- 1Department of Chemical Engineering, University of Engineering and Technology, Lahore, Pakistan
- 2Institute of Energy and Environmental Engineering, University of the Punjab, Lahore, Pakistan
- 3School of Environmental Engineering, University of Seoul, Seoul, South Korea
- 4Department of Chemical Engineering, COMSATS University Islamabad, Lahore, Pakistan
- 5Institute for Sustainable Industries and Liveable Cities, Victoria University, Melbourne, VA, Australia
- 6Energy Engineering Research and Development Centre, Faculty of Electrical, Energy and Environmental Engineering, University of the Punjab, Lahore, Pakistan
- 7Technical Services Department, Duqm Refinery and Petrochemical Industries Co, Duqm, Oman
Liquefied petroleum gas is an alternative, relatively clean and a supreme source of energy, which is being used as a key component in the global energy supply. The international trade agreements and the chemical and non-chemical demand of liquefied petroleum gas with the increase in the world’s population have brought its production from the processing of natural gas to the limelight. During its processing, a variety of different components are extracted from it, including methane and ethane which remains in the bulk as natural gas. The objective of this research work is to find the capability of investigating the liquefied petroleum gas recovery performance to make the process economical by saving the processing cost and energy. The novelty of this work is to deal with the design and simulation of a liquefied petroleum gas plant using Aspen HYSYS. To make this process energy efficient and economical, different schemes of process alternatives were applied by reducing the sizes of the exchanger and other pieces of equipment. Three cases are studied in which feed is precooled by rerouting the stream and/or by repositioning of the chiller for the recovery of liquefied petroleum gas from natural gas by analyzing their cost and process parameters. The modelling and simulation base case and three different case studies are realized in Aspen HYSYS. It has been observed that case study 2 results in about 10% increase in LPG production where the chiller is repositioned in the separation section of the LPG production flowsheet. Case study 3 shows a maximum decrease in hot side utilities in the flowsheet of about 20% while 10 and 14% decreases are observed for case studies 1 and 2, respectively. Furthermore, economic analysis indicates about 18 and 22% in the capital cost for case studies 2 and 3, respectively, due to the lower size of process units. The outcome of this investigation is to present plenty of suggestions to improve the process efficiency and minimize the requirement to over design the plant components.
Introduction
Energy consumption is an index which represents the industrial development and progress of any country. Due to increased globalization and urbanization, the energy resources are depleting rapidly. There is a massive increase in the per capita energy consumption and results in the increase in global energy demand; hence, dependence on the low-carbon and environment-friendly fuel is increasing (Hu et al., 2019). One of the clean energy resources is natural gas, which is a mixture of methane, ethane, propane, butane, and heavier hydrocarbons. There has been enormous change in technology in natural gas processing since the 1900s (Getu et al., 2013). Furthermore, the share of natural gas as an energy reserve in the developed countries has doubled during the past few decades and is on increase in developing countries. Raw natural gas is a source of dry natural gas; its variant natural gas liquids (NGLs), termed as natural gas condensates, contain sulfur and liquefied petroleum gas. In engineering context, butane and mere propane are designated as liquefied petroleum gas (LPG) (Liu et al., 2015; Faramawy et al., 2016). LPG is extracted from natural gas and is applied as fuel for multi purposes by providing energy to the vehicles and for cooking and heating appliances, and it is used as a refrigerant and aerosol propellant, thus hindering the damage of the ozone layer (Arteconi et al., 2010; Raslavičius et al., 2014; Hao et al., 2016). As per its historical preparation, Dr Walter Snelling is the main authority to prepare it on a laboratory scale in 1910 (Snelling et al., 1915). The said gas is a cleaner gas containing limited contents of sulfur while burning, and it gives no soot to the environment. Pressurized steel vessels are used for its transportation, and a maximum of 80–85% LPG is allowed to be filled in the stated vessels to compensate for its thermal expansion during the change in temperature (Totten and MacKenzie, 2003).
Pakistan is facing severe energy deficiency, and some parts of the countries are still not electrified. The country fossil reserves are near depletion and are pushing high demands on imports (WAPDA, 2021; Rafique and Rehman, 2017). Due to increased industrialization and interest in the energy sector after China–Pakistan Economic Corridor (CPEC) investment, there are short-term plans to increase the country’s thermal power generation (Lin and Ahmad, 2016). The finance-energy and energy-growth nexus have been evaluated by Komal and Abbas (Komal and Abbas, 2015), and it was found that urbanization and industrialization have a positive impact on energy consumption. However, fossil fuels or their derivatives play an important role in the energy sector of the country. In Pakistan, natural gas is used as burning fuel at the domestic level, in the combustion system of power generation, and industries to produce useful products. Its share is about 42% in plants producing electricity (Sayed et al., 2017). Due to the agronomical nature of Pakistan, 16% of natural gas is utilized in the fertilizer sector, 7% as commercial usage, and 3% for other purposes (Alam et al., 2017). Recoverable reserves of natural gas are estimated to be 29.671 Tcf (Asghar, 2015). The requirements of natural gas in the country are increasing together with about 10% per year increase in the production sector because of the excelling population and industrial sector. The government of Pakistan has also developed a new regulation to develop the LPG sector. Currently, 26 companies, including private and public, provide their services regarding the survey and production of natural gas. LPG was introduced in the market as an auto gas due to its sole usage in automobiles. Almost 10 companies are operating in Pakistan for the production of LPG while more than 80 companies are providing their services for its marketing purposes (Ullah et al., 2018).
