- 1College of Earth Science, Chengdu University of Technology, Chengdu, China
- 2Institute of Sedimentary Geology, Chengdu University of Technology, Chengdu, China
- 3Chengdu Center of China Geological Survey, Chengdu, China
Major and trace element zonation patterns were determined in ultrahigh-pressure eclogite garnets from the Changning-Menglian suture, Bangbing area. Several stages of eclogite metamorphism are recorded by garnet zonation. Cores of garnets are generally darker and inclusion-rich, while rims are brighter and inclusion-poor. Garnets were analyzed for the major element by EPMA and trace element by LA-ICP-MS. The results show that both major elements and rare earth elements in garnet have typical compositional bands. The major element zonation recorded the process of increasing temperature and pressure and the metamorphism of the eclogite from the core to rim, and the rare earth element zonation recorded different mineral chemical reactions during the formation of the garnet and its three stages of growth. Eclogites completely imply the process of rapid exhumation, cooling, and depressurization after short-term peak metamorphism. The contents of some trace elements (such as Y and Sr) in garnet are controlled by major elements and mineral combinations. The formation of new minerals in the process of retrograde metamorphism affects the distribution of trace elements.
Introduction
Eclogite is generally considered a typical product of the high-pressure metamorphism of modern plate tectonics (Carswell, 1990; Condie and Kröner, 2008). Eclogite-facies rocks including garnet + omphacite + SiO2 phase (quartz/coesite) assemblages are common components of the deeper part of subducted slabs and record information to constrain geochemical and geodynamic processes occurring at plate-convergent margins (Bebout, 2007; Hacker & Gerya, 2013; Taguchi et al., 2019). The Changning-Menglian suture zone is the southern extension of the Longmucuo-Shuanghu suture zone in the northern Qinghai–Tibetan Plateau. Many studies have been performed on these eclogites in the Longmucuo-Shuanghu suture zone. The eclogites are produced in the form of lenses that occur in garnet-phegite schist. Apart from Baqing eclogites, most eclogites from the Qiangtang block are characterized by low peak temperatures and the presence of lawsonite or its pseudomorphs. The peak stage of metamorphism takes place in the lawsonite-eclogite facies, which is the main metamorphic age occurring during the Late Triassic. Four stages of metamorphic evolution in eclogites represent the process of subduction of the Paleo-Tethys Ocean and collision orogeny between Gondwana and the continent in the Paleo-Tethys Ocean (Zhai, 2008; Dong et al., 2010; Zhai, 2013; Zhang et al., 2018). The lithology of the Changning-Menglian suture zone is similar to that of the Longmucuo-Shuanghu suction zone, and they likely represent a united residual of the Paleozoic Tethys Ocean (Wang et al., 2018). Eclogite was discovered in recent years in the Mengku, Qianmai, and Bangbing areas in the Changning-Menglian suture zone. The eclogites in the Mengku area feature strong retrograde metamorphism that is mainly in the form of garnet-amphibolite, while the eclogites from the Qianmai and Bangbing areas are fresh with weak retrogression (Li et al., 2015; Li et al., 2017; Sun et al., 2019; Peng et al., 2019). The Bangbing eclogite retains complete petrological information and has become an important research object to investigate the metamorphic evolution of the Changning-Menglian suction zone and to further explore the subduction-orogeny process of the Paleo-Tethys Ocean on the southeastern Tibetan Plateau.
Garnet is highly refractory and has low solubility in metamorphic fluids and anatectic melts, meaning that it is stable over a wide range of temperatures and pressures (Baxter et al., 2013; Xia et al., 2013). It may preserve geochemical zonation and mineral/fluid/melt inclusions in many conditions and has become an important mineral for the study of metamorphism. It has been demonstrated that the geochemical zonation of garnet is related to metamorphic pressure, temperature, and associated mineral assemblages (Xia and Zheng, 2011; Xia, 2019; Kohn, 2003). The change in the chemical composition reveals the environmental change and metamorphic processes during the growth of garnet, so it could play a vital role in the study of metamorphic rocks and metamorphic evolution. Major element zonation in garnet is susceptible to metamorphic modification via thermal diffusion or even be flattened at high temperatures in excess of 650°C. Trace elements in garnet are less susceptible to diffusion modification at high temperatures because of their larger cation radii (Chernoff C B and Carlson W D, 1999; Zhou et al., 2011). Trace element zonation in garnet is stronger than major element zoning and allows a much clearer identification of phases contributing to garnet-forming reactions and is thus a better-defined metamorphic evolution. The composition of trace elements is more sensitive to changes in petrochemical kinetics and mineral assemblage than that of major elements, which can well indicate the crystallization and growth process of garnet and related mineral chemical reactions.
As a major mineral in eclogite, garnet can grow at almost every stage during continental subduction and exhumation, which presents a lot of information about the metamorphic properties of the host rock. Zonation patterns were determined in garnet from the Bangbing area. Based on the analysis of the main and trace elements of garnet zonation, this study identifies the multistage growth of garnet, attempts to reveal the metamorphic evolution process of host eclogite, and explores the migration behavior of trace elements.
Geological setting
The Changning-Menglian suture zone at the southeastern Tibetan Plateau has widespread ophiolitic complexes, and the zone is connected to the Longmucuo-Shuanghu suture zone to the north and the Qingmai suture zone in Thailand to the south (Figure 1). It is adjacent to the Baoshan block in the west and the Lincang-Menghai magmatic arc to the east. The eclogite that we studied occurs in the Lancang Group. The Lancang Group is distributed along an elongated belt from Fengqing County in the north via Yun County, Lincang (Peng et al., 2018a; Wang et al., 2018). The group is mainly dominated by mica–quartz schist and biotite–muscovite schist, greenschist, albite schist, and amphibole schist. Meta-basic rocks, meta-acidic rocks, and high-pressure metamorphic rocks discretely occur as blocks and lenses. The eclogites occur in grayish-white garnet–mica schist and are produced in the form of varied sizes of lenses. This group of mica schists has light color and is coarse-grained, which is different from other non-host muscovite schists. Glaucophane with different contents is common in the schist around the eclogite belt, which forms a nearly north–south blueschist belt, referred to as the Suyi blueschist belt in the literature. In summary, the eclogites in this study occur in the Suyi blueschist belt, and they are produced in the form of lenses of varied sizes. The Lancang Group has experienced three stages of metamorphic deformation, and all lithologic layers are connected by tectonic foliation (Fu et al., 2021).
