- CO2 Utilisation Strategies and Society Research Group, Institute for Advanced Sustainability Studies, Potsdam, Germany
The implementation of the United Nations Sustainable Development Goals (SDGs) is indispensable for building a sustainable and just future for all humans and our planet. The SDGs are global goals. However, their implementation equally calls for action by a variety of actors in government, business, and civil society. Thus, policy making as well as industrial innovation efforts need to be designed to facilitate rather than hinder the implementation of the SDGs. Consequently, it is necessary to ensure that the possible environmental, economic, and societal impacts of technological innovations aiming for public support and funding in research, development, and market implementation are aligned with the respective objectives of the SDGs. Carbon capture and utilization (CCU) applications are an example of such innovations. By capturing and utilizing CO2, they are intended to have positive impacts on economy, society, and environment. Next to industries’ own efforts to advance such technologies, CCU is currently funded by governments in several countries, and such funding is likely to increase. Therefore, an assessment of the compatibility of CCU technologies with the SDGs is as much necessary as it is overdue. Hence, this paper elucidates on how CCU might contribute to or hinder the delivery of the SDGs. By comparing CCUs against the SDGs, it can be concluded that, under certain conditions, they might deliver contributions to several SDGs. The main contributions are expected within the context of energy transition processes, and in societal advancements that are linked to technological progress. For eight out of the seventeen SDGs, positive and indirect negative effects can be predicted. Therefore, the development and implementation of CCU aligned with the SDGs poses a challenge for policy makers when designing frameworks and funding schemes. Specific risks need to be monitored and considered in policy making. This paper therefore argues that the SDGs should be used as a framework for assessing potential societal effects of CCU technologies. The findings demonstrate that such an approach is necessary in order to identify and enhance the positive (and avoid indirect negative) effects that CCU technologies might have on people, prosperity and planet.
Introduction
The implementation of the Sustainable Development Goals (SDGs, see Figure 1), developed by the United Nations and adopted by all member states in 2015, is indispensable for building a sustainable and just future for all humans and our planet. The SDGs operationalize the United Nations 2030 Agenda for Sustainable Development and thus serve as tools to foster a global sustainable development with regard to people (society), planet (ecology) and prosperity (economy) (United Nations, 2015). The 17 SDGs are each operationalized by several targets. These targets define the goals more precisely and provide guidance for implementing and assessing the necessary steps toward the delivery of the goals. The SDGs provide a common basis for the design and shaping of more sustainable policies. Also, they serve as a reference system for governments and administrations in assessing efforts and actions (e.g., Bundesregierung, 2018; European Union, 2019; United States Government, 2019).
The SDGs are global goals. However, their implementation equally calls for action in regions and nations, undertaken by a variety of actors in government, business, and civil society (e.g., Spitz et al., 2016; Salvia et al., 2019). Thus, in order to achieve the objectives, policy making as well as industry innovation efforts need to be designed to promote the implementation of the SDGs. It is therefore necessary to ensure that the potential societal impacts of technology innovations seeking public support and funding in research, development, and market implementation – including carbon capture and utilization (CCU) applications – are aligned with the objectives of the SDGs. Such CCU technologies aim to utilize carbon dioxide as a resource, and at the same time deliver positive impacts for society and environment (BMBF, 2019).
In recent times, particularly among European policy makers, CCU has become widely discussed as a concept for industrial transformation toward sustainability (Group of Chief Scientific Advisors [SAM], 2018; Schlögl et al., 2018) and is also mentioned in current IPCC reports as a means to reduce carbon dioxide emissions (IPCC, 2018). The term carbon capture and utilization refers to technologies that utilize carbon dioxide as a source of carbon, thus usually replacing carbon from fossil resources and thereby saving on CO2 emissions (North and Styring, 2019), or providing other environmental benefits which vary depending on the specific technology. For example, applied CCU technologies in the field of mineralization1 may prove environmentally beneficial by replacing either non-renewable resources like limestone or other components with high production related carbon emissions (Favier et al., 2018). In all CCU applications, the utilized carbon dioxide would otherwise have been emitted but is instead obtained by on-site capture technologies for point sources or as a future option, via air capture technologies.
While the first research into making CO2 accessible as carbon source dates back to the 1970s (Aresta, 2010), the development of these technologies has gained momentum in recent years, primarily in light of the accelerating effects of climate change. The growing research activity in the field has led to several technical breakthroughs, and today the first products “made with CO2” have already entered the market (e.g., (Carbon8, 2019; Covestro, 2019). Similarly, to conventional uses of carbon from fossil resources, the options for utilizing CO2 span a wide range of production processes, products, and their respective chemical routes (for an overview, see for example, CarbonNext, 2018; European Commission, 2019a). These include fuels, chemicals, building materials from minerals, building materials from waste, and CO2 utilization paths to enhance the yields of biological processes (IEA, 2019).
While many CCU applications can be considered technically feasible, the main barriers to their industrial implementation and upscaling are higher costs compared with conventional production paths; high demand for energy from renewable resources for most but not all applications; and in some cases the need for hydrogen from renewable sources (Group of Chief Scientific Advisors [SAM], 2018); or, for some mineralization processes, the lack of or varying quality of the required feedstocks (Kremer et al., 2019). These barriers underline that the implementation of CCU technologies in conjunction with the SDGs requires political support, e.g., in the form of continued funding for R&D and support for market implementation on one hand, but on the other hand also require the most rigorous possible assessment of their interdependence with energy transformation policies and other sustainable societal developments, as defined in the SDGs.
Research into CCU technologies is currently funded by governments in several countries, for example Canada, the European Union, South Korea, and the United States [for an overview, see Olfe-Kräutlein et al. (2016)]. CCU technologies are presumed to play a role in future climate mitigation efforts (IPCC, 2018) and also hold promise for creating added value for industry and society, for example by providing an additional carbon source and contributing to a circular economy. Consequently, increased funding for CCU technologies is likely, for example via the European Union’s future ETS Innovation Fund (European Commission, 2019b) and in the context of the European Green Deal (European Commission, 2019c). In order to align the resulting national or regional funding schemes with policy targets such as the SDGs, it is necessary to describe and to determine CCU technologies’ potential impacts on the implementation of a broad range of sustainability aspirations. However, despite the hopes and expectations placed on CCU technologies, to date, their potential contributions to global climate goals and other areas of sustainable societal development remain unclear and will probably remain so in the near future. This is because, on the one hand, many CCU technologies are still in an early development phase (e.g., Group of Chief Scientific Advisors [SAM], 2018), making it difficult to quantify their potential effects, for example on targets such as job creation (target 8.5)2. On the other hand, CCU technologies involve diverse ranges of industries (e.g., ranging from production of cement to fuels) and CO2-based products (e.g., from building materials to kerosene). Subsequently, the technologies differ not only in technical, economic and maturity aspects, but also in their potential effects on societal and political developments. Their disparity hinders conclusive evaluation of the overall CCU effects and the development of common data for all CCU technologies (European Commission, 2019a). Nevertheless, the issue of environmental impacts is frequently addressed by scientific publications on specific effects of single applications, usually in Life Cycle Assessments (e.g., von der Assen and Bardow, 2014; Aldaco et al., 2019). Other economic and societal effects are occasionally outlined in policy oriented reports or studies (Piria et al., 2016; Zimmermann et al., 2017; Group of Chief Scientific Advisors [SAM], 2018; European Commission, 2019a). First publications have also drawn connections between SDGs and CCU technologies. Carus et al. (2019) discuss the sustainability aspects of CCU technologies, which they state directly address nine SDGs. However, the authors do not provide explanatory evidence for their assumptions, or consider negative impacts or limiting factors to the positive effects. Beuttler et al. (2019) highlight the benefits of direct air capture3 technologies for several SDGs. Thus, the literature examines individual positive aspects of some CCU applications on the SDGs, whereas overall economic or societal effects are subjects of policy analyses. However, systematic assessment of the compatibility of CCU technologies with the SDGs, elaborating on both potential positive and negative impacts, is as much necessary as it is overdue and will be undertaken in this paper for the first time.
Thus, while there is evidently a need to elaborate on CCU and the SDGs for target audiences involved in policy- and decision-making, such a debate might also prove useful for practitioners in the field: To date, there is no framework for policy-making or industrial actors in the field, providing guidance on how to embed the development of CCU technologies into a broader sustainability context beyond only environmental aspects, and allowing such issues to be integrated into their work considerations. Hence, this paper elucidates on two questions:
(1) Can CCU technologies contribute to delivering the SDGs?
(2) Can the SDGs provide a framework for the further development of CCU technologies?
To provide answers to these questions, the following section of this paper employs the best available knowledge to assess the potential contributions of CCU technologies to delivering the 17 SDGs and their targets. This assessment remains qualitative, since, as mentioned above, several factors currently limit the opportunity for quantitative comparison. Despite these limitations, this assessment provides policy-related recommendations based on the currently available data and will draw careful conclusions wherever possible, as a first move toward an overdue discussion of the interlinkages between CCU and the SDGs, highlighting gaps and needs for future research on these issues.
Assessing CCU Technologies Against the SDGs
Methodological Approach
To develop an approach for assessing CCU technologies against the SDGs, the following sub-section firstly discusses available tools and methods for aligning individual actions with the aims of the SDGs and examines two exemplary online tools. These observations inform the approach adopted in this study.
The SDGs are carefully worded and, at first sight, their formulations are striking and clear. Nevertheless, only their respective targets and the related indicators provide guidance on how to interpret the goals’ underlying aims and objectives in line with the original intentions. Many indicators appear to be aimed at governments or institutions as monitoring tools, but might not be applicable to industry or other societal actors. Therefore, the indicators may not always be helpful for individual industrial actors seeking to align their business activities with the SDGs. However, some online tools may enable the effects of technological applications to be assessed in relation to the SDGs.
