- 1Jilin Earthquake Agency, Jilin Changbaishan Volcano National Observation and Research Station, Changchun, China
- 2Institute of Volcanology, China Earthquake Administration, Changchun, China
- 3Ulanhot Earthquake Monitoring Center Station, Ulanhot, China
The latest research results show that there is a unified magma system and heating channel beneath the Arxan volcanic field, indicating a potential risk of eruption. The Arxan volcanic field features multiple gas emission sites (e.g., Jinjianggou hot springs and Yinjianggou hot springs) and exhibits strong hydrothermal activity. In this study, measurements of the hot spring gas composition and soil CO2 flux in the Arxan Holocene volcanic field were conducted, and the results were combined with previous research results to analyze the degassing characteristics of this region. The results show that the volcanic gases in the Arxan volcanic field are composed of 0.07%–1.09% CO2, 0.33–12 ppm CH4, 1.57–53 ppm H2, 800–30,241 ppm He, and 1.14%–1.86% Ar. The He content in this area is notably higher than that in other dormant volcanoes in China. This difference is possibly caused by U–Th decay in the Mesozoic granodiorite and acidic volcanic rocks in the study area, which can produce substantial radiogenic He. The soil gas concentrations near the Jinjianggou and Yinjianggou hot springs are higher than those of two Holocene volcanoes. The peak CO2 concentration in the soil near the Jinjianggou hot spring can reach 35,161 ppm. The single-site soil microseepage CO2 flux in the Arxan volcanic field is 4.66–107.18 g m−2 d−1, and the estimated annual CO2 emission flux from the volcanic field to the atmosphere is 0.63 × 105 t, which also demonstrates that soil CO2 flux of Arxan volcano is comparable to the soil CO2 emission level of the Iwojima volcano.
1 Introduction
Due to the existence of underground high-temperature magma chambers in active volcanic fields, a variety of geothermal fluids quickly escape to the surface along volcanic channels or faults, where they are emitted through hot springs, vents and soils. During the volcanic eruption intervals, large amounts of volcanic gases are emitted to the atmosphere because of the continuous heating of the country rock caused by the high-temperature chambers (Guo et al., 2015; Aiuppa et al., 2015; Tassi et al., 2016; Liu et al., 2021). It has been demonstrated that 14 volcanoes in mainland China erupted during the Holocene (wei et al., 1998; Wei et al., 2003; Pan et al., 2021) and are currently in a dormant state. Among the current dormant volcanoes in China, the Changbaishan, Tengchong, and Wudalianchi volcanoes have been investigated by numerous studies to determine their fluid backgrounds (Du et al., 1999; Shangguan et al., 2008; Zhang et al., 2011; 2015 ; 2016 ; 2018 ; Liu et al., 2011; Guo et al., 2015; Li, 2015; Zhao, 2012; Wei et al., 2021; Zhao et al., 2019). Some studies on the geothermal fluids in the Arxan volcano have been conducted, but studies focusing on the volcanic greenhouse gas emissions in this area are scarce. Therefore, to ascertain the components and emission levels of hot spring gases, this study investigates the CO2 emissions in the Arxan volcanic field and analyzes the emission characteristics of greenhouse gases in dormant volcanoes. This study provides significant information for deep magmatism investigations and volcano monitoring in the future.
A vast majority of previous investigations on the Arxan volcano focus on the volcanic geology, geophysical exploration, and geothermal heat in the peripheral area of the volcanic field (Liu, 1987; Bai et al., 2005; Tang, 2005; Zhao and Fan, 2010; Han et al., 2018; Gu, 2018; Cui et al., 2022; Li et al., 2023). The latest research illustrates that the Arxan volcanic cluster consists of 46 volcanoes ranging from Pliocene to Holocene in age, including four active volcanoes that have erupted during the Holocene, namely, the Gaoshan volcanoes, Yanshan volcanoes, Shihaogou basin volcanoes, and Xiaodonggou volcanoes. Among them, Gaoshan and Yanshan volcanoes are considered to be active volcanoes that erupted approximately 2,000 years ago (Bai et al., 2005). In recent years, some magnetotelluric sounding observations have shown that the region 10–12 km below the active volcanoes retains a high thermal state, and a mantle upwelling channel conducting heat may exist in the region at a depth of 30–50 km (Tang et al., 2005). Han et al. (2018) reports that the Arxan magma system is uniformly composed of arch bridge-shaped magma transportation channels and basaltic magma from the asthenosphere. In addition, two high-conductivity anomalies have been discovered, with burial depths from 40 to 90 km. Recent studies on fluid geochemistry in Arxan show that the excess N2, Ar, He and CO2 in hot springs are mainly from the crust and upper mantle, and approximately 3%–23% of the He in crater lake water and bubble gas in hot springs comes from the mantle (Cui et al., 2022).
The purpose of this study is to analyze the degassing characteristics and emission mechanisms of greenhouse gases in the Arxan volcano through the investigation of the relevant gas components, the concentrations of volcanic gas, and the emissions of soil gases. Moreover, this study aims to explore the emission characteristics of volcanic greenhouse gases during the dormant period and compare the results to those of other dormant volcanoes.
2 Geological setting
The Arxan volcanic cluster is located in the eastern section of the China–Mongolia border. The geographical coordinates are 120°14′–121°20′E, 47°15′–47°45′N. The volcanoes in this cluster are distributed in the upper reaches of the Halaha River in the southwest of the Greater Khingan Mountains situated in the back-arc region of the subducting western Pacific plate. Since the early Jurassic, affected by the subduction of the Pacific plate and the blocking of the Eurasian plate, a series of NE-oriented faults and volcanic rock belts with different scales have developed. Since the start of the Cenozoic, extensive basaltic magma has violently erupted (Liu, 1987; Liu et al., 2001; Pan et al., 2021). The volcanism in this region is multi-episodic and can be divided into the Pliocene, Pleistocene and Holocene eruption stages. The volcanic products overlie the Jurassic volcanic–intrusive rocks, with an overall NE trending distribution and an exposed area of approximately 1,300 km2. The Pliocene series is composed of tholeiite, and the Quaternary rocks are mainly alkaline olivine basalt.
