- 1Department of Botany, Faculty of Science, Hamdard University, New Delhi, India
- 2Department of Chemistry, CESAM-Centre for Environmental and Marine Studies, University of Aveiro, Aveiro, Portugal
- 3Plant Production Department, College of Food and Agricultural Sciences, King Saud University, Riyadh, Saudi Arabia
Impacts of increasing environmental stresses (such as drought) on crop productivity can be sustainably minimized by using plant-beneficial mineral nutrients (such as sulfur, S). This study, based on a pot-culture experiment conducted in greenhouse condition, investigates S-mediated influence of drought stress (imposed at pre-flowering, flowering, and pod-filling stages) on growth, photosynthesis and tolerance of mungbean (Vigna radiata L.) plants. Drought stress alone hampered photosynthesis functions, enhanced oxidative stress [measured in terms of H2O2; lipid peroxidation (LPO); electrolyte leakage (EL)] and decreased the pools of cellular redox buffers (namely ascorbate (AsA); glutathione (GSH)], and the overall plant growth (measured as leaf area and plant dry mass), maximally at flowering stage, followed by pre-flowering and pod-filling stages. Contrarily, S-supplementation to drought-affected plants (particularly at flowering stage) improved the growth- and photosynthesis-related parameters considerably. This may be ascribed to S-induced enhancements in the pools of reduced AsA and GSH, which jointly manage the balance between the production and scavenging of H2O2 and stabilize cell membrane by decreasing LPO and EL. It is inferred that alleviation of drought-caused oxidative stress depends largely on the status of AsA and GSH via S-supplementation to drought-stressed V. radiata at an appropriate stage of plant growth, when this nutrient is maximally or efficiently utilized.
Introduction
Recognized as one of the major environmental stress factors, and as a main constraint for crop production worldwide, drought affects virtually every aspect of plant growth, physiology and metabolism (Harb et al., 2010). In particular, at the whole-plant level, drought stress affects mainly the plant photosynthetic functions, causing imbalance in “CO2 fixation and electron transport.” This facilitates transfer of electrons to reactive oxygen species (ROS), including H2O2, as a result of over-reduction of the electron-transport-chain components (Anjum et al., 2008a; Lawlor and Tezara, 2009). Additionally, high concentration of ROS causes oxidative damage to photosynthetic pigments, bio-molecules such as lipids, proteins and nucleic acids, and leakage of electrolytes via lipid peroxidation (LPO), leading to cessation of normal plant cellular metabolism (Anjum et al., 2012a). The ascorbate-glutathione (AsA-GSH) pathway constitutes the major part of antioxidant defense system in plants where a number of ROS are effectively metabolized and/or detoxified by a network of reactions involving enzymes and metabolites with redox properties. Both AsA and GSH (tripeptide GSH; γ-glutamate-cysteine-glycine) are cellular redox buffers closely linked in major physiological functions. Nevertheless, in conjunction with other components of AsA-GSH pathway, both AsA and GSH together determine the lifetime of varied ROS and their reaction products within the cellular environment and provide crucial protection against oxidative damage (Anjum et al., 2010, 2012a, 2013). In recent studies, exogenous application of AsA or GSH was reported to help plants to withstand consequences caused by a range of abiotic stresses including Cd (Cai et al., 2011; Son et al., 2014), salinity (Wang et al., 2014) and high temperature (Nahar et al., 2015).
Maintenance of the status of mineral nutrients in plants is important for increasing the crop productivity and plant resistance to environmental stress (Cakmak, 2005; Anjum et al., 2008b, 2012b). The cumulative role of mineral nutrients in modulating cellular levels of AsA and GSH, and in strengthening the plant antioxidant defense system has been discussed extensively (Anjum et al., 2010, 2012a, 2013; Gill et al., 2011). Sulfur (S) is the fourth essential macronutrient for plants, after N, P and K, and plays a vital role in the regulation of plant growth, development and productivity (Hawkesford, 2000), via affecting leaf chlorophyll, N content and photosynthetic enzymes. Sulfur is required for protein synthesis, incorporated into organic molecules in plants, and is located in thiol (−SH) groups in proteins (cysteine-residues) or non-protein thiols (glutathione, GSH), the potential modulators of stress response (Anjum et al., 2008b; Lunde et al., 2008). Significance of plant ontogeny in the modulation of plant responses to abiotic stress factors such as drought (Anjum et al., 2008a) and heavy metals (Anjum et al., 2008c) has been reported. Also, considering a single plant growth-stage, the role of S nutrition in the improvement of plant growth, development and yield (Ahmad et al., 2005), and tolerance to stresses (such as Cd; Anjum et al., 2008b) has been evidenced. However, information is meager on the S-mediated control of plant responses to drought stress during plant ontogeny.
Given the paucity of information on drought sensitivity of legume crops, and on the physiological basis of mineral-nutrient-(like S)-assisted management of crop growth and productivity, the current study was undertaken (i) to screen the drought-sensitive stage(s) during plant ontogeny, (ii) to identify the plant-growth stage when S helps plants maximally to improve the pools of both cellular redox buffers (AsA, GSH) and mineral-nutrients (K, S, and Mg) in order to counteract the drought-accrued oxidative stress (measured as electrolyte leakage (EL), membrane lipid peroxidation and H2O2 levels). Mungbean (Vigna radiata L. Wilczek) was chosen as a model plant system for the current study, because it is a potential pulse crop in the Indian sub-continent due to its ready market, N2-fixation capability, early maturity and the ability to fit well in crop-rotation program (Anjum, 2006). Additionally, S-requirement of the pulse crops, for maintaining their normal growth and development, stands just second to that of the oil-yielding crops.
Materials and Methods
Experimental Materials, Procedure and Soil Characteristics
Seeds of mungbean (Vigna radiata L. Wilczek) cultivar Pusa 9531 were sown in 30-cm-diameter earthen pots filled with 8 kg soil. The soil was sandy loam in texture, with 7.8 pH 7.8, 0.38 dsm electrical conductivity, 0.43% organic carbon, 70 mg kg−1 soil available K and 5 mg kg−1 soil available S. Nitrogen (N; 120 mg kg−1 soil) and phosphorus (P; 30 mg kg−1 soil) were applied at the time of sowing. S was applied to V. radiata plants at the rate of 40 mg kg−1 soil, in the form of solution, 5 days before drought-stress imposition at various growth stages. The sources of N, P, K, and S were urea, single super phosphate, gypsum, and muriate of potash, respectively. After germination, three plants per pot were maintained until harvest. The pots were kept in green house under semi-controlled condition. A polythene plastic film was used to thwart the effects of rainfall, which allowed transmittance of 90% of visible wavelength (400–700 nm) under natural day and night conditions with a day/night temperature 25/20 ± 4°C and relative humidity of 70 ± 5%. All experiments were performed using completely expanded leaves from the second youngest nodes from the top of the plants.
