- Laboratory- 215, Department of Microbiology, University of Dhaka, Dhaka, Bangladesh
Bacillus thuringiensis (Bt) biopesticides, a recognized eco-friendly pest control agent, can be used to reduce many problems associated with indiscriminate use of chemical pesticides such as environmental pollutions, public health problems, emergence of resistance among pests in many developing countries etc. Bt strains were, therefore, isolated from different ecosystems of Bangladesh and characterized based on biochemical typing, 16S rRNA gene analysis, plasmid and cry genes profiles. Bt index was calculated 0.86 in this study and variations in abundance and distribution pattern of 16 different biotypes were demonstrated within 316 indigenous Bt strains which was compared to the other parts of the world. Bt indiana (17.8%), Bt kurstaki (16.7%), and Bt thuringiensis (12.7%) were found to be the most prevalent in Bangladesh among other biotypes. Hemolytic activity was variable among the biotypes and it was maximum for Bt biotype 10 (100%). Plasmids in the biotypes indiana, kurstaki, thuringiensis, and israelensis were observed to occupy a wider range than other biotypes. The screening for insecticidal genes viz. cry1, cry2, cry3, cry4A, cry8, cry9, cry10, and cry11 in the native Bt strains revealed their presence in varied proportion rendering cry1, cry2, and cry3 the most abundant. The abundance of Bt strains, their diversities and the cry genes profile were thus analyzed in this study which will be the basis for further research development with Bt biopesticide in Bangladesh.
Introduction
Pesticides are poisonous chemicals designed to impair the immune, reproductive, or nervous system of insects and killing thereby to protect agricultural and industrial products. Since an ideal pesticide must be lethal to the targeted pests, not to the non-target species including human, most of the chemical pesticide do not fall into this category because a wide range of acute and chronic human health problems, unfortunately, are linked to the chemical pesticides. Acute health effects appear shortly after the exposure to these pesticides such as skin and eye irritations, headaches, dizziness and nausea, weakness, breathing difficulty, mental confusion and disorientation, seizures, coma, and even death. Chronic health effects normally appear months or years after the exposure even at minimal level in the environment or from the ingested food and water contaminated with pesticide residues. These include disorders with nervous, reproductive or immune system and cancer as well (Aktar et al., 2009).
The high risk groups exposed to pesticides include production workers, formulators, sprayers, mixers, loaders, and agricultural farm workers. The epidemiological evidences suggest significantly higher rate of cancer incidences among farmers and farm workers in the US and Europe than among non-farm workers in some areas (Cantor et al., 1992). There are strong evidences for associations between lymphomas and soft-tissue sarcomas and certain herbicides (Zahm et al., 1990) as well as between lung cancer and exposure to organochlorine insecticides (Pesatori et al., 1994) in these high-risk populations. The annual occurrence of world-wide pesticide poisonings was estimated three million which resulted in 220,000 deaths (WHO, 1992).
On the other hand, pesticides damage the agricultural land firstly by harming beneficial insect species, soil microorganisms and worms which naturally limit pest populations and maintain soil health, secondly by weakening plant root systems and immune systems and finally reducing concentrations of essential plant nutrients in the soil such as nitrogen and phosphorous (The toxic action centre, 2012).
The consumption of chemical pesticides in Bangladesh has increased from 7350 metric tons in 1992–16,200 metric tons in 2001, more than doubling in the past decade and major chemical pesticides currently in use are, organophosphate, carbamate, pyrethroid, 2-dimethylaminopropane-1,3-dithiol, dithiocarbamate etc (Meisner and Dasgupta, 2004). A substantial body of anecdotal evidence also suggests that pesticide poisonings and ecological damage have become commonplace in Bangladesh (Jackson, 1992; Ramaswamy, 1992).
The contamination rate of pesticide is undoubtedly higher for fruits and vegetables because these foods receive the highest dosage of pesticides. In fact, it has been shown in a study performed by USDA that some pesticide residue remains in fruits and vegetables even after they have been washed, peeled, or cored (Wiles and Campbell, 1994).
So, alternatives of chemical pesticides remain obligatory and one of the most promising alternatives is the use of biological control agents or biopesticides from entomopathogenic microorganisms i.e., Bacillus thuringiensis (Bt). Bt is a gram-positive soil dwelling bacterium that synthesizes proteinaceous insecticidal crystals or δ-endotoxins during sporulation which can specifically kill insects belonging to the Lepidoptera, Coleoptera, Diptera, Hymenoptera, Hemiptera, and Mallophaga as well as some invertebrates (Ben-Dov et al., 1997; Schnepf et al., 1998; Feitelson et al., 1999). It has been successfully used for last few decades for its advantages like specific toxicity against target insects, lack of polluting residues and safety to non-target organisms such as mammals, birds, amphibians and reptiles etc. It has been used in agriculture, forestry and mosquito control and accounts for 95% of the 1% market share of biopesticides in the total pesticide market (Flexner and Belnavis, 1999). Over last 15 years, the global area devoted for production of transgenic Bt crops has got a rapid increase to more than 69 million hectares in 2012. It has been reported that insects can evolve resistance rapidly to Bt crops that are not high dose and raise concerns about the adequacy of current resistance management strategies (Gassmann et al., 2014).
Hence, the scopes and applications of Bt are increasing with their diversities around the world. Continuous searching for more and more Bt with diversities, therefore, keeps utmost importance and in this connection, Bt was isolated from different locations of Bangladesh to analyze the abundance and diversity with respect to phenotypic and genotypic characteristics.
