Process. 34, 2810–2822. doi:10.1002/hyp.13765
Li, Z., Gao, P., Hu, X., Yi, Y., Pan, B., and You, Y. (2020b). Coupled Impact of Decadal Precipitation and Evapotranspiration on Peatland Degradation in the Zoige basin, China. Phys. Geogr. 41, 145–168. doi:10.1080/02723646.2019.1620579
Li, Z., Lyu, S., Chen, H., Ao, Y., Zhao, L., and Wang, S. (2021). Changes in Climate and Snow Cover and Their Synergistic Influence on Spring Runoff in the Source Region of the Yellow River. Sci. Total Environ. 799, 149503. doi:10.1016/j.scitotenv.2021.149503
Liang, S., Wan, L., Li, Z., and Cao, W. (2007). The Effect of Permafrost on alpine Vegetation in the Source Regions of the Yellow River. J. Glaciol. Geocryol. 29, 45–52. (In Chinese with English abstract). doi:10.3969/j.issn.1000-0240.2007.01.008
Lin, M., Biswas, A., and Bennett, E. M. (2019). Spatio-Temporal Dynamics of Groundwater Storage Changes in the Yellow River Basin. J. Environ. Manage. 235, 84–95. doi:10.1016/j.jenvman.2019.01.016
Liu, C., Wang, P., Wen, T., Yu, D., and Bai, W. (2021). Spatio-Temporal Characteristics of Climate Change in the Yellow River Source Area from 1960 to 2019. Arid Zone Res. 38, 293–302. (In Chinese with English abstract). doi:10.13866/j.azr.2021.02.01
Luo, D., HuiJun, J., Marchenko, S., and Romanovsky, V. (2014). Distribution and Changes of Active Layer Thickness (ALT) and Soil Temperature (TTOP) in the Source Area of the Yellow River Using the GIPL Model. Sci. China Earth Sci. 57, 1834–1845. doi:10.1007/s11430-014-4852-1
Luo, D., Jin, H., Lü, L., and Zhou, J. (2016). Spatiotemporal Changes in Extreme Ground Surface Temperatures and the Relationship with Air Temperatures in the Three-River Source Regions during 1980-2013. Theor. Appl. Climatol. 123, 885–897. doi:10.1007/s00704-015-1543-6
Luo, D., Jin, H., Jin, X., He, R., Li, X., Muskett, R. R., et al. (2018). Elevation-Dependent Thermal Regime and Dynamics of Frozen Ground in the Bayan Har Mountains, Northeastern Qinghai-Tibet Plateau, Southwest China. Permafrost and Periglac Process 29, 257–270. doi:10.1002/ppp.1988
Luo, D., Jin, H., Bense, V. F., Jin, X., and Li, X. (2020a). Hydrothermal Processes of Near-Surface Warm Permafrost in Response to strong Precipitation Events in the Headwater Area of the Yellow River, Tibetan Plateau. Geoderma 376, 114531. doi:10.1016/j.geoderma.2020.114531
Luo, D., Liu, L., Jin, H., Wang, X., and Chen, F. (2020b). Characteristics of Ground Surface Temperature at Chalaping in the Source Area of the Yellow River, Northeastern Tibetan Plateau. Agric. For. Meteorology 281, 107819. doi:10.1016/j.agrformet.2019.107819
Luo, D., Jin, H., Du, H., Li, C., Ma, Q., Duan, S., et al. (2020c). Variation of alpine Lakes from 1986 to 2019 in the Headwater Area of the Yellow River, Tibetan Plateau Using Google Earth Engine. Adv. Clim. Change Res. 11, 11–21. doi:10.1016/j.accre.2020.05.007
Lv, M., Wang, Y., and Gao, Z. (2022). The Change Process of Soil Hydrological Properties in the Permafrost Active Layer of the Qinghai-Tibet Plateau. CATENA 210, 105938. doi:10.1016/j.catena.2021.105938
Ma, Q., Jin, H.-J., Bense, V. F., Luo, D.-L., Marchenko, S. S., Harris, S. A., et al. (2019). Impacts of Degrading Permafrost on Streamflow in the Source Area of Yellow River on the Qinghai-Tibet Plateau, China. Adv. Clim. Change Res. 10, 225–239. doi:10.1016/j.accre.2020.02.001
Man, Z., Weng, B., Yang, Y., Gong, X., Li, M., and Yu, Z. (2019). Effects of the Freezing-Thawing Cycle Mode on alpine Vegetation in the Nagqu River Basin of the Qinghai-Tibet Plateau. Water 11, 2122. doi:10.3390/w11102122
Mu, C., Li, L., Zhang, F., Li, Y., Xiao, X., Zhao, Q., et al. (2018). Impacts of Permafrost on above- and Belowground Biomass on the Northern Qinghai-Tibetan Plateau. Arctic, Antarctic, Alpine Res. 50, e1447192. doi:10.1080/15230430.2018.1447192
Niu, Y. (2020). Wetland Information Extraction of the Yellow River Source Based on Decision Tree Model. Master’s thesis. Beijing (China): China University of Geosciences.
