- 1Economics and Management School, Beijing University of Chemical Technology, Beijing, China
- 2National Science Library, Chinese Academy of Sciences, Beijing, China
Introduction: Detecting radical innovations in the solar energy domain could offer innovation references and support the promotion of solar energy. However, relevant studies in the solar energy domain are lacking, and the related methods need to be improved.
Methods: In this paper, a new framework to identify radical innovations in the solar energy domain is proposed by combining a technological convergence study and scientific relation analysis, and the link prediction method is utilized to detect potential radical innovations in this domain.
Results: 1) The distributions of both the technological classes and scientific categories are uneven in the solar energy domain. The top 15 technological classes account for nearly 75.46% of all classifications. Fifteen scientific categories are cited by all the patents, and applied physics, multidisciplinary material science, energy and fuels play important roles in this domain. 2) The relationships among technological classes have evolved over time and have mainly focused on neighbouring disciplines. 3) A total of 130 patents containing new convergence relationships and/or closely related to science are identified as radical innovations. Radical innovative topics are related to the subdomains of solar photovoltaic (solar PV), heat storage, heat exchangers, and solar collectors. 4) Five potential radical innovative topics are identified. Automatic plants for producing electric energy, solar energy ecology houses, and so on are considered to have great potential in the future.
Discussion: The results are consistent with the authoritative report and previous studies, which verify the viability of our methods. And the findings have important implications for scientists, policy-makers, and investors in this domain.
1 Introduction
The massive burning of mineral energy has caused global environmental pollution and ecological destruction, including the extreme overheating of the environment (Ma et al., 2021; Arsalan et al., 2022; Zakari et al., 2022). Solar energy is a free and environmentally friendly supply of power that has a negligible impact on the environment (Almutairi, et al., 2021) and has tremendous potential to replace fossil fuels with secure, clean, and sustainable energy (Trancik et al., 2015; Navothna and Thotakura, 2022). Many nations, such as the United States, Spain, Morocco, India, and China, have implemented solar energy systems in their electrical grids (Soomar, et al., 2022). The wide application of solar energy relies on technological development (Gao and Zhang, 2022). Hence, many countries have issued policies encouraging the technological innovation of solar energy. For example, the China National Energy Administration issued a notice on the construction of wind power and photovoltaic power generation projects in 2020, and the investment in solar technologies has reached CNY1.5 billion.
Radical innovation is very important for technological development. It has been regarded as a useful solution that leads to substantial improvements in products/services and can bring dramatic improvements to existing technologies (Haas and Ham, 2015; Rampa and Agogue, 2021). In the big data environment, the complex, multidimensional, and continuous evolutionary characteristics of science and technological development are becoming more observable than ever before. In particular, solar technology is moving forwards very fast, both in terms of its own technological advancement and its standing among global renewable energy technologies. It is important to identify radical innovations to offer innovation references for countries and enterprises to rationally allocate innovation resources and seek innovation improvements.
Radical innovation identification has been realized from different perspectives (Fleming, 2001; Hargadon, 2003; Dahlin and Behrens, 2005; Yun and Geum, 2019). Due to their high efficiency and objectivity, bibliometric methods have attracted much interest from researchers, and patent data, which contain rich technical information, have been widely used to study radical innovations. Among the various quantitative methods, technological convergence analysis has been applied widely in recent years and is mainly based on the cooccurrence relationship between different technological fields in patents (Fleming, 2001; Hargadon, 2003). It is regarded as the main source of radical innovation, and some researchers have also utilized this method in the innovation study of solar energy (Wang et al., 2018; Zhong, 2019; Zhang et al., 2021).
However, there are some limitations in radical innovation identification in the solar energy domain. First, the quantitative research related to solar energy is still lacking. Second, using a single approach that based on technological knowledge convergence to detect innovation performance may lead to missing some vital radical innovations. Many researchers have proven that the scientific knowledge base of patents has an essential effect on a patent’s value, and the scientific citations of patents have been regarded as a critical signal to distinguish radical innovations from other innovations (Sung et al., 2015). Thus, both the technological and scientific knowledge should be taken into considered when detecting the radical innovation. Last, the identified innovations based on technological convergence are often weak with respect to the technological detail information because studies are focused on the provision of the potential categories. To sum up, the timely, objective and sufficient information is not available for personnel in solar energy domain.
Based on the above, in this study, the knowledge convergence study that combines the technological convergence analysis and scientific signal analysis, as well as the topic analysis are integrated in the detection of radical innovations in solar energy domain. On the one hand, important and useful information for personnel in solar energy and related domains is provided. On the other hand, a novel and open framework is provided for radical innovation identification, which could improve the completeness of identification results.
The remainder of this paper is organized as follows. A literature review is presented in the next section, which introduces the theoretical background. Then, the data processing and methods used in this study are introduced. After that, the analysis results are presented and are followed by a discussion. Finally, the last section offers the conclusion and policy implications.
2 Literature review
2.1 Radical innovation identification
In organizational theory, a breakthrough innovation is defined as a novel and valuable solution that substantially improves products/services and exerts tremendous influence on industries and markets (Haas and Ham, 2015). This term is commonly used to explain competitive advantage and industry restructuring (Sung et al., 2015). Thus, radical innovation identification is studied in many disciplines and has been identified from different perspectives (Fleming, 2001; Hargadon, 2003; Dahlin and Behrens, 2005; Yun and Geum, 2019). In the era of big data, the qualitative method that based on the expert evaluation was inapplicable to the related study, and the bibliometric methods based on the patent analysis have attracted much interest from researchers due to the high efficiency and objectivity. Related studies can be classified into the following three types:
First, some works have detected radical innovation based on the measurement of novelty. For example, Dahlin and Behrens (2005) assumed that patents with more recent backwards citations tend to be more novel and could be identified as radical innovations. Sam and Reinhilde (2013) considered highly scientific knowledge to be related to more novel patents that are more likely to be radical innovations.
Second, network structure analysis has been widely used in radical innovation identification studies (Yun and Geum, 2019). Most of the related studies have regarded the knowledge network as a complex system. They identified radical innovation based on measuring the effects of their emergence on network stability.
Third, knowledge convergence has recently attracted much attention from researchers. Hargadon claimed that most radical innovations occur from the knowledge convergence of different technological fields (Hargadon, 2003). Many other scholars have also verified that technological knowledge convergence is the primary source of radical innovations (Fleming, 2001; Negroponte, 2003; Schoenmakers and Duysters, 2010). Meanwhile, the scientific knowledge convergence was also regarded as the main source of radical innovations (Narin et al., 1997; Tortoriello et al., 2014). And some researchers identified the radical innovations based on the diversity of scientific citations. Dahlin and Behrens (2005) defined the patents that have more scientific citations as radical innovations. Arts et al. (2013) also confirmed the strong link between the quantity of scientific citations and the radical innovations.
2.2 Technological convergence study
Technological convergence from crossing disciplines has become one of the critical ways to identify radical innovations (Flerming, 2001). Previous studies have focused on the general trend of convergence (Kong et al., 2020) and fusion degree studies (Park and Yoon, 2018). Recently, many researchers have put efforts into technological opportunity studies.
