- Department of Educational Science, University of Regensburg, Regensburg, Germany
Research has evidenced that digital educational games can be effective tools to impart knowledge. Researchers have recommended to focus on motivation and gaming load and their interaction when investigating learning process and success. Gaming expertise and the English proficiency of learners seem to be further important aspects of learning success, especially when non-native speakers play an English game. However, knowledge about the motivational and cognitive impact of games and learner characteristics on learning outcomes needs to be augmented and clarified. The present study aimed to address this need. We conducted an experimental media comparison to investigate the effects of game play and expertise in gaming and English on motivation, cognitive load, and performance. The participating German university students were randomly assigned to an educational gaming group and a hypertext group. Aspects of motivation were assessed before and after studying and gaming, cognitive load was rated during and after learning, and level of performance was measured before and after studying. The gaming group reported a higher level of interest, challenge, and anxiety of failing after introducing the task. Groups did not differ significantly in their perceived probability of success. The group levels of interest were the same after a 1 h learning phase. When learning, cognitive load increased after the initial phase in the gaming group and then stayed on a constant level, whereas the opposite pattern was found in the hypertext group. No differences were found in load ratings after learning between the two groups. Both groups improved their knowledge after learning, but the gain was larger for the hypertext group. Results point to gaming and English expertise as two mediating factors for learning success with educational games. We suggest that gaming expertise and English comprehension ability reduce cognitive load and thus enable learners to focus their resources on meaningful learning.
Introduction
Game-based digital learning has gained much interest in educational settings in the last 20 years (Connolly et al., 2012; Li and Tsai, 2013; All et al., 2014; Westera, 2015; Boyle et al., 2016; McLaren et al., 2017). Although game-based learning is a young field of systematic research (Westera, 2015), a few reviews exist (e.g., Egenfeldt-Nielsen, 2006; Divjak and Tomic, 2011; Connolly et al., 2012; Young et al., 2012; Li and Tsai, 2013; All et al., 2014; Tobias et al., 2014; Boyle et al., 2016; Hainey et al., 2016; Merino Campos and del Castillo Fernández, 2016) but meta-analyses are rare (e.g., Vogel et al., 2006; Sitzmann, 2011; Wouters et al., 2013; Clark et al., 2016). In general, the studies have revealed that game-based learning is linked to a broad range of perceptual, cognitive, behavioral, affective, and motivational effects and outcomes, but they also present a broad range of theories, research questions, designs, methods, and type of games used in the reviewed studies (e.g., Connolly et al., 2012; Li and Tsai, 2013; Tobias et al., 2014; Boyle et al., 2016; Clark et al., 2016; Merino Campos and del Castillo Fernández, 2016; Parong et al., 2017). In addition to this general knowledge about game-based learning, the existing research has also advanced detailed knowledge about designing effective educational games (e.g., Mayer and Johnson, 2010; Tobias et al., 2014). However, Connolly et al. (2012) pointed to the lack of empirical evidence on game-based learning, especially the lack of studies employing experimental designs with suitable control conditions. More recently, McLaren et al. (2017), in one of the latest experimental studies in this field, emphasized the need for sound experimental research on game-based learning. Westera (2015) critically discussed the key arguments for digital game-based learning (including the “games foster motivation” argument) as a response to many articles that have emphasized the positive effects of game-based learning despite the lack of empirical evidence.
Motivation and gaming load are two aspects often emphasized in the literature. Educational games are suggested to increase motivation (and thereby improve learning processes), but they are also suspected to require additional effort from the users to be successful gamers and hence successful learners (Keller, 2008; Schrader and Bastiaens, 2012; Woo, 2014; Nebel et al., 2015; Westera, 2015). Researchers have stressed that this “fine balance between increased mental effort and potential motivational benefits has to be addressed” (Nebel et al., 2015, p. 384); otherwise, students might fail in game-based learning. The systematic varying of important factors of educational games to investigate cause-and-effect paths in experimental studies (and thus improving the method, i.e., value-added research sensu Clark et al., 2016) is good scientific practice. However, keeping the balance between educational games and instructional material without game elements when a comparison is warranted is also very challenging (i.e., media comparison research sensu Connolly et al., 2012; Li and Tsai, 2013; Clark et al., 2016). This latter approach is more than pragmatic because it can inform educators about optimum methods that could be used to obtain learning objectives depending on learner characteristics. Taking a media-comparison perspective, the following study investigates the effects of an educational game on motivation, cognitive load, and performance in comparison to an educational hypertext in a repeated-measures experimental design. This method allows to compare educational systems and the effectiveness of each educational systems separately. Additionally, we investigated whether the learners could also benefit from instruction when the educational language is not their mother tongue or when they have only little or no gaming expertise. The need to study in English is common in international learning settings, which might add extra cognitive load to the challenge (i.e., difficulty) of the learning task in general when English is not the primary language of the learners. Our investigation was conducted against the background of the Cognitive Load Theory (CLT; de Jong, 2010; Sweller, 2010; Martin, 2014).
Educational Games and Game-Based Learning
Games are widely considered a subgroup of play. For Gray (2009, p. 480) play is “activity that is (1) self-chosen and self-directed; (2) intrinsically motivated; (3) structured by mental rules; (4) imaginative; and (5) produced in an active, alert, but non-stressed frame of mind.” Play mainly turns into a game by having a clearly defined goal and rules and a feedback mechanism focused on approaching the goal (McGonigal, 2011). Naturally, McGonigal (2011) also considers that gamers voluntarily participate in a game. Gray (2009) makes no distinction between game and play. He addresses the concept of formal game as players facing an already established fictional situation that provides the foundation for the explicit rules.
Game-based learning means using games in educational contexts to reach educational objectives (Connolly et al., 2012). These games could be entertaining games or educational games. While entertaining games are mainly designed for fun, entertainment, and recreation, educational games are a subgroup of games that are designed for educational purposes (Connolly et al., 2012). The main goal of game-based learning is learning and behavioral change (Connolly et al., 2012). Game-based learning is often used in the same context as serious games (Boyle et al., 2016), but serious games are often comprehended in a broader sense. Djaouti et al. (2011, p. 120), for example, defined serious games as “any piece of software that merges a non-entertaining purpose (serious) with a video game structure (game).” This definition includes also purposes that have both non-entertaining and non-educational purposes. More often, the broader purposes of serious games are scratched by naming (1) markets like government and non-governmental organization, defense, healthcare, marketing and communication, corporate, industry and education, and (2) purposes like games for health, advergames, games for training, games for education, games for science and research, production, and games as work (Sawyer and Smith, 2008).
One way to approach educational games is to describe them along important gaming dimensions or to classify them. Li and Tsai (2013) empirically found two characteristics that distinguish games: (1) the player modus and (2) the media type. The player modus provides the categories of single-player and the multiplayer modes, which can be further split into closed mode (playing is restricted to a defined group of players like in a classroom) or massive mode (playing is open to numerous players who are likely unknown to any single player). The media type refers to usage of immobile devices (like computers or laptops), of classic mobile devices (like mobile phones and tablets), and innovative mobile devices (like headsets and virtual reality glasses). Li and Tsai (2013) also accounted for the learning approach and differentiated between a game-playing and a game-making approach.
Connolly et al. (2012) developed a multidimensional framework for categorizing games along the following aspects: (1) Digital or non-digital game, (2) purpose of the game, (3) genre, (4) subject discipline, and (5) platform/delivery. This framework is a broader approach to classifying games and could be applied to any game. A game is classified as digital or non-digital depending on coding. Purposes of games were differentiated as games designed for entertainment, games for learning, and serious games. The game genre was classified according to the taxonomy of eight entertainment games provided by Herz (1997): Action games, adventure games, fighting games, puzzle games, role-playing games, simulations, sports games, and strategy games. The subject disciplines refer to the knowledge domain or curricular area of focus, which are health, society, mathematics, language, engineering, general knowledge, geography, and science. Finally, the mode of providing a game is categorized as video console, PC, online game, second life, mobile game, or alternate reality game.
Norman (2011) identified six general skill components necessary for playing games. The extracted general skill components are (1) perceptual-motor, (2) cognitive-verbal, (3) problem solving, (4) information utilization, (5) persistence, and (6) human-human interaction. Perceptual-motor abilities involve numerous components pertaining to perceptual speed, pattern recognition, object identification, simple and choice reaction time, tracking, targeting, timing, rhythm, and response mapping. Cognitive-verbal abilities involves processing or interpreting written and spoken information as well as detecting hidden objects and operating in secrecy and deception. Problem solving abilities comprise solving puzzles or problematic situations but also assessing and using probabilities in a game. Information utilization abilities includes the use of information from memory about events, names, and places in a game and also filtering out irrelevant information and adapting to changing requirements and opponents. Persistence refers primarily to having the patience to continue through difficult or boring parts of the game. Human -to-human interaction is about competing (playing as an opponent) or communicating (playing in a team) with other players. Naturally, games have their own set of required skills. Hence, some skills might be relevant for one game but not for another. Different games require different skills (Norman, 2011).
Fabricatore (2000) suggested a basic interactive cycle of four important phases of digital game playing, which provide opportunities for learning. A player starts a cycle with gathering information regarding the gaming world (Phase 1), then analyzes the gathered information (Phase 2), subsequently makes decisions based on the conducted analysis (Phase 3), and finally changes the status of the gaming world (Phase 4). Changing the gaming world leads to the start of a new interactive cycle. Therefore, Fabricatore emphasized the importance of managing ambiance and functional information. “Ambiance information encompasses merely perceptual elements that contribute to create an atmosphere capable of drawing and maintaining [a] player's attention on an emotive basis” (Fabricatore, 2000, p. 8). Ambiance information contributes to a player's feeling of being part of the gaming world and the player's immersion into the gaming world. “Functional information is inherent to whatever is needed to understand and control the game-play (i.e., what can be done during the game-playing, and the purposes to do it)” (p. 8).
Fabricatore (2000) also elaborated how opportunities for learning are linked to the phases of the cycle. In the first phase of the cycle, a player undertakes learning processes that contribute to the development of insight, visual-spatial perception, strategic thinking, and logical reasoning as a result of using these abilities or skills to gather actively relevant information about the gaming world. In addition, part of the gathered information could be educationally relevant information depending on the game content. In the second phase, a player is required to analyze the information gathered, which is an opportunity to develop analytical capabilities as part of reasoning processes. While game playing, “analyzing information means at least interpreting available data and identify relationships with previously acquired information in order to draw conclusions regarding the status of the gaming world” (Fabricatore, 2000, p. 11–12). The third phase is related to making decisions to approach the game goal. Making decisions in the game play usually implies managing risks and resources and considering the game rules, which in turn stresses the importance of strategic thinking and the opportunity to train this type of thinking. In the final phase, a player interacts with the gaming world by using the input interface. The need for psychomotor skills in this phase is important as the pace of the game challenges the player, which in turn is relevant for the psychomotor development of the player. In addition, performing the planned actions leads to feedback about the correctness of the decision. The feedback then gives an opportunity to refine skills or abilities and thus improve the player's mastery of the game.
