- Northwest Research Institute of Engineering Investigations and Design, Xi’an, Shaanxi, China
Loess mudflow is a unique geological hazard in the Loess Plateau, presenting a significant risk to the lives and properties of the region’s inhabitants. Defining and categorizing loess mudflow scientifically, as well as comprehending their disaster-prone environment and patterns of development, are crucial for implementing effective disaster prevention and mitigation strategies. Based on extensive field investigations and statistical analyses, this study defines and categorizes the loess mudflow into five types: liquefaction flow, freeze-thaw creep, subsurface erosion failure, collapse-mudflow, and erosion-enlargement. Statistical analysis reveals that the drainage area of loess mudflow is mostly concentrated between 0.15 and 30 km2, the bed slope typically ranges from 10‰–200‰, material storage in channels is mostly above 5 × 104 m3/km2, and the rainfall intensity triggering mudflow formation is generally greater than 10 mm/h; furthermore, a correlation analysis between the parameters of loess mudflow and the influencing factors reveals a significant linear growth relationship between mudflow volume and drainage area, and also reveals a significant power function relationship with the increase in channel bed slope. However, there is no significant correlation between mudflow volume and solid material storage, and mudflow density shows no significant correlation with drainage area, channel bed slope, or solid material storage. Finally, an empirical formula for estimating mudflow volume is proposed through regression analysis. The research findings aim to provide a theoretical basis for the study and risk assessment of loess mudflow disasters.
1 Introduction
The introduction of the national strategy for ecological conservation and high-quality development with the Yellow River basin have brought increased attention to geological processes, surface processes, and disaster effects in the region (Nicoll et al., 2013). The Loess Plateau, a critical part of the Yellow River basin, poses significant challenges to achieve ecological environmental protection in the Yellow River basin due to its fragile ecological environment, severe soil erosion, and frequent geological disasters (Baosheng et al., 2004; Wang et al., 2008). According to incomplete statistics, between 2002 and 2012, around 30% of national disasters were triggered by geological events in the loess areas (Hou et al., 2018; Leng et al., 2018; Zhuang et al., 2018; Bai et al., 2020). Loess mudflow, among the most frequent and destructive geological disasters in the Loess Plateau, has attracted considerable attention (Maher, 2016a; Peng et al., 2017).
Loess mudflow, unique to the Loess Plateau, exacerbates soil erosion in the region. Severe soil erosion not only causes significant sedimentation in the downstream areas of the Yellow River but also carries away fertile topsoil, leads to substantial degradation of agricultural quality and further deterioration of the ecological environment (Shi and Shao, 2000; Wu et al., 2018). Large-scale mudflow disasters can destroy villages, bury farmland, and block main rivers and reservoirs, leads to even more severe secondary disasters (Arouri et al., 2015). Loess mudflow, due to their relatively small scale during the initial stages of formation, are challenging to be identified promptly for implementing effective prevention measures. Moreover, once fully developed, the widespread occurrence of loess mudflows in the Loess Plateau can lead to serious disasters (Ostwald and Chen, 2006). For instance, intense rainfall in 2013 in Yan’an, on the Loess Plateau, triggered several large-scale mudflow disasters, led to significant casualties and economic losses. In Qinzhou district, Tianshui city, Gansu province, mudflows had resulted in dozens of casualties in Niangniang Town (Zhang et al., 2017). Between 2012 and 2015, the Zuli River basin in Gansu had experienced five consecutive mudflows in Kuzi valley, it caused severe mud accumulation on Gansu Provincial Road 207 and resulted in substantial property losses (Zhai et al., 2014). Since the 1990s, researchers have studied the initiation mechanisms and developmental patterns of mudflows. However, the recent progress in the study of loess mudflow is relatively slow, especially in addressing issues such as scientific definition, classification, and effective prevention of mudflow disasters (Guzzetti et al., 2008; Guo et al., 2013). Nevertheless, the frequency of loess mudflow occurrences has been gradually increasing. Due to the unique distribution of loess mudflow, their disaster effects and developmental patterns are differ significantly from typical mudflows in other regions.
Based on data collection and field investigations of loess mudflow, this paper provides a preliminary definition of loess mudflow. It analyzes their developmental types and patterns and conducts a correlation analysis of mudflow characteristics and influencing factors (Bai et al., 2022), serving as a reference for future research on loess mudflow.
