- 1School of Environment, Resources and Development, Asian Institute of Technology, Khlong Luang, Thailand
- 2Centre for Conservation Science and Technology, University of Adelaide, Adelaide, SA, Australia
- 3Graduate School of Applied Informatics, University of Hyogo, Kobe, Japan
- 4Department of Global Ecology, Carnegie Institution for Science, Stanford, CA, USA
- 5U.S. Department of Agriculture Forest Service, Newtown Square, PA, USA
- 6Department of Physical Geography and Ecosystem Science, Lund University, Lund, Sweden
- 7Food and Agriculture Organization of the United Nations Regional Office for Asia and the Pacific, Bangkok, Thailand
- 8International Tropical Timber Organization, Yokohama, Japan
- 9Faculty of Career Development, Kyoto Koka Women's University, Kyoto, Japan
- 10School of Biological Sciences, University of Adelaide, Adelaide, Australia
- 11Department of Biology, University of Florida, Gainesville, FL, USA
HIGHLIGHTS
• About 500 million hectares of tropical forests have been degraded due primarily to overexploitation
• Preventing premature re-entry into harvested areas can retain up to 34% of carbon stocks in the forests
• Adoption of reduced-impact logging and wood processing technologies (RIL+) along with financial incentives can reduce forest fires, forest degradation, maintain timber production, and retain carbon stocks
• About US$1.8 Mg or US$2 billion year−1 is needed for the adoption of RIL+ for the whole tropical production forests.
The REDD+ scheme of the United Nations Framework Convention on Climate Change has provided opportunities to manage tropical forests for timber production and carbon emission reductions. To determine the appropriate logging techniques, we analyzed potential timber production and carbon emission reductions under two logging techniques over a 40-year period of selective logging. We found that use of reduced-impact logging (RIL) techniques alone in tropical production forests (PdF) could reduce carbon emissions equivalent to 29–50% of net emissions from tropical deforestation and land use change, while also supplying 45% of global round-wood demand. Adopting RIL plus other improvements (RIL+) in forest management (adopting forest certification and DNA timber tracking to prevent illegal logging) and wood conversion practices (adopting technology to increase recovery of sawn wood), would result in increasing long-term carbon storage in sawn-wood and reduce logging-induced fire-prone wood wastes by 14–184%. For this to happen, about US$2 billion or $1.86 per Mg CO2 in financial incentives are needed annually for parties to adopt RIL+ and to prevent premature re-entry logging. Our findings suggest that future climate policies should explicitly include RIL+ to satisfy the “sustainable management of forests” proviso in the REDD+ scheme, and also count carbon in wood products as eligible credits for trading.
Introduction
Tropical forests are diverse in terms of flora and fauna species. Deforestation and forest degradation in the tropics have resulted in the annual loss of natural forests of 13 million ha, while degrading 500 million ha of primary and secondary forests (ITTO, 2002), and affecting up to 85% of the threatened and endangered species listed in the Red List of the International Union for Conservation of Nature (www.iucnredlist.org). In addition, tropical deforestation is responsible for net emissions of about 1 Pg C year−1 (Pan et al., 2011; Baccini et al., 2012), or 10% of global anthropogenic emissions. Not included in these estimates are emissions from unnecessarily destructive logging, which also unduly reduces commercial timber stocks and, worse yet, render many forests prone to burning and clearing (Asner et al., 2006). Losses of commercial timber stocks and associated carbon emissions (referred to collectively as forest degradation) are difficult to evaluate with the available remote sensing technology (Bustamante et al., 2016; Palace et al., 2016) because trees are selectively logged from forests with dense canopies, but the extent of logging far exceeds that of deforestation (Bicknell et al., 2014). Although carbon emissions from forest degradation usually go unreported (Asner et al., 2005), some estimate the magnitude of these emissions to be equivalent to that from deforestation (Pearson et al., 2014). From 2000 to 2005, high-resolution global remotely sensed images showed that humid tropical forest logging had at least 20-times the geographic footprint of deforestation (Asner et al., 2009). Unplanned logging by untrained crews causes severe damage to residual forests and leaves behind huge amounts of logging waste. Unnecessarily large canopy openings coupled with the presence of flammable logging waste also render forests susceptible to destructive fires (Cochrane, 2003).
Sustainable forest management is defined by the International Tropical Timber Organization (ITTO) as the process of managing forest to achieve one or more clearly specified objectives of management with regard to the production of a continuous flow of desired forest products and services without undue reduction of its inherent values and future productivity and without undue undesirable effects on the physical and social environment (www.itto.int). In our study, the desired objectives are to achieve the flow of timber production and to retain carbon stocks in the forests by switching from conventional logging (CVL) to reduced-impact logging (RIL). Defined in this article as a logging practice with well-defined planning, well-trained logging crews, and supervised implementation of timber harvesting operations, RIL is capable of significantly reducing logging damages and wood wastes caused by harvesting operations compared to CVL (see Sasaki and Putz, 2009, for comparison of damages and wastes). Main activities under RIL include pre-harvest activities such as boundary demarcation, pre-felling inventory, tree hunting, training, and tree marking and mapping and harvest activities such as felling and bucking, skidding, and log deck operation (Medjibe and Putz, 2012).
Sustainable forest management for timber and maximization of carbon retention are currently jeopardized in much of the tropics by poor logging practices and premature re-entry into harvested areas (i.e., re-logging before the end of the designated cutting cycle). Our previous studies suggest that replacing CVL with RIL could substantially reduce carbon emissions (Kim Phat et al., 2004; Putz et al., 2012; Sasaki et al., 2012). RIL can contribute to biodiversity conservation ranging from trees (Bicknell et al., 2014) to mammals (Putz et al., 2012). Furthermore, Miller et al. (2011) demonstrated with eddy covariance and ecological measurements that RIL impacts on forest carbon stocks are minimal, ranging from 26.0 ± 1.5 to 32.6 ± 1.3 Mg C ha−1 year−1 in forest before logging and 31.1 ± 1.4 to 32.0 ± 1.1 Mg C ha−1 year−1 in forests after 1–3 years of logging. These findings provide further impetus for explicit inclusion of RIL as a way to reach climate change mitigation objectives. We assess the potential contributions of improved tropical forest management to climate change mitigation through the adoption of RIL, along with strict prohibition of premature logging re-entry and technological changes in forest industries. A combination of RIL and the use (PLUS) of improved logging technology and wood processing technology is referred to here as RIL+.
Methods
Tropical Production Forests and Current Logging Practices
Production forest refers to forest, where commercial logging for timber production is allowed once per cutting cycle of 25–60 years on average, depending on forest types and management intervention (Rutishauser et al., 2015). Timber is exploited through selective logging systems, under which merchantable trees with diameter at breast height (DBH) greater than the DBH limits imposed by logging regulations in tropical countries (see Sasaki and Putz, 2009, for a list DBH limits in selected countries) are felled after logging permit is issued by the country in question. As unsustainable logging, industrial agriculture and smallholder subsistence crop cultivation, and agricultural cultivation continue to destroy and degrade tropical forests and biodiversity, international efforts to protect the forests began to show promising results. The roles of Reducing Emissions from Deforestation and forest Degradation PLUS (REDD+), which includes conservation of forests, sustainable management, and enhancement of forest carbon stocks, were fully recognized in the Copenhagen Accord adopted at the 15th session of the Conference of the Parties (COP15) to the UNFCCC in December 2009 (Burgess et al., 2010). Under the Warsaw Framework for REDD+ at COP 19, the need to protect tropical forests for climate change mitigation was further encouraged by offering result-based financial incentives to developing countries for reducing carbon emissions under REDD+ (Norman et al., 2014). In addition to pledging to reduce emissions by major emitters of greenhouse gases, such as the United States and European Union, and setting an emission cap for China, 24 countries pledged about $10 billion to Climate Finance at the COP20 in Lima in December 2014 (UNFCCC, 2014). REDD+ will inevitably be part of the future climate change regulations once Paris Agreement reached at the COP21 in December 2015 enters into force (UNFCCC, 2015). Sustainable forest management is an important element of REDD+ because of its multiple roles in commercial timber supply, job generation, biodiversity conservation, and reductions in carbon emissions from forest degradation. Sustainable forest management is a suitable goal for production forests (PdF)—the forests where logging for commercial timber is practiced. Logging in the tropics is practiced mainly under forest concessions in which governments grant logging licenses to companies to operate according to governmental regulations. Logging companies are usually required to submit a master plan outlining forest resource assessment methods, harvest planning, sequences of annual harvesting coupes, and socio-environmental impact assessments. Despite progress being made toward the writing of proper forest management plans, the called-for requirements are rarely met in practice and corruption is common. Therefore, sustainable forest management could play a positive role in supporting good governance, global timber supplies, and carbon retention if improved logging practices are adopted.