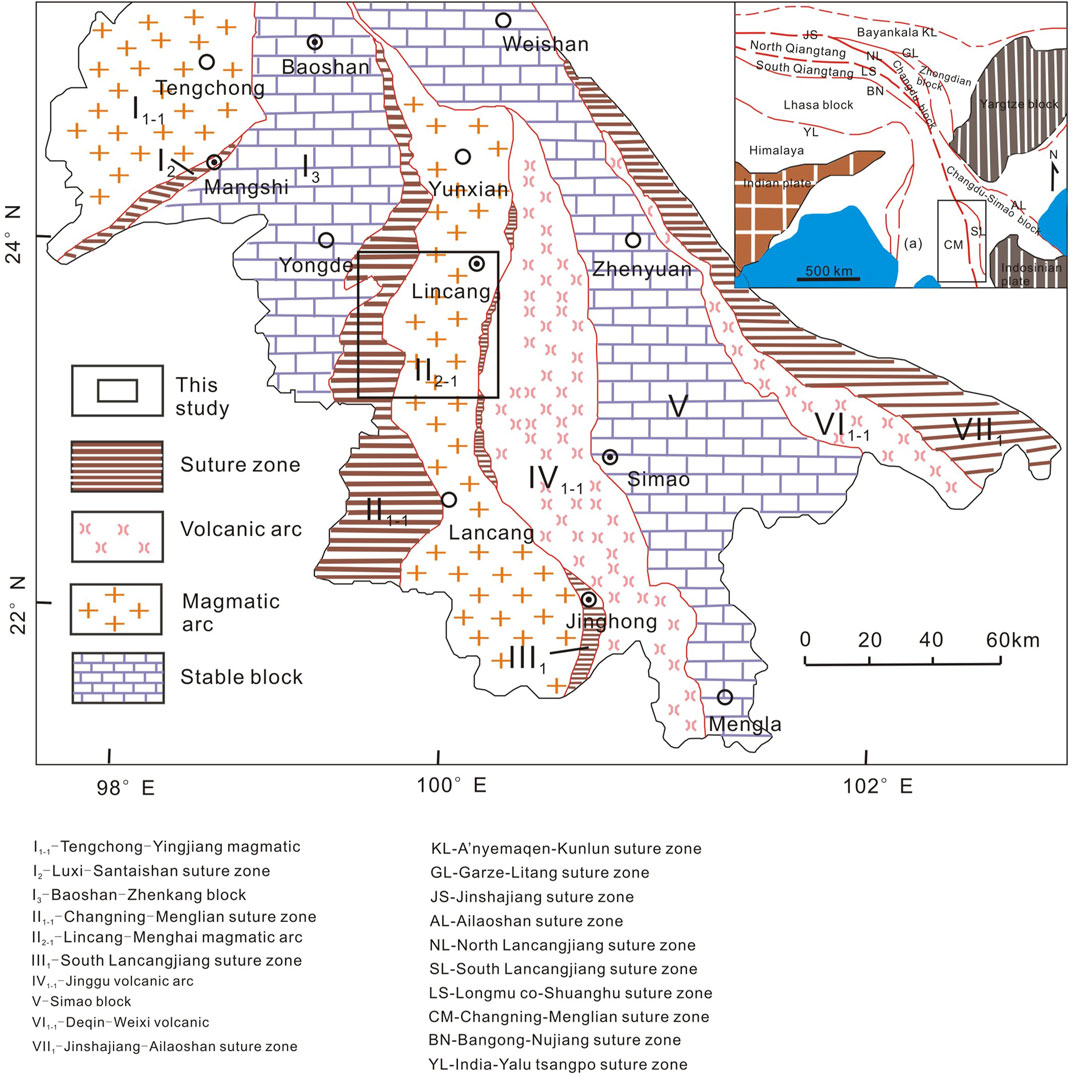
FIGURE 1. Geological map of the Changning-Menglian suture zone (after Fu et al., 2021)
Sample description
The samples are from the Bangbing area, including glaucophane eclogite and actinolite-bearing eclogite. Two kinds of eclogites all occur in the Lancang Group and Suyi blueschist belt. The eclogites are fresh and well preserved. According to the mineral composition of garnet, they are all type-C eclogites, typical of oceanic eclogites (Coleman et al., 1965).
Glaucophane eclogites are grayish-green with a porphyroblastic texture and a blocky structure. Porphyroblasts include garnet (25%), glaucophane (10%), and clinozoisite (5%–10%). The metamorphosed matrix with granular crystalloblastic texture consists of omphacite (40%), glaucophane (5%), quartz (5%), chlorite (5%), phengite (3%), and rutile (3%) (Figure 2A). Garnets from glaucophane eclogite in the selected samples are euhedral to subhedral granular, between 0.2 and 5 mm diameter, and rhombic dodecahedrons in shape and show visible growth zones. The boundary between omphacite and garnet in the metamorphosed matrix of glaucophane eclogite is clear and straight. The garnet is mostly dispersed in eclogite and occurs in the form of porphyroblasts. Small particle clusters and massive distribution can also be seen. Most of these garnets develop obvious zonation, which is mainly manifested in color zoning and inclusion zoning. The cores of garnet are dark red, and the rims are lighter. The cores of the garnets from glaucophane eclogites are generally inclusion-rich, while the rims are inclusion-poor. There are glaucophane, omphacite, chlorite, rutile, and minor amounts of quartz as inclusions in garnet. Subhedral glaucophane inclusions also show compositional zonation. The Na content from the core to the rim of the inclusion glaucophane increases, and the mineral composition is reflected in the change from winchite to riebeckite. This feature reflects the process of progressive metamorphism and temperature rise and shows that the host garnet experienced the process of progressive metamorphism during this period. Radial cracks around quartz inclusions may retrograde by coesite and indicate ultrahigh-pressure metamorphism (Figure 2C).