Faults with different directions are developed in the study area (Figure 1). The NE trending Halaha River fault cuts the lithosphere and has a length of approximately 500 km. Furthermore, it provides two channels transporting Quaternary magma from the mantle: one features a high-temperature and fluid-rich mass at a depth of 10–12 km, and the other features a low-temperature mass at a depth of 30 km (Tang et al., 2005). According to previous research (Cui et al., 2022), the hot springs exhibiting fluid derived from a deep mantle source include the Jinjianggou hot spring, Yinjianggou hot spring, Budonghe River, and the two volcanoes active in the Holocene.
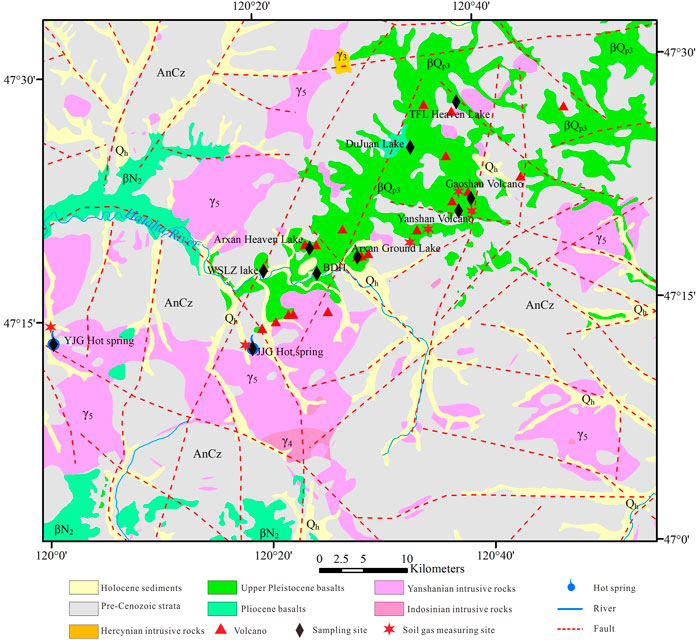
FIGURE 1. Geological map and sampling site locations in the Arxan active volcanic field (modified from 1:250000 geological structure map of Chaihe sheet).
3 Sampling and analytical techniques
The hot spring gas samples were collected by the drainage method. At the beginning of our research, the gas-collecting hood was placed in a bubble-free area of hot spring water, and water was filled into a 100 ml syringe to discharge the air inside. Then, the funnel was moved underwater to the bubbling area, and the water in the gas path was drained by the pressure of the hot spring gas itself and the syringe. The collected hot spring gases were subsequently injected into a vacuumized aluminum foil gas sample bag and an inverted water-sealed glass bottle. The gas components were analyzed using a gas chromatograph in the Key Laboratory of Earthquake Prediction, Institute of Earthquake Forecasting, China Earthquake Administration. A total of 11 gas samples were collected from the Jinjianggou hot spring(JJG), Yinjianggou hot spring(YJG), Budonghe river(BDH) and Arxan Tianchi Lake(TC). The locations of the sampling sites are shown in Figure 1.
Soil gas observation in the volcanic field was conducted using an EDK6900-X-type portable greenhouse gas analyzer, which can measure the concentrations of CO2 and CH4 with a range of 0–100,000 ppm and a measurement accuracy of less than ±1%. The instrument completes an air inflow observation every 3 min. Before the measurements, a pit with a depth of approximately 50 cm and a diameter of approximately 40 cm was dug in the surface soil layer. Then, the aluminum gas collector with a diameter of 35 cm and a height of 50 cm was buried in the pit, and the soil was backfilled to seal the gas collector (Figure 2). Then, the air inlet of the instrument was connected with the air inlet and outlet of the gas collecting hood, forming a closed loop. The instrument software can display the test results in real-time. After the real-time test data stabilized, the soil gas at this site was considered to have reached equilibrium. This study observed the soil gas of Gaoshan volcano, Yanshan volcano, and the Jinjianggou and Yinjianggou hot springs. The sample collection and field observations were conducted in August 2022.
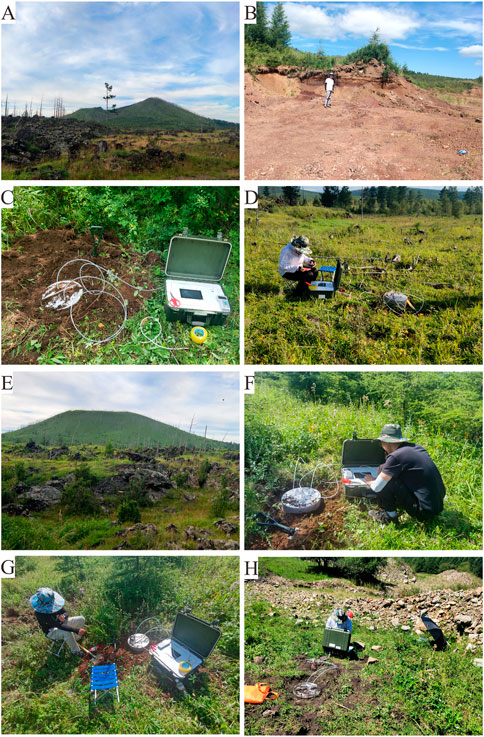
FIGURE 2. Soil gas observation in the field [(A) Gaoshan volcanic landscape; (B) Gaoshan volcanic pyroclastic flow; (C) Gaoshan volcanic cone measurement site; (D) Gaoshan volcanic periphery measurement site; (E) Yanshan volcanic landscape; (F) Yanshan volcanic cone measurement site; (G) Yanshan volcanic periphery measurement site; (H) Jinjianggou hot spring measurement site].