2 Definition and classification of mudflows
2.1 Definition of mudflows
Mudflows are widely considered as a special type of debris flow (Bowles et al., 2003). However, due to the unique material composition and distribution characteristics, mudflows have distinct differences in formation conditions, fluid characteristics, transportation and deposition features compared to typical debris flows (Jing et al., 2018). Currently, there is no unified definition standard for loess mudflow, distinctions can be made based on the composition of solid materials in the fluid, the presence of fine particles, and the variations in fluid mechanics properties. Mudflows can be classified into four types: high-sand water flow, mud flow, debris flow, and water-rock flow (Coussot and Meunier, 1996).
The composition of materials and the content of fine particles are crucial characteristics for distinguishing mudflows from debris flows and water-rock flows. Li (1999) stated that mudflows are mainly developed in the Loess Plateau, where the solid material composition is primarily composed from fine particles (silt, clay), making up 80%–90% of the composition, with sand and gravel accounting for only 10%–20%. Mudflows are characterized by the presence of numerous mud balls and significant cracking on the deposit surface post-drying. Tang et al. (2000) described that in mudflow fluids, sand, silt, and clay make up over 98% of the solid material in mudflow fluids, with rocks constituting less than 2%. After analyzed soil samples from 16 typical loess mudflow gullies in Yan’an (7 gullies) and Gansu (9 gullies) regions (Figure 1A), the author found that the content of fine particles in mudflow soil samples from Yan’an ranged from 50%–70%, with sand and gravel content between 30% and 50%. In contrast, mudflow samples from Gansu had a fine particle content of over 90%, and less than 10% sand and gravel content (Figure 1B).
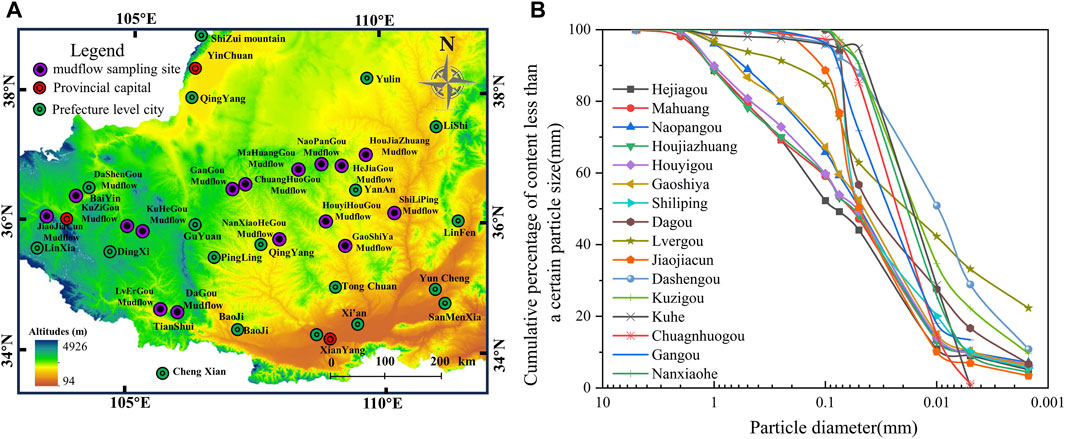
Figure 1. Particle size distribution curve of loess mudflow sample. (A) Distribution of sampling points; (B) Particle gradation curve of loess mudflow sample.
On the basis of meeting material composition and fine particle content requirements, mudflows are primarily composed of fine particles regarding fluid properties. They move in suspension and create a stable slurry with water. Due to the fine particle composition, mudflows are easily transit to laminar flow when in high concentration and exhibit turbulent flow when in low concentration. In contrast to water flows with high-sand content, mudflow fluids require a specific shear stress to initiate movement and are classified as non-Newtonian fluids according to Pearson and Tardy (2002); Kirwan (2016).
Based on the above statistics and combining with previous research, considering both material composition and fluid properties, we define a non-Newtonian fluid as a mudflow when the content of fine particles (particle size less than 2 mm) in the solid material composition is over 98%, and the content of fine particles (silt and clay) is not less than 50%.
2.2 Classification of mudflows
The classification of mudflows should have a certain basis and scientific rigor to better serve the identification and prevention of mudflows. Scientific classification is an essential prerequisite for studying the formation, transportation, and deposition patterns of mudflows. Due to different research purposes, the criteria and bases for classifying mudflows vary, and there is currently no systematic classification study specifically for mudflows. Chinese geologists primarily classify mudflows based on the solid material composition, genesis, fluid properties, soil source, geomorphic conditions, triggering factors, inducing factors, and dynamic characteristics (Li, 1999; Lei et al., 2000; Knight et al., 2010; Xin et al., 2015); (Table 1).