Cognitive Load Theory
As Norman (2011) demonstrated, digital game-based learning environments are often complex, which place demands on the player's working memory processes. If the demands and the corresponding cognitive working memory resources serve the educational learning objectives, then learning is likely to occur. However, if the demands fail to serve the intended educational processes, then learning might be disrupted or even prevented. For example, when learners are required to acquire factual knowledge or content understanding, the necessity to learn how to interact with the gaming world might hinder the intended learning process. The usage of working memory resources is one of the focuses of Cognitive Load Theory (CLT).
CLT is based on four pillars: (1) working memory with limited capacity, (2) long-term memory with unlimited capacity, (3) schemas in long-term memory, and (4) automation of schemas. CLT emphasizes the role of working memory in learning and the problem of occupying working memory by useful and unnecessary cognitive processes, that is, cognitive loads (de Jong, 2010; Sweller, 2010; Martin, 2014). The cognitive processes executed in working memory are thought to determine the effectiveness of learning. Only when information processing occurs without disturbances can learners build an adequate schema that will be stored effectively in long-term memory.
Working memory has a limited capacity, which allows processing of only a few information elements at a time. If rehearsal of memory items is not possible, items fade within seconds. Learning complex tasks is often difficult because of the working memory limitations. Learners must hold numerous elements (e.g., rules and states) in working memory while relating them, which often exceeds working memory capacity. The components of cognitive load are intrinsic, germane and extraneous loads. Intrinsic load is commonly assumed to be created by the difficulty of the subject matter related to the number of elements to be learned and their interactivity (de Jong, 2010). Intrinsic load is the basic amount of processing required for understanding a presentation. Germane load goes beyond understanding and is bound to processing information used to build schemas and store them in long-term memory (de Jong, 2010). Extraneous load is assumed to result from the presentation manner of the material and is often the main source that inhibits learning, because it is unrelated to the construction or automation of schemas (de Jong, 2010). Successful learning occurs when working memory capacity is not overburdened by overall cognitive load and when as much capacity as is available can be dedicated to schema acquisition and automation, which creates germane load. Cognitive overload is mostly created by extraneous and intrinsic load.
Long-term memory plays a special role for learning and higher cognitive processes and is beneficial for working memory in the sense that the quality of the existing knowledge and knowledge structures in long-term memory influences the quality of information processing in working memory, which in turn reduces working-memory load. Within CLT, this efficiency in processing is due to schemas. Sweller (1999, p. 10) defines a schema as a “cognitive construct that permits us to treat multiple elements of information as a single element categorized according to the manner in which it will be used.” Pollock et al. (2002; p. 63) later defined a schema as “a cognitive construct that organizes elements of information categorically and stores them in long-term memory.” Schemas represent interacting elements (i.e., elements and their interrelations; Pollock et al., 2002) and exist for flora (e.g., flowers and trees) and fauna (e.g., cats and dogs), objects (e.g., car, letters, and words), situations (e.g., danger and dating), and abstract concepts (e.g., democracy and square root). Schemas are handled in working memory as information units regardless of their complexity. “With expertise, interacting elements that would otherwise overwhelm working memory can be incorporated in a schema that acts as a single element and imposes a minimal working memory load” (p. 63). Possessing such schemas is the learner's solution to managing the problem of working memory load. When working memory processes one schematic element, it concurrently processes multiple sub-schematic interacting elements. Elements that are not bundled in a schema are most likely to overwhelm working memory. Hence, building adequate schemas is an instructional goal in helping learners. Once schemas are built, they can be perfected with automation. Automation of schemas is when the processing of the schemas occurs with minimal or no conscious effort. Thus, the automation of schema processing puts only minimal load on working memory and thus discharges it. The freed capacity can therefore be used for other processes that would not be possible when learners are in a novice state. Automation is important for expertise as well as schemas.
Cognitive Load Theory and Motivation
The CLT is not explicitly related to motivational aspects, although researchers implicitly assume a minimum of motivation to enable learners to attend to relevant information and to construct schemas (Moreno and Mayer, 2007; Schnotz et al., 2009). Recently, researchers have explored the link between motivational aspects and CLT (e.g., Schnotz et al., 2009; Ismail et al., 2013). Some researchers consider motivation as an expander of working memory capacity, although the general consensus is that working memory is an entity with a specific limited capacity for processing information (Miyake and Shah, 1999). Evidence also exists for a higher level of motivation resulting in a temporary increase in working memory capacity within some limits (Schnotz et al., 2009). A larger body of research has focused on the effects of motivation on the three types of load, especially germane load.
Motivation is especially assumed to regulate invested mental effort and the assigned memory resources to accomplish a task (Vollmeyer and Rheinberg, 2006; Schnotz et al., 2009). The role of motivation in the allocation of memory resources, however, is unclear and needs to be further explored (de Jong, 2010; Ismail et al., 2013). Motivation has been suggested to be responsible for allocating working memory resources to germane load, such as in the construction and automation of schemas (Moreno and Mayer, 2007), but motivation could also be a causal factor in the persistence of learning under high cognitive load or even overload due to intrinsic and extraneous load. Using strategies to counteract extraneous load and to manage intrinsic load, given the present context of learning and instructional presentation, could stem from motivation and invested effort into learning (Van Merriënboer and Ayres, 2005; Moreno, 2006; Orvis et al., 2008). Motivation is primarily discussed in relation to germane cognitive load (Ismail et al., 2013). Ismail et al. (2013) noted that “motivational factors, such as challenge, anxiety, interest, and probability of success can encourage or discourage students to engage in germane learning processes” (p. 329). Among these concepts, student interest and beliefs (e.g., self-efficacy) have been shown to determine the allocation of the necessary cognitive effort (Fisher and Ford, 1998; Hidi and Renninger, 2006; Moreno, 2006; Renninger and Hidi, 2011). Numerous studies have reported that a higher level of interest or motivation in a learning task is related to a higher level of invested cognitive effort (Fisher and Ford, 1998; Paas et al., 2005; Hidi and Renninger, 2006; Moreno, 2006).
Intrinsic motivation is connected to high-quality learning (Ryan and Deci, 2000). In general, positive correlations between motivation and performance have been reported (Fredericksen et al., 2000; Ali and Franklin, 2001; Aragon et al., 2001; Sankaran and Bui, 2001; Delialioglu, 2005; Waschull, 2005; Yukselturk and Bulut, 2007; Artino, 2008). A higher level of intrinsic motivation might make learners invest more resources in learning and particularly process information more deeply, thus contributing to successfully passing tests (Lazarević and Trebješanin, 2013; Lee, 2013; Yurdugül and Menzi Çetin, 2015). Research on self-regulated learning and learning strategies discuss deep and surface learning approaches, which are characterized by motives and strategies (Lee, 2013). These approaches refer to usage patterns of learning strategies that learners show when performing a specific learning task (Lee, 2013). Correspondingly, learners performing surface learning are more likely guided by extrinsic motives and strive for passing a course with minimal effort, whereas learners performing deep learning are more likely guided by intrinsic motives and strive for comprehension (Baeten et al., 2013; Lee, 2013; Laird et al., 2014). Deep learning was shown to be correlated with higher academic performance, whereas surface learning correlated negatively with academic performance (Lazarević and Trebješanin, 2013). The corresponding pattern of results could also be found with online and distance performance (Lee, 2013; Akçapinar, 2015; Yurdugül and Menzi Çetin, 2015). Considering the components of learning approaches, that is, motives (deep and surface motives) and learning strategies (deep and surface strategies), similar patterns of correlations with performance have been found for motives and strategies. Deep motives and strategies correlated positively with performance, and surface motives and strategies correlated negatively (Lazarević and Trebješanin, 2013; Lee, 2013; Akçapinar, 2015; Yurdugül and Menzi Çetin, 2015).
Effects of Game-Based Learning: Motivation, Cognitive Load, and Performance
Motivational effects of game-based learning have been studied less than cognitive outcomes (Li and Tsai, 2013; Wouters et al., 2013). For example, Connolly et al. (2012) reviewed 129 studies and identified 33 among them that reported motivational and affective effects of entertainment games (n = 26), learning games (n = 5), and serious games (n = 2). Of these, 18 high quality studies about entertainment games (n = 14), learning games (n = 4), and serious games (n = 0) were summarized. Results for learning games on motivation (n = 3) were mixed showing positive or no effects on motivation (Huizenga et al., 2008; Wijers et al., 2008; Fu et al., 2009). Divjak and Tomic (2011) investigated computer games to promote mathematics learning and found an overall positive impact on motivation. Wouters et al. (2013) conducted a meta-analysis on 38 studies about serious games from 1990 to 2012. Serious games were more effective in terms of learning (immediate measure of knowledge and cognitive skills; d = 0.29; 77 pairwise comparisons with n = 5,547) and maintenance of learning gains (delayed measure; d = 0.36; 16 pairwise comparisons with n = 499), but they were not significantly more motivating (d = 0.26; 31 pairwise comparisons with n = 2,216) in comparison to conventional learning methods.
Connolly et al. (2012) identified 32 among 129 studies that reported effects of entertainment games (n = 3), learning games (n = 26), and serious games (n = 3) on knowledge acquisition and content understanding. They summarized high quality studies about entertainment games (n = 3), learning games (n = 12), and serious games (n = 2) in their review. They reported that overall game-based learning is linked to positive effects on knowledge acquisition and content understanding, but they also noted that only few random control studies exist of which the results are mixed (e.g., Connolly et al., 2012). The effectiveness of game-based learning has also been reviewed in several other studies (Vogel et al., 2006; Divjak and Tomic, 2011; Young et al., 2012). Vogel et al. (2006) concluded in their meta-analysis that students who used computer games or interactive simulations showed higher cognitive gains than students who were instructed in a traditional way. Divjak and Tomic (2011) investigated whether computer games promote mathematics learning and found positive impacts on learning outcomes. Young et al. (2012) also reported positive effects of video games on language acquisition, history, and physical education.