The field work of this study mainly includes soil CO2 and CH4 gas observations (measured with a EDK6900-X portable greenhouse gas analyzer) in Gaoshan volcano, Yanshan volcano, and the Jinjianggou and Yinjianggou hot springs in the Arxan volcanic field. CO2 is one of the primary components of volcanic gases, and the results are easy to compare with those of other volcanoes. The field work of this study was carried out during sunny days in July and August. During the observations, the atmospheric temperature was approximately 29°C, the atmospheric pressure was approximately 88 kPa, and the soil moisture content was 5%. For the soil gas observations in the hot spring areas, we selected regions with loose soil and soil thicknesses greater than 80 cm. For the volcanic soil gas observations, the volcanic cone and the pyroclastic-covered peripheral area were selected. This field work was conducted based on previous studies and geological surveys. In the measurement process, we selected areas far from ponds, rivers, and regions with dense vegetation and human activities. Faults are highly developed in the study area, and the formation of hot springs is the result of the interaction between groundwater and faults. Some appropriate soil gas observation sites near the Jinjianggou and Yinjianggou hot springs were selected to determine the soil gas emission characteristics in the fault zone. Additionally, two sites on the cone and peripheral area of the two Holocene volcanoes (i.e., the Gaoshan and Yanshan volcanoes) were selected to show the level of soil gas emission in the volcanic field.
4 Results
4.1 Volcanic gas composition and content
The upward migration of magmatic gases is one of the most significant observable factors preceding volcanic eruptions. Due to the early and rapid upward gas migration, eruptions can be predicted earlier. Therefore, most volcanic monitoring studies focus on the gas geochemistry in dormant volcanic fields. In this study, we tested the gas components in Jinjianggou and Yinjianggou hot springs, Budonghe river and Tianchi volcanic field. The gas composition of the Arxan volcanic field is shown in Table 1, and previous observation data were also collected.
We analyzed the O2/N2 ratio (air = 0.268, Holland, 1987) of the hot spring gas components in the Arxan volcanic field. It can be found that the O2/N2 ratios of the samples JJG-1 and JJG-2 are close to that of the air. The Jinjianggou river, Budong river, and Arxan Tianchi lake samples are all surface water, which may be seriously contaminated by the atmosphere. The hot spring gases (Jinjianggou and Yinjianggou hot springs and Budonghe river) in the Arxan volcanic field are mainly composed of N2 (Figures 3, 4), accounting for 86.2%–97.2%. For volcanic gases, the CO2 content is low (0.07%–1.09%), while the He (800 × 10−6–30241 × 10−6) and Ar (1.14%–1.86%) contents are relatively high (Table 1). The ratios of He–CO2–N2 (Figure 3) in the triangular diagram show that the hot spring and surface water gases feature different components and that the hot spring gases are mainly enriched in N2. Specifically, the CO2/N2 ratio of the Jinjianggou hot spring gases is 0.001–0.004, while that of the Yinjianggou hot spring gases is 0.005–0.01. The Yinjianggou hot spring gases are more enriched in CO2 than the Jinjianggou hot spring gases. In addition, the hot spring gases in the volcanic field are generally enriched in N2, similar to the surface water in the Jinjianggou River, Arxan Tianchi and Dichi (DC) (Figure 5). The N2/Ar ratio (67–84) of the volcanic gases is close to that of the air (83.6, Hilton, 1996), but the O2 content (1.45%–5.57%) is low, and the 4He/20Ne ratio (152–384) is much higher than that of the air (0.32, Magro et al., 2013). The surface water in the Arxan volcanic field is characterized by high N2 contents (95.55%), low CO2 contents (0.34%) and low He contents (6%–9%), which is different from the hot spring samples.
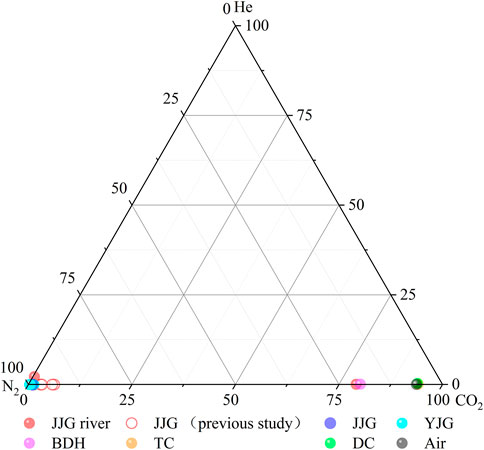
FIGURE 3. CO2–N2–He diagram comparing the hot spring and surface water gas compositions. Data sources are shown in Table 1. Filled and open symbols represent data from this study and published data, respectively.
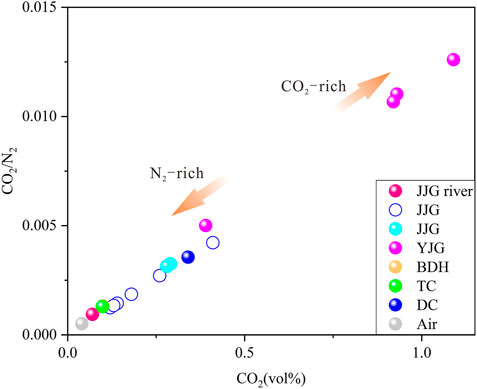
FIGURE 4. CO2/N2 vs. CO2 (vol%) for air, hot spring gases, and surface water gases in the Arxan volcanic field. Data sources are shown in Table 1. Filled and open symbols represent data from this study and published data, respectively.