Several studies inclusive of alterations in the gas recovery plant have been reported in the literature. A few of recent ones include the gas subcooled process comparison with the cold residue recycle and a conventional turbo expander for recovery of ethane from NG (Kherbeck and Chebbi, 2015). Moreover, different methods of natural gas dehydration including triethylene glycol, solid desiccant, and condensation were assessed (Netusil and Ditl, 2011). It was found that the addition of more equipment and even flow splitting may lead to less recovery of certain components (Kherbeck and Chebbi, 2015). Some studies also (Abdulrahman et al., 2015; Sayed et al., 2017) reported development of the optimized process models in Aspen HYSYS for the natural gas processing plant and estimated the preliminary capital cost for gas conditioning and processing. The specific impact on the turbo expander for optimal NGL recovery, feed compositions on different process schemes, gas subcooled, recycle vapor split, and cold residue has also been reported (Getu et al., 2013). The exergy analysis of the NGL recovery processes through modelling and simulation in Aspen HYSYS indicated that most of the exergy destruction happens at the air cooler and column present in the flowsheet (Hu et al., 2019). The exergy destruction in the LPG production process happens at the fractionation columns (Saadi et al., 2019). The exergy analysis carried out in a retrofitted refrigeration system, for the recovery of LPG from the natural gas plant located in Egypt, indicated an increase in the overall LPG recovery by 15.95% (Shehata et al., 2019). Simulations of the natural gas processing plant have been reported in good detail to provide insight into the containment removal with some cost analysis of different turbo expander systems (Mehrpooya et al., 2006; Roy and Amin, 2012; Çinar et al., 2016). Product recovery, energy requirements, and fixed capital cost for the LPG production from NGLs have been investigated, and it was reported that the single column with an overhead recycle is cost effective than a conventional fractional column (B. Raheem, 2015). In a separate study, the effect of vapor recompression and self-heat recuperation has been optimized for propane and propylene separation (Christopher et al., 2017).
A technological review of the process intensification of the oil and gas industry has been reported in the literature (Abdulrahman et al., 2021). Robust process intensification framework has been presented to avoid inconsistency between implementation and research for oil and gas sectors (Abdulrahman et al., 2021). It has been reported that the conceptual processes are much more complex than the commercial scale applications (Qyyum et al., 2022). A detailed assessment of the natural gas liquid processes has been presented with focus on feed condition, product purity and recovery, specific energy consumption, their economics, and their application through available pieces of software (Qyyum et al., 2022). It is found that CNG and LPG recovery is the least cost and most profitable alternative for the six different technological options, including gas to hydrate, gas to liquid, combined heat and power generation, and liquefied natural gas (Jokar et al., 2021). An attempt has been made for the process through which LPG can be recovered from the off gases of the fuel oil refinery to meet the demand of petroleum fuel in automotive, industrial, and domestic sectors (Qamar et al., 2020). The integration of the dehydration recovery and natural gas liquid unit tends to increase the LPG profit and capacity (Masnsour et al., 2020).
A number of studies have been reported across flow diagram alteration and proposed different refrigeration schemes in the NGL or LPG recovery sections of the natural gas processing plant. However, the effect of cooling, its position, and rerouting the process streams is seldom reported. Therefore, the present research work is focused on the improvement in the yield of LPG production by increasing or altering the process of cooling or their orientation in the separation and dehydration section of the natural gas processing plant. Furthermore, techno-economic investigation will be performed to get insight into the variation of the cooling effect on the cost parameters.
The novelty of this project is to deal with the design and simulation of the LPG plant while taking natural gas as a raw source and convert it into LPG in combination with natural gasoline (C5+) containing a greater value as a distinct product. Raw natural gas also contains some valued heavier hydrocarbons known as NGLs and favored to be used as raw feed in the petrochemical industry. To make this process energy efficient and economical, different schemes of raw material processing and heat transfer were applied, and their impact on process and economic parameters was evaluated. Three cases are studied in this research work to increase the recovery of LPG from natural gas by analyzing their cost and process parameters. In case study 1, the feed is precooled through cold box 1; in case study 2, the position of the chiller is in the separation section while in case study 3 the position is in the dehydration section. Aspen HYSYS v8.8 is used for the simulation of this research work. The process flow diagram is categorized into three of its parts. The first one deals with feed preparation usually obtained from the gas well, the second one discusses the liquefaction of natural gas, and the third one is the recovery process of LPG while two of the distillation columns are utilized in the recovery process. This research work is based on modelling and simulation to improve the currently used recovery processes in the industrial sector of Pakistan. Hence, the aim of this research work is to study the various flowsheet alternatives and compare the performance of different case studies. The evaluation criteria will be the capacity of different products for different alternatives, energy in terms of cold and hot utilities, and economic analysis.
Materials and Methods
Process Description
Reservoirs of natural gas are present under the earth’s crust and are combined with crude oil. After the discovery of the natural gas reservoirs by using modern techniques and different drilling steps, absolute processing of the natural gas is initiated. All other contaminants, such as sand, H2O, mud, and acidic gases including CO2 and H2S, NGLs are being extracted from the said process stream. The composition of the well head-treated natural gas is given in Table 1.
