- School of Chemistry, College of Science, University of Tehran, Tehran, Iran
A novel CO2 sorbent was prepared from the catalytic converters of spent automotive exhaust system by modifying with polyethyleneimine (PEI) through wet impregnation method. The prepared sorbent was characterized by scanning electron microscopy (SEM), Fourier transform infrared spectroscopy (FT-IR), thermogravimetric analysis (TGA), X-ray diffraction (XRD) and Brauer-Emmett-teller (BET) analysis before and after functionalization. Different PEI loadings were employed to study the adsorption performance of as-prepared sorbent. The characterization results showed no significant change in the structure, but the surface area was decreased after modification with amine groups. The adsorption was remarkably improved by increasing the PEI loading. The optimal PEI loading on the as-prepared sorbent was 60 wt.%. At optimal PEI loading, the CO2 adsorption reached to 101.3 mg (g sorbent)–1 at 70°C and partial pressure of 100 kPa. Further PEI loading had a negative effect on the adsorption. The CO2 adsorption capacity increased to 125.2 mg (g sorbent) –1 in the presence of 1 vol.% of the H2O moisture. Results confirmed the high performance of novel sorbent compared to other porous sorbents such as carbon-based materials. Adsorption/desorption cycles revealed that the PEI-impregnated sorbent can be satisfactorily regenerated after CO2 adsorption process.
Introduction
Fossil fuels had a drastic increase in CO2 emissions during past two centuries by a rapid increase of the world population and industrial activities (Dutcher et al., 2015; Li et al., 2015; Lee et al., 2016; Sanz-Pérez et al., 2016; Hu et al., 2017). The greenhouse effect results from the disruption in carbon balance in earth’s atmosphere leads to environmental impacts on the biological ecosystems (Mofarahi et al., 2008; Songolzadeh et al., 2014; Pasieka et al., 2015; Figueiredo et al., 2016; Irani et al., 2016; Luis, 2016; Singh et al., 2016). Therefore, academic and industrial communities have focused on developing promising methods to mitigate the CO2 concentration to standard levels (Lin et al., 2014; Shekhah et al., 2014; Wang J. et al., 2015). CO2 is known as the most prevalent greenhouse gas which need to be significantly decreased through various approaches such as pre-combustion, post-combustion and oxy-fuel combustion. Among them, post-combustion CO2 capture has received a wide attention form the scientific society due to the most popularity in the industrial processes. There are different procedures to reduce the CO2 emissions including aqueous solutions of amine (Maleki et al., 2018), membrane separation (Rezakazemi et al., 2011), hybrid systems and sorbent adsorption (Irani et al., 2018b,c).
Amine solution is commonly used in the industrial processes including gas sweetening in the natural gas refining plants. There are various amine solvents employed in the acid gas absorption including MDEA, DEA, MEA and a mix of mentioned solvents. MDEA play a significant role due to high absorption capacity and thermal and mechanical stability. However, amine solvents are restricted by some phenomenon including foaming, degradation, carbamate formation, toxicity and volatility (Ghasemi et al., 2020).
The adsorption process is known as an effective method for separation of CO2 in industrial applications. One of the most promising ways to separate the CO2 is adsorption by porous materials (Silva et al., 2017; Gil et al., 2018; Prasetyo et al., 2018). Porous materials have many remarkable features including stability, reusability, high surface area, the minimal energy requirement for regeneration and selectivity for the desired molecules (Nugent et al., 2013). Porous solid adsorbents such as metal oxides (Cuéllar-Franca and Azapagic, 2015; Gunathilake et al., 2016), porous carbon based materials, metal-organic frameworks (MOFs) (Irani et al., 2018b), covalent organic frameworks (COFs) (Didas et al., 2015; Huang et al., 2016) and zeolites (Lee and Park, 2015; Kim et al., 2016) have been separately investigated. Recently, studies have been focused on the CO2 separation using functionalized such adsorbents with amine-based compounds to form a composite (Best et al., 2018; Du et al., 2018). This functionalization process resulted in some excellent features such as high CO2 selectivity, ability to regeneration and excellent adsorption capacities. Amine functionalized solid adsorbents have shown the CO2 adsorption through separate or simultaneous chemical and physical bond formation as well as pore diffusion (Fan et al., 2018). Amine-modified adsorbents are prepared by various strategies including grafting nitrogen based groups on the solid surface, physical impregnation of solid sorbents with polyethyleneimine (PEI) and nitrogen-doping of some materials with gaseous NH3. Metal oxides have been widely modified with some organic functional groups to be employed in various applications such as catalysis, separation, drug delivery and bioactivation (Jung et al., 2016; Hu et al., 2017; Molavi et al., 2018). PEI (branched structure) is the most frequently used amine for CO2 adsorption by solids because of its amine-rich structure (Jung et al., 2018; Peng et al., 2018). Carbon-based materials are the most prevalent sorbents used in various studies to investigate the CO2 adsorption capability. Irani et al. studied modified carbon nanotubes/tetraethylenepentaamine for CO2 adsorption and the results showed the sorption capacity of 5 mmol/g-sorbent at 60°C (Irani et al., 2017).
Car industry annually generates a high amount of spent car catalysts which resulting in economic and environmental consequences. Automotive catalytic converters emerged in mid-1970s in the United States to reduce the emission of cars. This catalyst mainly contains platinum or a combination of platinum and palladium to convert the hydrocarbons and CO to H2O and CO2. Other main elements available in these catalytic converters are rhodium and gold (Sabegh et al., 2018). However, automotive industry suffers from some disadvantages such as non-renewability of these metals, high price and the environmental impacts related to their extraction. There is promising interest toward the recovery of these metals from spent car catalytic converters which result in the economic and environmental consequences (Jimenez de Aberasturi et al., 2011). Recovering the platinum metal groups (PMGs) has been focused by researchers in recent year. In a typical recovery process, PGMs can be extracted from scraps through melting and collecting them by collector metals including copper. In another process, scraps are dissolved in strong acid and metals are collected (Jimenez de Aberasturi et al., 2011).
In this study, a novel cost-effective sorbent was prepared and studied as a high performance support for CO2 capture. Multi-metal oxides were recovered via a cost-effective, green sol-gel procedure from catalytic converters of spent exhaust systems. The obtained multi-metal oxide was used as a novel adsorbent after modifying with PEI. Multi-metal oxide mixture was fully characterized before and after the functionalization process; and the different PEI loading, adsorption temperature, and CO2 partial pressure effects were studied using a thermo-gravimetric analyzer (TGA).
Experimental
Materials
Spent catalytic converters of exhaust systems were obtained by Iran and Research center of Auto parts and components company (ITRAC Co.) to recover the metal oxide nanoparticles as a novel solid CO2 adsorbent. Other materials found in this study with their source and specifications are summarized in Table 1.
Recovering Process of Metal Oxides From Spent Catalytic Converters
Following the crushing and milling catalytic converters of spent exhaust systems and obtaining the utmost particle size of 0.1 mm, the obtained powder was transferred to a beaker (250 ml) accompanied by addition of 10 ml of distilled water, 10 ml of HNO3 and 25 ml of HClO4 and 10 ml of HF. Then, the solution was cooled right down to ambient temperature after heating at 120°C for 3 h. Heating and cooling process was repeated while 25 ml of HCl was added to the solution. A larger beaker (500 ml) containing 300 ml distilled water was prepared and top of the solvent of the former beaker was transferred into it. Afterward, the NH3 solution was added dropwise to separate the elements from the mixture. After collecting, washing and drying the solid part, a solution containing sufficient level of nitric acid was prepared.
Finally, 10 g gelatin was included with a 100 ml deionized water container to be able to synthesize metal oxide nanoparticles. The container was heated at 80°C, while the solution prepared in the earlier stage was added into the container until obtaining a mild brown color. The ultimate product was calcined at 500°C for 4 h to acquire metal oxide nanoparticles.