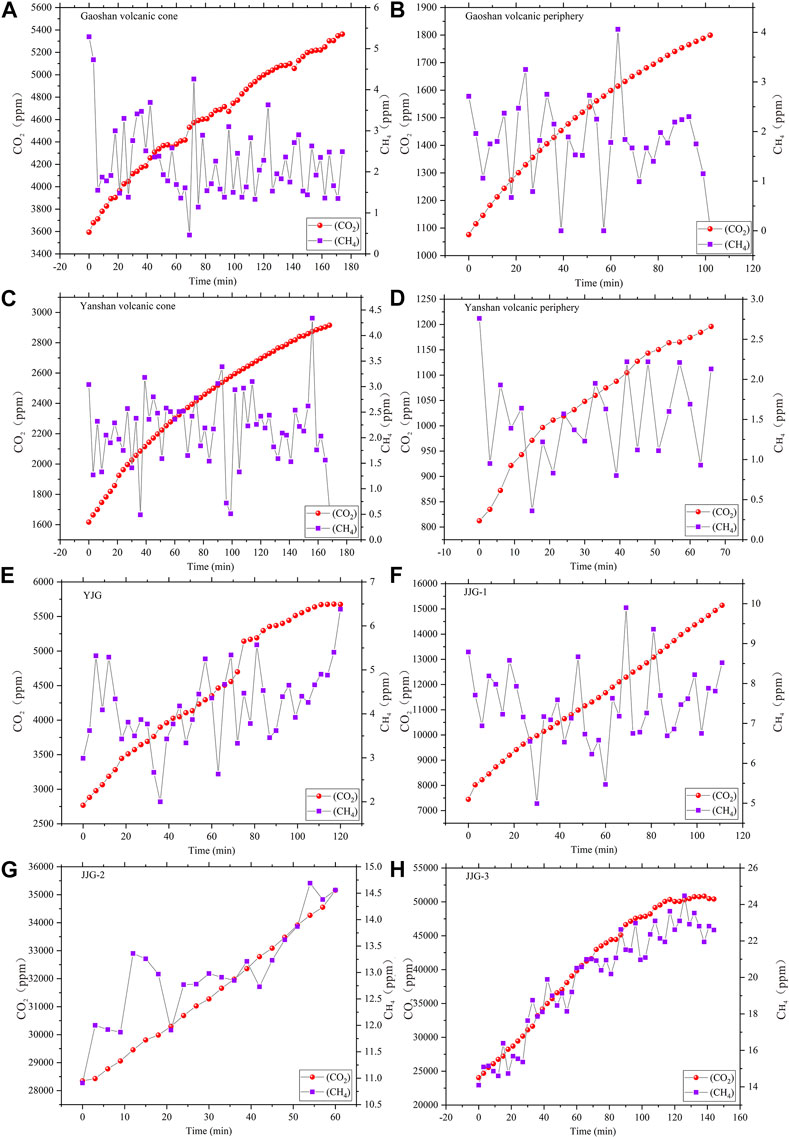
FIGURE 5. The measured curve of soil gas concentration in the volcanic field [(A) Gaoshan volcanic cone, (B) Gaoshan volcanic periphery, (C) Yanshan volcanic cone, (D) Yanshan volcanic periphery, (E) Yinjianggou hot spring, (F) Jinjianggou hot spring-1, (G) Jinjianggou hot spring-2, (H) Jinjianggou hot spring-3].
Previous investigations into the isotopic compositions of hot spring gases in the Arxan volcanic field have demonstrated that the 3He/4He ratio of the Arxan volcanic gas is low, ranging from 0.20 to 0.92 RA (Table 1). This value is higher than that of the crustal source (0.02 RA, Ballentine et al., 2002) but significantly lower than that of gas from the upper mantle or lithospheric mantle. This pattern suggests that the crustal source is the major contributor (Zhao et al., 2021). The 4He/20Ne ratio of surface water in the Arxan volcanic field is close to the atmospheric value (0.32, Ozima and Podosek, 2002), while the 4He/20Ne ratio of hot spring water is much higher than the atmospheric value. The δ13C ranges from −22.3‰ to −6.2‰, indicating that the CO2 in the hot springs may come from the mantle or be associated with biogenic and metamorphic gases in the crust (Cui et al., 2022). The excess N2, Ar, He, and CO2 in hot springs in the study area are mainly from the crust and upper mantle, and approximately 12%–63% of the He in the hot spring gas comes from the mantle (Cui et al., 2022). The CO2 content of the Arxan volcanic field is lower than that of the Changbaishan, Wudalianchi, and Tengchong volcanoes in China, where the CO2 contents are higher than 90%. The CH4 concentration of the Arxan volcanic field is slightly higher than that of the Changbaishan volcano (1,900–4,300 ppm) but lower than that of the Tengchong volcano (300–16,900 ppm); the H2 concentration is close to that of the Changbaishan volcano (1.1–29.2 ppm). However, the He concentrations in the gas from the Jinjianggou and Yinjianggou hot springs are prominently higher than those of the Changbaishan (7.6–53 ppm), Wudalianchi (10–330 ppm) (Gao and Li, 1999), and Tengchong (10–380 ppm) volcanoes (Wei et al., 2016).
4.2 Gas flux measurement
The curve of soil gas concentration measured in the field is shown in Figure 5. The accumulation chamber methodology (Figure 2) was employed in the gas flux observation to measure the diffusive emission of CO2 in the soil (Chiodini et al., 1998; Mazot et al., 2009; Mazot et al., 2011; Pérez et al., 2011). According to the methods described in Mazot et al. (2009) and Mazot et al. (2015), the soil temperature, atmospheric pressure and water temperature were measured during the field work. Moreover, we calibrated the instrument based on the atmospheric concentration before measurement. The gas diffusing through the soil accumulates and circulates in the closed-circuit system, migrating from the soil to the observation instrument and then returning to the collecting hood through the connecting tube. The soil CO2 flux was calculated using the following formula (Sun et al., 2018):
where T0 and P0 are the temperature and atmospheric pressure under standard temperature and pressure, respectively. Ts and Ps denote the soil temperature (°C) and atmospheric pressure (kPa) of each measurement site, respectively. dc/dt represents the concentration change rate for each measurement site.
The single-site soil microseepage CO2 fluxes in the Arxan volcanic field range from 4.66 to 107.18 g m−2 d−1, as shown in Table 2. Previous studies have shown that soil microseepage CO2 flux data in the range of 0.2–10,000 g m−2 d−1 are credible when adopting the closed-chamber method (Chiodini et al., 1998; Guo et al., 2014). Thus, the observed data in this study conform to the CO2 emission level of soil microseepage in most quiescent volcanoes. The CO2 fluxes in the Jinjianggou and Yinjianggou hot springs are high, whereas the soil CO2 fluxes in the two Holocene active volcanic non-volcanic fields are 4–13 times lower than those of the hot springs. Figure 1 shows that the faults of the entire Arxan volcanic field are highly developed, and the hot springs and active volcanic non-volcanic fields are all dissected or partially dissected by faults, providing channel for the release of deeply sourced gases.