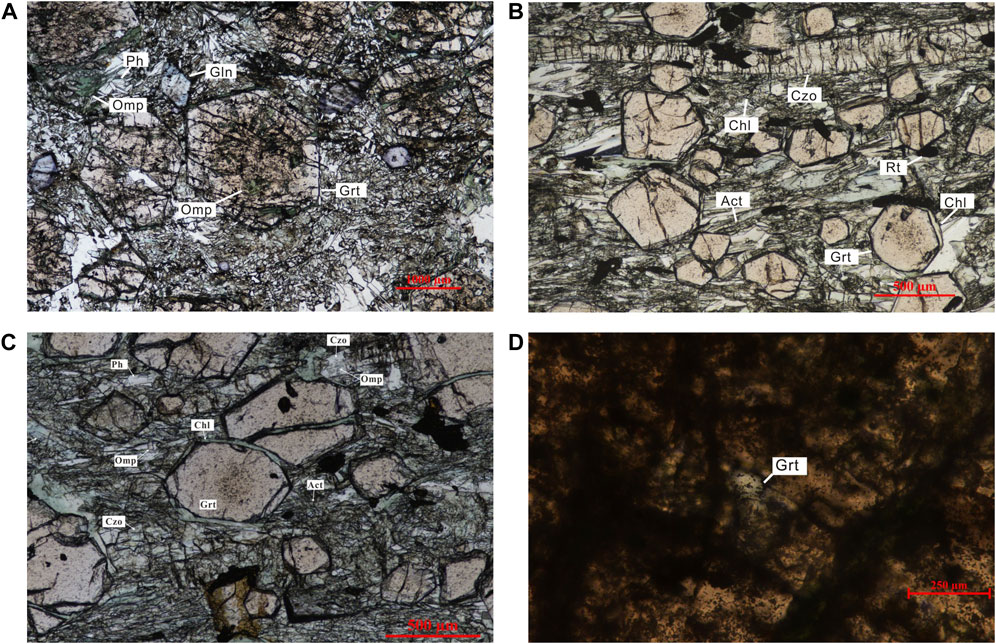
FIGURE 2. Photomicrograph images of eclogite. (A) Glaucophane eclogite with mineral assemblage of garnet + omphacite + glaucophane + phengite. (B) Actinolite-bearing eclogite with mineral assemblage of garnet + omphacite + actinolite + clinozoisite. (C) Chlorite surrounds garnet. (D) Radial cracks around quartz inclusion. Ph, phengite; Act, actinolite; Rt, rutile; Chl, chlorite; Czo, clinozoisite.
Actinolite-bearing eclogite features a porphyroblastic texture and a massive structure. Porphyroblasts include garnet (35%) and clinozoisite (5%). The metamorphosed matrix shows granular crystalloblastic texture, mainly including omphacite (40%), actinolite (10%), chlorite (5%), quartz (5%), and minor amounts of phengite and rutile (Figure 2B). Garnet from actinolite-bearing eclogite is mainly manifested in color zoning but is inclusion-poor. The actinolite-bearing eclogites may be retrograded by the glaucophane eclogites. The particle size of garnet from actinolite-bearing eclogite is less than that of garnet from glaucophane eclogite, while compositional and color zoning also developed. They are inclusion-poor and surrounded by chlorite. Chlorite surrounds garnet and is either circular or distributed along fractures of garnet grains (Figure 2D).
Analytical techniques
Mineral analyses were performed using a JEOL JXA- 8230 electron probe microanalyzer with four wavelength-dispersive spectrometers (WDSs) at the Center for Global Tectonics, School of Earth Sciences, China University of Geosciences (Wuhan). First, the samples were observed under a light microscope. A 15 kV accelerating voltage, 20 nA probe current, and 1- or 5-micron beam diameter were used based on different minerals. The precision and accuracy of the analyses were better than 2%, and most elements were within ∼1% of the relative error. The operating conditions were described in Wang J et al. (2019) and Ning et al. (2019) in detail.
Garnet was analyzed for REEs and trace elements in polished thin sections using laser ablation–inductively coupled plasma–mass spectrometry (LA-ICP-MS) using an Agilent 7900 ICP-MS system attached to a GeoLas HD laser ablation at Wuhan Sample Solution Analytical Technology Co., Ltd., Wuhan, China. The beam size was 44 µm with a 5 Hz repetition rate. The operating conditions followed those described in Liu et al. (2008) in detail.
Results
Major elements
The results of major element analysis of garnet in eclogite from the Bangbing area are listed in Table 1. Compositional zonation in the garnet from the glaucophane eclogites shows an increase in pyrope and grossular and a decrease in spessartine and almandine from the cores to rims, with the composition of the cores being Alm65–70Sps1.0–1.4Grs20–23Prp6–11 and that of the rims being Alm53–62Sps0.4–0.9Grs22–28Prp14–18 (Table 1; Figures 3A,B). The garnet has the highest almandine content and the lowest spessartine content. The composition of all garnets is in the field of C-type high-pressure and low-temperature eclogite, which is a typical marine eclogite.
