- 1Beijing Key Laboratory of Lignocellulosic Chemistry, Beijing Advanced Innovation Center for Tree Breeding by Molecular Design, Beijing Forestry University, Beijing, China
- 2Liaoning Key Laboratory of Pulp and Paper Engineering, Center for Lignocellulose Chemistry and Biomaterials, School of Light Industry and Chemical Engineering, Dalian Polytechnic University, Dalian, China
- 3Guangxi Key Laboratory of Clean Pulp and Papermaking and Pollution Control, College of Light Industry and Food Engineering, Guangxi University, Nanning, China
Deep eutectic solvents (DESs) can efficiently promote the efficiency of cellulose enzymatic hydrolysis through the removal of lignin component in lignocellulosic biomass pretreatment. Unraveling the fundamental structural variant of lignin during DES treatment would facilitate to understand the DES-based biomass pretreatment in a clear perspective. Herein, an enzymatic mild acidolysis lignin (EMAL) and a series of β-O-4 lignin model compounds were employed to be treated with choline chloride (ChCl)/lactic acid (LA) (1:2) DES, from which the structural variant of all lignin fractions can be realized in a detailed version. The β-O-4 linkages, existing in either realistic lignin or model compounds, could be cleaved by ChCl/LA, thus leading to the decrease of molecular weight and the rise of hydroxyl groups. The influence of reaction temperature and time was also examined in view of some key structural parameters. Experimental evidences from model compounds confirmed that the repolymerization occurs with the depolymerization of lignin, which may account for the low production of monomeric products during DES treatment.
Introduction
Lignocellulosic biomass, which possesses cellulose (ca. 30–50 wt%), hemicellulose (ca. 20–30 wt%), and lignin (ca. 15–30 wt%), is recognized as a complementary and alternative hydrocarbon resource to fossil feedstocks (Tuck et al., 2012; Galkin and Samec, 2016). In current biorefineries, the pretreatment of biomass, which aims to remove the lignin and promote the efficiency of cellulose enzymatic hydrolysis, remains an essential step (Behera et al., 2014; Rastogi and Shrivastava, 2017). The efforts at developing novel and efficient pretreatment technologies still stay an important one. Recently, deep eutectic solvents (DESs) have gained considerable attention as a new medium for biomass pretreatment due to their significant merits, including ease to prepare, stable chemical property, low cost, recyclability, and environment amity (Mbous et al., 2017; Satlewal et al., 2018). DESs are a mixture of a hydrogen bonding donor (HBD) and a hydrogen bonding acceptor (HBA), which present analogous solvent characteristics with ionic liquids (ILs) (Zhang et al., 2012; Smith et al., 2014). In DESs, the lignin and hemicellulose components can be deconstructed and removed from biomass matrix, while cellulose component can survive because of strong hydrogen bonding networks and stable cohesive energy (Vigier et al., 2015). Pioneering works have indicated that DES pretreatment can significantly promote the efficacy of residue cellulose enzymatic hydrolysis. The plant species, which on the pretreatment with different DESs, have been extended to gramineae [corncob (Procentese et al., 2015; Zhang et al., 2016), wheat straw (Jeong et al., 2015), sorghum (Das et al., 2018), and switchgrass (Chen et al., 2018; Kim et al., 2018)], hardwood [eucalyptus (Shen et al., 2019), willow (Lyu et al., 2018; Song et al., 2019), and poplar (Alvarez-Vasco et al., 2016; Liu et al., 2017)], softwood [pine (Lynam et al., 2017), spruce (Wahlström et al., 2016), and fir (Alvarez-Vasco et al., 2016)], and endocarp (Li et al., 2018).
Lignin is the only renewable aromatic hydrocarbon resource, and biorefinery industries have pursued efficient means for lignin valorization for more than a century (Ragauskas et al., 2014; Sun et al., 2018; Song, 2019; Wu et al., 2019; Zhang et al., 2019). Upon the treatment of biomass with DESs, lignin in lignocellulosic biomass matrix can be cleaved and solubilized (Satlewal et al., 2018). In such a process, more structural complexity has been added to chemical properties of DES-extracted lignin compared to its native version, and this would influence the subsequent upgrading of lignin remarkably. Zhang et al. illustrated that choline chloride (ChCl)/lactic acid (LA) (1:2 molar ratio) DES was capable of extracting lignin with low molecular weight (Mw) from poplar and Douglas fir trees, and they suggested that DES served as an acidic catalyst to rupture β-O-4 lignin linkages without subsequent condensation reactions (Alvarez-Vasco et al., 2016). Shi et al. reported that all ether linkages in sorghum straw lignin were ruptured with ChCl/LA DES, and the resulting lignin could be depolymerized into monophenols with Ru/C catalyst efficiently (Das et al., 2018). Singh et al. used the DESs from lignin-derived monophenols to treat switchgrass; instead, they found that major ether linkages still remained intact in the residual lignin after pretreatment/saccharification (Kim et al., 2018). Wan et al. used ternary DESs to treat switchgrass, which led to the removal of lignin and hemicellulose efficiently; depolymerization and repolymerization of lignin were corroborated on the analysis of NMR spectra (Chen et al., 2019). Obviously, different views on whether repolymerization reaction of lignin occurs or not in DES-pretreated biomass were both taken on in the past. Moreover, it is worthy to point out that only partial lignin fraction could be recovered and characterized in DES biomass pretreatment, thus leaving other lignin fractions in an unknown state. From the pretreatment of biomass and valorization of lignin viewpoints, the deep insight into lignin structural transformation with DES is great of interest and importance.
Herein, we used enzymatic mild acidolysis lignin (EMAL), derived from Eucalyptus tree, as well as dimeric and polymeric β-O-4 lignin models to undergo the treatment of ChCl/LA DES. The whole lignin fractions (i.e., regenerated and fragmented lignin) derived from DES treatment could be retrieved for investigation. Based on the NMR, gel permeation chromatography (GPC), gas chromatography (GC)–mass spectrometry (MS), liquid chromatography (LC)–mass spectrometry (MS), and biomass compositional analysis, the variations of lignin with reaction temperature and time were established in terms of Mw, monomeric products, as well as the abundance linkages and hydroxyl group. The profound comprehension on lignin structural transformation unraveled the depolymerization and repolymerization pathways of lignin during the treatment of DES.