Most scholars have contributed to disclosing the convergence process by using patent data (Faria, 2017), which is normally considered an important innovation indicator. For instance, Lee et al. (2022) investigated the technological convergence of artificial intelligence based on patent data registered at the United States Patent and Trademark Office (USPTO) from Google Patent Datasets. Network analysis is commonly used to analyse patent data, which covers cocitation analysis (Faria and Andersen, 2017), coclassification analysis (Ko et al., 2014), and so on. Among the diverse methods, coclassification analysis has been proven to be an effective approach (Ko et al., 2014). First, classification systems such as the International Patent Classification (IPC) provide us with a flexible option to analyse technological convergence at diverse levels. Second, coclassification analysis can be used to overcome some limitations, such as the time lag that exists in the citation network. For example, Zhu et al. (2022) examined technological convergence in 35 technical fields with 49, 687, 173 patents from 2000 to 2018 by using the coclassification of IPCs. Thus, the coclassification method is adopted to explore technological convergence in this study.
The IPC was established by the Strasbourg Agreement in 1971 and provides a hierarchical system for the classification of patents. If more than one IPC code is assigned to a patent, it means that the patent contains knowledge from more than one category, which demonstrates the technological convergence of these categories (Feng et al., 2020). Previous studies have shown that the partitioning of technology at the 4-digit IPC level has important implications when studying technological innovation (Wang et al., 2019). Based on this, our study defines technological convergence as the cooccurrence of 4-digit IPCs in patents in the solar energy domain.
Recently, many researchers have been involved in technological convergence opportunity studies and have put forwards various link prediction methods to detect potential relations that do not exist but have high potential to appear in the future. For example, based on patent data from 2010 to 2019, Kim and Cho (2022) explored potential technological convergence using a link prediction model and found that IT technologies are likely to play a crucial role in converging military technologies. The related methods can be divided into two categories: the first category is based on the structural similarity in the network domain, such as the Adamic–Adar (AA) index (Adamic and Adar, 2003), Resource Allocation (RA) index (Zhou et al., 2009), and Jaccard coefficient (Jaccard, 1901). The other category is network embedding algorithms in the field of machine learning (Isella et al., 2011). Our study applies the RA structural similarity method to link predictions to detect potential radical innovations because this kind of approach is not restricted by the distance function and has already been applied successfully in patent analysis by researchers (Cao et al., 2019).
The literature on technological convergence has laid the foundations for radical innovation detection. However, there is still a lack of a systematic analysis of radical innovation identification. First, using a single approach based on the technological knowledge convergence may lead to some key innovations being missed. The intimate relationships between science and technology have been widely studied (Narin et al., 1997; Huang et al., 2015; Sung et al., 2015). Moreover, previous results are often weak with respect to the technological detail design because they focus on the provision of the potential categories, while the true meaning of a potential technology is not specified. Thus, this paper combines IPC cooccurrence analysis, link prediction, text mining, and manual work to conduct a technological convergence study and integrates the role of the scientific knowledge base to detect radical technological topics.
2.3 Innovation study of solar energy technology
Patents are widely used as a measure of innovation. To study innovation in the field of solar energy, many scholars have studied patents in the field. These studies can be classified into the following three categories:
The first category is related to the study of the technology road map. For example, the trajectories of key technologies in the Chinese SWH and PV industries have been studied to understand the technology development status (Wang et al., 2018); the evolution of China’s solar energy patents has been analysed from different views, including the domain distribution, institution distribution, and the technology life cycle (Zhang et al., 2021); a solar power knowledge ontology schema has been constructed from the comprehensive literature and patent review, and the topics have been identified by using machine learning techniques and the Latent Dirichlet allocation (LDA) algorithm (Trappey et al., 2019). Zhong (2019) detected the evolutionary path and three key technology frontiers in building-integrated photovoltaics by network analysis and text clustering. Lai et al. (2021) used main path analysis to explore thin film solar technological trajectories by eliminating the self-citation effect. Luan et al. (2021) disclosed the driving forces of solar energy technology innovation and evolution and found that evolutionary trajectory analysis reveals different characteristics in different stages. The spatiotemporal evolution of the PV value chain was explored from a global perspective based on priority patent applications. Yuan et al. (2022) explored the spatiotemporal evolution of the PV value chain and found that global innovation activities in developing PV technologies are uneven in the temporal dimension.
In the second type of study, the key influencing factors of technological innovation are analysed. For example, Han and Magee (2018) studied how the scientific citations of patents in this domain impact technological innovations; based on the patent data of 25 countries from 1978 to 2003, it was proven that the public policies related to renewable energy have played an essential role in technological innovations in this field (Johnstone et al., 2010). Yun et al. (2019) showed that adopting a strategy of technological diversification and R&D collaboration exerts a positive effect on the solar PV firm performance based on 50 firms’ patent data. Taking the solar PV industry as a case study, Gao (2022) investigated the impact of international patent inflows on innovations in recipient countries and found that international patent inflows have a positive effect on innovations in recipient countries, but the impact is stronger for leading countries than for late-coming countries.
The third category is related to technological convergence. For example, Son and Cho (2020) conducted an IPC code cooccurrence network analysis of PV patents over a 15-year period to analyse technology fusion in the PV industry in South Korea and demonstrated that the structural pattern of fusion has been diversified or decentralized over time; the emergence of new combinations of unrelated technologies in the solar domain at the regional level was explored to prove that unrelated technologies are more likely to be recombined when they are strongly colocated in the same region (Li et al., 2020). Kangas et al. (2021) studied the technological convergence between information and communication technology (ICT) and solar energy. They found that the development of basic technologies has been even faster than the development of solar PV ICT solutions.
However, these studies have some limitations. First, there is still a lack of systematic analysis of radical innovations in the solar energy domain, most of the studies are focused on the solar PV sub-domain. Second, few of the convergence studies have combined different knowledge source (i.e., technological knowledge and scientific knowledge) into radical innovation detection; thus, some key radical innovations may have been lost. Last, the identified innovations based on technological convergence are often weak with respect to the technological detail information because studies are focused on the provision of the potential categories (i.e., the IPC or CPC categories). In contrast, the true meaning of a potential technology has not been specified. Based on this, in this paper, we incorporate technological convergence analysis and scientific knowledge linkage analysis into study. The patents with new technological convergence and more scientific citations in the solar energy domain are identified as the radical innovations. To provide more detailed information, the LDA model was utilized into study of the topics of radical innovations.
3 Methods
3.1 Data
The patent data in this study were collected from the Derwent Innovation Index, which contains patents from worldwide patent-issuing authorities. We searched the topic “solar energy” or “solar power” or “Sun’s energy” or “Sun’s power” and the IPC code “F24J002” or “H01L031” or “F03G006” or “F24D017” or “F24D003” and obtained 10,995 patents from 2000 to 2019 in the solar energy domain (downloaded on 20 October 2020, and the original data could be found in Supplementary Appendix S1).
3.2 Methodology
This study aims to explore the radical innovations in the solar energy domain by combining the technological convergence method and scientific relation analysis and to detect radical innovations that do not existing now but have high probabilities of appearing in the future. To detect more detailed information, the technological topics are explored using text mining and qualitative analysis.
Figure 1 shows the framework of this study. First, the technological convergence relationships are constructed based on the IPCs’ cooccurrence in the solar energy patents. Then the distribution of technological classifications and the evolution of their cooccurrence relationships are studied, and the radical innovations with new convergence relationships in recent years are identified. Second, the patents with more scientific citations are selected as possible radical innovations too. Third, the topics of patents containing the new technological convergence relationships as well as the strong scientific knowledge relations are analysed by using text mining. Finally, the potential radical innovative topics are identified by using the link prediction method and manual work through analyzing the content of related previous patents that contain the identified relationships.