Research on motivation, cognitive load, and performance (especially retention and transfer performance) based on CLT or related theories (e.g., the Cognitive Theory of Multimedia Learning; Mayer, 2014) that compare digital game-based methods with conventional media instruction methods (e.g., lectures, reading, drill and practice, or hypertext learning environments; see Wouters et al., 2013) are also rare. Clark et al. (2016) conducted a meta-analysis on digital game-based learning for K-16 students and reported from media comparison studies an improvement of learning in favor of games (d = 0.33; 57 comparison studies). Mayer (2014) evaluated qualified so-called media comparison studies in which an educational game was compared to a more traditional instructional approach in the sciences. He only identified 16 studies that met his rigorous scientific study criteria (e.g., having an experimental design with a control group, teaching the same academic content, measuring academic outcome as a dependent measure, reporting relevant descriptive statistics like M, SD, and n). He reported that 12 of the 16 studies showed superior learning for the games group (median of d = 0.69, using various outcome measures of performance). Most studies have reported higher retention performance for game-based learning (Moreno et al., 2001; Evans et al., 2008; Barab et al., 2009; Brom et al., 2011; Hwang et al., 2012), whereas other studies have reported no effects (Parchman et al., 2000; Hickey et al., 2009; Wrzesien and Raya, 2010) and lower performance (Swaak et al., 2004; Adams et al., 2012). Correspondingly, game-based learning groups outperformed control groups on knowledge transfer, problem solving, procedural knowledge and comprehension (Ricci et al., 1996; Moreno et al., 2001; Evans et al., 2008; Barab et al., 2009; Anderson and Barnett, 2011; Brom et al., 2011; McLaren et al., 2017), whereas no effect (Hickey et al., 2009) and lower transfer performance (Swaak et al., 2004; Adams et al., 2012) were rarely reported. The reported motivation and enjoyment were also higher with games (Ricci et al., 1996; Moreno et al., 2001; Wrzesien and Raya, 2010; Hwang et al., 2012; McLaren et al., 2017). Results from studies measuring cognitive load have been mixed. Some studies have found a higher cognitive load with games (Adams et al., 2012) and others having shown no effects (ratings of difficulty and comprehensibility; Moreno et al., 2001; McLaren et al., 2017). In addition, studies have shown that learning took longer when game-based (Evans et al., 2008; Adams et al., 2012; McLaren et al., 2017). Mayer (2014) also reported that four of the five studies showed superior second-language learning for the games group (median of d = 0.96), three of five in learning mathematics (median of d = 0.03), three out of three studies in learning language arts (median of d = 0.32), and two out of three in learning social studies (median of d = 0.62). These studies represent a media comparison approach to game research (Mayer, 2011).
Gaming Expertise and Language Comprehension
In general, Tobias et al. (2014) articulate that research on learner characteristics and their role in game-based learning is sparse and more research on this topic is needed. Gaming expertise and language comprehension abilities are two learner characteristics that are proposed to mainly affect extraneous cognitive load when learning. Extraneous load arises from the presentation manner of the material, including presentation codes, sensory modalities, dynamics, information structure, and presentational handling. Extraneous load is therefore dependent on presentation design and thus depends on instruction designers. Correspondingly, the instructional language (i.e., the verbal code) is a feature of the presentational design. Therefore, both gaming expertise and language are potential sources of extraneous load. Extraneous load is thought to be detrimental to learning because it is not involved in the construction or automation of schemas. For example, a lack of language comprehension abilities and gaming abilities might raise extraneous load. Sweller (1999) assumed that effects of extraneous load are more likely to occur with high intrinsic load; otherwise, the extraneous load is unlikely to lead to learning problems as long as the capacity of working memory is not exceeded. Extraneous and intrinsic load are additive in that way (Paas et al., 2003).
In game-based learning, the gaming process is thought to be a source of extraneous load because a player must first learn how to play the game, which competes with resources needed for learning the subject (Adams et al., 2012). Evidence for the effect of a lack of gaming experience on extraneous load was reported in the study conducted by Virvou and Katsionis (2008) in which novice players wasted time learning to navigate a game. Orvis et al. (2006, 2007, 2008) also reported that game experience is connected to better game performance and a higher level of perceived ease of game use. Games that are difficult to play can impose a high cognitive load on working memory, which limits the capacity available to construct schemas. Hence, learning might be hindered by the need to play a game. Researchers have suggested that gaming processes should be kept manageable when focusing on the gaming environment (i.e., the gaming environment should have high usability; International Organization for Standardization, 2006; Prümper and Hurtienne, 2007), and learners should get instructional support in form of guidance (Virvou and Katsionis, 2008). Another suggestion is to provide educational games only for learners with gaming expertise. Learners with gaming expertise might not suffer as much from gaming load because they can use their more or less automated gaming schemas to control the game environment. Without the expertise and the aid of automated schemas, gamers might experience excessive load because of the need to learn to play the game. In sum, gaming expertise is expected to reduce extraneous load caused by gaming but not at the expense of intrinsic or germane cognitive load, given that the skill components necessary for playing a game (see Norman, 2011) do not overlap with the skills to be learned or trained (Tobias et al., 2014). In addition, Orvis et al. (2008) reported that game experience was positively correlated to motivational aspects like task self-efficacy and self-set performance goals. In contrast, Anderson and Barnett (2011) reported no effects of gaming expertise on learning success.
An additional source of extraneous load is the instructional language. Not speaking the same primary language and relying on English is common in international learning settings, for example, at universities where the lack of language proficiency could add extraneous cognitive load to the difficulty of the learning task. When language comprehension is difficult, causing high cognitive load on working memory, less capacity is available to construct schemas. Hence, learning might be hindered by the need to understand a foreign language. Consequently, instructional texts should be kept simple when addressing the learning environment, or learners should have language comprehension expertise when addressing learner characteristics. Learners who can comprehend the language might not suffer as much from language load because they can use their more or less automated language comprehension schemas to understand the instruction. Without these schemas, they might experience excessive cognitive load because of comprehension difficulties. In sum, good language comprehension abilities are mainly expected to reduce extraneous load but to not affect intrinsic or germane cognitive load as long as the skills needed for comprehension do not overlap with the skills to be learned or trained.
Research Objectives and Expectations
Research has shown that games of all types affect motivation and cognitive load (e.g., Schrader and Bastiaens, 2012; Woo, 2014), two important factors that influence learning. The structure of games is capable of engaging people and in extreme cases even pushing them into addiction (Kuss and Griffiths, 2012). Yet, a game's structure and complexity create a high level of cognitive load (Keller, 2008; Schrader and Bastiaens, 2012; Woo, 2014; Nebel et al., 2015). Media comparison studies investigate the extent that motivation, cognitive load when learning and performance are influenced by using an educational game. Accordingly, we used the educational game CellCraft and compared it to hypertext instruction covering the same content.
Motivational effects of game-based learning are not studied as much by researchers as cognitive outcomes and have been mainly studied with entertainment games (Connolly et al., 2012; Li and Tsai, 2013; Wouters et al., 2013). In addition, mixed results of educational games on motivation have been reported, and level of motivation has been mostly rated after learning retrospectively (Huizenga et al., 2008; Wijers et al., 2008; Fu et al., 2009; Divjak and Tomic, 2011; Wouters et al., 2013). Furthermore, motivation has often been measured on a general level in research that focused primarily on cognitive aspects of learning. The various factors of motivation were mostly not assessed to the extent that would provide deeper insights into motivation. In the present study, the following four factors of motivation in learning situations were assessed before learning: anxiety about failing, challenge, interest, and probability of success1. Interest was also assessed after learning to analyze changes over time. Various assessments of interest have been regularly used in previous studies, which provides a basis for comparisons to the literature.
The literature review led to the following two hypotheses:
Hypothesis 1: Learners intending to play an educational game will experience a higher level of anxiety, challenge, and interest but a lower level of probability of success than learners intending to learn with a hypertext instruction.
Hypothesis 2: Learners having played an educational game will experience a higher level of interest than learners having studied a hypertext instruction.
In general, the reviewed literature had shown that educational games are cognitively challenging and therefore regarded as more effortful than other forms of instruction. Nevertheless, they often proved to be effective tools in imparting knowledge and skills. Based on the literature review the following hypotheses were tested:
Hypothesis 3: Learners playing an educational game will experience a higher level of cognitive load than learners studying a hypertext instruction.
Hypothesis 4: Learners playing an educational game will outperform learners studying a hypertext instruction on knowledge retention and transfer.
Tobias et al. (2014) emphasized that more research on learner characteristics is needed. Hence, we wanted to explore whether learners could also benefit from instruction when they have only minimal gaming experience or the instructional language is not their mother tongue. Not speaking the same primary language but English is quite common in international learning settings. It might add some (extraneous) cognitive load to the challenge (difficulty) of the learning task in general and thus influence the gaming experience as well as learning outcomes.
Hypothesis 5: When playing an educational game, successful learners will possess a higher level of gaming experience and are better in English comprehension.
Methods
Sample
Thirty-nine students [30 women and nine men; M = 23.9 years, SD = 1.80; age range = [19–28]] from the University of Regensburg (Universität Regensburg; n = 36) and the East Bavarian Technical University Regensburg (Ostbayerische Technische Hochschule Regensburg; n = 3) participated in this experiment. The average semester of study was 8.18 [SD = 2.48; semester range = [1–12]]. Overall, students were enrolled in 23 different subjects covering natural sciences and liberal arts of which 16 were studying their degree course that ends with a state examination (15 students studied to become teachers and one chemistry), six students were enrolled in a BA and 17 in an MA degree course. None of the students studied biology. All students' native language was German. Furthermore, no significant differences were found between the two treatment groups on sex (Fisher's exact test, p = 0.72), age (p = 0.43), semester of study (p = 0.73), and type of degree (Fisher's exact test, p = 0.32).
Seventeen of the 39 students (43.6%) reported that they play computer games, 22 reported that they did not (56.4%). All but one male student played games (88.9%) whereas only nine of 30 female students (30.0%) played. The players favored playing strategy games (n = 11), action and jump'n'run games (n = 8), shooter games (n = 6), simulations (n = 3), and learning games (n = 1). Ten students reported playing up to 2 h per day (58.8%), six students between 2 and 4 h (35.3%), and one student between 4 and 6 h (5.9%). The non-gaming students reported a lack of interest (n = 19) and a lack of time (n = 7) that prevented them from playing.
We recruited students from the joint campuses of Regensburg University and the East Bavarian Technical University of Regensburg. Despite our attempt to obtain a diverse sample of students from a broad range of study subjects, gaming expertise, and English expertise, we could not assume representativeness of the sample with respect to the population of students in Regensburg or the general population. Nevertheless, by assigning participants randomly to the treatment groups, we assumed the variance in the two groups would be the same, allowing for a reasonable comparison of treatments. The explorative analyses of the relations between gaming expertise and English expertise with motivation, learning experience, and success of learning is quite biased by the non-random sampling method, but the gaming and English expertise data from the present study is similar to data collected, for example, from psychology or pedagogy students at Regensburg University. Moreover, confronting students with unexpected instructional themes and methods is a typical aspect of learning for novices in any field of study at the university. Thus, we considered the conducted gaming and hypertext instruction to be adequate to investigate the research question.