Materials and Methods
Materials
Eucalyptus grandis (5 years old) were obtained from Guangxi Province, China. Eucalyptus EMAL was isolated according to the procedure developed by Wu and Argyropoulos (2003). The lignin chemical composition was measured according to NREL/TP-510-42618 protocol described previously (Sluiter et al., 2008). Polymeric and dimeric model compounds were synthesized according to our previous report (Li and Song, 2019), such as: dimeric model compound 1a had a complete β-O-4 structure and a phenolic group, dimeric model compound 1b had a complete β-O-4 structure but lacked a non-phenolic group, dimeric model compound 1c was a phenolic model but was lacking γ-CH2OH in the β-O-4 structure, dimeric model compounds phenethoxybenzene and 2-phenoxy-1-phenylethanol had no complete β-O-4 structure and methoxyl group on aromatic ring. ChCl and LA, purchased from Energy Chemical, were used for the preparation of DES in a 1:2 ratio without further purification. Commercial cellulase and xylanase were obtained from Shandong Longcott Enzyme Preparation Co., Ltd., China.
Treatment of Lignin With Deep Eutectic Solvent
EMAL (0.2 g) and ChCl/LA DES (2.0 g) were loaded in a 20-ml reactor, which was heated to the desired reaction temperature for a certain time with magnetic stirring (500 rpm). After the reaction, the reactor was cooled, and the thick reaction mixture was diluted with ethanol (5 ml). The addition of HCl solution (pH = 2) led to a precipitate, which was on the treatment of centrifugation, washing with ethanol/water (v/v, 1:9), and freeze-drying to afford regenerated lignin (RL). The liquid fraction from centrifugation was extracted with dichloromethane to obtain fragmented lignin samples (FL) in organic phase. The detailed post-processing flowchart of lignin treatment with DES was shown in Figure 1. The obtained regenerated and fragmented lignin samples were characterized by NMR [two-dimensional (2D) heteronuclear single quantum coherence spectroscopy (HSQC) and 31P], GPC, and GC-MS analysis. The used DES existed in the water phase after dichloromethane extraction, which could be recycled by the removal of water under vacuum conditions.
Analytic Methods
The NMR spectra were acquired on a Bruker AVANCE 400 MHz spectrometer by using a lignin sample (50 mg) dissolved in DMSO-d6 (0.5 ml). HSQC cross peaks and 31P signals were assigned by comparing the spectra with the literatures (Brosse et al., 2010; Mansfield et al., 2012; Wang et al., 2017; Xiao et al., 2017; Meng et al., 2019; Yang et al., 2020). GPC analysis of lignin samples [tetrahydrofuran (THF) solution, ca. 2 mg/ml] was performed on Shimadzu LC-20AD equipped with a PL-gel 10 μm Mixed-B 7.5 mm ID column (mixed) and UV detection detector (254 nm) at 50°C, using THF as the solvent (1 ml/min), which was calibrated with polystyrene standards (peak average Mw values of 96, 500, 1,320, 9,200, 66,000 g/mol; Polymer Laboratories Ltd.). GC-MS analysis of fragmented lignin samples was performed on Shimadzu GC-MS-QP2010SE equipped with an HP-5 MS (30 m × 250 mm × 0.25; Agilent) capillary column and a mass spectroscopy detector. LC-MS analysis of DES-treated dimeric compound was performed on Agilent 1290-6460 equipped with SB-C18 and electrospray ionization (ESI). The column oven was held at 30°C during analysis process. The mobile phase was a gradient methanol/water (with 0.1% formic acid and 5 mM ammonium acetate) at 0.2 ml/min flow rate.
Results and Discussion
The Treatment of Enzymatic Mild Acidolysis Lignin With Choline Chloride/Lactic Acid Deep Eutectic Solvent
An EMAL isolated from Eucalyptus was selected as a substrate (Guerra et al., 2006; Xiao et al., 2017; Wang et al., 2018). The total lignin and carbohydrate contents were measured as about 87 wt% and 7 wt% based on biomass compositional analysis (Table S1). 2D HSQC NMR spectra analysis indicated this EMAL featured abundant β-O-4 linkages (64%), as well as less β-β (3%) and β-5 (10%) substructures, being kin to native lignin in biomass (Figure 2A). Thereby, this EMAL is a suitable substrate to unravel the lignin structural variation. After the treatment of EMAL with ChCl/LA DES (1:2 of molar ratio), the reaction mixture was diluted with EtOH and HCl solution (pH = 2), thus leading to obtain regenerated lignin (RL, DES-1 to DES-5) as a precipitate. The leftover soluble fraction contained fragmented lignin (FL) species and DES, which could be separated by dichloromethane/water extraction (Figure 1). The analysis of 1H NMR spectra indicated no changing on the recycled DES (90%) (Figure S1). The detailed yields of RL and FL obtained at different reaction temperatures and times were presented in Table 1. With 80°C and 1 h treatment, 86% of RL (DES-1) and 10% of FL were obtained, respectively (Table 1, entry 2). The high mass balance (96%) indicated that all lignin fractions could be basically recovered for characterization. Thereby, the utilization of isolated EMAL can overcome the limitation in the biomass pretreatment by DES, wherein the illustration of the lignin variation is incomplete. With the rise of reaction temperature, the decreasing of RL and increasing of FL were observed simultaneously, as seen in the case of 100°C (83% and 16%; Table 1, entry 3), 120°C (73% and 23%; Table 1, entry 4) and 140°C (71% and 20%; Table 1, entry 5). This scenario may be because the harsher reaction condition enhances the fragmentation of lignin into small and soluble pieces. A similar trend was also observed at variable reaction times at 100°C, that is, prolonging the treatment time led to the low yield of RL and high yield of FL (Table 1, entries 6 and 7). The detailed structural changes to the EMAL were then assessed by GC-MS, GPC, and NMR spectroscopy.