Vegetation may have an effect on soil CO2 emissions, and the soil gas measurement sites of Gaoshan and Yanshan volcanoes had more vegetation cover, such as weeds and shrubs. We reviewed some relevant studies on CO2 fluxes emitted from non-volcanic soil vegetation. Norman et al. (1992) measured CO2 fluxes from a large number of vegetated soils in Wisconsin and obtained an average CO2 flux of 0.6 g m−2 d−1 (Norman et al., 1992). Mäki et al. (2019) conducted a soil CO2 flux study in a coniferous forest at Hyytiälä (Juupajoki, Finland) and observed fluxes of 0.72–5.04 μg m−2 d−1 (Mäki et al., 2019). Other scholars have performed a study on CO2 fluxes from natural vegetation in the Xilin River basin in the grasslands of Inner Mongolia, China, which has a similar geological background and climatic environment to the study area. Thus, our data can be compared to the CO2 emissions from natural vegetation in non-volcanic fields. That study found that the natural vegetation in the grasslands of the Xilin River basin in Inner Mongolia is associated with a CO2 flux of 2.4–12 g m−2 d−1 (Ma, 2006). We can see that the CO2 fluxes of natural vegetation in non-volcanic fields are all relatively low, and the CO2 fluxes near the Jinjianggou and Yinjianggou hot springs are markedly higher than those released by vegetation in the above non-volcanic fields. However, the CO2 fluxes in the Arxan and Yanshan volcanic fields are close to the levels released by natural vegetation in the grasslands of the Xilin River basin in Inner Mongolia. For the above reasons, it is likely that the surface faults of Gaoshan and Yanshan volcanoes are not well developed, and deep gases do not easily pass through the volcanic cones to reach the surface directly.
5 Discussion
We identified some unique characteristics of the hot spring gases in our study area during the sampling and observation process. The hot spring gas in the Arxan volcanic field is characterized by abnormally high He concentrations. The faults in the study area are highly developed, especially the NNE and NE oriented ones. Tang et al. (2005) observed two deep faults that acted as channels for the upward migration of magma from the mantle. One fault shows high temperatures and fluid enrichment at a depth of 10–12 km, and the other shows low temperatures at a depth of 30 km. The He content in the study area is significantly higher than that in other dormant volcanoes in China. According to previous studies on the petrological and geochemical characteristics of the volcanic rocks in the Yinjianggou of the Arxan volcanic field, the most remarkable feature is the anomalously strong enrichment of U in the regional volcanic rocks (the U content reaches 4.27–7.82 ug/g), which may be associated with the evolution of crust-derived magma (Wu et al., 2010). Thus, we speculate that the high He content is likely caused by the decay of U and Th in the Mesozoic granodiorite and acidic volcanic rocks.
Furthermore, the soil CO2 concentrations near the Jinjianggou and Yinjianggou hot springs are much higher than those in the Holocene volcanic field. The results of previous magnetotelluric and seismic surveys (Han et al., 2018; Li et al., 2023) have shown that NE and NNE trending faults are located in the vicinity of the two hot springs and may act as underlying magma channels. Therefore, the soil CO2 and the gases present in the two springs are remarkably higher in concentration than those in other regions. In contrast, the soil CO2 and CH4 concentrations in the Holocene Gaoshan and Yanshan volcanoes are lower than those in the Jinjianggou and Yinjianggou hot springs. These lower concentrations of volcanic gases at the surface may be due to the seal formed by lava flows generated by the eruption 2,000 years ago. The geological surveys of the volcanic cone and adjacent areas have not observed any obvious fault exposure.
Compared with the monitoring of erupting volcanoes, it is equally important to investigate the characteristics of gas release from dormant ones. The volcanic degassing trend is related to the type of volcanic gases. Previous studies have proposed that in response to the rise of magma, volcanic gases preferentially exsolve in the order of CO2, S, H2, HCl and HF (Werner et al., 2013). The analysis of the Arxan volcanic hot springs shows that the gas composition of the volcanic non-volcanic field is characterized by high N2, He, and Ar contents and low CO2 contents. In the hot spring gases, the δ15N value ranges from 1.3‰–1.9‰, and the N2/He ratio ranges from 303 to 663. The results suggest the existence of a high proportion of N2-rich organic matter (Zhao et al., 2021). Additionally, both the 3He/4He and CO2/3He ratios of the hot spring gas samples in the study area are low and differ from the patterns of the mantle endmember (high 3He/4He, low CO2/3He) and subduction recycling endmember (low 3He/4He, high CO2/3He). Furthermore, these values are far lower than the values of the volcanic and geothermal gases in volcanic arcs and mid-ocean ridges (Sano and Marty, 1995). The He concentrations in hot spring gases in the Arxan volcanic field (1,457 × 10−6 ∼ 30,241 × 10−6) are much higher than the atmospheric value (5.24 × 10−6), indicating a deep origin, i.e., a crustal and/or mantle source.
Several active volcanoes with the same tectonic background are compared to the Arxan volcanic field. Arxan, Changbaishan and Wudalianchi are all intraplate volcanoes, whereas Tengchong, Mount St. Helens and White Island are all subduction-related volcanoes. Additionally, Arxan, Changbaishan, Mount St. Helens, and Yellowstone are all currently dormant, while the White Island volcano has been in a slightly active state (2019 eruption, VEI = 2). Due to the large difference in the area of each volcanic field, it is not reasonable to directly compare the annual total flux of volcanic CO2. Therefore, the annual CO2 flux of each volcanic field was divided by the corresponding area in this study. According to Table 3, it can be seen that the soil CO2 flux of the Arxan volcano is approximately 20 times lower than that of the Mount St. Helens and Yellowstone volcanoes and approximately 2 times lower than that of the White Island volcano in New Zealand during the eruption stage. Iwo Jima volcano has erupted intermittently since 1922, with the most recent eruption occurring in July–August 2022. The historical eruption VEI maximum of one also indicates that soil CO2 fluxes from Alsan volcano are comparable to soil CO2 emission levels from Iwo Jima volcano, which is active and has erupted frequently in recent years (e.g., 2001, 2020, 2021 and 2022).
6 Conclusion
Based on the observation of soil gases and the composition of the gases escaping from hot springs, we analyzed the degassing characteristics of the Arxan volcanic field. The major conclusions include the following:
(1) In the studied hot springs in the Arxan volcanic field, the concentrations of CO2, CH4, H2, He, and Ar are 0.07%–1.09%, 0.33–12 ppm, 1.57–53 ppm, 800–30,241 ppm, and 1.14%–1.86%, respectively. The notably high He content is likely due to U–Th decay in the Mesozoic granodiorite and acidic volcanic rocks in the study area.