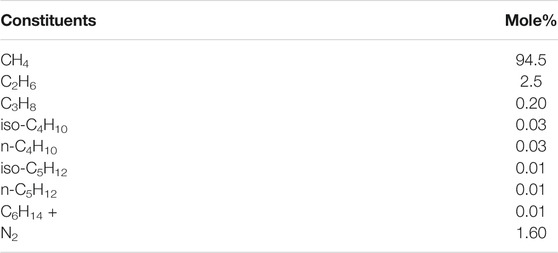
TABLE 1. Constitutions of natural gas (Thimm and Sullivan, 2004; Getzinger et al., 2015; Ullah et al., 2018).
The block flow diagram for the LPG production from natural gas is shown in Figure 1. It indicates that the LPG production from natural gas is composed of four subsections. Initially, the feed is treated which is designated by feed separation and dehydration section sections in Figure 1. Later, NGLs are recovered by using a refrigeration loop and cold box, and then, LPG recovery is attained by the use of distillation columns. These are indicated as NGL and LPG recovery sections in Figure 1, respectively.
Feed Treatment
The feed treatment section consists of removal of heavier hydrocarbons and water from natural gas before its processing. It consists of two subsections: the first one is a separation zone, and the second subsection is a dehydration zone.
Separation Section
The natural gas treatment procedure is initiated from the exit of the gas well. The composition of the feed in terms of liquid, gas, and water quantity is analyzed. To avoid any type of moisturizing contents, a saturator is also installed just before the section of the separator. Saturation of the feed stream is compulsory so that moisture contents present in the system does not absorb more moisture in the process and make the process inefficient. Because of the addition of water to saturate the system, the molar flow rate will exceed. The showering by a saturator to saturate the feed stream is performed, and some extra water is absorbed by the stream. Because of the sensitive nature of the material of construction of the downstream cold box, water is desired to be extracted in a three-phase separator. For LPG recovery, greater pressure is recommended; hence, pressure is set as higher as 600 psig. Dry gas stream containing zero impurities is recommended in the process; any type of free H2O is extracted from the stream. This separator will remove higher hydrocarbons, mud, and water from the stream. A stabilization unit is also installed to stabilize the condensate, and heavy liquids are removed from the stream and converted into fluid fuel. The red solid line region in Figure 2 indicates the separation section of the LPG production from natural gas.
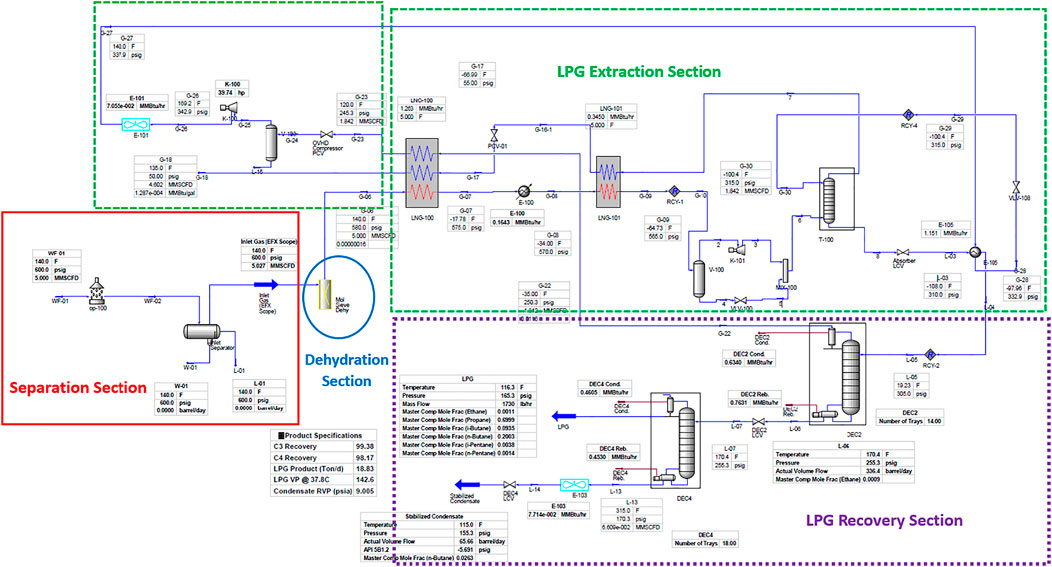
FIGURE 2. Process flow diagram and Aspen HYSYS simulated case study for the LPG production from natural gas.
Dehydration Section
This section deals with the removal of final traces of water proportions present in the feed. The material of construction of cold boxes is of aluminum alloy nature, so it may also corrode due to moisture presence. Meanwhile, operating process parameters can also elevate the effect of moisture contents in it. A number of industrial units are proposed for the stated objective in the literature. Molecular sieves are chosen for the dehydration purpose for the present research work. The material of construction of molecular sieves is of microporous nature consisting of small pours where the adsorption process will separate water contents from the feed. In comparison with other dehydration techniques, molecular sieves have a relatively higher efficiency to extract maximum contents of water from the natural gas feed stream. These sieve beds with tandem or parallel arrangement can be regenerated and reused by heating. In general, parallel orientation of two beds is recommended in LPG production.
Lighter contents of natural gas are found in major quantities (Table 1). Propane, iso-butane, and n-butane are the desired components for the extraction of LPG. The dehydration section is indicated by the blue solid circle region in Figure 2.