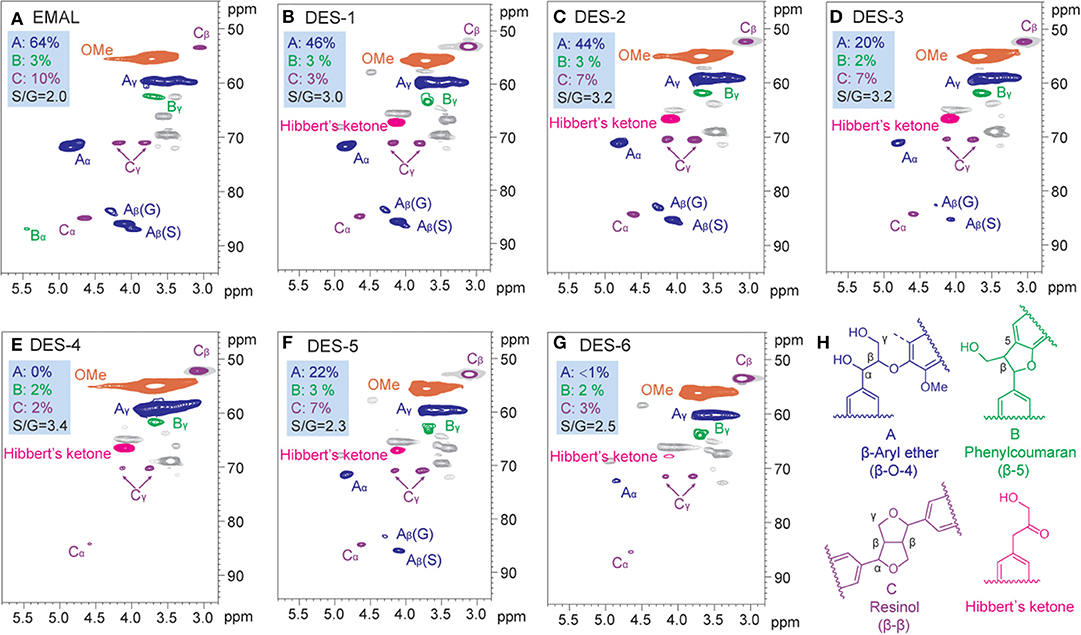
Figure 2. Side chain (δC/δH 45–100/2.8–5.3 ppm) in the two-dimensional (2D) heteronuclear single quantum coherence spectroscopy (HSQC) NMR spectra of regenerated lignin (RL) after deep eutectic solvent (DES) treatment. (A) Enzymatic Mild Acidolysis Lignin (EMAL), (B) DES-1 (Table 1, entry 2), (C) DES-2 (Table 1, entry 3), (D) DES-3 (Table 1, entry 4), (E) DES-4 (Table 1, entry 5), (F) DES-5 (Table 1, entry 6), (G) DES-6 (Table 1, entry 7), (H) main classical substructures and Hibbert's ketone structure in 2D HSQC NMR of the lignins.
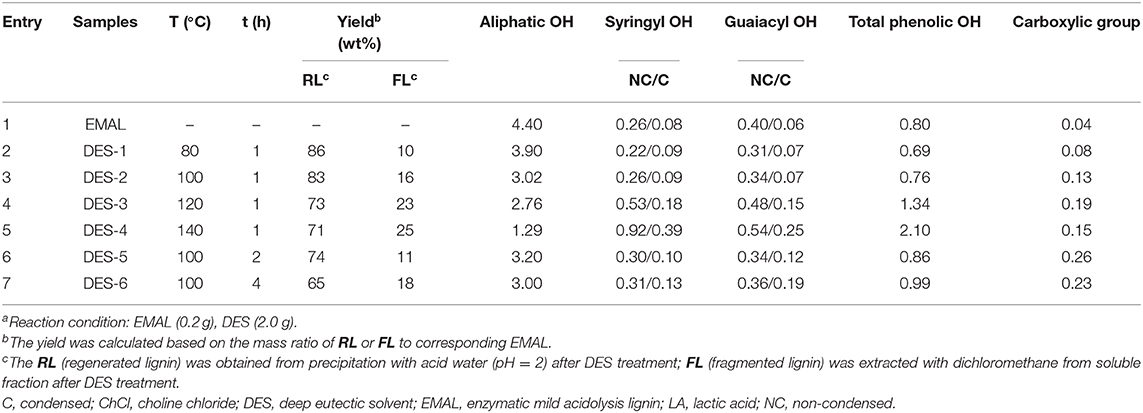
Table 1. ChCl/LA DES-treated Eucalyptus EMAL under various reaction conditions (the unit of hydroxyl and carboxylic group: mmol/g)a.
NMR Analysis
The abundance of hydroxyl group (OH) plays a critical role in future lignin valorization (Li and Song, 2019), which would be alternated with the cleavage of β-O-4 linkages and condensation reaction. To assess the variations of OH after DES treatment, regenerated lignin samples were treated with a phosphorylation reagent [2-chloro-4,4,5,5-tetramethyl-1,3,2-dioxaphospholate (TMDP)] and analyzed by typical 31P NMR technology (Brosse et al., 2010; Wang et al., 2017; Meng et al., 2019; Yang et al., 2020). The aliphatic OH (145.5–149.5 ppm), condensed phenolic OH (S unit, 143.5–144.5 ppm; G unit, 140.8–141.2), uncondensed phenolic OH (S unit, 141.8–143.5 ppm; G unit, 138.5–140.5) from syringyl and guaiacyl units, as well as carboxylic group (134.5–135.5 ppm) were all measured (Table 1; see also Figure S2). The abundance of aliphatic OH tapered off with the increasing of reaction temperature and time because the cleavage of β-O-4 linkages resulted in the loss of Cα-OH moiety. On the contrary, the total phenolic OH gradually increased with higher reaction temperature or longer time, which derived from the exposing of phenolic OH after the cleavage of β-O-4 linkages. The non-condensed OH for DES-1 (80°C, 0.53 mmol/g) and DES-2 (100°C, 0.60 mmol/g) at a relative low reaction temperature were comparable to initial EMAL (0.66 mmol/g), while significant increase of non-condensed OH was observed in DES-3 (120°C, 1.01 mmol/g) and DES-4 (140°C, 1.46 mmol/g) at high temperatures. In the case of condensed OH, a similar variation trend was also observed, and a maximum value of condensed OH was obtained in DES-4 (140°C, 0.64 mmol/g). These results suggested that the lignin experiences depolymerization and repolymerization upon the cleavage of β-O-4 linkages in a synchronous manner, and both of them would be enhanced under harsh conditions, being in line with a previous report (Chen et al., 2019). Similar variation trends occurred in DES treatment time (DES-5 and DES-6). The carboxylic group, probably generated from oxidation reaction with DES, was also increased, especially with longer reaction time (DES-5, 0.26 mmol/g; DES-6, 0.23 mmol/g).