3.2.1 Knowledge convergence analysis
The knowledge convergence analysis including technological and scientific knowledge convergence analysis. First, classification codes that assigned to the patents could represent the disciplinary knowledge. The cooccurrence of different codes in a patent could represent the technological knowledge convergence from different fields. In this study, we use the IPC because it is a widely used and international standard classification. The IPC contains eight sections, 131 classes and 642 subclasses. Previous studies have shown that the partitioning of technology at the subclass level (4-digit IPC) has important implications when studying technological innovation (Yun and Geum, 2019). Based on this, we define technological knowledge convergence as the cooccurrence of 4-digit IPCs in patents. And the patents with new convergence relationships are identified as the possible radical innovations. Second, we adopt the number of scientific citations of the patent as the metric of scientific knowledge convergence, which was proved to has strong relationship with radical innovations (Dahlin and Behrens, 2005; Arts et al., 2013).
3.2.2 Radical topic detection
To gain a deeper understanding of radical innovations, the topics of the identified radical innovations are explored using text mining. LDA is a popular text mining method that is widely used in patent analysis. In this study, we combine LDA with the term-extraction method (by using the Cortext tool) to summarize the topics. The detailed formulas of LDA are given as follows:
where D indicates the document set which contains
Furthermore, in this study, the coherence index is used to determine the number of topics (Stevens et al., 2012). The coherence index is a measure of the semantic similarity of keywords contained in each topic. The number of topics with the highest coherence value is the best number of topics. The formula is written as follows:
3.2.3 Link prediction
Link prediction method was utilized to study the promissing technologies in this paper. Among the various methods, the RA index has been proven to have good performance in many networks, especially in short-path networks (Zhou et al., 2009). Thus, in this study, RA is selected to predict technological convergence in the solar energy field.
The idea of the RA index is that if two disciplines have a greater number of common neighbours, they have a high possibility of a convergence relationship in the future. If their common neighbours have more convergence relationships with other disciplines, their contributions to the connection of these two disciplines are lower. The RA index can be calculated as follows:
where u and v are two IPCs in the network,
We use the area under the curve (AUC) to measure the accuracy of the link prediction algorithms. Ten percent of existing links are randomly selected as test links. A link prediction algorithm gives a score for each link in the test set to qualify its existence likelihood. The value of AUC can be viewed as the probability that the score of an existing link is higher than a randomly chosen non-existing link (Martinez et al., 2017). In the implementation, we compare the scores of a randomly chosen existing link and a randomly chosen non-existing link and compare them independently n times. n’ is the number of times that the score of an existing link is higher than that of a non-existent link, and n” is the number of times that they have the same score. The value of AUC is defined as follows:
4 Results
From 2000 to 2019, 373 IPCs covered 10,955 collected patents, and the total number of occurrence reached 23,957. The undirected weighted convergence networks from 2000 to 2019 are constructed based on the cooccurrence relations of the IPC codes. The nodes denote the technological disciplines (IPC codes), the links are the convergence relationships, and the weights are the frequency of cooccurrences of the two technological disciplines. Figure 2 displays the technological convergence map from 2000 to 2019. For better presentation, the weights of links that are greater than 10 are selected. As a result, 308 IPC codes are shown in the figure. Five components are detected using the Van Eck and Waltman association strength measure in VOSviewer (Van and Waltman, 2010).
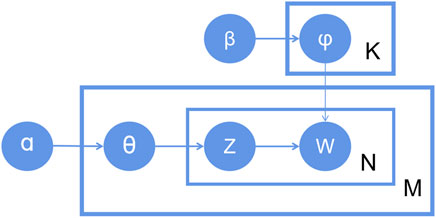
FIGURE 2. Technological convergence map of the solar energy domain; VOSviewer is used for visualization.
4.1 Distribution of technological classifications from 2000 to 2019
Figure 3 shows that with the increase in patents related to the domain, there is an evident increase in the number of categories from 2000 to 2010. From 2011 to 2019, there are downwards trends in the numbers of patents and categories. This indicates that the more technological classes of this domain tend to link with each other from 2000 to 2010 and that there is a decreasing trend after 2010.
Table 1 presents the technological classifications of patents from 2000 to 2019 with percentages greater than 1% for all IPCs. The highest percentage classification is the F24J IPC, which ranks highest among all classifications, followed by H01L, F03G, F24D, etc. These classifications mainly focus on the fields of heating, power generation, semiconductor devices, and machines or engines. The total occurrence of these 15 classifications accounts for nearly 75.46% of all classifications, indicating that researchers rely heavily on these dominant classifications in the field and implying an uneven distribution of classifications in the solar energy domain.
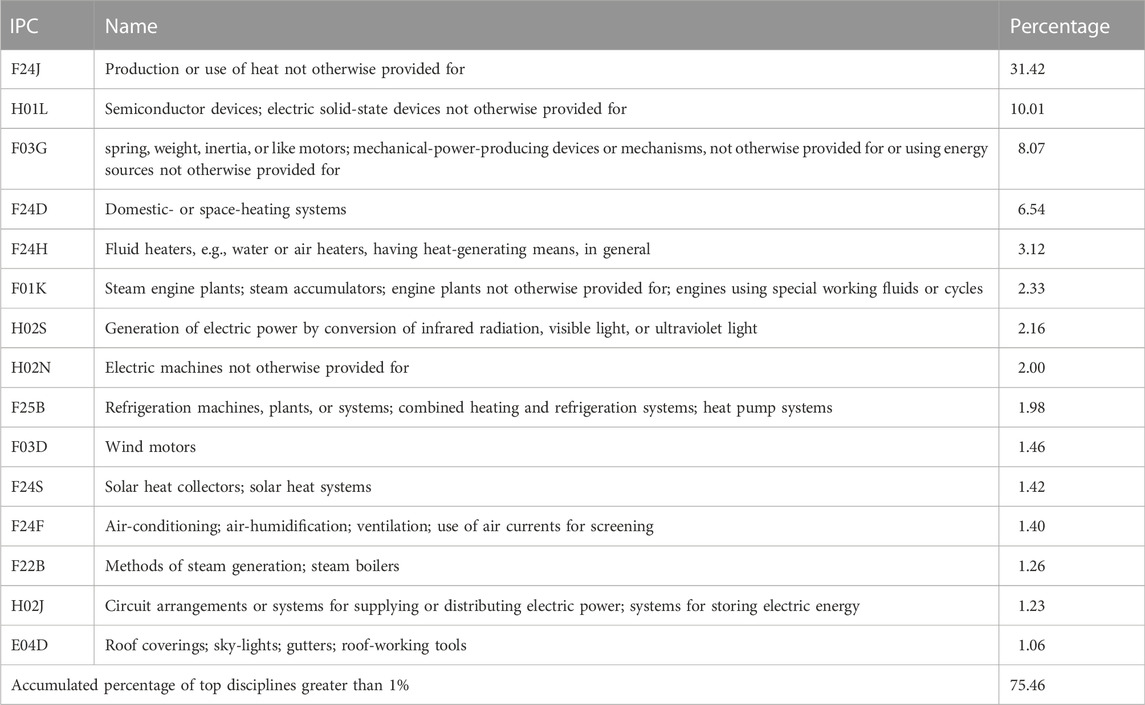
TABLE 1. Distribution and ranking of technological classifications with percentages greater than 1%.
4.2 The convergence process for technological fields
To trace the evolution of technological convergence, we use Cortext (cortext.net) to generate an evolution map for disciplines in the domain. This map aids in visualizing the details and components of relationships based on flow diagrams (also called “Sankey”) of classification relations (Rosvall and Bergstro, 2010). Cortext is used to apply the cosine similarity and Louvain algorithm (Blondel et al., 2008) for the decomposition. If one technological category (shown as bars in Figure 4) in a year is present in the following year, the flow of this category in the network will be represented as a belt linking the 2 bars (Leydesdorff and Goldstone, 2014).