Materials and Methods
Bacterial Strains
Bt kurstaki HD-73, B. thuringiensis sotto, B. thuringiensis japonensis Buibui were used as reference strains and were obtained from Bt stock collection of Okayama University, Japan.
Sampling and Isolation of Bacillus thuringiensis
Samples, comprising of soil, leaf, insect cadavers and stored product dust, were collected from different locations of Bangladesh (Figure 1) where Bt biopesticide was not applied before and sampling sites encompassed different ecosystems such as plane land, river basin, hill tracts, sea beaches etc. Soil (about 10.0 g each) samples were collected from 2 to 5 cm below the surface of shadowed and slightly moistened places, generally not exposed to sunlight with sanitized spatula into the sterile plastic bags to avoid contamination. Leaves, insects and stored dust products were also collected aseptically. Samples were kept at room temperature in an assigned dry place of laboratory. Then 1.0 g of each soil sample (for leaf, 1 piece in each flask and for each insect, gut was dissected out, crushed and mixed into the medium) was added in a 125 ml Erlenmeyer flask containing 20 ml of L- broth (per liter: tryptone 10.0 g, yeast extract 5.0 g, NaCl 5.0 g) supplemented with 0.25 M Na-acetate (pH 6.8) and incubated in an orbital shaker at 30°C and 200 rpm. After 4 h, 0.5 ml of suspension was transferred into a sterile test tube and heat treated for 10 min at 80°C in a water bath. Heat treated suspension was then diluted 10-folds and 0.1 ml was inoculated onto T3- agar medium (1.0 L: Tryptone 3.0 g, tryptose 2.0 g, yeast extract 1.5 g, MnCl2 0.005 g, phosphate buffer 50 mM, agar 15.0 g; pH: 6.8) (Travers et al., 1987) by spread plate method and incubated at 30°C. In case of appearance of any colony after overnight incubation, incubation period was extended up to 72 h to allow sporulation. Colonies were then picked and dispersed in sterile distilled water and examined with Phase Contrast Microscope. Colonies comprising of isolates with juxtaposed glowing spore and dark crystal protein as revealed under Phase Contrast Microscope (Primostar, Zeiss, Germany) were considered as Bt or Bacillus sphaericus. To distinguish Bt from Bs, starch hydrolysis test was performed according to the Bergey's Manual of Determinative Bacteriology and isolates with starch hydrolysing ability were identified as Bt. The diameter of spores was measured using the software ZEN (Zeiss, Germany).
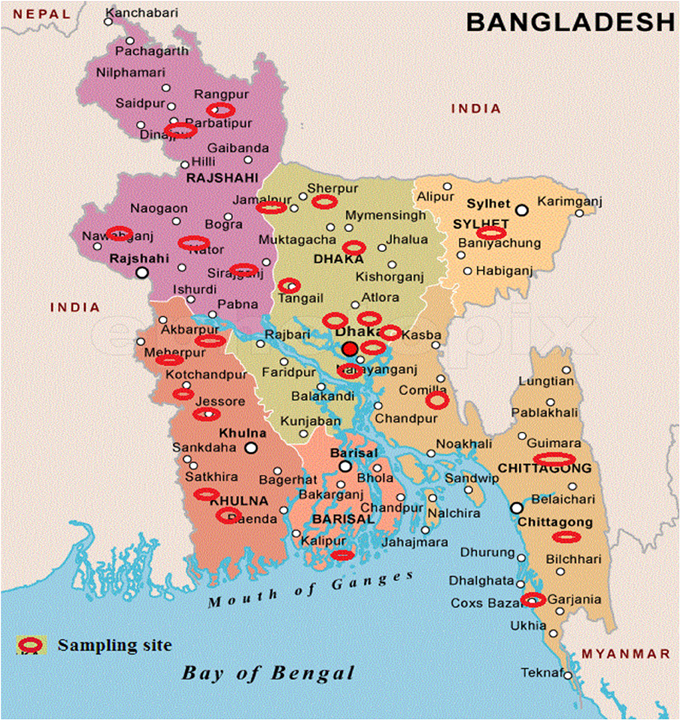
Figure 1. Sampling sites to isolate Bacillus thuringiensis from Bangladesh. Sampling sites are indicated by red eclipses over the map.
Hemolytic Test
Hemolytic test was performed to identify Bt isolates producing broad-spectrum cytolysins. Bt isolates were therefore inoculated (as dots with needle) onto Blood agar medium (nutrient agar containing 5% (v/v) sheep erythrocytes) and incubated overnight at 27°C. Isolates forming clear zone of hemolysis around their colonies were considered as hemolytic (Ichikawa et al., 2008).
Biochemical Typing
Four most relevant biochemical tests i.e., esculin utilization, acid formation from salicin and sucrose, and lecithinase production were carried out to classify Bt into 16 biotypes (Martin and Travers, 1989).
Total DNA Preparation
Total DNA was prepared from the single colonies of indigenous Bt isolates obtained after incubation by streaking on LB agar medium (Bravo et al., 1998). After 12 h of incubation at 30°C, a single colony was transferred into 100 μl sterile de-ionized water in a microfuge tube, vortexed and kept for 20 min at −70°C. It was then incubated in boiling water for 10 min to lyse the cells and briefly centrifuged for 20 s at 12,000 ×g. The upper aqueous phase transferred into sterile microfuge tubes was used as template and preserved at −20°C for further use. Fifty to Hundred nanograms of DNA from this suspension was used as template in PCR.