For descriptive purposes, participants were asked at the beginning of the experiment about their experience with multimedia educational games and learning environments and with reading and comprehending English texts in their studies, the ease at which they can comprehend English texts, and about whether they previously attended courses about cell biology. In addition, they rated their level of domain-specific knowledge and current interest in cell biology. Table S2 of the Supplementary File lists the English translations of the German items, and Table 1 shows the descriptive statistics. Overall, the sample reported to be less experienced in using e-learning environments and educational games, but English comprehension was rated to be fairly easy, given that the reading and comprehension of English texts is a regular feature of studies. Students also regard themselves as having only little prior knowledge about cell biology, and correspondingly most students had not attended any university course on this subject. On average, they were moderately interested in the subject of cell biology.
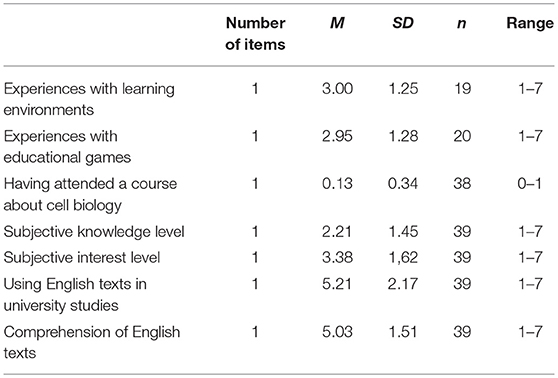
Table 1. Characteristics of the students' subjective ratings of their learning experience, prior knowledge, interest, and comprehension of English texts.
Design and Procedure
Before starting the experiment, the students were randomly assigned to a hypertext and a game learning group. At first, the students received the instruction about the procedure of the experiment and the learning task, which included the introduction of either the educational hypertext or game. The participants then provided demographic data and responded to questionnaire items that assessed domain-specific knowledge, experience with gaming, biology in general and English texts, and their current motivation to learn. Afterwards, the participants had 1 h to work through the hypertext or to play the game. While learning or gaming, the students were asked three times to rate their cognitive load. A final questionnaire was administered after the 1 h that assed participants' knowledge, their interest, and the experienced cognitive load while learning or gaming.
Description of the Training Material
CellCraft Game
We used CellCraft v. 1.0.1 for gaming based on the following two criteria: (1) the game should ensure a high level of ecologically validity and should be web-based so that everybody can access it. (2) The game should also have a high standard of quality in terms of programming, creativity, and factual accuracy. The game is developed by Carolina Biological Supply Company in the U.S., which has been offering science teaching materials since 1927 throughout the world. CellCraft is playable in full-screen mode without advertising, which could otherwise bind working memory resources and thus disturb learning and research on learning games. The game was retrieved from http://www.silvergames.com/cellcraft. It is programmed to work with Adobe Flash.
CellCraft consists of eight consecutive game levels in which the user applies the knowledge learned at each level to help a cell survive. The tasks of the levels are shown in Table 2. The plot of the story is revealed to the player through short animated scenes between levels (see Figure 1). The cell is the central theme in an animated story about the rescue of the platypus species on the planet Monotremes, which is in threat of being destroyed by a meteorite. The platypus species can only be rescued by sending a platypus cell to another planet and using it to generate a new population of platypuses, which is the task of the two platypus scientists, Sydney and Spike. They are introduced shortly after the start of level 1 but are referred to as mysterious figures and do not appear until the transition video between levels 1 and 2 when the background story is introduced (see Figure 1). The player's challenge is to support Sydney and Spike in their mission. The player is required to create a viable cell and protect it against various dangers like viruses, cold temperatures, and starvation on its way through space (see Table 2, Figure 2). To protect the cell effectively, the player should apply learned information about cell organelles and how they operate.
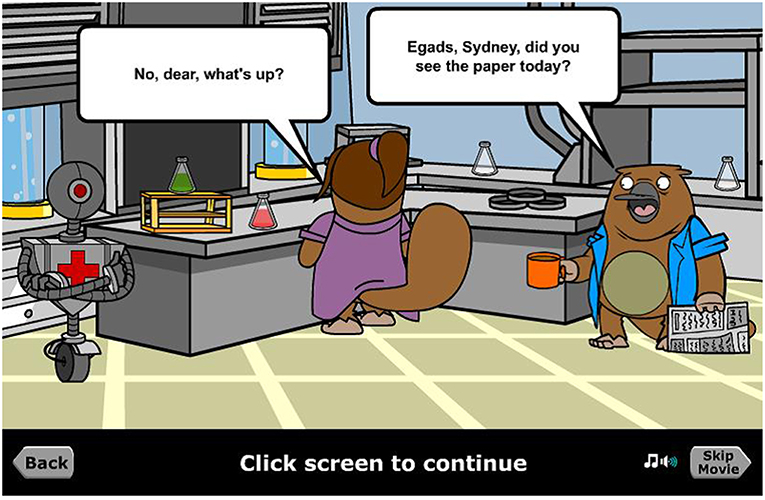
Figure 1. The screenshot shows a scene from the animated story that is used to reveal the plot during the transition between game levels (with permission of Carolina Biological Supply Company). The screenshot was taken from the transition between Level 1 and 2.
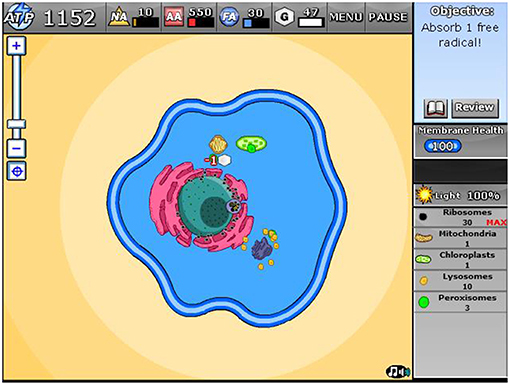
Figure 2. The screenshot shows a scene from Level 5 with the presentation of the current objective in the upper right-hand area and the available resources in the lower right-hand area (with permission of Carolina Biological Supply Company).
Players must learn how to apply their knowledge and transfer it to the game events. While controlling the cell throughout the different levels, reactions from the cell's environment informs players of their success of correctly applying the knowledge about cells. Each level of the game requires the player to use the knowledge gained in preceding levels. Therefore, with every mastered level, they are expected to improve in applying their knowledge and hence their gaming ability.
While fighting for the cell's survival, the player learns about the rules and regularities of the game. By defending against viruses and searching for resources for maintaining the cell metabolism, the player learns about the biochemical processes of the cell and the functions of the organelles that are involved in the various processes. After each attack of the viruses, the players receive feedback about their performance by showing a grade according to the American letter grading system (A to F) and by displaying how many viruses have multiplied in the cell or escaped. Performance evaluation is not transparent to the player. That is, the relationship between grades and game play or virus activity is not clear to the user. At the end of each level, the performance of the player is additionally evaluated by a grade and the time needed to finish the game level. No social comparison data are provided, for example, leaderboards or rankings of other players. Game difficulty is equal to all players and cannot be adjusted by the player. Successfully played game levels can be replayed, but players cannot skip a level.
Verbal information is presented in English as on-screen text. Specialist terms are used, but overall, the text is simple. The only audio in the game is background music, which changes when dangerous situations appear (e.g., viruses attacking the cell) and transitions between game levels are operated. Players can turn off the background music at any time. The transition videos can also be skipped.
After starting the game, the screen layout and functions are slowly introduced to the player by guiding attention to screen areas and functions and providing on-screen text explanations. The player can discover functions without the aid of the game. At the beginning, the game also provides strategy tips, for example, how to economize resources like the cell fuel Adenosintriphosphat (ATP). Strategy tips are mainly suitable for players having little experience with strategy games. The frequency of hints and strategy tips decrease with game progress. If the platypus cell gains a new organelle, the information is given. All types of information about screen areas, functions, strategies, and cell organelles are mainly given by on-screen text presented in a window in the middle of the screen. The explanations of cell organelles also includes illustrations and animation.
Hypertext CellCraft
The control group learned with a hypertext environment that provided the same content as the CellCraft game and approximated the amount and the duration of the text (see Figures 3, 4). Technically the hypertext was developed with PowerPoint. Users navigated by clicking on hypertext links that were blue, bolded, and underlined (see Figure 4). Additionally, a navigation menu allowed returning to the previous slide via an arrow button and to the slide map via a button depicting a house (see Figure 4). The information was sequenced to correspond with the game levels of CellCraft, starting with basic concepts and processes and then progressively providing more specific and elaborated information. The pictures, videos, and audios used in the hypertext version were taken from CellCraft. The hypertext comprised five modules (see Figure 3), whereas the game offers eight game levels. This difference is due to the game levels 6–8, which offer little relevant, new information but only elaboration through gaming. The only relevant information found in level 6 was integrated in one of the five hypertext modules. A click on the compass button presents an overview of the content. The learner has more control over sequencing and pacing of the information compared to CellCraft. The hypertext was designed without game elements and similar arousing elements.
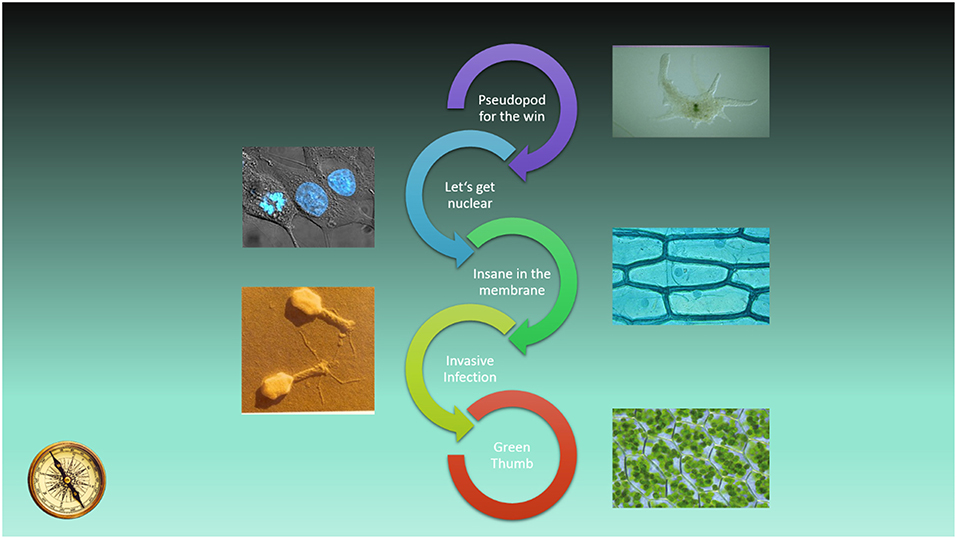
Figure 3. The screenshot shows the menu of the hypertext presenting its five sections (the six images are licensed for reprint or used with the permission of the copyright holder; Anonymous, 2007, 2011; Peters, 2006; TenOfAllTrades at English Wikipedia, 2007; Yuuji, 2010; Seilnacht, 2013). The circled arrows indicate the sequence of sections. The compass in the lower left-hand area of the screen leads to the content overview.