Pepin, N., Bradley, R. S., Diaz, H. F., Baraër, M., Caceres, E. B., Forsythe, N., et al. (2015). Elevation-dependent Warming in Mountain Regions of the World. Nat. Clim Change 5, 424–430. doi:10.1038/nclimate2563
Qin, Y., Yang, D., Gao, B., Wang, T., Chen, J., Chen, Y., et al. (2017). Impacts of Climate Warming on the Frozen Ground and Eco-Hydrology in the Yellow River Source Region, China. Sci. Total Environ. 605-606, 830–841. doi:10.1016/j.scitotenv.2017.06.188
Qin, Y., Zhang, P., Liu, W., Guo, Z., and Xue, S. (2020). The Application of Elevation Corrected MERRA2 Reanalysis Ground Surface Temperature in a Permafrost Model on the Qinghai-Tibet Plateau. Cold Regions Sci. Technol. 175, 103067. doi:10.1016/j.coldregions.2020.103067
Schuur, E. A. G., and Mack, M. C. (2018). Ecological Response to Permafrost Thaw and Consequences for Local and Global Ecosystem Services. Annu. Rev. Ecol. Evol. Syst. 49, 279–301. doi:10.1146/annurev-ecolsys-121415-032349
Șerban, R.-D., Jin, H., Șerban, M., Luo, D., Wang, Q., Jin, X., et al. (2020). Mapping Thermokarst Lakes and Ponds across Permafrost Landscapes in the Headwater Area of Yellow River on Northeastern Qinghai-Tibet Plateau. Int. J. Remote Sensing 41, 7042–7067. doi:10.1080/01431161.2020.1752954
Șerban, R. D., Jin, H., Șerban, M., and Luo, D. (2021). Shrinking Thermokarst Lakes and Ponds on the Northeastern Qinghai‐Tibet Plateau over the Past Three Decades. Permafrost and Periglac Process 32, 601–617. doi:10.1002/ppp.2127
Sheng, Y., Ma, S., Cao, W., and Wu, J. (2020). Spatiotemporal Changes of Permafrost in the Headwater Area of the Yellow River under a Changing Climate. Land Degrad. Dev. 31, 133–152. doi:10.1002/ldr.3434
Shi, R., Yang, H., and Yang, D. (2020). Spatiotemporal Variations in Frozen Ground and Their Impacts on Hydrological Components in the Source Region of the Yangtze River. J. Hydrol. 590, 125237. doi:10.1016/j.jhydrol.2020.125237
Sjöberg, Y., Coon, E., Sannel, A. B. K., Harp, D., Frampton, A., Painter, S. L., et al. (2016). Thermal Effects of Groundwater Flow through Subarctic Fens: A Case Study Based on Field Observations and Numerical Modeling. Water Resour. Res. 52, 1591–1606. doi:10.1002/2015WR017571
Sun, A., Zhou, J., Yu, Z., Jin, H., Sheng, Y., and Yang, C. (2019). Three-dimensional Distribution of Permafrost and Responses to Increasing Air Temperatures in the Head Waters of the Yellow River in High Asia. Sci. Total Environ. 666, 321–336. doi:10.1016/j.scitotenv.2019.02.110
Sun, A., Yu, Z., Zhou, J., Acharya, K., Ju, Q., Xing, R., et al. (2020). Quantified Hydrological Responses to Permafrost Degradation in the Headwaters of the Yellow River (HWYR) in High Asia. Sci. Total Environ. 712, 135632. doi:10.1016/j.scitotenv.2019.135632
Tian, H., Lan, Y., Wen, J., Jin, H., Wang, C., Wang, X., et al. (2015). Evidence for a Recent Warming and Wetting in the Source Area of the Yellow River (SAYR) and its Hydrological Impacts. J. Geogr. Sci. 25, 643–668. doi:10.1007/s11442-015-1194-7
Walvoord, M. A., and Kurylyk, B. L. (2016). Hydrologic Impacts of Thawing Permafrost-A Review. Vadose Zone J. 15, vzj2016.01.0010. doi:10.2136/vzj2016.01.0010
Wang, G., Li, Y., Wu, Q., and Wang, Y. (2006). Impacts of Permafrost Changes on Alpine Ecosystem in Qinghai-Tibet Plateau. Sci. China Ser. D 49, 1156–1169. doi:10.1007/s11430-006-1156-0
Wang, G., Bai, W., Li, N., and Hu, H. (2011). Climate Changes and its Impact on Tundra Ecosystem in Qinghai-Tibet Plateau, China. Climatic Change 106, 463–482. doi:10.1007/s10584-010-9952-0