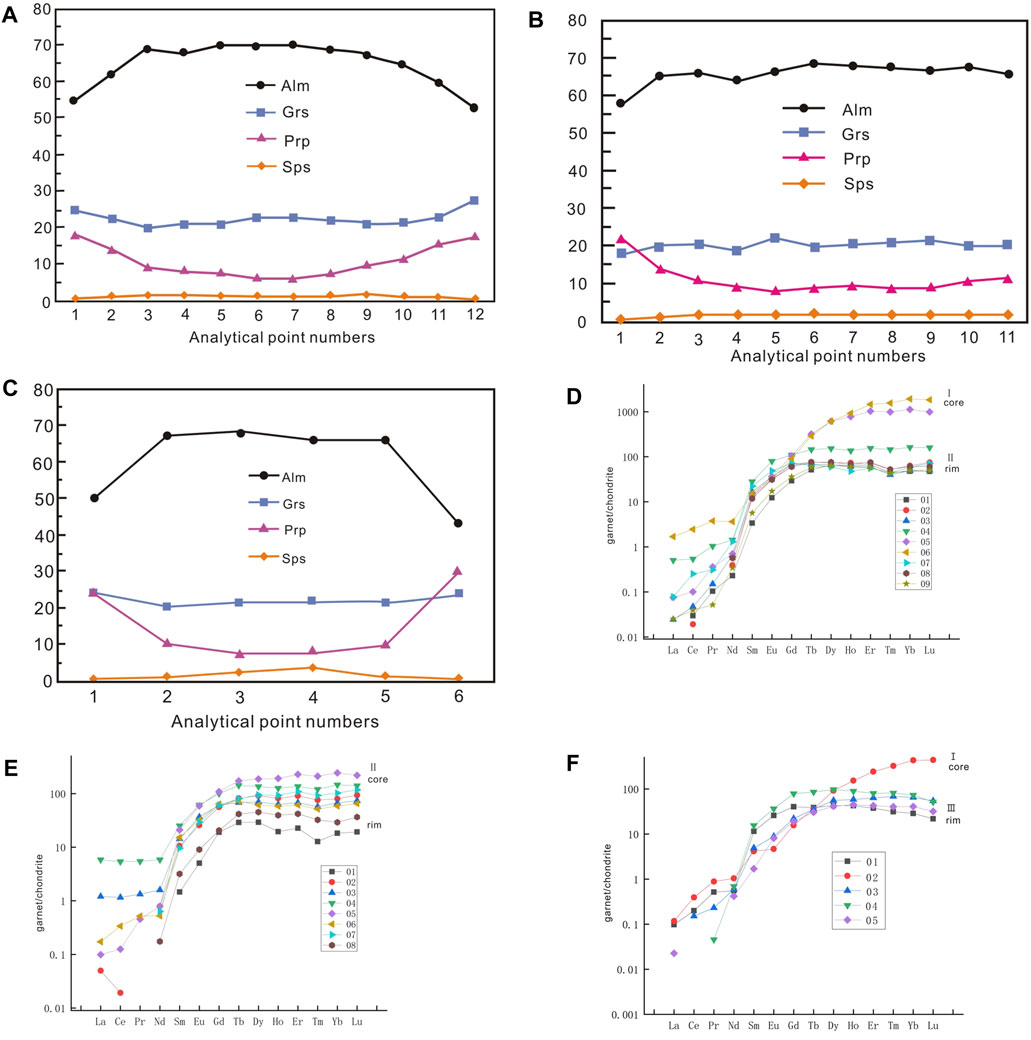
FIGURE 3. Compositional profile zonation of the garnet and chondrite-normalized REE patterns of garnet. (A,B) Major element compositional of garnet in glaucophane eclogite; (C) major element compositional of garnet in actinolite-bearing eclogite; (D,E) chondrite-normalized REE patterns of garnet in glaucophane eclogite; and (F) chondrite-normalized REE patterns of garnet in actinolite-bearing eclogite (Sun and Mcdough, 1989).
Garnet from the actinolite-bearing eclogites also developed compositional zoning. Garnet has the following chemical composition: cores of Alm66–68Sps0.9–3.5Grs21–22Prp8–10 to the rims of Alm44–50Prp24–30Grs24Sps0.3–0.5 (Table 2; Figure 3C). The garnet in the actinolite-bearing eclogites and the garnet in the glaucophane eclogites have similar variation tendencies: from the cores to rims, an increase in pyrope and grossular and a decrease in spessartine and almandine. The compositions of garnet grains are within the type C field except for one data point, which is typical of oceanic eclogites. However, the almandine in the cores of garnets in the actinolite-bearing eclogites is lower than that in the glaucophane eclogites, while the pyrope is significantly higher.
Rare earth elements
The results of trace elements in the simple ΣREE concentrations vary from 21.65 to 900.68 ppm (Table 3). The enrichment by HREEs is relative to LREEs, as well as the generally high concentrations of REEs. Evident fractionation among REEs indicate LREE/HREE 0.007–0.13, δEu (Eu/Eu* =0.29–1.16), and δCe (Ce/Ce* =0.12–3.84). Rare earth element zonation in the garnets is stronger than major element zoning. REEs are predicted to have a maximum in the core followed by a continuous decrease toward the rim, especially HREEs. HREEs positively correlate with grossular contents. REE can be divided into three models from the core to rim (Figures 3D–F): 1) the core is characterized by steeply increasing normalized REE patterns from LREEs to HREEs. The HREE decreases toward the outer core MREE content, which remains constant in mode 1. 2) The second model is a moderately convex upward normalized REE pattern in which the HREE concentration decreases suddenly and the MREE concentration decreases slightly. 3) The REE pattern is characterized by a drastic increase in MREEs, whereas the increase in the HREE concentrations is limited. Types 1) and 2) grow in the glaucophane eclogite garnet samples, and types 1) and 3) grow in the actinolite-bearing eclogite garnet samples. Type 1) appears in the cores of the samples, and types 2) and 3) appear in the rims of the samples. The chondrite-normalized REE patterns are similar in the cores of the two kinds of garnet, while there are differences in the rims.
