Negative emissions at negative cost-an opportunity for a scalable niche
- 1Climate Institute TU Delft, Delft, Netherlands
- 2Department of Water Management, TU Delft, Delft, Netherlands
- 3Energy and Sustainability Research Institute Groningen, Groningen University, Groningen, Netherlands
- 4Centre of Excellence in Systems, Energy and Environment, APJ Abdul Kalam Technological University, Kannur, Kerala, India
- 5M.S. Swaminathan Research Foundation, Chennai, India
- 6Sardar Swaran Singh National Institute of Bio-Energy (SSS-NIBE), Kapurthala, India
- 7Groningen Institute for Evolutionary Life Sciences, Groningen University, Groningen, Netherlands
- 8Department of Materials Science and Engineering, KTH Royal Institute of Technology, Stockholm, Sweden
- 9Institute of Materials Science, Universität der Bundeswehr München, Neubiberg, Germany
- 10Department of Biotechnology, TU Delft, Delft, Netherlands
- 11Central Plantation Crops Research Institute, Kasaragod, Kerala, India
In the face of the rapidly dwindling carbon budgets, negative emission technologies are widely suggested as required to stabilize the Earth’s climate. However, finding cost-effective, socially acceptable, and politically achievable means to enable such technologies remains a challenge. We propose solutions based on negative emission technologies to facilitate wealth creation for the stakeholders while helping to mitigate climate change. This paper comes up with suggestions and guidelines on significantly increasing carbon sequestration in coffee farms. A coffee and jackfruit agroforestry-based case study is presented along with an array of technical interventions, having a special focus on bioenergy and biochar, potentially leading to “negative emissions at negative cost.” The strategies for integrating food production with soil and water management, fuel production, adoption of renewable energy systems and timber management are outlined. The emphasis is on combining biological and engineering sciences to devise a practically viable niche that is easy to adopt, adapt and scale up for the communities and regions to achieve net negative emissions. The concerns expressed in the recent literature on the implementation of emission reduction and negative emission technologies are briefly presented. The novel opportunities to alleviate these concerns arising from our proposed interventions are then pointed out. Our analysis indicates that 1 ha coffee jackfruit-based agroforestry can additionally sequester around 10 tonnes of CO2-eq and lead to an income enhancement of up to 3,000–4,000 Euros in comparison to unshaded coffee. Finally, the global outlook for an easily adoptable nature-based approach is presented, suggesting an opportunity to implement revenue-generating negative emission technologies on a gigatonne scale. We anticipate that our approach presented in the paper results in increased attention to the development of practically viable science and technology-based interventions in order to support the speeding up of climate change mitigation efforts.
1 Introduction
1.1 A new science and technology pathway for negative emissions and climate change mitigation
An unabated increase in greenhouse gases (GHGs) and other atmospheric pollutants in the Earth’s atmosphere have reached a threshold at which reversing the emissions appears to be the only survival strategy for humanity. A recent editorial in Nature (Why current negative-emissions strategies remain “magical thinking,” 2018), another recent report from National Academies of Sciences, Engineering, and Medicine (NASEM) etc. have stressed the importance of “Negative Emissions” programmes and technologies in mitigating climate change (Negative Emissions Technologies and Reliable Sequestration, 2019). The pertinence of combining biological and engineering sciences based interventions to reduce emissions at a gigatonne scale is highlighted in recent literature (Majumdar and Deutch, 2018; Horton et al., 2021). However, the concerns around high costs, large land and energy requirements pose a major challenge in the execution of commonly promulgated negative emission technologies such as Bioenergy Carbon Capture and Storage (BECCS) and Direct Air Carbon Capture and storage (DACCS). Recently Hanna et al. argued that identifying niche markets as an approach for opening up new pathways in the search for solutions for emissions reduction challenges (Hanna and Victor, 2021).
Afforestation as a negative emission technology is gaining global significance for carbon sequestration. However, it directly competes with food production (Frank et al., 2017; Doelman et al., 2020). Similar challenges appear when various negative emission technologies are taken up separately for large-scale implementation. To this date, to the best of the authors’ knowledge, not many systematic approaches of global appeal appears to have been proposed and empirically tested, even though local initiatives and scattered scientific efforts have been reported on negative emission technologies. A potential solution is a globally adoptable and easily implementable nature-based programme to achieve food security, derive income, and generate clean energy while simultaneously removing CO2 from the atmosphere. In this context, we propose technology transition pathways, based on ongoing regional carbon neutrality programmes with community participation, to introduce several negative emission technologies in an integrated manner. We present an approach combining agricultural sciences and engineering sciences (agroforestry, bioenergy, soil engineering, remote sensing etc.). We also suggest an array of technological extensions for intercropped agroforestry and its by-products, biochar for soil amendment, and bioenergy. Many of these technological extensions, potentially rather easier to start without the infusion of too many high-end technologies, is expected to result in negative emissions and simultaneously increase farmers’ income through interventions in food, water, housing, waste, energy, and climate. Further, we explain how such an approach might help us to overcome several seemingly insurmountable techno-social challenges presented in recent literature and mass media (Fellmann et al., 2018; Gardezi and Arbuckle, 2020; Söderholm, 2020). Therefore, we discuss different strategies to fight climate change at a global scale by replicating the current initiative worldwide.
Climate summit discussions are often prolonged due to the very challenging goals, further reaching agreements on emission reduction targets and the financing needed is also not easy (Buchner et al., 2019; New Scientist, 2019; Lohan, 2021). Additionally, farming based negative emission technologies are now suggested as integral to climate related initiatives, as assuring food security is equally important while fighting climate change (Beerling et al., 2018). Our approach, targeting food security, wealth generation and climate change mitigation at the same time may hence help to ease out the rather difficult negotiations at climate summits.
1.2 Where to begin?
Fleischman et al. suggest that considering the needs of indigenous rural communities is of paramount importance for the success of any nature-based climate neutral solution (Fleischman et al., 2020). The support of stakeholders whose decisions and actions determine the long-term viability of climate-friendly initiatives is therefore critical. There are such places in Kerala, a state in India where environmentally friendly initiatives are taken up with large scale community involvement. More importantly, Kerala is considered to have one of the most developed and naturally evolved agroforestry systems in the world (Hart, 1996). This might make it easier for the stakeholders to quickly introduce innovative interventions in the state making it a niche market with a potential for agro-forestry based carbon sequestration.
1.2.1 Ongoing carbon neutral village initiatives in Kerala and the Meenangadi programme
Meenangadi village within the Wayanad district of Kerala (Figure 1) has initiated several environmentally friendly initiatives in the last couple of decades, and a carbon neutrality programme recently. Environment-friendly activities are conceived and implemented as a part of the “people-centric” development programmes with the support and involvement of the local people (Meenanagadi Gramapanchayat, 2015). The “Grama Panchayat”, the village local self-governance institution is in the lead. Care has been taken to ensure that interventions are socially acceptable and, wherever possible, also economically beneficial to the people. The focus areas include environmental protection, biodiversity conservation, and agricultural development. Panchayat’s interventions included large scale tree planting, agroforestry practices, bamboo cultivation, use of compost for soil amendment, plastic waste for road construction, roof water harvesting, and the replacement of plastic bags with environment-friendly cloth and paper bags. Cultivating fruit trees, conserving native crop diversity, and protecting water bodies have been promoted while sand mining and quarrying have been restricted. As per the records available at Meenangadi Grama Panchayat, more than 300,000 trees have been planted in the area since 2016 as the continuation of previous environmental protection measures. Along with that, plastic waste segregation was started in 2017 and till now more than 22 tonnes of waste has been collected and used for road construction (Muster roll, MGNREGP, Meenangadi Grama Panchayat, 2017). MS Swaminathan Research Foundation played an important role in providing knowledge support for many of their activities (Meenangadi marches to carbon-neutral tag-The Hindu; MSSRF CAbC—Regional Centre of MSSRF). The Panchayat started the carbon neutrality programme largely based on the above-mentioned activities. Doubling the farmers income is set as an important goal. The activities are supported by the state government (Isaac, 2016, 2018, Jayakumar, 2020), a non-governmental organization “Thanal” which has carried the baseline assessments on Carbon Neutral Meenangadi (Jayakumar et al., 2018), and several other organizations including the ones many of the authors are connected with (Rajeesh et al., 2021; Vasanth et al., 2021; Vijay et al., 2021; Nandakishor et al., 2022).
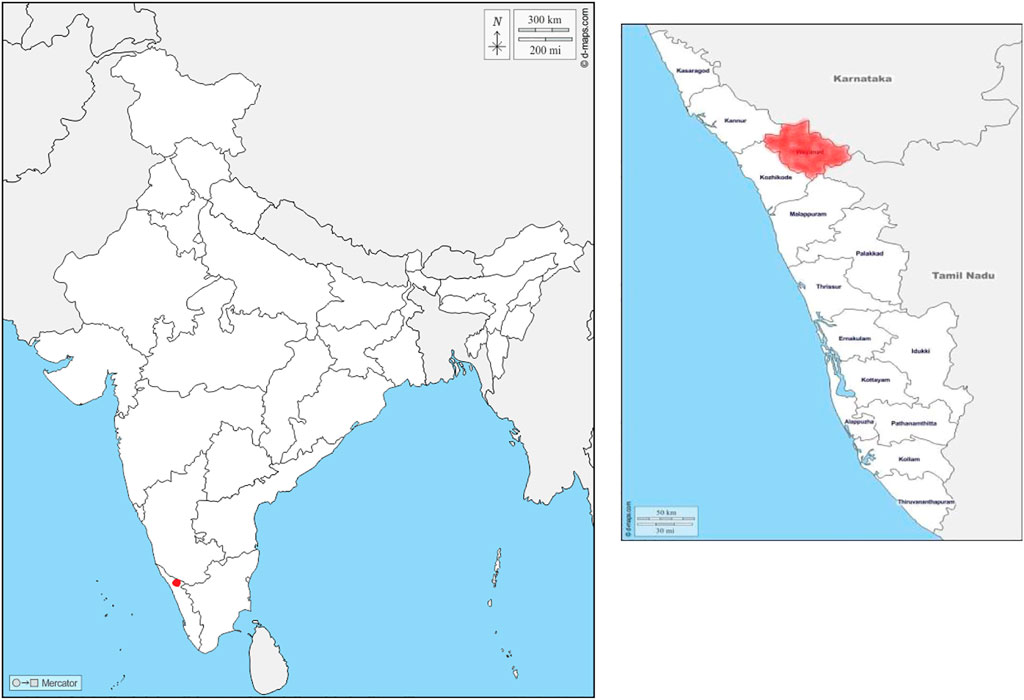
FIGURE 1. Wayanad in Kerala, India (Image Sources: https://d-maps.com/carte.php?num_car=4182&lang=en, https://d-maps.com/carte.php?num_car=8790&lang=en).