Wang, Z., Yang, G., Yi, S., Wu, Z., Guan, J., He, X., et al. (2012). Different Response of Vegetation to Permafrost Change in Semi-Arid and Semi-Humid Regions in Qinghai-Tibetan Plateau. Environ. Earth Sci. 66, 985–991. doi:10.1007/s12665-011-1405-1
Wang, S., Sheng, Y., Li, J., Wu, J., Cao, W., and Ma, S. (2018). An Estimation of Ground Ice Volumes in Permafrost Layers in Northeastern Qinghai-Tibet Plateau, China. Chin. Geogr. Sci. 28, 61–73. doi:10.1007/s11769-018-0932-z
Wang, T., Yang, H., Yang, D., Qin, Y., and Wang, Y. (2018). Quantifying the Streamflow Response to Frozen Ground Degradation in the Source Region of the Yellow River within the Budyko Framework. J. Hydrol. 558, 301–313. doi:10.1016/j.jhydrol.2018.01.050
Wang, Y., Yang, H., Gao, B., Wang, T., Qin, Y., and Yang, D. (2018). Frozen Ground Degradation May Reduce Future Runoff in the Headwaters of an Inland River on the Northeastern Tibetan Plateau. J. Hydrol. 564, 1153–1164. doi:10.1016/j.jhydrol.2018.07.078
Wang, R., Dong, Z.-B., and Zhou, Z.-V. C. (2019). Changes in the Depths of Seasonal Freezing and Thawing and Their Effects on Vegetation in the Three-River Headwater Region of the Tibetan Plateau. J. Mt. Sci. 16, 2810–2827. doi:10.1007/s11629-019-5450-7
Wang, R., Dong, Z., and Zhou, Z. (2020). Different Responses of Vegetation to Frozen Ground Degradation in the Source Region of the Yellow River from 1980 to 2018. Chin. Geogr. Sci. 30, 557–571. doi:10.1007/s11769-020-1135-y
Wang, J., Zhu, Q., Yang, Y., Zhang, X., Zhang, J., Yuan, M., et al. (2020). High Uncertainties Detected in the Wetlands Distribution of the Qinghai-Tibet Plateau Based on Multisource Data. Landscape Ecol. Eng. 16, 47–61. doi:10.1007/s11355-019-00402-w
Wang, M., Fu, J. E., Wu, Z., and Pang, Z. (2020). Spatiotemporal Variation of NDVI in the Vegetation Growing Season in the Source Region of the Yellow River, China. ISPRS Int. J. Geo-inf. 9, 282. doi:10.3390/ijgi9040282
Wang, X., Chen, D., Pang, G., Gou, X., and Yang, M. (2021). Historical and Future Climates over the Upper and Middle Reaches of the Yellow River Basin Simulated by a Regional Climate Model in CORDEX. Clim. Dyn. 56, 2749–2771. doi:10.1007/s00382-020-05617-4
Watts, J. D., Kimball, J. S., Bartsch, A., and McDonald, K. C. (2014). Surface Water Inundation in the Boreal-Arctic: Potential Impacts on Regional Methane Emissions. Environ. Res. Lett. 9, 075001. doi:10.1088/1748-9326/9/7/075001
Wrona, F. J., Johansson, M., Culp, J. M., Jenkins, A., Mård, J., Myers-Smith, I. H., et al. (2016). Transitions in Arctic Ecosystems: Ecological Implications of a Changing Hydrological Regime. J. Geophys. Res. Biogeosci. 121, 650–674. doi:10.1002/2015JG003133
Wu, X., Zhang, X., Xiang, X., Zhang, K., Jin, H., Chen, X., et al. (2018). Changing Runoff Generation in the Source Area of the Yellow River: Mechanisms, Seasonal Patterns and Trends. Cold Regions Sci. Technol. 155, 58–68. doi:10.1016/j.coldregions.2018.06.014
Wu, J., Li, M., Zhang, X., Fiedler, S., Gao, Q., Zhou, Y., et al. (2021). Disentangling Climatic and Anthropogenic Contributions to Nonlinear Dynamics of alpine Grassland Productivity on the Qinghai-Tibetan Plateau. J. Environ. Manage. 281, 111875. doi:10.1016/j.jenvman.2020.111875
Xiang, L., Wang, H., Steffen, H., Wu, P., Jia, L., Jiang, L., et al. (2016). Groundwater Storage Changes in the Tibetan Plateau and Adjacent Areas Revealed from GRACE Satellite Gravity Data. Earth Planet. Sci. Lett. 449, 228–239. doi:10.1016/j.epsl.2016.06.002