The above classifications have considered the differences in mudflow initiation mechanisms to a lesser extent, especially regarding the formation mechanism classification research of loess mudflow in the Loess Plateau. We attempt to depart from the perspective of mudflow formation mechanisms. Through a comparative analysis of various mudflow classifications and the physical significance of their classification criteria, we hope to achieve a more reasonable method of mudflow classification. Mudflow formation is influenced by various factors such as soil self-weight stress and hydraulic conditions. We categorize mudflows into five types based on their initial conditions, formation mechanisms, and modes of transportation, and the five types are listed as: Liquefied flow type (Figure 2A), Freeze-thaw creep-slip type (Figure 2B), subducting and ulcerating type (Figure 2C), trapping and oozing type (Figure 2D), and Erosion Expansion Type (Figure 2E); (Table 2).
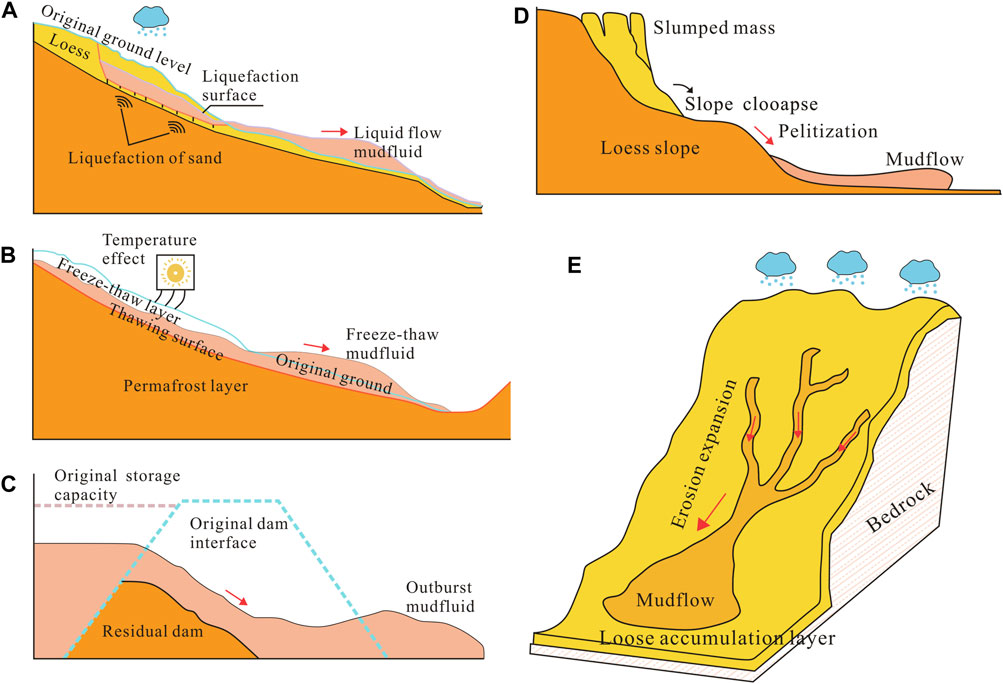
Figure 2. Schematic diagram of mudflow classification model. (A) Liquefied flow type; (B) Freeze-thaw creepslip type; (C) Subducting and ulcerating type; (D) Trapping and oozing type; (E) Erosion expansion type.
3 Development patterns of loess mudflow and their correlation with influencing factors
The influencing factors of debris flow mainly include terrain conditions, water source conditions and material sources. A detailed analysis of each influencing factor is stated as follows:
3.1 Formation conditions and development patterns of mudflows
According to the classification analysis of mudflows, mudflows form under specific conditions. Steep topography and abundant loose solid materials are intrinsic factors for mudflow formation, while intense rainfall, earthquakes, and hydraulic erosion are external dynamic factors that trigger the evolution of mudflow disasters (Hou et al., 2018).
Special geological structures are important conditions for the formation of mudflow channels. Through field investigations and data reviews, the researchers found that the areas where mudflow channels occur are typically hilly regions with widespread distribution of loess formations. The banks of mudflow channels are composed with pure loess, mainly include the Holocene Wucheng loess of the Lower Quaternary, as well as the Lishi loess of the Middle Quaternary and the Malan loess of the Upper Quaternary. The thickness of loess distribution in most channels is 60–70 m, some of which are even exceeding 100 m. The majority portion of solid material supply in mudflow channels comes from the collapse and landslides of loess slopes on both sides.