For example, an easily accessible SDG Impact Assessment Tool was developed by the Gothenburg Centre for Sustainable Development, at Chalmers University of Technology and University of Gothenburg, in collaboration with SDSN Northern Europe and Mistra Carbon Exit (Gothenburg Centre for Sustainable Development, n.d.). The website offers free use of a product defined as “a learning tool that visualizes the results from a self-assessment of how an activity, organization or innovation affects the SDGs.” It aims to stimulate users to improve their understanding of the complexity of sustainable development and the different aspects of the SDGs. As such, the tool guides the user along the SDGs and their operationalization, and mainly provides a good understanding of what the goals mean, a clear classification of impacts, and visualization of the results. The impact categories “direct positive” and “direct negative” define the immediate impacts of implementing the assessed technology. The categories “indirect positive” and “indirect negative” describe secondary impacts that might arise as consequences of the implementation. The category “more knowledge needed” is the recommended choice in cases where there is too little knowledge or high uncertainty. While the indicators, as developed by the UN, provide benchmark values for most SDG targets and thus provide tangible values as orientation for governments and related institutions, the difficulty in the application of this tool for other actors is that it cannot provide benchmark values or define targets more precisely. It remains entirely up to the user to estimate and state whether the described activity has an effect on the SDGs, to decide what can be considered an effect, and no quantification is required. No guidance or advice are available for assessing technologies in early developmental states or for making strategic decisions.
A second example: The United Nations Development Programme (UNDP) provides an online tool for SDG assessment of past and future projects or programs (United Nations Development Programme [UNDP], n.d.). While here, the assessment also explicitly aims to assess future activities, it requires users to quantify anticipated savings in CO2 emissions, as well as the applied assessment tool that provided this information. While this is a methodically sound procedure that calls on the required data and data obtaining transparency, the difficulty here is its inappropriateness for assessing types of technology, like CCU, or planned activities where such data cannot yet be provided.
Furthermore, other examples such as the “Chemie3” sustainability initiative of the German chemical industry also provide guidance on how actors might align their own actions with the SGDs (Chemie3, 2019). Here, the approach is to provide hands-on advice for specific industry sectors in their aspirations to become more sustainable.
A common feature of all these tools is that they do not offer assistance in aligning strategic decisions with the SDGs as required for CCU technologies. The tools either provide solely an overview and systematic understanding of the SDGs as such, or the assessment adopts a backward or cumulative perspective, when tangible effects can be quantified with the aim of reshaping certain actions for the future. These approaches require a body of knowledge, with accompanying data and experience, which are not yet available for CCU technologies. In this case, it is only possible to rely on estimates and assumptions that can be found in the relevant scientific literature or policy reports.
To the best knowledge of the author, none of the available tools specifically assess how the SDGs may be impacted by technologies that are currently in early developmental stages, or by related strategic options. This finding is supported by Grainger-Brown and Malekpour, who recently reviewed strategic tools and frameworks available for organizations for the implementation of the SDGs, and state that no tools were identified which substantially engaged with actual strategy development, the stage which can shape transformative change (Grainger-Brown and Malekpour, 2019)4.
Consequently, the present study systematically examines all SDGs and their possible links and relationships with expected economic, ecological, and societal effects of CCU technologies, to the extent that the current literature allows. The resulting assumptions are categorized according to the system recommended by the SDSN Northern Europe and utilized in the online SDG Assessment Tool described above, with one result being a visualization of this assessment. Thus, the following section relates each SDG and relevant targets with possible effects of CCU technologies, and derives specific recommendations when applicable. For an overview of all SDGs and their respective targets, please refer to the Supplementary Material or to the website of the United Nations.
Assessment of CCU Technologies Against the SDGs
SDG 1: End Poverty in All Its Forms Everywhere
Sustainable development goal 1 seeks to eradicate extreme poverty for all people everywhere, currently measured as people living on less than $1.25 USD per day. It is operationalized by eight targets, each with one to three assessment indicators that offer guidance on how exactly to understand the targets of SDG 1. The actions required to implement these targets are mostly located at the state or national level (e.g., target 1.3 “Implement nationally appropriate social protection systems and measures for all, and by 2030 achieve substantial coverage of the poor and the vulnerable”). Thus, any form of CCU deployment is presently unfavorable in developing countries, as the current state of these technologies still involves high costs for development and implementation. Nevertheless, CCU technologies might still contribute to SDG 1, regarding “…access to appropriate new technology…” (target 1.4) and “…implement programmes and policies to end poverty in all its dimensions” (target 1.A). This is possible with respect to the development of innovative, decentralized energy solutions (for details and references, see SDG 7), conditional upon intensive efforts toward technology transfer and external financing, and can be classified as an indirect positive effect.
Despite such a potential positive contribution to SDG 1, CCU technologies might also hinder attempts to end global poverty, since by further advancing and broadening the spectrum of available technologies in the developed countries, the implementation of CCU will enhance their competitive advantage and thus consolidate the global status quo, at least in the short term (Dollar, 1993). This can be classified as an indirect negative effect.
In the context of funding and policy making with the aim of aligning CCU technologies with the SDGs, it is therefore recommended to explore and support opportunities for international cooperation with regard to fostering appropriate technology transfer and designing policy measures specifically to enable cooperation with developing countries that have high potential for solar or geothermal energy production.
SDG 2: End Hunger, Achieve Food Security and Improved Nutrition and Promote Sustainable Agriculture
Sustainable development goal 2 aims to end hunger, achieve food security and improved nutrition, and promote sustainable agriculture. Despite possible contributions to production processes of comestible goods, CCU products in the chemical industry or the construction sector are often not directly involved in food production and the related issues as defined and addressed by SDG 2 and its eight targets, most importantly the fight against malnutrition (see targets 2.1 “By 2030, end hunger and ensure access by all people, in particular the poor and people in vulnerable situations, including infants, to safe, nutritious and sufficient food all year round,” and 2.2: “By 2030, end all forms of malnutrition, including achieving, by 2025, the internationally agreed targets on stunting and wasting in children under 5 years of age, and address the nutritional needs of adolescent girls, pregnant and lactating women and older persons”). Nevertheless, some applications are directly relevant for food production and have the potential to further enhance this influence in the future: The use of CO2 to enhance the yield of biological processes, either used as a gas in greenhouses or processed in fertilizers, can be considered as a possible contribution to SDG 2 (Mac Dowell et al., 2017). This might be significant for some agricultural sectors (e.g., industrial greenhouses), as utilizing CO2 may increase yields by up to 30 percent (Becker and Kläring, 2016)5 with direct implications for marketable volumes and supply. Nevertheless, this option can be limited by the availability of very pure CO2, utilizing infrastructure and heat sources in the proximity of greenhouses, where these exist (IEA, 2019). Thus, the utilization of CO2 as a yield booster requires circumstances that are likely to be unavailable in developing countries without financial support. Nevertheless, increased production of vegetables, for example, is possible and needs to be considered as a contribution to the provision of food for humans. Thus, where possible, for example in the vicinity of ports, the installation of such facilities should be supported with the aim of locally providing food or a source of income by supporting the export of vegetables. This effect can be considered an indirect positive contribution.
A second aspect for future consideration may be the development of novel food products by utilizing CO2 (Carus et al., 2019), as undertaken by the Norwegian company CO2Bio (CO2BIO, n.d.), which produces Omega 3 for fish food, or the Finnish company Solar Foods (Solar Foods, n.d), which utilizes CO2 in developing novel proteins for human nutrition. The significance of this application for SDG 2 is unclear. Furthermore, the capacities for scaling up and expected costs are currently unknown. This effect can be categorized as “More knowledge needed.”
With regard to SDG 2, one potentially confounding factor is that some CCU technologies might require the use of biological crops (Hepburn et al., 2019), and therefore agricultural land, which might lead to land use conflicts if adopted at large scale. This effect can also be categorized as “more knowledge needed.”
For funding and policy making, it is recommended that policies should be designed to fairly regulate potential land use conflicts and, where possible, to facilitate utilization of CO2 as yield booster with financial support.
SDG 3: Ensure Healthy Lives and Promote Well-Being for All at All Ages
Carbon capture and utilization technologies do not have any direct relations to the major targets associated with SDG 3, such as lowering maternity mortality rates or combatting epidemic diseases (targets 3.1: “By 2030, reduce the global maternal mortality ratio to less than 70 per 100,000 live births,” and 3.3: “By 2030, end the epidemics of AIDS, tuberculosis, malaria and neglected tropical diseases and combat hepatitis, water-borne diseases and other communicable diseases”). Also, based on current knowledge, the utilization of CO2 does not usually involve any direct health implications that occur either in production processes with CO2 or related to any CCU products.
Nevertheless, an exception may be mineralization processes that use waste materials, for example steel slags or municipal waste. Here, specific attention needs to be given to possible hazardous constituents such as heavy metals (Sanna et al., 2014; IEA, 2019). Also, CO2 separation technologies using amine scrubbing, as may be deployed for CCU or CCS (carbon capture and storage), may lead to health risks if applied at very large scales (Dautzenberg and Bruhn, 2013). Furthermore, depending on the location of potential CCU facilities, the transportation of CO2, especially in pipelines, might impose dangers to residents (Barrie et al., 2005; Koornneef et al., 2009). These can be categorized as indirect negative effects. An indirect positive effect on SDG 3 may be the use of CO2 in the production of pharmaceuticals (Zimmermann et al., 2017), thus potentially increasing the availability of more sustainable (although not necessarily cheaper) healthcare products.
