- Civil Engineering, School of Engineering, College of Engineering and Physical Sciences, University of Birmingham, Birmingham, UK
Anaerobic digestion requires a balanced availability of micro-nutrients with ideal growth conditions to reach optimal organic degradation and biogas production. Iron is the most abundant of the essential metals in an anaerobic digester and its mobility has a strong impact on microorganisms through its own bioavailability, but also through its influence on the bioavailability of other metals. Most previous research on iron mobility in anaerobic digestion has focused on sulfide as the controlling anion because digesters traditionally are sulfide rich and phosphate poor. However, chemical phosphorus removal (CPR) at wastewater treatment works (WWTW) can elevate phosphate concentrations in the digester 10-fold or more. The goal of this research was hence to examine the accepted wisdom of iron-sulfide dominance prevailing in all anaerobic digesters and by evaluating the potential for iron phosphate formation in municipal digesters treating CPR sludge. To fulfill this aim, iron compounds were identified experimentally from full-scale digesters at WWTW with CPR and the most likely iron species identified through modeling according to their thermodynamic probability of formation under the specific environmental conditions experienced in each anaerobic digester. Experimental and modeling data were then combined to identify the main chemical reactions controlling iron mobility in those anaerobic digesters. Results show that speciation of iron in the sampled anaerobic digesters was controlled by the solid phase through a primary reaction (sulfide precipitation to form pyrite and ferrous sulfide) and secondary reaction (phosphate precipitation to form vivianite). However, iron-sulfide precipitates represented only 10–30% of the total iron in the sampled digesters, while iron-phosphate precipitates represented more than 70%. The significance of the high quantity of vivianite in these digesters is that phosphate-rich anaerobic digesters will be more iron-mobile environments than sulfide-rich digesters, with iron being more readily exchanged between the solid and liquid phases during digestion, implying a higher level of bioavailability and the tendency to interact more readily with organic and inorganic counterparts.
Introduction
In the development of self-sustainable wastewater treatment plants, anaerobic digestion (AD) is a key process to reduce waste and produce renewable energy. The balanced availability of macro- and micro-nutrients, coupled with ideal growth conditions, is essential for a healthy anaerobic digester (Gustavsson, 2012). Any disruption of one of those factors can disturb the activity of micro-organisms and lead to the failure of the system. Some metals (such as iron, manganese, copper, cobalt, nickel, and zinc) are part of the essential micro-nutrients required for the well-being of the anaerobic digester and their presence, in a bioavailable form, are indispensable to reach the optimal performance of the anaerobic digester (Schattauer et al., 2011).
The bioavailability of metals is still the subject of considerable investigation both in natural and engineered ecosystems, but research to date generally agrees that most of the dissolved metals and a portion of weakly-bound metals (for example, biosorption) could be considered as available for microorganisms (Worms et al., 2006; Fuentes et al., 2008; Marcato et al., 2009). In the AD, metals undergo a complex series of reactions in the sludge matrix including sorption, complexation, or precipitation processes, influencing their speciation. Most of the metals are bound in the solid phase as precipitates or chelates (Oleszkiewicz and Sharma, 1990) and strongly bound compounds are unavailable for micro-organisms. However, weakly bound compounds can break down and release metals into the liquid phase, establishing a reserve of available metals. Any study of metal bioavailability must then consider the potential of a metals reserve moving from the solid phase to the liquid phase as the more readily bioavailable metals are removed by microbial activity and the equilibrium of the system shifts (Hassler et al., 2004; Jansen et al., 2007).
Iron is the most abundant of the essential metals in an anaerobic digester and hence, by virtue of its high concentration, may influence the speciation of other trace metals present in the digester by competing for the primary chemical reactions. Metcalf & Eddy Inc. (2003) report that the median concentration of iron in wastewater sludge is 17 g/kg Dried Solid (DS), 10 times higher than the median concentration of the second highest heavy metal, zinc, at 1.7 g/kg DS. Moreover, the iron concentration in anaerobic sludge digesters at municipal wastewater treatment works (WWTW) is often increased further by iron dosing for chemical phosphorus removal (CPR) and/or hydrogen sulfide control (Carliell-Marquet et al., 2010; Zhang et al., 2010). The establishment of a phosphorus limit discharge by Urban Wastewater Treatment Directive encouraged the development of CPR in the main WWTW in the UK. Carliell-Marquet et al. (2010) estimated that 300 WWTWs were removing phosphorus chemically in 2010 and this number was projected to increase to over 600 WWTWs by 2015 (Vale, 2012).
Iron, as most other metals in an anaerobic digester, should principally react with sulfide to form insoluble salts; sulfide precipitates being commonly accepted as the main thermodynamically stable compounds formed under anaerobic conditions (Callander and Barford, 1983; Morse and Luther, 1999; Zhang et al., 2010; Shakeri Yekta et al., 2014a). The two main iron sulfide compounds observed are pyrite (FeS2) and amorphous FeS (Kaksonen et al., 2003; van der Veen et al., 2007). Under anaerobic digestion conditions, pyrite is predicted to be the most stable inorganic precipitate when following the Pourbaix diagram (Pourbaix, 1963; Nielsen et al., 2005). However, pyritisation is a slow process and needs a reduction potential below −200 mV, so the formation of meta-stable amorphous FeS is likely to occur as a precursor to the transformation to pyrite (Nielsen et al., 2005). Other iron-sulfide precipitates have been detected in anaerobic digesters such as greigite (Fe3S4), mackinawite (monocrystalline FeS), and pyrrhotite (Fe1-xS) (Jong and Parry, 2004; Dewil et al., 2009; Gustavsson, 2012).
Anaerobic digesters are traditionally sulfide rich and phosphate poor, but phosphorus recovery processes at WWTW can elevate phosphate concentrations in a digester 10-fold or more (Carliell-Marquet et al., 2010). When iron is dosed to co-precipitate phosphorus in activated sludge plants prior to AD, it results in ion-rich feed sludge entering the digester. Once in the digester, reduction of ferric ions to ferrous ions under anaerobic condition disturbs all the Fe(III) binding. The change from trivalent to divalent iron species implies the formation of new thermodynamically stable compounds for iron such as pyrite (FeS2) or vivianite (Fe3(PO4)2.8H2O). Researchers that have studied the fate of iron in anaerobic digesters treating such iron-rich CPR sludge have indeed speculated that a not negligible quantity of iron would continue to be bound with phosphate in the anaerobic digester to form ferrous phosphate precipitates (Miot et al., 2009; Carliell-Marquet et al., 2010), with phosphate effectively competing with sulfide to precipitate iron. The formation of the proposed iron-phosphate precipitates goes against theories of thermodynamic evolution, but can be hypothesized from an availability/kinetic point of view. Zhang et al. (2009) and Miot et al. (2009) suggested that the phosphate creates a bulk phase around the iron (II) which limits sulfide's availability for precipitation of iron and favors the formation of ferrous phosphate.