Drought Stress Imposition and Sulfur (S) Application Schedule
Drought was imposed at pre-flowering (15 d after sowing) (group 1), flowering (30 d after sowing) (group 2) and at pod filling (50 d after sowing) (group 3) by withholding water for 5 days; this was followed by normal watering (without S). Other three groups (4–6) as well as the controls were supplied with an equal amount of S solution (40 mg S kg−1). All these (1–6) plant groups, and the control group, were maintained until harvest, and watered on alternate days. Soil moisture content was measured gravimetrically on dry weight basis at the time of pre-flowering, flowering, and post-flowering (pod-filling) stages (Table 1). Samplings were done after re-watering the drought-exposed plants for 5 days at the given growth stage i.e., at 25, 40, and 60 days after sowing. The treatments were arranged in a randomized complete block design, and each treatment was replicated five times.
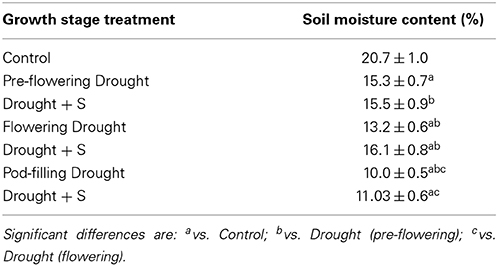
Table 1. Soil moisture content (%) measured in the control and drought-stressed conditions [with and without sulfur (S) supply], at pre-flowering, flowering and pod-filling stages of mungbean (Vigna radiata) plants. Values are the means of five replicates ± standard deviation.
Plant Growth, Photosynthesis and Biochemical Estimations
Leaf area was measured with a leaf area meter (LI-3000A, LI-COR, Lincoln, NE). Plant dry mass was determined after drying the plant at 80°C to a constant weight with the help of an electronic balance (SD-300). Net photosynthetic rate (Pn), stomatal conductance (Gs) and intercellular CO2 concentration (Ci) were recorded in fully expanded leaves of second youngest nodes, using infra-red gas analyzer (IRGA, LI-COR, 6400, Lincoln, NE) on a sunny day between 10:00 and 11:00 h. Chlorophyll content was estimated in fully expanded young leaves at each stage using the method given by Hiscox and Israelstam (1979). Estimation of soluble protein content was done according to Bradford (1976) using bovine serum albumin as standard.
Oxidative Stress Traits
We considered electrolyte leakage (EL), membrane lipid peroxidation and H2O2 levels as the biomarkers of oxidative stress. Cellular membrane integrity in leaves was assayed by measuring the EL according to Anjum et al. (2013). In brief, fresh leaves (1.0 g) were kept in glass vials containing 10 ml deionized water. The vials, covered with plastic caps, were placed in a shaking incubator at a constant temperature of 25°C for 6 h and the electrical conductivity (EC) of the solution was measured (EC1) using an electrical conductivity meter (WTW Cond 330i/SET, Weilheim, Germany). Subsequently, the same vials were kept in water bath shaker at 90°C for 2 h, cooled and EC2 was measured. EL was expressed following the formula EL = EC1/EC2 × 100.
Membrane lipid peroxidation was estimated in terms of thiobarbituric acid reactive substances (TBARS) contents adopting the method of Dhindsa et al. (1981) as described by Anjum et al. (2013). Briefly, fresh leaves (1.0 g) were ground in liquid nitrogen, mixed with 0.73% 2-thiobarbituric acid in 12% trichloroacetic acid, incubated for 30 min in boiling water, ice-cooled, centrifuged at 1000×g for 10 min at 4°C and the absorbance measured in the supernatant at 532 nm. The rate of lipid peroxidation was expressed as nmoles of TBARS formed per gram of fresh weight, using a molar extinction coefficient of 1.55 × 105 M−1 cm−1. Leaf-H2O2 content was determined following the method of Loreto and Velikova (2001) as adopted and described by Dipierro et al. (2005). In brief, leaf tissues (1.0 g) were homogenized in 2 ml of 0.1% (w/v) TCA. The homogenate was centrifuged at 12,000×g for 15 min and 0.5 ml of the supernatant were mixed with 0.5 ml of 10 mM K-phosphate buffer pH 7.0 and 1 ml of 1 M KI. The H2O2 content of the supernatant was evaluated by comparing its absorbance at 390 nm with a standard calibration curve.
Determination of Cellular Buffers
Both reduced GSH and AsA were considered as representative cellular redox buffers. The content of reduced glutathione (GSH) was estimated following the method of Anderson (1985). Fresh leaf tissues (1.0 g) were homogenized in 2 ml of 5% (w/v) sulphosalicylic acid at 4°C. The homogenate was centrifuged at 10,000×g for 10 min. To a 0.5 ml of supernatant, 0.6 ml of K-phosphate buffer (100 mM, pH 7.0) and 40 μl of 5′5′-dithiobis-2-nitrobenzoic acid (DTNB) were added, and absorbance was recorded after 2 min at 412 nm on a UV-VIS spectrophotometer (Lambda Bio 20, Perkin Elmer, MA, USA). The method of Law et al. (1983) was followed for estimation of reduced ascorbate (AsA). In brief, fresh leaf (0.5 g) was homogenized in 2.0 ml of K-phosphate buffer (100 mM, pH 7.0) containing 1 mM EDTA and centrifuged at 10,000×g for 10 min. To a 1.0 ml of supernatant, 0.5 ml of 10% (w/v) trichloroactetic acid (TCA) was added, thoroughly mixed and incubated for 5 min at 4°C. Then, 0.5 ml of NaOH (0.1 M) was mixed with 1.5 ml of the above solution and centrifuged at 5000×g for 10 min at 20°C. The aliquot thus obtained was equally distributed into two separate microfuge tubes (750 μl each). For estimation of AsA, 200 μl of K-phosphate buffer (150 mM, pH 7.4) was added to 750 μl of aliquot. For DHA estimation, 750 μl of aliquot was added to 100 μl of 1,4-dithiothreitol (DTT), followed by vortex-mixing, incubation for 15 min at 20°C, and addition of 100 μl of 0.5% (w/v) NEM. Both the microfuge tubes were then incubated for 30 s at room temperature. To each sample tube, 400 μl of 10% (w/v) TCA, 400 μl of H3PO4, 400 μl of 4% (w/v) bipyridyl dye (N'N-dimethyl bipyridyl) and 200 μl of 3% (w/v) FeCl3 were added and thoroughly mixed. Absorbance was recorded at 525 nm after incubation for 1 h at 37°C.