Liquefaction of NG and Extraction Zone
NG Liquefaction
The dashed line regions in Figure 2 encase the liquefaction process with steady refrigeration, and it is regarded as the second step. A molecular sieve dehydrator is used to remove the water from the gas stream to its minimum levels. The gas will be forwarded to the cryogenic section of the plant in which maximum removal of liquid from gas is performed. To obtain the cryogenic conditions, a turbo expander is utilized to apply the chilling process. To obtain a temperature less than −100°F, cold boxes are used. The temperature of the stream will be reduced by a steady refrigeration technique. The stream to be liquefied is passed through a multi-pass heat exchanger, aluminum alloy based with a plate and frame type arrangement. Transfer of heat occurs between the main stream of the process and downward streams approaching from the low temperature absorber and LPG recovery unit. The said exchanger (cold box) is not an ordinary type and is used for excessive temperature differences. The gas stream is introduced in the gas chiller which is based on mechanical refrigeration. The second cold box is also installed as a stand heat exchanger. The turbo expander and lower temperature are utilized to extract the liquid from the core stream. The gas stream is expanded by the stated turbo exchanger; ultimately, a decrease in the temperature of the stream is observed. Joule Thomson states that cooling of the gas stream takes place after its sudden expansion. Having greater pressure, a stream containing pure vapor is extracted from the separator and introduced into the turbo expander where during expansion, pressure is reduced. The gradual processing of the gas stream results in a steady decrease of the higher hydrocarbons to produce refined LPG. The expander provides some parts of the energy to increase the pressure of the desired stream and compressor. Two process streams are obtained in the low temperature absorption process: the first outlet is vapor overhead, which is returned to the initial process. This stream contains light hydrocarbons (unwanted for LPG) as side products and named as sale gas. The feed for the LPG recovery zone is obtained from the bottom of the low-temperature absorber.
LPG Extraction
LPG extraction is the closing and conclusive section of the stated scheme. The ultimate amount of C3H8 and C4H10, named LPG, is recovered through this section. Distillation columns (de-butanizer and de-ethanizer) will take out the required hydrocarbons completely. DEC-2 (De-ethanizer) is regarded as the first distillation column. A liquid-phase stream is introduced into it, and energy is provided to heat the feed and extract ethane from the stated stream. A de-butanizer is installed after it, which separates C3H8 and C4H10 in the upper part of the column by applying external energy through a reboiler. The square-type short-dotted line region in Figure 2 indicates the LPG recovery section of the LPG production from the natural gas.
Modelling Strategy
The purpose of this research work is to obtain the maximum yield of LPG from natural gas with increased purity. In doing so, the target is to reduce the overall utilization of energy and capital cost. Therefore, a steady-state simulation model for the complete LPG recovery process is prepared by utilizing the commercially available modelling and simulation software Aspen HYSYS (Version 8.8). It is decentralized into different sections, and afterward, it is integrated into the main flow pattern. The result from the steady-state model is further utilized to measure the chemical, physical, and total energy of each auxiliary. A complete energy analysis of the discussed liquefied petroleum gas recovery plant was performed to find the specific points of efficiency losses. The method chosen for the stated process is the Peng–Robinson equation of state. The base-case steady-state model as developed in Aspen HYSYS for the LPG recovery process from natural gas is shown in Figure 2. The process is decentralized into four zones: the separation zone, dehydration zone, NGL extraction zone, and LPG recovery zone. The LPG recovery target is set at predefined parameters, and the values for some of the specifications are listed in Table 2. The process input parameters for each of the units in the different case studies are reported in Table S1 of supporting information.
Results and Discussion
The conventional process needs to be modified for better efficiency and improvement. In this research work, a hybrid system is proposed for the cooling process to have an effective separation. Lower temperature absorption is responsible for processing via cold separation. A comprehensive model for LPG recovery from natural gas has been developed in Aspen HYSYS. The base-case model is tuned for steady-state simulation. By keeping all process parametric conditions the same as above, different alternatives have been proposed in the flowsheet of the LPG recovery plant. To investigate the equipment’s rearrangements, a comparison regarding the techno-commercial aspect has been conducted. This information is then utilized to decide about the best process for the efficient recovery of LPG.
Case Study 1
The cooling process is directly proportional to the recovery of LPG. By increasing the cooling of the natural gas, the conversion into the liquid form and the production of LPG will be increased. Similarly, as cooling of the gas is decreased, LPG recovery will decrease. To make the process cooler, only the orientation of the process stream is changed. In case study 1 (Figure 3), cold box 1 is the first and initial point from where the feed is passed before entering the three-phase separator. The process flow diagram and its model in Aspen HYSYS are indicated in Figure 3. Temperature of the feed is decreased, and separation is more perfect in the three-phase separator in comparison to the base case. The maximum liquid is extracted from the stream through a separation process, and due to its extraction from the stream, low contents are observed on the downstream gas equipment. The temperature of the feed stream is decreased from 140°F to 48°F which is a reasonable difference in temperature change, and ultimately, more drag force is applied to direct gas into the liquid side. Dehydration is effectively performed at the stated parametric conditions. At low temperature, the effectiveness of adsorption and absorption techniques is enough to extract the moisturizing contents easily containing a low load leading to better LPG recovery.