As the bioavailability of metals is not only dependent on their concentration in the sludge but on their speciation, the potential of phosphate to compete with sulfide for iron precipitation, could have an important impact on the bioavailability of iron in anaerobic digesters. The presence of vivianite or other iron-phosphate precipitates (weaker compounds than sulfide precipitates) in significant quantities, will change the overall speciation of iron in an anaerobic digester and so its behavior, potentially increasing iron mobility/availability for the microbial community.
The primary goal of this research was hence to examine the accepted wisdom of iron-sulfide dominance prevailing in all anaerobic digesters, by evaluating the potential for iron phosphate formation in municipal anaerobic digesters treating CPR sludge. To fulfill this aim, iron compounds were identified from full-scale digesters at WWTW to determine the main iron species according to their thermodynamic probability of formation under the specific environmental conditions experienced in each anaerobic digester. Experimental and modeling data were then combined to identify the main chemical reactions controlling iron mobility in those anaerobic digesters.
Materials and Methods
Sludge Collection
Seven UK WWTW were chosen for this experiment to obtain a wide range of iron concentrations in anaerobically digested sludge. Three types of sites were chosen; non-iron dosed sludge (NID), mixed sludge (MS), and iron dosed sludge (ID). The ratio primary:secondary sludge was around 60:40 across the seven anaerobically digested sludge and some digesters parameter are shown in Table 1. The three mixed sludge were taken from a digester receiving CPR sludge from the WWTW and imported no-iron dosed sludge from other WWTWs. The percentage of imported sludge for MS1-3 varied from 9 to 25%. The CPR was mostly accomplished by the dosage of iron chloride (FeCl3).
Sludge samples were collected directly from anaerobic digesters and kept sealed in a hermetic container during transport, after which the liquid and solid phases were separated by centrifugation (6000 rpm for 10 min). The solid phase was stored in polyethylene bottles at 4°C. The liquid phase was filtered at 0.45 μm and stored in polyethylene bottles at 4°C.
Metals Extraction
The total acid digestion method used an Aqua Regia solution (HCl:HNO3 3:1, 50% dilution, Heated 110–115°C) to dissolve all the metal compounds present in the sludge; as per Standard Methods (3030F, APHA-AWWA-WEF, 1985) and modified by Roussel (2013). Sequential extraction methods used in this research followed the modified BCR procedure described by Chao et al. (2006). The extractions were carried out with analytical grade reagents over a period of 4 days, in triplicate. The order of applied reagents in the BCR sequential extraction is: (1) weak acids, (2) reducing agent, (3) oxidizing agent, and (4) strong acid and so the four fractions produced from the BCR sequential extraction are classified as: (1) exchangeable, (2) reducible, (3) oxidisable, and (4) residual (Figure 1).
Metals Analysis
Flame atomic absorption spectroscopy (FAAS; Perkin Elmer AAS 800) was used to measure the concentration of iron at the absorption wavelength 248.3 nm. The calibration curves were calculated before any sample analysis and standard solutions were freshly made and diluted from a 1000 ppm standard iron solution provided by Fisher©. Calibration curves were only accepted with a correlation coefficient of 0.999 and standard solutions were used as control (10% error) for every 10 samples.
Scanning electron microscopy coupled with energy dispersive X-ray spectroscopy (SEM-EDS) requires dry compounds for analysis and so a fraction of the solid phase was dried at 105°C before being ground. The powdered compound was applied on an analytical disc and fixed with a carbon spray (carbon coated).
The microscope, XL-30 (with LaB6 filament) provided by Philips©, was fitted with a HKL EBSD system with NordlysS camera to obtain electron backscattering pictures to observe elements with high atomic number in the samples. The microscope was also fitted with INCA EDS system provided by Oxford Instrument to do microanalysis on the sample using X-ray spectroscopy. The spectrum of energy analyzed during the scan was 0–9.5 keV.
Chemical Modeling
The chemical environments of the seven anaerobic digesters were simulated using Phreeqc with the database minteq.v4 (USGS). Experimental values (element concentrations and sludge conditions) used as input values are detailed in Table 1. Each element was entered in the liquid phase in its atomic form; its speciation then being predicted based on the environmental input values and the exchanges/reactions allowed in the simulation, as detailed below.
The parameters described in the Phreeqc, which represented the anaerobic digester conditions, were limited to temperature, electric potential (pe) and pH. The input pH was 8–8.2 to allow a potential charge balance during the simulation and produced an output pH value agreeing with the measured pH of each sludge (7.1–7.4). The input potential electric (pe) was −6.7 to ensure anaerobic condition and avoid any oxidation during the simulation.
Phreeqc was limited to simulating inorganic reactions, with no organic complexation or biomass adsorption. The only exchange between phases allowed in this simulation was solid-liquid and no consideration of the gas composition or potential H2S exchange was considered. The exchange between solid-liquid phases was controlled by the saturation index of each precipitate (Roussel, 2013).
Results
BCR Sequential Extraction
Iron concentrations in the solid phase of the seven anaerobic digesters sampled were in the range 9.7–28.8 g/kg DS using the sum of the sequential extraction fractions and from 12.6 to 33 g/kg DS using total acid digestion (Table 2). An increase in total iron concentration of the digested sludge was observed when CPR processes influenced the digester sludge composition either through imported iron-dosed sludge being fed to the digester (MS), or when CPR was directly integrated into the WWTW (ID).
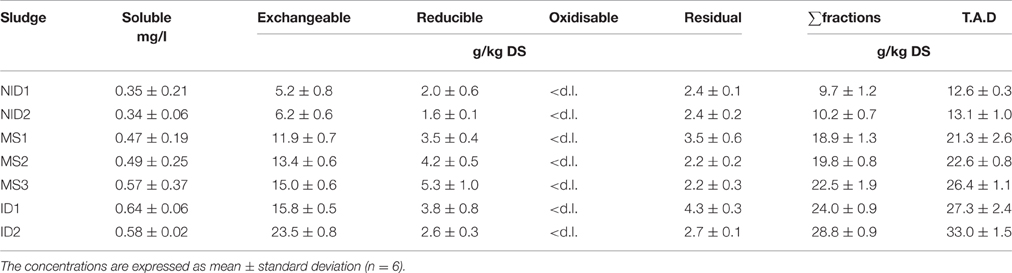
Table 2. Concentrations of iron in each BCR sequential extraction fraction and total acid digestion (T.A.D.) for the 7 anaerobically digested sludge.