Discussion
Major element zonation
Major zonation patterns in the garnets are a promising way to interpret the change in pressure and temperature evolution during the metamorphic evolution of eclogite. The content of pyrope is positively correlated with temperature (Chen et al., 2005; Carswell et al., 2000). It can be observed that pyrope increased from the core to rim in all samples, which reflects the temperature rise process of glaucophane eclogites and actinolite-bearing eclogites during metamorphism. In most cases, grossular contents in prograde garnet reach a maximum in the rim, suggesting that the highest grossular content in the garnet may represent the highest pressure during subduction. It can be observed that grossular increased from the core to rim in all samples as well. This increase in grossular reflects the pressure rise (Carswell et al., 2000; Hermann and Green, 2001; Hermann, 2002). The pyrope content in the garnet rim of the actinolite-bearing eclogites is significantly higher than that of the glaucophane eclogites, which indicates that the formation temperature of actinolite-bearing eclogites is higher than that of glaucophane eclogites. Pyrope and grossular are all predicted to have a maximum temperature in the rim. This result indicates that the peak metamorphic temperature and pressure of garnet should have been achieved at the same time. The prograde zoning of the garnet reserve records the rapid exhumation of the eclogite.
Rare earth element zonation
Three types of normalized REE patterns correspond to different mineral chemical reactions in the formation of garnet. Garnet growth along the considered P-T path (Fu et al., 2021) is predicted to occur in three stages. According to petrographic observations and thermodynamic simulations (Konrad-Schmolke et al., 2008) carried out by predecessors, three mineral chemical reactions correspond to three types of normalized REE patterns. 1) Chl + Ep + Qz→ (almandine—rich) Grt + (sodic—calcic) Amp + H2O. The almandine-rich garnet cores are formed mainly by the consumption of chlorite and grow during the early stage of prograde metamorphism. After chlorite is completely consumed, the growth of garnet in the first stage is interrupted, and the garnet formed in the second stage begins to grow. In the second stage, epidote became the main garnet-forming mineral. Epidote is an important mineral for the occurrence of REEs. Epidote is an important hydrous mineral that can remain stable during peak ultrahigh-pressure (UHP) metamorphism. The dissolution of hydrous minerals is closely related to the activity of metamorphic fluid or metamorphic anatexis. The continuous decrease in the REE content from the core to the rim indicates that garnet is decreasing in the REE reservoir. This process is known as the reservoir effect. 2) (sodic—calcic) Amp + Ep→ Grt (2) (with increasing grs content) + Czo (1) + Ky + Qz + H2O. 3) The rim of sample 3 (actinolite-bearing eclogites) is the third normalized REE pattern. Garnet is formed mainly by the consumption of amphibole in the third stage. Amp + Czo (1)→ Grt (3) + Czo (2) + Qz + H2O. Type 3 appears only in garnet in actinolite-bearing eclogites. The occurrence of type 3 garnet may be related to the formation of actinolite.
According to the changes in major elements and rare earth elements from the core to the rim of the garnets, there are two growth modes of garnet. One is the continuous growth mode. During metamorphism, the main and trace elements change uniformly with changes in temperature and pressure, indicating that the geological environment changes slowly. The other is the episodic growth mode. After a metamorphic event, the geological environment of garnet suddenly changed rapidly, resulting in a sudden change in composition (Chen et al., 2003). According to the composition change characteristics from the core to the rim of the sample, sample 1 and sample 3 reflect episodic growth, that is, the core and rim have significant concentration differentiation and different types of rare earth element-normalized patterns. The main elements in sample 2 change uniformly and slowly, and there is only one rare earth distribution mode, which reflects continuous growth.
It can be seen that the growth from the core to the rim of the garnet in the glaucophane eclogites is a process of increasing temperature and pressure, and the growth ends before the peak of metamorphism. The rim of garnet in actinolite-bearing eclogites continues to grow under increasing pressure. It can be divided into three stages of garnet growth. The three growth stages of garnet can correspond to three metamorphic evolution stages. Each metamorphic stage is described as follows: the prograde stage of metamorphism (M1), the peak stage of metamorphism (M2), and the post-peak stage of metamorphism. Pressures of 20.0–23.1 Kbar (average 21.5 Kbr) and temperatures of 407–480°C (average 452°C) were obtained for eclogites at the prograde stage of metamorphism. The P-T conditions of the peak metamorphism of the eclogites were 30.0–32.7 Kbar (average 30.6 Kbr) and 617°C–658°C (average 630°C) (Fu et al., 2021).
The zoning of large ion lithophile elements, transition metal elements, and high field strength elements is non-obvious. The ion radii of different trace elements are distinct. The diffusion rate in garnet is also different, and the degree of homogenization is distinct at high temperatures.
