- 1Laboratory of Geochronology and Geochemistry of Isotopes, Institute of Precambrian Geology and Geochronology (Russian Academy of Sciences), St. Petersburg, Russia
- 2Department of Geochemistry, Institute of Earth Sciences, St. Petersburg State University, St. Petersburg, Russia
- 3School of Geography, Geology and the Environment, University of Leicester, Leicester, United Kingdom
1 Introduction
The territory of northeastern Mongolia (Figure 1) forms part of the Central Asian intraplate volcanic province, which formed in the Late Mesozoic—Cenozoic between the Siberian and North China platforms (Yarmolyuk et al., 1995). Within northeastern Mongolia, volcanism was most active in the first half of the Early Cretaceous (∼120 Ma), when a thick lava cover known as the Cover Volcanic Complex (CVC) occurred (Yarmolyuk et al., 2020; Kuznetsov et al., 2022). The complex consists mostly of trachybasalts, basaltic trachyandesites, trachyandesites, and trachytes (Yarmolyuk et al., 2020; Kuznetsov et al., 2022). Geochemical and isotope (Sr, Nd, Pb) data from the CVC basalts indicates that the magmatism had a lithospheric mantle source, which possibly consisted of peridotite, eclogite, and pyroxenite lithologies (Dash et al., 2015; Bars et al., 2018; Sheldrick et al., 2020; Yarmolyuk et al., 2020; Kuznetsov et al., 2022). According to Kuznetsov et al. (2022) and Bars et al. (2018), the trachyandesites formed from a basaltic melt which underwent fractional crystallization processes. However, to date, the origin of the most felsic volcanism remains unclear. For example, do the CVC trachytes reflect fractional crystallization processes with, or without, mixing of mantle- and crust-derived melts? Thus, the felsic magmatism provides the opportunity to understand mantle–crust interaction processes in Mongolia during the Early Cretaceous volcanic activation period. This study aims to evaluate the significance and extent of any continental crust input via a Sr-Nd-Pb isotope study of Early Cretaceous CVC trachytes and basement rocks.
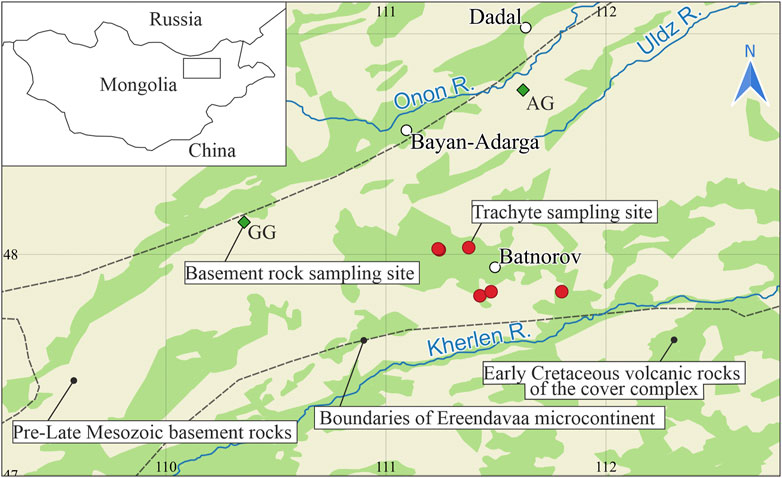
FIGURE 1. Schematic geological map of northeastern Mongolia with sampling sites. Abbreviations: AG, amphibole gneiss; GG, granitic gneiss.
2 Geological background
The formation of the CVC in northeastern Mongolia occurred in a post-collisional environment, in the Early Cretaceous, after the eruption of shoshonitic rocks (Sheldrick et al., 2020; Stupak et al., 2020; Yarmolyuk et al., 2020).
The formation of the CVC coincided with the formation of linear graben structures and horst systems with a northeastern strike (Kovalenko, 2010; Yarmolyuk et al., 2020). By ∼120 Ma, basaltic lava eruptions formed a cover of great thickness (>1,000 m) (Kovalenko, 2010; Yarmolyuk et al., 2020). This complex contains highly porous and massive glassy basalts which alternate along sections. Globular lavas and hyaloclastites are also common among the basalts, likely a consequence of eruptions occurring under lakes.
The basaltic volcanism phase ended during the Early Cretaceous (125—120 Ma) with trachytic volcanism in the form of short lava flows, large extrusions, small central volcanoes, and lava domes (Yarmolyuk et al., 2020). The trachytes predominantly occur alongside basaltic bedding, although elements of unconformable overlapping are observed occasionally. Dark gray vitrophyre horizons can be traced at the base of the felsic rocks, and agglomerate varieties predominate in their upper parts.