Thus, with regard to research funding, it is recommended to further explore possible health risks related to amine scrubbing technologies. With regard to products made with reused CO2, attention must be given to their compliance with relevant regulations and directives, if these novel products are not chemically identical to conventional products6. This applies, for example, to pharmaceuticals and products resulting from mineralization technologies.
SDG 4: Ensure Inclusive and Equitable Quality Education and Promote Lifelong Learning Opportunities for All
The implementation of SDG 4 also calls for actors, primarily nations and regions and their institutions, to improve the education situation in their area of accountability, as defined in targets such as ensuring free, equitable and quality primary and secondary education for boys and girls (target 4.1: “By 2030, ensure that all girls and boys complete free, equitable and quality primary and secondary education leading to relevant and Goal-4 effective learning outcomes”), or equal access for men and women to education, including university (target 4.3: “By 2030, ensure equal access for all women and men to affordable and quality technical, vocational and tertiary education, including university”).
While the development and implementation of CCU technologies requires specialist expertise in several academic disciplines and different industries, their development itself can only indirectly contribute to providing quality education by involving students and trainees in learning processes related to CCU. Additionally, by means of technology transfer, knowledge can be provided to nations that lack the funds or facilities to develop their own knowledge in the field (capacity building). This can be considered as an indirect positive effect.
Thus, policy makers and funding schemes should encourage industry actors in CCU to foster and provide learning options for students and professionals in order to develop and spread knowledge about CCU technologies.
SDG 5: Achieve Gender Equality and Empower All Women and Girls
Carbon capture and utilization technologies are not foreseen to have any positive or negative influence on gender equality and the empowerment of girls and women, other than very indirectly and not specific to CCU, such as by possibly fostering growth and development, which might then entail increased options and opportunities for girls and women. Consequently, CCU technologies have no implications for the delivery of SDG 5.
SDG 6: Ensure Availability and Sustainable Management of Water and Sanitation for All
Sustainable development goal 6 and its six targets mainly focus on the provision of clean water and sanitation (targets 6.1: “By 2030, achieve universal and equitable access to safe and affordable drinking water for all,” and 6.2: “By 2030, achieve access to adequate and equitable sanitation and hygiene for all and end open defecation, paying special attention to the needs of women and girls and those in vulnerable situations”) and the management of water as a resource for the sake of all (targets 6.4: “By 2030, substantially increase water-use efficiency across all sectors and ensure sustainable withdrawals and supply of freshwater to address water scarcity and substantially reduce the number of people suffering from water scarcity,” 6.5: “By 2030, implement integrated water resources management at all levels, including through transboundary cooperation as appropriate,” and 6.6: “By 2020, protect and restore water-related ecosystems, including mountains, forests, wetlands, rivers, aquifers and lakes”).
The application of CCU technologies has no known direct negative influence on water quality in areas where it is deployed (target 6.3). In contrast, indirect positive influences might occur due to the possible replacement of fossil resources with CCU technologies. For example, the extraction of natural gas or of fossil resources from oil sands puts a huge strain on water quality (Schindler, 2013; Vidic et al., 2013). A reduced need for fossil resources thus reduces the environmental burden of extracting them and potentially improves water quality.
The utilization of hydroelectric energy for CCU technologies might cause another indirect effect of these technologies on water related environmental issues. Since the ecological feasibility of CCU technologies depends on the availability of energy from renewable sources such as hydropower, a higher demand for such energy might intensify its negative effects on the affected ecosystems (Balzannikov and Vyshkin, 2011; Anderer et al., 2012).
If CCU facilities are located in areas where water is scarce, water consumption and withdrawal may become an issue and imply indirect negative effects. This is because the sustainable deployment of CCU technologies implies using energy from renewable sources, including solar. However, those regions with high solar energy potential may also experience water scarcity, which might be exacerbated by the introduction of CCU, as with other industrial facilities. Regionalized LCA is a suitable method for analyzing the water footprint in greater detail, as it takes local water conditions into account (European Commission, 2019a).
Some air capture installations, for example as developed by the Swiss company Climeworks (Climeworks, n.d.), produce water during the capture process and claim therefore to provide rather than consume water, and thus might be unlikely to strain global water supply even when implemented at large scale. Conversely, it is claimed that positive effects on SDG 6 can be achieved by providing fresh water and (after necessary purification) even drinking water (Beuttler et al., 2019). Once such technologies are implemented, this can be considered a positive direct effect.
In order to best align CCU technologies with the SDGs, it is recommended to apply regionalized LCA in order to account for actual local water conditions in location decisions. CCU applications with air capture should be considered for areas with high renewable energy potential.
SDG 7: Ensure Access to Affordable, Reliable, Sustainable, and Modern Energy for All
Carbon capture and utilization technologies, more precisely applications that produce fuels and chemicals, can contribute to some targets defined under SDG 7, which aims to “ensure access to affordable, reliable, sustainable and modern energy for all.” Regarding the goal of providing affordable, sustainable, and clean energy for all, this contribution is likely to be in the field of so-called Power-to-X (P2X) technologies (Sternberg and Bardow, 2015). In a sustainability context, P2X denominates technologies that provide storage or utilization options for excess electricity that will possibly be available due to the fluctuating energy production from solar, wind, or water. Examples include e-fuels, e-methanol, and e-methane, which involve CO2 in their production (Heinemann et al., 2019). Thus, the utilization of CO2 from air capture, or that would otherwise have been emitted, can contribute to solving the issue of energy storage and thereby support energy transition processes by enhancing the reliability of energy carriers from fluctuating renewable sources. It may simultaneously provide products for direct use (Beuttler et al., 2019; Carus et al., 2019). Furthermore, decentralized energy production pathways could be realized by applying CCU processes (Dittmeyer et al., 2019). The concept of decentralized energy production involving CCU is again of specific interest for countries that have high potential for renewable energies from solar, wind, or water, and may be a future option for providing energy in remote locations. Thus, with regard to SDG 7, direct positive effects can be achieved.
Nevertheless, it needs to be considered in this regard that most technologies that produce fuels and chemicals with CO2 require large amounts of energy. In order not to produce more CO2 than such processes can utilize, the required energy should come from renewable sources). This fact, on the one hand, makes fossil fuels more competitive by providing energy at lower costs, the exception being markets where cheap renewable energy allows commercial production, such as Iceland (Carbon Recycling International, n.d.) or Chile (IEA, 2019). On the other hand, the huge amount of renewable energy that such technologies require might also lead to competition among consumers of renewable energies, and raises the question of how the amount of renewable energy currently available can be used in the most efficient way (European Commission, 2019a). These can be considered as indirect negative effects.
While the continued use of coal for energy production is undesirable and not in line with global climate goals, in a transition period, the concept of “clean coal” might be adopted until coal-based energy production is completely phased out. Clean coal concepts are proposed in combination with CCS (e.g., Falcke et al., 2011), but might also involve CCU processes. In order to avoid unwanted path-dependencies and lock-in effects that might hinder the implementation of SDG 7, policy measures and funding must exclude all CCU applications that foster the continued use of new fossil resources or fossil-based technologies in energy production in combination with carbon capture, and should not foster measures in the field of enhanced oil and gas recovery (EOR and EGR) that use CO2 with the sole purpose of obtaining even larger amounts of oil or gas. Such indirect negative effects may occur as lock-in effects and path-dependencies with unwanted energy production.
As a consequence, in order to contribute to SDG 7, the development of energy storage options and decentralized energy solutions should be fostered by policy makers and funding mechanisms, with specific attention to avoiding path dependencies and lock-in effects.
SDG 8: Promote Sustained, Inclusive and Sustainable Economic Growth, Full and Productive Employment and Decent Work for All
While the improvement of working conditions as described in the targets of SDG 8 (e.g., target 8.5: “By 2030, achieve full and productive employment and decent work for all women and men, including for young people and persons with disabilities, and equal pay for work of equal value”) needs to be implemented by employers worldwide, a direct contribution of CCU technologies to the implementation of SDG 8 might be found in their innovative power. The general positive effects of innovation on economic growth and employment have been described many times (e.g., Cameron, 1996; Smolny and Schneeweis, 1996; Kinkel et al., 2004). Therefore, similar benefits may be reasonably anticipated for CCU technologies, although these cannot yet be demonstrated or quantified. Thus, CCU technologies can be considered as future contributors to sustainable economic growth within existing markets and to additional, growth-related employment (European Commission, 2019a).
While for the time being, CCU production processes are not likely to require more human effort than the equivalent conventional processes, some upstream and downstream processes, such as mechanical engineering or pipeline construction, might see increased demand through CCU and thus (European Commission, 2019a; Naims, 2020). Additionally, in less developed regions with high potential for renewable energies, the future application of CCU technologies might also create jobs and decent work, as work in high-technology facilities might be considered as such (Beuttler et al., 2019; Carus et al., 2019). This can be considered a direct positive effect.
For all kinds of CO2 utilization options, a lower material footprint by substituting fossil resources with CO2 (European Commission, 2019) represents a contribution to target 8.4, which aims to lower material footprints and domestic material consumption (“Improve progressively, through 2030, global resource efficiency in consumption and production and endeavor to decouple economic growth from environmental degradation, in accordance with the 10-year framework of programmes on sustainable consumption and production, with developed countries taking the lead”). This is a second direct positive effect. In decision-making processes concerning funding and policy making, especially for more mature CCU applications that are approaching market implementation, possible positive contributions to more sustainable growth, and other positive economic effects need to be taken into consideration (TEA). An advantage, as mentioned above, is also possible for less developed regions.