1.2.2 Tree banking and incentives for tree planting
Tree banking is a unique feature of the carbon neutral programme in Meenangadi and was recently inaugurated. The State and Local Self Governments have initiated innovative tree banking schemes to incentivize tree planting in the region (The Guardian, 2020). Under this scheme, individuals are given credit-linked incentives for planting and ensuring the survival of trees. Farmers may also use trees as collateral to get bank loans. Local Self Governments provide planting materials free and bear the cost (labour) of planting and weeding for the initial years. Till now 132 farmers have received a loan totalling around 4,000 Euros. Incentives for tree planting are critical for the success of the carbon neutral programme in two ways. First, they support the farmers to compensate for the short term losses during the juvenile period of the trees. Apprehension on yield loss and resultant financial loss is one of the major factors hindering tree planting. Second, they encourage farmers and local communities to participate in carbon neutral programmes. Thereby the initiatives ensure that carbon neutrality is a community movement, rather than just a government scheme.
1.2.3 The Mankulam programme
Similarly, Mankulam is another village in Kerala with a history in sustainable interventions including organic farming, agroforestry, village owned renewable electricity production and a village carbon neutrality programme is recently initiated. The United Nations Development Programme, Global Environment Facility, and the government agencies support the programme (with funding a project in which several of the authors are involved). The Mankulam programme focuses on achieving carbon neutrality and increasing farmer’s income using energy, farming and agroforestry interventions (coffee, tea, cardamom, jackfruit etc.).
1.3 Organized agroforestry and extended engineering interventions: The way forward
Based on the platform of ongoing efforts focusing on tree planting and other environmentally friendly initiatives, we propose the introduction of organized agroforestry together with new and renewable energy technologies, soil and water management practices, sustainable construction and an array of related science and technology-based interventions to achieve carbon neutrality and or negative emission futures while creating wealth and building climate resilience. Our suggested science and technology interventions include, pyrolysis and/or gasification for energy and biochar (as an early step with potentially other bioenergy technologies to be eventually introduced), biogas and slurry production, soil amendment, plant breeding, Information and Communication Technology (ICT) based remote and embedded sensing technologies for monitoring the water availability, agricultural productivity, and tree growth.
Agroforestry is a unique land use practice where farmers integrate trees with crops and/or livestock (Raintree, 1985). It has gained prominence in the context of climate change programmes (FAO, 2013) after the Kyoto protocol. As an interface between agriculture and forestry it is a sustainable land use practice in many developing countries. Agroforestry allows farmers to produce food, fodder, fuel, timber, and other forest resources from their farmlands (Jose, 2009). Therefore, improved agricultural practices and afforestation are potent measures to reduce GHG emissions (Rose et al., 2012; Smith et al., 2013).
Agroforestry can significantly mitigate tropical deforestation by offering alternate sources for wood products, thereby reducing the pressure on these forests (Iiyama et al., 2014). The tree-rich farming systems also reduce the need for fertilizers to improve soil quality, maintaining the nutritional balance and fertility (Shi et al., 2013). Trees in the croplands can thus improve the productivity of farming systems and also provide opportunities to create carbon sinks (Nair, 1993; Dixon et al., 1994; Dixon, 1995). Additionally, they could also offer benefits such as increased slope stability in hilly areas (Cohen and Schwarz, 2017).
2 Suggestions, guidelines, and implications: Negative emissions at negative costs
An agroforestry-based case study is presented together with suggestions and guidelines for achieving negative emissions. The case is based on the potential opportunities at Meenangadi village. Coffee is of prime importance in Meenangadi as it accounts for the largest area (around 3,000 ha) under cultivation in the village (Jayakumar et al., 2018). Coffee gardens are selected because they can accommodate shade trees (Figure 2B) if accompanied by agro-ecological interventions to reduce the competition for inputs like nutrients, water, and sunlight between plants and trees. More importantly, coffee production is expected to be severely affected by global warming (Climate & Coffee | NOAA Climate.gov; Time, 2018), and the cooling effect brought in by the shade trees is suggested as a local and natural climate change mitigation approach. A study on the impact of climate change on coffee production in Brazil indicated a 60% decline in the area suitable for coffee cultivation in unshaded plantations under projected climate change by 2050. However, it also suggested that agroforestry can mitigate climate change effects and maintain 75% of the area suitable for coffee cultivation since the trees bring in lower mean temperature, higher soil moistures and potentially more birds and bees, contributing towards pollination and pest control in coffee agroforestry systems (Gomes et al., 2020). In the present study, the expected revenue and the sequestration potential of Jackfruit-coffee-based agroforestry systems are calculated and indicative values are presented to demonstrate an alternative approach to strengthen the coffee growing regions in the face of climate change (Figure 3). The Meenangadi programme is potentially replicable in many other villages in the Wayanad district (and elsewhere), where coffee production is from 67,000 ha (Coffee board of India, 2015), partially shaded Robusta Coffee (except for a few plantations).
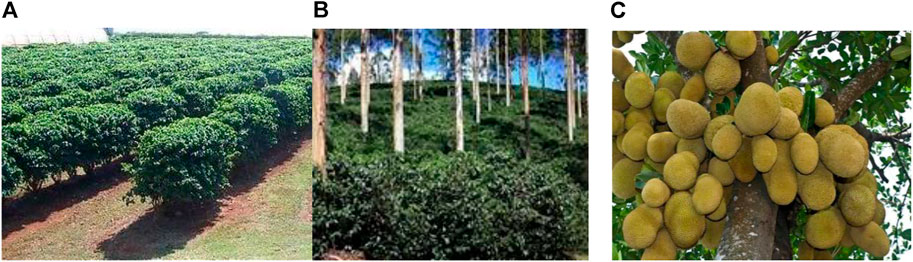
FIGURE 2. (A) Coffee Plantations without shade trees (B) with shade trees (C) Fruit bearing jackfruit tree.1) (Image Credit J. Stephen Conn is licensed under CC BY-NC 2.0)2) (Image Credit Nathan Darpen is licensed under CC BY-NC-SA 2.0) 3) (Image Credit: Balaram Mahalder is licensed under CC BY-SA 3.0).
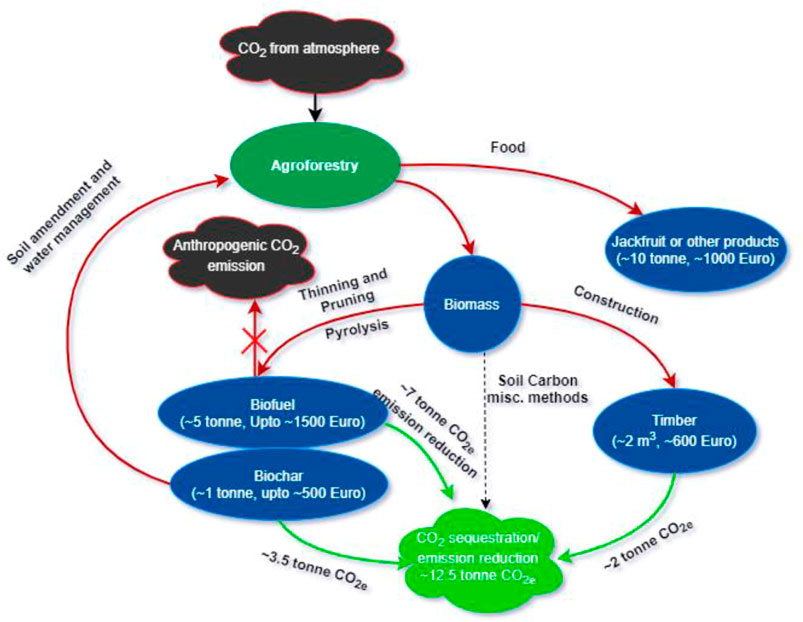
FIGURE 3. Revenue generation, emission reduction, and carbon sequestration potential (per hectare per year) of the coffee-jackfruit agroforest.
2.1 Food: Jackfruit-the jack of all fruits
Jackfruit tree is a versatile tree because apart from the direct use of its fruit, it is used as a vegetable in its tender stage and its seeds are also valuable. Jackfruit trees are suggested for coffee-jack tree agroforests as they are high food-yielding (upto 80 tonnes) and are known to have a high carbon sequestration capacity (Jithila and Prasadan, 2018). Also, the Jack tree is endemic to the Wayanad region and recently designated as the “state fruit” for the state of Kerala (Morton, 1987; The Indian Express, 2018). It is very suitable as a support tree for black pepper (Piper nigrum), whose vines can be trained on the stem. Jack trees are also recently planted along with a wide variety of tree species as part of the larger tree planting efforts in Meenangadi. Promoting jackfruit cultivation in coffee farms will gain more impact by introducing an organized industry around it. Producing and distributing quality seedlings, investing in the production, processing, and marketing of value-added products derived from jackfruit which all could contribute to such organized efforts which are already initiated in the region in small scales. However, science and technology supported large-scale efforts are yet to take place.
Jack trees are primarily cultivated in Asia- Pacific region. They are also grown in North and South America and Africa (Elevitch and Manner, 2006b). Countries like Vietnam, Philippines, Vietnam, Cambodia and Sri Lanka are aggressively promoting Jackfruit cultivation and its use. India is the second largest producer of the jackfruit in the world with more than 102,552 ha of farm areas with Jack trees, with Kerala as one of the leading cultivators in 97,000 ha annually (APAARI, 2012). In addition to its use as a staple food, a ripened fruit and a cooked vegetable, Jackfruit can also be used to prepare value added products like chips, squash, jam, pickles, ice creams, jelly, papad etc. Currently jackfruit has a market size of around $286 Million globally and is expected to grow at a rate of 3.2% annually (Jackfruit Market Share, 2021).
Jackfruit is increasingly being accepted as a meat substitute, especially for vegan and vegetarian diets, and is a potential staple food for the future (AP News, 2020; The New Indian Express, 2020). In coffee plantations, it is advisable to grow straight-growing Jack tree varieties bearing fruits on the main stem (Figure 2C). The suggested model is expected to support the farming community by providing food, income, ecological services, and rural employment. Any other tree species with food and timber values can also be included as a companion crop in such a farming system.