From 2000 to 2009, the complexity of the domain slightly increases—the number of communities increases from 7 to 8. The dominant relations of each community in each period are shown in Figure 5. Some of the relationships lack continuity and only exist for several years. It is worth noting that from 2011 to 2019, the number of communities decreased to 5. In addition, the map reveals that the convergence of IPC codes mainly occurs within the F0 category. For example, F03G & F03D, F01K & F03G, and F02C & F22G account for a large proportion of the different classification combinations. The F24J & F2D, H01G & H01L combinations have been the more prominent convergence relations in the recent period.
4.3 Radical innovations in the recent period
4.3.1 Radical innovative patent identification based on the analysis of new convergent relations
To detect new IPC relations (at the four-digit level) during the most recent period (2017–2019), we also downloaded all the related patents published from 1973 to 1999. Finally, among the total of 1,105 patents published from 2017 to 2019, 101 patents with 216 new relationships that had not previously appeared in the solar energy domain are identified in this study. These relationships indicate new knowledge recombination in the solar energy domain; thus, the patents containing these relationships are considered to have higher possibilities of being radical innovations. Table 2 lists the top_10 new relations that appear in recent patents. Radical innovations may be identified from these new combinations of knowledge from different fields. Using F24T, F24F, F24S, and G07F in new relation combinations seems to be popular. They are related to geothermal system, air conditioning, coin-free apparatus technologies. These new convergent technologies, which indicate the radical innovations in this domain, include the convergence of heating and geothermal systems, air conditioning and geothermal systems and horticulture and solar heat collectors. More detailed technological information is studied by utilizing semantic analysis based on the titles and abstracts of related patents (Section 4.3.2).
4.3.2 Identification of radical technologies based on scientific citations
In the total of 10,995 patents, there are 484 patents have scientific citations. The detailed information on the top 10 patents with more scientific citations is shown in Table 3. Besides, in the 14 patents that have scientific citations and published in 2017–2019, there is only one patent has new combinations of technological fields, the result shows that the two approaches are complementary since some strong scientific knowledge-related patents did not contain the new convergence relations.
To detect more detailed information, the topic LDA model is applied to the titles and abstracts of the 101 patents containing new knowledge convergence and the top 30 strong scientific knowledge-related patents (130 patents in total after removing duplicates). We set the number of topics based on the coherence value (Son and Cho, 2020). Finally, the patent documents are classified into four groups. Then, we use the term extraction tool in Cortext (cortext.net) to extract the top occurring terms. The topics can be summarized as solar PV, heat storage, heat exchangers, and solar collectors according to the terms (Table 4).
The recent radical innovations are mainly related to these four topics. First, in the topic of solar PV, radical innovations are focused on the solar cell structure and materials, which are related to the insulator layer, solar panel and PV modules. The enhanced structure is utilized to improve the solar heat energy utilization. Patent WO2019064218 designed a solar panel attachable-type hot water boiler that can increase the heat area of the waiting line and solar energy. Thin film technology has been widely used in radical innovations. For example, patent US2018209162 used the thin film in a heat sink system for collecting solar energy from sunlight in, e.g., agriculture applications. The porous structure also has been widely used in solar PV technology. In patent WO2017147463, an aerogel material comprising silica aerogel and a porous material with an average radius of less than or equal to 5 nm with a standard deviation of 3 nm were presented. This patent could be implemented to achieve high receiver efficiency at low cost. Besides, patent WO2017130224 provided an intelligent inline connector device for connecting photovoltaic modules of a solar power generation system, with a wireless communication module placed within the connector part.
In the topic of heat storage, radical innovative technologies are mainly related to thermal storage tanks, cleaning system-based geothermal energy, solar energy generating devices, absorbent films, aluminium solar panels, etc. In addition, the intelligent control system and hydroponics solar products are also possible radical innovations in this subdomain. Many patents are related to the thermal storage tank structure and aim at prevent the overheating of the storage tank. For example, patent JP2019199979 designs a road-heating layer on the outdoors adjacent to the building. Besides, patent CN206785572 and CN108375216 are solar energy and geothermal energy combined energy system, which fully utilizes geothermal energy, solar energy and other energy, effectively improves power generation efficiency, and makes full use of abundant natural resources. Patent CN107166747, CN106969512, and CN107289633 are related to the intelligent systems. For example, patent CN107166747 designed an intelligent solar water heater that has control circuit provided with main control board, power supply board and motor, and water temperature regulator formed with mixing cavity inside.
The heat exchanger topic includes remote control, semiconductor, condenser and sensor radical innovations. Waste heat utilization and cross-season multienergy complementary technologies are also emerging as radical innovations. Patent CN106802075 proposes a computer remote controlled drying solar heat collecting device that using distributed node network and can effectively reduce energy consumption and transmits fast signal transmission. Patent WO2017121830 is a solution of tungstate ions used to form semiconductor layer for photovoltaic devices, which has a dynamic viscosity of 2–64 cP, preferably 8–19 cP, at 25°C and atmospheric pressure of 101–325 Pa. It is stable over time, homogenous and compatible with printing techniques. And it can be prepared by simple and easy process without utilizing chemical pollutants or toxic compounds. Patent CN209386466 claims a energy complementary system cross-season solar and ground source heat pump, which combines the solar thermal technology, shallow ground temperature energy ground source heat pump technology and soil heat technology effectively fused together, which can ensure the stability and safety of the ground source heat pump system, and also promotes the development of the solar heating technology.
The solar collector topic mainly includes the radical innovations related to the PVT module that is designed as an organic solar cell, the power collector and the valve arrangement and spiral channel for the pump system. More specifically, the photovoltaic (PVT) module described in WO2017108984 could be used as a facade element, air collector, solar dryer, or domestic hot water module for use in insulated glazing, which enables space-saving use of both technologies and provides improved properties. The organic solar cell is operated reliably and at a high efficiency even at higher temperatures since the efficiency does not decrease at relatively high temperatures or decreases only to a lesser extent compared to silicon cells. In patent CN108661719, an in-cylinder engine formed with a spiral channel connected with a water pump impeller for driving the rotation of a water pump impeller and a lighting mechanism for collecting sunlight heat energy through a cylinder wall were proposed. In patent WO2017198777, a transparent conducting film for semiconductor devices was described. This film, which is based on nominally undoped ZnO, has enhanced environmental stability. The manufacturing method can be used as a relatively simple setup, especially if the plasma close to the substrate is generated by RF biasing, and maintenance and operation costs can be reduced in comparison with processes that use inductively coupled plasma to make the ZnO deposit conductive.
4.4 Potential radical technological topics
Potential radical innovation identification in the study is based on the analysis of convergence relationships. These include relationships that did not exist in this domain in 2017–2019 but have a high possibility of appearing in the future. The RA algorithm is applied in this study, and the AUC is applied to test the validity. In this study, the AUC value of RA is .96, which indicates that the RA algorithm is effective in estimating potential relationships.
In this study, the top five relationships with high RA values are viewed as potential convergence relationships and are shown in Table 5. The meanings of the IPC codes are listed in Table 6. F01K-F24D is the top potential convergence relationship in terms of RA value, meaning that patents containing knowledge from “F01K and F24D” have a notably high possibility of appearing in the future. In addition, two of the top five convergence links contain F24D, indicating that this discipline is the most popular in the convergence network with the most convergence partners.