Forest Areas Available for the RIL Practice
According to forest functions classified in FAO's Global Forest Resources Assessment 2010 (FAO, 2010), about 28.4% of the total area of tropical forests (1664 million ha in 2010) can be considered as PdF. RIL can be applied to manage these PdF for timber production and carbon retention. The area of PdF can be further classified to operable and inoperable areas. The former is the area suitable for logging operations, while the latter is the area of forests located in culturally, socially and environmentally sensitive areas such as around villages and culturally important sites, buffer zones along waterways, and on steep slopes, or other areas strictly protected by logging regulation. By subtracting inoperable areas from the total area of PdF, a net operable area of PdF can be obtained.
As reported in tropical forest resources assessment project (in the framework of the Global Environment Monitoring System of the FAO in 1984 (FAO, 1984), percentage of inoperable area ranges from 13% of PdF in central and Southern Sumatra to 30% in the whole of Sulawesi in Indonesia. In other tropical countries, inoperable area of PdF is about 30% of the total area of PdF (Kim Phat et al., 2004). For this study, we used 30% as the percentage of inoperable area and therefore the total net operable area of PdF is 330.8 million ha [= 1664*0.284*(1−0.3)] for a 40-year cutting cycle or 8.27 million ha is subject to annual harvesting.
Carbon Stocks for Each Logging Re-entry
Defined as re-logging before the end of the designated cutting cycle, premature re-entry logging has repeatedly caused the loss of carbon stocks until all marketable trees are harvested (Sasaki and Putz, 2009; Putz et al., 2012). To illustrate the impact of premature re-entry logging on carbon stocks on timber production and forest degradation, we assessed carbon stocks in natural PdF in the tropics under two logging methods—CVL and RIL. Under RIL, logging is implemented once at the beginning of a 40-year cutting cycle, while under CVL, two additional harvests are allowed in year 5 and year 20, well before the end of the 40-year cutting cycle. These two logging re-entries are referred to as premature re-entry logging. Equations (1)–(3) estimate forest carbon stocks for CVL and RIL. For the logging at the beginning, 30% of the aboveground biomass (including loss due to logging damages and wastes) are removed. Only merchantable trees (trees with DBH equal or greater than 50 cm) can be removed in the subsequently logging re-entries. Eq. 1 is carbon stocks affected by logging in the beginning of the cutting cycle under both logging methods, while the rest are subsequent premature re-entry logging under the CVL method:
Where
CS0(t), CS1(t), and CS2(t) are carbon stocks (Mg C ha−1) at time t under the RIL, the first premature re-entry and second premature re-entry logging, respectively.
CS0(t0), CS1(t0), and CS2(t0) are carbon stocks (Mg C ha−1) 1 year after RIL, first premature re-entry, and second premature re-entry logging, respectively.
CS0(t0−e1) and CS1(t0−e2) are carbon stocks affected by first re-entry (e1) and second re-entry (e2) until next harvest occurs
CSMAX is the highest carbon stocks of the mature PdF. It is assumed to be 600 Mg C ha−1 for all logging methods. This maximum value is equivalent to the stabilized level of carbon stocks in the forest ecosystems (Krankina and Harmon, 1995).
α = MAI/CS(t0) is the growth rate for each logging method. This calculation is based on the fact that forests grow faster when more space is created by logging (Pinard, 2009). MAI is Mean Annual Increment (Mg C ha−1year−1). Aboveground biomass increment (i.e., MAI) was reported to be about 1.30 Mg C ha−1 year−1 in Amazon forests (Mazzei et al., 2010), and 1.63 Mg C ha−1 year−1 in the northern Borneo (Berry et al., 2010). In a tropical wet forest in Nicaragua, it was estimated to be 2.68 Mg C ha−1 year−1 (Mascaro et al., 2005). For this study, MAI is assumed to be 1.5 Mg C ha−1 year−1.
The literatures on carbon stocks in tropical PdF have become increasingly available (Table 1). The data suggest that the average carbon stocks in PdF are 174.0 ± 11.6 Mg C ha−1 (± is for confidence interval of 90%). We took this average value for initial carbon stocks (aboveground carbon) in PdF prior to logging.
Merchantable trees account for 48.3% of total aboveground biomass in the Southwestern Brazilian Amazon (Cummings et al., 2002), 50.8% in Panama (Chave et al., 2005), and 56.2% in East Kalimantan, Indonesia (Sist et al., 1998). For this study, 50% is used for estimating aboveground biomass of merchantable trees. Biomass removal (biomass loss) at each harvest for both logging methods is assumed to be 30% of the total aboveground biomasses or 60% of the merchantable trees (including biomass in felled log, top log, stump, branches, and leaves). Subsequent first and second premature re-entry logging removes 30% of the total aboveground biomass until biomass of all merchantable trees is removed. When biomass of all merchantable trees is less than 30% of all biomass, that biomass is completely removed. However, no logging is assumed to occur when merchantable trees are completely logged.
Carbon Fluxes in Sawnwood and All Wood Wastes
Important variables or factors relevant to RIL and CVL methods are once-time logging at the beginning of cutting cycle, first and second premature re-entry logging, logging damages (LD) to residual stands, logging wastes (LW) due to tree felling, skidding of felled trees, and transporting of logs to sawmill, sawnwood (SW), and wood wastes (WW) at the sawmill where logs are further processed to produce wood for end use. Longevity of wood utilization is affected by wood processing technology and therefore wood technology (see CIRAD, 2015, for currently developed methods to increase wood durability and lifespan) can increase carbon storage in end-use wood products when appropriate wood processing technology is employed under the RIL. Current wood processing technology used in tropical countries results in a sawnwood recovery rate of only about 40–50% (Enters, 2001). Carbon biomass allocation for each component after harvesting is illustrated in Figure 1, and each wood component and parameter under conventional and RIL can be derived from:
where
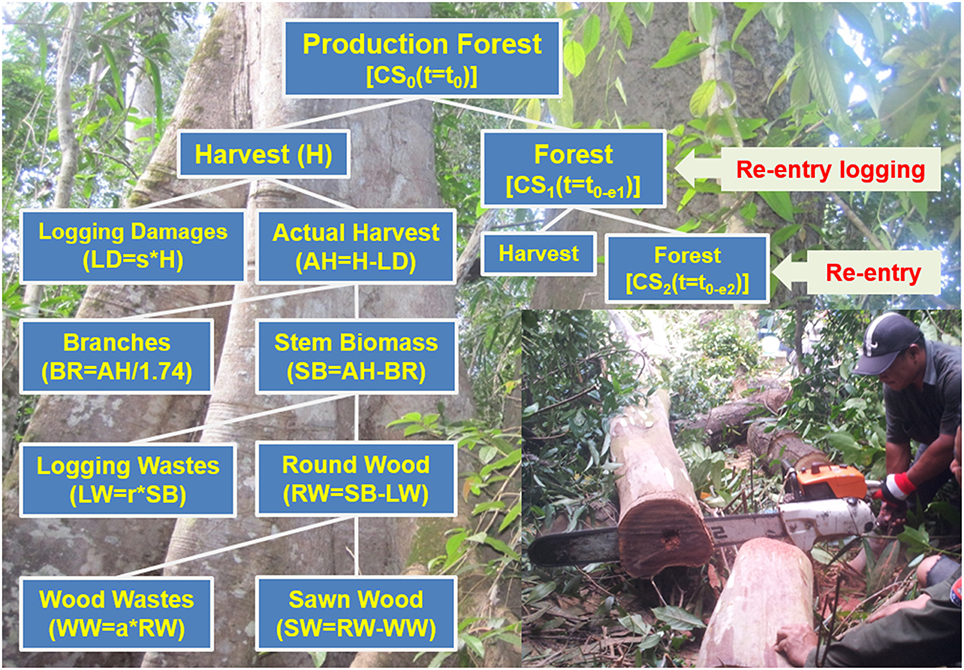
Figure 1. Schematic illustration of carbon biomass allocation after logging and premature re-entry logging. Background photos were taken by the first author in Kampong Thom and Koh Kong provinces in Cambodia.