It should also be noted that in all cases a loss of material was observed during the BCR sequential extraction; the recovery of iron (measured as the sum of the extracted fractions) ranged from 77 to 89% of the total iron concentration measured by the acid digestion procedure. This has also been reported by other researchers using the BCR sequential extraction procedure; 87% recovery was reported by Fuentes et al. (2008) in their research, which is comparable to the 85% average recovery found in this research. Finally, the concentration of iron in the liquid phase increased with the concentration of total iron in the solid phase from 0.3 mg/l for NID sludge to 0.6 mg/l for ID sludge (Table 2). The amount of iron present in the liquid phase represents around 0.1% of the total iron and the results were lower than the range of 0.5–4% suggested by Oleszkiewicz and Sharma (1990). The Pearson product-moment correlation coefficient was calculated between the concentration of iron in the liquid phase and the total iron concentration to statically confirm any linear correlation. The Pearson coefficient was 0.928 (p = 0.003) confirming a positive linear correlation between the variables, meaning that the concentration of soluble iron increases with the total concentration.
The BCR exchangeable fraction (first extracted fraction) represented the main iron fraction in all digested sludge samples, with more than 50% of the total iron extracted in this fraction and a maximum of 76% obtained for the sludge ID2 (Figure 2 and Table 2). This is in contrast to (last) fraction of sequential extraction schemes (Alvarez et al., 2002; van der Veen et al., 2007; Fuentes et al., 2008). However, Carliell-Marquet et al. (2010) and Dodd et al. (2000) found comparable results to those obtained during this research, with a high percentage of iron reported to be recovered in the first steps of the sequential extraction procedures (exchangeable and reducible fractions). The variation in iron extractability being reported by different researchers sampling different anaerobic digesters supports the argument that iron mobility can change markedly depending on the particular environmental conditions of each anaerobic digester. Notably, the concentration of iron extracted in the early fractions is heavily influenced by the presence of iron-phosphate rich CPR sludge in a digester. NID 1&2 sludge had 5.2 and 6.2 g/kg DS of iron extracted in the exchangeable fraction whereas 11.9–15.0 g/kg DS of iron was extracted in the same fraction in MS sludge and kept increasing to 23.5 g/kg DS for ID2. This linear correlation was statically confirmed by a Pearson coefficient between the iron extracted in the exchangeable fraction and the total iron (as sum of BCR fractions) of 0.984 (p = 0.000).
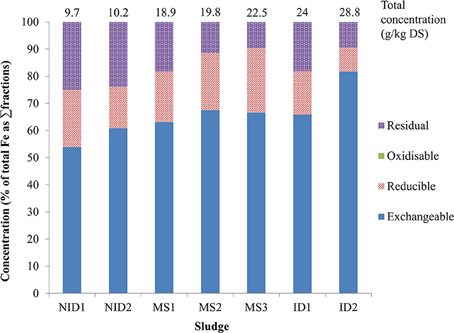
Figure 2. Iron fractionation profiles in the 7 anaerobically digested sludge (NID, non-iron dosed sludge; MS, mixed sludge; ID, iron dosed sludge).
The BCR sequential extraction procedure cannot be used to determine the exact metal species extracted in each fraction; so the presence of iron in the exchangeable fraction could result from dissolution of iron-carbonate or iron-phosphate precipitates or even the exchange of weakly bound iron from organic sites on the sludge matrix. In this research, vivianite was identified as one of the main compounds dissolved in the BCR exchangeable fraction, through the use of: SEM-EDS analysis, chemical equilibrium speciation modeling of the different AD environments, previous published research, and thermodynamic constants (Ofverstrom et al., 2011; Cheng et al., 2015).
Iron was also extracted in two other BCR sequential extraction fractions: the reducible and residual fractions, which represented each between 9 and 25% of the total iron. The concentration of iron in the BCR reducible fraction was between 2.0 g/kg DS (NID1) and 5.3 g/kg DS (MS3). No specific pattern was observed in the variation of iron extracted in the reducible fraction when compared with either the total iron concentration or the anaerobic digester environmental conditions (Pearson coefficient of 0.539; p = 0.211). Cheng et al. (2015) suggested that trivalent iron entering a digester in the feed sludge would not necessarily be reduced during the anaerobic digestion period in spite of the low oxidation-reduction potential of the anaerobic environment, if Fe(III) entered the digester as a well-ordered crystalline structure. This might occur, for example, when iron is dosed into an activated sludge process with a long sludge age, giving ample time for maturation of the crystalline ferric structure. Hence, the presence of ferric precipitates in the digested sludge should not be discounted as a possible contributing factor to the iron extracted during the reducible BCR fraction. Another hypothesis is that most Fe (III) entering an anaerobic digester is indeed reduced to Fe (II), leading to the rapid formation of ferrous phosphate or ferrous-hydroxyl-phosphate compounds (possibly amorphous; as suggested by Smith and Carliell-Marquet, 2009) that would then be readily dissolved by the low pH 2 of the reagent used in the second BCR extraction.
The iron concentration in the BCR residual fraction varied between 2.4 g/kg DS (NID1 and NID2) and 4.3 g/kg DS (ID1). As for the reducible fraction, no pattern was established between the concentration of iron extracted in the residual fraction and the total iron concentration of the digested sludge (Pearson coefficient of 0.298; p = 0.516). The iron extracted in the residual fraction was identified to be bound with sulfide and precipitated as pyrite or ferrous sulfide (SEM-EDS analysis). Sulfide precipitates are expected to be extracted in the oxidisable BCR fraction (Chao et al., 2006) but van der Veen et al. (2007) demonstrated that those precipitates were not systematically extracted in the oxidisable fraction and could be carried over to the residual fraction. In this research, measurement of the sulfur concentration in the different BCR sequential extraction fractions showed the presence of a high sulfur concentration in the residual fraction; suggesting that iron sulfide precipitates in the digested sludge were indeed being dissolved in this fraction, rather than the oxidisable fraction (Roussel, 2013); with concentrations of iron in the oxidisable BCR fractions below the detection limit and recorded as nil (Table 2).