On an average, 40 to 45 Jack trees per ha can be grown as a shade crop, based on the recommended wide spacing of 15 m × 15 m (Morton, 1987; Elevitch and Manner, 2006a). A conservative estimate shows that 10 tonne per year per ha (APAARI, 2012; India Together, 2013; Chandrakanth Reddy et al., 2019) jackfruit yield is achievable. Taking the minimum price of a tonne of jack fruit as 100 Euro (Chandrakanth Reddy et al., 2019), a revenue of 1,000 Euro per year per ha will be generated from the jackfruit trees.
2.2 Timber
Jack tree timber is excellent for furniture and construction purposes. Approximately 2 m3 (1.2 tonnes, considering density of timber as 600 kg/m3) of timber per jackfruit tree (Elevitch and Manner, 2006a; Kunhamu, 2011; Pandya et al., 2013) or 80 m3 of timber per ha can be obtained in 40 years. Assuming the price of timber to be 300 euro per m3 an additional revenue of 600 euro per year per ha will be generated that could also compensate for any possible reduction in revenue from coffee (Beer et al., 1997). Also, we estimate that nearly 2 tonnes of CO2-eq will be sequestered per year by 2 m3 of timber (from 40 trees per ha), as explained in Supplementary Appendix S1 (Pandya et al., 2013; Jithila and Prasadan, 2018).
2.3 Biofuels, biochar, and soil management
Biomass obtained from the thinning and pruning of trees can be used to produce biochar and biofuel using pyrolysis. In a previous study, our extended team has extensively reviewed the literature on the impact of field application of biochar on soil health and crop yield (Vijay et al., 2021). Based on the reported literature, we found that the effect of biochar application is higher in stressed soils of tropical regions in comparison to the temperate regions. Total biomass output from litter falls, and pruning from a coffee plantation with shade trees is around 10–12 tonne per ha per year (Beer, 1988; Evizal et al., 2009). Based on the feedstock used, biochar and biofuel production rate using fast pyrolysis varies from 15–25 wt% of solid char, 60–75 wt% of liquid bio-oil (Mohan et al., 2006; Carrasco et al., 2017), leading to approximately 1 tonne biochar and 5 tonne biofuel (bio-oil) per ha per year. We estimate that when a tonne of biochar is used to amend soil, 3.5 tonnes (approximately) of CO2-eq is sequestered. Depending on the replaced fuel mix, nearly 5 tonnes of biofuel (bio-oil) lead to an emission reduction of approximately 7 tonnes CO2-eq per hectare. Emissions reduction and sequestration potential from the different coffee-jackfruit shade agroforestry combinations considered in this study are presented in Supplementary Appendix S1. Income enhancement opportunities with the suggested interventions are also highlighted (Supplementary Appendix S2). Biochar and the biofuel produced could generate revenue up to 500 and 1,500 euro per ha per year, respectively (Campbell et al., 2018). In the case of fast pyrolysis, an internal rate of return (IRR) of above 30% is possible, if the cost of feedstock is zero and pyrolysis co-produces biochar for carbon sequestration and biofuel for transportation (Brown et al., 2011). Another extended team of ours has started early stage experiments and carried out an extensive review of literature on biochar production technologies for rural applications in the developing countries (Vasanth et al., 2021). It appears that there are easy to implement technologies such as pyrolysis or gasification stoves to start with biochar production (Birzer et al., 2014). However, it should be noted that biochar may not directly generate income as it will be primarily used as soil enhancer in the region. Biochar is known to support the rhizosphere and productivity of agricultural land and therefore contributes to sustain the long-term productivity (Kolton et al., 2017). Together with biochar application, other soil carbon improvement methods such as composting, slurry addition, soil moisture management, legume cultivation, and zero-tillage agriculture could also increase the soil carbon. However, further studies are required to come up with an optimal approach.
Supplementary Appendices S1, S2 presents the emissions and techno-economic analysis of coffee-jackfruit agroforestry system, respectively, when biochar producing cook stoves or advanced gasifier based energy systems are employed which incidently also results in somewhat similar emission values and income generation. Also, Supplementary Appendix S1B presents a simplified life cycle assessment of the coffee jackfruit system considering the proposed interventions.
2.4 Additional benefits
There are also several other potential benefits from Jack tree agroforests. They have a strong root system that could help in slope stabilization (S. Lukose Kuriakose et al., 2009; Giadrossich et al., 2019). Jack tree leaves are used for a variety of applications such as fodder, mulch etc.
Jack trees also provide a potential opportunity for significantly increased food production. Food (jackfruit) yield up to 80 tonnes or more in certain regions with well organised farming practices. This is probably one of the highest expected food yield per hectare from any staple food species. Figure 4A indicates that the yield of food from jackfruit is significantly higher than other edible food/crop species (FAOSTAT; Rahaman et al., 2018; Kreitzman et al., 2020),. Additionally, shade coffee plantations, or agroforests provide agricultural waste streams mostly throughout the year. Figure 4B shows comparison between biomass availability from shade tree plantations and wheat and paddy fields. Availability of biomass throughout the year offers the opportunity for deploying either small scale power plants or smaller storage units for producing similar amounts of bioenergy streams (electricity, biofuels) and biochar per year when compared to other highly seasonal food crops. This is expected to help bring down the capital costs required for installing such units and their operational costs. Supplementary Appendix S3 presents the strategic planning for farmer centered and agroforestry focused emission management initiatives. A triple helix concept for such initiatives is presented with the involvement of knowledge organizations, government agencies and the industry working together, based on the experiences gained with our initiatives till now. Supplementary Appendix S4 highlights the additional biodiversity benefits in coffee farms brought by trees in agroforestry systems. It is observed that biodiversity enhancement in farms can potentially influence the yield as well as size of coffee beans due to reduced temperatures under shade slowing down the fruit maturation. Presence of more birds, bees, increased pollination and pest control are other advantages (Raghuramulu, 2005).
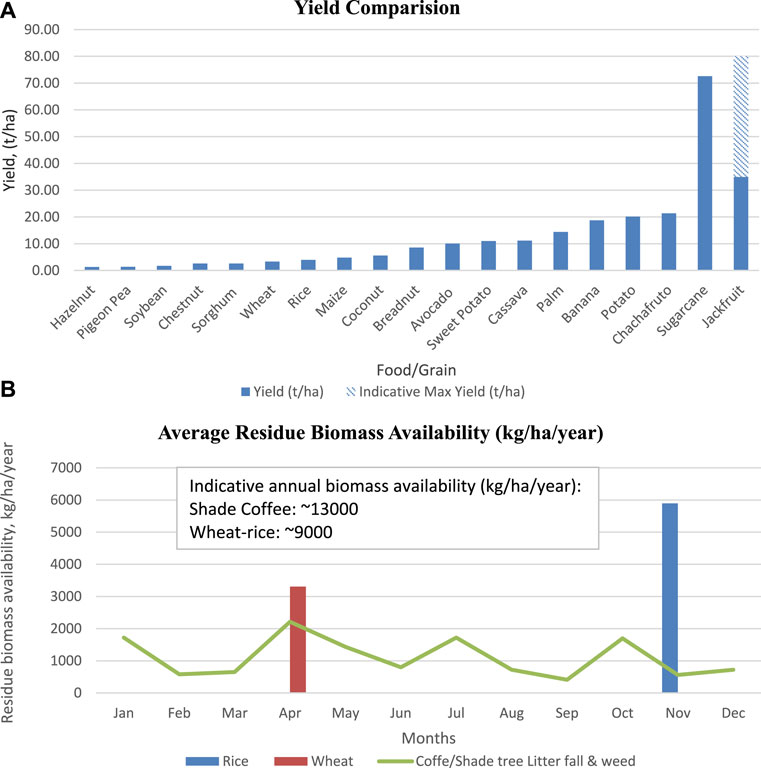
FIGURE 4. (A). Comparative yields of commonly grown high yielding food/grain species. Note: The yield of jackfruit per hectare in many places is reported to be upto 80 tonnes (Balamaze et al., 2019). The shaded sections of the bars show the indicative yield ranges. (B). Indicative values for residue biomass yield from rice, wheat (when rice and wheat are grown in consecutive seasons in the same field) and shaded coffee (Evizal et al., 2009).
2.5 The Meenangadi opportunity
Meenangadi certainly appears as a suitable village for early implementation of such a scheme. Supplementary Appendix S5 presents the results from an opinion survey conducted by our extended team highlighting the willingness of the local community for the suggested agroforestry approaches. The survey results indicate that the community members are aware of the climate change that is taking place and its implications. There is also willingness to adopt mitigation measures by planting timber and fruit yielding shade trees in farms allaying the acceptability concerns around tree planting among farmers. The reported acceptance rate of 94% appears as among the highest acceptance rates reported anywhere in the world. The detailed results are available elsewhere (Nandakishor et al., 2022). If 3,000 ha of coffee farms in Meenangadi are converted to properly managed shade coffee plantations, 6,000 tonnes of CO2-eq will be sequestered per year as timber, and 10,000 tonnes of CO2-eq will be sequestered per year as biochar (thinnings and prunings). Using biofuel for energy applications, though challenging, could help in avoiding approximately 20,000 tonnes of CO2-eq emissions per year. All of the above measures will sequester around 36,000 tonnes of CO2-eq per year, potentially making Meenangadi a carbon neutral territory (where the total calculated emissions are around 33,000 tonnes (Jayakumar et al., 2018)). Net-zero or net negative emissions could be achieved when all other interventions such as renewable energy (solar, wind, and hydro), and additional soil carbon management options mentioned above are considered. This is especially important as the renewable energy systems are rapidly becoming cost effective. The proposed interventions will generate a revenue of around 1.8 million Euro from timber, 4.5 million Euro from biofuel, and 3 million Euro from jackfruit, adding to a total revenue generation of 9.3 million Euro per year. All the above interventions may offset capital costs connected to the investments needed, any revenue drop arising from the conversion of coffee plantations to shade coffee, resulting in negative emissions at a negative cost. Supplementary Appendix S2B shows the capital cost required, potential revenue generation and the emission reduction potential of coffee-jacktree agroforestry systems.