The volcanic rocks of northeastern Mongolia lie on a peneplainized basement composed of different-age pre-Late Mesozoic complexes. The rocks of the Ereendavaa terrane mainly form the basement of the CVC rocks. The Ereendavaa terrane is a microcontinent composed of Paleoproterozoic granite gneisses, amphibolites, schists, and marbles (Bardach et al., 2002). According to Miao et al. (2017) and Miao et al. (2020), the Precambrian rocks of Ereendavaa could have been significantly reworked by Paleozoic and Mesozoic granitic magmatism.
3 Methods
3.1 Sampling
Trachytes (5 samples) and basement rocks of the Ereendavaa microcontinent (2 samples) were sampled during a Russian-Mongolian expedition in July—August 2017. The rock sampling sites are shown in Figure 1. A list of samples with coordinates is presented in Supplementary Table S2.
The first sample of basement rock is an amphibole gneiss (AG). The sample’s chemical composition (Supplementary Table S1) is close to the average composition of lower crustal granulite xenoliths found in Mongolia’s Mesozoic—Cenozoic lava fields (Stosch et al., 1997; Barry et al., 2003; Ancuta, 2017). Sample AG has a Mesoproterozoic (∼1.6 Ga) Sm-Nd model age utilizing a depleted mantle model composition (Dickin, 2014). Thus, sample AG is likely a good compositional proxy for the continental crust of the ancient Ereendavaa microcontinent, within which the Early Cretaceous volcanic fields are located. The second sample of basement rock is a porphyritic granitic gneiss (GG), similar in composition to S-type granites (Supplementary Table S1). The formation of these granitic gneisses was associated with the accretionary stage of the Mongol-Okhotsk belt (Yarmolyuk et al., 2019). The Sm-Nd model age of this sample (∼1.3 Ga) indicates that this granite was a partial melting product of the Ereendavaa ancient crust.
3.2 Isotope analyses
Isotopic compositions (Sr, Nd, and Pb) were determined on a Triton TI (Thermo Finnigan, Germany) multicollector solid-phase mass spectrometer at the Institute of Precambrian Geology and Geochronology (Russian Academy of Sciences, St. Petersburg).
Rb, Sr, Sm, and Nd concentrations and ratios (87Rb/86Sr and 147Sm/144Nd) were determined by isotopic dilution. Chemical extraction of Rb, Sr, Sm, and Nd was performed using methods described by Savatenkov et al. (2020). Analytical errors for Rb, Sr, Sm, and Nd concentrations were calculated based on multiple analyzes of standard BCR-1 and are ±0.5%. The total laboratory blank was 0.05 ng for Rb, 0.2 ng for Sr, 0.3 ng for Sm, and 0.5 ng for Nd. Averaged results for BCR-1 standard (50 measurements) was: [Sr] = 336.7 ppm, [Rb] = 47.46 ppm, [Sm] = 6.47 ppm, [Nd] = 28.13 ppm, 87Rb/86Sr = 0.4062, 87Sr/86Sr = 0.705035 ± 5, 147Sm/144Nd = 0.1380, and 143Nd/144Nd = 0.512643 ± 3. Isotopic analysis repeatability was controlled by determining the composition of certified standards JNdi-1 (143Nd/144Nd = 0.512117) (Tanaka et al., 2000) and SRM-987 (87Sr/86Sr = 0.710240). Over the period of measurements, the resulting 87Sr/86Sr value for SRM-987 was 0.710245 ± 5 (2σ, 50 measurements), and the 143Nd/144Nd value for JNdi-1 was 0.512105 ± 3 (2σ, 50 measurements). The Sr isotopic composition was normalized to 88Sr/86Sr = 8.37521, and the Nd composition was normalized to 146Nd/144Nd = 0.7219.
Since U loss likely occurred in the Late Mesozoic rocks due to post-magmatic alteration, age corrected Pb isotope estimates might not reflect initial compositions. To minimize this risk, fresh rocks with minimal secondary alteration were selected based on petrographic observations. Loss-on-ignition values for these samples did not exceed 1.71 wt%. Next, the rock fraction of 0.25—0.5 mm was treated in 2.2 N HCl on a hot plate (60 °C) for 1 h before the decomposition stage. Mineral decomposition and extraction of U and Pb were performed utilizing the method described by Manhes et al. (1984). The total laboratory blank for Pb and U did not exceed 0.1 and 0.01 ng, respectively. Correction for fractionation for the Pb isotopic ratios was performed using a double isotope dilution technique with a235U–204Pb–207Pb tracer (Melnikov, 2005). The inaccuracies (2σ) of the 206Pb/204Pb, 207Pb/204Pb, and 208Pb/204Pb isotope ratios were determined from a series of parallel analyzes of rock standard BCR-1 (206Pb/204Pb = 18.820 ± 0.005, 207Pb/204Pb = 15.6406 ± 0.0017, 208Pb/204Pb = 38.737 ± 0.010, n = 10) and did not exceed 0.03%, 0.03%, and 0.05%, respectively.