SEM-EDS Analysis
SEM-EDS analysis was performed on the solid phase of each sludge to obtain information on the iron species present in it. Ferrous phosphate and ferrous sulfide were the two main types of precipitates observed during the analysis and are described below (Figure 3 and their corresponding elemental compositions are given in Table 3). Several ferrous phosphate compounds were detected (Roussel, 2013) but the main compound was vivianite and it was observed in every AD sludge studied (compounds A and B, Figure 3). Vivianite was identified by stoichiometric analysis and corroborated by crystallographic analysis (Roussel, 2013). Three main elements (Fe, O, and P) were detected in compound A and B (Table 3) with a respective atomic percent of 13, 72, and 12 while Mg and Ca were also detected at low concentrations (1%). In order to simplify the stoichiometric analysis, Mg and Ca were included with the iron percentage as interchangeability between those cations and iron has been suggested by Nriagu (1972) and De Vrieze et al. (2013) for phosphate precipitation. Then three modified ratios Fe /P: 1.5, O/Fe: 5, and O/P: 7 gave the formula Fe3P2O14.5 written as Fe3(PO4)2.6.5H2O. Loss of water in the molecule in comparison with the vivianite formula (Fe3(PO4)2.8H2O) was likely to have occurred during the drying phase of the sample preparation for SEM-EDS. Frost et al. (2003) showed that natural vivianite is dehydrated in the temperature range 105–420°C and the dehydration occurs in five steps. The first step of dehydration incurs a loss of one water molecule and occurs at 105°C; this is the temperature that was used for drying the digested sludge samples prior to SEM-EDS and hence is likely to have affected the water content of vivianite detected by SEM-EDS.
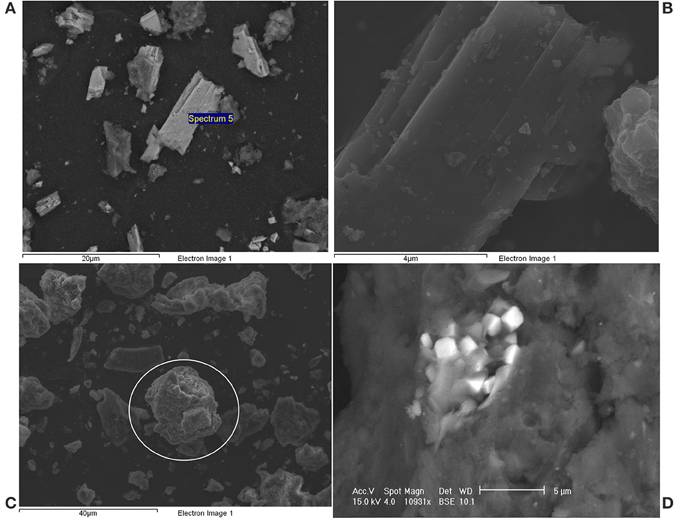
Figure 3. Pictures of iron precipitates detected in anaerobically digested sludge by SEM-EDS. (A,B) Vivianite, (C) Ferrous sulphide, (D) Pyrite.
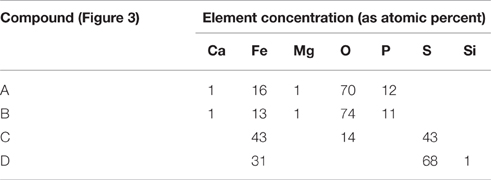
Table 3. Elemental analysis of the iron precipitates observed in the Figure 3.
The compounds C and D were identified as ferrous sulfide and pyrite, respectively. The identification was also done using stoichiometric analysis and crystallographic investigation. Both compounds contained mainly iron and sulfur but in different ratios (Table 3). The ratio Fe:S for the compound C was 1 and did not exhibit any specific crystalline structure (in comparison to compound D). It was identified as ferrous sulfide a meta-stable amorphous compound (Nielsen et al., 2005). The presence of oxygen in compound C was likely to be to surface oxidation during the SEM sample preparation as the percentage was too low to demonstrate the presence of sulfate precipitate. Finally, the ratio Fe:S for the compound D was 0.5 and the chemical formula was calculated as FeS2, pyrite. One of the pyrite crystal habits is cubic and this was clearly observed in the digested sludge, as shown in the Figure 3D (Haldar, 2014).
Chemical Modeling
The results from the predicted iron speciation are shown in the Table 4 for the seven types of anaerobically digested sludge under study. The input values for the simulation have been obtained from the total concentration (liquid and solid phases) measured in each sludge (Table 1). Phreeqc predicted that iron would be precipitated as pyrite and vivianite. The concentration of pyrite varied between 4.8 and 9.8 g/kg DS (Table 4). The amount of pyrite precipitated in the sludge was not dependent on the total iron concentration but was related to the total sulfide concentration. MS2 and MS3 were the two sludge with the lowest sulfide concentration and both had the lowest pyrite concentrations with 5.0 and 4.8 g/kg DS. By contrast, NID1 and ID2 were the two sulfide-rich sludge types and had the highest pyrite concentration with, respectively 7.2 and 9.8 g/kg DS. Vivianite precipitation was primarily related to the total iron concentration in the digested sludge, increasing from 2.7 g/kg DS for NID1 to 19.3 g/kg DS for ID2.
A relationship was observed between the predicted concentration of iron as vivianite or pyrite and experimental concentrations of iron in two BCR sequential extraction fractions (Figure 4). The iron concentrations in the exchangeable fraction and the concentrations of iron as vivianite presented comparable concentrations with the different sludges and both were dependent on the total iron concentration. The concentration of pyrite predicted by the model was also comparable with the concentration of iron extracted in the residual fraction and both were dependent on the total sulfur concentration.
The modeling is limited to calculations based on thermodynamic equilibrium, which cannot entirely capture the complexity of a living reactor. Specifically, equilibrium speciation modeling does not take into account the effect of kinetics, potential local reactions and co-precipitations, or the impact of micro-organisms on metal speciation. As an example, greigite (Fe3S4) and ferrous sulfide were only predicted by Phreeqc to precipitate for iron and sulfur if the model was set so that pyrite was not allowed to precipitate. Those two compounds have been found to be pyrite's precursor (Nielsen et al., 2005; Gustavsson, 2012) and SEM-EDS in this research showed that ferrous sulfide and pyrite were indeed both present in the digested sludge sampled, indicating that the sludge retention time was not long enough to obtain a complete transformation from ferrous sulfide to pyrite. Unless the model parameters are specifically altered by the user, it should be noted that pyrite will be the main predicted output. The kinetics can also modify the balance between predicted vivianite and pyrite. It is hypothesized that, following the dissolution of ferric phosphate (originally present in the feed sludge) in the low redox environment of an anaerobic digester, a high concentration of phosphate subsequently surrounds the iron, creating a bulk limitation of sulfide availability for iron (Zhang et al., 2009). This potential disturbance of the thermodynamic equilibrium was not taken into account by the equilibrium speciation model; hence it is likely that phosphorus-rich digesters will contain more vivianite than predicted by equilibrium speciation modeling, which will reduce pyrite formation. Finally, the presence of iron extracted in the second fraction (reducible) of the BCR sequential extraction was not clearly characterized and could be from non-reduced Fe(III) or undissolved ferrous phosphate from the first extraction or even co-precipitates. The amount of iron extracted in the reducible fraction was bound as pyrite or vivianite by the model.