SDG 9: Build Resilient Infrastructure, Promote Inclusive and Sustainable Industrialization and Foster Innovation
Sustainable development goal 9’s target 9.4 names several factors where CCU technologies provide viable options: “Upgrade infrastructure and retrofit industries to make them sustainable, with increased resource-use efficiency and greater adoption of clean and environmentally sound technologies and industrial processes, with all countries taking action in accordance with their respective capabilities.” Generally, the innovation effect may lead to upgraded infrastructure. Also, the possibility of retrofitting existing industrial facilities with capture technologies aims at this target (Scot Project, 2016; European Commission, 2019a). The envisaged resource-use efficiency, for example with regard to limestone in mineralization processes (Sanna et al., 2014) or with regard to fossil carbon in the production of polyols (Covestro, 2019), is inherent to the aims of CCU technologies as well as the greater adoption of clean and environmentally sound technologies and industrial processes (BMBF, 2015; WWF Deutschland, 2018; European Commission, 2019a; Olfe-Kräutlein et al., 2014, 2019). Thus, once implemented, CCU technologies can be considered as making positive contributions to the implementation of SDG 9. Targets 9.5, 9.A, and 9.B7 once again call for cooperation in capacity-building and technology transfer to developing countries, whenever possible and advisable according to local conditions (see SDG 1). CCU technologies inherently have innovative power, as they offer a variety of new production pathways and the delivery of more sustainable products (see section “Introduction”). Therefore, a contribution to SDG 9 through innovation is likely and can be considered a direct positive impact (Beuttler et al., 2019; Carus et al., 2019).
Analogous to the effects described with regard to SDG 7, special attention needs to be given to avoiding lock-in effects regarding the potential unwanted lifespan expansion of outdated industrial facilities, such as inadvertently perpetuating reliance on coal-fired power plants though the installation of carbon capture technologies. Thus, care must be taken to avoid potential indirect negative effects.
With regard to the rejuvenation of industrial facilities, possible lock-in effects in connection with retrofitting must be considered in policy-making and funding decisions. Again, international cooperation should be fostered in policy making in order to develop advantages for developing countries.
SDG 10: Reduce Inequality Within and Among Countries
The implementation of CCU technologies might have both positive and negative effects on inequalities within or among countries as defined by the targets of SDG 10. The installation of CCU facilities in less developed countries that are rich in solar energy can provide access to CO2 as an abundant carbon source (Carus et al., 2019), and therefore create additional value for such countries. CCU facilities may also provide sources of income and decent work for the local population. This differs from the one-sided advantage for countries with abundant fossil resources, which has shaped global geopolitics in recent decades. Thus, CCU technologies might compensate disadvantages of countries and regions without own access to fossil resources. These factors, in combination, might make an indirect contribution to reducing inequality between nations and regions.
As discussed above with regard to SDG 1, policy makers should keep the possibilities of technology transfer and international cooperation in mind, in order to avoid the indirect negative effect of exacerbating inequalities between developed and developing countries (see SDG 1 and accompanying recommendations). Hence, CCU technologies have potential for indirect positive and negative effects with respect to the aims of SDG 10.
SDG 11: Make Cities and Human Settlements Inclusive, Safe, Resilient and Sustainable
CO2 utilization technologies provide pathways to produce building materials from minerals and waste (e.g., Blue Planet Ltd., 2019; IEA, 2019; Mineral Carbonation and International, 2019). While they do not influence the future design and organization of urbanization processes and urban life in the sense defined by SDG 11 the targets8, the provision of more sustainable building materials is an indirect positive effect on the development of more sustainable future cities. Since cities are largely constructed using concrete and bricks, improved production pathways through CCU can make significant contributions to this field. Therefore, it is recommended that policy makers and governments recognize mineralization technologies as contributing to building more sustainable cities. This could be expressed, for example, by incorporation within government procurement procedures.
Furthermore, the local production of CO2-based fuels and chemicals might be considered a positive contribution toward more sustainable cities (Beuttler et al., 2019; Carus et al., 2019).
SDG 12: Ensure Sustainable Consumption and Production Patterns
With regard to the implementation of SDG 12, CCU technologies can specifically address targets 12.2 “more sustainable management and efficient use of natural resources”9, and 12.6, which aims to encourage companies, especially large and transnational companies, to adopt sustainable practices and integrate sustainability information into their reporting cycles10. CCU technologies do so by offering an overall contribution to more sustainable production processes, by making more efficient use of natural resources, and by contributing to the creation of an (industrial) carbon cycle (e.g., Mennicken et al., 2016). This goal specifically targets large and transnational companies, thus SDG 12 calls for action from the most relevant CCU actors, namely the chemical and fuel industries.
Target 12.5 focuses on waste reduction through measures for prevention, reduction, recycling, and reuse11. Here, CCU technologies contribute through their cyclic character: While it is not yet possible to completely close an industrial carbon cycle, CCU technologies already cover some of the required steps by developing capture technologies that provide CO2 as a feedstock (which would otherwise have been directly emitted), and by re-using that CO2 in different applications. Theoretically, the industrial carbon cycle could be closed by capturing CO2 from waste incineration plants (see Figure 2). This approach of CCU technologies is based on the idea of a circular economy (Naims, 2016; Carus et al., 2019) with the aim of eliminating all forms of waste, thereby making a positive contribution to SDG 12.
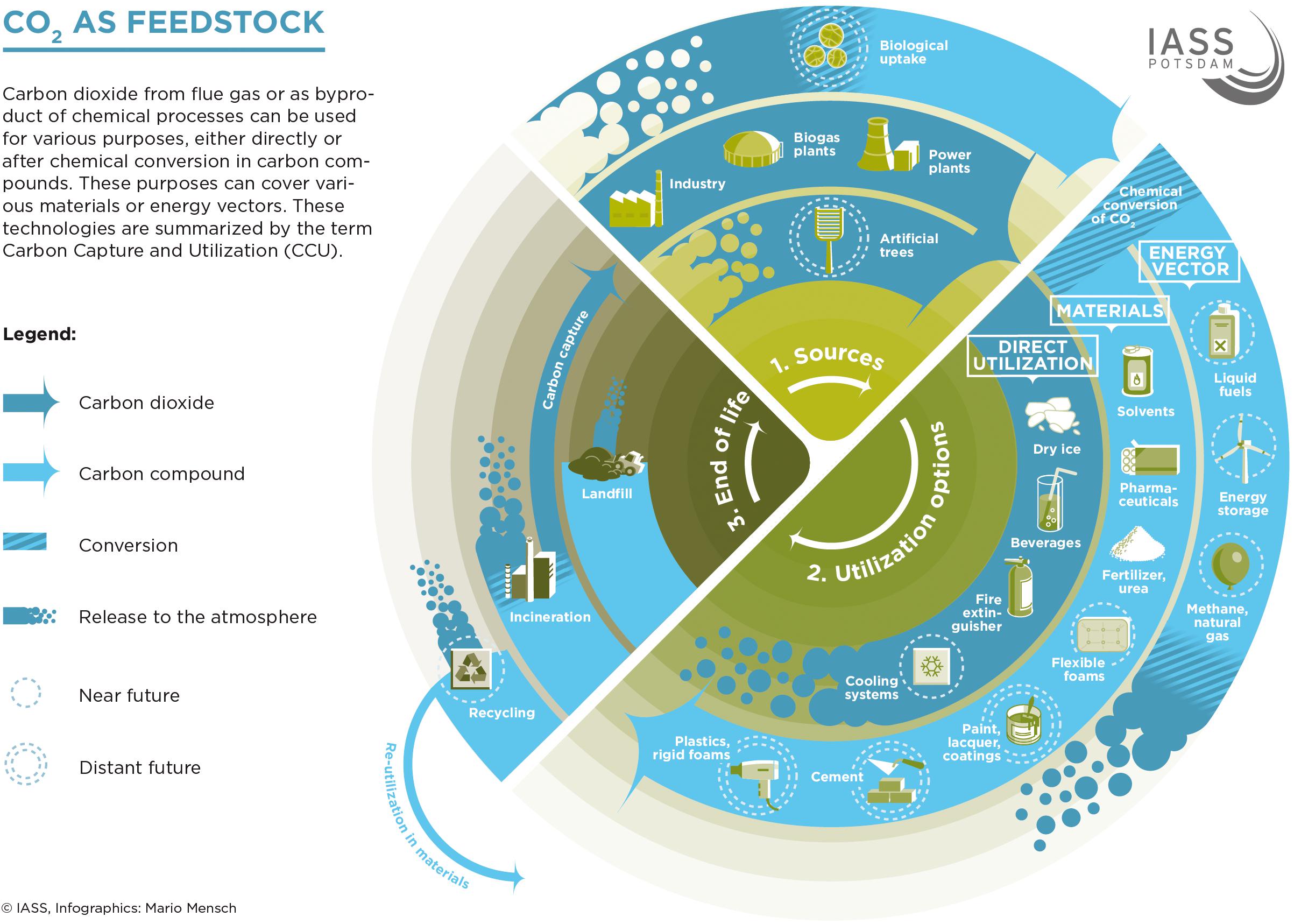
Figure 2. Overview of phases, pathways, and applications of CCU technologies, with focus on cyclic characteristics. Source: IASS/Mario Mensch.
Sustainable public procurement, a target mentioned in SDG 1212, is also important for the market implementation of CCU products. This is because, to date, the costs of such products are likely to be higher than for conventional equivalents (Naims, 2016). For example, cement made with CO2 could be subject to public procurement if it is proven that it has a lower CO2 footprint than conventional cement, and thus could positively contribute to national or regional sustainability goals and to SDG 12.