BL is total Biomass Loss or reduction during each logging entry. BL includes all harvested wood, branches, top logs, and destroyed trees. BL is same for both logging methods.
LD, HB, BR, SB, LW, RW, WW, and SW are Logging Damages, Harvested Biomass, BRanches of harvested wood, Stem Biomass of harvested wood, Logging Wastes, Round Wood, and Sawnwood under CVL and RIL, respectively.
s is logging damage to residual stands calculated as proportional to HB.
a is logging wastes such as top logs, broken trunks and high stumps created during tree felling, log skidding and transporting.
b is wood wastes created during processing of roundwood for sawnwood.
Values of BL, BEF and parameter s, a, and b are provided in Table 2.
Due to similarity of time to decay of wood products, cumulative carbon fluxes (carbon storage) in various harvested wood products can be grouped to two carbon pools. They are sawnwood (SW) and all logging and all wood waste (AW). AW is the sum of LD, BR, LW, and WW. Carbon fluxes in SW and AW (SWf and AWf) can be derived using the first-order equations below:
Where t is elapsed times (years) corresponding to time evolving after logging.
k1, k2 are the decay rates, which are derived from k = ln(2)/τ (τ is time to decay half of its volume or half-life time in year). Subscript f refers to flux. τ and k are dependent on wood processing technology to be employed under CVL or RIL.
Coupled with its trained staff, RIL is assumed to employ the more efficient wood processing technology that can increase end-use wood recovery rate by 10% more than that under the CVL and prolong the half-life time of sawnwood from 30 years as suggested in IPCC 2006 Guidelines to 50 years (IPCC, 2006). The UN's Clean Development Mechanism has an additional goal of assisting non-Annex 1 Parties to achieve sustainable development by providing an incentive for Annex 1 Parties to transfer climate friendly technology to non-Annex 1 Parties—the developing countries mainly in the tropics. It is anticipated that adoption of REDD+ scheme in the future climate agreement will also result in the need for technology transfer to developing countries. Half-life time for AW is 5 years under both logging scenarios.
Results
Sawnwood Production
Although same amount of biomass is removed under both CVL and RIL methods, removed biomass under RIL contains a smaller proportion of logging damages and other wood wastes, and therefore sawnwood production available for end use is much higher. Considering all tropical PdF, the RIL adoption could produce 287 million m3 year−1 of sawnwood production (about 478 million m3 of roundwood) over a 40-year cutting cycle (Table 3) or 29% of global roundwood consumption in 2012 (FAO, 2012). We compared the results of our findings with the data produced by the ITTO because ITTO is the only intergovernmental organization that has maintained good statistical data on global tropical timber trade. ITTO's member countries represent about 80% of the world's tropical forests and 90% of the global tropical timber trade. ITTO's member countries produced 173.6 million m3 of roundwood in 2011, or about an estimate of 204 million m3 from all tropical forests. Given the fact that up to 87% of logging in the tropics is illegal (Hansen and Treue, 2008; Lawson and MacFaul, 2010; Lawson, 2014) and was usually unreported (Colfer and Resosudarmo, 2002; Asner et al., 2005), and by assuming an average illegal logging rate of 60% (Lawson and MacFaul, 2010), a conservative estimate of roundwood production using the figure reported by ITTO would be 510 million m3 [= 204/(1−0.6)].
Our model suggests that sawnwood production under RIL is 124.7 m3 (207.8 m3 of roundwood) or 77.0% higher than that under the CVL if premature re-entry logging is prevented (Table 3). As first premature re-entry logging is allowed in the 5th year, the CVL method can produce total sawnwood similar to that of RIL. The CVL method with two logging re-entries produces by 345.9 million m3 of sawnwood (692 million m3 of roundwood) or 20.6% higher than that from RIL when sawnwood from the second premature re-entry logging in the 20th year is also included in the calculation (Table 3). However, when future commercial wood availability is compared (88.0 m3 ha−1 remaining at the year 40 under RIL compared to only 27.3 m3 under the CVL as shown in Figure 2X), the RIL adoption results in a 222% [222 = (88.0–27.3)/27.3] higher availability of merchantable timber than under CVL. Although total wood production from all premature re-entry logging is higher, CVL is likely to destroy all merchantable trees, and therefore putting tropical PdF at risk of being cleared for industrial agricultures as logging option is no longer feasible economically.
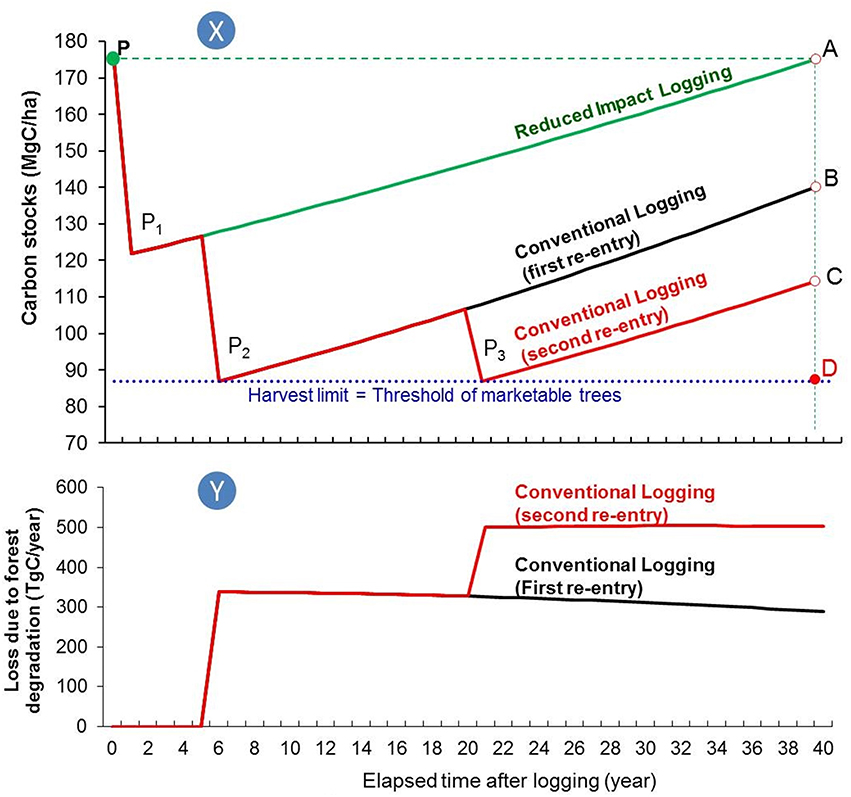
Figure 2. Forest carbon stocks, storage, and loss in a typical hectare of lowland tropical forest affected by harvest methods and re-entry logging. Under reduced-impact logging (X), carbon stocks dropped 30% to P1 after logging but carbon stocks recovered to the pre-logging level (A) after 40 years. If premature re-entry logging is allowed at the 5th and 20th years and CVL practices are employed, carbon stocks continue to drop to point P2 (losing all marketable trees) and P3, respectively. Commercial harvests for timber are unlikely when stocks are below D, but wood extraction for charcoal might continue. Over a 40-year time span, carbon stocks can recover back to point B and C (reaching 81–66% of pre-logging) if the area is not cleared. Reduced emissions from forest degradation are about 35–61 Mg C ha−1 over a 40-year period if the first and second episodes of premature re-entry logging are prevented, respectively. At the global level, carbon loss (Y) was derived by subtracting the carbon stocks under RIL from the baseline (i.e., carbon stocks under the first and second episodes of premature re-entry logging).