To further understand the potential convergence relationships and offer more detailed information on the potential radical topics, the patents that contain the five potential convergence relationships are collected and analysed. It is assumed that the topics of the potential convergence relationship are similar to those that the relationship covers in previous patents.
There is one patent containing F01K and F24D in 2016, and it is related to an automatic plant for producing electric energy. Thus, we consider that the potential convergence of F01K and F24D might also be related to this topic. There were two patents in 2016 containing F24D and E04H; the potential radical topic might be related to the building integrated photovoltaic system. The potential convergence of F21V and H01L might be associated with the topic of a solar concentrator apparatus. The potential convergence of F24H and B01D might be related to the system of supplying drinking water and electricity. The potential convergence of F23L and F24S might address a solar energy photothermal conversion heating furnace. Specially, in the research report on innovative solar technologies by APEC Energy Working Group (2018), the authors stressed that converting solar radiation into heat is the most simple and direct application of solar energy, with greater potential than other forms of renewable sources. While the building integrated photovoltaic system was regarded to “pose an opportunity to play an essential part in a new era of distributed power generation”. And they claimed that the technologies of aesthetic designs and flexible thin film solar PV module should be taken together into mitigate the barriers posed for current building integrated photovoltaic applications. Besides, the concentrator, which is one of the core parts of solar collector, was regarded to be important to the use efficiency of solar energy. The authoritative results in the report support the effectiveness of our predicting results.
5 Discussion
This study explores the radical technologies in the solar energy domain. Based on the combination of a technological convergence study and scientific relationship analysis, we investigate radical innovation using global patent data and reveal more details about radical innovative topics and potential topics in this domain.
5.1 The evolution of technological convergence relations
Based on the network structure analysis results, the average degree of the convergence networks demonstrates an increasing trend, indicating that more technological categories of this domain tend to link with each other. Meanwhile, the decreasing centrality indicators reveal that the network may be dominated by various groups of categories rather than a single category. The increasing cohesion indicators demonstrate the growing trend of convergence among various technological categories. These results show that solar energy technology has the characteristics of evolving trends running in a parallel manner between technological convergence and divergence, which are consistent with the conclusions by Son and Cho (2020), thus proving the validity of our research.
According to the intuitive visualization at the 3-digit IPC level, the technological categories are likely to link with those from neighbouring categories. At the same time, few relationships exist among distinct categories and often last for a short period. This may be because although technical diversity could breed disruptive innovation; it is also hazardous. Meanwhile, the dominant relations between adjacent categories are more stable. For example, the continuity of F24D-F24F and F03G-F03D lasted for almost the whole 20 years. This result reminds that researchers and engineers in the solar energy domain should collaborate with practitioners in adjacent categories.
5.2 Radical innovative technologies
The patents that contain new technological convergence relations and more scientific citations are regarded as having high possibilities to be radical innovations in this research. Radical technologies are related to solar PV, heat storage, heat exchangers and solar collectors. The detailed technologies are analysed by using semantic analysis.
The solar cell is the core technology of the solar power generation system and is also the most essential part. How to improve the storage efficiency and photoelectric conversion efficiency are the hotspots in the field. From the empirical evidence, carbon-based batteries are currently widely researched. This is due to the hydrophobic and heat resistance stability of the carbon material, which can significantly enhance battery performance (Wei et al., 2018). The ZnO transparent conducting film is regarded as the most advanced flexible transparent conductive thin film material; it has many advantages, such as abundant sources, a friendly environment, easy processing, and low cost (Song et al., 2014). In addition, array substrates, photovoltaic modules, and solar panels are recent radical topics.
Due to the seasonality and timeliness of solar energy, heat storage and exchanger technologies have been core themes in solar energy studies for a long time. Our result shows that the combination of solar energy with other renewable energy has become a hot spot, which is in accordance with a previous study (Chong et al., 2011; Guler et al., 2022). For instance, a hybrid system can provide better grid utilization due to different peak intervals of wind and solar radiation. Radical innovations are also related to the combination of solar and geothermal energy. In addition, intelligent systems are current hotspots.
In the solar collector topic, PVT technology is a new type of solar energy utilization technology that combines photovoltaic power generation and solar thermal utilization; its energy efficiency is significantly higher than that of traditional collectors (Shi, 2018). The solar power tower (SPT) has many advantages, such as large-scale and high thermal cycling efficiency, and has been regarded as the most promising technology (García et al., 2014). In addition, radical innovations are related to the valve arrangement and spiral channel for the pump system.
In the recent years, many researchers have proposed various solutions related to the aforementioned areas to improve the solar conversion efficiency and down the cost. For example, in the solar cell technosphere, Khurshid et al. (2022) have proposed the quantum dots ensitized ZnO nano-rods array solar cell, which could achieve high power conversion efficiency. And the perovskites cell have been explored as one of the most promising technologies (Sun et al., 2022). Besides, ultra-thin film solar cell, nanoparticles structured organic solar cells, and so on have been the focal points. Many researchers have paid attentions on multi-generation energy system driven by integrated renewable energy sources. Calise et al. (2016) designed a hybrid solar-geothermal plant for multi tasks; Das and Singh (2022) integrated a doubly-fed induction generator to a solar photovoltaic array for wind-solar hybrid power generation. In the solar collector topic, Adun et al. (2022) simulated the performance of the PVT system into residential settlement, and confirmed the potential benefits of this technology. In a word, both the researchers and engineers in the solar energy domain need to pay more attentions on these radical topics.
Based on the detailed information of radical innovative patents, solar energy is mainly applied to water heating, air conditioning and mobile charging. The research related to heating water is mainly about the water tank material, solar pipeline and control unit, which are the core technologies to heat water. Solar air conditioner technology has attracted global attention, and many researchers have focused on improving the utilization rate of solar energy and reducing energy consumption. In addition, portable solar chargers have attracted extensive attention.
5.3 Potential relations and topics
According to the link prediction algorithm, five relationships with high RA values are identified as potential relationships: F01K-F24D, F24D-E04H, F21V-H01L, F24H-B01D and F23L-F24S.
The potential relationship between F01K and F24D might be related to the topic of automatic plants for producing electric energy (Giuseppe, 2016). This is because solar energy has the characteristics of seasonal and regional diversity, and the light intensity could show significant differences at different times of the day in the same season. Automatic plants related to concentrating solar power technology with thermal energy storage can be used to overcome the intermittent and unstable nature of solar energy and reduce costs. The relation between F24D and E04H might be related to a solar energy ecology house (Li et al., 2015). The organic integration of solar energy and buildings has great potential in the future (Chen and Alateeq, 2021). For instance, a solar energy collector could be used to cover the rooftop of a building or as a substitute for the rooftop layer, thus achieving the holistic aesthetics of the building, reducing unnecessary extravagance and lowering costs.
The potential convergence of F21V and H01L might be related to a solar concentrator apparatus (Hebrink et al., 2010). The concentrator is significant for efficiently utilizing solar energy. Its unstable and intermittent nature makes the efficient use of solar energy difficult, and there is a great need to reduce the cost of power generation; light from the Sun needs to be concentrated in a smaller area. Many methods have been designed to achieve this goal (Bartłomie et al., 2021). The potential convergence of F24H and B01D might address the application of solar energy, which might be closely related to the system of supplying drinking water and electricity (Wang et al., 2012). This is also true for F23L and F24S, which might be related to the heating furnace (Cao et al., 2014).