16S rRNA Gene Analysis
PCR amplification of 16S rRNA gene from indigenous Bt isolates was performed with universal primers for Bacillus spp.: fwd (20F); 5′-GAGTTTGATCCTGGCTCAG-3′ (position 9–27), and rev (1500R); 5′-GTTACCTTGTTACGACTT-3′ (position 1492–1509). The PCR was performed in a thermal cycler (MJ, Bio-Rad) by 35 cycles (96°C for 50 s, 50°C for 45 s, 72°C for 2 min) with an initial denaturation step at 96°C for 10 min and a final extension step at 72°C for 10 min in 25 μl reaction mixture (forward and reverse primers 0.5 μM each, 50–100 ng of template, 0.5 U of Taq DNA polymerase (Promega, USA), 200 μM dNTPs, 10 mM Tris, 50 mM KCl and 1.5 mM MgCl2).
PCR product (5 μl) was analyzed in 1% (w/v) Agarose (Promega, USA) gel electrophoresis at 60 V for 1 h in 1×TBE buffer and visualized against UV trans-illumination in a gel documentation system (Alpha imager mini, USA) following staining in EtBr (Sigma, USA) solution (0.5 μg/ ml) and destaining in distilled water. Purified PCR products (Wizard® SV Gel and PCR Clean-Up System, Promega, USA) were sequenced at CARS, University of Dhaka, Bangladesh and the sequences were submitted in the NCBI database (Table 3).
Plasmid Profiling
Plasmid DNA was prepared by using the alkaline lysis method (Crosa and Falkow, 1981). Briefly, the pellet of a 5.0 ml culture grown in LB broth was lysed with 0.85 ml of TE buffer (50 mM Tris, 20 mM EDTA; pH 8.5) containing 2.0 mg/ml of lysozyme (Wako, Japan), 0.05 ml of 20% SDS solution, and 5.0 U proteinase-K (Nacalai tesque, inc, Japan). After mixing by gentle inversions, the cell suspension was incubated at 37°C for 30 min. 0.03 ml of 3.0 N NaOH was added subsequently to the suspension and mixed gently for 3 min. The suspension was neutralized by addition of 0.06 ml of 2.0 M Tris-HCl (pH 7.0) and mixed gently. Then 0.1 ml of 5.0 M NaCl was added, and the suspension was mixed by inversions, placed on ice for 15 min, and then centrifuged at 12,000 ×g for 15 min at 4°C (Tomy, MX-305, high speed Refrigerated micro centrifuge, Japan). The supernatant was transferred into a fresh centrifuge tube, and 2 volume of ice-cold ethanol was added. The tube was kept at −20°C for 15 min and then centrifuged at 12,000 ×g for 15 min. The supernatant was discarded, and the residue was dried by inverting the tube over a paper towel for a few minutes. The residue was dissolved in 50 μl of TE buffer (10 mM Tris, 1 mM EDTA) and kept at −20°C. Plasmid DNA was analyzed by electrophoresis on horizontal 0.8% agarose (Promega, USA) gel submerged in 1×TBE buffer at 70 V for 3 h. Gel was visualized in a gel documentation system following staining and de-staining.
Oligonucleotide Primers for PCR
Previously described primers for cry1, cry4A, cry8, cry10, and cry11 genes were used in this study (Table 1) whereas the primers for cry2, cry3, and cry9 genes were designed with the help of Primer-BLAST program from the conserved regions of respective gene sequences obtained from NCBI database.
Detection of cry Genes
DNA templates from Bt strains were mixed with PCR reaction mixture i.e., per μl containing 0.2 mM dNTPs, 0.5 μM of each primer, 1×PCR buffer and 0.5 U of Taq DNA polymerase (Promega, USA) in 25 μl reaction volume and amplification was performed in a DNA thermal cycler (MJ, Bio-Rad). For all primer sets, PCR was carried out with an initial single denaturation step at 95°C for 2 min and 30 amplification cycles including denaturation at 95°C for 45 s, annealing at temperatures specific for each primer sets (Table 1) for 45 s and extension at 72°C for 60 s. Finally an extra extension step was applied at 72°C for 10 min. PCR products (10 μl) were then electrophoresed in 1.5% agarose (Promega, USA) gel prepared and submerged in 1×TBE buffer at 60 V for 60 min. Gel was visualized in a gel documentation system following staining and de-staining.
Results
Distribution of Bacillus thuringiensis
Total 231 samples from 26 districts encompassing 6 different regions of Bangladesh (Figure 1) were processed and after acetate selection and heat treatment, 366 isolates were found to synthesis crystal protein during sporulation by Phase Contrast Microscopy (Figure 2). Among them, 317 isolates were distinguished as Bt for their starch hydrolysing capability and rest of them were considered Bacillus sphaericus. The distribution analysis of Bt in Bangladesh (Table 2) indicates that almost all parts of Bangladesh are rich in Bt as the average Bt index was calculated 0.86. Bt was most abundant in the Northern and Central part (index 0.90 and 0.89 respectively) and the least abundant was in the sandy beach (index- 0.73) (Table 2).
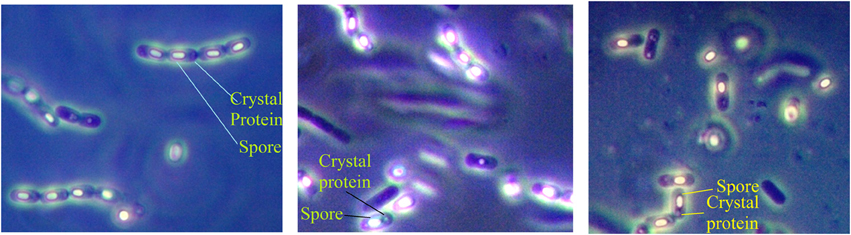
Figure 2. Examination of Bacterial isolates from the colonies incubated up to 72 h to allow sporulation by Phase contrast microscopy. Glowing spores and juxtaposed crystal protein as revealed under Phase Contrast microscope rendered them as Bacillus thuringiensis strains.