A series of theoretical simulations were done to represent a range of iron, phosphate, and sulfide concentrations in each digester. The iron concentration was simulated from 0 to 30 g/kg DS and the average (across the seven types of sludge) concentration of iron predicted as vivianite and as pyrite are shown in Figure 5. In the model simulation the first compound to be precipitated was pyrite, iron being converted into pyrite until sulfide became the limiting factor, after which the concentration of pyrite stayed constant and the iron precipitated as vivianite. Varying the sulfide concentration (0–40 g/kg DS, Figure 6) showed that, in the absence of sulfide, all the iron was precipitated as vivianite. Sulfide precipitates preferentially with copper, nickel, zinc and cobalt prior to the formation of pyrite, hence it is only when the sulfide concentration in a digester increases to 1 g/kg DS that pyrite formation is initiated in competition with vivianite formation. Varying the simulated phosphate content of the digested sludge (0–40 g/kg DS, results not shown) showed that, in absence of phosphate, iron was precipitated as pyrite and siderite (FeCO3). The concentration of pyrite stayed constant as phosphate content increased, however the siderite concentration decreased as phosphate competed with carbonate for iron, with iron precipitating increasingly as vivianite, until no siderite remained. This series of simulation demonstrates that, based on thermodynamic calculations alone iron speciation in a digester would be primarily controlled by sulfide, then phosphate and finally carbonate.
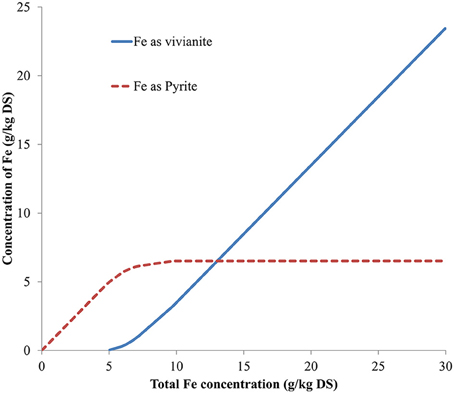
Figure 5. Predicted iron fractionation in function of total iron concentration (average of the predicted results obtained from all anaerobically digested sludge under study).
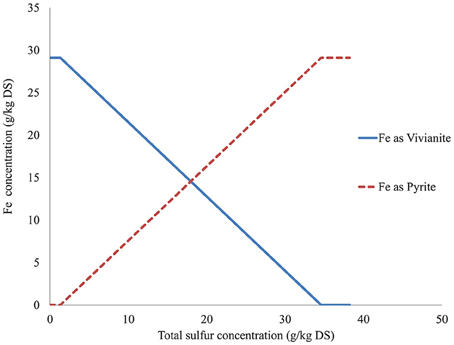
Figure 6. Predicted iron fractionation in function of total sulfur concentration (average of the predicted results obtained from all anaerobically digested sludge under study).
Discussion
Iron Speciation in Anaerobically Digested Sludge
Previous research has shown that sulfur plays an important role in the speciation of iron in many anaerobic digesters, but phosphate interaction with iron has traditionally been considered negligible, being dominated by full sulfide precipitation or by other binding such as carbonate, thiol, or organic material (Shakeri Yekta et al., 2014a). This research demonstrated that iron precipitates with both sulfide and phosphate in sewage sludge anaerobic digesters, which are becoming more phosphate-rich environments due to the increasing legislative drive to remove phosphorus from wastewater. In these digesters, iron bonded with phosphate to form vivianite; agreeing with previous results demonstrating its thermodynamic stability under anaerobic condition (Miot et al., 2009). Indeed, the solubility product of vivianite (pKsp = 35.8, Al-Borno and Tomson, 1994) agreed on the potential formation in anaerobically digested sludge from a thermodynamic approach. Rothe et al. (2016) found that vivianite is stable at pH conditions from 6 to 9 and its formation is detected in organic rich environment in presence of ferrous and orthophosphate ion. The pH range of vivianite stability agreed with its dissolution in the first BCR fraction (exchangeable, pH 4).
The precipitation of iron with phosphate has been previously qualitatively observed in anaerobically digested sludge (Miot et al., 2009; Carliell-Marquet et al., 2010). However, this study demonstrated that vivianite precipitation accounted for at least 50% of the total iron in non-sulfide rich AD and, when CPR was included in upstream wastewater treatment, vivianite could represent more than 90% of the total iron in an anaerobic digester. These results challenge the outputs from thermodynamic calculations (Callander and Barford, 1983) or previous experimental results (Morse and Luther, 1999; Zhang et al., 2010) who concluded that the majority of iron in anaerobic digesters would be present as sulfide precipitates. Previously, anaerobic digesters were often considered to be operating under sulfidic conditions with a threshold of 1 for S:Fe, however, a threshold of 2 is actually required for pyrite formation.
Concerning sulfide reacting with iron, SEM-EDS analysis showed the presence of two iron-sulfide compounds: amorphous ferrous sulfide and pyrite. Nielsen et al. (2005) demonstrated that pyrite is expected to be the most stable inorganic iron-sulfide precipitate. However, the relatively short time period for crystal formation and maturation in an anaerobic digester, coupled with redox potential variation (caused by the periodic addition of feed sludge) is likely to encourage ferrous sulfide formation over pyrite formation, as noted by Miot et al. (2009).
Concentration of different ferrous sulfide compounds varied from 10 to 25% of the total iron concentration in the seven studied digested sludge; no clear relationship could be seen between an increase of iron concentration in the digesters (resulting from CPR dosing) and the concentration of iron bound with sulfide (Table 2 and Figure 2). A relationship between total iron-sulfide precipitates and total sulfur concentration was demonstrated in this research through the BCR sequential extraction analysis, and had been suggested previously by Mosey and Hughes (1975). However, additional factors must also have been involved in controlling the precipitation of iron with sulfide in the digesters, as three sludges had the same amount of sulfur measured (NID2, MS1, and ID1) but demonstrated a variation in the concentration of iron extracted in the residual fraction, from 2.4 to 4.3 g/kg DS (Tables 1, 2). Moreover, the ID2 sludge represented the highest sulfur content but only 2.7 g/kg DS of iron was extracted as sulfide precipitates. Shakeri Yekta et al. (2014b) demonstrated that sulfur speciation in an anaerobic digester is an important factor determining the capacity of sulfur for iron precipitation, as each sulfur species possess a different binding capacity for iron. Sulfur species compete with each other for iron, and also with other anions such as phosphate. Moreover, kinetic effects can also enhance the preferential binding of iron with molecules other than sulfur in an anaerobic digester, due to a local unavailability of sulfide anions (Zhang et al., 2009).