Carbon Stock Changes, Emission Reductions, and Removals
Average carbon stocks decline from 174.0 Mg C ha−1 to 121.8 Mg C 1 year after RIL logging, but recovers to the pre-logging level or higher by year 40th if premature re-entry logging is prevented (Table 4 and point A in Figure 2X), depending upon forest growth rate and harvest intensity. Our model results agree with those of Keller et al. (2004). Similarly in Amazonian Brazil, where premature early-entry logging was prevented, it took only 16 years for forests to recover 100% of their biomass under RIL while during the same period only 77% was recovered under CVL (West et al., 2014). In contrast, under CVL with two premature re-entries, carbon stocks continue to decline until all merchantable trees are harvested (point D in Figure 2X). If premature re-entry logging is allowed, carbon stocks decline by 19.5 and 34.3% for first and second premature logging, respectively. Considering all PdF, and by taking carbon stocks under RIL for meeting the “sustainable management of forests” provision of REDD+ as carbon stocks retained through project activities, and using carbon stocks under CVL as the baseline, preventing first and second premature re-entry logging could reduce emissions of 279.9 and 493.7 Tg C year−1 (1026.4–1810.4 Tg CO2 year−1) respectively, in addition to removing (sequestering) −8.2 Tg C year−1 (−30.1 Tg CO2 year−1) from the atmosphere (Table 4 and Figure 2Y). These emission reductions and removals are equivalent to about 28.8–50.2% of carbon emissions from tropical deforestation between 2000 and 2010 (Baccini et al., 2012) or 93–162% of emission reductions committed by European Union for the second period of the Kyoto Protocol (European Commission, 2013). Furthermore, using logging emission factors of wood extraction and logging damages of 0.82 Mg C m−3 harvested wood in Indonesia (Pearson et al., 2014), total emissions from harvesting 324–692 (324 = 162.1/0.5 and 692 = 345.9/0.5 in Table 3) million m3 of roundwood are 266–567 TgC year−1, respectively. However, in practice, such logging-related emissions were usually unreported (Pearson et al., 2014).
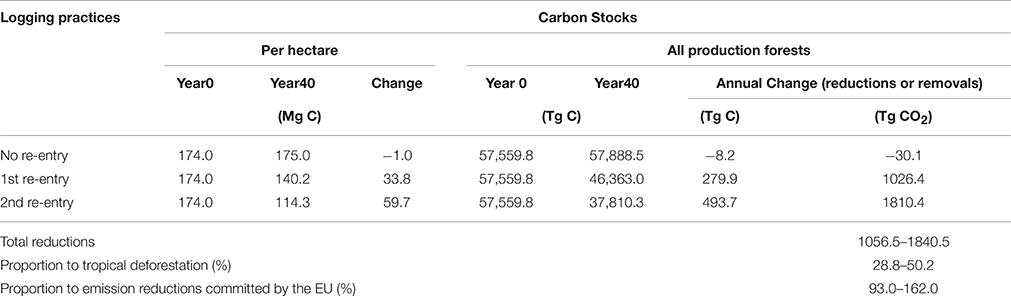
Table 4. Achieving emission reductions and removals by preventing premature re-entry logging in tropical production forests.
Minus (−) in Table 4 refers to carbon sequestration (sinks or removals). Emissions from tropical deforestation was based on Baccini et al. (2012). Emission reductions committed by EU were based on European Commission (2013).
Reducing Fire-Prone Wood Waste by Adopting RIL and Preventing Premature Re-entry Logging
In recent years, increasing fires in selectively logged forests were observed and pose threats to forest management. Humans are the direct cause of most fires in tropical forests and indirectly exacerbate the problem through anthropogenic climate change induced droughts and destructive logging (Cochrane, 2003). The presence of flammable logging wastes coupled with openings of tropical forest canopies render forests very susceptible to destructive fires (Siegert et al., 2001; Gerwing, 2002; Cochrane, 2003). Although CVL could produce more sawnwood when all premature re-entry logging is considered, it creates huge wood waste (371–920 TgC) or up to 183.9% higher if compared to waste created by RIL (Table 3). This wood waste is prone to destructive fires but can be reduced with the adoption of RIL combined with improved logging technology such as use of Logfisher, skyline, yarder, and/or helicopter (Abdul Rahim et al., 2009) and wood processing technology such as use of wood-Mizer milling technology rather than freehand chainsaw or chainsaw with frame attachments (Owusu et al., 2011). Forests become prone to destructive fires when the canopy is opened by selective logging and huge amounts of wood waste are left after timber felling. According to Matricardi et al. (2013), the total forest area affected by fires, selective logging, and a combination of logging and fires in the Amazon tripled from 5,889, 5,588, and 392 km2 in 1992 to 9038, 24,188, and 2471 km2 in 1999, respectively.
Carbon Storage in Harvested Wood Products
As short-life wood waste is reduced and long-life sawnwood is increased, more carbon storage in harvested wood products can be achieved under RIL+. Using equations (12, 13), adopting RIL+ could result in carbon storage in sawnwood of 82.6 TgC year−1, while it is only 39.7 TgC under the CVL if compared to one harvest under the “RIL+” (Figure 3). Carbon storage in all wood waste is 370.9 TgC and 324.1 TgC at the start of harvesting but reduces to 1.5 TgC and 1.3 TgC at the year 40th, representing the annual average storage of 69.6 TgC and 60.9 TgC under CVL and RIL+, respectively over a 40-year cutting cycle (Figure 3). Since carbon storage in all waste under RIL is 8.7 TgC (69.6–60.9) smaller than that under CVL, adopting RIL+ for managing all PdF could reduce emissions by 34.1 TgC year−1 (125 TgCO2) or about 21% of emission reductions committed by the United Kingdom for the first commitment period of the Kyoto Protocol between 2008 and 2012. If sawnwood and all waste obtained under the CVL from all premature re-entry loggings are compared, carbon storage in wood products (sawnwood plus waste) under CVL is higher but its declines faster because of a greater proportion and rapid decay of short-life wood waste.
Discussion
One of the major problems in managing tropical production forest is premature re-entry logging of stands that have not been allowed to recuperate for the entire length of the cutting cycle determined to be needed to sustain timber yields (Putz et al., 2012). Premature re-entry logging is driven by timber scarcity and prices, often coupled with governance failures; when market demands or timber prices increase, forests are often harvested prematurely. Low harvest costs due to the availability of previously constructed logging roads further encourage premature re-entry logging (Ravenel, 2004). The same phenomenon is also associated with changes in governmental and corporate leadership when concession maps are commonly redrawn and previous harvest plans are disregarded. Furthermore, in anticipation of unfavorable treatment by newly installed governments, logging companies often harvest as much timber as possible prior to or during elections (Burgess et al., 2012). Future climate agreements should include binding agreements that validated projects adopting RIL shall not be interrupted before the end of a harvest cycle.
In the wood processing sector, rapid technological developments provide new hope for increasing the use and lengthening the longevity of wood products (Fleming et al., 2014). Since RIL increases the amount of wood product from the same amount of harvested timber, RIL adoption can increase carbon storage in long-lived wood products and reduce short-lived wood waste. Furthermore, technological advancements in the logging industry can create more jobs (Clark, 2004) and facilitate low-carbon based economies. To encourage the use of advanced technologies for higher carbon retention in wood products, future climate agreement should also include carbon credits for long-lived wood products as eligible credits for trading.