K, S, and Mg Content Determinations
The method of Lindner (1944) was followed to estimate K content in digested samples using flame photometry; whereas, for S determination, 100 mg of dried fine powder of leaf was digested in a mixture of concentrated HNO3 and 60% HClO4 (85:1, v/v) and the content of sulfate was estimated using the turbidimetric method of Chesnin and Yien (1950). Leaf Mg content was determined by digesting samples in 5 ml of 96% H2SO4 and 3 ml of 30% H2O2 at 270°C; thereafter, Mg content was assayed by atomic absorption spectrometry at 285.2 nm wavelengths.
Data Analysis
SPSS (PASW statistics 18, Chicago, IL, USA) for Windows was used for statistical analysis. One-Way analysis of variance (ANOVA) was performed, followed by all pairwise multiple comparison procedures (Tukey test). Mann-Whitney U-test and Levene's test were performed in order to check the normal distribution and the homogeneity of variances, respectively. The data are expressed as mean values ± SD of five independent experiments with at least five replicates for each. The significance level was set at P ≤ 0.05.
Results
Significant results related to plant growth, photosynthetic functions, soluble-protein content, oxidative stress, cellular reducing buffers, plant mineral nutrients, and yield attributes are presented here, highlighting the significant changes observed at different (pre-flowering, flowering, and pod-filling) stages of plant growth.
Plant Growth and Photosynthetic Functions
Under drought stress, plant growth, in terms of leaf area and plant dry mass, decreased significantly at pre-flowering stage (vs. control, C). On application of S, significant change was noted in the drought-induced reduction in leaf area only. Drought stress imposed during pre-flowering stage also caused significant decrease in photosynthetic functions (viz., photosynthetic rate, Pn; stomatal conductance, Gs; intercellular CO2, Ci; chlorophyll content), as compared with the control. Supplementation of S significantly increased the drought-induced reductions in Pn, Gs, and Ci (Tables 2, 3).
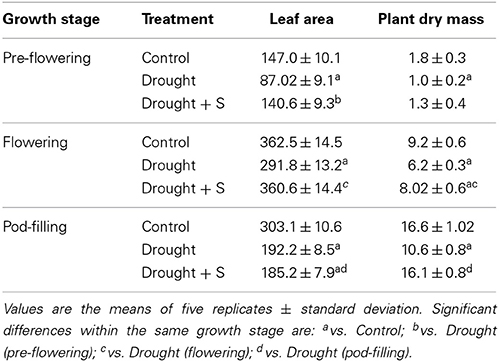
Table 2. Leaf area (cm2 plant−1) and plant dry mass (g plant−1) in mungbean (Vigna radiata) as influenced by drought stress and by drought + sulfur (S) application (mg kg−1 soil) at pre-flowering, flowering, and pod-filling stages.
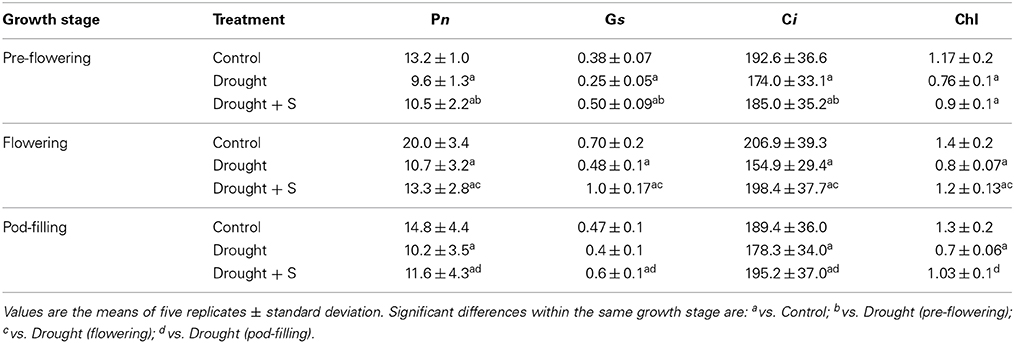
Table 3. Net photosynthetic rate (Pn; μmol CO2 m−1 s−1), stomatal conductance (Gs; mol m−2 s−1), intercellular CO2 concentration (Ci; μmol mol−1), chlorophyll (Chl) content (mg g−1 fresh weight, f.w.) and soluble protein content (mg g−1 f.w.) in mungbean (Vigna radiata), as influenced by drought stress and by drought + sulfur (S) application (mg kg−1 soil) at pre-flowering, flowering, and pod-filling stages.
During the flowering/reproductive stage, significant decrease in leaf area and plant dry mass was perceptible under drought stress alone (vs. C), whereas S application significantly increased the drought-induced reductions in these parameters. Pn, Gs, Ci and the chlorophyll content displayed significant decreases due to drought stress (vs. C); whereas, supplementation of S improved these traits (vs. drought at flowering). During the pod-filling stage, leaf area and plant dry mass decreased significantly due to imposition of drought stress (vs. C) and S application deepened the decline in leaf area and plant dry mass. Likewise, Pn, Gs and Ci and chlorophyll content displayed significant decreases due to drought stress imposed at the pod-filling stage (vs. C). The decrease in Pn, Ci and chlorophyll was significantly ameliorated with S supplementation (Tables 2, 3).
Oxidative Stress and Modulation of the Pools of Cellular Redox Buffers
With-holding water for 5-days during pre-flowering stage led to significant increases in electrolyte leakage (EL) and in the contents of thiobarbituric-acid-reactive substances (TBARS) and H2O2 (vs. C). However, S-application significantly decreased the impact of drought stress-impact at pre-flowering by reducing EL and the contents of TBARS and H2O2 (Figure 1). The pools of cellular redox buffers namely reduced ascorbate (AsA) and glutathione (GSH) declined significantly due to pre-flowering drought stress (vs. C); S-supplementation was insignificant to mitigate these declines. Oxidative stress traits such as EL, and the contents of TBARS and H2O2 significantly increased due to drought stress created at flowering stage (vs. C); however, S-application significantly decreased this drought-caused oxidative stress. In contrast, the contents of reduced AsA and GSH contents declined significantly (vs. C) due to drought stress at this stage, and supplementation of S significantly ameliorated these declines. Drought stress imposed at pod-filling stage significantly increased EL and the contents of TBARS and H2O2 (vs. C); whereas S-application significantly reduced the levels of H2O2 and EL elevated by drought at this stage. The reductions in AsA and GSH contents due to the drought stress imposed at pod-filling stage were insignificant (vs. C), and the effect of S supplementation in mitigating the impact of drought stress was also insignificant (Figure 2).