Case Study 2
The theme of this case study is to change the chiller position from the LPG extraction section to separation section. As a result, the temperature of the feed stream lower than case study 1 is observed. It goes from 140°F from the base case to 17.7°F in case study 2 (Figure 4). Therefore, the major portion of water in the separation section and heavy hydrocarbons (condensate) are removed and directly sent to the LPG recovery unit without passing through the dehydration as well as NGL extraction sections favorable in terms of capital as well as operational cost. A decrease in the size of equipment and cost results are obtained with this arrangement.
Case Study 3
As discussed in case study 2, the position of the process equipment is changed in case study 3 (Figure 5). The position of the chiller is moved from the NGL extraction zone to the dehydrating zone. Inlet gas entering in the dehydrating zone is chilled by the change in the position of the chiller; therefore, the size of the bed is lower than that to the base case and other case studies. While passing the stream through the dehydrating section, its temperature decreased to about −9.2°F from 140°F. The molecular sieve volume will also be decreased by decreasing the size of the bed. In the base case, a greater volume of the molecular sieve is available while in this stated case, a lower volume of the molecular sieve will be required. Because of the stated reason, activity of the amount of molecular sieve in the base case will be greater for a long time than that of the present case study.
Capacity Analysis
Figure 6 depicts the production capacity of all the case studies. The amount of basic feedstock or specific entity processed by a unit, or a plant, is known as the capacity of the unit. By changing the position of the chiller in the flowsheet or rerouting the feed natural gas through cold box 1, it was observed that the recovery of LPG varies. Sizing of the plant equipment has a greater effect on the cost of the process. The size specification of the process units changes due to the change in the position of the chiller in case studies 2 and 3. The process units in different case studies of LPG recovery are given in Table 3. The process units, including molecular sieve beds of the dehydrator and low temperature absorber, are different for case study 2 and case study 3 in comparison to the base case and case study 1. Large-sized equipment will cost high because of the greater usage of the material in it along with the maximum installation space. So, to make the plant capital cost and operational cost on an economical level, the size of the equipment is adjusted to the minimum level with a high efficiency and output so that the capacity of the plant should not be disturbed. Due to the change in the position of the chiller in case studies 2 and 3, the production capacity of LPG is higher in case study 2 supported by the change in the size of the process units.
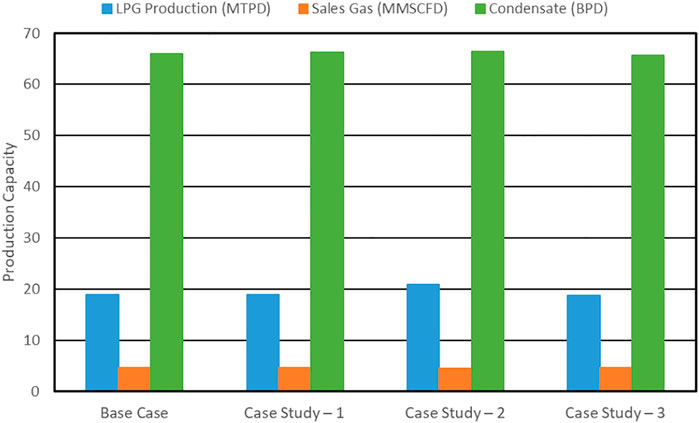
FIGURE 6. Production capacity of LPG and other by-products for the base case and other case studies.
Utility Analysis
In the base case and different case studies proposed in the present research work, natural gas feed is being cooled, either by rerouting it through cold box 1 or changing the position of the chiller in case studies 2 and 3. In the base case, cooling is applied only in the NGL extraction zone while cooling is effectively applied in separating and dehydrating sections of all other case studies. All other parameters are kept constant. Two case studies were investigated by changing the position of the chiller at dehydrating and separation sections. These alterations resulted in saving material costs. The ability to maintain efficient chilling requires the LTCS (Low-temperature carbon steel) section. However, its capital cost is higher than that of the ordinary carbon steel. Apropos to the minimum decreased temperature, i.e., about −20°F, the ordinary carbon steel for the chilling process of case studies 2 and 3 is preferred. This is the extreme limit for the usage of carbon steel after which LTCS is recommended. Hence, the duties of cold box units are decreased by altering the chiller position from the NGL extraction unit to dehydrating and separation sections. Past the chilling process, cooling is completed in the turbo expander and cold box units. Therefore, a change in cold utility requirement for different case studies has been observed. The cold utility requirements for different case studies including the base case are given in Figure 7.
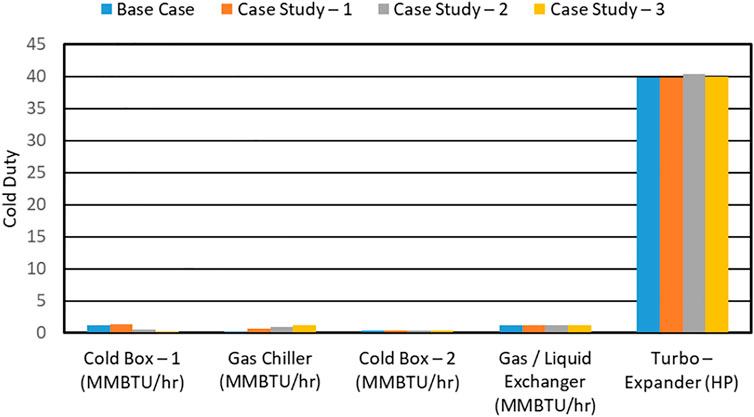
FIGURE 7. Cold utility duties for coolers, chillers, cold boxes, and refrigerators for the base case and different case studies.