4 Data description
4.1 Sr and Nd isotopic compositions
The results of the Rb-Sr and Sm-Nd isotope study are presented in Figure 2A, Supplementary Table S2, and Supplementary Table S3, respectively.
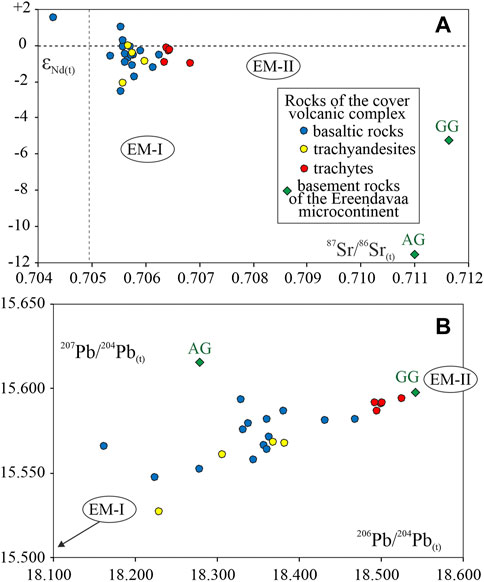
FIGURE 2. Sr, Nd (A), and Pb (B) isotope composition of the CVC rocks and basement rocks (northeastern Mongolia). The basaltic rocks and trachyandesites are from Bars et al. (2018); Kuznetsov et al. (2022); Sheldrick et al. (2020); Yarmolyuk et al. (2020). The trachytes and basement rocks of the Ereendavaa microcontinent are original data. All rocks are age corrected to 120.7 Ma (Sheldrick et al., 2020). The compositions for EM-1 and EM-2 are from Hofmann (2014).
Isotopic characteristics for the trachytes, for Sr and Nd, show minor variations. The range of values for 87Sr/86Sr is 0.707545—0.709554, and for 143Nd/144Nd is 0.512517—0.512559. Before plotting, the isotope ratios were age corrected to 120.7 Ma. This was based on an 40Ar–39Ar age obtained from the CVC trachybasalts which are associated with the trachytes (Sheldrick et al., 2020). On the diagram εNd(t)—87Sr/86Sr(t), trachytes are slightly shifted to the EM-II source composition area relative to the other rocks of the CVC (Figure 2A). The Nd isotopic composition of the trachytes does not differ from that of the basic and intermediate rocks of the CVC. The trachytes have a more radiogenic composition for Sr, though. This may indicate that assimilation processes, together with fractional crystallization, played a role in forming the trachytic melts. Nevertheless, the highly radiogenic composition of the Ereendavaa basement rocks indicates that the trachytes could not have formed only by melting of continental crust, as proposed by Yarmolyuk et al. (2020).
4.2 Pb isotopic compositions
Like the Sr isotopic compositions, the trachyte Pb isotopic characteristics show clear differences when compared to other CVC rocks. The range of values for 206Pb/204Pb is 18.603—18.653, and for 207Pb/204Pb is 15.593—15.600 (Supplementary Table S4). Utilizing a207Pb/204Pb(t)—206Pb/204Pb(t) diagram (Figure 2B), the trachytes plot closer to the EM-II source area and are shifted to the right, relative to the CVC basaltic rocks. It is also worth noting that the trachytes form a slight trend, which begins near the basaltic rocks and moves towards the composition of the granite gneiss of the Ereendavaa microcontinent. This may indicate that the trachytic melts were formed from basaltic melts, but then underwent late-stage fractional crystallization and assimilation. There may have even been some mixing between a basaltic melt with a melt derived from the ancient continental crust.
5 Conclusion
A combination of new Nd, Sr, and Pb isotope results from trachytes and basement rocks from the Ereendavaa microcontinent indicate that:
(1) The trachytes of the CVC formed from fractional crystallization and differentiation of more primitive basaltic trachyandesite—trachyandesite melts.
(2) The trachytic magma may have been contaminated by granitic crustal material. However, a further study utilizing detailed numerical modeling of assimilation processes, combined with thermodynamic modeling, is warranted to test crustal contamination processes.