2.6 Significance of integrated biofuel and biochar interventions in agroforestry initiatives
Figure 3 shows that integrated biofuel and biochar interventions significantly increases the emission reduction and carbon sequestration potential in the selected agroforestry system. For the combined soil amendment and bioenergy interventions, there are many emerging and attractive technological approaches. Easy to introduce routes could combine technologies ranging from small-scale biogas production and slurry utilization (Wasajja et al., 2021) to (solar) thermochemical approaches. Solid oxide fuel cells might help in CO2 separation in small scale bioenergy systems while helping to achieve very high thermodynamic efficiencies (Jaiganesh et al., 2022a). Small scale hydrogen production from biogas, pyrolysis, or gasification systems (Matthias Binder, Michael Kraussler, Matthias Kuba, 2018), based on simple and conventional approaches or based on cutting edge technologies such as internal reforming SOFCs (Saadabadi et al., 2021) or fuel assisted electrolysis is becoming very attractive, considering the significant momentum in the adoption of hydrogen technologies worldwide. Please refer to Supplementary Appendix S6 for the details of the technologies mentioned. Hydrogen or biofuels thus produced, could also provide an early-stage entry even to negative emission transportation (Jaspers et al., 2021).
3 Science and technology interventions for de-risking and the roadmap
There are several risks associated with the practical implementation of the suggested interventions in the field. Some of the major risks are acceptability problems with biofuels technologies, timber trade leading to deforestation in nearby areas, biodiversity concerns and the risk of economic leakage etc. The negative emission at negative cost programme, if properly de-risked, is an investment opportunity that can bring significant economic gains to the stakeholders while meeting the emissions targets. Supplementary Appendix S7 presents some of the risks and mitigation strategies including acceptability problems with biofuels technologies, timber trade leading to deforestation in nearby areas, and biodiversity concerns among others.
Detailed understanding in several knowledge domains, including the interaction dynamics of different intercropped species with the environment, the biochar application rate for soil amendment, technology choices for biofuels and carbon sequestration potential, is needed to come up with optimal strategies. Therefore, efforts are required to develop interlinkages between the proposed mechanisms and corresponding science and technological innovations. Biological techniques such as plant breeding are needed for developing appropriate plant varieties for agroforestry systems. Technological interventions coupled with monitoring systems in the form of Information Communication Technology (ICT) based remote and embedded sensing technologies are helpful in monitoring the water availability, agricultural productivity, and tree growth through geotagging. Remote sensing is also extremely important in developing cost-effective approaches for preserving existing forests, especially when large-scale timber programmes are conceived (Mitchell et al., 2017). Further, there are potentially rather easy to start techniques for remote sensing using mobile phones for image collection and processing (Ferster and Coops, 2016). However, advanced systems are expected to be based on drones or satellites (Tang and Shao, 2015). Figure 5 shows our proposed technological interventions and their interlinkages. In order to get the best possible results in income-generating carbon sequestration methods, some of the basic science areas to build clarity include, among others, thermodynamics, fluid dynamics, chemical sciences, material science, data sciences, artificial intelligence, and biological sciences. Engineering practices have to be developed based on scientific advancements. A fast-paced timeline to realize these technological interventions is critical to meet the international emission reduction commitments. Once the knowledge lines are well developed, the intercropped agroforestry approaches could be replicated worldwide by considering region-specific agroforestry approaches. Many of the perennial food crops presented in Figure 4A, among others, are potential candidates.
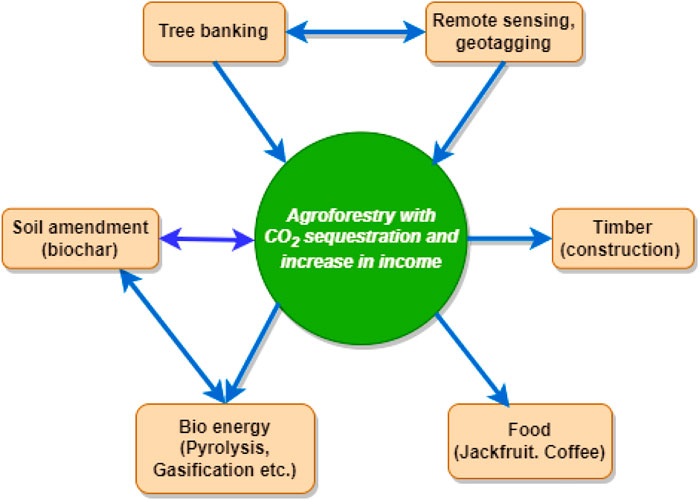
FIGURE 5. Mapping of the possible science and technology interventions for CO2-eq sequestration and revenue generation in agroforest.
While it is possible to start immediately making use of already available technologies, relevant technologies need to be developed to process and store jackfruit and other similar farm produce, biofuel and biochar production and utilization, all considering emission reduction and carbon sequestration. Further, the focus on large-scale timber-based construction technologies for carbon neutrality is new despite timber usage for construction in the past. Tailoring these technologies for achieving carbon neutrality and large-scale implementation may take a couple of years.
Advanced technologies might make these approaches even more attractive in the future (ultra-high efficiency bioenergy systems based on solid oxide fuel cells, fuel assisted electrolysers etc., as discussed before). For such technologies, one or 2 decades are needed for globally acceptable engineering practices and products to become widely available, if the technology development pace is comparable with the timelines of the average technology development and implementation cycles (from technology readiness level-1 to 9 and then to market (Peisen et al., 1999). With the technology pathways mentioned above, focussed efforts might result in such solutions becoming available sooner, especially since technology development is getting significantly faster (Butler, 2016). Dedicated and focused efforts are hence urgently needed. An interesting case study to learn from is the initiative by the coffee farmers from Costa Rica. They have obtained carbon neutrality certification based on a collective effort jointly with academia, government agencies, and companies.
Combining all these interventions and considering their importance, we are building multiple science and technology partnerships to involve in and contribute to such initiatives. Academic institutions, government agencies, business houses and global bodies (such as UN agencies), etc. are involved. This is well-aligned with the large-scale investments, the Government of Kerala (Isaac, 2016, 2018, 2020) and the Government of India are planning (WRI India, 2021) in carbon neutrality and renewable energy programmes (worth billions of Euros).
4 Our approach and answering the recently posed questions
Cox et al. looked at the public perception of carbon dioxide removal technologies and concluded that tangible and near-term results are helpful in receiving public support for such efforts (Cox et al., 2020). Hanssen et al. presented the potential for bioenergy with carbon capture and storage (BECCS) but expressed concern over the land requirement for BECCS systems (Hanssen et al., 2020). A major concern with negative emission technologies like Direct Air Capture (DAC) is that they are energy-intensive and costly (Fuhrman et al., 2020). Our approach alleviates such apprehensions, as food production and wealth creation are suggested parallel to carbon sequestration efforts. A niche opportunity is also identified in line with the suggestions from a previous study (Hanna and Victor, 2021). Chile’s afforestation programme through forest subsidies resulted in the expansion of exotic tree plantations and decreased the area of native forest and biodiversity without increasing total carbon stored (Heilmayr et al., 2020). Learning from the above, policymakers must ensure that afforestation/reforestation policies are carefully developed to increase vegetation and carbon sequestration and conserve biodiversity simultaneously.
Hasegawa et al. stated that implementing stringent climate mitigation policies across all regions and sectors will negatively impact global hunger and food consumption by 2050 (Hasegawa et al., 2018). Hayek et al. points out the need for carefully designed mitigation policies for agriculture and land use to simultaneously achieve climate stabilization and food security (Hayek et al., 2020). Similarly, based on a thorough review of literature on empirical evidence about the adoption of sustainable agricultural practices, Piñeiro et al. also affirmed that emission management programmes linked to short-term economic benefits have higher adoption rates than those aimed solely at providing an ecological service (Piñeiro et al., 2020). Therefore, an integrated approach of food and bioenergy production should be explored. Horton et al. evaluated the technologies to deliver food and climate security through agriculture, and pointed out the need for a series of new agricultural technologies in order to allow intensive agriculture to have a key role in climate change mitigation (Horton et al., 2021). Hepburn et al. compared several CO2 utilization methods and came up with several economically attractive propositions, including the land-based ones (Hepburn et al., 2019). However, they have stated that barriers to implementation remain substantial.
In a general perspective, climate change is attributed largely to increased GHG emissions. This overlooks the significant role many of the negative emission technologies play in land use, sustainable soil and water management, and consequently the hydrological cycle and the local climate (Roodari et al., 2021). The potential role of trees in forests in cooling and stabilizing local climates through moisture regeneration (Makarieva et al., 2009) may bring additional long-term benefits by mitigating the predicted reduction in growing areas due to climate change by 2050 (Climate & Coffee | NOAA Climate.gov).
Our novel approach makes bioenergy with CCS a much more attractive option avoiding concerns over the land requirement for BECCS. Our approach is also centered around agricultural practices that concurrently benefit the farmers and environment in both the short and long run and thus becomes a possible inclusive and participative solution at the community level. The solutions presented by us prioritizes food security and revenue generation along with climate stabilization, thus leading to negative emissions at a negative cost. Furthermore, we examined the possibilities of integrating climate stabilization with food production and food security, fuel production, and timber management as a well-integrated solution for the future.
It is our view that the novel integrated concepts presented here (including the steps given in Box 1) may encourage to forego the apprehensions and quickly start the efforts to test and deploy negative emission technologies at a large scale in the near future. These concepts also offer an opportunity to achieve environmental, economic, and social sustainability in an all-integrated manner.
BOX 1
(1) We propose full preparations for adopting Jackfruit agroforestry as a potential solution for climate-friendly and secure staple food production (if and when needed and if the climate crisis deepens). Conversion of robusta coffee plantations into shade coffee plantations appears as an appealing and easy to start first step. Other possible agroforestry combinations also need to be explored.(2) Bioenergy production and replacement of fossil fuels (especially combined with biochar/bio-slurry production) appear as offering the most significant CO2 emission reduction opportunity in agroforestry initiatives. Biohydrogen is certainly worth considering with carbon going to the soil and hydrogen used as fuel. Other methods for soil carbon management might also offer similar opportunities, but there are uncertainties (Tiefenbacher et al., 2021).(3) It is important to start such agroforestry initiatives where there is demonstrated local acceptance and active community participation, for instance the Meenangadi case presented in this study.(4) To the best of our knowledge, such a unique combination of easy to introduce coffee-jackfruit agroforestry with biofuels and biochar production, with an extremely appealing set of benefits, if (and only if) the systems are engineered very carefully, is not yet presented in literature. Significant research and development efforts along these lines need to be started across the globe, in co-creation mode, considering the sharp climate changes and the global urgency.
(1) We propose full preparations for adopting Jackfruit agroforestry as a potential solution for climate-friendly and secure staple food production (if and when needed and if the climate crisis deepens). Conversion of robusta coffee plantations into shade coffee plantations appears as an appealing and easy to start first step. Other possible agroforestry combinations also need to be explored.