Behavior of trace elements in garnet
At most points, a strong positive correlation exists between the contents of Ti and Ca (Figure 4A); the content of Ca increased from the core to the rim, and the content of Ti increased accordingly. Some samples have the highest Ca content at the rim, but the Ti content decreases greatly at this time. Ti in garnets is controlled by pressure and positively correlated with pressure. Combined with the analysis of petrographic characteristics and other element distributions, the content of Ti decreases when the edge reaches the peak pressure, which may be caused by the enrichment of Ti and the formation of rutile at this stage. The contents of Sr and Y elements are closely related to CaO (Figures 4B,C). Previous studies believe that the Y content in garnet is determined by CaO (Li TF et al., 2012), which is not affected by temperature and pressure. Because the ionic radius of Sr is similar to that of Ca, the lattice occupation is restricted by it (Harte and Kirkley, 1997). The CaO-Y and CaO-Sr projection maps of garnet from the Bangbing eclogite show that there is an obvious law of positive correlation between the contents. The samples with the highest Ca content at the rim and a decrease in Ti content are affected when Ti decreases. Therefore, the content of these two elements is not only controlled by CaO content but also affected by other factors, such as the formation of new minerals, which may migrate and enrich Y, Sr, and Ti from garnet to rutile together. The Si content in the composition of rutile inclusions can reflect the pressure at the time of formation. This feature is reflected from the garnet core to the mantle in the eclogite in the Bangbing area. The rim with the highest Mg content decreases, which is similar to the Ca–Ti relationship. The Si content in the composition of rutile inclusions can reflect the pressure at the time of formation. It is generally considered that more than 500 ppm is a sign of ultrahigh pressure (Zhu et al., 2017). The Si content of rutile inclusions in the sample is 291–1,480 ppm, so the eclogite in the sample may have experienced high pressure–ultrahigh pressure. It is generally believed that Ni and Mg have similar ionic radii. In garnet, the Ni content is controlled by the MgO content and affected by temperature (O’ Reilly and Griffin, 1995), which is positively correlated with temperature. This feature is reflected from the garnet core to the mantle in the eclogite in the Bangbing area. The rim with the highest Mg content decreases, which is similar to the Ca–Ti relationship (Figure 4D). The content of Zr gradually decreases from the core to the rim of garnet. Zr is mainly controlled by temperature and is positively correlated with the formation temperature of garnet (O’ Reilly S. M. and Griffin W. L. 1995), which is contrary to the heating process reflected by the main elements. The possible reason is similar to that for the change in Ti content, which is the formation of rutile, and the content of Zr in rutile is high.
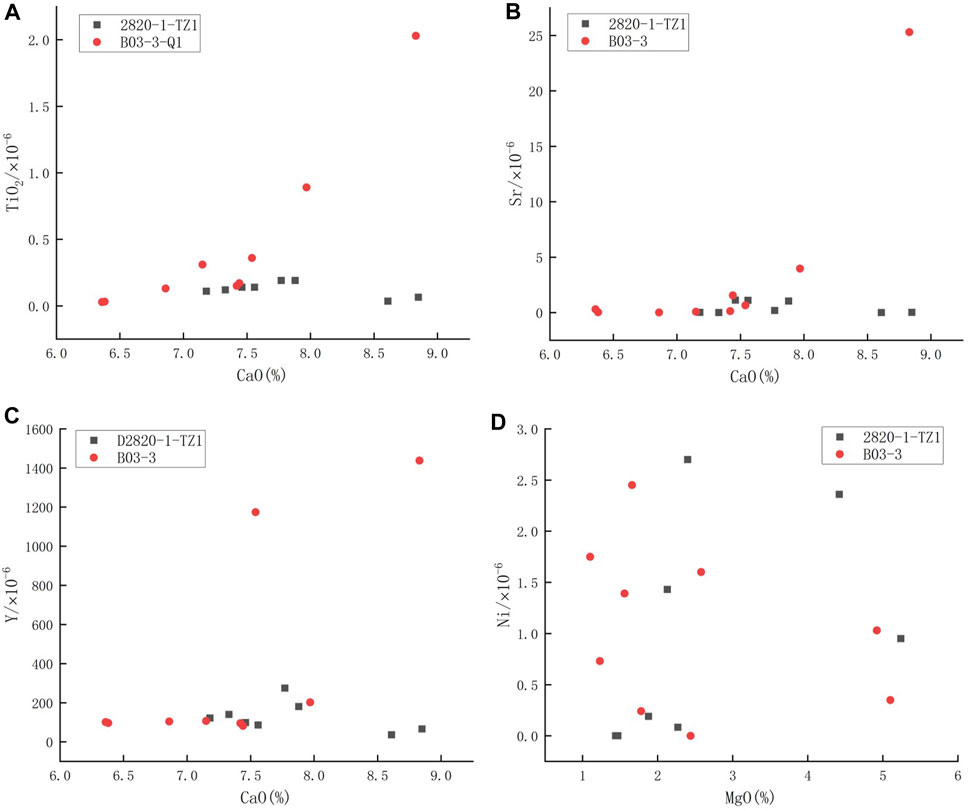
FIGURE 4. Variations between CaO and MgO and some trace elements of garnet. (A) variation of CaO-Ti; (B) variation of CaO-Sr; (C) variation of CaO-Y; and (D) variation of MgO-Ni.
Conclusion
1) The garnet from the Bangbing area is generally euhedral, with color, inclusion, and composition zoning. Major element growth zonation exhibits pyrope and grossular increasing from the core to rim, showing the prograde growth of the garnet. Three types of normalized REE patterns in garnet correspond to different mineral chemical reactions in the formation of garnet and three stages of growth.
2) The contents of pyrope and grossular reached their highest values at the same time, indicating that the eclogite in the Bangbing area peaks in temperature and pressure at the same time after subduction. The garnet is surrounded by chlorite, suggesting a depressurization process after peak metamorphism. The zonation of garnet is well preserved, suggesting a relatively short peak metamorphic time and relatively rapid exhumation process.
3) The controlling factors of the chemical behavior of trace elements in garnet are the result of many aspects. Trace elements are not only controlled by the main elements but also affected by temperature, pressure, fluid, mineral combination, and other factors.
Data availability statement
The raw data supporting the conclusion of this article will be made available by the authors, without undue reservation.
Author contributions
NL: conceptualization, formal analysis, investigation, writing—original draft, and writing—review and editing. GW: formal analysis, resources, writing—review and editing, supervision, and funding acquisition. ZP: conceptualization, resources, and funding acquisition. YF: conceptualization, formal analysis, and investigation.
Funding
This study was supported by the National Natural Science Foundation of China (92055314) and the China Geological Survey (DD 20221635).