To elaborate the changes of inter-unit linkages, 2D HSQC NMR analysis for regenerated lignin samples DES-1 to DES-6 were performed (Figure 2; see also Figure S3). The detailed signal peaks were assigned according to previous reports (Mansfield et al., 2012; Xiao et al., 2017). A decline of β-O-4 linkage (46%) was observed after DES treatment 80°C for 1 h, being lower than that from EMAL (64%). This decline was intensified with the rise of reaction temperature and/or time until almost all cross signals for β-O-4 units disappeared in DES-4 and DES-6. The lessening of β-O-4 linkage corresponded to the rise of phenolic hydroxyl groups. In the case of carbon–carbon linkages (β-β and β-5), partial degradation was detected by comparison with those from EMAL. Thereby, the cleavage of β-O-4 linkage occurred preferentially in the DES treatment process. Of note, cross signals for Hibbert's ketone (labeled in red) were also detected in the regenerated lignin samples, which kept consistent in the conclusion that DES treatment process is an acid-catalyzed process (Brandt et al., 2015; Alvarez-Vasco et al., 2016; Das et al., 2018). Further analysis of HSQC NMR spectra showed that the signal intensity of Hibbert's ketone gradually decreased with prolonging reaction time until disappearance in DES-6 (Figure 2G) probably because Hibbert's ketone moiety could be released or retransformed.
The ratios of S/G subunits of regenerated lignin were also measured by semiquantitative integration of cross signals at aromatic/unsaturated region (δC/δH 100–135/5.9–8.0 ppm) (Figure 2 and Figure S3). The increased S/G ratio values were detected after DES treatment by comparison with unreacted EMAL. The high ratios of S/G in regenerated lignin indicated that more guaiacyl subunits have been solubilized in fragmented lignin.
Gel Permeation Chromatography and Gas Chromatography–Mass Spectrometry Analysis
To address changes in lignin polymerization degree during DES treatment, analysis of the resulting samples by GPC was performed, as shown in Figure 3. The average Mw slightly decreased from 8,590 g/mol for initial EMAL to 6,110 g/mol for regenerated lignin sample DES-1, in line with the observation in 2D NMR spectra, where most β-O-4 linkages still remained. The corresponding fragmented lignin recovered from soluble fraction showed a low Mw of 1,890 g/mol. With the elevation of reaction temperature or time, the degree of lignin polymerization declined continuously. DES-4, obtained at 140°C and 1 h, exhibited the lowest Mw values of regenerated lignin (2,350 g/mol) and fragmented lignin (780 g/mol). Under such a condition, GPC corroborated the prevalence of full dissociation of lignin biopolymer, in alignment with NMR analysis, wherein highest abundance of hydroxyl groups and lowest content of β-O-4 moiety were both detected. In addition, the polydispersity values (Mw/Mn) for regenerated lignin (1.35–1.57) and fragmented lignin (1.12–1.38) were both decreased compared to initial EMAL (2.08), indicating that the resulted lignin samples have a narrow distribution.
In view of the low polymerization degree in fragmented lignin, we speculated that some monomeric products have been generated. Product distributions based on GC-MS analysis showed that vanillin and syringaldehyde were produced as the major monomeric phenols in all cases. Interestingly, syringyl- and guaiacyl-derived monoketones and diketones were also detected (Figure S4), which were promoted with the rise of reaction temperature. It should be noted that either aldehyde or diketone derivatives from lignin depolymerization proceeded through an acid-catalyzed reaction according to previous reports (Lundquist and Hedlund, 1967; Deuss et al., 2015). Unfortunately, the combined yield of total monomers is less than 3 wt% by comparison with the authentic samples, which is rather lower than that from metal-catalyzed hydrogenolysis of EMAL (Xiao et al., 2017). The severe recondensation, which accompanies the depolymerization of lignin, may account for this scenario.
Treatment of β-O-4 Mimics With Deep Eutectic Solvent
Currently used EMAL contained 7 wt% carbohydrate, which, together with β-β and β-5 linkages, will exhibit in the 2D NMR spectra after DES treatment and will disturb the distinction of lignin structure changes. To recognize the lignin-derived signals in a clear version, a synthetic polymer composed exclusively of the β-O-4 substructure was treated with ChCl/LA DES at 100°C for 4 h (Figure 4A). The detailed workup procedure to acquire the soluble and insoluble fractions is similar with realistic lignin sample. For the case of insoluble fraction, the changes in 2D NMR spectra proceeded in a fashion akin to EMAL, that is, the sharp decline of β-O-4 signals and the emergence of Hibbert's ketone specie (Alvarez-Vasco et al., 2016; Das et al., 2018). Additionally, the cross peaks at aliphatic area (62.1/3.6 ppm, 64.3/3.5 ppm, 80.6/4.6 ppm), as well as at aromatic (114.6/6.7 ppm, 120.0/6.8 ppm), were emerged and detected (Figure 4B). Some of these signals could be found in NMR spectra of DES-treated EMAL (Figure 2). Taking into consideration the decline of β-O-4 moiety, together with current polymeric state, these new signals may be ascribed to the recondensation product (Shuai et al., 2010). This observation is different with previous report by using lignocellulosic biomass as substrates, where no condensation structures were distinguished probably because of the overlapping of signals (Alvarez-Vasco et al., 2016). The DES-treated β-O-4 polymeric mimics also led to a decrease in Mw from 3,200 g/mol to 2,100 g/mol (insoluble fraction) and 590 g/mol (soluble fraction) (Figure 4C). Further analysis of the soluble fraction suggested the vanillin, guaiacyl-derived monoketones, and diketone were all generated, being in line with scenario of realistic lignin (Figure S5).