Preparation of PEI-Loaded Metal Oxide Nanoparticles
In order to prepare the PEI functionalized metal oxide nanoparticles, wet impregnation method was employed (Liu J. et al., 2012; Wang W. et al., 2015; Martín et al., 2016). For this specific purpose, PEI (in a desired amount) was dissolved in 20 ml of methanol under stirring for 15 min. Then, 0.5 g of metal oxide nanoparticles were added to the solution. The mixture was continuously stirred at 50°C for 3.5 h, followed closely by sealing at 50°C for 12 h. Afterward, the resultant slurry was dried at 80°C to obtain the amine modified sorbent. The as-prepared sorbent was impregnated with the different mass fraction of PEI (30, 40, 50, 60, and 70 wt.%). The morphology of recovered sorbent was characterized before and after impregnation with PEI. X-ray diffraction (XRD) pattern was performed using Xpert MPD diffractometer. Field emission scanning electron was recorded by a Tuscan MIR3 LM. The thermal stability was examined with TGA (Perkin-Elmer). Finally, FT-IR absorption was employed on a Bruker ISS-88 to verify the sorbent nature.
CO2 Adsorption and Regeneration Process
CO2 adsorption measurements of PEI functionalized MMO nanoparticles were performed by TGA (Pyris 1, PerkinElmer TG analyzer, United States) (Lee et al., 2015). In this task, the specified amount (10 mg) of PEI impregnated MMO was placed into an alumina crucible. The temperature was changed from room temperature to 100°C with an interest rate of 5°C/min under a natural N2 flow (50 ml/min) to eliminate the adsorbed CO2 and moisture available in the structure. Then, after cooling the sample to the required temperature (25, 40, 65, 70, 90°C), N2 flow was switched to CO2 (pure) or CO2/N2 (mixture) and any change in the sample weight was simultaneously recorded. To be able to desorption measurements, the gas flow was switched to N2 (pure) with a temperature rate of 10°C/min to 110°C.
Results and Discussion
Characterization of Pristine MMO and PEI Functionalized MMO Recovered From Spent Car Catalytic Converter
FESEM-EDS analysis was performed to study the morphological structure of nanoparticles and verify amounts and types of metals available in the structure of the sorbent. As shown in Figure 1, a porous structure has been verified as a result of chemical composition of the coat which will be mainly Al2O3 and ZrO2 nanoparticles. Different size range (9–60 nm) of the cubic crystalline particles is due to the presence of CeO2, MgO, and ZrO2 compounds in the structure. The higher degree of oxygen detected in the structure is definitely an indicator of the accessibility of metals within their oxide form that will be desired for amine modification. Aluminum is the absolute most prevalent substance in the structure of as-prepared sorbent, while the automotive catalytic converters are often formed by an alumina-coated ceramic structure to aid the platinum group metals (PGMs) (Jimenez de Aberasturi et al., 2011). The SEM images of PEI modified MMO reveal a retained structure after modification. However, the meso and micro-pores are blocked after functionalization because of the presence of amine groups in the structure. The EDS analysis verifies the clear presence of N atoms in the structure after modification by the amine.
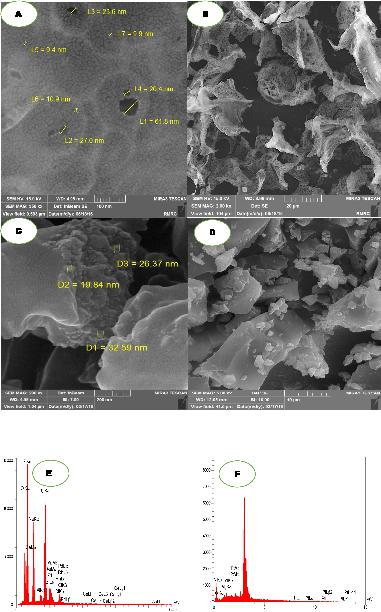
Figure 1. SEM images of (A,B) pristine MMO, (C,D) MMO-PEI-60, EDS analysis of recycled multi-metal oxide prepared from the spent car catalytic converter (E) and EDS analysis of 60 wt.% PEI loading (F).
X-ray diffraction (XRD) patterns of nanoparticles show the Bragg’s peaks in the product range of 2Theta values between 5° and 90°. The presence of Al2O3 and Au particles were completely confirmed in the line with the standard patterns. The sample shows the exact same diffraction peaks with lower intensities after impregnation which indicates the retained structure after modification process, and intensities of the peaks became lower with increasing the PEI contents (Zaman et al., 2010; Strunk et al., 2011; Cortes Vega et al., 2017; Figure 2). According to EDS analysis presented in Figure 1, The catalyst has a porous structure which could be in association with the chemical composition of the coat (mostly Al2O3, ZrO2). The cubic crystalline particles, which are in the different size ranges between 9 and 60 nm, are assigned to CeO2, ZrO2, MgO compounds. The higher amount of Oxygen (42.06 wt%) show that the detected elements such as Al, Au, Ce, Zr, Si, Mg and Ba are available in their oxide form. Because the car catalytic converters are formed by an alumina-coated ceramic structure which supports the PGMs, So, aluminum must be the most prevalent substance in the prepared catalyst.
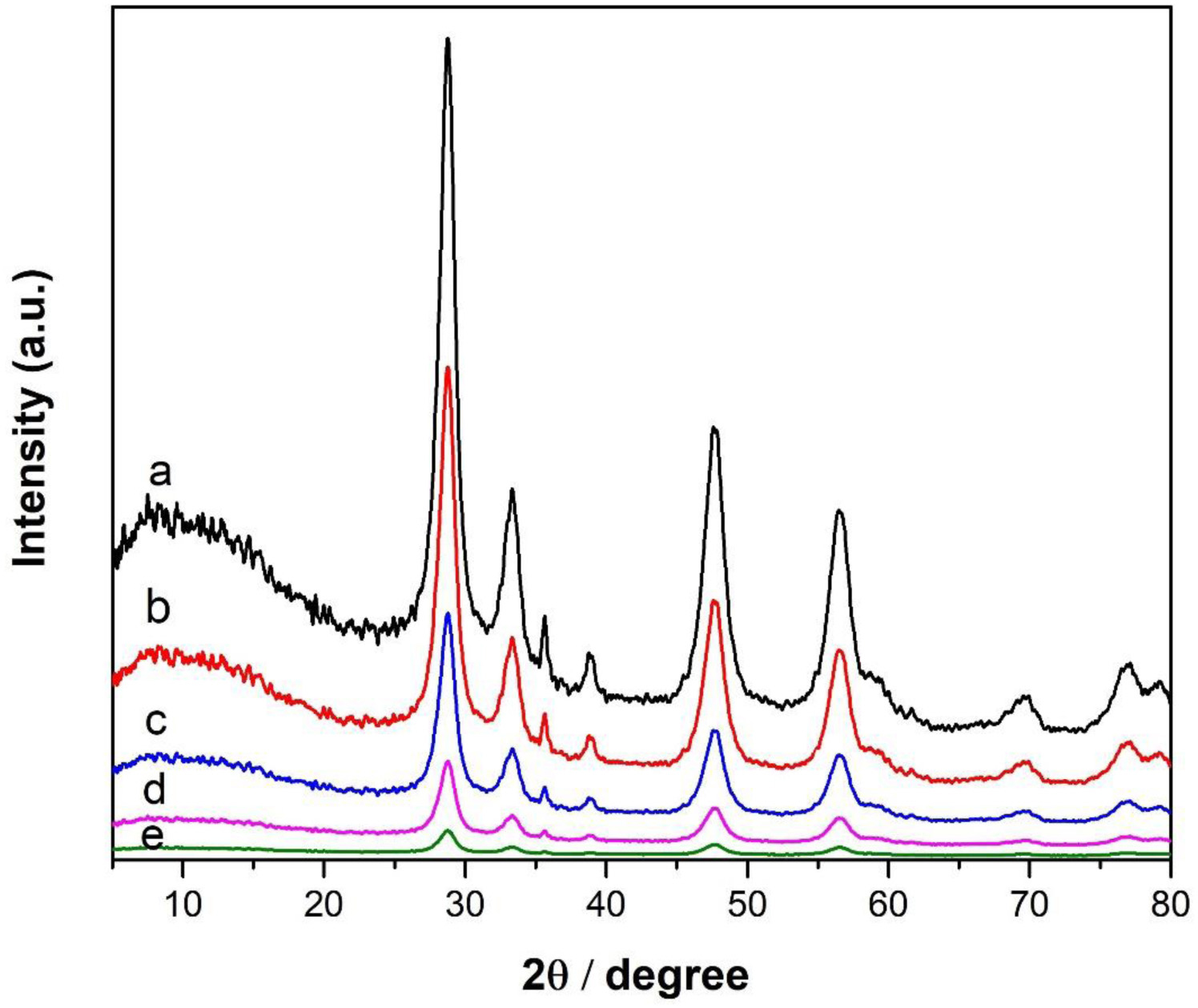
Figure 2. XRD patterns of pristine MMO before and after PEI loading: (a) pristine MMO; (b) MMO-PEI-30; (c) MMO-PEI-40; (d) MMO-PEI-50; (e) MMO-PEI-60.