Nevertheless, possible negative effects must also be taken into consideration. More attractive CCU products or production pathways (e.g., those that are “greener” or lead to lower costs), may result in rebound effects, where higher consumption partially counteracts emission reduction effects (Olfe-Kräutlein et al., 2016; European Commission, 2019a).
Therefore, it is recommended that policy making and public procurement foster CCU technologies alongside other sustainable production patterns, with regard to the risk of rebound effects.
SDG 13: Take Urgent Action to Combat Climate Change and Its Impacts
The main aim of actors in developing and implementing CCU technologies is to reduce CO2 emissions and thereby contribute to action against climate change (for an overview of different CCU actors’ motivations, see Olfe-Kräutlein et al., 2019). However, other effects, such as a decreasing need for fossil resources or other raw material inputs, and in some cases efficiency effects, might make a positive contribution to SDG 13 (e.g., Mennicken et al., 2016; CarbonNext, 2018; Carus et al., 2019). While these aims are the most important motivation for advancing and implementing CCU technologies (Olfe-Kräutlein et al., 2019), it is not yet possible to accurately estimate the significance of this potential positive contribution.
Varying estimates of the CO2 saving from CCU technologies have been published in recent years (e.g., The Royal Society, 2017; European Commission, 2019a; Global CO2 Initiative, 2019; Hepburn et al., 2019). These estimates vary significantly, which seems inevitable, given that: underlying energy scenarios differ; there is no clear definition of what a CCU technology is or is not (for example, inclusion or exclusion of enhanced oil or gas recovery EOR/EGR); there is no common approach to managing differing degrees of maturity; and that the individual production processes differ widely, even for similar products, and thus would each require specific calculations. Not least due to the individual circumstances of each proposed industrial CCU process, a transparent assessment of possible emission reductions and other environmental issues needs to be undertaken to demonstrate environmental benefit for all applications individually, and therewith confirm their possible direct positive contributions. Several publications already provide guidance on specific aspects of assessing the environmental impacts of CCU applications (e.g., von der Assen et al., 2013; Zimmermann et al., 2018). In Europe and the United States, efforts are currently underway to align and develop standards for life cycle and techno-economic assessments of CCU, and have already led to initial publications on this objective (Zimmermann et al., 2018).
Thus, to verify future or existing contributions to SDG 13, it is recommended to assess the possible environmental effects of each CCU process individually by means of appropriate methods such as life cycle assessment. In policy- and funding-related decision-making processes, it must be ensured that the scope and methodology of such assessments produce the required data in a transparent and standardized manner. All public funding for CCU technologies needs to be contingent upon demonstrable positive effects for SDG 13.
SDG 14 and 15: Conserve and Sustainably Use the Oceans, Seas and Marine Resources for Sustainable Development; Protect, Restore and Promote Sustainable Use of Terrestrial Ecosystems, Sustainably Manage Forests, Combat Desertification, and Halt and Reverse Land Degradation and Halt Biodiversity Loss
For SDGs 14 and 15, as defined in their respective targets, the development and implementation of CCU technologies (based on current knowledge) is likely to have no specific direct effects, but rather to evoke impacts similar to industrial facilities in general. This means that, in general, the installation, expansion, and transformation of all industrial facilities may be harmful to terrestrial and aquatic biodiversity, if undertaken without due consideration. These effects can be reduced if the roll-out of industrial facilities is undertaken in line with regional and supranational sustainability goals and takes regard of environmental effects. At present, there are no known, specific environmental risks directly linked to the implementation of CCU technologies.
An indirect positive effect for aquatic and terrestrial life can be assumed via CCU’s possible general environmental contributions toward reducing reliance on fossil resources, facilitating recycling processes and the closing of an industrial carbon cycle (Global CO2 Initiative, 2019).
Another indirect negative effect that all industrial facilities have in common is the possibility of land use conflicts. As with other facilities, these should be avoided when making decisions on locating industrial plants applying CCU. It is currently unknown whether those effects might occur at all, and if so, whether they might be compensated by possible positive effects of CCU in terms of preventing land degradation due to drilling and mining; by reducing the consumption of fossil fuels; or, compared to the use of biomass, by not requiring the use of arable land (Carus et al., 2019).
Consequently, local administrations and policy makers must apply the highest environmental standards to all proposed industrial facilities engaged in CCU processes, in order to avoid negative effects on biodiversity.
SDG 16: Promote Peaceful and Inclusive Societies for Sustainable Development, Provide Access to Justice for All and Build Effective, Accountable and Inclusive Institutions at All Levels
The implementation of CCU technologies is unlikely to have any direct effects on the goals of SDG 16 as defined in its targets13. Nevertheless, indirect positive effects might occur with regard to maintaining and further fostering a peaceful world under certain conditions. While the majority of emissions are caused by developed nations, most of the consequences of global warming are presently borne by developing countries that are more vulnerable to desertification as well as rising sea levels. Therefore, all measures that developed countries undertake in order to avoid and reduce emissions or even to extract CO2 from the atmosphere (negative emission technologies, NET) may be regarded as measures that foster peaceful relations. Under certain conditions, this also includes CCU technologies, amongst others. Technology transfer, as discussed with relation to SDG 1 and 10, can add to this possible indirect effect.
SDG 17: Strengthen the Means of Implementation and Revitalize the Global Partnership for Sustainable Development
The question of whether or not the development and implementation of CCU technologies will contribute to SDG 17 and its targets largely depends on those being active in the field, be it in research, industry, or policy making: Whether CCU technologies will be solely a regional or national technology success, or follow a more global approach and claim to contribute to societal goals also in other parts of the world, is – for policy makers – a matter of designing policies and fostering technology transfer and research accordingly, for example with a focus on decentralized energy or fuel solutions (see SDG 7). For industry, this means strongly considering options for transferring knowledge and technologies, and supporting capacity-building in developing countries that might have future potential for implementing CCU technologies. Enhanced international cooperation in the field of CCU, designed to equally fit the needs of less developed countries, can be part of the additional support that SDG 17 requires from the developed countries to the less developed ones (targets 17.3 and 17.5)14. Tied to many necessary, maybe partly altruistic preconditions, it is currently unknown whether CCU will make a positive contribution to SDG 17 or not.
From a policy perspective, mechanisms for funding the development and implementation of CCU technologies should not only address regional needs, but also contribute to global societal goals, avoiding simply consolidating the competitive advantages of the developed countries without any compensatory activities. Policies should specifically target international cooperation and negotiation to consider worldwide implications. Industry actors should be intensely encouraged to engage in technology transfer and capacity building.
Actionable Recommendations
The assessment of CCU technologies points to considerable leeway in designing their further development and implementation, in addition to the necessary technological advancements. In order to shape CCU pathways in accordance with the SDGs, the recommendations presented in the previous section provide guidance for policy decision processes, and also a basis for action in industry and administrations. The following section summarizes those recommendations according to the United Nations’ grouping of the SDGs as they relate to the three pillars of sustainable development, i.e., society (people), ecology (planet), and economy (prosperity) (e.g., Hansmann et al., 2012; United Nations, 2015).
Recommendations for CCU Contributions to Achieving SDGs Related to People (Society)
The group of SDGs that mainly focuses on people (the “society” pillar of sustainability), comprises SDGs 1 to 5. To shape the development of CCU technologies according to these SDGs and bring their positive potential to the full, the most important aspect for research and industry is to be ready to share knowledge and for policy makers to foster and enable such processes.
• Support opportunities for international cooperation, especially with developing countries that have high potential for solar, wind, or geothermal energy production.
• Design policy measures that foster and enable technology transfer.
• Provide learning options for students and professionals, including from developing countries, in order to develop and spread knowledge about the possibilities of integrating CCU technologies in future energy systems.
In order to avoid direct or indirect negative effects on the delivery of SDGs 1 to 5, attention needs to be given to possible conflicts in land use as well as to health aspects in the production and use phase of products made with CO2.
• Design policies with regard to the need to avoid conflicts in land use.
• Further explore health risks related to amine scrubbing technologies.
• With regard to products made with CO2, attention must be given to their compliance with respective product regulations and directives.
The deployment of CCU technologies has least potential for meeting the SDG targets relating to society/people, compared with the anticipated contributions to the pillars of ecology and economy. Given the consideration of the recommendations above, they may positively contribute only in an indirect way. Nevertheless, at the same time, the deployment of CCU technologies has little or no potential to hinder the implementation of SDGs 1 to 5.
Recommendations for CCU Contributions to Achieving SDGs Related to Prosperity (Economy)
The group of SDGs that mainly focuses on prosperity (the “economy” pillar of sustainability) comprises SDGs 7 to 11. Among these, the alignment of CCU development with SDG 7 is of fundamental importance, as it involves the complex relationship between CO2 utilization and different aspects of energy production and consumption. Here, it is crucial to adopt a systems perspective and to consider possible effects beyond those of any given application in isolation. CCU technologies have major promise for future energy systems, but their implementation can also hinder the advancement of sustainable energy systems. Therefore, respective policies and funding schemes need to consider and address possible opposite effects.
• In order to contribute to SDG 7, priority should be given to the development and deployment of energy storage options and decentralized energy solutions.
• Specific attention must be given to avoiding path dependencies involving unsustainable energy production and lock-in effects.
Also with regard to SDG 9, positive and negative direct effects of CCU technologies on industrial innovation and infrastructure are possible and should be addressed accordingly.