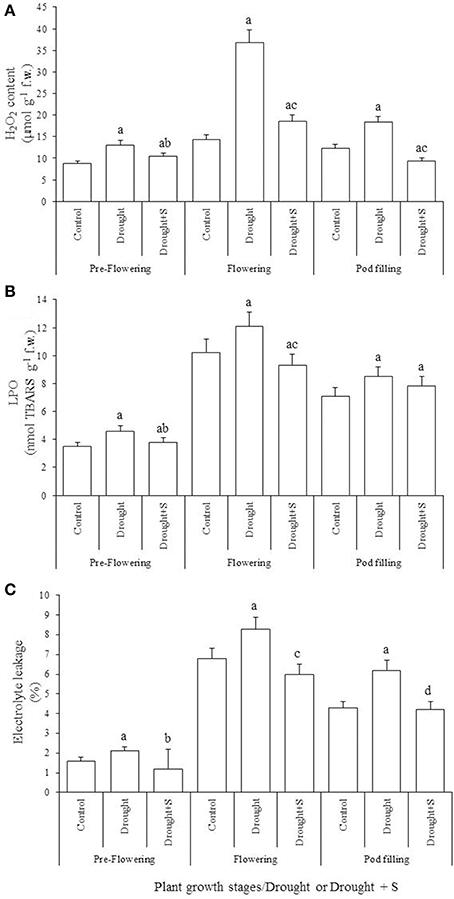
Figure 1. Levels of H2O2 (nmol g−1 fresh weight, f.w.) (A), lipid peroxidation (LPO; nmol thiobarbituric acid reactive substances, TBARS g−1 f.w.) (B) and electrolyte leakage (%) (C) in the mungbean (Vigna radiata) leaf as influenced by drought stress and by drought + sulfur (S) application (mg kg−1 soil) at pre-flowering, flowering, and pod-filling stages of plant growth. Values are the means of five replicates ± standard deviation. Significant differences within the same growth stage are: avs. Control; bvs. Drought (pre-flowering); cvs. Drought (flowering); and dvs. Drought (pod-filling).
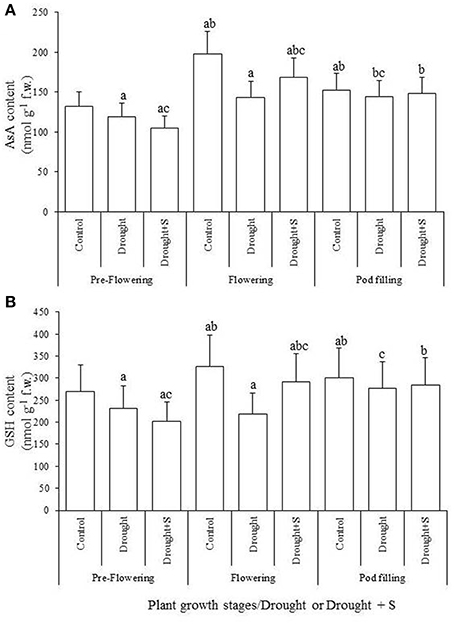
Figure 2. The reduced ascorbate (AsA) (A) and reduced glutathione (GSH) (B) contents (nmol g−1 fresh weight) in the mungbean (Vigna radiata) leaf as influenced by drought stress and sulfur (S) application (mg kg−1 soil) at pre-flowering, flowering, and pod-filling stages of plant growth. Values are the means of five replicates ± standard deviation. Significant differences within same growth stage are: avs. Control; bvs. Drought (pre-flowering); cvs. Drought (flowering); and dvs. Drought (pod-filling).
Mineral Nutrients
Plant nutrients, such as K, S, and Mg, displayed significant reductions due to drought stress imposed at pre-flowering stage (vs. C); however, no significant difference was observed when drought-stressed plants were supplemented with S. Drought imposition during flowering stage caused significant reduction in K, S, and Mg levels in the leaf tissue (vs. C); whereas their contents significantly increased when plants facing drought at flowering stage were supplemented with S. Among the plant nutrients studied, only K content displayed a significant reduction due to drought at pod-filling stage (vs. C); whereas S and Mg contents did not differ significantly under the stress of drought alone or drought + S imposed at pod-filling stage (vs. C). Moreover, the K content significantly increased when drought-stressed plants were supplemented with S (Table 4).
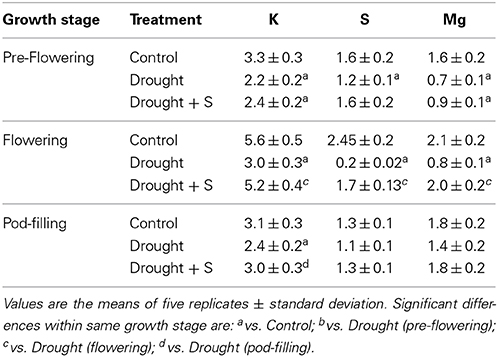
Table 4. Potassium (K), sulfur (S), and magnesium (Mg) contents (μmol g−1 dry weight) in mungbean (Vigna radiata) leaves as influenced by drought stress and by drought +S application (mg kg−1 soil) at pre-flowering, flowering, and pod-filling stages.
Discussion
Plant Growth and Photosynthetic Functions
Plant growth is the outcome of coordination of major physiological/biochemical processes in plants. In the present study, plant dry mass and leaf area showed a significant relationship with the severity of water deficit stress, irrespective of the phase of plant ontogeny. Earlier, plant growth in terms of dry mass accumulation and leaf area has been used as a tool for the assessment of crop productivity (Sundaravalli et al., 2005; Anjum et al., 2008a). Cell division, enlargement and differentiation and also the plant genetic make-up are significantly influenced by water-deficit stress, which in turn affects plant growth (Aref et al., 2013). In the present study, previously mentioned processes might be impacted by drought tress severely during vegetative/flowering stage which coincides with drought-mediated considerable decreases in leaf area and photosynthesis, as observed earlier also (Sundaravalli et al., 2005; Anjum et al., 2008a; Husen et al., 2014). However, the drought-induced huge reduction in leaf area (a major component of plant growth) may be a strategy that plants adopt to adjust with water-deficit stress. Earlier, the reduced leaf-expansion/area was evidenced to conserve the internal water/moisture through the reduced rate of transpiration (reviewed by Mahajan and Tuteja, 2005).