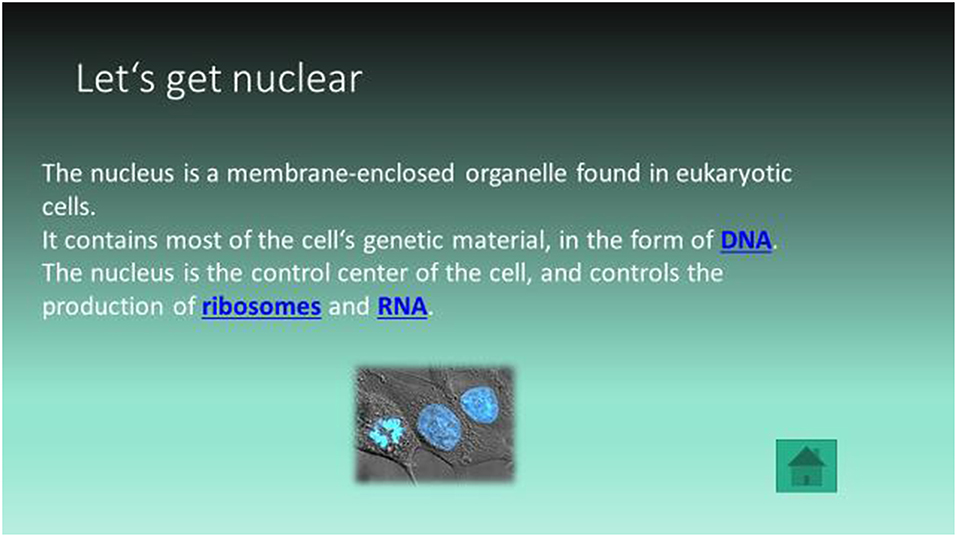
Figure 4. The screenshot was taken from the second section of the hypertext showing blue hyperlinks (the image is licensed for reprint or used with the permission of the copyright holder; TenOfAllTrades at English Wikipedia, 2007). The house button leads the learners back to the menu page (the button is our own creation).
Means of Measurements
The Supplementary File presents the questionnaires used in the study. Tables S1–S5 of the Supplementary File list the English translations of the German items of the measurement scales and other items, Tables S6–S10 list the German items (see Supplementary Material section). Table 3 presents the features of the measurement scales (number of items, M, SD, score range, number of subjects, Cronbach's alpha).
Prequestionnaire
The students were asked about their sex, age, mother language, and studies (type of university, major or area of study, degree program, and current semester).
The domain-specific prior knowledge was measured by 10 single-choice questions, six questions assessing factual knowledge and four questions assessing comprehension of processes. Each question comprised four alternative answers and an option “I don't know.”
Participants then reported and rated their experience with computer games (four questions) and learning environments (one question). In addition, students rated their experience with cell biology in general (two questions), their current interest in cell biology (one question) and their experience with reading English texts (one question) and their ease of comprehension of English texts (one question).
Finally, the students' current motivation was measured by the Questionnaire on Current Motivation (QCM) of Rheinberg et al. (2001), Freund et al. (2011). The questionnaire assesses four factors of current motivation in learning situations: anxiety (five items), challenge (four items), interest (five items), and probability of success (four items). Items were rated on 7-point scales from disagree to agree.
Questions While Learning
Three times while learning, the students rated their current level of cognitive load by placing a check mark on a 7-point scale from very, very low to very, very high. Students were instructed to pause learning when an audio signal was given and to immediately rate their level of cognitive load.
Postquestionnaire
After learning, only the game-based learning group was required to report the number of trials they needed to finish a game level. Then performance was assessed by using the pretest of domain-specific prior knowledge. Subsequently the students' interest was rated again by the interest scale of the QCM (already used in the prequestionnaire) of Rheinberg et al. (2001). Finally, the students reported their level of domain-specific knowledge after learning and again their level of cognitive load while learning retrospectively on a 7-point scale from very, very low to very, very high.
Annotation to Analyses
Means of items were calculated for prior knowledge, current motivation (i.e., anxiety, challenge, interest, and probability of success), and performance. Cognitive load and level of knowledge after learning were measured by one-item subjective ratings. A high score expresses a higher level of the feature. In addition, prior knowledge and performance scores were rescaled as a percentage of correct answers.
Results
Motivation
The learning groups differed significantly in experienced interest, anxiety, and challenge but only marginally in probability of success (see Table 4). The gaming group was more interested before the learning phase but also more anxious about failing. They also felt more challenged by the educational game. The gaming group also rated its probability of success lower than the hypertext group, but unexpectedly no differences were found between the groups (resulting also in a small effect size of d = 0.43). After the learning phase, the groups still showed about the same levels of interest. Thus, nearly the same difference in motivational levels between groups was found. This pattern of results is also reflected by the non-significant effect of time and the non-significant interaction effect of the repeated measurement ANOVA. All learning group effects analyses revealed large partial eta-squared effect sizes. Thus, Hypotheses 1 and 2 could be confirmed.
Cognitive Load
The repeated-measures ANOVA revealed two different courses over time of cognitive load while learning between the learning groups (see Table 5). This pattern is reflected by a significant interaction between the learning group and time and the non-significant main effects of group and time. The level of self-reported cognitive load increased in the gaming group from the first measure after 15 min of gaming to the second measure after 30 min and then stayed at the same level, whereas the course of cognitive load for students in the hypertext group showed the reverse pattern. Cognitive load stayed at the same level for the first and second assessment and then decreased to a lower level. The experienced cognitive load with hypertext learning is noteworthy because it was higher than with gaming for the first two measures and then changed order. Ratings of cognitive load after learning showed no difference between learning groups. Therefore, Hypothesis 3 could not be confirmed.
Performance
The repeated-measures ANOVAs of retention and transfer performance showed similar results. Students in both learning groups improved their knowledge (see Table 6; significant effects of time), but the hypertext group outperformed the gaming group. Students in the hypertext group started at an equal level of prior knowledge in terms of prior retention performance and prior transfer performance, and then they descriptively gained more knowledge than the gaming group (see Table 6) as indicated by the interaction effect of time and learning type on retention performance that became significant and the interaction effect on transfer performance that remained non-significant.

Table 6. Descriptive statistics and the test results of the retention and transfer performance analyses.
We considered whether the students in the gaming group could reach level 5 of the game, given that all relevant information (tested in the performance test) could have been already gained from level 1 up to the beginning of level 5. Table 7 summarizes the number of trials needed and the number of students who completed each level and the highest game levels achieved. Four out of the 20 students reported not having reached level 5. The higher the game level, the lower the completion rate within the 1 h learning time. Calculating repeated-measures ANOVAs of retention and transfer performance entering only the gamers who completed level 4 replicated the result shown above (see Table 8). Thus, overall, Hypothesis 4 could not be confirmed.

Table 8. Descriptive statistics and the test results of the retention and transfer performance analyses for learners who completed Level 4.
Gaming Experience and English Comprehension Skills
Inspecting the descriptive statistics of the gaming group on ratings of English comprehension ability and gaming experience shows differences between students who completed and failed Level 4 (see Table 9). Overall, the failing students seem to have had less experience in gaming and in comprehension of English texts.

Table 9. Descriptive statistics of the students' subjective ratings of their learning experience and comprehension of English texts.
English comprehension skills and gaming expertise scores each covaried in the expected directions with interest after gaming, cognitive load ratings (post-study measure), retention performance, transfer performance, and completed level (see Table 10). Overall, gaming expertise and English comprehension skills positively correlated with interest, performance, and completed level of gaming but negatively with cognitive load. The correlation between performance and gaming expertise was non-significant, which could have occurred because starters of game Level 5 had received all the necessary information for the performance test. In general, Hypothesis 5 could be confirmed.

Table 10. Correlations of learning experience and English comprehension skills with interest, cognitive load, performance, and completed level.
Discussion
The aim of the media comparison study was to investigate the extent that an educational game affects motivation, cognitive load while learning, and performance of students compared to a more traditional text-based hypertext instruction. Generally, we expected that using a game for learning should lead to higher levels of motivation, cognitive load, and learning outcomes. The results showed that the level of motivation before learning was already higher when students expected to learn while playing a game. They reported more interest and anxiety of failing, and they experienced a higher level of challenge and reported a tendency toward a lower probability of success than students in the hypertext condition. Students' interest was also higher after learning via the game. Unexpectedly, students reported the same level of overall cognitive load in the game and hypertext conditions, but the curves of load while learning differed. The gaming students reported a lower level of load at the beginning, but it raised afterwards and stayed on the same level until the end of the study period. In contrast, the hypertext group started with a higher level of load but decreased to a lower level until the final period of the study phase. Both types of instruction fostered retention and transfer performance, but the hypertext group outperformed the game-based group on retention. This result is essentially due to a subgroup of students in the game group who had less gaming experience and was worse in English text comprehension.
Motivation and Performance
We found evidence for the assumption that educational games have a high potential to motivate. The present study showed differentiated knowledge about motivational conditions of learners even before starting to learn. Students were more interested, experienced a higher level of challenge, had more anxiety of failure, and a tendency to report a lower likelihood of succeeding than students in the hypertext condition. These results of the motivation measures are noteworthy because motivation, and in particular interest, has been assessed after the learning phase and very often with one-item ratings in most published studies (Huizenga et al., 2008; Wijers et al., 2008; Fu et al., 2009; Divjak and Tomic, 2011; Wouters et al., 2013; Mayer, 2014). We also found that the level of interest after learning did not change in comparison to the level before learning for both hypertext and game-based learning. The results correspond to research on digital entertainment games and educational games (Huizenga et al., 2008; Wijers et al., 2008; Fu et al., 2009; Divjak and Tomic, 2011; Connolly et al., 2012; Li and Tsai, 2013; Wouters et al., 2013) and research on motivation (Divjak and Tomic, 2011, but not Wouters et al., 2013, and Connolly et al., 2012).