• With regard to the rejuvenation of industrial facilities, possible lock-in effects in connection with retrofitting must be considered.
Again, as described with regard to “people”-related SDGs in the preceding section, international cooperation should be fostered in policy making in order to support developing countries and ameliorate their disadvantages. This is necessary to align CCU development with SDGs 9 and 10 (reduce inequalities).
Among this group of SDGs, another effect of CCUs that calls for policy action involves support for more sustainable building materials as a contribution to SDG 11, which addresses sustainable cities and infrastructures.
• Mineralization technologies should be recognized by policy making as a contribution to building more sustainable cities. This could be expressed, for example, by incorporation in government procurement procedures.
Sustainable development goals 7 to 11 have considerable significance for the sustainable implementation of CCU technologies. Besides the financial feasibility of industrial choices, actors within the CCU industry will also have to give serious consideration to the systemic consequences that such choices may entail concerning possible lock-in effects and path dependencies. Here, specific political attention and action is required in order to foster the potential of CCU technologies and to avoid any negative effects.
Recommendations for CCU Contributions to Achieving SDGs Related to Planet (Ecology)
Sustainable development goals 6 and 12 to 15 address the “planet” (the “ecology” pillar of sustainability). It is essential for CCU technologies to comply with these SDGs, since their main aim is to contribute to a more sustainable and environmentally sensitive economic system. Therefore, the main action required by decision makers in governance, industry, and research is to continue to develop and apply transparent assessment standards, specifically tailored to the needs of CCU technologies, in order to ensure that they contribute to the SDGs.
• To verify future or existing contributions to SDG 13, the possible environmental effects of each CCU process must be assessed individually through appropriate methods such as life cycle assessment.
• Policy- and funding-related decision-making processes must ensure that the scope and methodology applied to LCAs produce the required data in a transparent manner.
• Regionalized LCA needs to be applied in order to take into account actual local resource conditions for location decisions.
Attention must also be given to avoiding direct or indirect negative effects, also specifically on the achievement of SDGs 12, 14, and 15.
• Policy making should foster CCU alongside other sustainable production patterns, while considering the potential negative implications of rebound effects.
• For all industrial facilities that engage in CCU processes, the highest environmental standards must be applied in order to avoid negative effects aquatic and terrestrial life.
The planet-related SDGs pose a substantial assessment framework for the basic prerequisites for CCU technologies. This is recognized by actors in the field (Beuttler et al., 2019; Carus et al., 2019). Nevertheless, there is a need for greater progress on such assessment frameworks, particularly if seeking public funding (which is anticipated to increase) for the development of CCU technologies.
Discussion
Can CCU Technologies Contribute to Delivering the SDGs by 2030?
Based on this qualitative assessment of CCU technologies in relation to the SGDs, no evident incompatibility has been identified, insofar as: From the present perspective, no direct negative effects are described in the most recent literature. However, the assessment reveals that the implementation of CCU technologies may involve many indirect effects, both positive and negative (see Figure 3). These broader effects may lie beyond the direct responsibility of the developing entity (whether academic or industrial actors), or might remain unnoticed if investigations such as interactions with the SDGs are not undertaken. Therefore, it is the role of policy makers and respective funding agencies to act accordingly: Future policies and funding schemes need to ensure that the further development and deployment of CCU technologies are aligned with the societal, environmental, and economic targets defined by the SDGs. The SDGs provide a valuable tool to assess individual technology applications so that possible indirect negative effects can be avoided or at least minimized by anticipatory policy making, and possible direct and indirect positive effects can be fostered and enhanced.
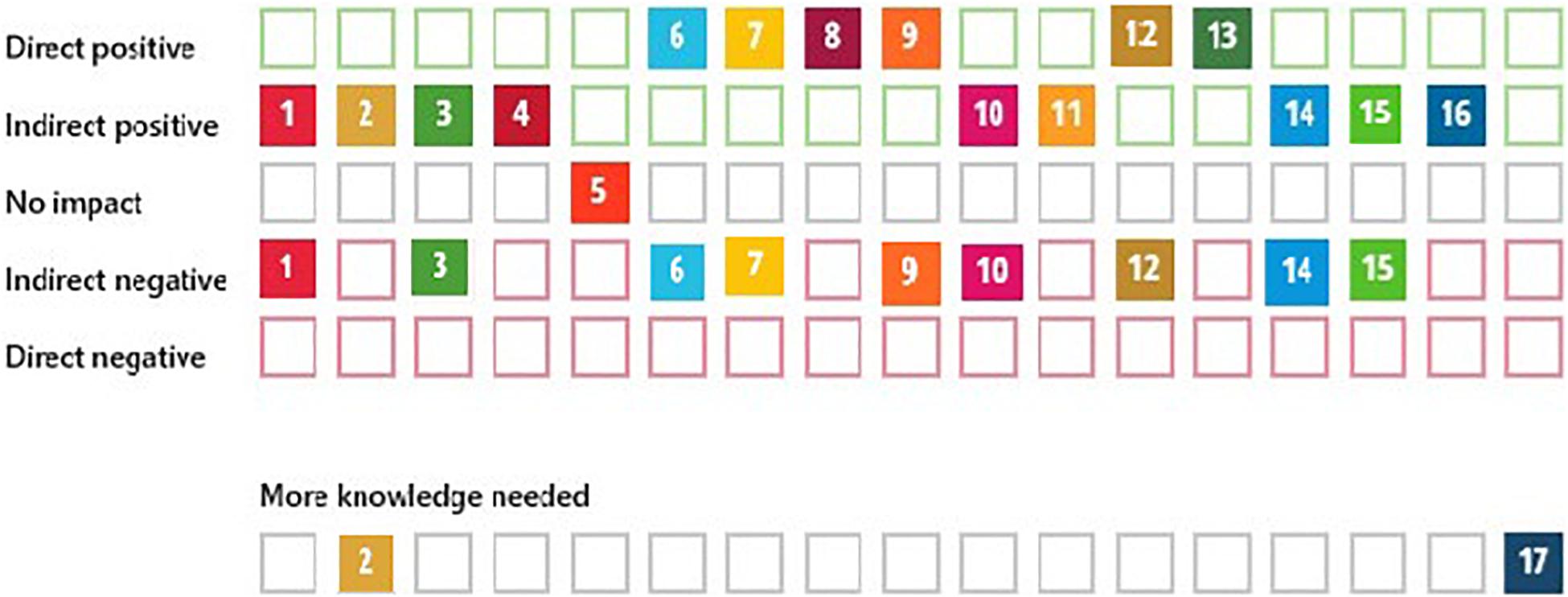
Figure 3. Overview of CCU/SDG assessment. Source: Author, based on graphical representation of the SDG impact assessment tool.
This study articulates the areas and conditions in which opportunities for positive contributions to the SDGs are possible, and which interrelations should be avoided. The main contributions are to be expected in the context of energy transition processes and in societal advancements, as defined by the scope of the SDGs that are linked to technological progress. It has been shown that it is necessary to assess the consequences of CCU not only through Life Cycle Assessments (LCA) or Techno-Economic Analyses (TEA), as – in the best case – they are currently undertaken. In practice, a broader assessment approach, as provided by the SDGs, is vital for identifying and subsequently addressing other important effects that CCU technologies might have on people, prosperity, and planet, such as interrelations with energy or industry policies and targets. In future implementation processes, the specific risks need to be constantly monitored and considered in policy making.
Can the SDGs Provide a Framework for the Further Development of CCU Technologies?
The alignment of CCUs to the SDGs is essential in order to guarantee continued support in policy making and through public funding. It has been shown that possible positive and negative effects can be identified by systematically assessing such technologies against the SDGs. The SDGs thus provide an appropriate tool through which one’s own doing can be evaluated. The effective alignment of CCU technologies with the SDGs depends on the actions taken by relevant actors: Either CCU will be solely an interesting technological innovation with little relevance to global climate goals and a peaceful, more sustainable world; Or, additionally to its single purposes in selected industry branches, CCU will unfold its potential to serve as a global tool to reduce emissions and, at the same time, provide new options for local energy supply systems and a means to reduce the strain on finite natural resources. The challenge for policy making is to establish frameworks and shape policies and funding accordingly. In order to proving worthy of the invested public resources, all actors should pay close attention to ensuring that the steering, development, and implementation of CCU comply with the SDGs.
For this purpose, the SDGs need to be used as a global framework for assessing the societal impacts of CCU technologies. As shown in this analysis, even this rather general overall assessment of CCU technologies reveals clear messages on which aspects must be considered, to ensure alignment with the SDGs. To facilitate effective transfer of these findings into practice, supportive tools on how to assess specific technology applications in relation to the SDGs are available from various providers in different branches of industry (e.g., for a systematic assessment if no retrospective data are available: Gothenburg Centre for Sustainable Development, n.d.; if data on a specific application is already available: United Nations Development Programme [UNDP], n.d.; specific to the chemical industry: Chemie3, 2019). If the state of development of a technological application does not yet enable conclusions on specific SDG-related aspects, knowledge gaps might be temporarily bridged with the results of more general discussions on the issue, as made available in this article, which thus provide a basis for preliminary conclusions.
Also, for the CCU community, alongside the relevant IPCC reports, the SDGs provide an additional system of reference when framing and positioning CCU as a family of technologies that fosters sustainable development. This might prove helpful in communication processes involving all kinds of stakeholders, and it further enhances the meaningfulness and necessity of such an assessment. The implementation of CCU technologies as a means toward the sustainable transformation of the planet places the emphasis for action where it belongs: on industry, particularly those that account for large proportions of global emissions, such as the chemical industry or cement production. Their commitment to the SDGs is essential to meet global goals and implement the 2030 Agenda for Sustainable Development. Accordingly, incorporating these aims when designing policies and funding measures for CCU might provide an additional motivation to take on this responsibility and, consequently, contribute to a more just and peaceful world.