6 Conclusion and policy implications
The development of solar energy technology is of great significance for the sustainable development of human society. This paper studies radical innovation in the solar technological field based on a new framework by using patent literature. It provides more details about radical innovative topics and potential innovative topics in this domain.
The findings have implications for scientists, policymakers, and investors in this domain. For example, the potential convergence relationships could offer references for researchers seeking technical cooperation since technological convergence could promote innovation and cross the technical barrier in the domain. For methodological implications, this work combines a technological convergence analysis and scientific relationship study, which could present some critical findings from being lost by comparing the results with previous studies using only a single approach. In addition, text mining and link prediction are applied to identify radical innovative topics and potential topics in the domain. Thus, the detailed information could be detected further.
Despite its contributions, several limitations also exist in this study. First, the solar energy domain is studied as a whole, while this domain consists of several subdomains, such as heat storage and photoelectric conversion. These subdomains are significantly different from each other; thus, further division of the solar energy domain into several parts could allow for more detailed research about technological convergence. Second, the technological convergence study is mainly dependent on the classification method of technology. While the technological categories vary in different databases, other classification methods are also used in other works (Jaffe and Trajtenberg, 1999; Han and Magee, 2018), which may lead to different convergence trend results. In addition, due to the few scientific citations of patents in the solar energy domain, the scientific relation study is only related to the variety of citations. The relationships between science and radical innovations could be delved into deeply by adding other technological domains. For example, diversity indicators, such as the indicator proposed by Rao-Stirling et al., 2007, could be utilized to explore the relations between scientific categories and radical innovations in the future. Stevens et al., 2012.
Data availability statement
The original contributions presented in the study are included in the article/Supplementary Material, further inquiries can be directed to the corresponding author.
Author contributions
SF: Data curation; Methodology; Software; Funding acquisition; Writing-original draft. FH: Supervision; Methodology; Visualization; Funding acquisition; Writing-original draft.
Funding
This research is supported by grants from the National Natural Science Foundation of China (Grant No.42101176); Xi’an Guokehulian Innovation and Development Institute Limited (Grant No. E1491403).
Conflict of interest
The authors declare that the research was conducted in the absence of any commercial or financial relationships that could be construed as a potential conflict of interest.
Publisher’s note
All claims expressed in this article are solely those of the authors and do not necessarily represent those of their affiliated organizations, or those of the publisher, the editors and the reviewers. Any product that may be evaluated in this article, or claim that may be made by its manufacturer, is not guaranteed or endorsed by the publisher.
Supplementary material
The Supplementary Material for this article can be found online at: https://www.frontiersin.org/articles/10.3389/fenrg.2022.1056564/full#supplementary-material
References
Adamic, L. A., and Adar, E. (2003). Friends and neighbors on the web. Soc. Netw. 25 (3), 211–230. doi:10.1016/s0378-8733(03)00009-1
Adun, H., Ishaku, H. P., Titus, O. A., and Shefik, A. (2022). 3-E feasibility analysis on photovoltaic/thermal application for residential buildings: A case study of sub-saharan africa. Energy Sources Part A-Recovery Util. Environ. Eff. 44 (4), 9901–9919. doi:10.1080/15567036.2022.2143962
Almutairi, K., Mostafaeipour, A., Baghaei, N., Techato, K., Chowdhury, S., Jahangiri, M., et al. (2021). Techno-Economic investigation of using solar energy for heating swimming pools in buildings and producing hydrogen: A case study. Front. Energy Res. 9, 680103. doi:10.3389/fenrg.2021.680103
Apec Energy Working Group, (2018). Research report on innovative solar technologies. Tokyo, Japan: APEC Energy Working Group.
Arsalan, M. S., Abdul, H., Mustapha, M., Musznicki, P., Iqbal, A., and Czapp, S. (2022). Solar photovoltaic energy optimization and challenges. Front. Energy Res. 10, 879985. doi:10.3389/fenrg.2022.879985
Arts, S., Appio, F. P., and Looy, B. V. (2013). Inventions shaping technological trajectories: Do existing patent indicators provide a comprehensive picture?. Scientometrics 97 (3), 397–419. doi:10.1007/s11192-013-1045-1
Bartłomiej, M., Magdalena, B., and Krzysztof, P. (2021). Influence of solar concentrator in the form of luminescent PMMA on the performance of a silicon cell. Sustainbility 13 (4), 2086–2086. doi:10.3390/su13042086
Blondel, V. D., Guillaume, J. L., Lambiotte, R., and Lefebvre, E. (2008). Fast unfolding of communities in large networks. J. Stat. Mech. Theory Exp. 8 (10), 10008. doi:10.1088/1742-5468/2008/10/p10008
Calise, F., d'Accadia, M. D., Macaluso, A., Piacentino, A., and Vanoli, L. (2016). Exergetic and exergoeconomic analysis of a novel hybrid solar-geothermal polygeneration system producing energy and water. Energy Convers. Manag. 115, 200–220. doi:10.1016/j.enconman.2016.02.029
Cao, J. F., Di, J. J., Ren, H. T., Zhang, X., Song, Y., and Wang, Y. (2014). Solar energy photo-thermal converting heating furnace has production medium pipeline that is connected with first heat exchanger to make production medium and heat conduction oil heat transfer. Bihaozhou Beijing Energy-Saving & Environment, Beijing, China,
Cao, R. M., Liu, S. Y., and Xu, X. K. (2019). Network embedding for link prediction: The pitfall and improvement. Chaos 29, 103102. doi:10.1063/1.5120724
Chen, A., and Alateeq, A. (2021). Performance of solar cells integrated with rigid and flexible building substrates under compression. J. Build. Eng. 34, 101938. doi:10.1016/j.jobe.2020.101938
Chong, W. T., Naghavi, M. S., Poh, S. C., Mahlia, T., and Pan, K. (2011). Techno-economic analysis of a wind-solar hybrid renewable energy system with rainwater collection feature for urban high-rise application. Appl. Energy 88 (11), 4067–4077. doi:10.1016/j.apenergy.2011.04.042
Dahlin, K. B., and Behrens, D. M. (2005). When is an invention really radical? Defining and measuring technological radicalness. Res. Policy 34 (5), 717–737. doi:10.1016/j.respol.2005.03.009
Das, S., and Singh, B. (2022). Normalized maximum correntropy criterion based ripple mitigation strategy for wind-solar hybrid generation system under nonideal grid conditions. IEEE Trans. Power Electron. 38 (1), 956–967. doi:10.1109/tpel.2022.3199387
Faria, L. G. D., and Andersen, M. M. (2017). Sectoral dynamics and technological convergence: An evolutionary analysis of eco-innovation in the automotive sector. Industry Innovation 24, 837–857. doi:10.1080/13662716.2017.1319801
Feng, S. D., An, H. Z., Li, H. J., Qi, Y., Wang, Z., Guan, Q., et al. (2020). The technology convergence of electric vehicles: Exploring promising and potential technology convergence relationships and topics. J. Clean. Prod. 260, 120992. doi:10.1016/j.jclepro.2020.120992
Fleming, L. (2001). Recombinant uncertainty in technological search. Manag. Sci. 47 (1), 117–132. doi:10.1287/mnsc.47.1.117.10671
Gao, X. (2022). International patent inflows and innovative capacity in recipient countries: Evidence from the solar photovoltaics (PV) industry. Energy Sustain. Dev. 68, 449–456. doi:10.1016/j.esd.2022.05.002
Gao, X., and Zhang, Y. (2022). What is behind the globalization of technology? Exploring the interplay of multi-level drivers of international patent extension in the solar photovoltaic industry. Renew. Sustain. Energy Rev. 163, 112510. doi:10.1016/j.rser.2022.112510
García-Barberena, J., Monreal, A., Mutuberria, A., and Sanchez, M. (2014). Towards cost-competitive solar towers-energy cost reductions based on decoupled solar combined cycles (DSCC). Energy Procedia 49, 1350–1360. doi:10.1016/j.egypro.2014.03.144
Giuseppe, M. (2016). “Automatic plant for producing electric energy, has thermostatic actuator that activates fuel-type auxiliary system to preset value and to deactivate it when pressure in tank reaches maximum value,”. WO2016147210.Marocco Giuseppe, Cristaldi Angelo, Benvenuti Marilena
Guler, O. F., Sen, O., Yilmaz, C., and Kanoglu, M. (2022). Performance evaluation of a geothermal and solar-based multigeneration system and comparison with alternative case studies: Energy, exergy, and exergoeconomic aspects. Renew. Energy 200, 1517–1532. doi:10.1016/j.renene.2022.10.064
Haas, M. R., and Ham, W. (2015). Microfoundations of knowledge recombination: Peripheral knowledge and breakthrough innovation in teams. Adv. Strategic Manag. 32, 47–87. doi:10.1108/S0742-332220150000032002
Han, F., and Magee, C. L. (2018). Testing the science/technology relationship by analysis of patent citations of scientific papers after decomposition of both science and technology. Scientometrics 116 (2), 767–796. doi:10.1007/s11192-018-2774-y
Hargadon, A. (2003). How breakthroughs happen: The surprising truth about how companies innovate. Massachusetts, MA, USA: Harvard Business Press.