Photosynthesis (Pn) and its related variables (Gs, Ci, chlorophyll content) are highly regulated multi-step processes and exhibit great sensitivity to drought stress (Zlatev et al., 2006; Lawlor and Tezara, 2009; Husen et al., 2014). In the current study, drought stress alone significantly decreased Pn, Gs, Ci and chlorophyll content irrespective of the plant ontogenetic stages. In fact, photosynthesis and its related variables are tightly interwoven and hence changes in one component significantly affect the performance of others (Lawlor and Tezara, 2009). Our findings on the drought stress-accrued reductions in Gs and leaf Ci coincide with those of Zlatev et al. (2006) and Meyer and Genty (1998), who considered Gs as the major factor for controlling Ci and hence the Pn. Additionally, unavailability of chlorophyll also contributes to drought-induced decrease in Pn (Lawlor and Tezara, 2009). The drought-induced decrease in chlorophyll content has been reported earlier also due to reduction in the lamellar content of the light-harvesting chlorophyll a/b protein, inhibition in biosynthesis of chlorophyll-precursors and/or degradation of chlorophyll (Khanna-Chopra et al., 1980). Our findings on drought-mediated decrease in Pn, Gs, Ci and the content of chlorophyll confirm some earlier reports (Khanna-Chopra et al., 1980; Anjum et al., 2008a; Husen et al., 2014).
Regardless of irrigation treatments, our results also revealed that S-application significantly increased the growth and chlorophyll content and Pn. It was more effective when applied at flowering stage of the plant. The adequate and balanced supply of mineral nutrients has been shown to play a vital role in sustaining food security (Cakmak, 2005). S is involved in the light reaction of photosynthesis as an integral part of ferredoxin, a non-haem iron-sulfur protein (Marschner, 1995). Additionally, it plays essential roles in mechanisms like vitamin co-factors, GSH in redox homeostasis, and detoxification of xenobiotics (Anjum et al., 2012b). The S requirement by plants varies with growth stage and with species, varying normally between 0.1 and 1.5% of dry weight. Anjum et al. (2008b) suggested that adequate S supply may improve the pools of these compounds in plants to a great extent that may lead to increased photosynthetic efficiency, dry mass and crop yield. Sufficient S supplies improved photosynthesis and growth of Brassica juncea through regulating N assimilation (Khan et al., 2005). The maximum utilization of S in Brassica campestris crop takes place when applied at flowering stage (Ahmad et al., 2005; Anjum, 2006). Application of S increased the seed yield and attributing characters in other crops also (Anjum et al., 2012b).
Oxidative Stress and Modulation of the Pools of Cellular Redox Buffers and Mineral Nutrients
Production of ROS, such as H2O2, is mediated by O2 reduction and subsequent oxidative damages in drought-exposed plants (Khanna-Chopra and Selote, 2007; Anjum et al., 2012a). Plant membrane is regarded as the first target of many plant stresses due to increase in its permeability and loss of integrity under environmental stresses including the drought stress (Candan and Tarhan, 2003). In the present study, the drought-stress sensitivity of the reproductive phase of drought-exposed V. radiata was evidenced by significantly high levels of H2O2, the content of TBARS (the cytotoxic products of lipid peroxidation and indicator of extent of stress-led ROS-mediated high oxidative stress) and the EL (the measure of stress-mediated changes in membrane leakage and injury) at flowering stage, followed by pre-flowering and post-flowering stages. These results are in close agreement with the findings of Qureshi et al. (2007). Earlier, the least peroxidation of membrane lipids and the ability of cell membranes to tightly control the rate of ion movement in and out of cells have been used as tests of damage to a great range of tissues (Candan and Tarhan, 2003). However, the drought-stressed plants exhibited least contents of H2O2, TBARS and percent EL, when supplemented with S at their flowering and pod-filling stages. These results suggested that the S-mediated decrease in contents of H2O2, TBARS and percent EL depends on application of S to drought-stressed plants at appropriate growth stage when S is efficiently and differentially utilized to strengthen plants to withstand the enhanced lipid peroxidation and subsequent leakage of electrolytes due to elevated levels of H2O2. Thus, S-application protected differentially the drought-stressed plants against H2O2-mediated localized oxidative damage, disruption of metabolic functions, LPO and leakage of electrolytes (Zlatev et al., 2006). Our observations on drought alone-mediated significant increases in H2O2 content, lipid peroxidation (in terms of TBARS content) and percent EL in V. radiata plants coincide with the findings of Sreenivasulu et al. (2000) and Selote and Khanna-Chopra (2006) on different crop plants.
Plant resistance to stresses is closely associated with the efficiency of the antioxidant defense system (comprising both enzymatic and non-enzymatic components of AsA-GSH pathway) in the maintenance of the balance between the basal production of ROS and their elimination (Anjum et al., 2010, 2012c). In this perspective, AsA and GSH are important water-soluble non-enzymatic antioxidants and major cellular redox buffers in plants (Anjum et al., 2010, 2012c, 2014). Both are interlinked in terms of their physiological role in AsA-GSH pathway for effective elimination of ROS (such as H2O2) in plant cells (Anjum et al., 2010, 2012a,c). Contrary to an earlier report (Shehab et al., 2010) on drought-induced increase in AsA and GSH levels in different plant species, our study revealed a significant decrease in the contents of both AsA and GSH in V. radiata, irrespective of the growth stage at which the drought stress was imposed. However, our findings are in conformity with those of Khanna-Chopra and Selote (2007) on drought-exposed Triticum aestivum. The application of S improved the AsA and GSH contents and was thus beneficial when applied to drought-stressed plants at their flowering or post-flowering stages. It was, therefore, significant for protection of V. radiata against ROS-mediated oxidative stress. This substantiates our earlier report suggesting improved AsA and GSH contents in Cd-stressed Brassica campestris plants by S supplementation (Anjum et al., 2008b). However, it is imperative to mention here that exhibition of higher levels of AsA and GSH in plants receiving drought stress + S supply at pod-filling stage may be due to S-mediated maintenance of elevated activities of AsA-GSH-regenerating enzymes such as dehydroascorbate reductase, monodehydroascorbate reductase, and GSH reductase (Eltayeb et al., 2007; Anjum et al., 2008b). Moreover, as AsA and GSH are key players in cellular redox homeostasis; the S-mediated improvement in their reduced pools must help plants to run normally the ascorbate peroxide-dependent H2O2 metabolism under drought-stress conditions. Therefore, S application mitigated, although partially, the drought-induced decrease in AsA content by maintaining elevated activities of dehydroascorbate reductase and monodehydroascorbate reductase (data not shown)—the key components in maintaining the reduced pool of AsA and hence the plant tolerance to oxidative stress (Eltayeb et al., 2007). Considering K, S, and Mg responses to drought and S, the uptake of the available nutrient ions dissolved in the soil solution by plants depends upon water flow through the soil-root-shoot pathway. It also depends on root growth and nutrient mobility in the soil (Fageria et al., 2002). In this study, drought stress significantly impacted the contents of K, S, and Mg in leaves contingent upon the plant-growth stage exposed. However, as reported also in earlier studies (Abdin et al., 2003; Malvi, 2011), a synergistic interaction of S with K and Mg was revealed herein, where S-application ameliorated drought-induced reductions in the leaf K, S, and Mg contents, maximally when applied at reproductive stage.