(2) The soil gas concentrations near the Jinjianggou and Yinjianggou hot springs are higher than those near two Holocene volcanoes. The peak CO2 concentration in the soil near the Jinjianggou hot spring can reach 35,161 ppm. The single-site soil microseepage CO2 flux in the Arxan volcanic field is 4.66–107.18 gm−2 d−1, and the estimated annual CO2 emission flux from the volcanic non-volcanic field to the atmosphere is 0.63 × 105 t, which also demonstrates that soil CO2 flux of Arxan volcano is comparable to the soil CO2 emission level of the Iwojima volcano.
Data availability statement
The original contributions presented in the study are included in the article/Supplementary Material, further inquiries can be directed to the corresponding author.
Author contributions
XP, GG, DH, BB, and SG conducted the field survey. XP and YS processed the data and prepared the first draft. All authors contributed to the article and approved the submitted version.
Funding
This work was supported by the Science for Earthquake Resilience (XH23013B), Jilin Scientific and Technological Development Program-Key technologies for early warning of volcanic eruption in Changbaishan Tianchi based on multi-source volcano monitoring (20230203132SF), Chinese Academy of Engineering and Local Cooperation Strategic Consulting Project (JL2021-03-01), and the National Observation and Research Station, Institute of Geology, China Earthquake Administration (NORSCBS21-04, NORSCBS21-07, and NORSCBS22-07).
Acknowledgments
We are grateful to Baojin Jia from the Arxan Volcano Monitoring Station for his support during the field investigation and to Jianguo Du and Yueju Cui from the Institute of Earthquake Forecasting, China Earthquake Administration, for their helpful suggestions.
Conflict of interest
The authors declare that the research was conducted in the absence of any commercial or financial relationships that could be construed as a potential conflict of interest.
Publisher’s note
All claims expressed in this article are solely those of the authors and do not necessarily represent those of their affiliated organizations, or those of the publisher, the editors and the reviewers. Any product that may be evaluated in this article, or claim that may be made by its manufacturer, is not guaranteed or endorsed by the publisher.
References
Aiuppa, A., Bani, P., Moussallam, Y., Napoli, R. D., Allard, P., Gunawan, H., et al. (2015). First determination of magma-derived gas emissions from bromo volcano, eastern java (Indonesia). J. Volcanol. Geotherm. Res. 304 (OCT.1), 206–213. doi:10.1016/j.jvolgeores.2015.09.008
Bai, Z., Tian, M., Wu, F., Xu, D., and Li, T. (2005). Yanshan, gaoshan-two active volcanos of the volcanic cluster in arshan, inner Mongolia. Earthq. Res. China 21 (1), 5. (In Chinese with English abstract).
Ballentine, C. J., Burgess, R., and Marty, B. (2002). Tracing fluid origin, transport and interaction in the crust. Rev. Mineral. Geochem. 47, 539–614. doi:10.2138/rmg.2002.47.13
Cheng, Z., Guo, Z., Zhang, M., and Zhang, L. (2014). Carbon dioxide emissions from Tengchong cenozoic volcanic field.Yunnan province,SW China. Acta Petrol. Sin. 30 (12), 3657–3670. (In Chinese with English abstract).
Chiodini, G., Cioni, R., Guidi, M., Raco, B., and Marini, L. (1998). Soil CO2 flux measurements in volcanic and geothermal areas. Appl. Geochem. 13 (5), 543–552. doi:10.1016/S0883-2927(97)00076-0
Cui, Y., Sun, F., Liu, L., Xie, C., Li, J., Chen, Z., et al. (2023). Contribution of deep-earth fluids to the geothermal system: A case study in the arxan volcanic region, northeastern China. Front. Earth Sci. 10, 996583. doi:10.3389/feart.2022.996583
Du, J., Li, S., Zhao, Y., Ren, J., Sun, R., and Duanmu, H. (1999). Geochemicalcharacteristics of gases from the volcanic area Wudalianchi, Northeastern China. Acta Geol. Sin. 73 (2), 103–107. doi:10.1111/j.1755-6724.1999.tb00830.x
Gao, Q., and Li, N. (1999). A discussion on fluid geochemistry and origin of the Tengchong and Wudalianchi volcanic areas. Geol. Rev. (04), 345–351. (In Chinese with English abstract).
Gu, X. (2018). Geochemical characteristics and evolution mechanism of thermal and mineral springs in Arxan. north eastern Beijing China: China University of Geosciences. (In Chinese with English abstract).dissertation
Guo, Z., Zhang, M., Cheng, Z., Zhang, L., and Lu, J. (2014). Fluxes and Genesis of greenhouse gases emissions fromtypical volcanic fields in China. Acta Petrol. Sin. 30 (11), 3467–3480. (In Chinese with English abstract).
Guo, Z., Zhang, M., Sun, Y., Cheng, Z., Zhang, L., and Liu, J. (2015). Research advances in greenhouse gases degassingfrom cenozoic volcanic active fields. J.Bulletin Mineralogy, Petrology Geochem. 34 (4), 690–700. (In Chinese with English abstract). doi:10.3969/i.issn.1007-2802.2015.04.003
Han, J., Wang, T., Liu, W., Liu, G., Han, S., and Liu, L. (2018). Deep“arch-bridge”magmatic system of the Aershan volcanic group and its stability analysis. J. Seismol. And Egology. 40 (3), 21. (In Chinese with English abstract). doi:10.3969/jissn.0253-4967.2018.03.007
Harris, D. M., and Rose, W. I. (1996). Dynamics of carbon dioxide emissions, crystallization, and magma ascent: Hypotheses, theory, and applications to volcano monitoring at Mount St. Helens. Bull. Volcanol. 58, 163–174. doi:10.1007/s004450050133
Hilton, D. R. (1996). The helium and carbon isotope systematics of a continental geothermal system: Results from monitoring studies at long valley caldera (California, USA). Chem. Geol. 127 (4), 269–295. doi:10.1016/0009-2541(95)00134-4
Holland, P. W., and Emerson, D. E. (1987). A determination of the helium 4 content of near-surface atmospheric air within the continental United States. J. Geophys. Res. Solid Earth 92 (B12), 12557–12566. doi:10.1029/jb092ib12p12557
Li, J., Tian, Y., Zhao, D., Yan, D., Li, Z., and Li, H. (2023). Magmatic system and seismicity of the Arxan volcanic group in Northeast China. Geophys. Res. Lett. 50 (6), e2022GL101105. doi:10.1029/2022GL101105
Li, T., Liu, J., Wang, X., Guo, Z., Guo, W., Cheng, Z., et al. (2015). Geochemical characteristics and genesis of gases from Tianchi volcanic Springs,Changbai Mountains, Jilin, China. J4 34 (6), 1192–1202. (In Chinese with English abstract).