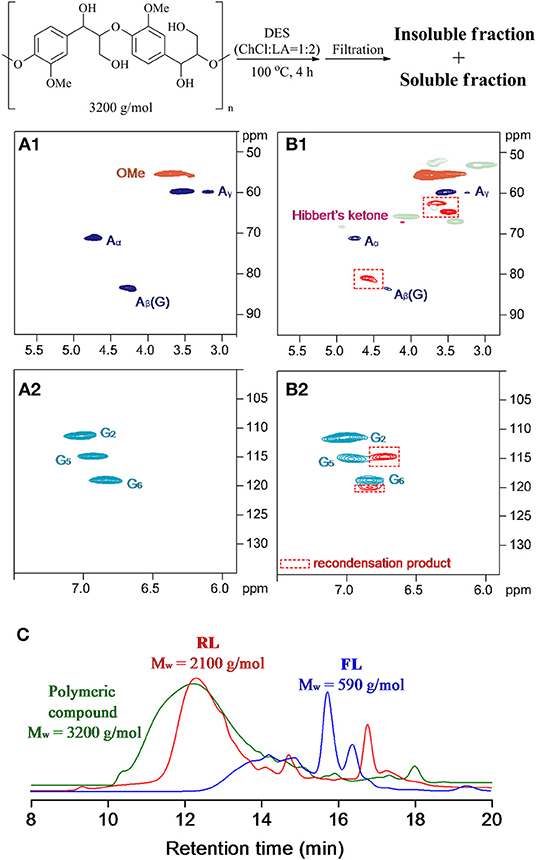
Figure 4. Two-dimensional (2D) heteronuclear single quantum coherence spectroscopy (HSQC) spectra of (A) synthetic lignin polymeric compounds and (B) insoluble fraction after deep eutectic solvent (DES) treatment. (C) The molecular weight distribution.
A series of dimeric lignin mimics were also tested in DES at 100°C for 2 h, and some representative results were summarized in Table 2 and Figure S6. Compound 1a, having a complete β-O-4 structure and a phenolic group, underwent a full conversion after DES treatment. By comparison with authentic samples on GC-MS, some monophenols, such as vanillin (3a, 0.1%), guaiacyl-derived and Hibbert's ketone (7a, 2%), coniferol (8a, 1%), and guaiacol (2, 10%), were identified. It is worth mentioning that an enol ether derivative from dehydration reaction of 1a, which is often generated through an acid-catalyzed hydrolysis reaction and considered as intermediates toward Hibbert's ketone or aldehyde, was also observed in DES treatment (Table 2, entry 1) (Lundquist and Lundgren, 1972; Lundquist, 1976). Compared to metal catalysis systems (Hossain et al., 2019; Li and Song, 2019), the rather low mass balance from monomeric and dimeric products suggested that a severe repolymerization has occurred in DES treatment. The analysis of resulted mixture on LC-MS showed that trimers, tetramers, and oligomers with corresponding mass values have been generated, despite that the exact structures of the resulting products were hard to identify (Figure S7). GPC analysis of the resulting mixture indicated a broader polydispersity after compound 1a was treated with DES (Figure 5). A broad peak with a longer retention time (from 13.22 to 14.51 min) appeared, which is assigned to oligomers in accordance with their larger molecular structure by comparison with compound 1a. The peak also having two shorter retention times (15.86 and 16.79 min) corresponded to the monophenols. The above results confirmed that the depolymerization of β-O-4 units and the repolymerization reactions occurred concurrently during DES treatment, being in accordance with realistic lignin and synthetic polymer. Given that few free phenolic groups exit in lignin before the cleavage of β-O-4 unit, a non-phenolic model compound 1b with β-O-4 structure was also tested by DES. This reaction gave similar monomeric products in lower yields (Table 2, entry 2), albeit a full conversion of 1b was observed. In the case of another phenolic lignin model 1c, which lacks γ-CH2OH in β-O-4 structure, aldehyde derivatives were generated as dominated monomers (Table 2, entry 3). Dimeric compounds that do not contain complete β-O-4 structure and methoxyl group on aromatic ring, i.e., phenethoxybenzene and 2-phenoxy-1-phenylethanol, were also treated with ChCl/LA DES, while no obvious conversion was detected (Figure S8).
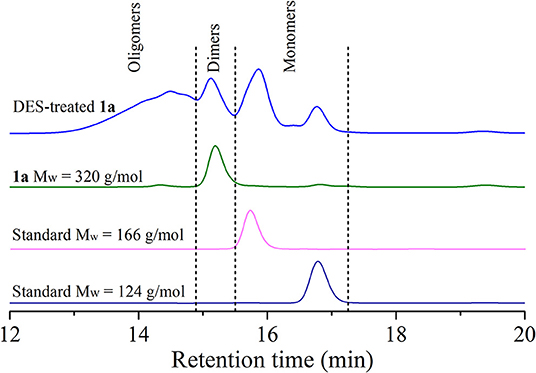
Figure 5. Gel permeation chromatography (GPC) spectra of deep eutectic solvent (DES)-treated 1a, compound 1a and standard compounds [guaiacol: molecular weight (Mw) = 124 g/mol and dihydroeugenol: Mw = 166 g/mol].