The spore diameters of indigenous Bt from Bangladesh were observed to be in the range of 0.6–1.02 μm and the average spore diameter was calculated to be 0.76 ± 0.097 μm whereas it was 0.63 ± 0.081 μm for reference Btk HD-73 in this study.
Prevalence of Biotypes
Biochemical typing helped in classification of indigenous Bt strains into 16 biotypes. From this study, B. thuringiensis indiana (17.8%), kurstaki (16.7%), thuringiensis (12.7%) etc. biotypes were found to be the most prevalent in Bangladesh (Figure 3). Bt biotypes 11, sotto, 9, 13 and israelensis were almost equal in their abundances (5% of total).
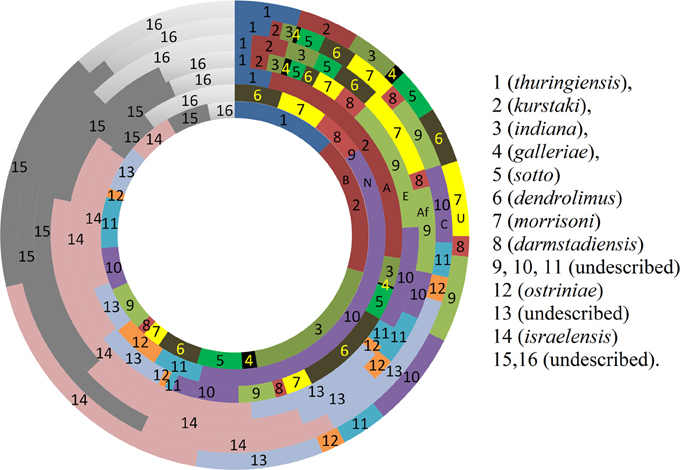
Figure 3. Comparison of prevalence (%) pattern of different biotypes around the world. (Rings from centre to periphery: B, Bangladesh; N, New Zealand; A, Asia; E, Europe; Af, Africa; C, Central and South America; U, United States).
The distribution of different biotypes in different sampling sites was also analyzed. The distribution pattern implies that almost all biotypes are present in the sampling sites in an unbiased manner except few. Bt thuringiensis found to be abundant in the central zone of Bangladesh and Bt kurstaki was abundant in Jhenidah. However, the distribution pattern of Bt biotypes seemed unique when compared with the rest of the world (Figure 3). Bt thuringiensis, kurstaki and indiana together account for 47% of the total isolates of Bangladesh whereas it is 0, 30, 4.4, 6.9, 4.6, and 12% for New Zealand, other countries of Asia, Europe, Africa, Central & South America and United States respectively (Martin and Travers, 1989). Abundance of Bt israelensis is low in Bangladesh whereas it is remarkable in other parts of the world.
Broad Spectrum Cytolysin Activity
Three hundred and seventeen Bt strains were tested for broad spectrum cytolysin activity and 58.36% (189) of them were found to have this property hence presumed as insecticidal and rest 41.64% can be explored for anti-cancer cell protein, parasporin. Based on this property, percentages of insecticidal strains in each biotype were also determined (Figure 4). The order of percentage of insecticidal strains was thus made- Bt 10> Bt kurstaki> Bt dendrolimus> Bt thuringiensis> Bt 9> Bt indiana> Bt israelensis.
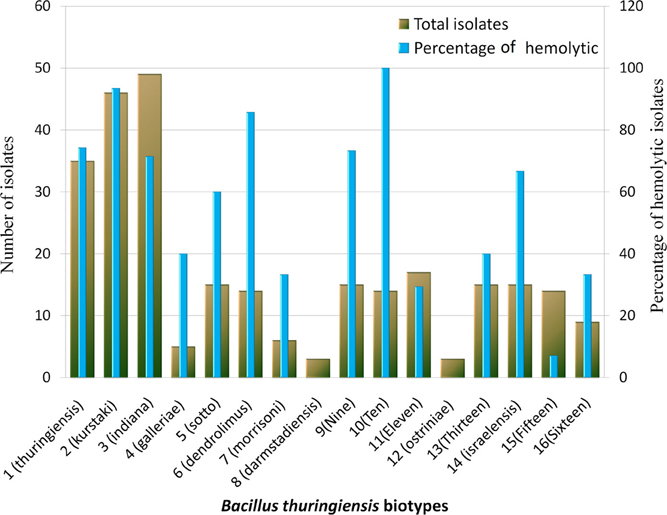
Figure 4. Distribution of hemolytic Bacillus thuringiensis strains in different biotypes. Figure shows the number of isolate and the percentage of isolates with Broad spectrum cytolytic activity in each biotype which was determined by Hemolytic test.
16S rRNA Gene Sequence Analysis
Amplicons of about 1500 bp were obtained from PCR amplification of 16S rRNA gene of indigenous Bt isolates (Figure 5). The sequences obtained from the amplicons were used for nucleotide blast in NCBI database (http://www.ncbi.nlm.nih.gov/) and the sequences producing significant alignments to them with more than 96% identity were observed to be from different strains of Bt. GenBank Accession numbers for these strains are provided (Table 3).