For the regeneration purposes of molecular sieve beds, heat energy is utilized in the dehydrating zone. This heat is utilized to dry the molecular sieve and extract moisture from the feed gas in an efficient way. The stated energy being utilized in the LPG recovery section is to vaporize the C2H8 in de-ethanizer to elevate the LPG purity. This energy is also utilized to separate C4 and C3 from higher hydrocarbon in the de-butanizer to make it purified. The heat utility duties are indicated in Figure 8 for the base case, and case studies are shown in Figure 8.
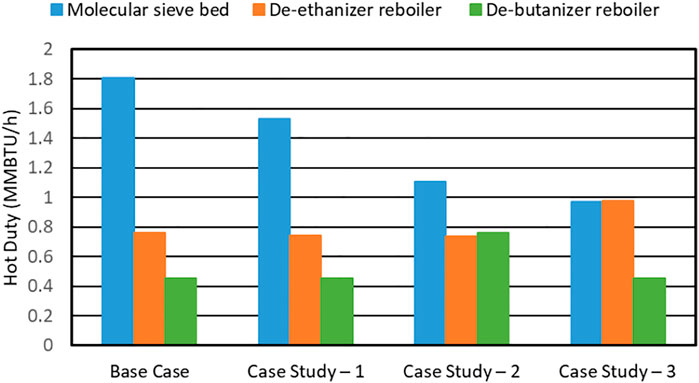
FIGURE 8. Hot utility duties for the molecular sieve bed, de-ethanizer, and de-butanizer reboilers for the base case and different case studies.
Economic Analysis
The material of construction of the equipment, their hot and cold utilities, and their relative sizes are directly related to the total cost of the plant. There are two types of costs which are associated with the feasibility of a plant (i.e., from commissioning to operation). One is named as the capital cost, and the second one is named as the operational cost. There is no drift change in the operational cost for the base case and any alteration proposed in the base case for the present research work while a reasonable change in the capital cost is observed when comparing the capital cost of the base case with other case studies. The capital cost of different case studies is represented for comparison in Figure 9. It is observed that there is a small increase in the capital cost of about 2.5% in case study 1 in comparison to the base case due to the extra load of feed stream in cold box 1. However, there is a sharp decrease of nearly 18 and 22% in the capital cost for case studies 2 and 3, respectively, due to the lower size of absorption and adsorption units. The decrease in temperature to an acceptable minimum level for maximum recovery of LPG, refrigeration process, and chilling process will have a greater effect on the overall capital cost.
The detailed cost of each unit of the LPG recovery plant for the base case and three different case studies for comparison is presented in Figure 10. It is observed that case study 3 will have the least cost in comparison to the base case and case studies 1 and 2. It must be kept in mind that these cost numbers are estimates obtained through modelling and simulation. However, their trend will remain the same.
Conclusion
This research work clearly focuses on the energy optimization and cost optimization for the recovery of LPG from natural gas by utilizing different process configurations of equipment and their positioning while keeping the process parametric conditions the same as in the base case. In case study 1, the natural gas is rerouted through cold box 1. In case study 2, the chiller position is in the separation section while it is in the dehydration section for case study 3. It is observed that case study 3 is the optimized configuration in the context of the process and economic parameters when compared with all the other case studies. In case study 3, gas cooling is accomplished just after the separation, and it is upstream of the dehydration section with optimized size specification of the molecular sieve dehydrator bed and low temperature absorber. Furthermore, the turbo expander and low temperature absorption configuration system produced greater recovery of liquefied petroleum gas, i.e., 1910 lb/hr of LPG was obtained out of 5 MMSCFD raw natural gas with a maximum purity of 99.9%. Therefore, the present research work will contribute toward the process improvement by a change in the process flowsheet and observing better utilities and economic values.
Data Availability Statement
The original contributions presented in the study are included in the article/Supplementary Material; further inquiries can be directed to the corresponding authors.
Author Contributions
UA, MZ, and AA developed the conceptualization and methodology of the study. HZ and Y-KP managed resources and valuable research insights into the study. AR and SD provided literature resources and helped in analysis. MB contributed to the writing and provided valuable research insights. All authors have read and agreed to the published version of the manuscript.
Funding
The financial support from the National Research Foundation of Korea under the project (NRF-2020M1A2A2079801) is gratefully acknowledged. Also, this work was supported by the National Research Foundation of Korea (NRF) grants funded by the Korean government (MSIT) (NRF-2021R1A2C3011274).
Conflict of Interest
Author MB was employed by the company Duqm Refinery and Petrochemical Industries Co.
The remaining authors declare that the research was conducted in the absence of any commercial or financial relationships that could be construed as a potential conflict of interest.
Publisher’s Note
All claims expressed in this article are solely those of the authors and do not necessarily represent those of their affiliated organizations, or those of the publisher, the editors, and the reviewers. Any product that may be evaluated in this article, or claim that may be made by its manufacturer, is not guaranteed or endorsed by the publisher.