The motivational differences found between the game-based and hypertext learning groups did not result in superior performance of the game-based group. This finding is contradictory to the literature, which has often shown games to be effective tools in imparting knowledge and skills (Vogel et al., 2006; Divjak and Tomic, 2011; Connolly et al., 2012; Young et al., 2012; Wouters et al., 2013). This inconsistency can be explained by differences in gaming time and the number of training sessions among studies. Wouters et al. (2013, p. 251) raised the question of “whether a training of only one session is sufficient to ensure cognitive changes.” They suggested that games show their effectiveness “only after multiple training sessions in which the players get used to the game” (p. 251). In other words, the players must first adjust to the game procedures before deeper learning can result in better performance. The meta-analyses performed by Wouters et al. (2013) and Clark et al. (2016) support this point. They reported higher learning gains with multiple sessions for serious games than conventional instruction methods and that multiple sessions are more effective than only one session. The results of this study using a 1 h session also supports this claim. Some learners had difficulties with the game and therefore did not proceed as fast as the other users. Consequently, they could not process information provided in higher levels of the game and hence performed worse than students in the hypertext group. English skills also contributed to this result. Poor English skills might have contributed to worse comprehension of instruction and game play compared to students with adequate English skills. This influence of English skills and gaming expertise in addition to a possible interaction of skills should be considered in future studies that investigate game-based learning in a foreign language. Exploring which students and in which contexts educational games can be helpful is an important research focus for practical purposes, which can be addressed by investigating individual difference variables of students.
Cognitive Load and Mental Effort
The reported levels of cognitive load experienced while learning did not differ between groups using CellCraft and a comparable hypertext for learning. This result does not support the assumption that educational games are cognitively more challenging and therefore regarded as more effortful than other traditional forms of instruction. Hence, this result is also contradictory to literature (Keller, 2008; Schrader and Bastiaens, 2012; Woo, 2014; Nebel et al., 2015; Westera, 2015). The assumption is partly strengthened based on the finding that some students in the game-based learning group could not complete the necessary level that had presented the last relevant information of cell biology. This result can be interpreted that playing CellCraft was effortful and challenging, causing at least some students to proceed slower than expected because of gaming difficulty.
To explain the cognitive load results, two aspects must be considered. First, subjective ratings of cognitive load and mental effort are under criticism. As in this study, cognitive loads are often measured by one-item ratings (Sweller, 2010; Martin, 2014). de Jong (2010) summarized a multitude of issues related to subjective cognitive load ratings, especially when using one-item rating scales. He questioned the validity, reliability and sensitivity of the instrument, particularly the variation of scales used in self-report assessments (e.g., number of points, anchor terms), and how often and when self-report measures are used (e.g., during or after learning). Furthermore, scores are not consistently interpreted (e.g., as a high or low cognitive load score) and easily comparable across studies (e.g., when scores are associated with poor performance conditions in one study and with high performance conditions in another study). These aspects should be considered when interpreting results of this study. The one-item subjective ratings used in this study might simply be inadequate measures in the context of learning via games, which would make them a poor basis for the comparison of load scores. The second aspect is that game-based learning might rapidly bring students to the point at which their level of gaming skills meets the difficulty of the gaming task. This rapid skill development is assumed to be a precondition for experiencing flow, and students experiencing flow are not expected to rate their effort validly. In general, students experiencing flow are expected to underestimate their invested effort (Schnotz et al., 2009).
The reported load curves in the learning groups nevertheless reflect some plausibility. The gaming students reported a lower level of load at the beginning, which then increased and remained at the same level until the end of the study period. This pattern might reflect a normal flow of gaming with CellCraft. At the beginning, the cover story is set up and gamers are introduced to the game procedures (Level 1). The story and procedures are relatively easy at this point and are thus expected to not place much load on gamers. When the game becomes more difficult, cognitive load also increases. Given the equilibrium mechanism between skill level and difficulty of the game, it might be held relatively constant until the end of the game (or learning period). In contrast, the hypertext group started with a higher level of load that decreased to a lower level until the final period of the study phase. This curve could reflect the learning process adequately. Students using hypertext start from the beginning with information processing and knowledge construction given a fixed level of difficulty of navigating the hypertext. Then, when students reach the end of studying (i.e., they have worked on mostly all parts of the hypertext or have completed it and now spend the available time with reviewing information), the cognitive load decreases. Our findings point to this interpretation of load curves.
Limits
The present study is a media comparison study, comparing students who learned about cell biology using an educational game called CellCraft with students who learned the same content using hypertext. This method was chosen to investigate whether educational games offer learning advantages over traditional instruction. The criticism of media comparison studies by Clark (1994) is relevant. Clark criticized that media is often confounded with instructional methods in media studies and thus effects are not clearly attributable. Clark also stated that media are only vehicles for the instructional method which accounts for learning. To address this point, we ensured that at least the instructional content was equivalent and that two active learning situations were compared. This study provides no advice on how to design an educational game that fosters motivation and learning, which would be better achieved with a value-added approach.
The main limitation of the present study is the short duration of the gaming and studying time, which resulted in providing only a limited learning experience. Limiting learning to a 1 h session and letting non-English native speaking students play alone in groups constitutes a special learning environment, especially when using CellCraft. The results therefore cannot be generalized to other learning situations and users. CellCraft is an example of a real-time single-player strategy game (genre category) that uses a cover story and a simple mouse event play procedure. Nevertheless, our findings revealed the potential of games in motivating students. Furthermore, using subjective one-item ratings of cognitive load or mental effort in a gaming context, as in process assessments, was shown to be problematic. The results from one item does not seem to represent fluctuations of cognitive load well and may not provide a standardized comparison of media. Therefore, more advanced and objective measures of cognitive load should be applied (de Jong, 2010; Clark, 2012; Martin, 2014).
Future Perspective
We recommend that studies should investigate motivation more broadly and more comprehensively by focusing on the factors of motivation guided by theory. In addition, the course of motivation in single sessions as well as over multiple sessions should also be tracked, including assessments before gaming. This would help to clarify motivational effects of games and game design characteristics.
More adequate, objective measures of cognitive load should also be used. As with motivation, the variation of cognitive load in single sessions as well as over multiple sessions should be tracked. This would help to clarify cognitive load effects of games and game design characteristics.
We also recommend that gaming duration and the number of sessions should be included as a research focus to clarify when educational games are more effective (e.g., in terms of performance) or desirable (e.g., in terms of motivation) than traditional instruction. In addition, even when retention and transfer knowledge is regularly assessed in studies based on CLT, researchers should note that games seem to affect a broader range of learning outcomes. Thus, a game for learning might be provided for more educated learners, although the game may not be the most efficient method to reach the desired cognitive learning objectives. Instead, the game could be used to achieve other valued goals, such as motivational or emotional goals. This approach would imply a more detailed task analyses of games like Tobias et al. (2014) had suggested.
Finally, more research on learner characteristics is needed (Tobias et al., 2014). Although gaming expertise is recognized as a crucial characteristic, it is rarely investigated. Gaming expertise is particularly suggested to be a mediator of the effects of the number of gaming sessions and game design features on gaming processes, cognitive load while learning, and learning outcomes.
Data Availability
The datasets generated for this study are available on request to the corresponding author.
Ethics Statement
An ethics approval was not required per the guidelines of the Department of Educational Science, University of Regensburg and national regulations. For the study, no ethical issues were encountered. Students completed questionnaires and no misleading information about the goal of the study was given. The materials and procedures were also not invasive. In accordance with the guidelines of the Ethical Committee of the German Psychological Society, anonymity of data was ensured and all subjects gave written informed consent for data collection and analyses and data usage for scientific purposes.
Author Contributions
SS has developed the conception and design of the study and collected the data. KS has analyzed, interpreted the data, and written the paper.
Funding
This work was supported by the German Research Foundation (DFG) within the funding programme Open Access Publishing.
Conflict of Interest Statement
The authors declare that the research was conducted in the absence of any commercial or financial relationships that could be construed as a potential conflict of interest.
Supplementary Material
The Supplementary Material for this article can be found online at: https://www.frontiersin.org/articles/10.3389/feduc.2019.00018/full#supplementary-material
Footnotes
1. ^Probability of success refers to the participants' perception of their abilities and the difficulty of the task (Vollmeyer and Rheinberg, 2006). Vollmeyer and Rheinberg (2006) prefer the concept of a person's belief of being able to succeed in the task. Our usage of the term should not be confused with how the term is used in Classical Test Theory.
References
Adams, D. M., Mayer, R. E., MacNamara, A., Koening, A., and Wainess, R. (2012). Narrative games for learning: testing the discovery and narrative hypothesis. J. Educ. Psychol. 104, 235–249. doi: 10.1037/a0025595
Akçapinar, G. (2015). “Profiling students' approaches to learning through Moodle logs,” in Proceedings of MAC-ETL 2015 in Prague (Prague: MAC Prague consulting Ltd.), 242–248. Available online at: https://books.google.de/books?id=SkAmCwAAQBAJ&printsec=frontcover&hl=de#v=onepage&q&f=false
Ali, A., and Franklin, T. (2001). Internet use in the classroom: potentials and pitfalls for student learning and teacher-student relationships. Educ. Technol. 41, 57–59. Available online at: http://www.jstor.org/stable/44428684
All, A., Nunez Castellar, E. P., and van Looy, J. (2014). Measuring effectiveness in digital game-based learning: a methodological review. Int. J. Serious Games 1, 3–20. doi: 10.17083/ijsg.v1i2.18
Anderson, J., and Barnett, M. (2011). Using video games to support pre-service elementary teachers learning of basic physics principles. J. Sci. Educ. Technol. 20, 347–362. doi: 10.1007/s10956-010-9257-0
Anonymous (2007). Download PNG Image: Compass PNG. Available online at: http://pngimg.com/download/25569
Anonymous (2011). Biology Wallpaper. Available online at: http://editimage.club/newakc560426.html
Aragon, S. R., Johnson, S. D., and Shaik, N. (2001). “A preliminary analysis of learning style influence on student success in online vs. face-to-face environments,” in Learning for the Future, eds B. Cope and M. Kalantzis (Melbourne, VIC: Common Ground Publishing), 3–17.