FT-IR spectroscopy showed two bands at 3600 and 3199 cm–1 which are caused by O-H bonds. As inferred from Figure 3, the bands at 2099 and 1652 cm–1 are linked to H-O-H bonds. The peak at 1072 cm–1 is attributed to the Al-O-H (Liu C. et al., 2012). The clear presence of Alumina in the structure is completely confirmed by these bands obtained in the FT-IR analysis. Ce-O stretching vibration is verified by bands at 848 and 521 cm–1. The bands round the 1700 cm–1 verify the current presence of metal oxides in the structure (Liu C. et al., 2012). The presence of PEI could be observed via FT-IR spectra. There are two important bands after functionalization emerged by amine groups. The absorption peak at 1556 cm–1 is attributed to stretching vibration along with symmetric and asymmetric bending vibration of the NH2 group. The stretching vibration of the CH2 groups at the absorption band of 2945 cm–1 demonstrates the successful loading of PEI which is also verified by literature (Thi Le et al., 2014; Niu et al., 2016; Irani et al., 2018b).
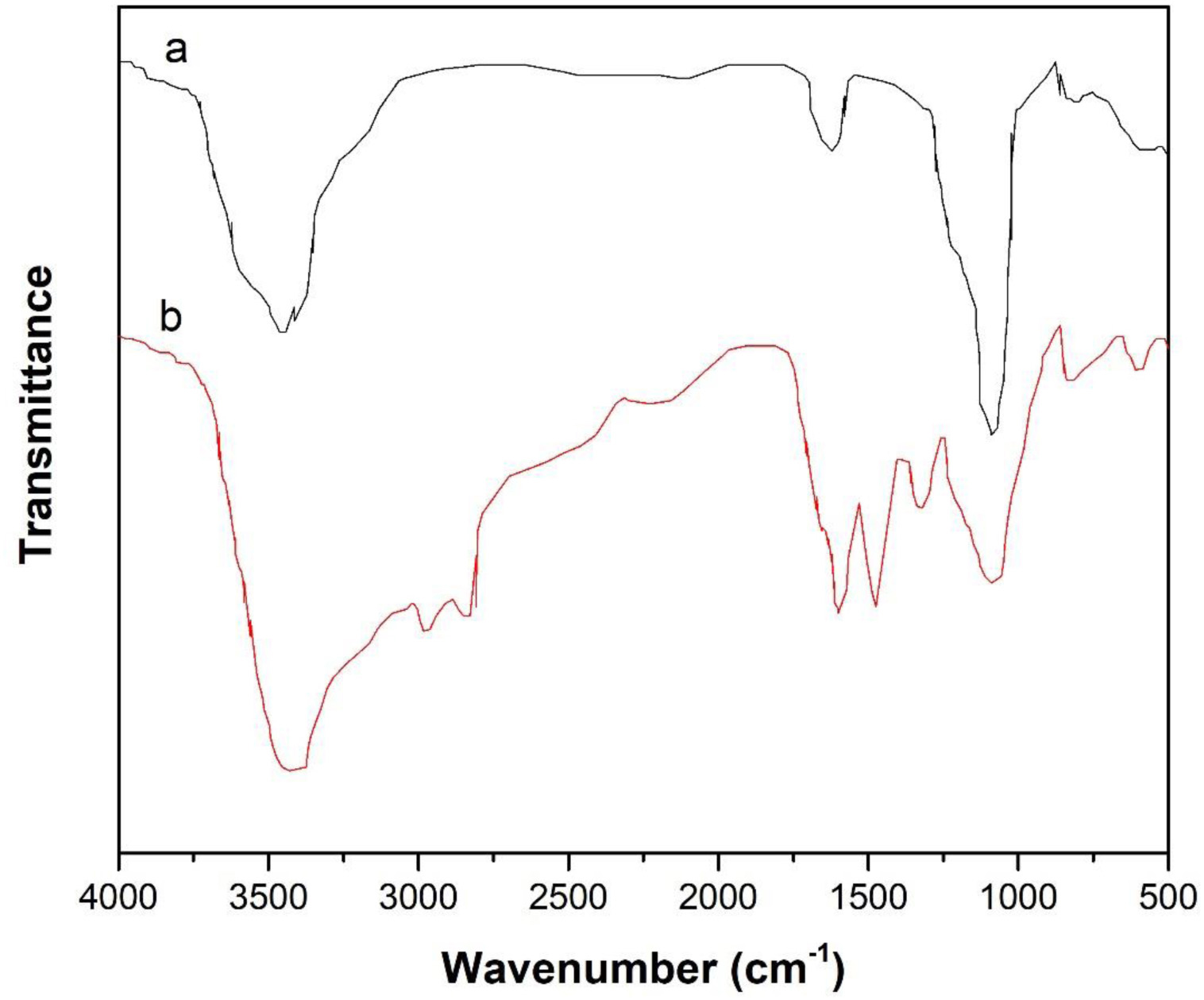
Figure 3. FTIR spectrum of pristine MMO before and after PEI loading: (a) pristine MMO and (b) MMO-PEI-60.
Thermo-gravimetric analyzer profile, which was performed from 40°C to 800°C with a rate of 40°C/min, shows a mass loss (10–15%) peaks around 150°C, which is mainly due to the evaporation of moisture. According to Figure 4, TGA profile also shows a mass loss peak around 250 which is associated the decomposition of PEI. So, based on these observations, MMO-PEI tolerates the temperatures up to 250°C, which is desirable for regeneration process at 110°C. The as-prepared sorbent showed an acceptable stability compared to carbon-based materials such as graphene and CNTs. As reported (Irani et al., 2017), MWCNTs/TEPA with TEPA loading of 75 wt.% showed a similar thermal stability in comparison with the novel sorbent prepared in this study. Carbon-based materials are well-known for their high thermal and mechanical stability and the TGA profile confirmed the high thermal stability of the novel sorbent compared with carbon-based materials.
N2 adsorption/desorption isotherms of MMO was also examined before and following the modification process by PEI. The hysteric loops observed at 0.2 < P/P0 < 1 indicates the mesoporous structure. As shown in Figure 5, obvious changes are determined after PEI loading as a result of presence of amine groups in the pore channels of MMO nanoparticles. So, decreases in N2 capacity adsorption confirmed this fact that the pores were gradually filled by PEI molecules resulted in slimmer hysteric loops. According to Figure 6, the mesopores were centered at 1 nm. Therefore, increasing the PEI loading contributes to an entire filling in the pores (Samanta et al., 2012; Molavi et al., 2018). The decrease of pore size of MMO after PEI loading was because of the modification of amine groups on MMO. Table 2 presents the specific surface area and total pore volumes for pristine MMO and functionalized MMOs. As inferred from Table 2, the total pore volume of mesopores as well as SBET decreased with increasing the amount of PEI loading. So, impregnation of MMO with PEI triggered covering the micropores and mesopores of MMO with amine groups.
CO2 Adsorption of MMO-PEI
After impregnation of MMO with amine groups, the modified surface comes with an affinity for acidic gases. CO2 will react with PEI at first glance of MMO as opposed to inner PEI (Irani et al., 2015). The adsorption capacity is mainly depended on the accessibility of amine groups on the surface sample to interact with carbon dioxide. More sites were provided after more loadings. However, too much PEI loading agglomerated together resulted in the hindered pores for adsorption. The reactions associated with CO2 adsorption process are presented as follows.