The scale and frequency of mudflows are determined by the combined influence of the above factors. According to the analysis of collected historical events of mudflow outbreaks and influencing factors, it is found that heavy rainfall is the main driving force behind mudflow disasters among the external forces mentioned above (Li, 1999; Lei et al., 2000; Wang et al., 2008; Xin et al., 2015). Therefore, a statistical analysis of 186 mudflow channels in Qingyang City, Gansu Province (Figure 3), was conducted using statistical methods to clarify the characteristics of their disaster-prone environments and development patterns. The rainfall data used in the article are from literature records collected during mudflow disasters (Lanzhou Institute of Glaciology and Geocryology, Chinese Academy of Sciences, 1982; Wang et al., 1997; Li, 1999; Ding Pan, 2020). Mudflow bulk density, loose solid material mass, and total mudflow volume data refer to geological hazard investigation and zoning reports of six counties in Qingyang City (Geological and Environmental Monitoring Institute of Gansu Province, 2007, 2008). The reported mudflow bulk density is obtained from collected historical records and by on-site measurement methods; while the mass of loose solid material is obtained through field investigations and remote sensing image interpretation; and the total mudflow volume is calculated using empirical formula 1 (Zhongxin, 2014).
Where: Q is the total volume of mudflow (m3); t is the duration of the mudflow (s); QC is the peak flow rate of the mudflow (m3/s).
3.1.1 Channel morphology
Channel morphology significantly constrains the initiation characteristics of loess mudflow. The watershed area and channel slope are two important indicators which reflect channel morphology. The schematic diagram of watershed area and longitudinal ratio drop is shown in Figure 4.
(1) Watershed area
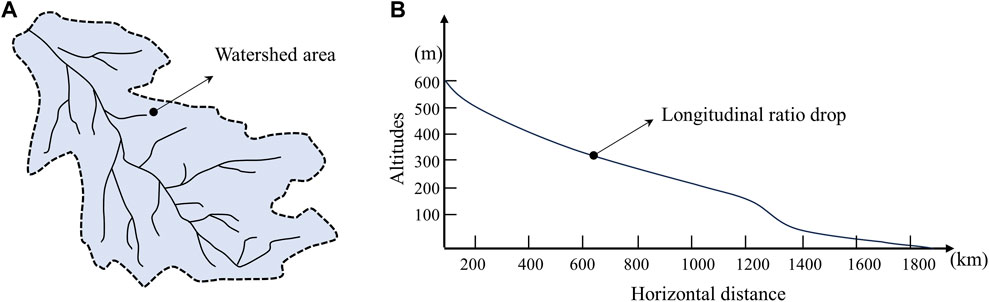
Figure 4. Schematic conceptualization of watershed area and longitudinal specific drop. (A) Watershed area; (B) Longitudinal ratio drop.
The size of the watershed area has a significant impact on the formation, nature, and scale of mudflows. Through statistical analysis, it was found that the watershed areas of loess mudflow channels are mostly concentrated in the range of 0.15–30 km2, accounting for 82.7% of the total number of channels (Figure 5). This is close to the 90% proportion of watershed areas in loess mudflow channels reported by Wang (1997). In summary, it can be inferred that the watershed area of mudflow channels in the Loess Plateau is generally less than 30 km2, providing an area range for evaluating the high incidence rate of mudflows in the region.
(2) Longitudinal ratio drop of the trench
The channel slope, represented by the longitudinal slope, can visually reflect the gradient of runoff in the channel, and it directly affects the hydraulic conditions of the channel. When the channel slope is small and the hydraulic conditions are poor, the initiation of loose materials in the channel bed is severely restricted. When the longitudinal slope increases and approaches or exceeds the natural angle of repose of loose materials, solid materials are more likely to be mobilized, and form the source of mudflows. Observations show that the channel slopes of mudflow channels are mostly concentrated in the range of 10‰–200‰, accounting for 90.91% of the total (Figure 6). This is also close to the criterion proposed by Wang (1997), that the channel slope of mudflow channels should be less than 250‰.
(3) Relationship Between watershed area and Longitudinal ratio drop of the trench
The size of the longitudinal slope of mudflow channels is also related to the watershed area. Figure 7 presents the relationship between watershed area and channel slope. The results show that the longitudinal slope tends to decrease with the increase of the watershed area and exhibits a good power-law relationship. When the composition and distribution of solid loose materials in the channel are the same, the upstream longitudinal slope of the watershed is relatively large. However, because of the small drainage area in this region, hydraulic conditions are insufficient, and it cannot cause severe erosion, which provides limited material for mudflow initiation. As the watershed area increases, the drainage area also increases, leading to larger hydraulic conditions, and mudflows can occur even with relatively small longitudinal slopes. Therefore, with the increase in watershed area, there is a tendency for the required channel slope for mudflow formation to decrease.