Acknowledgments
The authors thank the Center for Global Tectonics, School of Earth Sciences, China University of Geosciences (Wuhan) for their contribution to the EMPA work and Wuhan Sample Solution Analytical Technology Co., Ltd., Wuhan, China, for their contribution to the LA-ICP-MS work.
Conflict of interest
The authors declare that the research was conducted in the absence of any commercial or financial relationships that could be construed as a potential conflict of interest.
Publisher’s note
All claims expressed in this article are solely those of the authors and do not necessarily represent those of their affiliated organizations, or those of the publisher, the editors, and the reviewers. Any product that may be evaluated in this article, or claim that may be made by its manufacturer, is not guaranteed, or endorsed by the publisher.
References
Baxter, E. F., Caddick, M. J., and Dragovic, B. (2013). Garnet: A rock-forming mineral petrochronometer. Rev. Mineral. Geochem. 83, 469–533. doi:10.2138/rmg.2017.83.15
Bebout, G. E. (2007). Metamorphic chemical geodynamics of subduction zones. Earth Planet. Sci. Lett. 260, 373–393. doi:10.1016/j.epsl.2007.05.050
Carswell, D. A. (1990). “Eclogites and the eclogite facies rocks:Definitions and classification,” in Eclogite facies rocks. Editor D. A. Carswell (Glasgow, London: Blackie & Son), 1 13.
Carswell, D. A., Wilson, R. N., and Zhai, M. (2000). Metamorphic evolution, mineral chemistry and thermobarometry of schists and orthogneisses hosting ultra-high pressure eclogites in the Dabieshan of central China. LITHOS 52 (1), 121–155. doi:10.1016/S0024-4937(99)00088-2
Chen, D. L., Sun, Y., Liu, L., Zhang, A. D., Luo, J. H., and Wang, Y. (2005). Metamorphic evolution of the yuka eclogite in the north qaidam, NW China: evidences from the compositional zonation of the garnet and reaction texture in the rock. Acta Petrol. Sin. 21 (4), 1039–1048. (in Chinese with English abstract). doi:10.3321/j.issn:1000-0569.2005.04.002
Chen, N. S., Sun, M., Yang, Y., Liu, R., and Wang, Q. Y. (2003). Major- and trace-element zoning in metamorphic gatnets and their THEIR metamorphic process implications. Earth Sci. Front. 10 (03), 315–320. (in Chinese with English abstract). doi:10.1016/S0955-2219(02)00073-0
Coleman, R., Lee, D., Beatty, L. B., and Brannock, W. W. (1965). Eclogites and eclogites: their differences and similarities. Geol. Soc. Am. Bull. 76 (5), 483–508. doi:10.1130/0016-7606(1965)76[483:EAETDA]2.0.CO
Condie, K. C., and Kröner, A. (2008). When did plate tectonics begin? Evidence from the geologic record, in Geol. Soc. Am. Editor K. C. Condie, and V. Pease 440, 281–294.
Dong, Y. S., Li, C., Shi, J. R., Zhang, X. Z., and Wang, S. Y. (2010). Forming process of the high pressure metamorphic belt in central Qiangtang Tibet. Acta Petrol. Sin. 26 (7), 2099–2105. (in Chinese with English abstract).
Fu, Y. Z., Peng, Z. M., Wang, B. D., Wang, G. Z., Hu, J. F., Guan, J. L., et al. (2021). Petrology and metamorphism of glaucophane eclogites in changning-menglian suture zone, bangbing area, southeast Tibetan plateau: A evidence for paleo-tethyan subduction. China Geol. 4 (01), 111–116. doi:10.31035/cg2021017
Hacker, B., and Gerya, T. V. (2013). Paradigms, new and old, for ultrahigh-pressure tectonism. Tectonophysics 603, 79–88. doi:10.1016/j.tecto.2013.05.026
Harte, B., and Kirkley, M. B. (1997). Partitioning of trace elements between clinopyroxene and garnet:data from mantle eclogites. Chem. Geol. 136, 1–24. doi:10.1016/S0009-2541(96)00127-1
Hermann, J., and Green, D. H. (2001). Experimentalconstraints on high pressure meltingin subducted crust. Earth Planet. Sci. Lett. 188, 149–168. doi:10.1016/s0012-821x(01)00321-1
Hermann, J. (2002). Allanite:Thorium andlight rare Earth element carrier insubducted crust. Chem. Geol. 192, 289–306. doi:10.1016/s0009-2541(02)00222-x
Kohn, M. J. (2003). Geochemical zoning in metamorphic minerals. Treatise Geochem. 3, 229–261. doi:10.1016/B0-08-043751-6/03176-5
Konrad-Schmolke, M., Zack T, P. J., O'Brien, P. J., and Jacob, D. E. (2008). Combined thermodynamic and rare Earth element modelling of garnet growth during subduction: Examples from ultrahigh-pressure eclogite of the Western Gneiss Region, Norway. Earth Planet. Sci. Lett. 272, 488–498. doi:10.1016/j.epsl.2008.05.018
Li, J., Sun, Z. B., Huang, L., Xu, G. X., Tian, S. M., Deng, R. H., et al. (2017). P-T-t path and geological significance ofretrograded eclogites from Mengku area in Western Yunnnan Province, China. Acta Petrol. Sin. 33 (7), 2285–2301. (in Chinese with English abstract).