Artino, A. R. (2008). Motivational beliefs and perceptions of instructional quality: predicting satisfaction with online training. J. Comput. Assist. Learn. 24, 260–270. doi: 10.1111/j.1365-2729.2007.00258.x
Baeten, M., Struyven, K., and Dochy, F. (2013). Student-centered teaching methods: can they optimize students? approaches to learning in professional higher education? Stud. Educ. Eval. 39, 14–22. doi: 10.1016/j.stueduc.2012.11.001
Barab, S. A., Scott, B., Siyahhan, S., Goldstone, R., Ingram-Goble, A., Zuiker, S. J., et al. (2009). Transformational play as a curricular scaffold: using videogames to support science education. J. Sci. Educ. Technol. 18, 305–320. doi: 10.1007/s10956-009-9171-5
Boyle, E. A., Hainey, T., Connolly, T. M., Gray, G., Earp, J., Ott, M., et al. (2016). An update to the systematic literature review of empirical evidence of the impacts and outcomes of computer games and serious games. Comput. Educ. 94, 178–192. doi: 10.1016/j.compedu.2015.11.003
Brom, C., Preuss, M., and Klement, D. (2011). Are educational computer micro-games engaging and effective for knowledge acquisition at high schools? A quasi-experimental study. Comput. Educ. 57, 1971–1988. doi: 10.1016/j.compedu.2011.04.007
Clark, D. B., Tanner-Smith, E. E., and Killingsworth, S. S. (2016). Digital games, design, and learning: a systematic review and meta-analysis. Rev. Educ. Res. 86, 79–122. doi: 10.3102/0034654315582065
Clark, R. E. (1994). Media will never influence learning. Educ. Tech. Res. Dev. 42, 21–29. doi: 10.1007/BF02299088
Clark, R. E. (2012). “What is next in the media and methods debate?,” in Learning From Media: Arguments, Analysis, and Evidence, ed R. E. Clark (Greenwich, NC: Information Age Publishers), 327–337.
Connolly, T. M., Boyle, E. A., MacArthur, E., Hainey, T., and Boyle, J. M. (2012). A systematic literature review of empirical evidence on computer games and serious games. Comput. Educ. 59, 661–686. doi: 10.1016/j.compedu.2012.03.004
de Jong, T. (2010). Cognitive load theory, educational research, and instructional design: some food for thought. Instr. Sci. 38, 105–134. doi: 10.1007/s11251-009-9110-0
Delialioglu, O. (2005). “Investigation of source of motivation in a hybrid course,” Presented at Association for Educational Communications and Technology Annual Meeting 2005. Available online at: https://eric.ed.gov/?id=ED485032
Divjak, B., and Tomic, D. (2011). The impact of game-based learning on the achievement of learning goals and motivation for learning mathematics - literature review. J. Inform. Organ. Sci. 35, 15–30. Available online at: https://jios.foi.hr/index.php/jios/article/view/182
Djaouti, D., Alvarez, J., and Jessel, J.-P. (2011). “Classifying serious games: the G/P/S model,” in Handbook of Research on Improving Learning and Motivation Through Educational Games: Multidisciplinary Approaches, ed P. Felicia (Hershey, PA: IGI Global), 118–136. doi: 10.4018/978-1-60960-495-0.ch006
Egenfeldt-Nielsen, S. (2006). Overview of research on the educational use of video games. Nordic. J. Digit. Lit. 1, 184–213. Available online at: https://www.idunn.no/dk/2006/03/overview_of_research_on_the_educationaluseof_video_games
Evans, K. L., Yaron, D., and Leinhardt, G. (2008). Learning stoichiometry: a comparison of text and multimedia formats. Chem. Educ. Res. Pract. 9, 208–218. doi: 10.1039/B812409B
Fabricatore, C. (2000). “Learning and videogames: an unexploited synergy,” in 2000 Annual Convention of the Association for Educational Communications and Technology (AECT). Workshop: In Search of the Meaning of Learning (Long Beach, CA). Available online at: http://eprints.hud.ac.uk/28000/
Fisher, F. L., and Ford, J. K. (1998). Differential effects of learner effort and goal orientation on two learning outcomes. Pers. Psychol. 51, 397–420. doi: 10.1111/j.1744-6570.1998.tb00731.x
Fredericksen, E., Pickett, A., Shea, P., Pelz, W., and Swan, K. (2000). Student satisfaction and perceived learning with on-line courses: principles and examples from the SUNY Learning Network. Online Learn. 4, 7–38. Available online at: https://onlinelearningconsortium.org/jaln_full_issue/volume-4-issue-2-september-2000/
Freund, P. A., Kuhn, J.-T., and Holling, H. (2011). Measuring current achievement motivation with the QCM: short form development and investigation of measurement invariance. Pers. Indiv. Differ. 51, 629–634. doi: 10.1016/j.paid.2011.05.033
Fu, F.-L., Su, R.-C., and Yu, S.-C. (2009). EGameFlow: a scale to measure learners' enjoyment of e-learning games. Comput. Educ. 52, 101–112. doi: 10.1016/j.compedu.2008.07.004
Gray, P. (2009). Play as a foundation for hunter-gatherer social existence. Am. J. Play 1, 476–522. Available online at: https://eric.ed.gov/?id=EJ1069037
Hainey, T., Connolly, T. M., Boyle, E. A., Wilson, A., and Razak, A. (2016). A systematic literature review of games-based learning empirical evidence in primary education. Comput. Educ. 102, 202–223. doi: 10.1016/j.compedu.2016.09.001
Herz, J. C. (1997). Joystick Nation: How Video Games Ate Our Quarters, Stole Our Hearts, and Rewired Our Minds. Boston, MA: Little, Brown and Company.
Hickey, D. T., Ingram-Goble, A. A., and Jameson, E. M. (2009). Designing assessments and assign designs in virtual educational environments. J. Sci. Educ. Technol. 18, 187–208. doi: 10.1007/s10956-008-9143-1
Hidi, S., and Renninger, K. A. (2006). The four-phase model of interest development. Educ. Psychol. 41, 111–127. doi: 10.1207/s15326985ep4102_4
Huizenga, J., Admiraal, W., Akkerman, S., and ten Dam, G. (2008). “Cognitive and affective effects of learning history by playing a mobile game,” in The 2nd European Conference on Games Based Learning: ECGBL 2008 (Reading: Academic Publishing International), 207–212. Available online at: https://www.researchgate.net/publication/252575434_Cognitive_and_affective_effects_of_learning_History_by_playing_a_mobile_game
Hwang, G.-C., Wu, P.-H., and Chen, C.-C. (2012). An online game approach for improving students' learning performance in web-based problem-solving activities. Comput. Educ. 59, 1246–1256. doi: 10.1016/j.compedu.2012.05.009
International Organization for Standardization (2006). ISO 9241-110:2006. Ergonomics of Human-System Interaction - Part 110: Dialogue Principles. Available online at: http://www.iso.org/iso/iso_catalogue/catalogue_tc/catalogue_detail.htm?csnumber=38009
Ismail, H. N., Kuldas, S., and Hamzah, A. (2013). Do students need more motivational resources or more cognitive resources for better learning? Proc. Soc. Behav. Sci. 97, 325–332. doi: 10.1016/j.sbspro.2013.10.241
Keller, J. (2008). An integrative theory of motivation, volition, and performance. Technol. Instr. Cogn. Learn. 6, 79–104. Available online at: https://www.oldcitypublishing.com/journals/ticl-home/ticl-issue-contents/ticl-volume-6-number-2-2008/ticl-6-2-p-79-104/
Kuss, D. J., and Griffiths, M. D. (2012). Internet gaming addiction: a systematic review of empirical research. Int. J. Ment. Health Ad. 10, 278–296. doi: 10.1007/s11469-011-9318-5
Laird, T. F. N., Seifert, T. A., Pascarella, E. T., Mayhew, M. J., and Blaich, C. F. (2014). Deeply affecting first-year students' thinking: deep approaches to learning and three dimensions of cognitive development. J. Higher Educ. 85, 402–432. doi: 10.1353/jhe.2014.0017
Lazarević, D., and Trebješanin, B. (2013). Karakteristike i činioci pristupa studiranju studenata nastavničkih fakulteta [Characteristics and factors of learning approaches of the prospective teachers]. Psihologija 46, 299–314. doi: 10.2298/PSI130601006L
Lee, S. W.-Y. (2013). Investigating students' learning approaches, perceptions of online discussions, and students' online and academic performance. Comput. Educ. 68, 345–352. doi: 10.1016/j.compedu.2013.05.019
Li, M.-C., and Tsai, C.-C. (2013). Game-based learning in science education: a review of relevant research. J. Sci. Educ. Technol. 22, 877–898. doi: 10.1007/s10956-013-9436-x
Martin, S. (2014). Measuring cognitive load and cognition: metrics for technology-enhanced learning. Educ. Res. Eval. 20, 592–621. doi: 10.1080/13803611.2014.997140
Mayer, R. E. (2011). “Multimedia learning and games,” in Computer Games and Instruction, eds S. Tobias and J. D. Fletcher (Charlotte, NC: Information Age), 281–305.
Mayer, R. E. (2014). Computer Games for Learning: An Evidence-Based Approach. Cambridge, MA: MIT Press.
Mayer, R. E., and Johnson, C. I. (2010). Adding instructional features that promote learning in a game-like environment. J. Educ. Comput. Res. 42, 241–265. doi: 10.2190/EC.42.3.a
McGonigal, J. (2011). Reality is Broken: Why Games Make Us Better and How They Can Change the World. New York, NY: The Penguin Press.
McLaren, B. M., Adams, D. M., Mayer, R. E., and Forlizzi, J. (2017). A computer-based game that promotes mathematics learning more than a conventional approach. Int. J. Game Based Learn. 7, 36–56. doi: 10.4018/IJGBL.2017010103
Merino Campos, C., and del Castillo Fernández, H. (2016). The benefits of active video games for educational and physical activity approaches: a systematic review. J. New Approach. Educ. Res. 5, 115–122. doi: 10.7821/naer.2016.7.164
Miyake, A., and Shah, P. (Eds.) (1999). Models of Working Memory: Mechanisms of Active Maintenance and Executive Control. New York, NY: Cambridge University Press.
Moreno, R. (2006). When worked examples don't work: is cognitive load theory at an impasse? Learn. Instr. 16, 170–181. doi: 10.1016/j.learninstruc.2006.02.006
Moreno, R., and Mayer, R. (2007). Interactive multimodal learning environments: special issue on interactive learning environments: contemporary issues and trends. Educ. Psychol. Rev. 19, 309–326. doi: 10.1007/s10648-007-9047-2
Moreno, R., Mayer, R. E., Spires, H., and Lester, J. (2001). The case for social agency in computer-based teaching: do students learn more deeply when they interact with animated pedagogical agents? Cogn. Instr. 19, 177–214. doi: 10.1207/S1532690XCI1902_02
Nebel, S., Schneider, S., and Rey, G. D. (2015). From duels to classroom competition: social competition and learning in educational videogames within different group sizes. Comput. Hum. Behav. 55, 384–398. doi: 10.1016/j.chb.2015.09.035
Norman, K. L. (2011). Assessing the Components of Skill Necessary for Playing Video Games. Human-Computer Interaction Technical Report 11-11-11. University of Maryland. Available online at: http://hcil2.cs.umd.edu/trs/2011-27/2011-27.pdf
Orvis, K. A., Horn, D. B., and Belanich, J. (2006). Videogame-Based Training Success: The Impact of Trainee Characteristics – Year 2 (Technical Report, No. 1188). Arlington, VA: U.S. Army Research Institute for the Behavioral and Social Sciences.