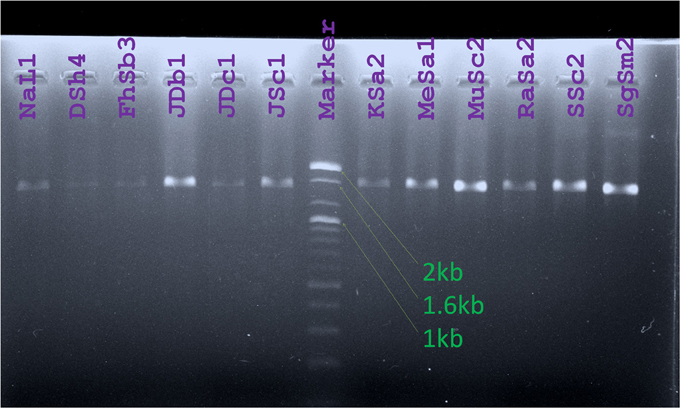
Figure 5. Amplicons obtained from PCR for 16S rRNA gene amplification were visualized against UV light after Agarose gel electrophoresis. Lanes were labeled with the names of Bt strains studied. (Marker: 100 bp DNA ladder, Bioneer, Korea).
Diversity in Plasmid Profile
Diversity was observed in the plasmid profiles of indigenous Bt strains. Clearly visible supercoiled plasmids were present in the range of 3 kb to more than 16 kb. 81% of the visible plasmid bands fall into the range of 10–13 kb (Figure 6). The pattern for small plasmids was compared and molecular weight based distribution of plasmids among the biotypes was determined which in other sense renders the diversity too. Plasmids in Bt thuringiensis, kurstaki, indiana, and israelensis exhibited more diversity as the plasmids from these biotypes were present in the range of 3 to more than 16 kb (Figure 7).
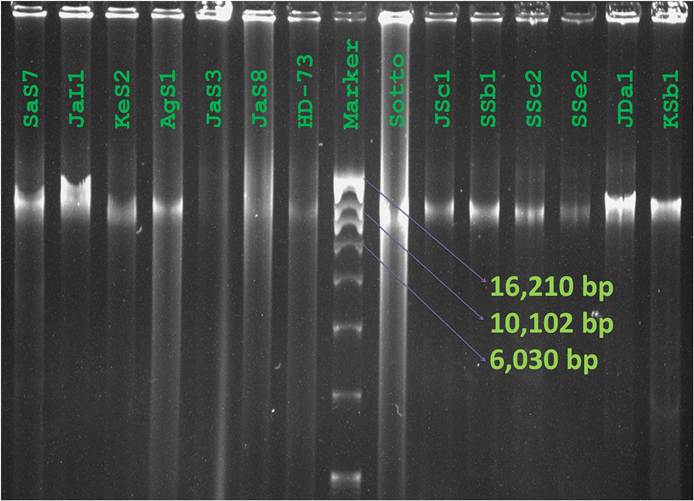
Figure 6. Plasmid profiles of Bacillus thuringiensis strains of Bangladesh. Image of Plasmid DNA was captured as visualized against UV light after agarose gel electrophoresis and staining with ethidium bromide. Names of the isolates and reference strain were labeled over lanes (Marker: Supercoiled DNA ladder, Invitrogen, USA).
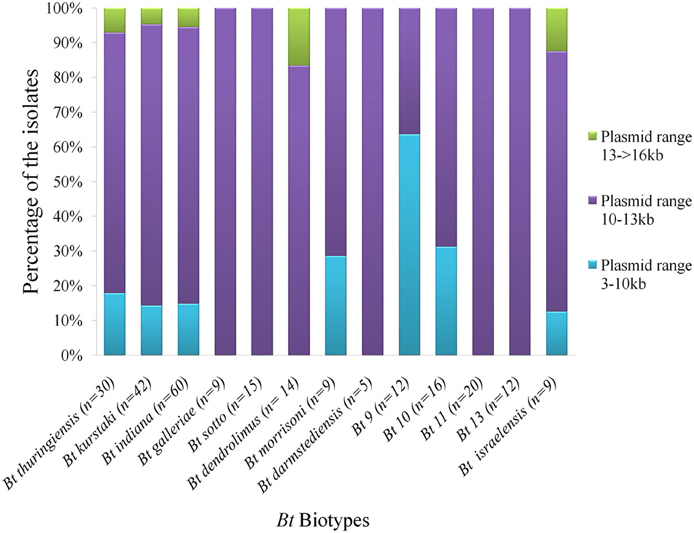
Figure 7. Distribution of plasmids based on molecular sizes in the prevailing Bt biotypes of Bangladesh. “n” is the number of isolates from each biotypes used in plasmid profiling. Three different colors indicate graphically the percentage of isolates containing plasmids of 3 different ranges.
cry Gene Profile
Upon electrophoresis of PCR products in 1.5% Agarose gel, amplicons for the target sequences of cry1, cry2, cry3, cry4A, cry8, cry9, and cry10 genes were observed in the native Bt strains (Figure 8). Bt strains that produced amplicons of desired sizes (Table 1), were considered positive for respective genes. The abundances of targeted cry genes in the Bt of Bangladesh were calculated (Figure 9) and cry1 gene was the most abundant with (30.8%) followed by cry2 (25.5%), cry3 (22.2%), and cry9 (7.2%) genes. Abundance of cry4A and cry10 genes were less than 5% and no strains with cry11 gene was found.