(2) Bioenergy production and replacement of fossil fuels (especially combined with biochar/bio-slurry production) appear as offering the most significant CO2 emission reduction opportunity in agroforestry initiatives. Biohydrogen is certainly worth considering with carbon going to the soil and hydrogen used as fuel. Other methods for soil carbon management might also offer similar opportunities, but there are uncertainties (Tiefenbacher et al., 2021).
(3) It is important to start such agroforestry initiatives where there is demonstrated local acceptance and active community participation, for instance the Meenangadi case presented in this study.
(4) To the best of our knowledge, such a unique combination of easy to introduce coffee-jackfruit agroforestry with biofuels and biochar production, with an extremely appealing set of benefits, if (and only if) the systems are engineered very carefully, is not yet presented in literature. Significant research and development efforts along these lines need to be started across the globe, in co-creation mode, considering the sharp climate changes and the global urgency.
5 Global outlook—A brief discussion
The proposed approach, if expanded globally, has the potential for achieving negative emissions (gigatonne scale) at negative costs. Globally, coffee is grown in around 11 Mha. Approximately 4 million ha (Jha et al., 2014) of unshaded coffee plantations could be converted to shade coffee plantation with perennial food producing trees as shade trees. It is anticipated that a part of the approximately 3.5 million ha of sparsely shaded coffee farms (Jha et al., 2014) could offer the opportunities for planting food yielding trees. In total this could result in a CO2-eq sequestration/emission avoidance somewhere between 40 million tonnes CO2-eq to 75 million tonnes CO2-eq per year.
From a food production perspective, our assessment indicates that more than 10 tonnes of food can be obtained per ha (from 40 trees) per year from Coffee-jackfruit tree-agroforestry. Intercropped coffee-jackfruit tree agroforests also could be developed without coffee but with other crops (such as black pepper, cardamom, vegetables, fruits etc.) Considering 500 kg per year as the food requirement per person (Serra-Majem et al., 2003), and on an average 40 Jack trees are planted per ha in all cases around 400 million hectares of intercropped-jackfruit based agroforestry (not necessarily with coffee) or agroforestry practices based on other perennial food crops taking a few hundred million hectares more) can meet a major part of the food requirements for the current 7.8 billion world population. This estimation is in line with the detailed discussion on the advantage of perennial food crops vs. annual food crops (wheat, soya and rice) that was recently presented by Kreitzman et al shows that a conversion of a fraction of the 1.29 billion hectares of agricultural land used for annual crops into perennial crops has an underutilised potential for the global food supply of nutritious staple foods (Kreitzman et al., 2020).
Timber from jackfruit trees is another highly valuable product. Presently, the cement industry emits around 8% of the world’s total GHG emissions (Carbon Brief, 2018). Promotion of timber-based construction can reduce the dependency on highly emission-intensive cement and steel sectors, and hence reduce the GHG emissions (Skullestad et al., 2016; Sandanayake et al., 2018). Our estimation indicates that 80 m3 of jackfruit tree timber can be produced per hectare from coffee-jackfruit agroforestry in 40 years, which can be used for construction purposes. Thus, extending Jack fruit, or other staple food yielding tree-based agroforestry with a variety of intercrops to nearly 400 million hectares and using the timber to replace a fraction of cement and steel, will offer significant emission reduction from the construction sector around the world. More details on emission reduction potential from concrete replacement with timber are presented in Supplementary Appendix S8. For example, Chachafruto (Erythrina edulis) is another appealing shade tree species with significant food (upto 85 t/km fencing) and fodder production (upto 30 t/km fencing) (Orwa et al., 2009) potential that can be explored on similar lines as jackfruit in suitable agro-climatic zones globally.
Following this approach, a significant share of more than one billion hectares of the world’s arable land can be used for tree planting which is otherwise used for farming to produce food. Apart from timber, biomass can be used to produce biochar, a wide range of industrial chemicals, and other bioproducts. The energy alternatives from Jack tree biomass can also provide biofuels (including biohydrogen) as a substituent to fossil fuels for future transportation fuel requirements leading to negative emission transportation opportunities (Jaspers et al., 2021). Bioenergy integrated shade coffee systems could be replicated in many other places, to begin with in other major coffee-producing regions such as Brazil, Colombia, Indonesia, Vietnam, Ethiopia etc. Other similar agroforestry systems with different crop combinations could then be introduced in many other countries. While a coffee- jackfruit tree agroforestry system is initially proposed, many other combinations might be possible and they include- Coffee or Cocoa with coconut, mango, cashew nut etc. Chachafruto fruto found in South America could be another option.
Wider replication of the proposed programme with large-scale afforestation, biochar for soil carbon sequestration, and fossil fuel replacement with biofuels can help to reduce 5%–10% (or more) of the current global GHG emissions. This shows the importance of bioenergy initiatives in global agroforestry-based emission reduction efforts and even beyond. Biofuels, if judiciously employed, might also help the difficult to decarbonize sectors such as shipping and aviation becoming emission negative. In extremely well-designed cases this could perhaps make even aviation more environment friendly than riding bicycle or even walking, if one considers the well to wheel fuel production and utilization chain resulting in active removal of carbon dioxide from atmospheric air. With the emerging uncertainties in the future fuel options worldwide (Speirs et al., 2015) and the energy conversion technologies for the chosen fuels, and the wide variety of technology options cropping up in biofuel production and utilization, it is becoming imperative that the scientific and engineering communities start giving significantly increased attention to bioresource management and their efficient utilization.
The opportunities for generating wealth, could also help to foster international solidarity in finding climate solutions. Our suggested approach offers a solution for the simultaneous achievement of climate stabilization and food security. We believe that the global hunger and food consumption-related problems resulting from mitigation policies can be bypassed through the suggested interventions. Further, the increase in solar and wind production capacity indicates that a negative emission global society may not be a distant dream anymore. Such an effort will, however, need well-coordinated science and technology development programmes, accompanied with socio-technical studies. Nonetheless, taking up such initiatives is suggested as worthwhile considering the environmental challenges the humanity faces.
6 Summary
Negative emission technologies are gaining global significance and the need for combining agricultural sciences and engineering sciences is critical for a cleaner and healthier future. A global programme of this nature, based on agroforestry, might lead to increased food security and income for the rural population while stabilizing climate. Our indicative analysis shows that 1 ha of coffee-jackfruit-agroforestry system has the potential to sequester approximately 10.5 tonnes of CO2-eq per year while also presenting an income generating opportunity of the order of 3,000–4,000 Euro per year. Other agroforestry systems based on different perennial food crops also appear as worth considering. We have presented an empirically testable integrated approach (encompassing bioenergy systems, geo-tagging, remote sensing, soil carbon and water management, biochar application, biodiversity conservation) implementable in a rather short time period, well connected to an ongoing government programme. The energy, water, waste, food, and housing emissions nexus pointed out here brings a unique opportunity to potentially achieve negative emission features at negative costs, with regional efforts first, eventually leading to a global effort–driven by local farmers and the governments. Ongoing and the expected future efforts include knowledge development in all the domains discussed in this manuscript and field level implementation with a participatory approach, well supported by a triple helix consortium. The success of the programme, if realized, is expected to open up similar opportunities elsewhere in the world and hence is of international significance.
Author contributions
PA conceived of the paper and drafted the first outline. VC, VV, and PA finalised the manuscript. GG supported the Meenangadi Panchayat officials in implementing the sustainability and the carbon neutrality programmes for more than a decade. All authors contributed in developing the concepts, provided critical input on estimates, and were involved in drafting the manuscript. Additionally, all authors have read and approved the manuscript.
Acknowledgments
Authors acknowledge the support and leadership provided by former Finance Minister of Kerala, Dr. Thomas Isaac, in setting the carbon neutral Meenangadi and Wayanad initiatives. Authors also thank the President of the Meenangadi Village Panchayat, Beena Vijayan, Ajit Mathai of mByom, Executive Manager of TU Delft Climate Institute H Schoffelen, and the Director of the TU Delft Climate Institute Prof H Russchenberg for their help in setting up and contributing to the science and technology related discussions on and around the concepts presented in this paper. Authors are also thankful to Nadia van Pelt, Centre for Languages and Academic Skills, TU Delft, for her help in language editing of several sections of manuscript. The authors are grateful to the Panchayat officials and the experts who have attended several work meetings and events organized in support of the initiative. We also acknowledge the early-stage activities carried out in Meenangadi by the NGO, Thanal. All the speakers from various organizations and countries who contributed to the science and technology related discussions during the workshop and the webinar organized in connection with the carbon neutral Wayanad programme are also thanked by the authors (one workshop organised by Kerala Government Agencies in 2019 and the webinar by TU Delft in 2020).
Conflict of interest
The authors declare that the research was conducted in the absence of any commercial or financial relationships that could be construed as a potential conflict of interest.
Publisher’s note
All claims expressed in this article are solely those of the authors and do not necessarily represent those of their affiliated organizations, or those of the publisher, the editors and the reviewers. Any product that may be evaluated in this article, or claim that may be made by its manufacturer, is not guaranteed or endorsed by the publisher.
Supplementary Material
The Supplementary Material for this article can be found online at: https://www.frontiersin.org/articles/10.3389/fenrg.2022.806435/full#supplementary-material
References
Aman, R. (2022). A guide to jackfruit cultivation. Available at: https://rfcarchives.org.au/Next/Fruits/Jakfruit/JackfruitCultiv2-84.htm (Accessed June 22, 2022).
AP News (2020). apnews. Available at: https://apnews.com/article/2b1890ed42eb4689a11f77c2f4dfbfb6 (Accessed October 13, 2020).
APAARI (2012). Jackfruit improvement in the asia-pacific region-A status report. Asia-pacific assoc. Agric. Res. Institutions, 182. Available at: www.apaari.org.