Orvis, K. A., Horn, D. B., and Belanich, J. (2008). The roles of task difficulty and prior videogame experience on performance and motivation in instructional videogames. Comput. Hum. Behav. 24, 2415–2433. doi: 10.1016/j.chb.2008.02.016
Orvis, K. A., Orvis, K. L., Belanich, J., and Mullin, L. N. (2007). “The influence of trainee gaming experience on affective and motivational learner outcomes of videogame-based training environments,” in Computer Games and Team and Individual Learning, eds H. F. O'Neil and R. S. Perez (Oxford: Elsevier Ltd.), 125–143.
Paas, F., Renkl, A., and Sweller, J. (2003). Cognitive load theory and instructional design: recent developements. Educ. Psychol. 38, 1–4. doi: 10.1207/S15326985EP3801_1
Paas, F., Tuovinen, J. E., van Merriënboer, J. J. G., and Darabi, A. A. (2005). A motivational perspective on the relation between mental effort and performance: optimizing learner involvement in instruction. Educ. Tech. Res. Dev. 53, 25–34. doi: 10.1007/BF02504795
Parchman, S. W., Ellis, J. A., Christinaz, D., and Vogel, M. (2000). An evaluation of three computer-based instructional strategies in basic electricity and electronics training. Mil. Psychol. 12, 73–87. doi: 10.1207/S15327876MP1201_4
Parong, J., Mayer, R. E., Fiorella, L., MacNamara, A., Homer, B. D., and Plass, J. L. (2017). Learning executive function skills by playing focused video games. Contemp. Educ. Psychol. 51, 141–151. doi: 10.1016/j.cedpsych.2017.07.002
Peters, K. (2006). File:Plagiomnium Affine Laminazellen.jpeg. Available online at: http://www.wikizeroo.net/index.php?q=aHR0c HM6Ly9lbi53aWtpcGVkaWEub3JnL3dpa2kvRmlsZTpQbGFnaW9tbml1bV 9hZmZpbmVfbGFtaW5hemVsbGVuLmpwZWc
Pollock, E., Chandler, P., and Sweller, J. (2002). Assimilating complex information. Learn. Instr. 12, 61–86. doi: 10.1016/S0959-4752(01)00016-0
Prümper, J., and Hurtienne, J. (2007). “Usability is easy to use: Some background on standards and processes,” in User-Driven IT Design and Quality Assurance, eds Y. Sundblad and A. Walldius (Stockholm: KTH 2007), 47–52.
Renninger, A. K., and Hidi, S. (2011). Revisiting the conceptualization, measurement, and generation of interest. Educ. Psychol. 46, 168–184. doi: 10.1080/00461520.2011.587723
Rheinberg, F., Vollmeyer, R., and Burns, B. (2001). FAM: Ein Fragebogen zur Erfassung aktueller motivation in lern- und leistungssituationen [QCM: a questionnaire to assess current motivation in learning situations]. Diagnostica 47, 57–66. doi: 10.1026//0012-1924.47.2.57
Ricci, K. E., Salas, E., and Cannon-Bowers, J. A. (1996). Do computer-based games facilitate knowledge acquisition and retention? Mil. Psychol. 8, 295–307. doi: 10.1207/s15327876mp0804_3
Ryan, R. M., and Deci, E. L. (2000). Intrinsic and extrinsic motivations: classic definitions and new directions. Contemp. Educ. Psychol. 25, 54–67. doi: 10.1006/ceps.1999.1020
Sankaran, S. R., and Bui, T. (2001). Impact of learning strategies and motivation on performance: a study in web-based instruction. J. Instr. Psychol. 28, 191–198.
Sawyer, B., and Smith, P. (2008). “Serious game taxonomy,” Paper Presented at the Serious Game Summit 2008 (San Francisco, CA).
Schnotz, W., Fries, S., and Horz, H. (2009). “Some motivational aspects of cognitive load theory,” in Contemporary Motivation Research: From Global to Local Perspectives, eds S. Wosnitza, S. A. Karabenick, A. Efklides and P. Nenniger (Göttingen: Hogrefe), 86–113.
Schrader, C., and Bastiaens, T. (2012). Educational computer games and learning: the relationship between design, cognitive load, emotions and outcomes. J. Interact. Learn. Res. 23, 251–271. Available online at: https://www.learntechlib.org/primary/p/36201/
Seilnacht, T. (2013). Zellen in Einem Zwiebelhäutchen [Cells in an Onion Skin]. Available online at: http://www.digitalefolien.de/biologie/didaktik/tnzwieb.JPG
Sitzmann, T. (2011). A meta-analytic examination of the instructional effectiveness of computer-based simulation games. Pers. Psychol. 64, 489–528. doi: 10.1111/j.1744-6570.2011.01190.x
Swaak, J., Jong, T. D., and Joolingen, W. R. V. (2004). The Effects of discovery learning and expository instruction on the acquisition of definitional and intuitive knowledge. J Comput Assist Lear 20, 225–234. doi: 10.1111/j.1365-2729.2004.00092.x
Sweller, J. (2010). Element interactivity and intrinsic, extraneous, and germane cognitive load. Educ. Psychol. Rev. 22, 123–138. doi: 10.1007/s10648-010-9128-5
TenOfAllTrades at English Wikipedia (2007). File:HeLa Cells Stained With Hoechst 33258.jpg. Available online at: https://commons.wikimedia.org/wiki/File:HeLa_cells_stained_with_Hoechst_33258.jpg
Tobias, S., Fletcher, J., Bediou, B., Wind, A., and Chen, F. (2014). “Multimedia learning with computer games,” in The Cambridge Handbook of Multimedia Learning, ed R. Mayer (Cambridge: Cambridge University Press), 762–784. doi: 10.1017/CBO9781139547369.037
Van Merriënboer, J. J. G., and Ayres, P. (2005). Research on cognitive load theory and its design implications for e-learning. Educ. Tech. Res. 53, 5–13. doi: 10.1007/BF02504793
Virvou, M., and Katsionis, G. (2008). On the usability and likeability of virtual reality games for education: the case of VR-ENGAGE. Comput. Educ. 50, 154–178. doi: 10.1016/j.compedu.2006.04.004
Vogel, J. J., Vogel, D. S., Cannon-Bowers, J., Bowers, G. A., Muse, K., and Wright, M. (2006). Computer gaming and interactive simulations for learning: a meta-analysis. J. Educ. Comput. Res. 34, 229–243. doi: 10.2190/FLHV-K4WA-WPVQ-H0YM
Vollmeyer, R., and Rheinberg, F. (2006). Motivational effects on self-regulated learning with different tasks. Educ. Psychol. Rev. 18, 239–253. doi: 10.1007/s10648-006-9017-0
Waschull, S. B. (2005). Predicting success in online psychology courses: self-discipline and motivation. Teach. Psychol. 32, 190–192. doi: 10.1207/s15328023top3203_11
Westera, W. (2015). Games are motivating, aren't they? Disputing the arguments for digital game-based learning. Int. J. Serious Games 2, 4–17. doi: 10.17083/ijsg.v2i2.58
Wijers, M., Jonker, V., and Kerstens, K. (2008). “MobileMath: the phone, the game and the math,” in The 2nd European Conference on Games Based Learning: ECGBL 2008 (Reading: Academic Publishing International), 507–517. Available online at: https://www.researchgate.net/publication/228107365_MobileMath_the_Phone_the_Game_and_the_Math
Woo, J.-C. (2014). Digital game-based learning supports student motivation, cognitive success, and performance outcomes. Educ. Technol. Soc. 17, 291–307. Available online at: https://www.j-ets.net/ETS/issues3ebc.html?id=64
Wouters, P., van Nimwegen, C., van Oostendorp, H., and van der Spek, E. D. (2013). A meta-analysis of the cognitive and motivational effects of serious games. J. Educ. Psychol. 105, 249–265. doi: 10.1037/a0031311
Wrzesien, M., and Raya, M. A. (2010). Learning in serious virtual worlds: evaluation of learning effectiveness and appeal to students in the E-Junior project. Comput. Educ. 55, 178–187. doi: 10.1016/j.compedu.2010.01.003
Young, M. F., Slota, S., Cutter, A. B., Jalette, G., Mullin, G., Lai, B., et al. (2012). Our princess is in another castle: a review of trends in serious gaming for education. Rev. Educ. Res. 82, 61–89. doi: 10.3102/0034654312436980
Yukselturk, E., and Bulut, S. (2007). Predictors for student success in an online course. J. Educ. Technol. Soc. 10, 71–83. Available online at: http://anitacrawley.net/Resources/Articles/Yukselturk.pdf
Yurdugül, H., and Menzi Çetin, N. (2015). Investigation of the relationship between learning process and learning outcomes in e-learning environments. Eurasian J. Educ. Res. 59, 57–74. doi: 10.14689/ejer.2015.59.4
Yuuji, T. (2010). File:Chaos Carolinense.jpg. Available online at: https://commons.wikimedia.org/wiki/File:Chaoss_carolinense.jpg
Keywords: games, digital game-based learning, motivation, cognitive load, learning in a foreign language
Citation: Stiller KD and Schworm S (2019) Game-Based Learning of the Structure and Functioning of Body Cells in a Foreign Language: Effects on Motivation, Cognitive Load, and Performance. Front. Educ. 4:18. doi: 10.3389/feduc.2019.00018
Received: 05 December 2018; Accepted: 19 February 2019;
Published: 19 March 2019.
Edited by:
Fabrizio Consorti, Sapienza University of Rome, ItalyReviewed by:
Gabriella Agrusti, Libera Università Maria SS. Assunta, ItalyAnna Dipace, University of Modena and Reggio Emilia, Italy
Copyright © 2019 Stiller and Schworm. This is an open-access article distributed under the terms of the Creative Commons Attribution License (CC BY). The use, distribution or reproduction in other forums is permitted, provided the original author(s) and the copyright owner(s) are credited and that the original publication in this journal is cited, in accordance with accepted academic practice. No use, distribution or reproduction is permitted which does not comply with these terms.
*Correspondence: Klaus Dieter Stiller, a2xhdXMuc3RpbGxlckB1ci5kZQ==