3.1.2 Rainfall factors
Adequate water supply is another essential condition for the formation of loess mudflow. The water sources for mudflows in the Loess Plateau mainly come from atmospheric precipitation and irrigation (Wang, 1997). The main characteristics of precipitation on the Loess Plateau are concentrated and intense, often taking the form of heavy rainfall. Strong rainfall conditions can easily gather large hydraulic conditions in the watershed area, which could cause the loose materials to accumulate in the channel bed and further lead the slope deposits to be eroded and transported, and at last form the source of mudflows. Based on the collected rainfall intensity data during the outbreak of loess mudflow channels, it was found that the rainfall intensity which causes mudflow outbreaks was mostly greater than 10 mm/h, accounting for 95.65% of the total, with a significant portion falling between 10 and 20 mm/h, accounting for 41.3% of the total (Figure 8).
3.1.3 Solid loose material storage
The storage of solid loose material is one of the essential conditions for the formation of mudflows. The material that been storage in mudflow channels is usually greater than 5 × 104 m3/km2, accounting for 96.77% of the total number of channels. Among them, cases with storage greater than 20 × 104 m3/km2 are most common, accounting for 61.83% of the total (Figure 9). This indicates that a certain level of solid material storage is necessary for the formation of mudflows. Through field investigations, it was found that the main ways of loose material supply include slope erosion, landslides, and collapse sources.
3.2 Correlation analysis of mudflow characteristics and influencing factors
The formation of mudflows is a complex physical process influenced by multiple factors (Pen, 2015). Watershed area, channel slope, solid material storage, and rainfall intensity have decisive effects on mudflow formation. However, under the influence of different factors, the scale and bulk density of mudflows exhibit distinct characteristics. Moreover, the interactive effects of various factors also have diverse impacts on mudflow characteristics, making the formation process relatively intricate. This section analyzes the main influencing factors of mudflow volume and density under different factor conditions and combinations of multiple factors. Due to the absence of some rainfall data for certain channels, this section only analyzes watershed area, channel slope, and solid material storage as factors.
3.2.1 Analysis of correlation between mudflow volume and influencing factors
Through the correlation analysis of mudflow volume and watershed area, channel slope, and solid material storage, it is observed that the mudflow volume exhibits a significant linear growth relationship with the watershed area and a significant power function relationship with the increase of channel bed slope (Figure 10). However, there is no significant correlation between mudflow volume and solid material storage. When the watershed area increases, the channel’s drainage capacity increases, enhancing hydraulic conditions, and thus increasing its transport and erosion capacity, lead to the involvement of more loose material in mudflow and subsequently increases the mudflow volume. However, the mudflow volume decreases in a power function as the channel bed slope increases. The main reason is illustrated in Figure 9, where the longitudinal slope decreases with the increase in watershed area. When the channel bed slope is large, the corresponding watershed area is small, and the hydraulic conditions in the small valley are relatively weak, hindering the initiation of loose material and limiting the supply of loose material to the channel, resulting in a relatively small mudflow volume. When the solid material storage increases, the mudflow volume does not significantly increase. One reason is that although there is a considerable amount of solid material storage in the channel, interference from factors such as watershed area and slope may reduce the erosion and transport capacity of runoff in the channel, result in less loose material been participated in mudflow; on the other hand, when there is a large amount of solid material in the channel, it is only under the conditions of extensive heavy rainfall, which forms significant hydraulic conditions, with what the channel may have the capability to transport and erode solid material. Even if mudflows are formed locally, they may be blocked and stopped due to the blocking effect of the accumulation of loose material in the channel, and only smaller-scale mudflows can be formed.