The present assessment is limited by the specific attributes of CCU technologies, foremost the broad range of technological applications that are subsumed as CCU, and their mostly low level of maturity. Both render the quantification of possible overall effects as challenging to unfeasible at present. This limitation is reinforced by CCU’s possible contribution to climate targets being strongly dependency on the availability of abundant renewable energy. Therefore, the reasonable implementation of CCU, and alongside this its alignment with the SDGs, strongly depends on the delivery of energy targets, as defined for example by the European Commission (2018) or the German Federal Government (Bundesministerium für Umwelt Naturschutz, Bau und Reaktorsicherheit, 2016). Despite these limitations, it is advisable for actors involved with CCU to continuously dedicate efforts and future research toward quantifying individual CCU effects with respect to each SDG.
To conclude, some SDGs are likely to be supported by the implementation of CCU, whereas others will require specific policy support in order to realize their full potential or to avoid unwanted negative effects. To align the further development and future implementation of CCU technologies with the SDGs, policy and decision makers at regional, national, and supranational levels must devote special attention to several factors, foremost to avoiding possible indirect negative effects.
The assessment shows that the SDGs can and should serve as an assessment framework for the CCU community – spanning industry, research, and policy – in making CCU technologies what they claim to be: A technological innovation that facilitates progress toward delivering a better planet for all. It now requires all actors in the field to live up to this challenge, by taking action to ensure that the further development and future implementation of CCU technologies are “SDG-proof.”
Author Contributions
The author confirms being the sole contributor of this work and has approved it for publication.
Funding
This study has been undertaken as part of the project “CO2sistent”, funded by the Global CO2 Initiative.
Conflict of Interest
The authors declare that the research was conducted in the absence of any commercial or financial relationships that could be construed as a potential conflict of interest.
Acknowledgments
I thank Till Strunge and Lorenzo Cremonese for their critical review and comments and Volker Sick for his ongoing support in our common projects.
Supplementary Material
The Supplementary Material for this article can be found online at: https://www.frontiersin.org/articles/10.3389/fenrg.2020.00198/full#supplementary-material
Footnotes
- ^ The term carbonization is often used as an equivalent to mineralization.
- ^ All references to the SDGs refer to the website of the United Nations https://sustainabledevelopment.un.org/, accessed on 12 December 2019.
- ^ DAC refers to a range of technological solutions that are able to extract CO2 from ambient air at any location on the planet.
- ^ “No tools were identified which substantially engaged with actual strategy development, the stage which can shape transformative change.”
- ^ The study by Becker and Kläring investigated the growth of red leaf lettuce.
- ^ If products “made with CO2” only differ in the production phase, but the end product is chemically identical to that of conventional processes, no new interrelations with valid product regulations are to be expected.
- ^ For all SDGs and their targets, please refer to the Supplementary Material.
- ^ For all targets, please refer to the annex.
- ^ SDG 12.2: “By 2030, achieve the sustainable management and efficient use of natural resources.”
- ^ SDG 12.6: “Encourage companies, especially large and transnational companies, to adopt sustainable practices and to integrate sustainability information into their reporting cycle.”
- ^ SDG 12.5: “By 2030, substantially reduce waste generation through prevention, reduction, recycling and reuse.”
- ^ SDG 12.7: “Promote public procurement practices that are sustainable, in accordance with national policies and priorities.”
- ^ e.g., SDG 16.1: “Significantly reduce all forms of violence and related death rates everywhere” or 16.3: “Promote the rule of law at the national and international levels and ensure equal access to justice for all.”
- ^ SDG 17.3: “Mobilize additional financial resources for developing countries from multiple sources; 17.5: “Adopt and implement investment promotion regimes for least developed countries.”
References
Aldaco, R., Butnar, I., Margallo, M., Laso, J., Rumayor, M., Dominguez-Ramos, A., et al. (2019). Bringing value to the chemical industry from capture, storage and use of CO2, A dynamic LCA of formic acid production. Sci. Total Environ. 663, 738–753. doi: 10.1016/j.scitotenv.2019.01.395
Anderer, P., Dumont, U., Massmann, E., and Keuneke, R. (2012). Wasserkraftnutzung in Deutschland. Dessau-Roßlau: Umweltbundesamt.
Balzannikov, M., and Vyshkin, E. (2011). “Hydroelectric power plants reservoirs and their impact on the environment. Environment. technology, resources,” in Proceedings of the 8-th International Scientific and Practical Conference, Yurga.
Barrie, J., Brown, K., Hatcher, P., and Schellhase, H. (2005). Carbon Dioxide Pipelines: A Preliminary Review of Design and Risks. Greenhouse Gas Control Technologies 7. Amsterdam: Elsevier, 315–320.
Becker, C., and Kläring, H.-P. (2016). CO2 enrichment can produce high red leaf lettuce yield while increasing most flavonoid glycoside and some caffeic acid derivative concentrations. Food Chem. 199, 736–745. doi: 10.1016/j.foodchem.2015.12.059
Beuttler, C., Charles, L., and Wurzbacher, J. (2019). The role of direct air capture in mitigation of anthropogenic greenhouse gas emissions. Front. Clim. 1:10. doi: 10.3389/fclim.2019.00010
Blue Planet Ltd (2019). Solving the Carbon Capture Problem by Converting CO2 in High-Value Building Materials. Available online at: http://www.blueplanet-ltd.com/ (accessed December 12, 2019).
BMBF (2015). Bekanntmachung Richtlinie zur Förderung von CO2Plus – Stoffliche Nutzung von CO2 zur Verbreiterung der Rohstoffbasis. Available online at: https://www.ptj.de/lw_resource/datapool/_items/item_6478/banz_at_25.06.2015_b4.pdf (accessed October 25, 2016).
BMBF (2019). Bundesministerium für Bildung und Forschung, Bekanntnmachung der Fördermaßnahme CO2 als nachhaltige Kohlenstoffquelle – Wege zur industriellen Nutzung (CO2-WIN). Available online at: https://www.bmbf.de/foerderungen/bekanntmachung-1875.html (accessed August 15, 2019).
Bundesministerium für Umwelt Naturschutz, Bau und Reaktorsicherheit (2016). Klimaschutzplan 2050–Klimapolitische Grundsätze und Ziele der Bundesregierung. Bonn: Bundesministerium für Umwelt, Naturschutz, Bau und Reaktorsicherheit.
Cameron, G. (1996). Innovation and economic growth. CEPDP (277). Centre for Economic Performance. London: London School of Economics and Political Science.
Carbon Recycling International (n.d.). Driving Change Clean from Industrial Emissions. Available online at: https://www.carbonrecycling.is/ (accessed 6 July 2020).
Carbon8 (2019). Using Carbon Dioxide to Give Waste Value. Available online at: http://c8s.co.uk/. (accessed December 12, 2019).
CarbonNext (2018). The Next Generation of Carbon for The Process Industry: Selection of Most Relevant Processes for Further Investigation. Sheffield, UK: CarbonNext.
Carus, M., Vom Berg, C., and Breitmayer, E. (2019). “CO2 utilisation and sustainability,” in Carbon Dioxide Utilization: From Fundamentals to Production Processes, eds M. North and P. Styring (Berlin: Walter de Gruyter, GmbH), 13–30. doi: 10.1515/9783110563191-002
Chemie3 (2019). Chemie3-Nachhaltigkeitcheck Aktualisiert. Available online at: https://www.chemiehoch3.de/home/chemie3-aktivitaeten/chemie3-nachhaltigkeits-check.html (accessed January, 2020).
Climeworks (n.d.). Let’s Reverse Climate Change. Available online at: https://www.climeworks.com/ (accessed 6 July 2020).
CO2BIO (no date). The Future is Here. Available online at: https://www.co2bio.no/?lang=en (accessed 11 March 2020).
Covestro (2019). CO2 as a Raw Material. Available online at: https://www.covestro.com/en/company/strategy/attitude/co2-dreams (accessed August 16, 2019).
Dautzenberg, G., and Bruhn, T. (2013). Environmental Impacts of Carbon Capture Technologies. An overview of the state of development, potential side effects and current challenges for science and society. IASS Working Paper. Potsdam: IASS.
Dittmeyer, R., Klumpp, M., Kant, P., and Ozin, G. (2019). Crowd oil not crude oil. Nat. Commun. 10, 1–8.
Dollar, D. (1993). Technological differences as a source of comparative advantage. Am. Econ. Rev. 83, 431–435.
European Commission (2019a). Identification and Analysis of Promising Carbon Capture and Utilisation Technologies, Including Their Regulatory Aspects. Brussels: European Commission.
European Commission (2019b). Innovation Fund. Available online at: https://ec.europa.eu/clima/policies/innovation-fund_en (accessed December 12, 2019).
European Union (2019). Sustainable Development in The European Union. Luxembourg: Publications Office of the European Union.
Falcke, T. J., Hoadley, A. F., Brennan, D. J., and Sinclair, S. E. (2011). The sustainability of clean coal technology, IGCC with/without CCS. Process Saf. Environ. 89, 41–52. doi: 10.1016/j.psep.2010.08.002
Favier, A., De Wolf, C., Scrivener, K., and Habert, G. (2018). A Sustainable Future for the European Cement and Concrete Industry: Technology assessment for full decarbonisation of the industry by 2050. Zürich: ETH Zurich.