Conclusions
Drought stress in isolation enhanced ROS generation and decreased the cellular redox buffers (AsA and GSH) and eventually hampered photosynthetic functions. These results were significant at flowering stage, followed by the pre-flowering and post-flowering (pod-filling) stages (Figure 3). However, improvements in these parameters due to S application was apparent (at the flowering/reproductive stage), which enhanced the pools of cellular redox buffers (AsA and GSH), which in turn managed a balance between the production and scavenging of H2O2 and stabilized the cell membrane by decreasing LPO (Figure 3). Overall, the study inferred that supplementation of S to drought-exposed plants at their flowering stage can improve their growth, photosynthesis and related variables via efficiently being utilized, and in turn managing the pools of AsA and GSH, and subsequently controlling the drought-accrued oxidative stress.
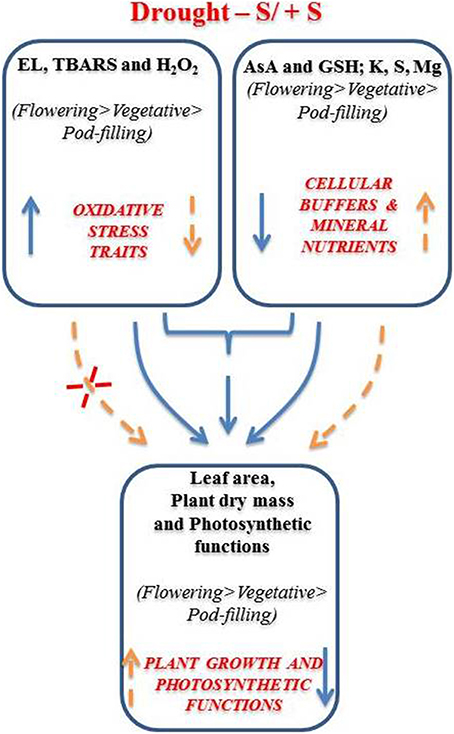
Figure 3. Schematic representation of drought stress impacts and the role of sulfur (S) in mungbean (Vigna radiata) during its ontogeny. Complete and broken lines indicate respectively drought alone and drought + S conditions; whereas, increase and decrease have been indicated by the up and down arrows respectively.
Conflict of Interest Statement
The authors declare that the research was conducted in the absence of any commercial or financial relationships that could be construed as a potential conflict of interest.
Acknowledgments
Partial financial support received from the Hamdard National Foundation (HNF), New Delhi, India (DSW/HNF-18/2006) and the Portuguese Foundation for Science and Technology (FCT) (SFRH/BPD/64690/2009; SFRH/BPD/84671/2012) is gratefully acknowledged.
References
Abdin, M. Z., Ahmad, A., Khan, N., Khan, I., Jamal, A., and Iqbal, M. (2003). “Sulphur interaction with other nutrients,” in Sulphur in Plants, eds Y. Abrol and A. Ahmad (Dordrecht: Kluwer Academic Publishers), 359–374. doi: 10.1007/978-94-017-0289-8_20
Ahmad, A., Khan, I., Anjum, N. A., Diva, I., Abdin, M. Z., and Iqbal, M. (2005). Effect of timing of sulfur fertilizer application on growth and yield of rapeseed. J. Plant Nutr. 28, 1049–1059. doi: 10.1081/PLN-200058905
Anderson, M. E. (1985). Determination of glutathione and glutathione disulfides in biological samples. Methods Enzymol. 113, 548–570. doi: 10.1016/S0076-6879(85)13073-9
Anjum, N. A. (2006). Effect of Abiotic Stresses on Growth and Yield of Brassica campestris L. and Vigna radiata (L.) Wilczek under Different Sulfur Regimes. Ph.D. thesis, New Delhi: Jamia Hamdard.
Anjum, N. A., Ahmad, I., Mohmood, I., Pacheco, M., Duarte, A. C., Pereira, E., et al. (2012c). Modulation of glutathione and its related enzymes in plants' responses to toxic metals and metalloids - a review. Environ. Exp. Bot. 75, 307–324. doi: 10.1016/j.envexpbot.2011.07.002
Anjum, N. A., Ahmad, I., Rodrigues, S., Henriques, B., Cruz, N., Coelho, C., et al. (2013). Eriophorum angustifolium and Lolium perenne metabolic adaptations to metals- and metalloids-induced anomalies in the vicinity of a chemical industrial complex. Environ. Sci. Pollut. Res. 20, 568–581. doi: 10.1007/s11356-012-1062-2
Pubmed Abstract | Pubmed Full Text | CrossRef Full Text | Google Scholar
Anjum, N. A., Gill, S. S., Gill, R., Hasanuzzaman, M., Duarte, A. C., Pereira, E., et al. (2014). Metal/metalloid stress tolerance in plants: role of ascorbate, its redox couple and associated enzymes. Protoplasma 251, 1265–1283. doi: 10.1007/s00709-014-0636-x
Pubmed Abstract | Pubmed Full Text | CrossRef Full Text | Google Scholar
Anjum, N. A., Gill, S. S., Umar, S., Ahmad, I., Duarte, A. C., and Pereira, E. (2012b). Improving growth and productivity of oleiferous Brassicas under changing environment: significance of nitrogen and sulphur nutrition, and underlying mechanisms. Sci. World J. 2012:657808. doi: 10.1100/2012/657808
Pubmed Abstract | Pubmed Full Text | CrossRef Full Text | Google Scholar
Anjum, N. A., Umar, S., and Ahmad, A. (2012a). Oxidative Stress in Plants: Causes, Consequences and Tolerance. New Delhi: IK International Publishing House Pvt. Ltd.