Liu, E. J., Wood, K., Aiuppa, A., Giudice, G., Bitetto, M., Fischer, T. P., et al. (2021). Volcanic activity and gas emissions along the south sandwich arc. Bull. Volcanol. 83, 3–23. doi:10.1007/s00445-020-01415-2
Liu, G., Sun, Y., and Guo, Z. (2011). The newest monitoring information of Changbaishan volcano, NE China. J.Acta Petrol. Sin. 27 (10), 2905–2911. (In Chinese with English abstract). doi:10.1016/j.pgeola.2011.03.006
Liu, J., Han, J., and Fyfe, W. S. (2001). Cenozoic episodic volcanism and continental rifting in northeast China and possible link to Japan Sea development as revealed from K–Ar geochronology. Tectonophysics 339 (3-4), 385–401. doi:10.1016/S0040-1951(01)00132-9
Liu, J. (1987). Study on geochronology of the Cenozoic volcanic rocks in Northeast China. J.Acta Petrol. Sin. (04), 23–33. (In Chinese with English abstract).
Ma, X. (2006). Dissertation. The Institute of botany, Chinese Academy of Sciences. Effects of cultivation and grazing on SoilCarbon pool and GreenhouseGasesFluxes in the inner Mongolia steppes. Institute of Botany, The Chinese Academy of Science Beijing, Beijing China
Magro, G., Gherardi, F., and Bayon, F. E. B. (2013). Noble and reactive gases of Palinpinon geothermal field (Philippines): Origin, reservoir processes and geodynamic implications. Chem. Geol. 339, 4–15. doi:10.1016/j.chemgeo.2012.09.036
Mäki, M., Aaltonen, H., Heinonsalo, J., Hellén, H., Pumpanen, J., and Bäck, J. (2019). Boreal forest soil is a significant and diverse source of volatile organic compounds. Plant Soil 441, 89–110. doi:10.1007/s11104-019-04092-z
Mazot, A., and Bernard, A. (2015). “CO2 degassing from volcanic lakes,” in Volcanic lakes. Advances in volcanology. Editors D. Rouwet, B. Christenson, F. Tassi, and J. Vandemeulebrouck (Berlin, Heidelberg: Springer). doi:10.1007/978-3-642-36833-2_15
Mazot, A., Rouwet, D., Taran, Y., Inguaggiato, S., and Varley, N. (2011). CO2 and He degassing at el chichón volcano, chiapas, Mexico: Gas flux, origin and relationship with local and regional tectonics. Bull. Volcanol. 73, 423–441. doi:10.1007/s00445-010-0443-y
Mazot, A., and Taran, Y. (2009). CO2 flux from the volcanic lake of El Chichón (Mexico). Geofísica Int. 48 (1), 73–83. doi:10.22201/igeof.00167169p.2009.48.1.100
Norman, J. M., Garcia, R., and Verma, S. B. (1992). Soil surface CO2 fluxes and the carbon budget of a grassland. J. Geophys. Res. Atmos. 97 (D17), 18845–18853. doi:10.1029/92JD01348
Notsu, K., Sugiyama, K., Hosoe, M., Uemura, A., Shimoike, Y., Tsunomori, F., et al. (2005). Diffuse CO2 efflux from Iwojima volcano, Izu-Ogasawara arc, Japan. J. Volcanol. Geotherm. Res. 139 (3-4), 147–161. doi:10.1016/j.jvolgeores.2004.08.003
Ozima, M., and Podosek, F. A. (2002). Noble gas geochemistry. Cambridge University Press. Cambridge, United Kingdom.
Padrón, E., Pérez, N. M., Hernández, P. A., Sumino, H., Melián, G. V., Barrancos, J., et al. (2013). Diffusive helium emissions as a precursory sign of volcanic unrest. Geology 41 (5), 539–542. doi:10.1130/G34027.1
Pan, B., Liu, G., Cheng, T., Zhang, J., Sun, Z., Ma, B., et al. (2021). Development and status of active volcano monitoring in China. Geol. Soc. Lond. Spec. Publ. 510 (1), 227–252. doi:10.1144/SP510-2020-62
Sano, Y., and Marty, B. (1995). Origin of carbon in fumarolic gas from island arcs. Chem. Geol. 119 (1-4), 265–274. doi:10.1016/0009-2541(94)00097-R
Shangguan, Z., and Wu, C. (2008). Geochemical features of magmatic gases in the regions of dormant volcanoes in China. J.Acta Petrol. Sin. 24 (11), 2638–2646. (In Chinese with English abstract).