The outcomes of CO2 adsorption of modified MMO with various quantities of PEI at 70°C and in CO2 partial pressure of 100 kPa are shown in Figure 7. Adsorption capacity was improved by increasing the total amount of PEI loading on the MMO. Actually, the interaction between PEI and CO2 molecules will be through acid-base chemical reaction by increasing the loading of PEI onto MMO surface in accordance with to Eq. 1, 2. The utmost adsorption capacity as a function of time for pristine MMO was just 10.8 mg/(g sorbent). The adsorption capacity of MMO-PEI-30 increased significantly to 55.6 mg/g as a result of amine loading onto the surface of MMO. These observations confirmed the effective capture of CO2 with amine loading. The adsorption capacity increased with increasing the PEI loading amount due to the presence of more amine sites available on the surface of the MMO (Irani et al., 2016). The maximum adsorption capacities for MMO-PEI-40 and MMO-PEI-50 was 76.3 and 81 mg/g, respectively. On the list of samples, MMO-PEI-60 showed the best CO2 adsorption capacity. The adsorption capacity with this sample as a function of time was 101.3 mg/g. Further PEI loading generated a reduction in adsorption capacity. For MMO-PEI-70, the adsorption capacity decreased to 66.2 mg/g weighed against MMO-PEI-60. Therefore, further PEI loading will cover the out lining of MMO and avoid the diffusion of CO2 molecules in to the inner spaces modified MMO with amine groups, and so the accessible sorption sites will undoubtedly be reduced after further functionalization (Irani et al., 2017). Compared to the literature, results showed a high adsorption capacity of the sorbent so that the sample with the optimum performance (MMO-PEI-60) revealed the adsorption capacity of 101.3 mg/g-sorbents (Irani et al., 2017, 2018a).
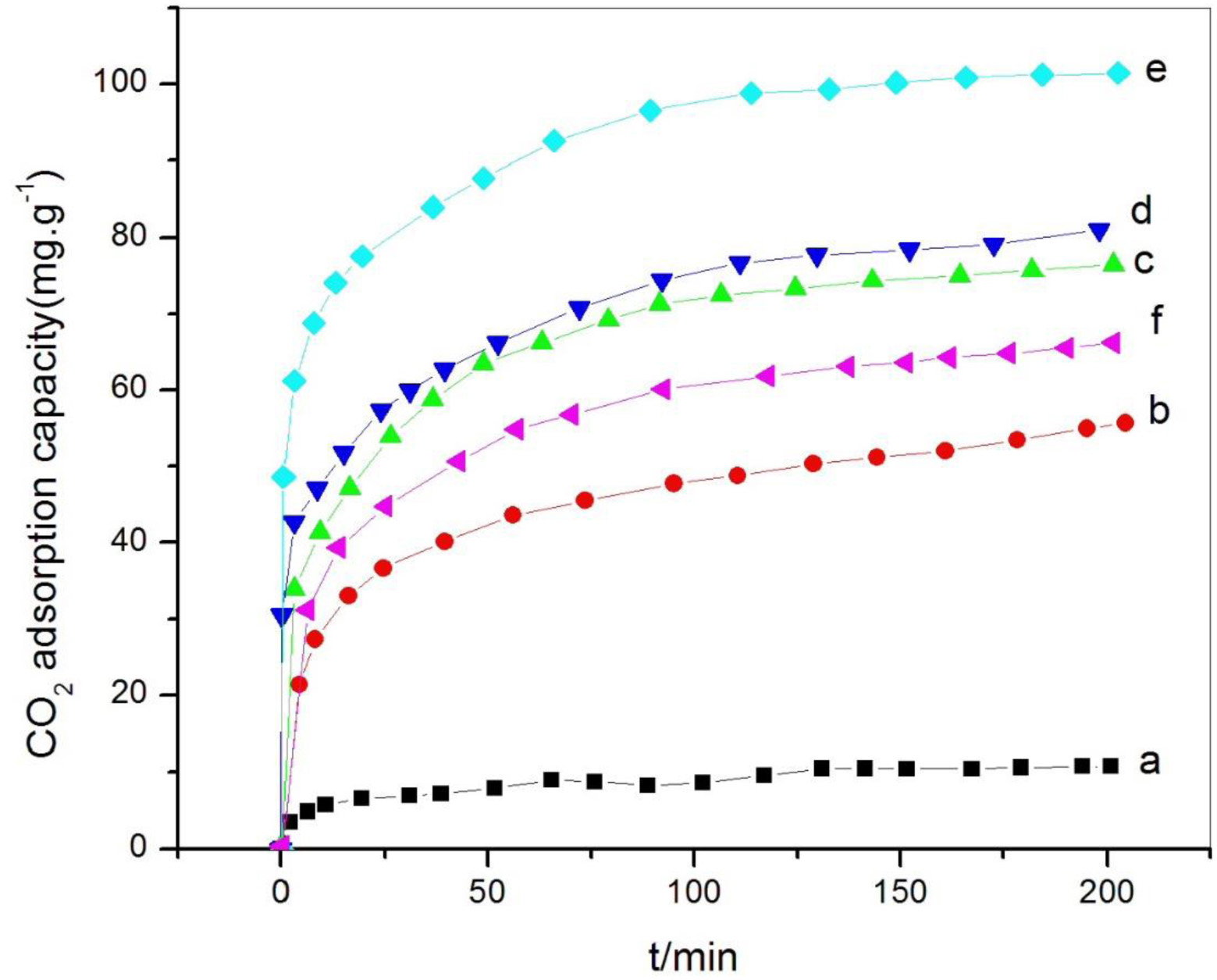
Figure 7. CO2 sorption capacities of pristine MMO before and after PEI loading: (a) pristine MMO; (b) MMO-PEI-30; (c) MMO-PEI-40; (d) MMO-PEI-50; (e) MMO-PEI-60 and (f) MMO-PEI-70 at 70°C.
In amine-based sorbents, the CO2 adsorption capacity is affected directly by the total amount of amine loading. As shown in Figure 8, increasing the PEI loading features a positive effect on the CO2 sorption. The CO2 sorption risen up to 101.3 mg/g with increasing the PEI loading from 30 to 60 wt.%. Further PEI loading resulted in a reduced amount of CO2 sorption capacity. This phenomenon could be linked to the reduced accessible amine sites available on the sorbent surface as a result of amine agglomeration.