Plausible Reaction Pathway
Based on the above results acquired from realistic lignin and model compounds, a plausible reaction pathway for the DES-treated lignin was proposed in Figure 6. β-O-4 units in lignin should be efficiently cleaved probably through an acid-catalyzed process during DES. The reaction starts from a hydrogen ion attack on the α-hydroxyl group in A, which gives a carbocation species B via the release of one molecular unit of H2O (Lundquist and Lundgren, 1972; Lundquist, 1976; Ito et al., 2011; Sturgeon et al., 2014). The elimination reaction between α positive charge and β-H results in an enol ether intermediate C, together with regeneration of a hydrogen ion. Following hydrolysis of C leads to the cleavage of C-O bond, thus affording Hibbert's ketone moiety E (Lundquist and Lundgren, 1972; Lundquist, 1976; Ito et al., 2011; Sturgeon et al., 2014). Alternatively, allylic rearrangement of C forms isomeric enol ether G1 through D, and G1 can also be generated from the rearrangement reaction of E and F under acidic conditions (Lundquist and Hedlund, 1967). The isomerization of G1 intermediate gives G2 probably via an enediol, and an equilibrium is proposed between G1 and G2 (Lundquist and Hedlund, 1967; Lundquist and Lundgren, 1972). Finally, an oxidoreduction of the mixture G1 and G2 leads to the formation of monoketone H and diketone I (Lundquist and Hedlund, 1967; Lundquist and Lundgren, 1972). Experimental evidences have substantiated the formation of oligomers rapidly during DES treatment, which may derive from the fact that the carbocation B or enol ether C is most likely responsible for the repolymerization rather than depolymerization (Lohr et al., 2015; Li and Song, 2019).
Conclusion
The detailed structural transformation of lignin during DES (ChCl:LA, 1:2) treatment was systematically unraveled by means of experiments using isolated lignin and a series of β-O-4 lignin model compounds. Entire fractions derived from lignin which were recovered from DES-treated EMAL showed different characteristics. The cleavage of β-O-4 linkages of EMAL is exacerbated with the increasing of reaction temperature and/or time, which leads to a sustained fall of insoluble lignin fraction and average Mw values, as well as a sustained rise of hydroxyl groups. The monomeric phenols derived from EMAL, polymeric and dimeric β-O-4 mimics were also identified, albeit in low combined yields. Experimental evidences from the reactions of β-O-4 models substantiated that the repolymerization reaction is accompanied by the depolymerization process. Mechanistic study suggested the main steps during DES-treated wood lignin, such as the cleavage of β-O-4, the repolymerization of active species, the production and derivation of mono products, should involve acid-catalyzed processes. This contribution provided a clear and beneficial information on lignin variation during DES treatment, which would be beneficial to design new DES-based pretreatment tailored for biorefinery and biotransformation.
Data Availability Statement
All datasets generated for this study are included in the article/Supplementary Material.
Author Contributions
SW carried out all experiments and wrote the manuscript. HL performed the lignin model compounds analysis. L-PX and GS designed the work and revised the manuscript. All authors discussed the results.
Funding
This work was supported by the National Natural Science Foundation of China (21776020, 31971607, 51961125207), the Natural Science Foundation of Liaoning Province (No. 2019-MS-019), the Opening Project of Guangxi Key Laboratory of Clean Pulp & Papermaking and Pollution Control (No. 2019KF14), and Youth Technology Talents of Dalian (No. 2019RQ035).
Conflict of Interest
The authors declare that the research was conducted in the absence of any commercial or financial relationships that could be construed as a potential conflict of interest.
Supplementary Material
The Supplementary Material for this article can be found online at: https://www.frontiersin.org/articles/10.3389/fenrg.2020.00048/full#supplementary-material
References
Alvarez-Vasco, C., Ma, R., Quintero, M., Guo, M., Geleynse, S., Ramasamy, K. K., et al. (2016). Unique low-molecular-weight lignin with high purity extracted from wood by deep eutectic solvents (DES): a source of lignin for valorization. Green Chem. 18, 5133–5141. doi: 10.1039/C6GC01007E
Behera, S., Arora, R., Nandhagopal, N., and Kumar, S. (2014). Importance of chemical pretreatment for bioconversion of lignocellulosic biomass. Renew. Sust. Energy Rev. 36, 91–106. doi: 10.1016/j.rser.2014.04.047
Brandt, A., Chen, L., van Dongen, B. E., Welton, T., and Hallett, J. P. (2015). Structural changes in lignins isolated using an acidic ionic liquid water mixture. Green Chem. 17, 5019–5034. doi: 10.1039/C5GC01314C
Brosse, N., El Hage, R., Chaouch, M., Pétrissans, M., Dumarçay, S., and Gérardin, P. (2010). Investigation of the chemical modifications of beech wood lignin during heat treatment. Polym. Degrad. Stab. 95, 1721–1726. doi: 10.1016/j.polymdegradstab.2010.05.018
Chen, Z., Jacoby, W. A., and Wan, C. (2019). Ternary deep eutectic solvents for effective biomass deconstruction at high solids and low enzyme loadings. Bioresour. Technol. 279, 281–286. doi: 10.1016/j.biortech.2019.01.126
Chen, Z., Reznicek, W. D., and Wan, C. (2018). Aqueous choline chloride: a novel solvent for switchgrass fractionation and subsequent hemicellulose conversion into furfural. ACS Sust. Chem. Eng. 6, 6910–6919. doi: 10.1021/acssuschemeng.8b00728
Das, L., Li, M., Stevens, J., Li, W., Pu, Y., Ragauskas, A. J., et al. (2018). Characterization and catalytic transfer hydrogenolysis of deep eutectic solvent extracted sorghum lignin to phenolic compounds. ACS Sust. Chem. Eng. 6, 10408–10420. doi: 10.1021/acssuschemeng.8b01763