Ayanleye, S., Udele, K., Nasir, V., Zhang, X., and Militz, H. (2022). Durability and protection of mass timber structures: A review. J. Build. Eng. 46, 103731. doi:10.1016/j.jobe.2021.103731
Balamaze, J., Muyonga, J. H., and Byaruhanga, Y. B.Food Technology, Business Incubation Centre, School of Food Technology, Nutrition and Bio Engineering Makerere University, P. O. Box 7062 Kampala UgandaFood Technology, Business Incubation Centre, School of Food Technology, Nutrition and Bio Engineering Makerere University, P. O. Box 7062 Kampala Uganda (2019). Production and utilization of jackfruit (Artocarpus heterophyllus) in Uganda. Afr. J. Food Agric. Nutr. Dev. 19, 14289–14302. doi:10.18697/AJFAND.85.17290
Bavec, F., and Bavec, M. (2015). “Underutilized crops and intercrops in crop rotation as factors for increasing biodiversity on fields,” in Biodiversity in ecosystems - linking structure and function (London: InTech). doi:10.5772/59131
Beer, J. (1988). Litter production and nutrient cycling in coffee (Coffea arabica) or cacao (Theobroma cacao) plantations with shade trees. Agroforest. Syst. 7, 103–114. doi:10.1007/BF00046846
Beer, J., Muschler, R., Kass, D., and Somarriba, E. (1997). Shade management in coffee and cacao plantations. Agrofor. Syst. 38, 139–164. doi:10.1023/a:1005956528316
Beerling, D. J., Leake, J. R., Long, S. P., Scholes, J. D., Ton, J., Nelson, P. N., et al. (2018). Farming with crops and rocks to address global climate. Nat. Plants 4, 138–147. food and soil security/631/449/706/1143/704/47/704/106 perspective. doi:10.1038/s41477-018-0108-y
Bhagwat, S. A., Willis, K. J., Birks, H. J. B., and Whittaker, R. J. (2008). Agroforestry: A refuge for tropical biodiversity? Trends Ecol. Evol. 23, 261–267. doi:10.1016/j.tree.2008.01.005
Birzer, C., Medwell, P., MacFarlane, G., Read, M., Wilkey, J., Higgins, M., et al. (2014). “A biochar-producing, dung-burning cookstove for humanitarian purposes,” in Procedia engineering (Elsevier), 243–249. doi:10.1016/j.proeng.2014.07.063
Bote, A. D., and Struik, P. C. (2011). Effects of shade on growth, production and quality of coffee (Coffea arabica) in Ethiopia. J. Hortic. For. 3, 336–341. Available at: https://research.wur.nl/en/publications/effects-of-shade-on-growth-production-and-quality-of-coffee-coffe (Accessed January 15, 2022).
Brown, T. R., Wright, M. M., and Brown, R. C. (2011). Estimating profitability of two biochar production scenarios: Slow pyrolysis vs fast pyrolysis. Biofuels Bioprod. Bioref. 5, 54–68. doi:10.1002/bbb.254
Buchner, B., Clark, A., Falconer, A., Macquarie, C., Tolentino, R., and Watherbee, C. (2019). Global landscape of climate finance 2019. Clim. Policy Initiat., 15. Available at: https://www.climatepolicyinitiative.org/wp-content/uploads/2019/11/2019-Global-Landscape-of-Climate-Finance.pdf.
Butler, D. (2016). Tomorrow’s world. Bus. Strategy Rev. 530, 11–17. doi:10.1111/j.0955-6419.2004.00334.x
Campbell, R. M., Anderson, N. M., Daugaard, D. E., and Naughton, H. T. (2018). Financial viability of biofuel and biochar production from forest biomass in the face of market price volatility and uncertainty. Appl. Energy 230, 330–343. doi:10.1016/j.apenergy.2018.08.085
Carbon Brief (2018). Carbon Brief. Available at: https://www.carbonbrief.org/qa-why-cement-emissions-matter-for-climate-change (Accessed September 6, 2020).
Carbon emissions of different fuels - Forest Research (2022). Carbon emissions of different fuels - forest Research. Available at: https://www.forestresearch.gov.uk/tools-and-resources/fthr/biomass-energy-resources/reference-biomass/facts-figures/carbon-emissions-of-different-fuels/ (Accessed January 25, 2022).
Carrasco, J. L., Gunukula, S., Boateng, A. A., Mullen, C. A., DeSisto, W. J., Wheeler, M. C., et al. (2017). Pyrolysis of forest residues: An approach to techno-economics for bio-fuel production. Fuel 193, 477–484. doi:10.1016/j.fuel.2016.12.063
Cement (2022). Bureau of energy efficiency. Available at: https://beeindia.gov.in/node/166 (Accessed January 15, 2022).
Chain-Guadarrama, A., Martínez-Salinas, A., Aristizábal, N., and Ricketts, T. H. (2019). Ecosystem services by birds and bees to coffee in a changing climate: A review of coffee berry borer control and pollination. Agric. Ecosyst. Environ. 280, 53–67. doi:10.1016/j.agee.2019.04.011
Chandrakanth Reddy, I., Prabakar, C., Sita Devi, K., Ponnarasi, T., and Shelton Peter, Y. (2019). An economic analysis on jackfruit production and marketingincuddaloredistrictofTamilnadu, India. Plant Arch. 19, 2801–2809.
Cinti, G., Bidini, G., and Hemmes, K. (2016). An experimental investigation of fuel assisted electrolysis as a function of fuel and reactant utilization. Int. J. Hydrogen Energy 41, 11857–11867. doi:10.1016/j.ijhydene.2016.05.205
Climate and Coffee (2022). NOAA Climate.gov. Available at: https://www.climate.gov/news-features/climate-and/climate-coffee (Accessed January 15, 2022).
Coffee board of India (2015). Database on coffee. Available at: http://www.indiacoffee.org/Database/DATABASE_Mar15_I.pdf.
Cohen, D., and Schwarz, M. (2017). Tree-root control of shallow landslides. Earth Surf. Dynam. 5, 451–477. doi:10.5194/esurf-5-451-2017
Lohan, L. (2021). On foreign relations. Available at: https://www.cfr.org/backgrounder/paris-global-climate-change-agreements (Accessed May 17, 2021).
Cox, E., Spence, E., and Pidgeon, N. (2020). Public perceptions of carbon dioxide removal in the United States and the United Kingdom. Nat. Clim. Chang. 10, 744–749. doi:10.1038/s41558-020-0823-z
Dixon, R. K. (1995). Agroforestry systems: Sources or sinks of greenhouse gases? Agroforest. Syst. 31, 99–116. doi:10.1007/BF00711719
Dixon, R. K., Brown, S., Houghton, R. A., Solomon, A. M., Trexler, M. C., Wisniewski, J., et al. (1994). Carbon pools and flux of global forest ecosystems. Science 263, 185–190. doi:10.1126/science.263.5144.185
Doelman, J. C., Stehfest, E., Vuuren, D. P., Tabeau, A., Hof, A. F., Braakhekke, M. C., et al. (2020). Afforestation for climate change mitigation: Potentials, risks and trade‐offs. Glob. Chang. Biol. 26, 1576–1591. doi:10.1111/gcb.14887
Elevitch, C. R., and Manner, H. I. (2006a). Artocarpus heterophyllus (jackfruit) ver. 1.1v. 4–5. Available at: http://eprints.soton.ac.uk/53417/.
Elevitch, C. R., and Manner, H. I. (2006b). “Artocarpus heterophyllus (jackfruit) ver. I.I,” in Species profiles for pacific island agroforestry (Hawaii, United States: Hōlualoa, Hawai‘i: Permanent Agriculture Resources).
Elevitch, C. R., and Manner, H. I. (2006). “Artocarpus heterophyllus (jackfruit) ver. I.I,” in Species profiles for pacific island agroforestry hōlualoa, Hawai‘i (Hawaii, United States: Permanent Agriculture Resources).
Erol, M., Haykiri-Acma, H., and Küçükbayrak, S. (2010). Calorific value estimation of biomass from their proximate analyses data. Renew. Energy 35, 170–173. doi:10.1016/j.renene.2009.05.008
Evizal, R., Prijambada, I. D., Mada, U. G., Widada, J., Mada, U. G., Widianto, D., et al. (2009). “Biomass production of shade grown coffee agroecosystems,” in International seminar on sustainable biomass production and utilization challenges and oppurtunities.
FAO (2013). Advancing agroforestry on the policy agenda: A guide for decision-makers. FAOSTAT Available at: http://www.fao.org/faostat/en/#data/QC (Accessed August 2, 2021).
Fellmann, T., Witzke, P., Weiss, F., Van Doorslaer, B., Drabik, D., Huck, I., et al. (2018). Major challenges of integrating agriculture into climate change mitigation policy frameworks. Mitig. Adapt. Strateg. Glob. Chang. 23, 451–468. doi:10.1007/s11027-017-9743-2
Ferster, C. J., and Coops, N. C. (2016). Integrating volunteered smartphone data with multispectral remote sensing to estimate forest fuels. Int. J. Digit. Earth 9, 171–196. doi:10.1080/17538947.2014.1002865
Fleischman, F., Basant, S., Chhatre, A., Coleman, E. A., Fischer, H. W., Gupta, D., et al. (2020). Pitfalls of tree planting show why we need people-centered natural climate solutions. Bioscience. doi:10.1093/biosci/biaa094
Frank, S., Havlík, P., Soussana, J. F., Levesque, A., Valin, H., Wollenberg, E., et al. (2017). Reducing greenhouse gas emissions in agriculture without compromising food security? Environ. Res. Lett. 12, 105004. doi:10.1088/1748-9326/aa8c83
Fuhrman, J., McJeon, H., Patel, P., Doney, S. C., Shobe, W. M., Clarens, A. F., et al. (2020). Food–energy–water implications of negative emissions technologies in a +1.5 °C future. Nat. Clim. Chang. 10, 920–927. doi:10.1038/s41558-020-0876-z
Gao, Y., Jiang, J., Meng, Y., Yan, F., and Aihemaiti, A. (2018). A review of recent developments in hydrogen production via biogas dry reforming. Energy Convers. Manag. 171, 133–155. doi:10.1016/j.enconman.2018.05.083
Gardezi, M., and Arbuckle, J. G. (2020). Techno-optimism and farmers’ attitudes toward climate change adaptation. Environ. Behav. 52, 82–105. doi:10.1177/0013916518793482
Available at: GCEK (2022). gcek. https://www.gcek.ac.in/ (Accessed June 13, 2022).
Giadrossich, F., Cohen, D., Schwarz, M., Ganga, A., Marrosu, R., Pirastru, M., et al. (2019). Large roots dominate the contribution of trees to slope stability. Earth Surf. Process. Landforms 44, 1602–1609. doi:10.1002/esp.4597
Gomes, L. C., Bianchi, F. J. J. A., Cardoso, I. M., Fernandes, R. B. A., Filho, E. I. F., Schulte, R. P. O., et al. (2020). Agroforestry systems can mitigate the impacts of climate change on coffee production: A spatially explicit assessment in Brazil. Agric. Ecosyst. Environ. 294, 106858. doi:10.1016/j.agee.2020.106858
Hanna, R., and Victor, D. G. (2021). Marking the decarbonization revolutions. Nat. Energy 6, 568–571. doi:10.1038/s41560-021-00854-1
Hanssen, S. V., Daioglou, V., Steinmann, Z. J. N., Doelman, J. C., Van Vuuren, D. P., Huijbregts, M. A. J., et al. (2020). The climate change mitigation potential of bioenergy with carbon capture and storage. Nat. Clim. Chang. 10, 1023–1029. doi:10.1038/s41558-020-0885-y
Hart, R. (1996). Forest gardening: Cultivating an edible landscape. 2nd ed. Chelsea Green Publishing.