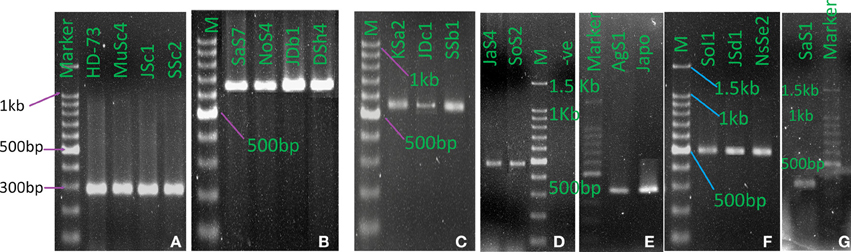
Figure 8. cry genes profile of indigenous Bacillus thuringiensis. (A) cry1 (277 bp), (B). cry2 (639 bp), (C) cry3 (525 bp), (D) cry4A (459 bp), (E) cry8 (376 bp), (F) cry9 (492 bp), (G) cry10 (348 bp). (M: 100 bp DNA Marker, Takara, Japan. HD-73: B. thuringiensis kurstaki HD-73, japo: Bt japonensis Buibui).
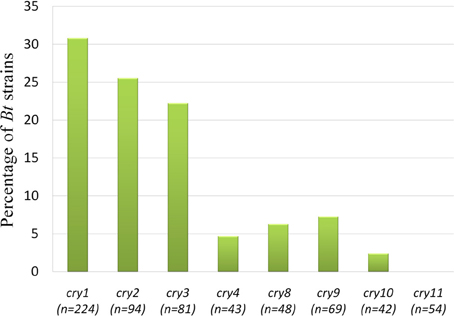
Figure 9. Prevalence rate of cry genes in the Bacillus thuringiensis strains of Bangladesh. “n” indicates the number of isolates tested for that particular gene. “n” was determined as subspecies and/or biotype based analysis of cry genes were performed.
Discussion
Continuous searching for Bt strains expressing toxins with novel and diverse activity keeps tremendous importance for replacing the prevailing chemical pesticides as well as to fight the pest resistance. The research was, therefore, carried out with a view to develop a Bt collection source characterized with their genetic and toxic properties leading to biopesticide production.
Sample was hence collected with the objectives of deducing the abundance, distribution and diversity of Bt in different eco-systems of Bangladesh and the cry gene profiles. Sampling was performed from 26 different districts of Bangladesh that covered plane lands, river basin, hilly regions, sandy beaches and agricultural lands (Figure 1). Two hundred and thirty one samples were processed with acetate selection and heat treatment so that the spores other than Bt are germinated and heat-killed. Then the left alone Bt spores were allowed to germinate in T3- agar medium for overnight which was extended up to 72 h for sporulation as crystal proteins are synthesized during this period. Phase contrast microscopy was performed to identify the isolates producing crystal proteins (Figure 2) which produced 366 isolates with this property.
It was discussed that Bacillus sphaericus responded similarly in this isolation process but was distinguished by Phase contrast microscopy as lacking parasporal crystal proteins (Travers et al., 1987). Again some strains of Bacillus sphaericus synthesize a parasporal inclusion or crystal which contains proteins toxic against the larvae of a variety of mosquito species (Baumann et al., 1991). So these ambiguities should be resolved for confident discrimination of Bt from Bs. According to the Bergey's Manual of Determinative Bacteriology B. thuringiensis can be distinguished from B. sphaericus by starch hydrolysis test and this test was performed in this study. Isolates with starch hydrolyzing ability were identified as Bt. Upon starch hydrolysis test, 317 out of 366 parasporal crystal protein synthesizing isolates were confirmed as Bt.
The spore diameter of native Bt strains ranged from 0.6 to 1.02 μm and the average spore diameter was calculated 0.76 ± 0.097 μm in this study and native Bt spores were found 20% bigger than the reference Btk HD-73 (0.63 ± 0.081 μm). Deviation in spore diameters up to ±0.097 for local Bt strains is a clear indication of high diversities among the strains.
Bt index is often used as an indication of how easily the Bt was isolated which was 0.86 for Bangladesh in this study. This index was variable across different ecosystem of Bangladesh. Northern and central parts of the country were indexed higher than the rest parts. Bt was less available in the sandy beaches, yet it was 0.73 (Table 2).
Bt has got diverse functions like bacteriocin, chitinase, Vip toxins, cytocidal proteins and delta endotoxin production. These functions are not common for all and to sort out the strains with specific functions, classification is highly important. Serotyping and biotyping are two established methods for rapid classification into subspecies with a presumptive function.
Biotyping based on esculin and lecithinase hydrolysis as well as salicin and sucrose utilization which are the most variable among different Bt subspecies enabled in devising a simplified system of classification into 16 biochemical types (Martin and Travers, 1989). Ten out of 16 biotypes were described for known subspecies and in this study, further characterization and analysis were performed based on the classification. Biochemical tests results of this study estimated biotypes describing Bt indiana, kurstki, and thuringiensis as the most prevalent in Bangladesh.
The abundance of Bt biotypes around the world (Martin and Travers, 1989) was compared with that of Bangladesh. The abundance pattern in Bangladesh was slightly similar with that of Asian countries but high variations were discernible with other portions of the world (Figure 3). Biotypes 14 (israelensis), 15 and 16 account for 30–58% of all Bt strains all over the world whereas it was 13.8% for Bangladesh. On the other hand, biotype 1 (thuringiensis), 2 (kurstaki), and 3 (indiana) together account for 47.2% of all Bt strains in Bangladesh while only 4.4–12% was observed in the other parts of the world. For Asian countries, it accounts up to 30%. Martin and Travers showed that biotypes not yet described represented 51.9% of the total isolates worldwide (Martin and Travers, 1989). It was calculated 30.5% For Bangladesh in this study.