3.2.2 Correlation analysis of mudflow capacity and influence factors
Through the correlation analysis of mudflow density (this density represents the bulk density of a single mudflow) with watershed area, channel slope, and solid material storage, it is found that the mudflow density has no significant correlation with watershed area, channel slope, or solid material storage. It can be seen from Figure 11 that the density of mudflow is mostly concentrated between 1.55 and 1.65, and there is no significant variation with changes in watershed area, channel slope, or solid material storage. Mudflows are mostly dilute or transitional mudflows (Cui et al., 2018), with only three channels classified as viscous mudflows. The main reason is that viscous mudflows mostly belong to the category of cohesive mudflows. Their formation is characterized by the increase in the water content of the solid loose deposits in the channel, which lead to a decrease in the internal strength of the soil, an increase in its self-weight. When the ultimate shear strength is less than the shear force, the accumulated material will undergo a quick transition, that gradually liquefying into a mudflow during the movement (Zhu et al., 2017). The characteristic of rainfall in the Loess Plateau region is concentrated and intense, often occurs in the form of one or several heavy rains. The formation of mudflows in this region is mainly through the source of loose deposits in the channel bed by surface runoff that formed in the channel watershed. Therefore, based on the rainfall characteristics of the region, the mudflows formed in the Loess Plateau region are generally hydraulic mudflows, namely, dilute or transitional mudflows.
3.2.3 Comprehensive analysis of factors influencing mudflow characterization
In order to comprehensively analyze the combined influence of watershed area, channel slope, solid material storage, and their interactions on mudflow characteristics, a correlation analysis was conducted for watershed area(A), channel slope(λ), solid material storage(Ψ), watershed area-channel slope interaction (IAλ), watershed area-solid material storage interaction (IAΨ), channel slope-solid material storage interaction (IλΨ), and the watershed area-channel slope-solid material storage interaction (IAλΨ). As shown in Table 3, mudflow volume is highly correlated with watershed area and channel slope. It also exhibits significant correlations with the interaction of watershed area-channel slope, watershed area-solid material storage, and watershed area-channel slope-solid material storage. The order of magnitude for these correlations is A>λ>IAΨ>IAλΨ>IAλ (Table 3). However, mudflow volume shows no significant correlation with solid material storage and channel slope-solid material storage interaction. These results indicate that watershed area and channel slope are the most crucial factors which influence mudflow volume, and the interactions between various factors also significantly affect mudflow volume. Larger watershed areas provide sufficient water sources for mudflows, while steeper channel slopes provide greater hydraulic conditions. The combination enhances the erosive transport capacity of channel runoff. It is also observed from Table 1 that mudflow density does not exhibit significant correlations with the related factors.
Through the regression analysis of the highly significant correlation between mudflow volume and watershed area, as well as channel slope which obtained from the above analysis, the regression formula for mudflow volume is derived:
In the formula, Q represents the mudflow volume (m3);A is the watershed area (km2);λ denotes the channel slope (‰). The F-test and t-tests for each parameter in the fitted equation all reach a significant level.
Equation 2 of the regression model indicates that during the variation process of mudflow volume, the watershed area plays a prominent role, while an increase in channel slope leads to a decrease in mudflow volume.
4 Conclusion
This paper firstly provided a preliminary definition of loess mudflows and classified them based on their formation conditions. Subsequently, a statistical analysis of the disaster-prone characteristics and developmental patterns of 186 mudflow channels in Gansu Province was conducted. Finally, a correlation analysis of mudflow characteristics and influencing factors was performed, lead to the following preliminary conclusions:
(1) Based on the initial conditions and formation mechanisms of mudflows, they can be categorized into five types: liquefaction flow-type mudflows, freeze-thaw creep-type mudflows, erosion-collapse-type mudflows, subsidence-liquefaction-type mudflows, and erosion-expansion-type mudflows.
(2) The watershed areas of loess mudflow gullies are mostly concentrated in the range of 0.15–30 km2, and the channel bed slopes are mainly within 10‰–200‰. The material storage in the channels is generally above 5 × 104 m3/km2. The rainfall intensity causing mudflow formation is generally greater than 10 mm/h. This data can be used to assess the factor intervals and contributes to the high incidence of mudflows in the Loess Plateau region.
(3) A correlation analysis was conducted on the various influencing factors and their interactions with mudflow volume and mudflow density in loess regions. The order of correlation for factors influencing mudflow volume is A>λ>IAΨ>IAλΨ>IAλ. An empirical formula for estimating mudflow volume in loess regions was proposed through regression analysis.
Data availability statement
The original contributions presented in the study are included in the article/Supplementary Material, further inquiries can be directed to the corresponding author.
Author contributions
BW: Conceptualization, Formal Analysis, Methodology, Resources, Writing–original draft, Writing–review and editing. ZZ: Investigation, Software, Visualization, Writing–review and editing. XH: Supervision, Validation, Writing–review and editing. GG: Validation, Writing–review and editing. XD: Data curation, Writing–review and editing. YY: Data curation, Writing–review and editing.