Harvey, C. A., and González Villalobos, J. A. (2007). Agroforestry systems conserve species-rich but modified assemblages of tropical birds and bats. Biodivers. Conserv. 16, 2257–2292. doi:10.1007/s10531-007-9194-2
Hasegawa, T., Fujimori, S., Havlík, P., Valin, H., Bodirsky, B. L., Doelman, J. C., et al. (2018). Risk of increased food insecurity under stringent global climate change mitigation policy. Nat. Clim. Chang. 8, 699–703. doi:10.1038/s41558-018-0230-x
Hayek, M. N., McDermid, S. P., and Jamieson, D. W. (2020). An appeal to cost undermines food security risks of delayed mitigation. Nat. Clim. Chang. 10, 418–419. doi:10.1038/s41558-020-0766-4
Heilmayr, R., Echeverría, C., and Lambin, E. F. (2020). Impacts of Chilean forest subsidies on forest cover, carbon and biodiversity. Nat. Sustain. 3, 701–709. doi:10.1038/s41893-020-0547-0
Hepburn, C., Adlen, E., Beddington, J., Carter, E. A., Fuss, S., Mac Dowell, N., et al. (2019). The technological and economic prospects for CO2 utilization and removal. Nature 575, 87–97. doi:10.1038/s41586-019-1681-6
Home (2022). Thanal. Available at: https://thanaltrust.org/ (Accessed June 13, 2022).
Horton, P., Long, S. P., Smith, P., Banwart, S. A., and Beerling, D. J. (2021). Technologies to deliver food and climate security through agriculture. Nat. Plants 7, 250–255. doi:10.1038/s41477-021-00877-2
Hydrogen Valley (2022). New energy coalition. Available at: https://www.newenergycoalition.org/en/hydrogen-valley/ (Accessed January 1, 2022).
Iiyama, M., Neufeldt, H., Dobie, P., Njenga, M., Ndegwa, G., Jamnadass, R., et al. (2014). The potential of agroforestry in the provision of sustainable woodfuel in sub-Saharan Africa. Curr. Opin. Environ. Sustain. 6, 138–147. doi:10.1016/j.cosust.2013.12.003
India Together (2013). India together. Available at: https://indiatogether.org/jackfruit-agriculture (Accessed August 9, 2021).
Isaac, T. M. T. (2020). Budget speech 2020-2021. Available at: file:///C:/Users/youhe/Downloads/kdoc_o_00042_01.pdf.
Isaac, T. M. T. (2016). Government of Kerala revised budget speech. Available at: https://kerala.gov.in/documents/10180/5b6cb7dd-d019-42f6-b9a5-836abada51f0.
Jackfruit Market Share (2021). Size and industry growth analysis 2021. Available at: https://www.industryarc.com/Research/Jackfruit-Market-Research-507377 (Accessed January 9, 2022).
Jaiganesh, N., Kuo, P., Woudstra, T., Kumar, R. A., and Aravind, P. V (2022a). Negative emission power plants: Thermodynamic modeling and evaluation of a biomass-based integrated gasification solid oxide fuel cell/gas turbine system for power, heat, and biochar co-production-part 1. 10, 1–20. doi:10.3389/fenrg.2022.803756
Jaiganesh, N., Kuo, P-C., Champatan, V., Girigan Gopi, R., and Aravind, P. V. A. (2022b). Negative emission power plants: Techno-economic analysis (TEA) of a biomass-based integrated gasification solid oxide fuel cell/gas turbine system for power, heat and biochar co-production - Part 2. Front. Energy Res. Sect. Carbon Capture, Util. Storage. doi:10.3389/fenrg.2022.826227
Jaspers, B. C., Kuo, P.-C., Amladi, A., van Neerbos, W., and Aravind, P. V. (2021). Negative CO2 emissions for transportation. Front. Energy Res. 9, 151. doi:10.3389/fenrg.2021.626538
Jayakumar, C., Ushakumari, S., and Nair, S. K. (2018). Carbon neutral Meenangadi – assessment and recommendations. Kerala: Thiruvananthapuram.
Jezeer, R. E., Verweij, P. A., Santos, M. J., and Boot, R. G. A. (2017). Shaded coffee and Cocoa – double dividend for biodiversity and small-scale farmers. Ecol. Econ. 140, 136–145. doi:10.1016/j.ecolecon.2017.04.019
Jha, S., Bacon, C. M., Philpott, S. M., Ernesto Méndez, V., Läderach, P., Rice, R. A., et al. (2014). Shade coffee: Update on a disappearing refuge for biodiversity. Bioscience 64, 416–428. doi:10.1093/biosci/biu038
Jithila, P. J., and Prasadan, P. K. (2018). Carbon sequestration by trees-A study in the Western Ghats, Wayanad region. Indian J. Ecol. 45, 479–482.
Jose, S. (2012). Agroforestry for conserving and enhancing biodiversity. Agroforest. Syst. 85, 1–8. doi:10.1007/s10457-012-9517-5
Jose, S. (2009). Agroforestry for ecosystem services and environmental benefits: An overview. Agroforest. Syst. 76, 1–10. doi:10.1007/s10457-009-9229-7
Joy, C. V. (2004). Small coffee growers of sulthan bathery. Wayanad. Kerala Res. Program. Local Lev. Dev. Cent. Dev. Stud. Thiruvanathapuram.
Killian, B., Rivera, L., Soto, M., and Navichoc, D. (2013). Carbon footprint across the coffee supply chain : The case of Costa Rican coffee track : Supply-chain and operation management carbon footprint across the coffee supply chain. J. Agric. Sci. Technol. B 3, 151–170.
Kim, T. H., Chae, C. U., Kim, G. H., and Jang, H. J. (2016). Analysis of CO2 emission characteristics of concrete used at construction sites. Sustainability 8, 348. doi:10.3390/su8040348
Kolton, M., Graber, E. R., Tsehansky, L., Elad, Y., and Cytryn, E. (2017). Biochar-stimulated plant performance is strongly linked to microbial diversity and metabolic potential in the rhizosphere. New Phytol. 213, 1393–1404. doi:10.1111/nph.14253
Kosakowski, W., Bryszewska, M. A., and Dziugan, P. (2020). Biochars from post-production biomass and waste from wood management: Analysis of carbonization products. Mater. (Basel) 13, 4971. doi:10.3390/ma13214971
Kreitzman, M., Toensmeier, E., Chan, K. M. A., Smukler, S., and Ramankutty, N. (2020). Perennial staple crops: Yields, distribution, and nutrition in the global food system. Front. Sustain. Food Syst. 4, 1–21. doi:10.3389/fsufs.2020.588988
Kunhamu, T. K. (2011). “Jack and agroforestry,” in The jackfruit (Houston, Texas: Stadium Press LLC), 177–189.
Levin, D. B., and Chahine, R. (2010). Challenges for renewable hydrogen production from biomass. Int. J. Hydrogen Energy 35, 4962–4969. doi:10.1016/j.ijhydene.2009.08.067
Long, N. Van, Ngoc, N. Q., Dung, N. N., Kristiansen, P., Yunusa, I., Fyfe, C., et al. (2015). The effects of shade tree types on light variation and robusta coffee production in Vietnam. Engineering 07, 742–753. doi:10.4236/eng.2015.711015
Lukose Kuriakose, S., van Beek, L. P. H., and van Westen, C. J. (2009). Root strength of tropical plants - an investigation in the Western Ghats of Kerala , India. EGU Gen. Assem. 11, 2896.
Majumdar, A., and Deutch, J. (2018). Research opportunities for CO2 utilization and negative emissions at the gigatonne scale. Joule 2, 805–809. doi:10.1016/j.joule.2018.04.018
Makarieva, A. M., Gorshkov, V. G., and Li, B. L. (2009). Precipitation on land versus distance from the ocean: Evidence for a forest pump of atmospheric moisture. Ecol. Complex. 6, 302–307. doi:10.1016/j.ecocom.2008.11.004
Meenanagadi Gramapanchayat (2015). Vikasanarekha, 2015–2016. Meenangadi marches to carbon-neutral tag - The Hindu Available at: https://www.thehindu.com/news/national/kerala/meenangadi-marches-to-carbon-neutral-tag/article17664043.ece (Accessed June 16, 2022).
Mitchell, A. L., Rosenqvist, A., and Mora, B. (2017). Current remote sensing approaches to monitoring forest degradation in support of countries measurement, reporting and verification (MRV) systems for REDD+. Carbon Balance Manag. 12, 9. doi:10.1186/s13021-017-0078-9
Moguel, P., and Toledo, V. M. (1999). Biodiversity conservation in traditional coffee systems of Mexico. Conserv. Biol. 13, 11–21. doi:10.1046/j.1523-1739.1999.97153.x
Mohan, D., Pittman, C. U., and Steele, P. H. (2006). Pyrolysis of wood/biomass for bio-oil: A critical review. Energy fuels. 20, 848–889. doi:10.1021/ef0502397
Morton, J. F. (1987). Jackfruit in: Fruits of warm climates. Miami, FL. MSSRF CAbC – Regional Centre of MSSRF Available at: http://mssrfcabc.res.in/ (Accessed June 13, 2022).
Muschler, R. G. (2001). Shade improves coffee quality in a sub-optimal coffee-zone of Costa Rica. Agrofor. Syst. 51, 131–139. doi:10.1023/A:1010603320653
Nair, P. K. R. (2011). Agroforestry systems and environmental quality: Introduction. J. Environ. Qual. 40, 784–790. doi:10.2134/jeq2011.0076
Nair, P. K. R. (1993). The history of agroforestry. Introd. Agrofor., 3–12. doi:10.1007/978-94-011-1608-4_1
Nandakishor, T. M., Gopi, G., Champatan, V., Sukesh, A., and Aravind, P. V ( (2022). Agroforestry in shade coffee plantations as an emission reduction strategy for tropical regions : Public acceptance and the role of tree banking. Front. Energy Res. 10, 1–11. doi:10.3389/fenrg.2022.758372
Negative Emissions Technologies and Reliable Sequestration (2019). Negative emissions technologies and reliable sequestration. Washington, DC: National Academies Press. doi:10.17226/25259
Nesper, M., Kueffer, C., Krishnan, S., Kushalappa, C. G., and Ghazoul, J. (2017). Shade tree diversity enhances coffee production and quality in agroforestry systems in the Western Ghats. Agric. Ecosyst. Environ. 247, 172–181. doi:10.1016/j.agee.2017.06.024
New Scientist (2019). New scientist. Available at: https://www.newscientist.com/article/2227541-cop25-climate-summit-ends-in-staggering-failure-of-leadership/ (Accessed May 17, 2021).