Sun, Y., Guo, Z., Liu, J., and Du, J. (2018). CO2 diffuse emission from maar lake: An example in Changbai volcanic field, NE China. J. Volcanol. Geotherm. Res. 349, 146–162. doi:10.1016/j.jvolgeores.2017.10.012
Tang, J., Wang, J., Chen, X., Zhao, G., and Zhan, Y. (2005). A PreliminaryInvestigation on electric structure of the crust and upper mantle in ArxanVolcanic area. Chin.J. Geophys. 48 (1), 214–221. doi:10.1002/cjg2.642
Tassi, F., Cabassi, J., Calabrese, S., Nisi, B., Venturi, S., Capecchiacci, F., et al. (2016). Diffuse soil gas emissions of gaseous elemental mercury (GEM) from hydrothermal-volcanic systems: An innovative approach by using the static closed-chamber method. Appl. Geochem. 66, 234–241. doi:10.1016/j.apgeochem.2016.01.002
Wardell, L. J., Kyle, P. R., Dunbar, N., and Christenson, B. (2001). White Island volcano, New Zealand: Carbon dioxide and sulfur dioxide emission rates and melt inclusion studies. Chem. Geol. 177 (1-2), 187–200. doi:10.1016/S0009-2541(00)00391-0
Wei, F., Xu, J., Kong, Q., Liu, S., Xu, D., and Pan, B. (2021). Sources of CH4 with variable carbon isotopes from Changbaishan volcano in NE China: Implications for the feeding system. J. Volcanol. Geotherm. Res. 419, 107355. doi:10.1016/j.jvolgeores.2021.107355
Wei, F., Xu, J., Shangguan, Z., Pan, B., Yu, H., Wei, W., et al. (2016). Heliumand carbon isotopes in the hot springs of Changbaishan volcano, northeasternChina: A material connection between Changbaishan volcano and the westPacifc plate? J. Volcanol. Geotherm. Res. 327, 398–406. doi:10.1016/jjvolgeores.2016.09.005
Wei, H., Liu, R., Fan, Q., and Li, N. (1998). Active volcanoes in China and their relavant hazards. Chin. J. Nat. 20 (4), 5. (In Chinese with English abstract)
Wei, H., Sparks, R. S. J., Liu, R., Fan, Q., Wang, Y., Hong, H., et al. (2003). Three active volcanoes in China and their hazards. J. Asian Earth Sci. 21 (5), 515–526. doi:10.1016/S1367-9120(02)00081-0
Werner, C., Hurwitz, S., Evans, W. C., Lowenstern, J. B., Bergfeld, D., Heasler, H., et al. (2008). Volatile emissions and gas geochemistry of hot spring basin, Yellowstone national park, USA. J. Volcanol. Geotherm. Res. 178 (4), 751–762. doi:10.1016/j.jvolgeores.2008.09.016
Werner, C., Kelly, P. J., Doukas, M., Lopez, T., Pfeffer, M., McGimsey, R., et al. (2013). Degassing of CO2, SO2, and H2S associated with the 2009 eruption of redoubt volcano, Alaska. J. Volcanol. Geotherm. Res. 259, 270–284. doi:10.1016/j.jvolgeores.2012.04.012
Wu, C., Yin, H., Shen, K., Zhu, Y., Xie, H., Cao, T., et al. (2010). Characteristics of petrology and geochenistry of volcanic rocks in the Yin jianggou Aershan area. Geol. Explor. 46 (3), 0515–0524. (In Chinese with English abstract).
Zhang, M., Guo, Z., Liu, J., Liu, G., Zhang, L., Lei, M., et al. (2018). The intraplate changbaishan volcanic field (China/north Korea): A review on eruptive history, magma Genesis, geodynamic significance, recent dynamics and potential hazards. Earth Sci. Rev. 187, 19–52. doi:10.1016/j.earscirev.2018.07.011
Zhang, M., Guo, Z., Sano, Y., Cheng, Z., and Zhang, L. (2015). Stagnant subducted Pacific slab-derived CO2 emissions: Insights into magma degassing at Changbaishan volcano, NE China. J. Asian Earth Sci. 106, 49–63. doi:10.1016/j.jseaes.2015.01.029
Zhang, M., Guo, Z., Sano, Y., Zhang, L., Sun, Y., Cheng, Z., et al. (2016). Magma-derived CO2 emissions in the Tengchong volcanic field, SE Tibet: Implications for deep carbon cycle at intra-continent subduction zone. J. Asian Earth Sci. 127, 76–90. doi:10.1016/j.jseaes.2016.06.009
Zhang, M. L., Guo, Z. F., Cheng, Z. H., Zhang, L. H., and Wen, X. Y. (2011). Greenhouse gases flux estimation of hot springs in changbaishan volcanic field, ne China. Acta Petrol. Sin. 27 (10), 2898–2904. (In Chinese with English abstract). doi:10.1016/j.pgeola.2011.03.006
Zhao, C., Ran, H., and Wang, Y. (2012). Present-day mantle-derived helium release in the Tengchong volcanic field, Southwest China: Implications for tectonics and magmatism. J. Acta Petrol. Sin. 28 (4), 1189–1204.
Zhao, W., Guo, Z., Lei, M., Zhang, M., Zheng, G., Fortin, D., et al. (2019). Volcanogenic CO2 degassing in the songliao continental rift system, ne China. Geofluids 2019 (6), 1–14. doi:10.1155/2019/8053579
Zhao, W., Guo, Z., Liu, J., Zhang, M., Sun, Y., Lei, M., et al. (2021). Fluxes and Genesis of carbon dioxide emissions from Cenozoic volcanic fields in NE China. Acta Petrol. Sin. 37 (4), 1255–1269. (in Chinese with English abstract). doi:10.18654/1000-0569/2021.04.17
Keywords: dormant volcano monitoring, volcanic gas emissions, soil gas fluxes, hot spring gas components, Arxan volcanic field
Citation: Pan X, Gu G, Han D, Bao B, Guan S and Song Y (2023) Investigation of hot spring gas components and soil gas fluxes in Arxan Holocene volcanic field, Inner Mongolia, NE China. Front. Earth Sci. 11:1174315. doi: 10.3389/feart.2023.1174315
Received: 26 February 2023; Accepted: 10 May 2023;
Published: 24 May 2023.
Edited by:
Maoliang Zhang, Tianjin University, ChinaReviewed by:
Wenbin Zhao, Chinese Academy of Sciences, ChinaZhi Chen, China Earthquake Administration, China
Copyright © 2023 Pan, Gu, Han, Bao, Guan and Song. This is an open-access article distributed under the terms of the Creative Commons Attribution License (CC BY). The use, distribution or reproduction in other forums is permitted, provided the original author(s) and the copyright owner(s) are credited and that the original publication in this journal is cited, in accordance with accepted academic practice. No use, distribution or reproduction is permitted which does not comply with these terms.
*Correspondence: Guohui Gu, MTU5NDMwODU3NTNAMTYzLmNvbQ==