Reactions Controlling the Iron Behavior in Anaerobically Digested Sludge
The results of this study have been used to obtain a better understanding of iron speciation in municipal anaerobic digesters and also, importantly, to determine the most probable order of the sulfide and phosphate precipitation reactions (Figure 7). Results have shown that the first reaction controlling the behavior of iron in the solid phase is precipitation with sulfide to form ferrous sulfide first and then pyrite, regardless of the higher iron or phosphate concentrations in the digesters we studied. Iron sulfide precipitation is, however, strictly constrained by the iron/sulfur ratio in the anaerobic digester and is likely to be limited (Shakeri Yekta et al., 2014a). Following precipitation with sulfide, iron reacts with phosphate to precipitate as vivianite and various ferrous-(hydroxyl)-phosphate compounds. Vivianite represented the largest fraction of iron in all the digesters studied, without being the primary reaction.
These results hence agree with traditional thermodynamic calculations in predicting sulfide as the primary factor controlling iron speciation (Pourbaix, 1963; Callander and Barford, 1983) even if 90% of the total iron was found to be present as vivianite in the digesters studied. However, thermodynamic calculations do seem to overestimate the precipitation of iron with sulfide when compared to experimental data. Shakeri Yekta et al. (2014a) controlled this overestimation in their model by including ligands in the liquid phase and thiols in the solid phase for sulfidic sludge; when sulfide became limiting in their simulated anaerobic digesters, carbonate was used to precipitate iron as siderite. This study has shown that phosphate can also compete kinetically with sulfide to react with iron, especially through the formation of stable iron-phosphate compounds such as vivianite. CPR at a WWTW enhances the ability of phosphate to compete for iron in an anaerobic digester by introducing iron as ferric phosphate in the digester feed sludge, effectively limiting the access of sulfide to iron (Miot et al., 2009; Zhang et al., 2009).
More research is required to complete the overall picture of iron mobility in phosphorus enriched anaerobic digesters, by understanding the speciation of iron in the liquid phase, particularly in terms of bioavailability. Quantitative determination of different iron species in the liquid phase is complicated by low detectable concentrations of individual species (Fermoso et al., 2009). Shakeri Yekta et al. (2014a) approached this subject by creating a model simulation from two sub-models developed by Rickard (2006) and Davison et al. (1999). Their results showed that the iron concentration in the liquid phase of an anaerobic digester, and its speciation, depended primarily on the ratio of sulfur to iron in their digester. Iron solubility was observed to increase when secondary iron precipitates were recorded alongside sulfide precipitation.
In this study, increased total iron concentration in the digested sludge also increased the concentration of iron measured in the liquid and could be linked with the solubility product constant of vivianite formation. Hence, the speciation of iron in the solid phase impacts directly on the reactions controlling the behavior of iron in the liquid phase and, ultimately, on the concentration of iron in the liquid phase.
Impact of Vivianite Precipitation on the Anaerobic Digestion Process
The presence of more than 50% and up to 80% of iron precipitated as vivianite in an anaerobic digester, instead of precipitated with sulfide as traditionally expected, means that iron in these digesters is likely to be more mobile and hence more bioavailable; this is likely to have a secondary effect on other metals speciation.
The optimal balance for metals bioavailability is a fragile equilibrium and any change in their speciation might tip a digester into the deficiency or toxicity zone (Zandvoort et al., 2006). The presence of CPR at a WWTW increases the total concentration of iron and phosphorus in the feed sludge, leading to an increase of vivianite precipitation and solubility of iron in the anaerobic digester. Previous results showed that CPR had negative impact on biogas production (Smith and Carliell-Marquet, 2009; Ofverstrom et al., 2011) that could be correlated to a concentration of iron in the liquid phase coupled with a higher mobility of iron in the solid phase. By contrast, De Vrieze et al. (2013) found that the addition of iron-rich activated sludge stabilized kitchen waste anaerobic digesters, primarily because iron was a rate-limiting element and secondarily by reducing the high concentration of soluble phosphate in these digesters.
Previous research on metal solubility and bioavailability in digesters used the sulfide element to estimate metal solubility in sewage sludge digesters, assuming sulfide as the dominant anion (Mosey et al., 1971; Mosey and Hughes, 1975). Specifically, Mosey et al. (1971) used sulfide solubility (threshold at pS = 17.2) as a measure of the potential bioavailability and hence, toxicity, of heavy metals in anaerobic digestion. This hypothesis could still be used for sulfidic digesters, but sewage sludge today generally has a higher amount of iron than sulfur, due primarily to the prevalence of CPR. Under these new phosphate-rich conditions, phosphate availability must also be taken into consideration in order to calculate iron solubility. Vivianite is defined by the BCR sequential extraction analysis as a weakly bound compound; this weak binding capacity of vivianite enhances the transfer of iron between solid and liquid phase creating a reserved pool of iron able to quickly shift depending on the AD condition. This means that the overall mobility of iron in sewage sludge anaerobic digesters has changed over time as upstream wastewater treatment processes have changed to include phosphorus removal, in response to increasingly strict discharge requirements.
The large amount of iron bound with phosphate in sewage sludge digesters today, also reduces phosphate availability for other cations such as calcium or magnesium. Struvite is a high value end product from anaerobic digestion that can be precipitated through magnesium addition, post anaerobic digestion; struvite precipitation relying on phosphate having been released into the liquid phase during digestion. Phosphate entering an anaerobic digester from CPR is bound with iron and even though it is likely to be released temporarily as a result of Fe(III) reduction, this research showed that most phosphate is re-precipitated as vivianite and will remain in this form, preventing post-digestion struvite recovery.
Finally, the speciation of iron also has an impact on the other metals behavior in the anaerobic digester. Cobalt and nickel are both metals that kinetically precipitate with sulfide after iron and can potentially be absorbed onto ferrous sulfide compounds (Morse and Luther, 1999). Shakeri Yekta et al. (2014b) demonstrated that nickel solubility was controlled by three reactions, including interaction with ferrous sulfide. Hence, reduction of ferrous sulfide precipitates as a result of vivianite formation might reduce the effect of co-precipitation for nickel and cobalt, enhancing secondary reactions and consequently impacting on their solubility. Finally, increased iron mobility could also influence the kinetics of essential trace metals being supplemented to anaerobic digesters to boost biogas production. Roussel (2013) observed a quicker dissolution of supplemented cobalt bound to EDTA in iron-rich sludge than in iron-poor sludge. It was demonstrated that the acceleration of cobalt dissolution was due to higher availability of iron to react with EDTA and release the cobalt, which in turn made cobalt more bioavailable and increased the rate of biogas production from those digesters.