Orwa, C., Mutua, A., Kindt, R., and Jamnadass, R. (2009). Erythrina edulis. Agroforestry database: A tree species reference and selection guide version 4.0. Agrofor. Database 1–5.
Pandya, I. Y., Salvi, H., Chahar, O., and Vaghela, N. (2013). Quantitative analysis on carbon storage of 25 valuable tree species of Gujarat , incredible India. Indian J. Sci. Res. 4, 137–141.
Peisen, D. J., Schulz, C. L., Golaszewski, R. S., Ballard, B. D., and Smith, J. J. (1999). Case studies : Time required to mature aeronautic technologies to operational readiness. Pennsylvania: Jenkintown.
Piñeiro, V., Arias, J., Dürr, J., Elverdin, P., Ibáñez, A. M., Kinengyere, A., et al. (2020). A scoping review on incentives for adoption of sustainable agricultural practices and their outcomes. Nat. Sustain. 3, 809–820. doi:10.1038/s41893-020-00617-y
Rahaman, M. A., Rahman, A., Miah, M. G., Hoque, M. A., and Rahman, M. M. (2018). Productivity and profitability of jackfruit-eggplant agroforestry system in the terrace ecosystem of Bangladesh. Turk. JAF. Sci. Tech. 6, 124. doi:10.24925/turjaf.v6i2.124-129.1330
Raghuramulu, Y. (2005). Significance of shade for sustainable coffee production in India. Indian Coffee, 21–24.
Raintree, J. B. (1985). , shifting cultivation and sustainable agricul. Available at: https://www.fao.org/3/50630e/50630e02.htm#agroforestry pathways: land tenure (Accessed June 13, 2022). Agroforestry pathways: Land tenure, shifting cultivation and sustainable agricultureUnasylva - No. 154 - Agrofor. - Pathw.
Rajeesh, Abhinand (2021). Tree monitoring system for carbon neutral wayanad. The APJ Abdul Kalam Technological University.
Roodari, A., Hrachowitz, M., Hassanpour, F., and Yaghoobzadeh, M. (2021). Signatures of human intervention – or not? Downstream intensification of hydrological drought along a large central asian river: The individual roles of climate variability and land use change. Hydrol. Earth Syst. Sci. 25, 1943–1967. doi:10.5194/hess-25-1943-2021
Rose, S. K., Ahammad, H., Eickhout, B., Fisher, B., Kurosawa, A., Rao, S., et al. (2012). Land-based mitigation in climate stabilization. Energy Econ. 34, 365–380. doi:10.1016/j.eneco.2011.06.004
Saadabadi, S. A., Illathukandy, B., and Aravind, P. V. (2021). Direct internal methane reforming in biogas fuelled solid oxide fuel cell; the influence of operating parameters. Energy Sci. Eng. 1, 1232–1248. –17. doi:10.1002/ese3.887
Sahoo, K., Bilek, E., Bergman, R., and Mani, S. (2019). Techno-economic analysis of producing solid biofuels and biochar from forest residues using portable systems. Appl. Energy 235, 578–590. doi:10.1016/j.apenergy.2018.10.076
Sandanayake, M., Lokuge, W., Zhang, G., Setunge, S., and Thushar, Q. (2018). Greenhouse gas emissions during timber and concrete building construction —a scenario based comparative case study. Sustain. Cities Soc. 38, 91–97. doi:10.1016/j.scs.2017.12.017
Shahrier, F., Eva, I. J., Afrin, M., Alam, C. S., and Rashid, A. R. M. H. (2020). Literature review on LCA of LPG as a transportation and cooking fuel. Proc. Int. Conf. Ind. Mech. Eng. Oper. Manag.
Shi, S., Zhang, W., Zhang, P., Yu, Y., and Ding, F. (2013). A synthesis of change in deep soil organic carbon stores with afforestation of agricultural soils. For. Ecol. Manage. 296, 53–63. doi:10.1016/j.foreco.2013.01.026
Skullestad, J. L., Bohne, R. A., and Lohne, J. (2016). High-rise timber buildings as a climate change mitigation measure - a comparative LCA of structural system Alternatives. Energy Procedia 96, 112–123. doi:10.1016/j.egypro.2016.09.112
Smith, I., and Snow, M. A. (2008). Timber: An ancient construction material with a bright future. For. Chron. 84, 504–510. doi:10.5558/tfc84504-4
Smith, P., Haberl, H., Popp, A., Erb, K. H., Lauk, C., Harper, R., et al. (2013). How much land-based greenhouse gas mitigation can be achieved without compromising food security and environmental goals? Glob. Chang. Biol. 19, 2285–2302. doi:10.1111/gcb.12160
Söderholm, P. (2020). The green economy transition: The challenges of technological change for sustainability. Sustain. Earth 3, 6. doi:10.1186/s42055-020-00029-y
Speirs, J., McGlade, C., and Slade, R. (2015). Uncertainty in the availability of natural resources: Fossil fuels, critical metals and biomass. Energy Policy 87, 654–664. doi:10.1016/j.enpol.2015.02.031
Tang, L., and Shao, G. (2015). Drone remote sensing for forestry research and practices. J. For. Res. 26, 791–797. doi:10.1007/s11676-015-0088-y
Thanuja, P., and Singh, N. K. (2017). An economic analysis of cost and returns of coffee production in Kodagu district of Karnataka. Int. Res. J. Agric. Econ. Stat. 8, 366–375. doi:10.15740/has/irjaes/8.2/366-375
The Guardian (2020). The guardian. Available at: https://www.theguardian.com/global-development/2020/dec/28/how-a-tree-mortgage-scheme-could-turn-an-indian-town-carbon-neutral (Accessed May 18, 2021).
The Indian Express (2018). The Indian express. Available at: https://indianexpress.com/article/india/jackfruit-to-be-keralas-state-fruit-declaration-on-march-21-5101170/ (Accessed May 17, 2021).
The New Indian Express (2020). The new Indian express. Available at: https://www.newindianexpress.com/world/2020/may/18/kerala-grown-superfood-jackfruit-goes-global-as-a-meat-substitute-2144909.html (Accessed October 13, 2020).
Tiefenbacher, A., Sandén, T., Haslmayr, H.-P., Miloczki, J., Wenzel, W., Spiegel, H., et al. (2021). Optimizing carbon sequestration in croplands: A synthesis. Agronomy 11, 882. doi:10.3390/agronomy11050882
Time (2018). Available at: https://time.com/5318245/coffee-industry-climate-change/(Accessed June 11, 2021).
Usva, K., Sinkko, T., Silvenius, F., Riipi, I., and Heusala, H. (2020). Carbon and water footprint of coffee consumed in Finland—Life cycle assessment. Int. J. Life Cycle Assess. 25, 1976–1990. doi:10.1007/s11367-020-01799-5
van Biert, L., Godjevac, M., Visser, K., and Aravind, P. V. (2019). Dynamic modelling of a direct internal reforming solid oxide fuel cell stack based on single cell experiments. Appl. Energy 250, 976–990. doi:10.1016/j.apenergy.2019.05.053
Vasanth, P., Roekaerts, D., Dupont, C., Kaushal, P., John, T. D., and Pv, A. (2021). Biochar production technologies for soil carbon amendment in rural areas in developing countries: A step towards carbon neutrality. (Abstract accepted, manuscript in preparation).
Vijay, V., Shreedhar, S., Adlak, K., Payyanad, S., Sreedharan, V., Gopi, G., et al. (2021). Review of large-scale biochar field-trials for soil amendment and the observed influences on crop yield variations. Front. Energy Res. 9, 1–21. doi:10.3389/fenrg.2021.710766
Wasajja, H., Al-muraisy, S. A. A., Piaggio, A. L., Ceron-chafla, P., Aravind, P. V., Spanjers, H., et al. (2021). Improvement of biogas quality and quantity for small-scale biogas-electricity generation application in off-grid settings : A field-based study.
Why current negative-emissions strategies remain (2018). )Why current negative-emissions strategies remain ‘magical thinking’. Nature 554, 404. doi:10.1038/d41586-018-02184-x
WRI India (2021). Exploring carbon neutral development India’s subnational regions. Available at: https://wri-india.org/blog/exploring-carbon-neutral-development-india’s-subnational-regions (Accessed May 18, 2021).
Keywords: climate–change, negative emission technologies, biochar, agroforesry systems, carbon sequeatration, sustainable agriculture, bioenergy
Citation: Aravind PV, Champatan V, Gopi G, Vijay V, Smit C, Pande S, van den Broeke LJP, John TD, Illathukandy B, Sukesh A, Shreedhar S, Nandakishor TM, Purushothaman SJ, Posada J, Lindeboom REF and Nampoothiri KUK (2022) Negative emissions at negative cost-an opportunity for a scalable niche. Front. Energy Res. 10:806435. doi: 10.3389/fenrg.2022.806435
Received: 31 October 2021; Accepted: 05 July 2022;
Published: 26 August 2022.
Edited by:
Anne Mae Gaffney, Idaho National Laboratory (DOE), United StatesReviewed by:
Gerardo J. Ruiz-Mercado, United States Environmental Protection Agency (EPA), United StatesRafael Capaz, Federal University of Itajubá, Brazil
Luis Rincon, Food and Agriculture Organization of the United Nations, Italy
Sapan Thapar, TERI School of Advanced Studies (TERI SAS), India
Copyright © 2022 Aravind, Champatan, Gopi, Vijay, Smit, Pande, van den Broeke, John, Illathukandy, Sukesh, Shreedhar, Nandakishor, Purushothaman, Posada, Lindeboom and Nampoothiri. This is an open-access article distributed under the terms of the Creative Commons Attribution License (CC BY). The use, distribution or reproduction in other forums is permitted, provided the original author(s) and the copyright owner(s) are credited and that the original publication in this journal is cited, in accordance with accepted academic practice. No use, distribution or reproduction is permitted which does not comply with these terms.
*Correspondence: P. V. Aravind, A.PurushothamanVellayani@tudelft.nl