Again, hemolytic activity or broad spectrum cytolysin activity for presumptive identification of insecticidal isolates was performed with all the Bt isolates obtained. Our previous observation for strains with hemolytic activity was modified from 58.36 to 77.7% as the sampling sites and isolates increased in number (Shishir et al., 2012). The percentage of hemolytic strains in each biotype was calculated. It was found that biotype darmstadiensis and ostriniae possess no hemolytic strains and only 7% of the strains were hemolytic in biotype 15. Less than 40% hemolytic strains were present in biotypes galleriae, morrisoni, 11, 13, 16 and more than 60% hemolytic strains were present in rest of the biotypes (Figure 4). 100% strains from Bt 10, 93% from kurstaki and 85% from dendrolimus were hemolytic. No such relationship was observed between percentage of hemolytic strains and abundance of cry genes in a certain biotype (Data not shown).
Then 16S rRNA gene sequencing was performed with randomly selected 19 strains and 1 reference strains. Sequences obtained following PCR with universal primer set for Bacillus were analyzed and they were identified as Bt by blastn program. It was performed in order to evaluating the isolation process of Bt by acetate selection and starch hydrolysis method.
Usually mega plasmids are present in low copy numbers and small or cryptic plasmids are present in high copy numbers in Bt. So it is likely to observe more small plasmids than mega plasmids in agarose gel electrophoresis. Again depending on the variation in techniques, successful extraction of small plasmids may vary also. Plasmid was therefore extracted with a view to compare their pattern among indigenous Bt strains. In our study, large plasmids were observed in many strains but due to their presence in low copy numbers, the bands were not intense enough. So the small plasmid pattern was compared and molecular weight based distribution of plasmids among the biotypes was determined which in other sense renders the diversity within the biotypes (Figure 7).
From the full list of δ-endotoxins, correlation between the subspecies and cry genes was observed (Crickmore et al., 2012). Based on the information, subspecies based investigation of cry1, cry2, cry3, cry4A, cry8, cry9, cry10, and cry11 genes was performed into the indigenous Bt strains. Both expected and spurious bands were observed and the Bt strains with desired amplicons of about 277, 639, 525, 459, 376, 492, and 348 bp for cry1, cry2, cry3, cry4A, cry8, cry9, and cry10 genes respectively were considered as positive. Spurious products are actually generated if mismatch in priming occurs which enhances the getting of putative novel cry genes as reported in many studies (Jouzani et al., 2008; Bozlağan et al., 2010).
Total 73 Bt strains out of 230 were positive for eight different cry genes examined. The number of Bt strains containing cry1 gene was the maximum (69) as compared to cry2 (24), cry3 (18), cry4A (2), cry8 (3), cry9 (5), and cry10 (1) genes (Figure 9). Bravo et al. (1998) have found cry1 genes the most frequent (49.5%), then cry3 gene as highly abundant (21.7%) and cry9 gene as less abundant (2.6%). These results showed both similarity and dissimilarity across different geographic regions which might affect the diversity of cry gene content of Bt strains. In addition, it is probable that the remaining 158 isolates of this study producing no amplicons for the cry genes examined may contain other genes as 72 different cry gene groups and many subgroups have been defined in the literature to date (Crickmore et al., 2012).
Conflict of Interest Statement
The authors declare that the research was conducted in the absence of any commercial or financial relationships that could be construed as a potential conflict of interest.
Acknowledgments
This work was supported by a Grant-in-Aid from the USDA as a project entitled “Production of Bacillus thuringiensis biopesticides by biotechnological approach for the control of vegetable pests in Bangladesh.” We thank Okayama University, Japan for providing references strains. We also thank CARS, University of Dhaka, Dhaka-1000, Bangladesh for providing sequencing facility.
References
Aktar, M. W., Gupta, D. S., and Chowdhury, A. (2009). Impact of pesticides use in agriculture: their benefits and hazards. Interdiscip. Toxicol. 2, 1–12. doi: 10.2478/v10102-009-0001-7
Baumann, P., Clark, M. A., Baumann, L., and Broadwell, A. H. (1991). Bacillus sphaericus as a mosquito pathogen: properties of the organism and its toxins. Microbiol. Mol. Biol. Rev. 55, 425–436.
Ben-Dov, E. A., Zaritsky, E., Dahan, Z., Barak, R., Sinai, R., Manasherob, A., et al. (1997). Extended screening by PCR for seven cry-group genes from field-collected strains of Bacillus thuringiensis. Appl. Environ. Microbiol. 63, 4883–4890.
Bozlağan, I., Ayvaz, A. R., Ozturk, F., Acik, L., Akbulut, M., and Yilmaz, S. (2010). Detection of the cry1 gene in Bacillus thuringiensis isolates from agricultural fields and their bioactivity against two stored product moth larvae. Turk. J. Agric. For. 34, 145–154. doi: 10.3906/tar-0905-19
Bravo, A., Sarabia, S., Lopez, L., Ontiveros, H., Abarca, C., Ortiz, A., et al. (1998). Characterization of cry genes in a Mexican Bacillus thuringiensis strain collection. Appl. Environ. Microbiol. 64, 4965–4972.
Cantor, K. P., Blair, G., Everett, A., Gibson, R., Burmeister, L. F., Brown, L. M., et al. (1992). Pesticides and other agricultural risk factors for non-Hodgkin's lymphoma among men in Iowa and Minnesota. Cancer Res. 52, 2447–2455.
Crickmore, N., Zeigler, D. R., Schnepf, E., Van, R. J., Lereclus, D., Baum, J., et al. (2012). Bacillus thuringiensis Toxin Nomenclature. Available online at: http://www.lifesci.sussex.ac.uk/Home/Neil_Crickmore/Bt/
Crosa, J. H., and Falkow, S. (1981). “Plasmids,” in Manual of Methods for General Bacteriology, eds P. Gerhardt, R. G. E. Murray, R. N. Costilow, E. W. Nester, W. A. Wood, N. R. Krieg, and G. B. Phillips (Washington, DC: American Society for Microbiology), 266–282.