Global CO2 Initiative (2019). Global Roadmap for Implementing CO2 Utilization. Michigan: Global CO2 Initiative.
Gothenburg Centre for Sustainable Development (n.d.). SDG Impact Assessment Tool. Available online at: https://sdgimpactassessmenttool.org/about (accessed January, 2020).
Grainger-Brown, J., and Malekpour, S. (2019). Implementing the sustainable development goals. A review of strategic tools and frameworks available to organisations. Sustainability 11:1381. doi: 10.3390/su11051381
Group of Chief Scientific Advisors [SAM] (2018). Novel carbon capture and utilisation technologies. Brussels: Group of Chief Scientific Advisors [SAM].
Hansmann, R., Mieg, H. A., and Frischknecht, P. (2012). Principal sustainability components, empirical analysis of synergies between the three pillars of sustainability. Int. J. Sust. Dev. World 19, 451–459. doi: 10.1080/13504509.2012.696220
Heinemann, C., Kasten, P., Bauknecht, D., Bracker, J., Bürger, V., Emele, L., et al. (2019). Die Bedeutung Strombasierter Stoffe für den Klimaschutz in Deutschland. Freiburg: Institute of Applied Ecology.
Hepburn, C., Adlen, E., Beddington, J., Carter, E. A., Fuss, S., Mac Dowell, N., et al. (2019). The technological and economic prospects for CO 2 utilization and removal. Nature 575, 87–97. doi: 10.1038/s41586-019-1681-6
IPCC (2018). Global Warming of 1.5°C. An IPCC Special Report on the Impacts of Global Warming of 1.5°C Above Pre-Industrial Levels and Related Global Greenhouse Gas Emission Pathways, in The Context of Strengthening the Global Response to the Threat of Climate Change, Sustainable Development, and Efforts to Eradicate Poverty. Geneva: IPCC.
Kinkel, S., Lay, G., and Wengel, J. (2004). Innovation, mehr als Forschung und Entwicklung, Wachstumschancen auf anderen Innovationspfaden, Mitteilungen aus der Produktionsinnovationserhebung. Karlsruhe: Fraunhofer ISI.
Koornneef, J., Spruijt, M., Molag, M., Ramirez, A., Faaij, A., and Turkenburg, W. (2009). Uncertainties in risk assessment of CO2 pipelines. Energy Proc. 1, 1587–1594. doi: 10.1016/j.egypro.2009.01.208
Kremer, D., Etzold, S., Boldt, J., Blaum, P., Hahn, K. M., Wotruba, H., et al. (2019). Geological mapping and characterization of possible primary input materials for the mineral sequestration of carbon dioxide in Europe. Minerals 9:485. doi: 10.3390/min9080485
Mac Dowell, N., Fennell, P. S., Shah, N., and Maitland, G. C. (2017). The role of CO2 capture and utilization in mitigating climate change. Nat. Clim. Change 7, 243–249. doi: 10.1038/nclimate3231
Mennicken, L., Janz, A., and Roth, S. (2016). The German R&D program for CO 2 utilization—innovations for a green economy. Environ. Sci. Pollut. Res. 23, 11386–11392.
Mineral Carbonation and International (2019). MCI and Carbon Utilisation. Available online at: https://www.mineralcarbonation.com/ (accessed December 12, 2019).
Naims, H. (2016). Economics of carbon dioxide capture and utilization—a supply and demand perspective. Environ. Sci. Pollut. Res. 23, 22226–22241. doi: 10.1007/s11356-016-6810-2
Naims, H. (2020). Economic aspirations connected to innovations in carbon capture and utilization value chains. J. Ind. Ecol. 1–14. doi: 10.1111/jiec.13003
North, M., and Styring, P. (eds) (2019). Carbon Dioxide Utilization. From Fundamentals to Production Processes. Berlin: Walter de Gruyter, GmbH.
Olfe-Kräutlein, B., Naims, H., Bruhn, T., and Lorente Lafuente, A. M. (2016). CO2 als Wertstoff - Herausforderungen und Potenziale für die Gesellschaft. Potsdam: IASS.
Olfe-Kräutlein, B., Naims, H., Bruhn, T., Lorente Lafuente, A. M., and Tobias, M. (2014). CO2 as an asset? IASS Fact Sheet 2/2014. Potsdam: IASS.
Olfe-Kräutlein, B., Naims, H., Strunge, T., and Chanin, A. (2019). “Societal contributions and policy needs of CCU technologies,” in Summary of the 4th round table CO2 utilization contextualizing carbon dioxide utilization - International policy perspectives on CCU. Workshop Reports I (Potsdam: IASS).
Piria, R., Naims, H., and Lorente Lafuente, A. M. (2016). CCU, Klimapolitische Einordnung und innovationspolitische Bewertung. Adelphi Discussion Paper. Potsdam: IASS.
Salvia, A. L., Leal Filho, W., Brandli, L. L., and Griebeler, J. S. (2019). Assessing research trends related to Sustainable Development Goals. Local and global issues. J. Clean Prod. 208, 841–849. doi: 10.1016/j.jclepro.2018.09.242
Sanna, A., Uibu, M., Caramanna, G., Kuusik, R., and Maroto-Valer, M. (2014). A review of mineral carbonation technologies to sequester CO2. Chem Soc. Rev. 43, 8049–8080. doi: 10.1039/c4cs00035h
Schindler, D. (2013). Geoscience of climate and energy 12. Water quality issues in the oil sands region of the Lower Athabasca River, Alberta. Geosci. Can. 40, 202–214.
Schlögl, R., Abanades, C., Aresta, M., Azapagic, A., Blekkan, E. A., Cantat, T., et al. (2018). Novel Carbon Capture and Utilisation Technologies, Research and Climate Aspects. SAPEA Evidence Review Report. Brussels: European Commission.
Scot Project (2016). Smart CO2 transformation, challenges and opportunities CCU. Available online at: https://www.scotproject.org/content/challenges-and-opportunities-ccu-training-module (accessed December 12, 2019).
Smolny, W., and Schneeweis, T. (1996). Innovation, Wachstum und Beschäftigung, Eine empirische Untersuchung auf der Basis des ifo-Unternehmenspanels, Discussion Paper, Center for International Labor Economics (CILE). Konstanz: University of Konstanz.
Solar Foods (no date). Food out of Thin Air. Available online at: https://solarfoods.fi/ (accessed 11 March 2020).
Spitz, G., Van Ewijk, E., and Kamphof, R. (2016). Global Goals, Local Action? Approaches of Dutch Local Governments to the Sustainable Development Goals. Discussion Paper. Amsterdam: Kaleidos Research.
Sternberg, A., and Bardow, A. (2015). Power-to-what? Environmental assessment of energy storage systems. Energy Environ. Sci. 8, 389–400. doi: 10.1039/c4ee03051f
The Royal Society (2017). The Potential and Limitations of Using Carbon Dioxide. Policy Briefing. London: Royal Society.
United Nations (2015). The Sustainable Development Goals Knowledge Platform. Available online at: https://sustainabledevelopment.un.org/ (accessed December 12, 2019).
United Nations Development Programme [UNDP] (n.d.). Available online at: https://climateimpact.undp.org/#!/toolbar/about-the-tool (accessed March 10, 2020).
United States Government (2019). U.S. national statistics for the U.N. Sustainable Development Goals. Available online at: https://sdg.data.gov/ (accessed December 12, 2019).
Vidic, R. D., Brantley, S. L., Vandenbossche, J. M., Yoxtheimer, D., and Abad, J. D. (2013). Impact of shale gas development on regional water quality. Science 340:6134.
von der Assen, N., and Bardow, A. (2014). Life cycle assessment of polyols for polyurethane production using CO2 as feedstock, insights from an industrial case study. Green Chem. 16, 3272–3280. doi: 10.1039/c4gc00513a
von der Assen, N., Jung, J., and Bardow, A. (2013). Life-cycle assessment of carbon dioxide capture and utilization, avoiding the pitfalls. Energ. Environ. Sci. 6, 2721–2734.
WWF Deutschland (2018). Wie klimaneutral ist CO2 als Rohstoff wirklich? WWF Position zu Carbon Capture and Utilization (CCU). Berlin: WWF Deutschland.
Zimmermann, A., Kant, M., Strunge, T., Tzimas, E., Leitner, W., Arlt, W., et al. (2017). CO2 Utilisation Today, Report 2017. Berlin: Technische Universität Berlin.
Keywords: carbon capture and utilization, sustainable development goals, sustainable technologies, carbon dioxide utilization, carbon recycling
Citation: Olfe-Kräutlein B (2020) Advancing CCU Technologies Pursuant to the SDGs: A Challenge for Policy Making. Front. Energy Res. 8:198. doi: 10.3389/fenrg.2020.00198
Received: 07 May 2020; Accepted: 28 July 2020;
Published: 27 August 2020.
Edited by:
Greeshma Gadikota, Cornell University, United StatesReviewed by:
Rafael Mattos Dos Santos, University of Guelph, CanadaEduardo René Perez Gonzalez, São Paulo State University, Brazil
Copyright © 2020 Olfe-Kräutlein. This is an open-access article distributed under the terms of the Creative Commons Attribution License (CC BY). The use, distribution or reproduction in other forums is permitted, provided the original author(s) and the copyright owner(s) are credited and that the original publication in this journal is cited, in accordance with accepted academic practice. No use, distribution or reproduction is permitted which does not comply with these terms.
*Correspondence: Barbara Olfe-Kräutlein, YmFyYmFyYS5vbGZlLWtyYWV1dGxlaW5AaWFzcy1wb3RzZGFtLmRl