As up to 87% of the timber harvests can be illegal in a given (Lawson, 2014), about $30–100 billion year−1 is lost globally due to this illegal logging (UNEP INTERPOL, 2013). Forest certification schemes have played an important role in certifying the timber products from sustainably managed forests (Durst et al., 2006; Cerutti et al., 2011; Putz et al., 2012), thereby reducing illegal and unsustainable logging practices to some extent (Cerutti et al., 2011; Kishor and Lescuyer, 2012). Nevertheless, as forest certification is based commonly on paper, and logging in the tropics is being practiced with corruption and falsification of logging permits (Nellemann, 2012), forest certification alone is still vulnerable to document falsification. A combination of forest certification and newly developed timber tracking technologies (Dormontt et al., 2015) to monitor and control wood products is critical for reducing illegal logging and for the prevention of premature re-entry logging. According to a recently established working group of the United Nations Office on Drugs and Crime, a range of technologies are available to verify timber source claims (e.g., anatomy, stable isotopes), but DNA timber tracking technology seems like the best source of information about the tree species, country, forest concession and even individual tree from which wood was derived (Dormontt et al., 2015). Further development, integration, and support for this verification technologies is now required.
Costs are currently the major concern for adopting the RIL or RIL+. RIL has higher costs for pre-harvest and harvest planning activities, and infrastructure development (depending on locations, it may also include costs for helicopter or cable system for logging on steep terrain or wet soil) and for adopting chainsaws to increase wood recovery rate (Holmes et al., 2002; Medjibe and Putz, 2012) but lower costs for harvest operations (Sasaki and Putz, 2009). In Malaysia, although average costs for helicopter logging are $50–60 compared to US$10 m−3 for CVL, logging damage to surrounded trees were 3.8 times lower than damage created by CVL (Abdul Rahim et al., 2009). Our previous study (Medjibe and Putz, 2012) found that the costs for RIL techniques are lower in four of the ten case studies than costs of using CVL for logging in PdF in Brazil, Gabon, Guyana, and Malaysia. On average, costs for adopting RIL are US$1.43 ± 1.57 (90% of CI) higher than that under CVL (Table 5). For the whole tropical PdF, total additional costs for adopting RIL are about US$821 million year−1 in order to harvest 574 million m3 of roundwood. The cost for certifying forests where RIL has been adopted are estimated to be US$2.55 to $10.06 m−3 for every 5 years in Brazil and Indonesia, respectively (White, 2011) or $0.51 to $2.00 m−3 year−1. Assuming $2.00 m−3 for certifying forests implementing RIL, it would cost US$1969 million year−1 to certify all 574 million m3 of roundwood.
As adopting RIL can result in emission reductions of 288–502 TgC (1056–1840 TgCO2), the costs for adopting RIL techniques with certified timber products are US$0.60–1.86 per Mg CO2. This is well below the carbon price for reducing deforestation project traded in the voluntary carbon markets in 2013 and 2012, which was US$ 4.20 and 7.40, respectively. As Paris Agreement was reached at the COP21 by the parties to the UNFCCC to limit the greenhouse gas emission emissions, new demand for carbon is likely to increase, especially after this agreement enters into effect after 55 countries responsible for 55% of global carbon emissions ratify the agreement (UNFCCC, 2015). Thus, carbon price is also likely to increase. Furthermore, costs for DNA timber tracking (to enforce legislation as well as to reduce export/import of illegally sources timber) is less than 0.01% of the timber values but these costs can be covered from the premium price of the certified timber. Although using DNA technology to track the tropical timber trade is still in its infancy stage, a study on 38,000 log transactions from sustainable forestry in the Japanese market found that certified timber has a price premium of 1.4–4.0% depending on timber species (Yamamoto et al., 2014). Furthermore, as some governments such as European Union, Australia, the United States of America began to enforce the legality of timber import from tropical countries, DNA timber tracking is the only natural identification technology that cannot be falsified (Lowe and Cross, 2011). Financial incentives are therefore still required to get tropical forest industries to reform their harvesting practices such as appropriate logging planning; use of trained crews for forest inventory, tree marking, tree directional felling, log skidding and trimming; and supervision of logging, to adopt less destructive technologies whenever possible such as the use of directional felling, cable system, or helicopter logging system or a combination, and to increase efficiencies (i.e., through the use of trained crews and advanced chainsaws at wood processing mills) from harvest to the final product.
Conclusion
Improved tropical forest management is an important element of the UNFCCC's REDD+ scheme that also supports the long-term supply of commercial timber products and safeguards local social welfare and biodiversity. Our study suggests that about 28% of the total area of tropical forests (i.e., all the officially designated tropical PdF) can be targeted for RIL+ and RIL+ can produce 287 million m3 year−1 of sawnwood, reduce emissions of up to 494 Tg C year−1 or 50% of carbon emissions from tropical deforestation, and increase carbon storage of 8.2 Tg C year−1 in sawnwood, while preventing logging-promoted and enhanced forest fires. RIL+ also requires technological development because it requires proper planning at every stage of logging and wood processing operations. Our study also suggests that technological development throughout the logging industry can further reduce carbon emissions through the use of improved machinery used in logging operations, transport, wood processing and source verification. Recent advances in DNA timber tracking technology along with forest certification scheme will further enforce the legality of tropical timber import. To achieve long-term production of tropical sawnwood and logging-induced emission reductions, financial incentives of US$ 1–2 billion per year or up to $2 Mg CO2 will be needed at least initially for the management of all tropical PdF.
Author Contributions
NS designed research and performed data analysis. NS, GA, YP, WK, PD, HM, IA, AL, LK, and FP equally wrote the article.
Conflict of Interest Statement
The authors declare that the research was conducted in the absence of any commercial or financial relationships that could be construed as a potential conflict of interest.
Acknowledgments
The first author was supported by a Grant-in-Aid for Scientific Research Category A (No. 24252002) from the Ministry of Education, Culture, Sports, Science and Technology of Japan for his research activities at the University of Adelaide.
References
Abdul Rahim, S. A., Mohd Shahwahid, H. O., and Zariyawati, M. A. (2009). A comparison analysis of logging cost between conventional and reduce impact logging practices. Int. J. Econ. Manage. 3, 354–366.
Asner, G. P., Broadbent, E. N., Oliveira, P. J. C., Keller, M., Knapp, D. E., and Silva, J. N. (2006). Condition and fate of logged forests in the Brazilian Amazon. Proc. Natl. Acad. Sci. U.S.A. 103, 12947–12950, doi: 10.1073/pnas.0604093103
Asner, G. P., Knapp, D. E., Broadbent, E. N., Oliveira, P. J. C., Keller, M., and Silva, J. N. M. (2005). Selective logging in the Brazilian Amazon. Science 310, 480–482. doi: 10.1126/science.1118051
Asner, G. P., Rudel, T. K., Aide, T. M., Defries, R., and Emerson, R. A. (2009). contemporary assessment of change in humid tropical forests. Conserv. Biol. 23, 1386–1395. doi: 10.1111/j.1523-1739.2009.01333.x
Baccini, A., Goetz, S. J., Walker, W. S., Laporte, N. T., Sun, M., Sulla-Menashe, D., et al. (2012). Estimated carbon dioxide emissions from tropical deforestation improved by carbon-density maps. Nat. Clim. Chang. 2, 182–185. doi: 10.1038/nclimate1354
Barreto, P., Amaral, P., Vidal, E., and Uhl, C. (1998). Costs and benefits of forest management for timber production in eastern Amazonia. For. Ecol. Manage. 108, 9–26. doi: 10.1016/s0378-1127(97)00251-x
Berry, N. J., Phillips, O. L., Lewis, S. L., Hill, J. K., Edwards, D. P., Tawatao, N. B., et al. (2010). The high value of logged tropical forests: lessons from northern Borneo. Biodivers. Conserv. 19, 985–997. doi: 10.1007/s10531-010-9779-z
Bicknell, J. E., Struebig, M. J., Edwards, D. P., and Davies, Z. G. (2014). Improved timber harvest techniques maintain biodiversity in tropical forests. Curr. Biol. 24, R1119–R1120. doi: 10.1016/j.cub.2014.10.067
Blanc, L., Echard, M., Herault, B., Bonal, D., Marcon, E., Chave, J., et al. (2009). Dynamics of above-ground carbon stocks in a selectively logged tropical forest. Ecol. Appl. 19, 1397–1404. doi: 10.1890/08-1572.1
Boltz, F., Carter, D. R., Holmes, T. P., and Pereira, R., J. (2001). Financial returns under uncertainty for conventional and reduced-impact logging in permanent production forests of the Brazilian Amazon. Ecol. Econ. 39, 387–398. doi: 10.1016/S0921-8009(01)00231-2
Brown, S. (1997). Estimating Biomass and Biomass Change of Tropical Forests: A Primer. Rome: FAO Forestry Paper, 134.