Data availability statement
The original contributions presented in the study are included in the article/Supplementary Material, further inquiries can be directed to the corresponding author.
Author contributions
MK is the corresponding and the first author of this article. He wrote the first draft of the article. VS is responsible for comprehensive guidance in the research process. TS reviewed and finalized the article. MK and VS sampled volcanic and continental crust rocks during fieldwork, extracted Pb and U from rock samples, and measured the isotopic composition of samples. LS performed sample preparation for chemical decomposition and extracted Rb, Sr, Sm, and Nd from samples. All authors contributed to the article and approved the submitted version.
Funding
This research was subsidized by the Russian Science Foundation (project no. 23-27-00165).
Conflict of interest
The authors declare that the research was conducted in the absence of any commercial or financial relationships that could be construed as a potential conflict of interest.
Publisher’s note
All claims expressed in this article are solely those of the authors and do not necessarily represent those of their affiliated organizations, or those of the publisher, the editors and the reviewers. Any product that may be evaluated in this article, or claim that may be made by its manufacturer, is not guaranteed or endorsed by the publisher.
Supplementary material
The Supplementary Material for this article can be found online at: https://www.frontiersin.org/articles/10.3389/feart.2023.1156559/full#supplementary-material
References
Ancuta, L. (2017). “Toward an improved understanding of intraplate uplift and volcanism: Geochronology and geochemistry of intraplate volcanic rocks and lower-crustal xenoliths,”. doctoral thesis (Bethlehem (PA): Lehigh University).
Badarch, G., Cunningham, D., and Windley, B. (2002). A new terrane subdivision for Mongolia: Implications for the phanerozoic crustal growth of central asia. J. Asian Earth Sci. 21, 87–110. doi:10.1016/S1367-9120(02)00017-2
Barry, T., Saunders, A., Kempton, P., Windley, B., Pringle, M., Dorjnamjaa, D., et al. (2003). Petrogenesis of cenozoic basalts from Mongolia: Evidence for the role of asthenospheric versus metasomatized lithospheric mantle sources. J. Petrol. 44 (1), 55–91. doi:10.1093/petrology/44.1.55
Bars, А., Miao, L., Fochin, Z., Baatar, M., Anaad, Ch., and Togtokh, Kh. (2018). Petrogenesis and tectonic implication of the Late Mesozoic volcanic rocks in East Mongolia. Geol. J. 53 (6), 2449–2470. doi:10.1002/gj.3080
Dash, B., Yinb, A., Jiang, N., Tseveendorj, B., and Han, B. (2015). Petrology, structural setting, timing, and geochemistry of Cretaceous volcanic rocks in eastern Mongolia: Constraints on their tectonic origin. Gondwana Res. 27, 281–299. doi:10.1016/j.gr.2013.10.001
Dickin, A. P. (2014). Model ages (Sm-Nd)” in encyclopedia of scientific dating methods. Germany: Springer. doi:10.1007/978-94-007-6326-5_2-2
Hofmann, A. W. (2014). “Sampling mantle heterogeneity through oceanic basalts: Isotopes and trace elements,” in Treatise on Geochemistry (Cambrige, MA: Harvard University), 67–101. doi:10.1016/B0-08-043751-6/02123-X
Kovalenko, D. V. (2010). A comparison of Late Mesozoic and Cenozoic intraplate magmatic areas in Central Asia and paleomagnetic reconstructions of the anomalous-mantle location. Russ. Geol. Geophys. 51 (7), 774–784. doi:10.1016/j.rgg.2010.06.005
Kuznetsov, M. V., Savatenkov, V. M., Shpakovich, L. V., Yarmolyuk, V. V., and Kozlovsky, A. M. (2022). Evolution of the magmatic sources of the eastern Mongolian volcanic area: Evidence from geochemical and Sr–Nd–Pb isotope data. Petrology 30 (5), 441–461. doi:10.1134/S0869591122050034
Manhes, G., Allegre, C. J., and Provost, A. (1984). U-Th-Pb systematics of the eucrite “Juvinas”. Precise age determination and evidence for exotic lead. Geochim. Cosmochim. Acta. 148, 2247–2264. doi:10.1016/0016-7037(84)90221-7
Melnikov, N. N. (2005). Errors of double isotope dilution method in isotope analysis of lead. Geochem. Int. 43 (12), 1228–1234.