Funding
The author(s) declare that no financial support was received for the research, authorship, and/or publication of this article.
Conflict of interest
The authors declare that the research was conducted in the absence of any commercial or financial relationships that could be construed as a potential conflict of interest.
Publisher’s note
All claims expressed in this article are solely those of the authors and do not necessarily represent those of their affiliated organizations, or those of the publisher, the editors and the reviewers. Any product that may be evaluated in this article, or claim that may be made by its manufacturer, is not guaranteed or endorsed by the publisher.
References
Arouri, M., Nguyen, C., and Youssef, A. B. (2015). Natural disasters, household welfare, and resilience: evidence from rural vietnam. World Dev. 70, 59–77. doi:10.1016/j.worlddev.2014.12.017
Bai, B., Bai, F., Li, X., Nie, Q., Jia, X., and Wu, H. (2022). The remediation efficiency of heavy metal pollutants in water by industrial red mud particle waste. Environ. Technol. Innov. 28, 102944. doi:10.1016/j.eti.2022.102944
Bai, B., Xu, T., Nie, Q., and Li, P. (2020). Temperature-driven migration of heavy metal Pb2+ along with moisture movement in unsaturated soils. Int. J. Heat. Mass Transf. 153, 119573. doi:10.1016/j.ijheatmasstransfer.2020.119573
Baosheng, W., Zhaoyin, W., and Changzhi, L. (2004). Yellow River Basin management and current issues. J. Geogr. Sci. 14, 29–37. doi:10.1007/BF02841104
Bowles, F. A., Faas, R. W., Vogt, P. R., Sawyer, W. B., and Stephens, K. (2003). Sediment properties, flow characteristics, and depositional environment of submarine mudflows, Bear Island Fan. Mar. Geol. 197, 63–74. doi:10.1016/S0025-3227(03)00089-6
Coussot, P., and Meunier, M. (1996). Recognition, classification and mechanical description of debris flows. Earth-Sci. Rev. 40, 209–227. doi:10.1016/0012-8252(95)00065-8
Guo, X., Cui, P., and Li, Y. (2013). Debris flow warning threshold based on antecedent rainfall: a case study in Jiangjia Ravine, Yunnan, China. J. Mt. Sci. 10, 305–314. doi:10.1007/s11629-013-2521-z
Guzzetti, F., Peruccacci, S., Rossi, M., and Stark, C. P. (2008). The rainfall intensity–duration control of shallow landslides and debris flows: an update. Landslides 5, 3–17. doi:10.1007/s10346-007-0112-1
Hou, X., Vanapalli, S. K., and Li, T. (2018). Water infiltration characteristics in loess associated with irrigation activities and its influence on the slope stability in Heifangtai loess highland, China. Eng. Geol. 234, 27–37. doi:10.1016/j.enggeo.2017.12.020
Jing, L., Kwok, C. Y., Leung, Y. F., Zhang, Z., and Dai, L. (2018). Runout scaling and deposit morphology of rapid mudflows. J. Geophys. Res. Earth Surf. 123, 2004–2023. doi:10.1029/2018JF004667
Kirwan, A. (2016). On objectivity, irreversibility and non-Newtonian fluids. Fluids 1, 3. doi:10.3390/fluids1010003
Knight, R., Pyrak-Nolte, L. J., Slater, L., Atekwana, E., Endres, A., Geller, J., et al. (2010). Geophysics at the interface: response of geophysical properties to solid-fluid, fluid-fluid, and solid-solid interfaces. Rev. Geophys. 48. doi:10.1029/2007RG000242
Lei, X. Y., Huang, Y. H., and Wang, W. (2000). Disasters of mud flow and human activities on the loess plateau of China. Geol. Shaanxi (01), 28–39.
Leng, Y., Peng, J., Wang, Q., Meng, Z., and Huang, W. (2018). A fluidized landslide occurred in the Loess Plateau: a study on loess landslide in South Jingyang tableland. Eng. Geol. 236, 129–136. doi:10.1016/j.enggeo.2017.05.006
Li, Z. S. (1999). Disaster and prevention of debris flow in Shaanxi province. Environ. Shaanxi (01), 3–6.