Xie, J., Xu, Y.-P., Guo, Y., and Wang, Y. (2021). Detecting the Dominant Contributions of Runoff Variance across the Source Region of the Yellow River Using a New Decomposition Framework. Hydrol. Res. 52, 1015–1032. doi:10.2166/nh.2021.179
Yan, D., Lai, Z., and Ji, G. (2020). Using Budyko-type Equations for Separating the Impacts of Climate and Vegetation Change on Runoff in the Source Area of the Yellow River. Water 12, 3418. doi:10.3390/w12123418
Yang, M., Nelson, F. E., Shiklomanov, N. I., Guo, D., and Wan, G. (2010). Permafrost Degradation and its Environmental Effects on the Tibetan Plateau: A Review of Recent Research. Earth-Science Rev. 103, 31–44. doi:10.1016/j.earscirev.2010.07.002
Yang, Z.-p., Gao, J.-x., Zhao, L., Xu, X.-l., and Ouyang, H. (2013). Linking Thaw Depth with Soil Moisture and Plant Community Composition: Effects of Permafrost Degradation on alpine Ecosystems on the Qinghai-Tibet Plateau. Plant Soil 367, 687–700. doi:10.1007/s11104-012-1511-1
Yang, Y., Wu, Q., Jin, H., Wang, Q., Huang, Y., Luo, D., et al. (2019). Delineating the Hydrological Processes and Hydraulic Connectivities under Permafrost Degradation on Northeastern Qinghai-Tibet Plateau, China. J. Hydrol. 569, 359–372. doi:10.1016/j.jhydrol.2018.11.068
Yi, S., Zhou, Z., Ren, S., Xu, M., Qin, Y., Chen, S., et al. (2011). Effects of Permafrost Degradation on alpine Grassland in a Semi-arid basin on the Qinghai-Tibetan Plateau. Environ. Res. Lett. 6, 045403. doi:10.1088/1748-9326/6/4/045403
Yuan, Q., Yuan, Q., and Ren, P. (2021). Coupled Effect of Climate Change and Human Activities on the Restoration/degradation of the Qinghai-Tibet Plateau Grassland. J. Geogr. Sci. 31, 1299–1327. doi:10.1007/s11442-021-1899-8
Zhao, X., Zhao, L., Li, Q., Chen, H., Zhou, H., Xu, S., et al. (2018). Using Balance of Seasonal Herbage Supply and Demand to Inform Sustainable Grassland Management on the Qinghai-Tibetan Plateau. Front. Agr. Sci. Eng. 5, 1–8. doi:10.15302/J-FASE-2018203
Keywords: permafrost degradation, ecological impacts, alpine permafrost ecosystems, burial depth of ecologically-safe groundwater table, source area of the Yellow River
Citation: Jin X, Jin H, Luo D, Sheng Y, Wu Q, Wu J, Wang W, Huang S, Li X, Liang S, Wang Q, He R, Serban RD, Ma Q, Gao S and Li Y (2022) Impacts of Permafrost Degradation on Hydrology and Vegetation in the Source Area of the Yellow River on Northeastern Qinghai-Tibet Plateau, Southwest China. Front. Earth Sci. 10:845824. doi: 10.3389/feart.2022.845824
Received: 30 December 2021; Accepted: 31 January 2022;
Published: 15 March 2022.
Edited by:
Fahu Chen, Institute of Tibetan Plateau Research (CAS), ChinaReviewed by:
Jing Luo, Northwest Institute of Eco-Environment and Resources (CAS), ChinaZhanju Lin, Lanzhou Branch of Chinese Academy of Sciences, China
Copyright © 2022 Jin, Jin, Luo, Sheng, Wu, Wu, Wang, Huang, Li, Liang, Wang, He, Serban, Ma, Gao and Li. This is an open-access article distributed under the terms of the Creative Commons Attribution License (CC BY). The use, distribution or reproduction in other forums is permitted, provided the original author(s) and the copyright owner(s) are credited and that the original publication in this journal is cited, in accordance with accepted academic practice. No use, distribution or reproduction is permitted which does not comply with these terms.
*Correspondence: Huijun Jin, aGpqaW5AbHpiLmFjLmNu