Feitelson, J. S., Payne, J., and Kim, L. (1999). Bacillus thuringiensis: insects and beyond. Biotechnology 10, 271–275. doi: 10.1038/nbt0392-271
Flexner, J. L., and Belnavis, D. L. (1999). “Microbial insecticides,” in Biological and Biotechnological Control of Insect Pests, eds J. E. Rechcigl and N. A. Rechcigl (Boca Raton, FL: Lewis Publishers), 35–62.
Gassmann, A. J., Petzold-Maxwell, J. L., Clifton, E. H., Dunbar, M. W., Hoffmann, A. M., Ingber, D. A., et al. (2014). Field-evolved resistance by western corn rootworm to multiple Bacillus thuringiensis toxins in transgenic maize. Proc. Natl. Acad. Sci. U.S.A. 111, 5141–5146. doi: 10.1073/pnas.1317179111
Ichikawa, M., Uemori, A., Yasutak, K., Kagoshima, K., Mizuki, E., and Ohba, M. (2008). Failure to phenotypically discriminate between non-insecticidal Bacillus thuringiensis strains with anticancer parasporins (PS2, PS3, and PS4) and Bacillus thuringiensis strains that produce insecticidal Cry proteins. Appl. Entomol. Zool. 43, 421–426. doi: 10.1303/aez.2008.421
Jackson, G. (1992). Agrochemical Usage in Asia Region: A Reference Compendium. Washington, DC: Asia Agriculture Technical Department, The World Bank.
Jouzani, G. S., Pourjan, A. A., Seifinejad, A., Marzban, R., Kariman, K., and Maleki, B. (2008). Distribution and diversity of Dipteran-specific cry and cyt genes in native Bacillus thuringiensis strains obtained from different ecosystems of Iran. J. Ind. Microbiol. Biotechnol. 35, 83–94. doi: 10.1007/s10295-007-0269-6
Martin, P. A. W., and Travers, R. S. (1989). Worldwide abundance and distribution of Bacillus thuringiensis isolates. Appl. Environ. Microbiol. 55, 2437–2442.
Meisner, C., M., and Dasgupta, S. (2004). Identifying Pesticide Hotspots: the Case of Bangladesh. Mimeo. Available online at: http://www.worldbank/nipr
Pesatori, A. C., Sontag, J. M., Lubin, J. H., Consonni, D., and Blair, A. (1994). Cohort mortality and nested case-control study of lung cancer among structural pest control workers in Florida (United States). Cancer Causes Control 5, 310–318. doi: 10.1007/BF01804981
Ramaswamy, S. (1992). Pest Control and Environment. Notes for Discussion at a Seminar on Environment and Agriculture. Dhaka: Agriculturalist Association of Bangladesh.
Schnepf, E., Crickmore, N., Rie, V. J., Lereclus, D., Baum, J., Feitelson, J., et al. (1998). Bacillus thuringiensis and its pesticidal crystal proteins. Microbiol. Mol. Biol. Rev. 62, 775–806.
Shishir, A., Akter, A., Bodiuzzaman, Aktar, N., Rahman, M., Khan, S. A., et al. (2012). “Molecular characterization of indigenous Bacillus thuringiensis kurstaki isolates from Bangladesh and toxicity of Btk HD-73 against melon fruit fly, Bactrocera cucurbitae,” in Paper Presented at the 1st AFSA Conferences on Food Safety and Food Security (Osaka).
The toxic action centre. (2012). Available online at: http://www.toxicsaction.org/problems-and-solutions/pesticides
Travers, R. S., Martin, P. A. W., and Reichelderfer, C. F. (1987). Selective process for efficient isolation of soil Bacillus spp. Appl. Environ. Microbiol. 56, 1263–1266.
WHO. (1992). Our Planet, Our Health: Report of the WHO Commission on Health and Environment. (Geneva: World Health Organization).
Wiles, R., and Campbell, C. (1994). Washed, Peeled–Contaminated: Pesticide Residues in Ready-To-Eat Fruits and Vegetables. (Washington, DC: Environmental Working Group).
Keywords: public health, Bacillus thuringiensis, biotyping, abundance and diversity, cry genes
Citation: Shishir A, Roy A, Islam N, Rahman A, Khan SN and Hoq MM (2014) Abundance and diversity of Bacillus thuringiensis in Bangladesh and their cry genes profile. Front. Environ. Sci. 2:20. doi: 10.3389/fenvs.2014.00020
Received: 06 March 2014; Accepted: 23 May 2014;
Published online: 12 June 2014.
Edited by:
Khwaja M. Sultanul Aziz, Bangladesh Academy of Sciences, BangladeshReviewed by:
Gulnihal Ozbay, Delaware State University, USAWilliam Hsiao, University of British Columbia, Canada
Copyright © 2014 Shishir, Roy, Islam, Rahman, Khan and Hoq. This is an open-access article distributed under the terms of the Creative Commons Attribution License (CC BY). The use, distribution or reproduction in other forums is permitted, provided the original author(s) or licensor are credited and that the original publication in this journal is cited, in accordance with accepted academic practice. No use, distribution or reproduction is permitted which does not comply with these terms.
*Correspondence: Md. Mozammel Hoq, Laboratory- 215, Department of Microbiology, University of Dhaka, Dhaka- 1000, Bangladesh e-mail:bW16aG9xQGdtYWlsLmNvbQ==