Supplementary Material
The Supplementary Material for this article can be found online at: https://www.frontiersin.org/articles/10.3389/fenrg.2021.785827/full#supplementary-material
References
Abdulrahman, I., Máša, V., and Teng, S. Y. (2021). Process Intensification in the Oil and Gas Industry: A Technological Framework. Chem. Eng. Process. - Process Intensification 159, 108208. doi:10.1016/J.CEP.2020.108208
Abdulrahman, R. K., Zangana, M. H. S., and Sebastine, I. M. (2015). Optimal NGL Recovery from Natural Gas Using Turboexpander: A Case Study and Simulation. Chem. Technol. Fuels Oils 51 (5), 536–538. doi:10.1007/s10553-015-0636-3
Alam, M. S., Paramati, S. R., Shahbaz, M., and Bhattacharya, M. (2017). Natural Gas, Trade and Sustainable Growth: Empirical Evidence from the Top Gas Consumers of the Developing World. Appl. Econ. 49 (7), 635–649. doi:10.1080/00036846.2016.1203064
Arteconi, A., Brandoni, C., Evangelista, D., and Polonara, F. (2010). Life-cycle Greenhouse Gas Analysis of LNG as a Heavy Vehicle Fuel in Europe. Appl. Energ. 87 (6), 2005–2013. doi:10.1016/j.apenergy.2009.11.012
Asghar, Z. (2015). Identification and the Strategic Role of Stakeholders along Mining Value Chain in Pakistan. Available at: https://core.ac.uk/download/pdf/188774525.pdf (Accessed Jun 09, 2021).
B. Raheem, A. (2015). Comparative Economic Investigation Options for Liquefied Petroleum Gas Production from Natural Gas Liquids. Ajche 3 (2), 55. doi:10.11648/j.ajche.s.2015030201.17
Christopher, C. C. E., Dutta, A., Farooq, S., and Karimi, I. A. (2017). Process Synthesis and Optimization of Propylene/Propane Separation Using Vapor Recompression and Self-Heat Recuperation. Ind. Eng. Chem. Res. 56 (49), 14557–14564. doi:10.1021/acs.iecr.7b03432
Çinar, C., Şahin, F., Can, Ö., and Uyumaz, A. (2016). A Comparison of Performance and Exhaust Emissions with Different Valve Lift Profiles between Gasoline and LPG Fuels in a SI Engine. Appl. Therm. Eng. 107, 1261–1268. doi:10.1016/j.applthermaleng.2016.07.031
Faramawy, S., Zaki, T., and Sakr, A. A.-E. (2016). Natural Gas Origin, Composition, and Processing: A Review. J. Nat. Gas Sci. Eng. 34, 34–54. doi:10.1016/j.jngse.2016.06.030
Getu, M., Mahadzir, S., Long, N. V. D., and Lee, M. (2013). Techno-economic Analysis of Potential Natural Gas Liquid (NGL) Recovery Processes under Variations of Feed Compositions. Chem. Eng. Res. Des. 91 (7), 1272–1283. doi:10.1016/j.cherd.2013.01.015
Getzinger, G. J., O’Connor, M. P., Hoelzer, K., Drollette, B. D., Karatum, O., Deshusses, M. A., et al. (2015). Natural Gas Residual Fluids: Sources, Endpoints, and Organic Chemical Composition after Centralized Waste Treatment in Pennsylvania. Environ. Sci. Technol. 49 (14), 8347–8355. doi:10.1021/acs.est.5b00471
Hao, H., Liu, Z., Zhao, F., and Li, W. (2016). Natural Gas as Vehicle Fuel in China: A Review. Renew. Sustain. Energ. Rev. 62, 521–533. doi:10.1016/j.rser.2016.05.015
Hu, H., Jiang, H., Jing, J., Pu, H., Tan, J., and Leng, N. (2019). Optimization and Exergy Analysis of Natural Gas Liquid Recovery Processes for the Maximization of Plant Profits. Chem. Eng. Technol. 42 (1), 182–195. doi:10.1002/ceat.201800238
Jokar, S. M., Wood, D. A., Sinehbaghizadeh, S., Parvasi, P., and Javanmardi, J. (2021). Transformation of Associated Natural Gas into Valuable Products to Avoid Gas Wastage in the Form of Flaring. J. Nat. Gas Sci. Eng. 94 (104078), 202178. doi:10.1016/J.JNGSE.2021.104078
Kherbeck, L., and Chebbi, R. (2015). Optimizing Ethane Recovery in Turboexpander Processes. J. Ind. Eng. Chem. 21, 292–297. doi:10.1016/j.jiec.2014.02.035
Komal, R., and Abbas, F. (2015). Linking Financial Development, Economic Growth and Energy Consumption in Pakistan. Renew. Sustain. Energ. Rev. 44, 211–220. doi:10.1016/j.rser.2014.12.015
Lin, B., and Ahmad, I. (2016). Technical Change, Inter-factor and Inter-fuel Substitution Possibilities in Pakistan: A Trans-log Production Function Approach. J. Clean. Prod. 126, 537–549. doi:10.1016/j.jclepro.2016.03.065
Liu, K., Zhang, B. J., and Chen, Q. L. (2015). A New Adsorption Process to Intensify Liquefied Petroleum Gas Recovery from Raw Natural Gas. Energ. Proced. 75, 853–859. doi:10.1016/j.egypro.2015.07.183
Masnsour, A. A., Shehata, W. M., and Khalifa Gas, F. (2020). Integrating Dehydration and Natural Gas Liquids Processes for Maximization of Natural Gas Liquids Production. Int. J. Eng. Res. Technol. 13, 5055–5065. Available: http://www.irphouse.com5055.