Hebrink, T. J., Clear, S. C., Gilbert, L. R., Weber, M. F., and Yu, T. H. (2010). Broadband reflectors, concentrated solar power systems, and methods of using the same. Minnesota, MN, USA: 3M Innovative Properties Company.
Huang, M. H., Yang, H. W., and Chen, D. Z. (2015). Increasing science and technology linkage in fuel cells: A cross citation analysis of papers and patents. J. Inf. 9 (2), 237–249. doi:10.1016/j.joi.2015.02.001
Isella, L., Stehlé, J., Barrat, A., Cattuto, C., Pinton, J. F., and Van den Broeck, W. (2011). What’s in a crowd? Analysis of face-to-face behavioral networks. J. Theor. Biol. 271, 166–180. doi:10.1016/j.jtbi.2010.11.033
Jaccard, P. (1901). étude comparative de la distribution florale dans une portion des Jura. Bull. Soc. Vaudoise Sci. Nat. 37, 547–547. doi:10.5169/seals-266450
Jaffe, A., and Trajtenberg, M. (1999). International knowledge flows: Evidence from patent citations. Econ. Innovation New Technol. 8 (1-2), 105–136. doi:10.1080/10438599900000006
Johnstone, N., Hascic, I., and Popp, D. (2010). Renewable energy policies and technological innovation: Evidence based on patent counts. Environ. Resour. Econ. 45 (1), 133–155. doi:10.1007/s10640-009-9309-1
Kangas, H. L., Ollikka, K., Ahola, J., and Kim, Y. (2021). Digitalisation in wind and solar power technologies. Renew. Sustain. Energy Rev. 150, 111356. doi:10.1016/j.rser.2021.111356
Khurshid, S., Latif, H., Rasheed, S., Sharif, R., Sattar, A., and Amjad, R. J. (2022). Enhancement in absorption spectrum by ITO coated, down converting glass as a photoanode substrate for efficient PbS/CdS quantum dots sensitized ZnO nano-rods array solar cell. Opt. Mater. 124, 111991. doi:10.1016/j.optmat.2022.111991
Kim, K. S., and Cho, N. W. “Predicting the patterns of technology convergence in defense technologies,” in Proceedings of the IEEE International Conference on Big Data and Smart Computing (BigComp), Daegu, Korea, January 2022, 72–75. doi:10.1109/BigComp54360.2022.00024
Ko, N., Yoon, J., and Seo, W. (2014). Analyzing interdisciplinarity of technology fusion using knowledge flows of patents. Expert Syst. Appl. 41, 1955–1963. doi:10.1016/j.eswa.2013.08.091
Kong, D. J., Yang, J. Z., and Li, L. F. (2020). Early identification of technological convergence in numerical control machine tool: A deep learning approach. Scientometrics 125 (3), 1983–2009. doi:10.1007/s11192-020-03696-y
Lai, K. K., Bhatt, P. C., Kumar, V., Chen, H. C., Chang, Y. H., and Su, F. P. (2021). Identifying the impact of patent family on the patent trajectory: A case of thin film solar cells technological trajectories. J. Inf. (2), 15. doi:10.1016/j.joi.2021.101143
Lee, S., Hwang, J., and Cho, E. S. (2022). Comparing technology convergence of artificial intelligence on the industrial sectors: Two-way approaches on network analysis and clustering analysis. Scientometrics 127 (1), 407–452. doi:10.1007/s11192-021-04170-z
Leydesdorff, L., and Goldstone, R. (2014). Interdisciplinarity at the journal and specialty level: The changing knowledge bases of the journal cognitive science. J. Assoc. Inf. Sci. Technol. 65 (1), 164–177. doi:10.1002/asi.22953
Li, D. Y., Heimeriks, G., and Alkemade, F. (2020). Recombinant invention in solar photovoltaic technology: Can geographical proximity bridge technological distance?. Reg. Stud. 55 (4), 605–616. doi:10.1080/00343404.2020.1839639
Li, F. M., Liu, H. J., Liu, S. Z., Li, J. P., Zheng, J., Feng, R., et al. (2015). Ecosystem for rural housing comprises ecological farmhouse, solar hot water system, solar photovoltaic panels, biogas room and kitchen, and the ecological farmhouse comprises indoor heating system and power distribution system. Beijing, China: Beijing SuThink InC.
Luan, C. J., Sun, X. M., and Wang, Y. L. (2021). Driving forces of solar energy technology innovation and evolution. J. Clean. Prod. 287, 125019. doi:10.1016/j.jclepro.2020.125019
Ma, N., Shum, W. Y., Han, T. T., and Lai, F. (2021). Can machine learning be applied to carbon emissions analysis: An application to the CO2 emissions analysis using Gaussian process regression. Front. Energy Res. 9, 756311. doi:10.3389/fenrg.2021.756311
Martinez, V., Berzal, F., and Cubero, J. C. (2017). A survey of link prediction in complex networks. ACM Comput. Surv. 49 (4), 1–33. doi:10.1145/3012704
Narin, F., Hamilton, K. S., and Olivastro, D. (1997). The increasing linkage between U.S. technology and public science. Res. Policy 26 (3), 317–330. doi:10.1016/s0048-7333(97)00013-9
Navothna, B., and Thotakura, S. (2022). Analysis on large-scale solar PV plant energy performance–loss–degradation in coastal climates of India. Front. Energy Res. 10, 857948. doi:10.3389/fenrg.2022.857948
Negroponte, N. (2003). Creating a culture of ideas: What sparks the ideas that beget new technologies? The cofounder of MIT's media lab says celebrating wrong answers and listening to youth make a good start. (Emerging technologies). Technol. Rev. 106 (1), 34–35.