Deuss, P. J., Scott, M., Tran, F., Westwood, N. J., de Vries, J. G., and Barta, K. (2015). Aromatic monomers by in situ conversion of reactive intermediates in the acid-catalyzed depolymerization of lignin. J. Am. Chem. Soc. 137, 7456–7467. doi: 10.1021/jacs.5b03693
Galkin, M. V., and Samec, J. S. M. (2016). Lignin valorization through catalytic lignocellulose fractionation: a fundamental platform for the future biorefinery. ChemSusChem 9, 1544–1558. doi: 10.1002/cssc.201600237
Guerra, A., Filpponen, I., Lucia, L. A., Saquing, C., Baumberger, S., and Argyropoulos, D. S. (2006). Toward a better understanding of the lignin isolation process from wood. J. Agric. Food Chem. 54, 5939–5947. doi: 10.1021/jf060722v
Hossain, M. A., Phung, T. K., Rahaman, M. S., Tulaphol, S., Jasinski, J. B., and Sathitsuksanoh, N. (2019). Catalytic cleavage of the β-O-4 aryl ether bonds of lignin model compounds by Ru/C catalyst. Appl. Catal. A 582, 117100–117106. doi: 10.1016/j.apcata.2019.05.034
Ito, H., Imai, T., Lundquist, K., Yokoyama, T., and Matsumoto, Y. (2011). Revisiting the mechanism of β-O-4 bond cleavage during acidolysis of lignin. Part 3: search for the rate-determining step of a non-phenolic C6-C3 type model compound. J. Wood Chem. Technol. 31, 172–182. doi: 10.1080/02773813.2010.515050
Jeong, K. M., Lee, M. S., Nam, M. W., Zhao, J., Jin, Y., Lee, D.-K., et al. (2015). Tailoring and recycling of deep eutectic solvents as sustainable and efficient extraction media. J. Chromatogr. A 1424, 10–17. doi: 10.1016/j.chroma.2015.10.083
Kim, K. H., Dutta, T., Sun, J., Simmons, B., and Singh, S. (2018). Biomass pretreatment using deep eutectic solvents from lignin derived phenols. Green Chem. 20, 809–815. doi: 10.1039/C7GC03029K
Li, H., and Song, G. (2019). Ru-catalyzed hydrogenolysis of lignin: base-dependent tunability of monomeric phenols and mechanistic study. ACS Catal. 9, 4054–4064. doi: 10.1021/acscatal.9b00556
Li, W., Amos, K., Li, M., Pu, Y., Debolt, S., Ragauskas, A. J., et al. (2018). Fractionation and characterization of lignin streams from unique high-lignin content endocarp feedstocks. Biotechnol. Biofuels 11, 304–317. doi: 10.1186/s13068-018-1305-7
Liu, Y., Chen, W., Xia, Q., Guo, B., Wang, Q., Liu, S., et al. (2017). Efficient cleavage of lignin-carbohydrate complexes and ultrafast extraction of lignin oligomers from wood biomass by microwave-assisted treatment with deep eutectic solvent. ChemSusChem 10, 1692–1700. doi: 10.1002/cssc.201601795
Lohr, T. L., Li, Z., and Marks, T. J. (2015). Selective ether/ester C-O cleavage of an acetylated lignin model via tandem catalysis. ACS Catal. 5, 7004–7007. doi: 10.1021/acscatal.5b01972
Lundquist, K. (1976). Low-molecular weight lignin hydrolysis products. Appl. Polym. Symp. 28, 1393–1407
Lundquist, K., and Hedlund, K. (1967). Acid degradation of lignin I. the formation of ketones of the guaiacylpropane series. Acta Chem. Scand. 21, 1750–1754. doi: 10.3891/acta.chem.scand.21-1750
Lundquist, K., and Lundgren, R. (1972). Acid degradation of lignin part VII.* the cleavage of ether bonds. Acta Chem. Scand. 26, 2005–2023. doi: 10.3891/acta.chem.scand.26-2005
Lynam, J. G., Kumar, N., and Wong, M. J. (2017). Deep eutectic solvents' ability to solubilize lignin, cellulose, and hemicellulose; thermal stability; and density. Bioresour. Technol. 238, 684–689. doi: 10.1016/j.biortech.2017.04.079
Lyu, G., Li, T., Ji, X., Yang, G., Liu, Y., Lucia, A. L., et al. (2018). Characterization of lignin extracted from willow by deep eutectic solvent treatments. Polymers 10, 869–879. doi: 10.3390/polym10080869
Mansfield, S. D., Kim, H., Lu, F., and Ralph, J. (2012). Whole plant cell wall characterization using solution-state 2D NMR. Nat. Protoc. 7, 1579–1589. doi: 10.1038/nprot.2012.064
Mbous, Y. P., Hayyan, M., Hayyan, A., Wong, W. F., Hashim, M. A., and Looi, C. Y. (2017). Applications of deep eutectic solvents in biotechnology and bioengineering-promises and challenges. Biotechnol. Adv. 35, 105–134. doi: 10.1016/j.biotechadv.2016.11.006
Meng, X., Crestini, C., Ben, H., Hao, N., Pu, Y., Ragauskas, A. J., et al. (2019). Determination of hydroxyl groups in biorefinery resources via quantitative 31P NMR spectroscopy. Nat. Protoc. 14, 2627–2647. doi: 10.1038/s41596-019-0191-1
Procentese, A., Johnson, E., Orr, V., Garruto Campanile, A., Wood, J. A., Marzocchella, A., et al. (2015). Deep eutectic solvent pretreatment and subsequent saccharification of corncob. Bioresour. Technol. 192, 31–36. doi: 10.1016/j.biortech.2015.05.053
Ragauskas, A. J., Beckham, G. T., Biddy, M. J., Chandra, R., Chen, F., Davis, M. F., et al. (2014). Lignin valorization: improving lignin processing in the biorefinery. Science 344:1246843. doi: 10.1126/science.1246843
Rastogi, M., and Shrivastava, S. (2017). Recent advances in second generation bioethanol production: An insight to pretreatment, saccharification and fermentation processes. Renew. Sust. Energy Rev. 80, 330–340. doi: 10.1016/j.rser.2017.05.225
Satlewal, A., Agrawal, R., Bhagia, S., Sangoro, J., and Ragauskas, A. J. (2018). Natural deep eutectic solvents for lignocellulosic biomass pretreatment: recent developments, challenges and novel opportunities. Biotechnol. Adv. 36, 2032–2050. doi: 10.1016/j.biotechadv.2018.08.009
Shen, X.-J., Wen, J.-L., Mei, Q.-Q., Chen, X., Sun, D., Yuan, T.-Q., et al. (2019). Facile fractionation of lignocelluloses by biomass-derived deep eutectic solvent (DES) pretreatment for cellulose enzymatic hydrolysis and lignin valorization. Green Chem. 21, 275–283. doi: 10.1039/C8GC03064B
Shuai, L., Yang, Q., Zhu, J. Y., Lu, F. C., Weimer, P. J., Ralph, J., et al. (2010). Comparative study of SPORL and dilute-acid pretreatments of spruce for cellulosic ethanol production. Bioresour. Technol. 101, 3106–3114. doi: 10.1016/j.biortech.2009.12.044
Sluiter, A., Hames, B., Ruiz, R., Scarlata, C., Sluiter, J., Templeton, D., et al. (2008). Determination of Structural Carbohydrates and Lignin in Biomass. Golden, CO: National Renewable Energy Laboratory (NREL).