Conclusion
• Municipal sewage sludge digesters are today more phosphorus-rich environments than in the past, due to the increasing legislative requirements for WWTW to remove phosphorus from wastewater, which they achieve with iron dosing, resulting in an iron and phosphorus rich sludge being fed to the anaerobic digesters.
• Increasing iron concentrations in municipal sewage sludge digesters was linked to increasing concentrations of vivianite precipitated in the digested sludge.
• The first reaction controlling the behavior of iron in the solid phase is precipitation with sulfide to form ferrous sulfide initially and then pyrite, regardless of the higher iron or phosphate concentrations in the digesters we studied.
• Following precipitation with sulfide, iron reacts with phosphate to precipitate as vivianite and various ferrous-(hydroxyl)-phosphate compounds. Vivianite represented the largest fraction of iron in all the digesters studied, without being the primary reaction.
• Vivianite is defined by the BCR sequential extraction analysis as a weakly bound compound; this weak binding capacity of vivianite enhances the transfer of iron between solid and liquid phase creating a reserved pool of iron able to quickly shift depending on the AD condition.
Author Contributions
JR performed the doctoral research from which this paper was written. CC was the primary supervisor for that research. Both JR and CC have been involved in preparing, writing, and editing this manuscript.
Funding
The research in this paper was supported by the Engineering and Physical Sciences Research Council (UK) through a Doctoral Industrial CASE Studentship award (Voucher number: 07001986) “Increasing biogas quantity and quality from anaerobic digestion of wastewater sludge, using nutrient supplementation”; with industrial funding provided by Severn Trent Water Ltd.
Conflict of Interest Statement
The authors declare that the research was conducted in the absence of any commercial or financial relationships that could be construed as a potential conflict of interest.
References
Al-Borno, A., and Tomson, M. B. (1994). The temperature dependence of the solubility product constant of vivianite. Geochim. Cosmochim. Acta 58, 5373–5378.
Alvarez, E. A., Mochón, M. C., Jiménez Sánchez, J. C., and Ternero Rodríguez, M. (2002). Heavy metal extractable forms in sludge from wastewater treatment plants. Chemosphere 47, 765–775. doi: 10.1016/S0045-6535(02)00021-8
APHA-AWWA-WEF (1985). Standard Methods for the Examination of Water and Wastewater, 16th Edn. Washington, DC: APHA.
Callander, I. J., and Barford, J. P. (1983). Precipitation, chelation, and the availability of metals as nutrients in anaerobic-digestion 1. Methodology 25, 1947–1957.
Carliell-Marquet, C. M., Smith, J., Oikonomidis, I., and Wheatley, A. (2010). Inorganic profiles of chemical phosphorus removal sludge. Water Manag. 163, 65–77. doi: 10.1680/wama.2010.163.2.65
Chao, W., Li, X. C., Wang, P. F., Zou, L. M., and Ma, H. T. (2006). Extractable fractions of metals in sewage sludges from five typical urban wastewater treatment plants of China. Pedosphere 16, 756–761. doi: 10.1016/S1002-0160(06)60111-2
Cheng, X., Chen, B., Cui, Y., Sun, D., and Wang, X. (2015). Iron(III) reduction-induced phosphate precipitation during anaerobic digestion of waste activated sludge. Sep. Purif. Tech. 140, 6–11. doi: 10.1016/j.seppur.2015.01.002
Davison, W., Phillips, N., and Tabner, B. J. (1999). Soluble iron sulfide species in natural waters: reappraisal of their stoichiometry and stability constants. Aquat. Sci. 61, 23–43.
De Vrieze, J., De Lathouwer, L., Verstraete, W., and Boon, N. (2013). High-rate iron-rich activated sludge as stabilizing agent for the anaerobic digestion of kitchen waste. Water Res. 47, 3732–3741. doi: 10.1016/j.watres.2013.04.020
Dewil, R., Baeyens, J., Roels, J., and Steene, D. (2009). Evolution of the total sulphur content in full-scale wastewater sludge treatment. Environ. Eng. Sci. 26, 867–872. doi: 10.1089/ees.2007.0335
Dodd, J., Large, D. J., Fortey, N. J., Milodowski, A. E., and Kemp, S. (2000). A petrographic investigation of two sequential extraction techniques applied to anaerobic canal bed mud. Environ. Geochem. Health 22, 281–296. doi: 10.1023/A:1006743630918
Fermoso, F. G., Bartacek, J., Jansen, S., and Lens, P. N. (2009). Metal supplementation to UASB bioreactors: from cell-metal interactions to full-scale application. Sci. Tot. Environ. 407, 3652–3667. doi: 10.1016/j.scitotenv.2008.10.043
Frost, R. L., Weier, M. L., Martens, W., Kloprogge, J. T., and Ding, Z. (2003). Dehydration of synthetic and natural vivianite. Thermochim. Acta 401, 121–130. doi: 10.1016/S0040-6031(02)00505-1
Fuentes, A., Lloréns, M., Sáez, J., Isabel Aguilar, M. A., Ortuño, J. F., and Meseguer, V. F. (2008). Comparative study of six different sludges by sequential speciation of heavy metals. Bioresour. Technol. 99, 517–525. doi: 10.1016/j.biortech.2007.01.025
Gustavsson, J. (2012). Cobalt and Nickel Bioavailability for Biogas Formation. Ph.D. thesis, Linköping University, Linköping Studies in Arts and Science, 549.
Haldar, S. K. (2014). “Basic mineralogy,” in Introduction to Mineralogy and Petrology (Oxford, UK: Elsevier), 39–79.
Hassler, C. S., Slaveykova, V. I., and Wilkinson, K. J. (2004). Some fundamental (and often overlooked) considerations underlying the free ion activity and biotic ligand models. Environ. Toxicol. Chem. 23, 283–291. doi: 10.1897/03-149
Jansen, S., Gonzalez-Gil, G., and van Leeuwen, H. P. (2007). The impact of Co and Ni speciation on methanogenesis in sulfidic media - Biouptake versus metal dissolution. Enzyme Microb. Technol. 40, 823–830. doi: 10.1016/j.enzmictec.2006.06.019
Jong, T., and Parry, D. L. (2004). Adsorption of Pb(II), Cu(II), Cd(II), Zn(II), Ni(II), Fe(II), and As(V) on bacterially produced metal sulfides. J. Colloid Interface Sci. 275, 61–71. doi: 10.1016/j.jcis.2004.01.046
Kaksonen, A. H., Riekkola-Vanhanen, M. L., and Puhakka, J. A. (2003). Optimization of metal sulphide precipitation in fluidized-bed treatment of acidic wastewater. Water Res. 37, 255–266. doi: 10.1016/S0043-1354(02)00267-1
Marcato, C.-E., Pinelli, E., Cecchi, M., Silvestre, J., Winterton, P., and Guiresse, M. (2009). Ecotoxicology and environmental safety bioavailability of Cu and Zn in raw and anaerobically digested pig slurry. Ecotoxicol. Environ. Saf. 72, 1538–1544. doi: 10.1016/j.ecoenv.2008.12.010
Metcalf & Eddy Inc. (2003). Wastewater Engineering: Treatment and Reuse, 4th Edn. Boston, MA: McGraw-Hill.