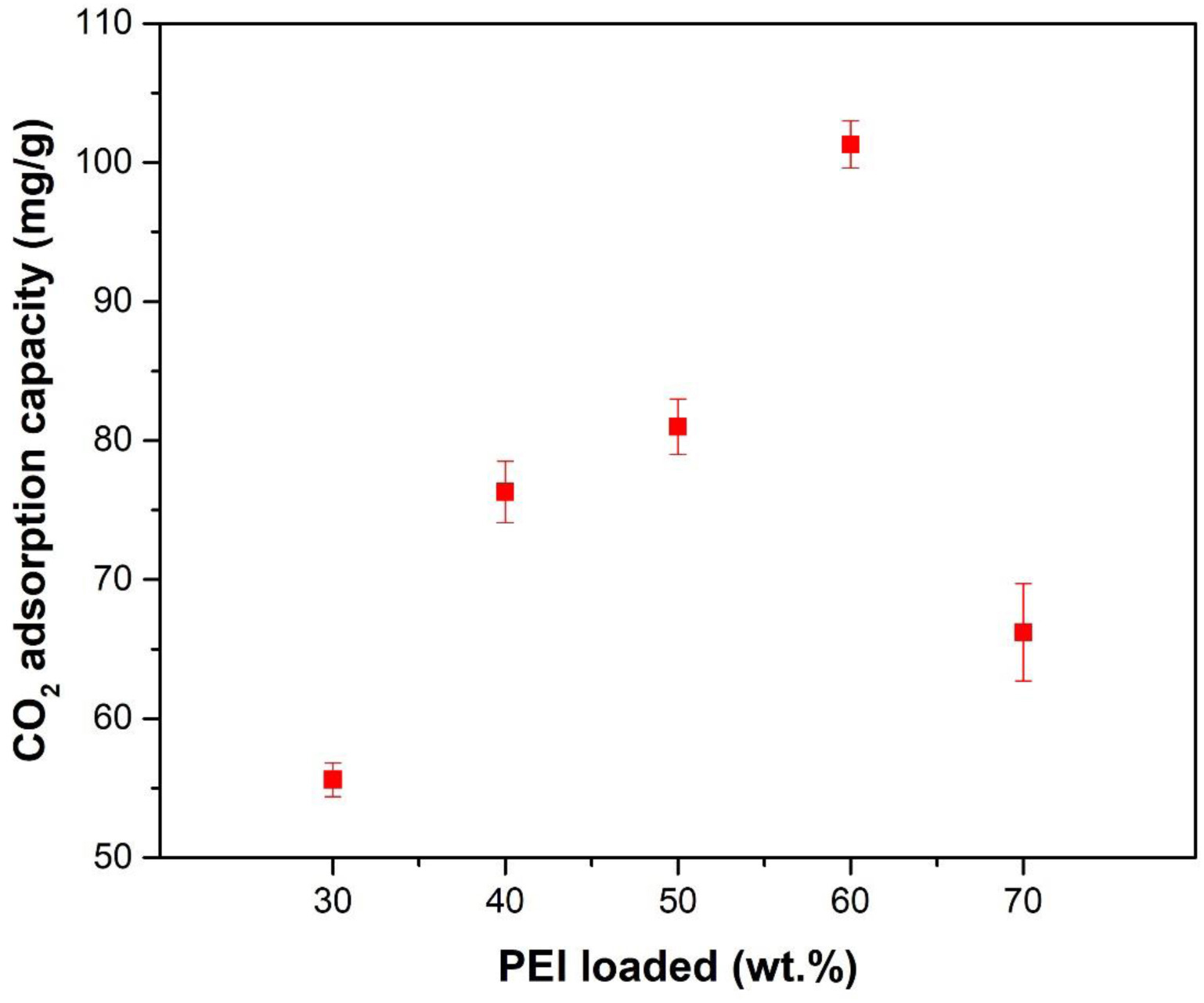
Figure 8. The effect of PEI loading on the CO2 adsorption capacity at 70°C and in CO2 partial pressure of 100 kPa.
Effect of Partial Pressure on CO2 Adsorption Capacity
Figure 9 shows the adsorption capacity of MMO-PEI-60 at 70°C and in partial pressures of 7, 25, 50, 70, 80, and 90 kPa. Partial pressure had a positive effect on the adsorption capacity of samples. The maximum adsorption capacity was 92.7 mg/g in partial pressure of 90 kPa. Partial pressure has always a direct effect on both absorption and adsorption processes.
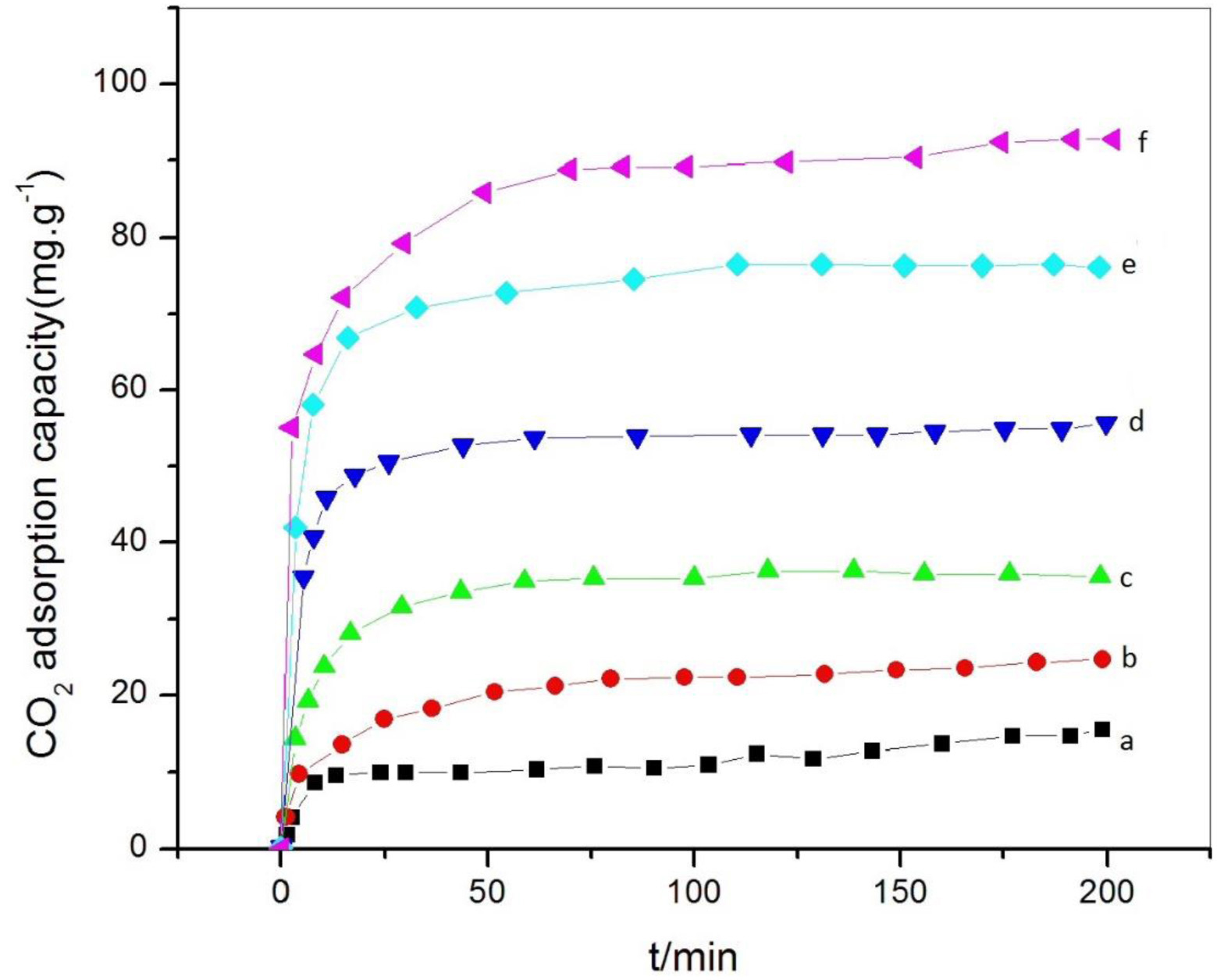
Figure 9. CO2 adsorption capacity of MMO-PEI-60 at 70°C and in partial pressures of (a) 7; (b) 25; (c) 50; (d) 70; (e) 80 and (f) 90 kPa.
Effect of Temperature on CO2 Adsorption Capacity
The adsorption capacity of MMO-PEI-60 was evaluated at different temperatures and in partial pressure of 100 kPa. As given in Figure 10, the adsorption capacity raised with increasing the temperature to 75°C. Increasing temperature accelerated the amine molecules motion which led to the enhanced sorption capacity. However, the further increase in temperature triggered a slight decline in adsorption capacity because of the thermodynamic effects. In reality, at higher temperatures, desorption could be the dominant reaction compared to adsorption. CO2 adsorption is controlled by kinetics at low temperature. Only at that array of temperature, PEI includes a high viscosity and adsorption capacity is reduced by this factor. At higher temperatures, the reaction is principally controlled by thermodynamic factors and active CO2-affinity sites may be utilized. When the temperature raised up to 70°C, the equilibrium shifted to desorption process and adsorption capacity decreased. Two stage process can be observed for adsorption. The beginning sharp linear adsorption is due to the surface chemical reaction and the second is attributed to the slow diffusion stage. Therefore, at the beginning stage, the chemical reaction on the sample surface determines the adsorption rate. So, the perfect adsorption temperature for MMO-PEI-60 was 70°C in line with the effect of kinetics and thermodynamics.