Smith, E. L., Abbott, A. P., and Ryder, K. S. (2014). Deep eutectic solvents (DESs) and their applications. Chem. Rev. 114, 11060–11082. doi: 10.1021/cr300162p
Song, G. (2019). The development of catalytic fractionation and conversion of lignocellulosic biomass under lignin-first strategy. J. For. Eng. 4, 1–10. doi: 10.13360/j.issn.2096-1359.2019.05.001
Song, Y., Chandra, R. P., Zhang, X., Tan, T., and Saddler, J. N. (2019). Comparing a deep eutectic solvent (DES) to a hydrotrope for their ability to enhance the fractionation and enzymatic hydrolysis of willow and corn stover. Sust. Energy Fuels 3, 1329–1337. doi: 10.1039/C8SE00617B
Sturgeon, M. R., Kim, S., Lawrence, K., Paton, R. S., Chmely, S. C., Nimlos, M., et al. (2014). A mechanistic investigation of acid-catalyzed cleavage of aryl-ether linkages: implications for lignin depolymerization in acidic environments. ACS Sust. Chem. Eng. 2, 472–485. doi: 10.1021/sc400384w
Sun, Z., Fridrich, B., de Santi, A., Elangovan, S., and Barta, K. (2018). Bright side of lignin depolymerization: toward new platform chemicals. Chem. Rev. 118, 614–678. doi: 10.1021/acs.chemrev.7b00588
Tuck, C. O., Pérez, E., Horváth, I. T., Sheldon, R. A., and Poliakoff, M. (2012). Valorization of biomass: deriving more value from waste. Science 337, 695–699. doi: 10.1126/science.1218930
Vigier, K. D. O., Chatel, G., and Jérôme, F. (2015). Contribution of deep eutectic solvents for biomass processing: opportunities, challenges, and limitations. ChemCatChem 7, 1250–1260. doi: 10.1002/cctc.201500134
Wahlström, R., Hiltunen, J., Pitaluga de Souza Nascente Sirkka, M., Vuoti, S., and Kruus, K. (2016). Comparison of three deep eutectic solvents and 1-ethyl-3-methylimidazolium acetate in the pretreatment of lignocellulose: effect on enzyme stability, lignocellulose digestibility and one-pot hydrolysis. RSC Adv. 6, 68100–68110. doi: 10.1039/C6RA11719H
Wang, H.-M., Wang, B., Wen, J.-L., Yuan, T.-Q., and Sun, R.-C. (2017). Structural characteristics of lignin macromolecules from different eucalyptus species. ACS Sust. Chem. Eng. 5, 11618–11627. doi: 10.1021/acssuschemeng.7b02970
Wang, S., Gao, W., Li, H., Xiao, L.-P., Sun, R.-C., and Song, G. (2018). Selective fragmentation of biorefinery corncob lignin into p-hydroxycinnamic esters with a supported zinc molybdate catalyst. ChemSusChem 11, 2114–2123. doi: 10.1002/cssc.201800455
Wu, S., and Argyropoulos, D. S. (2003). An improved method for isolating lignin in high yield and purity. J. Pulp Pap. Sci. 29, 235–240.
Wu, Y., Qian, Y., Lou, H., Yang, D., and Qiu, X. (2019). Enhancing the broad-spectrum adsorption of lignin through methoxyl activation, grafting modification, and reverse self-assembly. ACS Sust. Chem. Eng. 7, 15966–15973. doi: 10.1021/acssuschemeng.9b02317
Xiao, L.-P., Wang, S., Li, H., Li, Z., Shi, Z.-J., Xiao, L., et al. (2017). Catalytic hydrogenolysis of lignins into phenolic compounds over carbon nanotube supported molybdenum oxide. ACS Catal. 7, 7535–7542. doi: 10.1021/acscatal.7b02563
Yang, H., Yoo, C. G., Meng, X., Pu, Y., Muchero, W., Tuskan, G. A., et al. (2020). Structural changes of lignins in natural populus variants during different pretreatments. Bioresour. Technol. 295, 122240–122246. doi: 10.1016/j.biortech.2019.122240
Zhang, C.-W., Xia, S.-Q., and Ma, P.-S. (2016). Facile pretreatment of lignocellulosic biomass using deep eutectic solvents. Bioresour. Technol. 219, 1–5. doi: 10.1016/j.biortech.2016.07.026
Zhang, Q., De Oliveira Vigier, K., Royer, S., and Jérôme, F. (2012). Deep eutectic solvents: syntheses, properties and applications. Chem. Soc. Rev. 41, 7108–7146. doi: 10.1039/C2CS35178A
Keywords: lignin, deep eutectic solvents (DESs), β-O-4 mimics, repolymerization, depolymerization
Citation: Wang S, Li H, Xiao L-P and Song G (2020) Unraveling the Structural Transformation of Wood Lignin During Deep Eutectic Solvent Treatment. Front. Energy Res. 8:48. doi: 10.3389/fenrg.2020.00048
Received: 12 February 2020; Accepted: 10 March 2020;
Published: 14 April 2020.
Edited by:
Chang Geun Yoo, SUNY College of Environmental Science and Forestry, United StatesReviewed by:
Wen Wang, Guangzhou Institute of Energy Conversion (CAS), ChinaQiong Wang, Guangzhou Institute of Energy Conversion (CAS), China
Copyright © 2020 Wang, Li, Xiao and Song. This is an open-access article distributed under the terms of the Creative Commons Attribution License (CC BY). The use, distribution or reproduction in other forums is permitted, provided the original author(s) and the copyright owner(s) are credited and that the original publication in this journal is cited, in accordance with accepted academic practice. No use, distribution or reproduction is permitted which does not comply with these terms.
*Correspondence: Ling-Ping Xiao, lpxiao@dlpu.edu.cn; Guoyong Song, songg@bjfu.edu.cn
†These authors have contributed equally to this work