Miot, J., Benzerara, K., Morin, G., Bernard, S., Beyssac, O., Larquet, E., et al. (2009). Transformation of vivianite by anaerobic nitrate-reducing iron-oxidizing bacteria. Geobiology 7, 373–384. doi: 10.1111/j.1472-4669.2009.00203.x
Morse, J. W., and Luther, G. W. (1999). Chemical influences on trace metal-sulfide interactions in anoxic sediments. Geochim. Cosmochim. Acta 63, 3373–3378.
Mosey, F. E., and Hughes, D. A. (1975). The toxicity of heavy metal ions to anaerobic digestion. Water Pollut. Control 74, 18–39.
Mosey, F. E., Swanwick, J. D., and Hughes, D. A. (1971). Factors affecting the availability of heavy metals to inhibit anaerobic digestion. Water Pollut. Control 70, 668–679.
Nielsen, A. H., Lens, P. N. L., Vollersten, J., and Hvitved-Jacobsen, T. (2005). Sulfide-iron interactions in domestic wastewater from a gravity sewer. Water Res. 39, 2747–2755. doi: 10.1016/j.watres.2005.04.048
Nriagu, J. O. (1972). Stability of vivianite and ion-pair formation in the system Fe3(PO4)2-H3PO4-H2O. Geochim. Cosmochim. Acta 36, 459–470
Ofverstrom, S., Regimantas, D., and Sapkaite, I. (2011). The effect of iron salt on anaerobic digestion and phosphate release to sludge liquor. Science 3, 123–126. doi: 10.3846/mla.2011.97
Oleszkiewicz, J. A., and Sharma, V. K. (1990). Stimulation and inhibition of anaerobic processes by heavy-metals - a review. Biol. Wastes 31, 45–67.
Rickard, D. (2006). The solubility of FeS. Geochim. Cosmochim. Acta 70, 5779–5789. doi: 10.1016/j.gca.2006.02.029
Rothe, M., Kleeberg, A., and Hupfer, M. (2016). The occurrence, identification and environmental relevance of vivianite in waterlogged soils and aquatic sediments. Earth-Sci. Rev. 158, 51–64. doi: 10.1016/j.earscirev.2016.04.008
Roussel, J. (2013). Metal Behaviour in Anaerobic Sludge Digesters Supplemented with Trace Nutrients. Ph.D. thesis, University of Birmingham.
Schattauer, A., Abdoun, E., Weiland, P., Plöchl, M., and Heiermann, M. (2011). Abundance of trace elements in demonstration biogas plant. Biosyst. Eng. 108, 57–65. doi: 10.1016/j.biosystemseng.2010.10.010
Shakeri Yekta, S., Lindmark, A., and Skyllberg, U. (2014b). Importance of reduced sulfur for the equilibrium chemistry andkinetics of Fe(II), Co(II) and Ni(II) supplemented to semi-continuousstirred tank biogas reactors fed with stillage. J. Hazard. Mater. 269, 83–88. doi: 10.1016/j.jhazmat.2014.01.051
Shakeri Yekta, S., Svensson, B., Bjorn, A., and Skyllberg, U. (2014a). Thermodynamic modeling of iron and trace metal solubility and speciation under sulfidic and ferruginous conditions in full scale continuous stirred tank biogas reactors. Appl. Geochem. 47, 61–73. doi: 10.1016/j.apgeochem.2014.05.001
Smith, J. A., and Carliell-Marquet, C. M. (2009). A novel laboratory method to determine the biogas potential of iron-dosed activated sludge. Bioresour. Technol. 100, 1767–1774. doi: 10.1016/j.biortech.2008.10.004
Vale, P. (2012). “Preparing for the 2nd cycle of the WFD - the twin challenge of phosphorus and iron,” in Wastewater Forum. Available online at: http://www.fwr.org/wastewat/2012%200702%20Preparing%20for%20the%202nd%20cycle%20of%20the%20WFD_P&Fe%20-%20Vale-SevernTrent-FWR.pdf (Accessed 6 June, 2016).
van der Veen, A., Fermoso, F. G., and Lens, P. N. L. (2007). Bonding form analysis of metals and sulfur fractionation in methanol-grown anaerobic granular sludge. Eng. Life Sci. 7, 480–489. doi: 10.1002/elsc.200720208
Worms, I., Simon, D. F., Hassler, C. S., and Wilkinson, K. J. (2006). Bioavailability of trace metals to aquatic microorganisms: importance of chemical, biological and physical processes on biouptake. Biochimie 88, 1721–1731. doi: 10.1016/j.biochi.2006.09.008
Zandvoort, M. H., van Hullebusch, E. D., Fermoso, F. G., and Lens, P. N. L. (2006). Trace metals in anaerobic granular sludge reactors: bioavailability and dosing strategies. Eng. Life Sci. 6, 293–301. doi: 10.1002/elsc.200620129
Zhang, L., Keller, J., and Yuang, Z. (2009). Inhibition of sulfate-reducing and methanogenic activities of anaerobic sewer biofilms by ferric iron dosing. Water Res. 43, 4123–4132. doi: 10.1016/j.watres.2009.06.013
Keywords: iron bioavailability, vivianite, anaerobic digestion, chemical phosphorus removal, sulfide, phosphate, equilibrium speciation modeling
Citation: Roussel J and Carliell-Marquet C (2016) Significance of Vivianite Precipitation on the Mobility of Iron in Anaerobically Digested Sludge. Front. Environ. Sci. 4:60. doi: 10.3389/fenvs.2016.00060
Received: 22 December 2015; Accepted: 29 August 2016;
Published: 13 September 2016.
Edited by:
Kartik Chandran, Columbia University, USAReviewed by:
Bharath Prithiviraj, MetaSUB Consortium, USASeung Gu Shin, Pohang University of Science and Technology, South Korea
Copyright © 2016 Roussel and Carliell-Marquet. This is an open-access article distributed under the terms of the Creative Commons Attribution License (CC BY). The use, distribution or reproduction in other forums is permitted, provided the original author(s) or licensor are credited and that the original publication in this journal is cited, in accordance with accepted academic practice. No use, distribution or reproduction is permitted which does not comply with these terms.
*Correspondence: Jimmy Roussel, ai5yb3Vzc2VsQGJoYW0uYWMudWs=