Li, J., Sun, Z. B., Xu, G. X., Zhou, K., Zeng, W. T., Huang, L., et al. (2015). Firstly discovered garnet-amphibolite from Mengku area, shuangjiang county, western yunnan province, China. Acta Mineral. Sin. 35 (04), 421–424. (in Chinese with English abstract). doi:10.16461/j.cnki.1000-4734.2015.04.001
Liu, Y. S., Hu, Z. C., Gao, S., Günther, D., Xu, J., Gao, C. G., et al. (2008). In situ analysis of major and trace elements of anhydrous minerals by LA-ICP-MS without applying an internalstandard. Chem. Geol. 257, 34–43. doi:10.1016/j.chemgeo.2008.08.004
Ning, W. B., Fu, D., Wang, J. P., Xiao, D., Li, F. F., and Huang, B. (2019). Electron probe microanalysis of monazite and its applications to U-th-Pb dating of geological samples. J. Earth Sci. 30 (5), 952–963. doi:10.1007/s12583-019-1020-8
O' Reilly, S. M., and Griffin, W. L. (1995). Trace-element partitioning between garnet and clinopyroxene in mantle-derived pyroxenites and eclogites: P-T-X controls. Chem. Geol. 121, 105. doi:10.1016/0009-2541(94)00147-Z
Peng, Z. M., Hu, J. F., Fu, Y. Z., Zhang, J., Guan, J. L., Han, W. W., et al. (2018a). Geological map of wendong sheet (F47e005016)(1:50000), the people’s republic of China. In Press.
Peng, Z. M., Wang, G. Z., Wang, B. D., Wang, L. Q., Fu, Y. Z., Guan, J. L., et al. (2019). Discovery of glaucophane eclogites within the Lancang group in bangbing area, Western yunnan. J. Chengdu Univ. Technol. Sci. Technol. Ed. 46 (5), 639–640. (in Chinese with English abstract).
Spear, F. S., Selverstone, J., Hickmott, D., Crowley, P., and Hodges, K. V. (1984). P-T paths from garnet zoning: A new technique for deciphering tectonic processes in crystalline terranes. Geology 12 (2), 87–90. doi:10.1130/0091-7613(1984)12<87:PPFGZA>2.0.CO;2
Sun, S. S., and McDonough, W. F. (1989). “Chemical and isotopic systematic of oceanic basalt: Implications for mantle composition and processes,” in Magmatism in the ocean basins. Editors A. D. Sanders, and M. J. Norry (London, United Kingdom: Geol Soc Spec Publ), 42, 313–345.
Sun, Z. B., Hu, S. B., Zhou, K., Zhou, T. Q., Zhao, J. T., Wang, Y. X., et al. (2019). Petrology, mineralogy and metamorphic p-T path of eclogites from the Qianmai area, Lancang County, western Yunnan Province. Geol. Bull. China 38 (7), 1105–1115.
Taguchi, Tomoki, Enami, Masaki, and Kouketsu, Yui (2019). Metamorphic record of the Asemi-gawa eclogite unit in the Sanbagawa belt, Southwest Japan: Constraints from Inclusions study in garnet porphyroblasts. J. Metamorph. Geol. 37 (2), 181–201. doi:10.1111/jmg.12456
Wang, B. D., Wang, L. Q., Wang, D. B., Yin, F. G., He, J., Peng, Z. M., et al. (2018). Tectonic evolution of the changning-menglian proto-paleo Tethys Ocean in the sanjiang area, south Western China. Earth Sci. 43 (8), 2527–2550. doi:10.3799/dqkx.2018.160
Xia, Q. X. (2019). Different origins of garnet in high pressure to ultralpressure metamorphic rocks. Earth Sci. 44 (12), 4042–4049. (in Chinese with English abstract).
Xia, Q. X., and Zheng, Y. F. (2011). The composition and chemical zoning in garnet from high to ultrapressure metamorphic rocks. Acta Petrol. Sin. 27 (2), 433–450. (in Chinese with English abstract).
Xia, Q. X., Zhou, L. G., and Zheng, Y. F. (2013). Zonation and multiphase growth of garnet in UHP metamorphic rocks of continental subduciton zone. Chin. Sci. Bull. 58, 2138–2144. (in Chinese with English abstract). doi:10.1360/972013-599
Zhai, Q. G. (2008). “Petrology, Geochemistry and tectonic evolution of the eclogite from central Qiangtang area,North Tibet,” (China, Beijing: Chinese Academy of Geological Sciences). dissertation’s thesis.
Zhai, Q. G. (2013). Triassic metamorphic belt in central Qiangtang area, North Tibet. Acta Geol. Sin. 87 (S1), 19.
Zhang, X. Z., Dong, Y. S., Wang, Q., and Dan, W. (2018). High pressure metamorphic belt in central Qiangtang, Tibetan Plateau: Progressand unsolved problems. Geol. Bull. China 37 (8), 1406–1416. (in Chinese with English abstract).
Zhou, L. G., Xia, Q. X., Zheng, Y. F., and Chen, R. X. (2011). Multistage growth of garnet in ultrahigh-pressure eclogite during continental collision in the dabie orogen: constrained by trace elements and U-Pb ages. Lithos 127, 101–127. doi:10.1016/j.lithos.2011.08.010
Keywords: garnet, eclogite, zoning, HP/UHP metamorphism, Changning-Menglian suture
Citation: Lu N, Wang G, Fu Y and Peng Z (2022) Implication of garnet zoning in high pressure- ultrahighpressure eclogite from Changning-Menglian suture zone, Bangbing area, Southeast Tibetan Plateau. Front. Earth Sci. 10:930880. doi: 10.3389/feart.2022.930880
Received: 28 April 2022; Accepted: 28 July 2022;
Published: 30 August 2022.
Edited by:
Gillian Foulger, Durham University, United KingdomCopyright © 2022 Lu, Wang, Fu and Peng. This is an open-access article distributed under the terms of the Creative Commons Attribution License (CC BY). The use, distribution or reproduction in other forums is permitted, provided the original author(s) and the copyright owner(s) are credited and that the original publication in this journal is cited, in accordance with accepted academic practice. No use, distribution or reproduction is permitted which does not comply with these terms.
*Correspondence: Guozhi Wang, d2FuZ2d1b3poaTY2QDE2My5jb20=; Yuzhen Fu, ZnV5dXpoZW4xNUBjZHV0LmVkdS5jbg==