Brown, S., Casarim, F. M., Grimland, S. K., and Pearson, T. (2011). Carbon Impacts from Selective Logging of Forests in Berau, East Kalimantan, Indonesia. Final Report to The Nature Conservancy. Winrock International. Available online at: https://goo.gl/qL3Zf0 (Accessed 20/11/2015)
Brown, S., Pearson, T., Moore, N., Parveen, A., Ambagis, S., and Shoch, D. (2005). Impact of Logging on Carbon Stocks of Forests: Republic of Congo As a Case Study. Report submitted to the United States Agency for International Development. Winrock International. Available online at: http://goo.gl/CQJZpq (Accessed 18/11/2015).
Bryan, J., Shearman, P., Ash, J., and Kirkpatrick, J. B. (2010). Impact of logging on aboveground biomass stocks in lowland rain forest, Papua New Guinea. Ecol. Appl. 20, 2096–2103. doi: 10.1890/09-1818.1
Burgess, N. D., Bahane, B., Clairs, T., Danielsen, F., Dalsgaard, S., Funder, M., et al. (2010). Getting ready for REDD +in Tanzania: a case study of progress and challenges. Oryx 44, 339–351. doi: 10.1017/S0030605310000554
Burgess, R., Hansen, M., Olken, B. A., Potapov, P., and Sieber, S. (2012). The political economy of deforestation in the tropics. Q. J. Econ. 127, 1707–1754. doi: 10.1093/qje/qjs034
Bustamante, M. C., Roitman, I., Aide, T., Alencar, A., Anderson, L., Aragão, L. E., et al. (2016). Towards an integrated monitoring framework to assess the effects of tropical forest degradation and recovery on carbon stocks and biodiversity. Glob. Chang Biol. 22, 92–109. doi: 10.1111/gcb.13087
Cerutti, P., Tacconi, L., Nasi, R., and Lescuyer, G. (2011). Legal vs. certified timber: preliminary impacts of forest certification in Cameroon. Forest Policy Econ. 13, 184–190. doi: 10.1016/j.forpol.2010.11.005
Chave, J., Andalo, C., Brown, S., Cairns, M. A., Chambers, J. Q., Eamus, D., et al. (2005). Tree allometry and improved estimation of carbon stocks and balance in tropical forests. Oecologia 145, 87–99. doi: 10.1007/s00442-005-0100-x
CIRAD (2015). Improving the Lifespan of Wood Products: Natural Durability and Low-Impact Preservative Treatments. CIRAD Online Document. Available online at: http://goo.gl/wZUZW5 (Accessed 20/11/2015).
Clark, J. (2004). Forest policy for sustainable commodity wood production: an examination drawing on the Australian experience. Ecol. Econ. 50, 219–232. doi: 10.1016/j.ecolecon.2004.03.032
Colfer, C. J. P., and Resosudarmo, I. A. P. (eds.). (2002). Which Way Forward? People, Forests and Policy Making in Indonesia. Washington, DC: Resources for the Future; Bogor: CIFOR; Singapore: Institute of Southeast Asian Studies.
Culmsee, H., Leuschner, C., Moser, G., and Pitopang, R. (2010). Forest aboveground biomass along an elevational transect in Sulawesi, Indonesia, and the role of Fagaceae in tropical montane rain forests. J. Biogeogr. 37, 960–974. doi: 10.1111/j.1365-2699.2009.02269.x
Cummings, D. L., Boone Kauffman, J., Perry, D. A., and Flint Hughes, R. (2002). Aboveground biomass and structure of rainforests in the southwestern Brazilian Amazon. For. Ecol. Manage. 163, 293–307. doi: 10.1016/S0378-1127(01)00587-4
Dagang, A. A., Richter, F., Hahn-Schilling, and Manggil, P. (2002). “Financial and economic analyses of conventional and reduced impact harvesting systems in Sarawak,” in Applying Reduced Impact Logging to Advance Sustainable Forest Management, eds T. Enters, P. B. Durst, G. B., Applegate, C. S. Kho Peter, and G. Man (FAO RAP Publication). Availabe online at: http://goo.gl/OEOLHt (Accessed 25/11/2015).
Djomo, A. N., Knohl, A., and Gravenhorst, G. (2011). Estimations of total ecosystem carbon pools distribution and carbon biomass current annual increment of a moist tropical forest. For. Ecol. Manage. 261, 1448–1459. doi: 10.1016/j.foreco.2011.01.031
Dormontt, E. E., Boner, M., Braun, B., Breulmann, G., Degen, B., Espinoza, E., et al. (2015). Forensic timber identification: it's time to integrate disciplines to combat illegal logging. Biol. Conserv. 191, 790–798. doi: 10.1016/j.biocon.2015.06.038
Durst, P. B., McKenzie, P. J., Brown, C. L., and Appanah, S. (2006). Challenges facing certification and eco-labelling of forest products in developing countries. Int. Fores. Rev. 8, 193–200. doi: 10.1505/ifor.8.2.193
Dwiprabowo, H., Grulois, S., Sist, S., and Kartawinata, K. (2002). Cost–Benefit Analysis of Reduced-Impact Logging in a Lowland Dipterocarp Forest of Malinau, East Kalimantan. Forest, Science and Sustainability: The Bulungan Model Forest. ITTO Technical Report, Yokohama.
Enters, T. (2001). Trash or Treasure? Logging and Mill Residues in Asia and the Pacific. Bangkok: FAO, RAP Publication. FAO.
European Commission (2013). Ratification of the Second Commitment Period of the Kyoto Protocol to the United Nations Framework Convention on Climate Change. Proposal for a COUNCIL DECISION, COM 768 final. Brussels: European Commission.
FAO (1984). Tropical Forest Resources Assessment Project (in the Framework of the Global Environment Monitoring System - GEMS). Rome: FAO.
FAO (2010). Global Forest Resources Assessment 2010. Rome: FAO. Available online at: http://www.fao.org/docrep/013/i1757e/i1757e.pdf (Accessed 10/11/2015).
FAO (2012). Global Forest Products Facts and Figures. Rome: FAO. Available online at: http://goo.gl/7leJWt (Accessed 12/11/2015).