Anjum, N. A., Umar, S., Ahmad, A., Iqbal, M., and Khan, N. A. (2008b). Sulphur protects mustard (Brassica campestris L.) from cadmium toxicity by improving leaf ascorbate and glutathione. Plant Growth Regul. 54, 271–279. doi: 10.1007/s10725-007-9251-6
Anjum, N. A., Umar, S., Ahmad, A., Iqbal, M., and Khan, N. A. (2008c). Ontogenic variation in response of Brassica campestris L. to cadmium toxicity. J. Plant Interact. 3, 189–198. doi: 10.1080/17429140701823164
Anjum, N. A., Umar, S., and Chan, M. T. (2010). Ascorbate-Glutathione Pathway and Stress Tolerance in Plants. Dordrecht: Springer. doi: 10.1007/978-90-481-9404-9
Anjum, N. A., Umar, S., Iqbal, M., and Khan, N. A. (2008a). Growth characteristics and antioxidant metabolism of moongbean genotypes differing in photosynthetic capacity subjected to water deficit stress. J. Plant Interact. 3, 127–136. doi: 10.1080/17429140701810732
Aref, M. I., Ahmed, A. I., Khan, P. R., El-Atta, H., and Iqbal, M. (2013). Drought-induced adaptive changes in the seedling anatomy of Acacia ehrenbergiana and Acacia tortilis subsp. raddiana. Trees 27, 959–971. doi: 10.1007/s00468-013-0848-2
Bradford, M. M. (1976). A rapid and sensitive method for the quantitation of microgram quantities of protein utilizing the principle of protein-dye binding. Anal. Biochem. 72, 248–254. doi: 10.1016/0003-2697(76)90527-3
Pubmed Abstract | Pubmed Full Text | CrossRef Full Text | Google Scholar
Cai, Y., Cao, F., Wei, K., Zhang, G., and Wu, F. (2011). Genotypic dependent effect of exogenous glutathione on Cd-induced changes in proteins, ultrastructure and antioxidant defense enzymes in rice seedlings. J. Hazard. Mater. 192, 1056–1066. doi: 10.1016/j.jhazmat.2011.06.011
Pubmed Abstract | Pubmed Full Text | CrossRef Full Text | Google Scholar
Cakmak, I. (2005). The role of potassium in alleviating detrimental effects of abiotic stresses in plants. J. Plant Nutr. Soil Sci. 168, 521–530. doi: 10.1002/jpln.200420485
Candan, N., and Tarhan, L. (2003). The correlation between antioxidant enzyme activities and lipid peroxidation levels in Mentha pulegium organs grown in Ca2+, Mg2+, Cu2+, Zn2+ and Mn2+ stress conditions. Plant Sci. 163, 769–779. doi: 10.1016/S0168-9452(03)00269-3
Chesnin, L., and Yien, C. H. (1950). Turbidimetric determination of available sulphates. Proc. Soil Sci. Soc. Am. 51, 149–151.
Dhindsa, R. H., Plumb-Dhindsa, P., and Thorpe, T. A. (1981). Leaf senescence correlated with increased level of membrane permeability, lipid per oxidation and decreased level of SOD and CAT. J. Exp. Bot. 32, 93–101. doi: 10.1093/jxb/32.1.93
Dipierro, N., Mondelli, D., Paciolla, C., Brunetti, G., and Dipierro, S. (2005). Changes in the ascorbate system in the response of pumpkin (Cucurbita pepo L.) roots to aluminium stress. Plant Physiol. 162, 529–536. doi: 10.1016/j.jplph.2004.06.008
Pubmed Abstract | Pubmed Full Text | CrossRef Full Text | Google Scholar
Eltayeb, A. M., Kawano, N., Badawi, G. H., Kaminaka, H., Sanekata, T., Shibahara, T., et al. (2007). Overexpression of monodehydroascorbate reductase in transgenic tobacco confers enhanced tolerance to ozone, salt and polyethylene glycol stresses. Planta 225, 1255–1264. doi: 10.1007/s00425-006-0417-7
Pubmed Abstract | Pubmed Full Text | CrossRef Full Text | Google Scholar
Fageria, N. K., Baligar, V. C., and Clark, R. B. (2002). Micronutrients in crop production. Adv. Agron. 77, 185–187. doi: 10.1016/S0065-2113(02)77015-6
Gill, S. S., Khan, N. A., Anjum, N. A., and Tuteja, N. (2011). Amelioration of cadmium stress in crop plants by nutrients management: morphological, physiological and biochemical aspects. Plant Stress 5, 1–23.
Harb, A., Krishnan, A., Ambavaram, M. M., and Pereira, A. (2010). Molecular and physiological analysis of drought stress in Arabidopsis reveals early responses leading to acclimation in plant growth. Plant Physiol. 154, 1254–1271. doi: 10.1104/pp.110.161752
Pubmed Abstract | Pubmed Full Text | CrossRef Full Text | Google Scholar
Hawkesford, M. J. (2000). Plant responses to sulfur deficiency and the genetic manipulation of sulfate transporters to improve S-utilization efficiency. J. Exp. Bot. 51, 131–138. doi: 10.1093/jexbot/51.342.131
Pubmed Abstract | Pubmed Full Text | CrossRef Full Text | Google Scholar
Hiscox, J. H., and Israelstam, G. F. (1979). A method for extraction of chlorophyll from leaf tissues without maceration. Can. J. Bot. 57, 1332–1334. doi: 10.1139/b79-163
Husen, A., Iqbal, M., and Aref, I. M. (2014). Water status and leaf characteristics of Brassica carinata under drought stress and re-hydration conditions. Braz. J. Bot. 37, 217–227. doi: 10.1007/s40415-014-0066-1
Khan, N. A., Mobin, M., and Samiullah. (2005). The influence of gibberellic acid and sulfur fertilization rate on growth and S-use efficiency of mustard (Brassica juncea). Plant Soil 270, 269–274. doi: 10.1007/s11104-004-1606-4
Khanna-Chopra, R., Chaturverdi, G., Aggarwal, P., and Sinha, S. (1980). Effect of potassium on growth and nitrate reductase during water stress and recovery in maize. Physiol. Plant 49, 495–500. doi: 10.1111/j.1399-3054.1980.tb03340.x
Khanna-Chopra, R., and Selote, D. S. (2007). Acclimation to drought stress generates oxidative stress tolerance in drought resistant than susceptible wheat cultivar under field conditions. Environ. Exp. Bot. 60, 276–283. doi: 10.1016/j.envexpbot.2006.11.004
Law, M. Y., Charles, S. A., and Halliwell, B. (1983). Glutathione and ascorbic acid in spinach (Spinaceu oleraceu) chloroplasts. The effect of hydrogen peroxide and of paraquat. Biochem. J. 210, 899–903.