Effect of Moisture on the CO2 Adsorption Capacity
All types of gases typically contain some moisture; so, examination the aftereffect of presence of moisture is crucial for CO2 adsorption measurement. As shown in Figure 11, addition of 1 vol.% resulted in increase in the CO2 sorption capacity to 125.2 mg/g. Further concentration of moisture caused a lowering of CO2 sorption capacity. This could be due to the occupying some accessible surface area by water molecules. As previously mentioned, under dry conditions, carbamates are the product of the reaction between amine groups and CO2 molecule. In the current presence of the moisture, both bicarbonates could be formed when CO2 molecule reacts with one amine groups (Irani et al., 2015, 2016; Guo et al., 2016). The reactions under wet conditions are presented as follows:
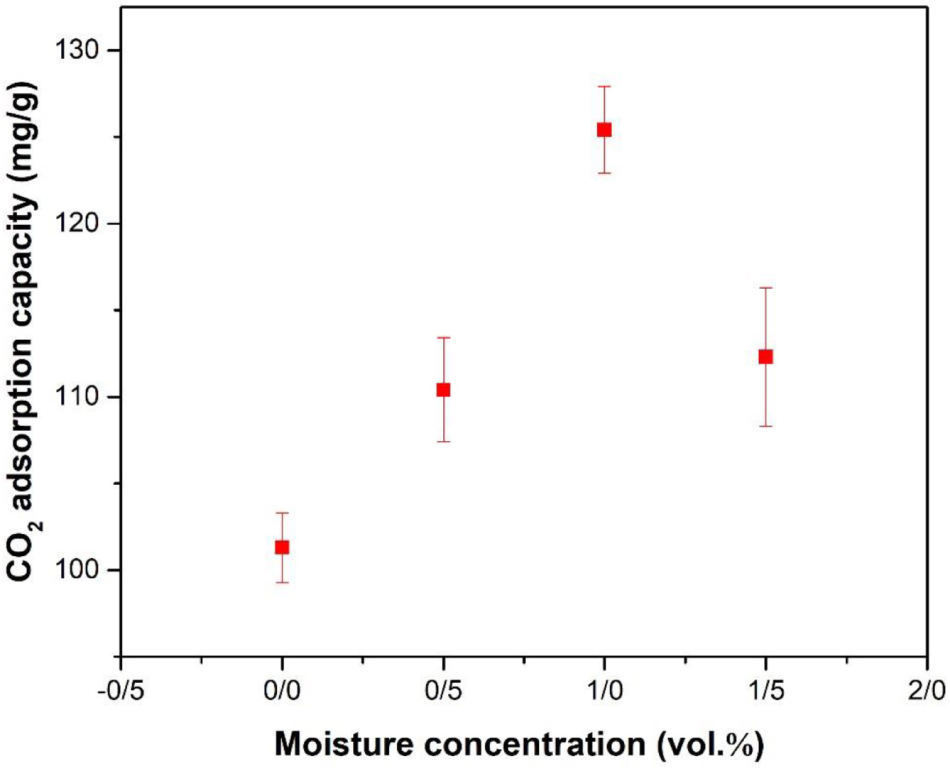
Figure 11. Effect of moisture on CO2 adsorption capacity at 70°C in the presence of 0.5, 1, 1.5 vol.% of the H2O moisture.
Determining the Selectivity of CO2 Over N2
Kinetic diameters for CO2 and N2 are 3.3 and 3.64 Å, respectively. However, coulombic forces are present in the interactions between CO2 and amine groups. CO2 shows a strong chemisorption and physisorption interactions with polar amine groups. On the other side, N2 only interacts via physisorption and intermolecular interactions (van der waals) (Shin et al., 2016). So, Henry’s law selectivity can be calculated through Toth model at low pressure (Bali et al., 2015).
Where Q presents the gas capacity, Qmax is the maximum gas capacity, and A and n are constant values.
Thus, Henry’s constant can be calculated as follows:
So, the adsorption selectivity for x over y is calculated as
The selectivity of MMO-PEI-60 was calculated using the mentioned equations. Figure 12 shows the CO2 and N2 adsorption isotherms of MMO-PEI-60 at 70°C and partial pressure range of 0 to 100 kPa.
The selectivity of MMO-PEI-60 toward CO2 over N2 was calculated as high as 50.2. This selectivity value confirms that MMO-PEI has a high affinity for CO2 because impregnated amine groups lead to an enhancement in the interactions between CO2 gases and the impregnated sorbents.
Regenability of the Sorbent
Cyclic CO2 adsorption was performed at 70°C and partial pressure of 100 kPa to investigate the regeneration properties of the MMO-PEI-60. The regeneration process plays a critical role in the case of lowering the cost of power plants (Lee et al., 2016; Ntiamoah et al., 2016). The results of the regeneration measurements for MMO-PEI-60 over 7 cycles are shown in Figure 13. These results indicate that the MMO-PEI sorbents show a good stability and regeneration.
Conclusion
An inexpensive and recovered sorbent for CO2 adsorption has been prepared from catalytic converters of spent exhaust systems and functionalized with polyethyleneimine through wet impregnation method. The thermal stability of sorbent and functionalized samples showed that the modified multi-metal oxides/PEI are stable below 250°C. The sorbent was treated by acid to increase the surface area. The PEI loading on the sorbent was optimized as 60 wt.% with maximum CO2 sorption capacity of 101.3 mg (g sorbent) –1 at 70°C and partial pressure of 100 kPa. Higher adsorption capacity was achieved by increasing the CO2 partial pressure. The CO2 adsorption capacity increased to 125.2 in the presence of 1 vol.% of the H2O moisture. The selectivity of MMO-PEI-60 toward CO2 over N2 was calculated as high as 50.2. The regeneration measurements for MMO-PEI-60 over 7 cycles confirmed a good regenerability for modified MMO/PEI sorbent.
Data Availability Statement
The raw data supporting the conclusions of this article will be made available by the authors, without undue reservation.
Author Contributions
All authors listed have made a substantial, direct and intellectual contribution to the work, and approved it for publication.
Conflict of Interest
The authors declare that the research was conducted in the absence of any commercial or financial relationships that could be construed as a potential conflict of interest.
Supplementary Material
The Supplementary Material for this article can be found online at: https://www.frontiersin.org/articles/10.3389/fenrg.2020.00196/full#supplementary-material
FIGURE S1 | EDS analysis of recycled multi-metal oxide prepared from the spent car catalytic converter.
FIGURE S2 | EDS analysis of 60 wt.% PEI loading.