Figueira, A. M. S., Miller, S. D., de Sousa, C. A. D., Menton, M. C., Maia, A. R., da Rocha, H. R., et al. (2008). Effects of selective logging on tropical forest tree growth. J. Geophys. Res. 113, 1–11. doi: 10.1029/2007JG000577
Fleming, P., Smith, S., and Ramage, M. (2014). Measuring-up in timber: a critical perspective on mid- and high-rise timber building design. Archit. Res. Q. 18, 20–30. doi: 10.1017/S1359135514000268
Fox, J. C., Yosi, C. K., Nimiago, P., Oavika, F., Pokana, J. J. N., Lavong, K., et al. (2010). Assessment of aboveground carbon in primary and selectively harvested tropical forest in Papua New Guinea. Biotropica 42, 410–419. doi: 10.1111/j.1744-7429.2009.00617.x
Gerwing, J. J. (2002). Degradation of forests through logging and fire in the eastern Brazilian Amazon. For. Ecol. Manage. 157, 131–141. doi: 10.1016/S0378-1127(00)00644-7
Hansen, C. P., and Treue, T. (2008). Assessing illegal logging in Ghana. Int. Forest. Rev. 10, 573–590. doi: 10.1505/ifor.10.4.573
Healey, J. R., Price, C., and Tay, J. (2000). The cost of carbon retention by reduced impact logging. For. Ecol. Manage. 139, 237–255. doi: 10.1016/S0378-1127(00)00385-6
Holmes, T. P., Blate, G. M., Zweede, J. C., Pereira, J.r, R., Barreto, P., Boltz, F., et al. (2002). Financial and ecological indicators of reduced impact logging performance in the eastern Amazon. For. Ecol. Manage. 163, 93–110. doi: 10.1016/S0378-1127(01)00530-8
Huang, M., and Asner, G. P. (2010). Long-term carbon loss and recovery following selective logging in Amazon forests. Glob. Biogeochem. Cycles 24, 1–15. doi: 10.1029/2009GB003727
Imai, N., Samejima, H., Langner, A., Ong, R. C., Kita, S., Titin, J., et al. (2009). Co-benefits of sustainable forest management in biodiversity conservation and carbon sequestration. PLoS ONE 4:e8267. doi: 10.1371/journal.pone.0008267
IPCC (2003). Good Practice Guidance for Land Use, Land Use Change and Forestry, eds J. Penman, M. Gytarsky, T. Hiraishi, T. Krug, D. Kruger, R. Pipatti et al. Hayama: IPCC/OECD/IEA/IGES.
IPCC (2006). IPCC Guidelines for National Greenhouse Gas Inventories. Prepared by the National Greenhouse Gas Inventories Programme. Kanagawa: IGES.
ITTO (2002). Guidelines for the Restoration, Management and Rehabilitation of Degraded and Secondary Tropical Forests. Yokohama: ITTO.
Johns, J. S., Barreto, P., and Uhl, C. (1996). Logging damage during planned and unplanned logging operations in the eastern Amazon. For. Ecol. Manage. 89, 59–77. doi: 10.1016/S0378-1127(96)03869-8
Keller, M., Palace, M., Asner, G. P., Pereira, R., and Silva, J. N. M. (2004). Coarse woody debris in undisturbed and logged forests in the eastern Brazilian Amazon. Glob. Chang. Biol. 10, 784–795. doi: 10.1111/j.1529-8817.2003.00770.x
Kim Phat, N., Knorr, W., and Kim, S. (2004). Appropriate measures for conservation of terrestrial carbon stocks - analysis of trends of forest management in Southeast Asia. For. Ecol. Manage. 191, 283–299. doi: 10.1016/j.foreco.2003.12.019
Kim Phat, N., Ouk, S., Uozumi, Y., and Ueki, T. (2000). Stand dynamics of Dipterocarp trees in Cambodia's evergreen forest and management implications- a case study in Sandan district, Kampong Thom. Jpn. J. For. Plan. 6, 13–23.
Kishor, N., and Lescuyer, G. (2012). Controlling illegal logging in domestic and international markets by harnessing multi-level governance opportunities. Int. J. Commons 6, 255–270. doi: 10.18352/ijc.327
Krankina, O. N., and Harmon, M. E. (1995). The impact of intensive forest management on carbon stores in forest ecosystems. World Resour. Rev. 6, 161–177.
Lasco, R. D., Macdicken, K. G., Pulhin, F. B., Guillermo, I. Q., Sales, R. F., and Cruz, R. V. O. (2006). Carbon stocks assessment of a selectively logged dipterocarp forest and wood processing mill in the Philippines. J. Trop. For. Sci. 18, 212–221.
Lawson, S. (2014). Illegal Logging in the Democratic Republic of the Congo. Energy, Environment and Resources EER PP 2014/03. London: Chatham House. Available online at: http://goo.gl/rM3zP6 (Accessed 20/11/2015).
Lawson, S., and MacFaul, L. (2010). Illegal Logging and Related Trade: Indicators of the Global Responses. London: Chatham House, 154. Available online at: http://goo.gl/DFskg1 (Accessed 7 June 2016)
Lowe, A. J., and Cross, H. B. (2011). The application of DNA methods to timber tracking and origin verification. IAWA J. 32, 251–262. doi: 10.1163/22941932-90000055
Mascaro, J., Perfecto, I., Barros, O., Boucher, D. H., de la Cerda, I. G., Ruiz, J., et al. (2005). Aboveground biomass accumulation in a tropical wet forest in nicaragua following a catastrophic hurricane disturbance. Biotropica 37, 600–608. doi: 10.1111/j.1744-7429.2005.00077.x
Matricardi, E. A. T., Skole, D. L., Pedlowski, M. A., and Chomentowski, W. (2013). Assessment of forest disturbances by selective logging and forest fires in the Brazilian Amazon using Landsat data. Int. J. Remote Sens. 34, 1057–1086. doi: 10.1080/01431161.2012.717182
Mazzei, L., Sist, P., Ruschel, A., Putz, F. E., Marco, P., Pena, W., et al. (2010). Above-ground biomass dynamics after reduced-impact logging in the Eastern Amazon. For. Ecol. Manage. 259, 367–373. doi: 10.1016/j.foreco.2009.10.031
Medjibe, V. P., and Putz, F. E. (2012). Cost comparisons of reduced-impact and conventional logging in the tropics. J. For. Econ. 18, 242–256. doi: 10.1016/j.jfe.2012.05.001
Medjibe, V. P., Putz, F. E., Starkey, M. P., Ndouna, A. A., and Memiaghe, H. R. (2011). Impacts of selective logging on above-ground forest biomass in the Monts de Cristal in Gabon. For. Ecol. Manage. 262, 1799–1806. doi: 10.1016/j.foreco.2011.07.014
Miller, S. D., Goulden, M. L., Hutrya, L. R., et al. (2011). Reduced impact logging minimally alters tropical rainforest carbon and energy exchange. Proc. Natl. Acad. Sci. U.S.A. 108, 19431–19435. doi: 10.1073/pnas.1105068108
Morel, A. C., Saatchi, S. S., Malhi, Y., Berry, N. J., Banin, L., Burslem, D., et al. (2011). Estimating aboveground biomass in forest and oil palm plantation in Sabah, Malaysian Borneo using ALOS PALSAR data. For. Ecol. Manage. 262, 1786–1798. doi: 10.1016/j.foreco.2011.07.008
Nasi, R., and Frost, P. G. H. (2009). Sustainable forest management in the tropics: is everything in order but the patient still dying? Ecol. Soc. 14:40. Available online at: http://www.ecologyandsociety.org/vol14/iss2/art40/
Nellemann, C. (2012). Green Carbon, Black Trade: Illegal Logging, Tax Fraud and Laundering in the Worlds Tropical Forests. A Rapid Response Assessment. Arendal: United Nations Environment Programme, GRIDArendal.
Norman, M., Caravani, A., Nakhooda, S., Watson, C., and Schalatek, L. (2014). Climate Finance Thematic Briefing: REDD+ Finance, Climate Finance Fundamentals, Climate Policy Initiative. London: Overseas Development Institute. Available online at: http://goo.gl/2OphWX (Accessed 19/11/2015).
Okuda, T., Suzuki, M., Numata, S., Yoshida, K., Nishimura, S., Adachi, N., et al. (2004). Estimation of aboveground biomass in logged and primary lowland rainforests using 3-D photogrammetric analysis. For. Ecol. Manage. 203, 63–75. doi: 10.1016/j.foreco.2004.07.056
Owusu, F. W., Appiah, J. K., Damnyag, L., and Blay, D. (2011). Comparative analysis of recovery efficiencies of some milling techniques in Ghana. Ghana J. Forest. 27, 87–100.