Mehrpooya, M., Gharagheizi, F., and Vatani, A. (2006). An Optimization of Capital and Operating Alternatives in a NGL Recovery Unit. Chem. Eng. Technol. 29 (12), 1469–1480. doi:10.1002/ceat.200600220
Netusil, M., and Ditl, P. (2011). Comparison of Three Methods for Natural Gas Dehydration. J. Nat. Gas Chem. 20 (5), 471–476. doi:10.1016/S1003-9953(10)60218-6
Qamar, R. A., Mushtaq, A., Ullah, A., and Uddin Ali, Z. (2020). Simulation of Liquefied Petroleum Gas Recovery from off Gases in a Fuel Oil Refinery. Arfmts 73 (1), 109–130. doi:10.37934/arfmts.73.1.109130
Qyyum, M. A., Naquash, A., Haider, J., Al-Sobhi, S. A., and Lee, M. (2022). State-of-the-art Assessment of Natural Gas Liquids Recovery Processes: Techno-Economic Evaluation, Policy Implications, Open Issues, and the Way Forward. Energy 238, 121684. doi:10.1016/J.ENERGY.2021.121684
Rafique, M. M., and Rehman, S. (2017). National Energy Scenario of Pakistan - Current Status, Future Alternatives, and Institutional Infrastructure: An Overview. Renew. Sustain. Energ. Rev. 69, 156–167. doi:10.1016/j.rser.2016.11.057
Raslavičius, L., Keršys, A., Mockus, S., Keršienė, N., and Starevičius, M. (2014). Liquefied Petroleum Gas (LPG) as a Medium-Term Option in the Transition to Sustainable Fuels and Transport. Renew. Sustain. Energ. Rev. 32, 513–525. doi:10.1016/j.rser.2014.01.052
Roy, P. S., and Amin, M. R. (2012). Aspen-HYSYS Simulation of Natural Gas Processing Plant. J. Chem. Engg 26 (1), 62–65. doi:10.3329/jce.v26i1.10186
Saadi, T., Jeday, M.-R., and Jaubert, J.-N. (2019). Exergetic Analysis of an LPG Production Plant Using HYSYS Software. Energ. Proced. 157 (2018), 1385–1390. doi:10.1016/j.egypro.2018.11.303
Sayed, A. E.-R., Ashour, I., and Gadalla, M. (2017). Integrated Process Development for an Optimum Gas Processing Plant. Chem. Eng. Res. Des. 124, 114–123. doi:10.1016/j.cherd.2017.05.031
Shehata, W. M., Bhran, A. A., Shoaib, A. M., Ibrahim, A. A., and Gad, F. K. (2019). Liquefied Petroleum Gas Recovery Enhancement via Retrofitting the Refrigeration System of an Existing Natural Gas Liquid Plant. Asia‐pac J. Chem. Eng. 14 (2), e2292. doi:10.1002/apj.2292
Snelling, W. O., Claudy, C. H., and Kirsch, J. P. (1915). Two Startling Discoveries in Oil Refining on JSTOR. Weekly J. Pract. Inf. 112, 266–277. Available at: https://www.jstor.org/stable/26015802?seq=1#metadata_info_tab_contents.
Thimm, H. F., and Sullivan, J. (2004). Natural Gas Processing Principles and Technology-Part I. Available at: www.hfthimm.com (Accessed Jun 09, 2021).
Totten, G. E., and MacKenzie, S. (2003). Handbook of Aluminum: Vol. 1: Physical Metallurgy and Processes. Florida, USA: CRC Press.
Ullah, K., Hamid, S., Mirza, F. M., and Shakoor, U. (2018). Prioritizing the Gaseous Alternatives for the Road Transport Sector of Pakistan: A Multi Criteria Decision Making Analysis. Energy 165, 1072–1084. doi:10.1016/j.energy.2018.10.055
WAPDA (2021). Water & Power Development Authority. Available at: http://www.wapda.gov.pk/(accessed Jun 07, 2021).
Keywords: LPG recovery, modelling and simulation, utilities, process integration, HYSYS
Citation: Ali U, Zafar M, Ahmed A, Zaman HK, Razzaq A, Daood SS, Bashir M and Park Y-K (2021) Techno Commercial Analysis of Liquefied Petroleum Gas Recovery From Natural Gas Using Aspen HYSYS. Front. Energy Res. 9:785827. doi: 10.3389/fenrg.2021.785827
Received: 29 September 2021; Accepted: 19 November 2021;
Published: 24 December 2021.
Edited by:
Shiliang Yang, Kunming University of Science and Technology, ChinaReviewed by:
Shuanshi Fan, South China University of Technology, ChinaBheru Lal Salvi, Maharana Pratap University of Agriculture and Technology, India
Copyright © 2021 Ali, Zafar, Ahmed, Zaman, Razzaq, Daood, Bashir and Park. This is an open-access article distributed under the terms of the Creative Commons Attribution License (CC BY). The use, distribution or reproduction in other forums is permitted, provided the original author(s) and the copyright owner(s) are credited and that the original publication in this journal is cited, in accordance with accepted academic practice. No use, distribution or reproduction is permitted which does not comply with these terms.
*Correspondence: Syed Sheraz Daood, c2Rhb29kLmljZXRAcHUuZWR1LnBr; Young-Kwon Park, Y2F0YWxpY2FAdW9zLmFjLmty
†These authors have contributed equally to this work