References
Bali, S., Sakwa-Novak, M. A., and Jones, C. W. (2015). Potassium incorporated alumina based CO2 capture sorbents: Comparison with supported amine sorbents under ultra-dilute capture conditions. Colloids Surfaces A Physicochem. Eng. Asp. 486, 78–85. doi: 10.1016/j.colsurfa.2015.09.020
Best, T., Finney, K. N., Ingham, D. B., and Pourkashanian, M. (2018). CO2-enhanced and humidified operation of a micro-gas turbine for carbon capture. J. Clean. Prod. 176, 370–381. doi: 10.1016/j.jclepro.2017.12.062
Cortes Vega, F. D., Martinez Torres, P. G., Pichardo Molina, J., Gomez Ortiz, N. M., Hadjiev, V. G., Zarate Medina, J., et al. (2017). Gold nanoparticle SERS substrates sustainable at extremely high temperatures. J. Mater. Chem. C 5, 4959–4966. doi: 10.1039/C7TC00527J
Cuéllar-Franca, R. M., and Azapagic, A. (2015). Carbon capture, storage and utilisation technologies: A critical analysis and comparison of their life cycle environmental impacts. J. CO2 Util. 9, 82–102. doi: 10.1016/j.jcou.2014.12.001
Didas, S. A., Choi, S., Chaikittisilp, W., and Jones, C. W. (2015). Amine-Oxide Hybrid Materials for CO2 Capture from Ambient Air. Acc. Chem. Res. 48, 2680–2687. doi: 10.1021/acs.accounts.5b00284
Du, Y., Gai, W. M., and Jin, L. Z. (2018). A novel and green CO2adsorbent developed with high adsorption properties in a coal mine refuge chamber. J. Clean. Prod. 176, 216–229. doi: 10.1016/j.jclepro.2017.12.019
Dutcher, B., Fan, M., and Russell, A. G. (2015). Amine-based CO2 capture technology development from the beginning of 2013-A review. ACS Appl. Mater. Interfaces. 7, 2137–2148. doi: 10.1021/am507465f
Fan, Y. H., Zhang, S. W., Bin Qin, S., Li, X. S., and Qi, S. H. (2018). An enhanced adsorption of organic dyes onto NH2functionalization titanium-based metal-organic frameworks and the mechanism investigation. Microporous Mesoporous Mater. 263, 120–127. doi: 10.1016/j.micromeso.2017.12.016
Figueiredo, M. C., Ledezma-Yanez, I., and Koper, M. T. M. (2016). In situ Spectroscopic study of CO2 electroreduction at copper electrodes in acetonitrile. ACS Catal. 6, 2382–2392. doi: 10.1021/acscatal.5b02543
Ghasemi, M. H., Irani, V., and Tavasoli, A. (2020). Amino functionalized ZIF-90@GO/MDEA nanofluid: As a new class of multi-hybrid systems to enhance the performance of amine solutions in CO2 absorption. J. Nat. Gas Sci. Eng. 74:103110. doi: 10.1016/j.jngse.2019.103110
Gil, A., Arrieta, E., Vicente, M. A., and Korili, S. A. (2018). Synthesis and CO2adsorption properties of hydrotalcite-like compounds prepared from aluminum saline slag wastes. Chem. Eng. J. 334, 1341–1350. doi: 10.1016/j.cej.2017.11.100
Gunathilake, C., Gangoda, M., and Jaroniec, M. (2016). Mesoporous alumina with amidoxime groups for CO 2 sorption at ambient and elevated temperatures. Ind. Eng. Chem. Res. 55, 5598–5607. doi: 10.1021/acs.iecr.6b00674
Guo, L., Yang, J., Hu, G., Hu, X., DaCosta, H., and Fan, M. (2016). CO2 removal from flue gas with amine-impregnated titanate nanotubes. Nano Energy. 25, 1–8. doi: 10.1016/j.nanoen.2016.04.038
Hu, H., Zhang, T., Yuan, S., and Tang, S. (2017). Functionalization of multi-walled carbon nanotubes with phenylenediamine for enhanced CO<inf>2</inf> adsorption. Adsorption 23, 73–85. doi: 10.1007/s10450-016-9820-y
Huang, K., Chai, S. H., Mayes, R. T., Tan, S., Jones, C. W., and Dai, S. (2016). Significantly increasing porosity of mesoporous carbon by NaNH2 activation for enhanced CO2 adsorption. Microporous Mesoporous Mater. 230, 100–108. doi: 10.1016/j.micromeso.2016.04.041
Irani, M., Fan, M., Tuwati, A., Dutcher, B., and Russell, A. G. (2015). Modi fi ed nanosepiolite as an inexpensive support of tetraethylenepentamine for CO 2 sorption. Nano Energy 11, 235–246. doi: 10.1016/j.nanoen.2014.11.005
Irani, M., Gasem, K. A. M., Dutcher, B., and Fan, M. (2016). CO 2 capture using nanoporous TiO (OH) 2/tetraethylenepentamine. Fuel 183, 601–608. doi: 10.1016/j.fuel.2016.06.129
Irani, M., Jacobson, A. T., Gasem, K. A. M., and Fan, M. (2017). Modified carbon nanotubes/tetraethylenepentamine for CO 2 capture. Fuel 206, 10–18. doi: 10.1016/j.fuel.2017.05.087
Irani, M., Jacobson, A. T., Gasem, K. A. M., and Fan, M. (2018a). Facilely synthesized porous polymer as support of poly (ethyleneimine) for effective CO 2 capture. Energy 157, 1–9. doi: 10.1016/j.energy.2018.05.141
Irani, V., Tavasoli, A., Maleki, A., and Vahidi, M. (2018b). Polyethyleneimine-functionalized HKUST-1/MDEA nanofluid to enhance the absorption of CO2in gas sweetening process. Int. J. Hydrogen Energy 43, 5610–5619. doi: 10.1016/j.ijhydene.2018.01.120
Irani, V., Tavasoli, A., and Vahidi, M. (2018c). Journal of Colloid and Interface Science Preparation of amine functionalized reduced graphene oxide/methyl diethanolamine nanofluid and its application for improving the CO 2 and H 2 S absorption. J. Colloid Interface Sci. 527, 57–67. doi: 10.1016/j.jcis.2018.05.018
Jimenez de Aberasturi, D., Pinedo, R., Ruiz de Larramendi, I., Ruiz de Larramendi, J. I., and Rojo, T. (2011). Recovery by hydrometallurgical extraction of the platinum-group metals from car catalytic converters. Miner. Eng. 24, 505–513. doi: 10.1016/j.mineng.2010.12.009
Jung, H., Lee, C. H., Jeon, S., Jo, D. H., Huh, J., and Kim, S. H. (2016). Effect of amine double-functionalization on CO2adsorption behaviors of silica gel-supported adsorbents. Adsorption 22, 1137–1146. doi: 10.1007/s10450-016-9837-2
Jung, W., Park, J., and Lee, K. S. (2018). Kinetic modeling of CO 2 adsorption on an amine-functionalized solid sorbent. Chem. Eng. Sci. 177, 122–131. doi: 10.1016/j.ces.2017.11.003
Kim, C., Cho, H. S., Chang, S., Cho, S. J., and Choi, M. (2016). An ethylenediamine-grafted Y zeolite: a highly regenerable carbon dioxide adsorbent via temperature swing adsorption without urea formation. Energy Environ. Sci. 9, 1803–1811. doi: 10.1039/C6EE00601A
Lee, J. W., Torres Pineda, I., Lee, J. H., and Kang, Y. T. (2016). Combined CO2absorption/regeneration performance enhancement by using nanoabsorbents. Appl. Energy 178, 164–176. doi: 10.1016/j.apenergy.2016.06.048
Lee, M.-S., Lee, S.-Y., and Park, S.-J. (2015). Preparation and characterization of multi-walled carbon nanotubes impregnated with polyethyleneimine for carbon dioxide capture. Int. J. Hydrogen Energy. 40, 3415–3421. doi: 10.1016/j.ijhydene.2014.12.104
Lee, S. Y., and Park, S. J. (2015). A review on solid adsorbents for carbon dioxide capture. J. Ind. Eng. Chem. 23, 1–11. doi: 10.1016/j.jiec.2014.09.001
Li, C., Shi, H., Cao, Y., Kuang, Y., Zhang, Y., Gao, D., et al. (2015). Modeling and optimal operation of carbon capture from the air driven by intermittent and volatile wind power. Energy 87, 201–211. doi: 10.1016/j.energy.2015.04.098
Lin, Y., Lin, H., Wang, H., Suo, Y., Li, B., Kong, C., et al. (2014). Enhanced selective CO 2 adsorption on polyamine/MIL-101(Cr) composites. J. Mater. Chem. A 2, 1–9. doi: 10.1039/C4TA01174K
Liu, C., Shih, K., Gao, Y., Li, F., and Wei, L. (2012). Dechlorinating transformation of propachlor through nucleophilic substitution by dithionite on the surface of alumina. J. Soils Sediments 12, 724–733. doi: 10.1007/s11368-012-0506-0