Palace, M., Sullivan, F. B., Ducey, M., and Herrick, C. (2016). Estimating tropical forest structure using a terrestrial lidar. PLoS ONE 11:e0154115. doi: 10.1371/journal.pone.0154115
Pan, Y., Birdsey, R. A., Fang, J., Houghton, R., Kauppi, P. E., et al. (2011). A large and persistent carbon sink in the world's forests. Science 333, 988–993. doi: 10.1126/science.1201609
Pearson, T. R. H., Brown, S., and Casarim, F. M. (2014). Carbon emissions from tropical forest degradation caused by logging. Environ. Res. Lett. 9:034017. doi: 10.1088/1748-9326/9/3/034017
Pearson, T., Walker, S., Grimland, S., and Brown, S. (2006). Impact of Logging on Carbon Stocks of Forests : The Brazilian Amazon as a Case Study. Arlington: Winrock International. Available online at: http://goo.gl/XgLAeQ (Accessed 13/11/2015).
Pinard, M. (2009). “Carbon-neutral logging in a Malaysian rainforest: reduced collateral damage fosters rapid recovery,” in Realising REDD+: National Strategy and Policy Options, ed A. Angelsen (Bogor: Center for International Forestry Research), 256.
Pinard, M. A., Putz, F. E., and Tay, J. (2000). Lessons learned from the implementation of reduced-impact logging in hilly terrain in Sabah, Malaysia. Int. Forest. Rev. 2, 33–39.
Pinard, M., and Putz, F. E. (1996). Retaining forest biomass by reducing logging damage. Biotropica 28, 278–295. doi: 10.2307/2389193
Putz, F. E., Zuidema, P. A., Synnott, T., Peña-Claros, M., Pinard, M. A., et al. (2012). Sustaining conservation values in selectively logged tropical forests: the attained and the attainable. Conserv. Lett. 5, 296–303. doi: 10.1111/j.1755-263X.2012.00242.x
Ravenel, R. M. (2004). Community-based logging and de facto decentralization: illegal logging in the Gunung Palung area of West Kalimantan, Indonesia. J. Sust. Forest. 19, 213–237. doi: 10.1300/J091v19n01_10
Rutishauser, E., Hérault, B., Baraloto, C., Blanc, L., Descroix, L., Sotta, E. D., et al. (2015). Rapid tree carbon stock recovery in managed Amazonian forests. Curr. Biol. 25, R775–R792. doi: 10.1016/j.cub.2015.07.034
Saharudin, A. S., Brodie, J. D., and Sessions, J. (1999). Analysis of two alternative harvesting systems in Peninsular Malaysia: sensitivity analysis of costs, logging damage and buffers. J. Trop. For. Sci. 11, 809–821.
Sasaki, N., Chheng, K., and Ty, S. (2012). Managing production forests for timber production and carbon emission reductions under the REDD+ scheme. Environ. Sci. Policy 23, 35–44. doi: 10.1016/j.envsci.2012.06.009
Sasaki, N., and Putz, F. E. (2009). Critical need for new definitions of ‘forest’ and ‘forest degradation’ in global climate change agreements. Conserv. Lett. 2, 226–232. doi: 10.1111/j.1755-263X.2009.00067.x
Schwab, O., Pulkki, R., and Bull, G. Q. (2001). Reduced Impact Logging in Tropical Forests: Literature Synthesis, Analysis and Prototype Statistical Framework. Forest Production Division, Working Paper Series FOP/08. Rome: FAO. Available online at: http://www.fao.org/docrep/007/ae359e/ae359e00.htm (Accessed 23/10/2015).
Siegert, F., Ruecker, G., Hinrichs, A., and Hoffmann, A. A. (2001). Increased damage from fires in logged forests during droughts caused by El Niño. Nature 414, 437–440. doi: 10.1038/35106547
Simpson, W. T. (1999). “Drying and control of moisture content and dimensional changes,” in Wood Handbook - Wood as an Engineering Material. General Technical Report FPLGTR-113 (Madison: USDA Forest Service, Forest Products Laboratory), Chap. 12, 12.1–12.20.
Sist, P., Mazzei, L., Blanc, L., and Rutishauser, E. (2014). Large trees as key elements of carbon storage and dynamics after selective logging in the Eastern Amazon. For. Ecol. Manage. 318, 103–109. doi: 10.1016/j.foreco.2014.01.005
Sist, P., Nolan, T., Bertault, J. G., and Dykstra, D. (1998). Harvesting intensity versus sustainability in Indonesia. For. Ecol. Manage. 108, 251–260. doi: 10.1016/S0378-1127(98)00228-X
Sist, P., and Saridan, A. (1999). Stand structure and floristic composition of a primary lowland dipterocarp forest in East Kalimantan. J. Trop. For. Sci. 11, 704–722.
Stanley, S. A. (2009). Preliminary Biomass Estimate in Pt. Mamberamo Alas Mandiri Concession Papua, Indonesia. Report for Conservation International. Available online at: http://goo.gl/zixUfv (Accessed 16/11/2015).
Stas, S. M. (2014). Above-Ground Biomass and Carbon Stocks in a Secondary Forest in Comparison with Adjacent Primary Forest on Limestone in Seram, the Moluccas, Indonesia. CIFOR, Bogor, Working Paper 145. Available online at: http://goo.gl/RIR1ym (Accessed 23/10/2015).
UNEP and INTERPOL (2013). UNEP and INTERPOL Assess Impacts of Environmental Crime on Security and Development. Press Release, 6 November 2013. Online document Available online at: http://goo.gl/WHazqA (Accessed 08 June 2016).
UNFCCC (2014). Green Climate Fund Exceeds $10 Billion. PRESS RELEASE/10. DEC, 2014. Online document: Available online at: http://goo.gl/dTsnqC (Accessed 30 June 2016).
UNFCCC (2015). Adoption of the Paris Agreement. UNFCCC/CP/2015/L.9/Rev.1. Online Document: Available online at: https://unfccc.int/resource/docs/2015/cop21/eng/l09r01.pdf (Accessed 01/3/2016).
Van der Hout, P. (1999). Reduced Impact Logging in the Tropical Rain Forest of Guyana: Ecological, Economic and Silvicultural Consequences. Tropenbos-Guyana Series 6. Georgetown: Tropenbos-Guyana Programme.
West, T. A. P., Vidal, E., and Putz, F. E. (2014). Forest biomass recovery after conventional and reduced-impact logging in Amazonian Brazil. For. Ecol. Manage. 314, 59–63. doi: 10.1016/j.foreco.2013.11.022
White, G. (2011). Suppliers or Supply Chain Partners? Pay More to Get More. Understanding FSC costs. Washington, DC: Global Forest & Trade, World Wildlife Fund.
Keywords: forest degradation, production forest, REDD, selective logging, timber production, carbon emissions
Citation: Sasaki N, Asner GP, Pan Y, Knorr W, Durst PB, Ma HO, Abe I, Lowe AJ, Koh LP and Putz FE (2016) Sustainable Management of Tropical Forests Can Reduce Carbon Emissions and Stabilize Timber Production. Front. Environ. Sci. 4:50. doi: 10.3389/fenvs.2016.00050
Received: 17 March 2016; Accepted: 19 July 2016;
Published: 03 August 2016.
Edited by:
Luuk Fleskens, University of Leeds, UKReviewed by:
Shoana Humphries, Earth Innovation Institute, USARobert Jandl, Bundesamt und Forschungszentrum für Wald, Austria
Copyright © 2016 Sasaki, Asner, Pan, Knorr, Durst, Ma, Abe, Lowe, Koh and Putz. This is an open-access article distributed under the terms of the Creative Commons Attribution License (CC BY). The use, distribution or reproduction in other forums is permitted, provided the original author(s) or licensor are credited and that the original publication in this journal is cited, in accordance with accepted academic practice. No use, distribution or reproduction is permitted which does not comply with these terms.
*Correspondence: Nophea Sasaki, bm9waGVhc0BhaXQuYXNpYQ==