Park, I., and Yoon, B. (2018). Technological opportunity discovery for technological convergence based on the prediction of technology knowledge flow in a citation network. J. Inf. 12, 1199–1222. doi:10.1016/j.joi.2018.09.007
Rampa, R. (2021). Developing radical innovation capabilities: Exploring the effects of training employees for creativity and innovation. Creat. Innov. Proj. Manag. 30 (1), 211–227. doi:10.1111/caim.12423
Rosvall, M., and Bergstrom, C. T. (2010). Mapping change in large networks. PLoS One 5 (1), e8694. doi:10.1371/journal.pone.0008694
Sam, A., and Reinhilde, V. (2013). “The technological origins and novelty of breakthrough inventions,” in 35th DRUID Celebration Conference, 2013, Barcelona, Spain, June 17–19.
Schoenmakers, W., and Duysters, G. (2010). The technological origins of radical inventions. Res. Policy 39 (8), 1051–1059. doi:10.1016/j.respol.2010.05.013
Shi, N. B. (2018). Performance analysis of solar absorption refregeration system based on PVT. Fujian, China: Fuzhou University.
Son, S., and Cho, N. W. (2020). Technology fusion characteristics in the solar photovoltaic industry of South Korea: A patent network analysis using ipc co-occurrence. Sustainability 12, 9084. doi:10.3390/su12219084
Song, M., Park, J. H., Kim, C. S., Kim, D. H., Kang, Y. C., Jin, S. H., et al. (2014). Highly flexible and transparent conducting silver nanowire/ZnO composite film for organic solar cells. Nano Res. 7 (9), 1370–1379. doi:10.1007/s12274-014-0502-3
Soomar, A. M., Hakeem, A., Messaoudi, M., Musznicki, P., Iqbal, A., and Czapp, S. (2022). Solar photovoltaic energy optimization and challenges. Front. Energy Res. 10, 879985. doi:10.3389/fenrg.2022.879985
Stevens, K., Kegelmeyer, P., Andrzejewski, D., and Buttler, D. (2012). “Exploring topic coherence over many models and many topics,” in Proceedings of the 2012 Joint Conference on Empirical Methods in Natural Language Processing and Computational Natural Language Learning, Jeju Island, Korea, July 2012, 952–961.
Stirling, A. (2007). A general framework for analysing diversity in science, technology and society. J. R. Soc. Interface 4 (15), 707–719. doi:10.1098/rsif.2007.0213
Sun, X. L., Zhu, Z. L., and Li, Z. A. (2022). Recent advances in developing high-performance organic hole transporting materials for inverted perovskite solar cells. Front. Optoelectron. 15 (1), 46. doi:10.1007/s12200-022-00050-3
Sung, H. Y., Wang, C. C., Huang, M. H., and Chen, D. Z. (2015). Measuring science-based science linkage and non-science-based linkage of patents through non-patent references. J. Inf. 9 (3), 488–498. doi:10.1016/j.joi.2015.04.004
Tortoriello, M., McEvily, B., and Krackhardt, D. (2014). Being a catalyst of innovation: The role of knowledge diversity and network closure. Organ. Sci. 10 (4), 1–16. doi:10.1287/orsc.2014.0942
Trancik, J., Brown, P., Jean, J., Kavlak, G., and Needell, Z. (2015). Technology improvement and emissions reductions as mutually reinforcing efforts: Observations from the global development of solar and wind energy (Technical Report). Massachusetts, MA, USA: MIT.
Trappey, A. J. C., Trappey, C. V., Chang, A. C., and Li, J. X. (2019). Deriving competitive foresight using an ontology-based patent roadmap and valuation analysis. Int. J. Semantic Web Inf. Syst. (IJSWIS) 15 (2), 68–91. doi:10.4018/ijswis.2019040104
Van, E. N. J., and Waltman, L. (2010). Software survey: VOSviewer, a computer program for bibliometric mapping. Scientometrics 84 (2), 523–538. doi:10.1007/s11192-009-0146-3
Wang, M., Yau, W., and Chen, M. (2012). Disaster relief system of supplying drinking water and electricity, has manual generator that is used to resupply in case of solar generator shortage. Taichung, Taiwan: Univ Nat Chin Yi Technology.
Wang, Y. W., Urban, F., Zhou, Y., and Chen, L. (2018). Comparing the technology trajectories of solar pv and solar water heaters in China: Using a patent lens. Sustainability 10, 4166. doi:10.3390/su10114166
Wang, Z. N., Porter, A. L., Wang, X. F., and Carley, S. (2019). An approach to identify emergent topics of technological convergence: A case study for 3D printing. Technol. Forecast. Soc. Change 146, 723–732. doi:10.1016/j.techfore.2018.12.015
Wei, J., Chen, W., Chen, D. M., and Yang, K. (2018). An amorphous carbongraphite composite cathode for long cycle life rechargeable aluminum ion batteries. J. Mater. Sci. Technol. 34 (6), 983–989. doi:10.1016/j.jmst.2017.06.012
Yuan, X. D., Song, W. L., Zhang, C. X., and Yuan, Y. (2022). Understanding the evolution of photovoltaic value chain from a global perspective: Based on the patent analysis. J. Clean. Prod. 377, 134466. doi:10.1016/j.jclepro.2022.134466
Yun, J., and Geum, Y. (2019). Analysing the dynamics of technological convergence using a co-classification approach: A case of healthcare services. Technol. Analysis Strategic Manag. 31 (12), 1412–1429. doi:10.1080/09537325.2019.1616082
Yun, S. Y., Lee, J., and Lee, S. (2019). Technology development strategies and policy support for the solar energy industry under technological turbulence. Energy Policy 124, 206–214. doi:10.1016/j.enpol.2018.09.003
Zakari, Y., Vuille, F., and Lehning, M. (2022). Climate change impact assessment for future wind and solar energy installations in India. Front. Energy Res. 10, 859321. doi:10.3389/fenrg.2022.859321
Zhang, Y. T., Tsai, C. H., and Chung, C. C. (2021). The transitions of technological innovation systems in the transnational context: The example of China's solar photovoltaic industry (1970s-2010s). Technol. Analysis Strategic Manag. 33 (12), 1467–1483. doi:10.1080/09537325.2021.1879380
Zhong, B. T., Hei, Y. J., Li, L., Luo, H. B., and Tang, J. Q. (2019). Technology frontiers of building-integrated photovoltaics (bipv): A patent co-citation analysis. Int. J. Low-Carbon Technol. 2, 1–12. doi:10.1093/ijlct/ctz068
Zhou, T., Lu, L. Y., and Zhang, Y. C. (2009). Predicting missing links via local information. Eur. Phys. J. B 71 (4), 623–630. doi:10.1140/epjb/e2009-00335-8
Keywords: solar energy, technology convergence, scientific citation, link prediction, radical innovation
Citation: Feng S and Han F (2023) Radical innovation detection in the solar energy domain based on patent analysis. Front. Energy Res. 10:1056564. doi: 10.3389/fenrg.2022.1056564
Received: 29 September 2022; Accepted: 12 December 2022;
Published: 06 January 2023.
Edited by:
Fouzi Harrou, King Abdullah University of Science and Technology, Saudi ArabiaReviewed by:
Seyyed Jalaladdin Hosseini Dehshiri, Allameh Tabataba’i University, IranRamalingam Senthil, SRM Institute of Science and Technology, India
Copyright © 2023 Feng and Han. This is an open-access article distributed under the terms of the Creative Commons Attribution License (CC BY). The use, distribution or reproduction in other forums is permitted, provided the original author(s) and the copyright owner(s) are credited and that the original publication in this journal is cited, in accordance with accepted academic practice. No use, distribution or reproduction is permitted which does not comply with these terms.
*Correspondence: Fang Han, aGFuZmFuZ0BtYWlsLmxhcy5hYy5jbg==