Liu, J., Liu, Y., Wu, Z., Chen, X., Wang, H., and Weng, X. (2012). Polyethyleneimine functionalized protonated titanate nanotubes as superior carbon dioxide adsorbents. J. Colloid Interface Sci. 386, 392–397. doi: 10.1016/j.jcis.2012.07.048
Luis, P. (2016). Use of monoethanolamine (MEA) for CO2 capture in a global scenario: Consequences and alternatives. Desalination 380, 93–99. doi: 10.1016/j.desal.2015.08.004
Maleki, A., Irani, V., Tavasoli, A., and Vahidi, M. (2018). Enhancement of CO2 solubility in a mixture of 40wt% aqueous N-Methyldiethanolamine solution and diethylenetriamine functionalized graphene oxide. J. Nat. Gas Sci. Eng. 55, 219–234. doi: 10.1016/j.jngse.2018.04.032
Martín, C. F., Sweatman, M. B., Brandani, S., and Fan, X. (2016). Wet impregnation of a commercial low cost silica using DETA for a fast post-combustion CO2 capture process. Appl. Energy 183, 1705–1721. doi: 10.1016/j.apenergy.2016.09.081
Mofarahi, M., Khojasteh, Y., Khaledi, H., and Farahnak, A. (2008). Design of CO2 absorption plant for recovery of CO2 from flue gases of gas turbine. Energy 33, 1311–1319. doi: 10.1016/j.energy.2008.02.013
Molavi, H., Eskandari, A., Shojaei, A., and Mousavi, S. A. (2018). Enhancing CO2/N2 adsorption selectivity via post-synthetic modification of NH2-UiO-66(Zr). Microporous Mesoporous Mater. 257, 193–201. doi: 10.1016/j.micromeso.2017.08.043
Niu, M., Yang, H., Zhang, X., Wang, Y., and Tang, A. (2016). Amine-Impregnated Mesoporous Silica Nanotube as an Emerging Nanocomposite for CO2 Capture. ACS Appl. Mater. Interfaces 8, 17312–17320. doi: 10.1021/acsami.6b05044
Ntiamoah, A., Ling, J., Xiao, P., Webley, P. A., and Zhai, Y. (2016). CO2 Capture by Temperature Swing Adsorption: Use of Hot CO2-Rich Gas for Regeneration. Ind. Eng. Chem. Res. 55, 703–713. doi: 10.1021/acs.iecr.5b01384
Nugent, P., Belmabkhout, Y., Burd, S. D., Cairns, A. J., Luebke, R., Forrest, K., et al. (2013). Porous materials with optimal adsorption thermodynamics and kinetics for CO2 separation. Nature 495, 80–84. doi: 10.1038/nature11893
Pasieka, J., Jorge, L., Coulombe, S., and Servio, P. (2015). Effects of as-produced and amine-functionalized multi-wall carbon nanotubes on carbon dioxide hydrate formation. Energy Fuels 29, 5259–5266. doi: 10.1021/acs.energyfuels.5b01036
Peng, J., Iruretagoyena, D., and Chadwick, D. (2018). Hydrotalcite/SBA15 composites for pre-combustion CO 2 capture: CO 2 adsorption characteristics. J. CO2 Util. 24, 73–80. doi: 10.1016/j.jcou.2017.12.004
Prasetyo, L., Do, D. D., and Nicholson, D. (2018). A coherent definition of Henry constant and isosteric heat at zero loading for adsorption in solids – An absolute accessible volume. Chem. Eng. J. 334, 143–152. doi: 10.1016/j.cej.2017.10.022
Rezakazemi, M., Niazi, Z., Mirfendereski, M., Shirazian, S., Mohammadi, T., and Pak, A. (2011). CFD simulation of natural gas sweetening in a gas-liquid hollow-fiber membrane contactor. Chem. Eng. J. 168, 1217–1226. doi: 10.1016/j.cej.2011.02.019
Sabegh, M. Y., Norouzi, O., Jafarian, S., Khosh, A. G., and Tavasoli, A. (2018). Pyrolysis of marine biomass to produce bio-oil and its upgrading using a novel multi-metal catalyst prepared from the spent car catalytic converter. Bioresour. Technol. 249, 473–478. doi: 10.1016/j.biortech.2017.10.017
Samanta, A., Zhao, A., Shimizu, G. K. H., Sarkar, P., and Gupta, R. (2012). Post-combustion CO2 capture using solid sorbents: A review. Ind. Eng. Chem. Res. 51, 1438–1463. doi: 10.1021/ie200686q
Sanz-Pérez, E. S., Murdock, C. R., Didas, S. A., and Jones, C. W. (2016). Direct Capture of CO2 from ambient air. Chem. Rev. 116, 11840–11876. doi: 10.1021/acs.chemrev.6b00173
Shekhah, O., Belmabkhout, Y., Chen, Z., Guillerm, V., Cairns, A., Adil, K., et al. (2014). Made-to-order metal-organic frameworks for trace carbon dioxide removal and air capture. Nat. Commun. 5:4228. doi: 10.1038/ncomms5228
Shin, G. J., Rhee, K. Y., and Park, S. J. (2016). Improvement of CO2 capture by graphite oxide in presence of polyethylenimine. Int. J. Hydrogen Energy 41, 14351–14359. doi: 10.1016/j.ijhydene.2016.05.162
Silva, J. M., Trujillano, R., Rives, V., Soria, M. A., and Madeira, L. M. (2017). High temperature CO2 sorption over modified hydrotalcites. Chem. Eng. J. 325, 25–34. doi: 10.1016/j.cej.2017.05.032
Singh, A., Sharma, Y., Wupardrasta, Y., and Desai, K. (2016). Selection of amine combination for CO2 capture in a packed bed scrubber. Resour. Technol. 2, S165–S170. doi: 10.1016/j.reffit.2016.11.014
Songolzadeh, M., Soleimani, M., Takht Ravanchi, M., and Songolzadeh, R. (2014). Carbon dioxide separation from flue gases: A technological review emphasizing reduction in greenhouse gas emissions. Sci. World J. 2014:828131. doi: 10.1155/2014/828131
Strunk, J., Vining, W. C., and Bell, A. T. (2011). Synthesis of Different CeO2 structures on mesoporous silica and characterization of their reduction properties. J. Phys. Chem. C 115, 4114–4126. doi: 10.1021/jp1105746
Thi Le, M. U., Lee, S. Y., and Park, S. J. (2014). Preparation and characterization of PEI-loaded MCM-41 for CO2 capture. Int. J. Hydrogen Energy. 39, 12340–12346. doi: 10.1016/j.ijhydene.2014.04.112
Wang, J., Huang, H., Wang, M., Yao, L., Qiao, W., Long, D., et al. (2015). Direct capture of low-concentration CO<inf>2</inf> on mesoporous carbon-supported solid amine adsorbents at ambient temperature. Ind. Eng. Chem. Res. 54, 5319–5327. doi: 10.1021/acs.iecr.5b01060
Wang, W., Li, J., Wei, X., Ding, J., Feng, H., Yan, J., et al. (2015). Carbon dioxide adsorption thermodynamics and mechanisms on MCM-41 supported polyethylenimine prepared by wet impregnation method. Appl. Energy 142, 221–228. doi: 10.1016/j.apenergy.2014.12.072
Keywords: spent catalytic converter, polyethyleneimine, multi-metal oxides, CO2 adsorption, functionalizability
Citation: Irani V, Khosh AG and Tavasoli A (2020) Polyethyleneimine (PEI) Functionalized Metal Oxide Nanoparticles Recovered From the Catalytic Converters of Spent Automotive Exhaust Systems and Application for CO2 Adsorption. Front. Energy Res. 8:196. doi: 10.3389/fenrg.2020.00196
Received: 26 October 2019; Accepted: 27 July 2020;
Published: 05 November 2020.
Edited by:
Qinfen Gu, Australian Nuclear Science and Technology Organisation, AustraliaReviewed by:
Junfeng Wang, State Key Laboratory of Multiphase Complex Systems, Institute of Process Engineering (CAS), ChinaWei Liu, Molecule Works Inc., United States
Copyright © 2020 Irani, Khosh and Tavasoli. This is an open-access article distributed under the terms of the Creative Commons Attribution License (CC BY). The use, distribution or reproduction in other forums is permitted, provided the original author(s) and the copyright owner(s) are credited and that the original publication in this journal is cited, in accordance with accepted academic practice. No use, distribution or reproduction is permitted which does not comply with these terms.
*Correspondence: Vahid Irani, dmFoaWRpcmFuaTE0QHV0LmFjLmly; Ahmad Tavasoli, dGF2YXNvbGkuYUB1dC5hYy5pcg==; dmFoaWRpcmFuaTE0QGdtYWlsLmNvbQ==