Maher, B. A. (2016a). Palaeoclimatic records of the loess/palaeosol sequences of the Chinese Loess Plateau. Quat. Sci. Rev. 154, 23–84. doi:10.1016/j.quascirev.2016.08.004
Maher, B. A. (2016b). Palaeoclimatic records of the loess/palaeosol sequences of the Chinese Loess Plateau. Quat. Sci. Rev. 154, 23–84. doi:10.1016/j.quascirev.2016.08.004
Nicoll, T., Brierley, G., and Yu, G. (2013). A broad overview of landscape diversity of the Yellow River source zone. J. Geogr. Sci. 23, 793–816. doi:10.1007/s11442-013-1045-3
Ostwald, M., and Chen, D. (2006). Land-use change: impacts of climate variations and policies among small-scale farmers in the Loess Plateau, China. Land Use Policy 23, 361–371. doi:10.1016/j.landusepol.2005.04.004
Pearson, J. R. A., and Tardy, P. M. J. (2002). Models for flow of non-Newtonian and complex fluids through porous media. J. Newt. Fluid Mech. 102, 447–473. doi:10.1016/S0377-0257(01)00191-4
Pen, X. (2015). Comment on the progress in,problems and countermeasure on mudflow induced by rainfall. Geol. Rev., Available at: https://www.semanticscholar.org/paper/Comment-on-the-Progress-in%2CProblems-and-on-Mudflow-Pen/533eac4fce4649d4f78b010acbb59992de192a68 (Accessed December 23, 2023).
Peng, J., Huo, A., Cheng, Y., Dang, J., Wei, H., Wang, X., et al. (2017). Submersion simulation in a typical debris flow watershed of Jianzhuangchuan catchment, Loess Plateau. Environ. Earth Sci. 76, 462. doi:10.1007/s12665-017-6797-0
Shi, H., and Shao, M. (2000). Soil and water loss from the Loess Plateau in China. J. Arid. Environ. 45, 9–20. doi:10.1006/jare.1999.0618
Tang, B. X., Zhou, B. F., Wu, J. S., Zhong, D. L., Luo, D. F., Liu, S. Q., et al. (2000). Debris flows in China. Beijing: The Commercial Press.
Wang, S.-Y., Liu, J.-S., and Yang, C.-J. (2008). Eco-environmental vulnerability evaluation in the Yellow River basin, China. Pedosphere 18, 171–182. doi:10.1016/S1002-0160(08)60005-3
Wu, L., Jiang, J., Li, G., and Ma, X. (2018). Characteristics of pulsed runoff-erosion events under typical rainstorms in a small watershed on the Loess Plateau of China. Sci. Rep. 8, 3672. doi:10.1038/s41598-018-22045-x
Xin, P., Wu, S. R., Shi, J. S., Wang, T., and Shi, L. (2015). Comment on the progress in,problems and countermeasure on mudflow induced by rainfall. Geol. Rev. 61 (03), 485–493.
Zhai, X., Xia, J., and Zhang, Y. (2014). Water quality variation in the highly disturbed Huai River Basin, China from 1994 to 2005 by multi-statistical analyses. Sci. Total Environ. 496, 594–606. doi:10.1016/j.scitotenv.2014.06.101
Zhang, X., Li, P., Li, Z. B., and Yu, G. (2017). Characteristics and formation mechanism of the July 25, 2013, Tianshui group-occurring geohazards. Environ. Earth Sci. 76, 219. doi:10.1007/s12665-017-6542-8
Zhu, X. H., Peng, J. B., Tong, X., and Ma, P. H. (2017). Preliminary research on geological disaster chains in loess area. J. Eng. Geol. 25 (01), 117–122.
Keywords: loess mudflow, basin form, developmental characteristics, statistical analysis, correlation analysis
Citation: Wang B, Zhao Zh, Huang X, Gu G, Du Xh and Yang Y (2024) Developmental characteristics of loess mudflow and correlation analysis with influencing factors. Front. Earth Sci. 12:1373126. doi: 10.3389/feart.2024.1373126
Received: 19 January 2024; Accepted: 02 April 2024;
Published: 16 April 2024.
Edited by:
Hans-Balder Havenith, University of Liège, BelgiumReviewed by:
Qian-Gong Cheng, Southwest Jiaotong University, ChinaBing Bai, Beijing Jiaotong University, China
Copyright © 2024 Wang, Zhao, Huang, Gu, Du and Yang. This is an open-access article distributed under the terms of the Creative Commons Attribution License (CC BY). The use, distribution or reproduction in other forums is permitted, provided the original author(s) and the copyright owner(s) are credited and that the original publication in this journal is cited, in accordance with accepted academic practice. No use, distribution or reproduction is permitted which does not comply with these terms.
*Correspondence: Zhi hai Zhao, emhhb3poaWhhaTE5OTBAMTYzLmNvbQ==