Miao, L., Zhang, F., Baatar, M., Zhu, M., and Anaad, C. (2017). SHRIMP zircon U-Pb ages and tectonic implications of igneous events in the Ereendavaa metamorphic terrane in NE Mongolia. J. Asian Earth Sci. 144, 243–260. doi:10.1016/j.jseaes.2017.03.005
Miao, L., Zhu, M., Liu, C., Baatar, M., Anaad, C., Yang, S., et al. (2020). Detrital-Zircon age spectra of neoproterozoic-paleozoic sedimentary rocks from the Ereendavaa terrane in NE Mongolia: Implications for the early-stage evolution of the Ereendavaa terrane and the mongol-okhotsk ocean. Minerals 10 (9), 742. doi:10.3390/min10090742
Savatenkov, V. M., Kozlovsky, A. M., Yarmolyuk, V. V., Rudnev, S. N., and Oyunchimeg, Ts. (2020). Pb and Nd isotopic data on granitoids from the lake zone, Mongolian and gobi altai with implications for the crustal growth of the central asian orogenic belt. Petrology 28, 403–417. doi:10.1134/S0869591120050045
Sheldrick, T., Barry, T., Millar, I., Barfod, D., Halton, A., and Smith, D. (2020). Evidence for southward subduction of the Mongol-Okhotsk oceanic plate: Implications from Mesozoic adakitic lavas from Mongolia. Gondwana Res. 79, 140–156. doi:10.1016/j.gr.2019.09.007
Stosch, H., Ionov, D., Puchtel, I., Galer, S., and Sharpouri, A. (1995). Lower crustal xenoliths from Mongolia and their bearing on the nature of the deep crust beneath central Asia. Lithos 36 (3), 227–242. doi:10.1016/0024-4937(95)00019-4
Stupak, F. M., Yarmolyuk, V. V., and Kudryashova, E. A. (2020). Late mesozoic volcanism in the ust’-kara basin (eastern transbaikalia) and its relationship with magmatism of the great xing’an and east Mongolian volcanic belts. Russ. Geol. Geophys. 61 (1), 14–25. doi:10.15372/RGG2019113
Tanaka, T., Togashi, S., Kamioka, H., Amakawa, H., Kagami, H., Hamamoto, T., et al. (2000). JNdi-1: A neodymium isotopic reference in consistency with LaJolla neodymium. Chem. Geol. 168, 279–281. doi:10.1016/S0009-2541(00)00198-4
Yarmolyuk, V. V., Kovalenko, V. I., and Ivanov, V. G. (1995). Intraplate Late Mesozoic–Cenozoic volcanic province of Asia – projection of the hot mantle field. Geotectonics 5, 41–67.
Yarmolyuk, V. V., Kozlovskiy, A. M., Savatenkov, V. M., Kudryashova, E. A., and Kuznetsov, M. V. (2020). Late mesozoic eastern Mongolia volcanic area: Structure, magmatic associations, and sources of melts. Petrology 28 (6), 563–590. doi:10.1134/S0869591120060053
Yarmolyuk, V. V., Kozlovsky, A. M., Travin, A. V., Kirnozova, T. I., Fugzan, M. M., Kozakov, I. K., et al. (2019). Duration and geodynamic nature of giant central asian batholiths: Geological and geochronological studies of the khangai batholith. Stratigr. Geol. Correl. 27 (1), 73–94. doi:10.1134/S0869593819010088
Keywords: Early Cretaceous, Sr-Nd-Pb isotopes, northeastern Mongolia, trachytes, intraplate, cover volcanic complex
Citation: Kuznetsov MV, Savatenkov VM, Sheldrick TC and Shpakovich LV (2023) Early Cretaceous trachytes and basement rocks from northeastern Mongolia: a Sr-Nd-Pb isotope study. Front. Earth Sci. 11:1156559. doi: 10.3389/feart.2023.1156559
Received: 01 February 2023; Accepted: 25 May 2023;
Published: 02 June 2023.
Edited by:
Hossein Azizi, University of Kurdistan, IranReviewed by:
Mathieu Benoit, UMR5563 Géosciences Environnement Toulouse (GET), FranceMichel Grégoire, UMR5563 Géosciences Environnement Toulouse (GET), France
Copyright © 2023 Kuznetsov, Savatenkov, Sheldrick and Shpakovich. This is an open-access article distributed under the terms of the Creative Commons Attribution License (CC BY). The use, distribution or reproduction in other forums is permitted, provided the original author(s) and the copyright owner(s) are credited and that the original publication in this journal is cited, in accordance with accepted academic practice. No use, distribution or reproduction is permitted which does not comply with these terms.
*Correspondence: Maksim V. Kuznetsov, Z2FybmV0ZWNsb2dpdGVAZ21haWwuY29t