Lawlor, D. W., and Tezara, W. (2009). Causes of decreased photosynthetic rate and metabolic capacity in water-deficient leaf cells: a critical evaluation of mechanisms and integration of processes. Ann. Bot. 103, 561–579. doi: 10.1093/aob/mcn244
Pubmed Abstract | Pubmed Full Text | CrossRef Full Text | Google Scholar
Lindner, R. C. (1944). Rapid analytical method for some of the more common organic substances of plant and soil. Plant Physiol. 19, 76–84. doi: 10.1104/pp.19.1.76
Loreto, F., and Velikova, V. (2001). Isoprene produced by leaves protects the photosynthetic apparatus against ozone damage, quenches ozone products, and reduces lipid peroxidation of cellular membranes. Plant Physiol. 127, 1781–1787. doi: 10.1104/pp.010497
Pubmed Abstract | Pubmed Full Text | CrossRef Full Text | Google Scholar
Lunde, C., Zygadlo, A., Simonsen, H. T., Nielsen, P. L., Blennow, A., and Haldrup, A. (2008). Sulfur starvation in rice: the effect on photosynthesis, carbohydrate metabolism, and oxidative stress protective pathways. Physiol. Plant 134, 508–521. doi: 10.1111/j.1399-3054.2008.01159.x
Pubmed Abstract | Pubmed Full Text | CrossRef Full Text | Google Scholar
Mahajan, S., and Tuteja, N. (2005). Cold, salinity and drought stresses: an overview. Arch. Biochem. Biophys. 444, 139–158. doi: 10.1016/j.abb.2005.10.018
Pubmed Abstract | Pubmed Full Text | CrossRef Full Text | Google Scholar
Malvi, U. R. (2011). Interaction of micronutrients with major nutrients with special reference to potassium. Karnataka J. Agric. Sci. 24, 206–109.
Meyer, S., and Genty, B. (1998). Mapping intercellular CO2 mole fraction (Ci) in Rosa leaves fed with ABA by using chlorophyll fluorescence imaging. Significance of Ci estimated from leaf gas exchange. Plant Physiol. 116, 947–958. doi: 10.1104/pp.116.3.947
Pubmed Abstract | Pubmed Full Text | CrossRef Full Text | Google Scholar
Nahar, K., Hasanuzzaman, M., Alam, M. M., and Fujita, M. (2015). Exogenous glutathione confers high temperature stress tolerance in mung bean (Vigna radiata L.) by modulating antioxidant defense and methylglyoxal detoxification system. Environ. Exp. Bot. 112, 44–54. doi: 10.1016/j.envexpbot.2014.12.001
Qureshi, M. I., Qadir, S., and Zolla, L. (2007). Proteomics-based dissection of stress-responsive pathways in plants. J. Plant Physiol. 164, 1239–1260. doi: 10.1016/j.jplph.2007.01.013
Pubmed Abstract | Pubmed Full Text | CrossRef Full Text | Google Scholar
Selote, D. S., and Khanna-Chopra, R. (2006). Drought acclimation confers oxidative stress tolerance by inducing co-ordinated antioxidant defence at cellular and subcellular level in leaves of heat seedlings. Physiol. Plant 127, 494–506. doi: 10.1111/j.1399-3054.2006.00678.x
Shehab, G. G., Ahmed, O. K., and El-Beltagi, H. S. (2010). Effects of various chemical agents for alleviation of drought stress in rice plants (Oryza sativa L.). Not. Bot. Hort. Agrobot. Cluj-Napoca 38, 139–148.
Son, J. A., Narayanankutty, D. P., and Roh, K. S. (2014). Influence of exogenous application of glutathione on rubisco and rubisco activase in heavy metal-stressed tobacco plant grown in vitro. Saudi J. Biol. Sci. 21, 89–97. doi: 10.1016/j.sjbs.2013.06.002
Pubmed Abstract | Pubmed Full Text | CrossRef Full Text | Google Scholar
Sreenivasulu, N., Grimm, B., Wobus, U., and Weschke, W. (2000). Differential response of antioxidant compounds to salinity stress in salt-tolerant and salt sensitive seedlings of foxtail millet (Setaria italica). Physiol. Plant 109, 435–442. doi: 10.1034/j.1399-3054.2000.100410.x
Sundaravalli, V., Paliwal, K., and Ruckmani, A. (2005). Effect of water stress on photosynthesis, protein content and nitrate reductases activity of Albizzia seedlings. J. Plant Biol. 32, 13–17.
Wang, R., Liu, S., Zhou, F., Ding, C., and Hua, C. (2014). Exogenous ascorbic acid and glutathione alleviate oxidative stress induced by salt stress in the chloroplasts of Oryza sativa L. Z. Naturforsch. 69c, 226–236. doi: 10.5560/ZNC.2013-0117
Pubmed Abstract | Pubmed Full Text | CrossRef Full Text | Google Scholar
Zlatev, Z. S., Lidon, F. C., Ramalho, J. C., and Yordanov, I. T. (2006). Comparison of resistance to drought of three bean cultivars. Biol. Plant 50, 389–394. doi: 10.1007/s10535-006-0054-9
Pubmed Abstract | Pubmed Full Text | CrossRef Full Text | Google Scholar
Keywords: cellular buffers, drought stress, mungbean ontogeny, oxidative stress, sulfur, Vigna radiata
Citation: Anjum NA, Umar S, Aref IM and Iqbal M (2015) Managing the pools of cellular redox buffers and the control of oxidative stress during the ontogeny of drought-exposed mungbean (Vigna radiata L.)—role of sulfur nutrition. Front. Environ. Sci. 2:66. doi: 10.3389/fenvs.2014.00066
Received: 11 December 2014; Accepted: 17 December 2014;
Published online: 08 January 2015.
Edited by:
Adriano Sofo, Università degli Studi della Basilicata, ItalyReviewed by:
Yogesh Abrol, Bhagalpur University, IndiaKumar Ajit, University of South Australia, Australia
Copyright © 2015 Anjum, Umar, Aref and Iqbal. This is an open-access article distributed under the terms of the Creative Commons Attribution License (CC BY). The use, distribution or reproduction in other forums is permitted, provided the original author(s) or licensor are credited and that the original publication in this journal is cited, in accordance with accepted academic practice. No use, distribution or reproduction is permitted which does not comply with these terms.
*Correspondence: Muhammad Iqbal, Department of Botany, Faculty of Science, Hamdard University, New Delhi, 110062, India e-mail:aXFiYWxnNUB5YWhvby5jby5pbg==