- 1Department of Civil and Environmental Engineering, National University of Singapore, Singapore, Singapore
- 2School of Environmental Science and Engineering, Shanghai Jiao Tong University, Shanghai, China
Increased concentrations of airborne particulate matter in megacities within China, caused by rapid economic growth, urbanization and industrialization, have become a major health concern. High energy consumption, emissions from a large fleet of on-road vehicles and intense industrial activities all contributed to the deterioration of urban air quality in China. In this study, we investigated the bioaccessibility of 12 particulate-bound trace elements (Al, Co, Cd, Cr, Cu, Fe, K, Mg, Mn, Ni, Pb, and Zn) using simulated lung fluids [Gamble's solution (pH = 7.4) and artificial lysosomal fluid (ALF) (pH = 4.5)] following the collection of PM in Shanghai, a megacity in China, to gain a better understanding of their fate upon inhalation by adults and children. All the trace elements showed higher bioaccessibility in ALF, compared to that in the Gamble's solution, due to lower pH and higher ionic strength of the former over the latter. In most cases, the kinetic response of trace elements in terms of their solubility followed a curvilinear pattern and approached near steady-state characteristics after a protracted period of incubation in the lung fluids. Health risk assessment was conducted for adults and children based on the measured bioaccessible fractions of trace elements, which showed significant excess lifetime cancer risk to both children and adults.
Introduction
The rapid urbanization and industrialization since the introduction of the economic reform in 1970s in China brought tremendous economic growth, but also caused severe air pollution problems (Chan and Yao, 2008; Tie and Cao, 2009). The energy consumption tripled from 1973 to 2002 with nearly 70% of the energy generated by coal-fired power plants (Chan and Yao, 2008; Tie and Cao, 2009). Such a high consumption of coal in the energy sector has resulted in the emission of SO2 and particulate-bound trace elements with high levels into the ambient air in the megacities of China such as Beijing, Shanghai, Guangzhou, Tianjing, Xi'an, etc (Bi et al., 2007; Wang et al., 2008; Lü et al., 2012; Wang D. et al., 2014). Other than coal burning, a substantial increase in the number of on-road vehicles coupled with widespread industrial processes and resuspension of soil dust also contributed to the exacerbation of urban air quality in China (Chan and Yao, 2008; Han et al., 2014). More than 70% of the cities within China had high annual average concentrations of total suspended particles (TSP), exceeding the PRC class II residential standard, 200 μg/m3 (Zhang et al., 2010). The number of days with bad air quality and reduced atmospheric visibility (the so-called haze events) have increased in the past few years in the megacities of China, raising serious concerns about adverse effects of particulate air pollution on the environment, the national economy and human health (Tie and Cao, 2009; Qiao et al., 2014; Wang X. et al., 2014). In the year of 2010, outdoor air pollution was responsible for more than 1 million premature deaths in China (Health Effects Institute, 2013). Measures have been taken to mitigate the increasing levels of ambient air pollution such as the enactment of stringent ambient air quality standards and the expansion of air quality monitoring networks in major polluted cities (Chan and Yao, 2008). To help control PM pollution effectively, its sources, chemical composition, and impacts on human health as well as the ecosystem should be investigated. A number of research studies on the physical, chemical, and toxicological properties of PM and related socio-economic effects have been conducted in China, especially during the last decade (e.g., Chan and Yao, 2008; Tie and Cao, 2009; Tan and Zhao, 2014; Andersson et al., 2015).
Shanghai is the major economic center of the eastern China, located at the Yangtze River Delta (YRD) region, the most urbanized and industrialized area of China (Tan and Zhao, 2014). Being one of the biggest megacities (population exceeding 10 million) in China, Shanghai has experienced severe air pollution episodes, especially in 2013, when the PM2.5 concentration exceeded 400 μg/m3. This megacity has a total area of 6340 km2 with more than 20 million residents (permanent residents together with migrants). Finance and other service sectors are growing in Shanghai rapidly, but still heavy industries such as chemical and metal processing industries are major players in the business sector (Chan and Yao, 2008). It was reported that 43% of the days in 2009 with bad air quality were categorized as hazy days in Shanghai (Wang X. et al., 2014). Hua et al. (2014) revealed a significant association between PM2.5 concentrations and the number of asthma cases among children in Shanghai. Qiao et al. (2014) also observed an increase in the number of emergency room visits associated with exposure to the increased PM2.5 concentrations in Shanghai. Many studies were conducted to examine the chemical and physical properties and health effects of PM in Shanghai air (Lu et al., 2011; Lü et al., 2012; Hua et al., 2014; Qiao et al., 2014; Wang X. et al., 2014).
Among the many complex chemical compounds bound to PM, trace elements have gained particular attention due to their ability to induce adverse health impacts (e.g., Pavagadhi et al., 2013; Betha et al., 2014). Elements such as Chromium VI (Cr), Nickel (Ni), Cadmium (Cd), Cobalt (Co), and Arsenic (As) are highly carcinogenic, and classified as carcinogenic compounds by the International Agency for Research on Cancer (IARC, 2013). However, not all the elements present in PM are readily available for biochemical reactions within the body which may produce reactive oxygen species (ROS) that affect human health. Few studies have focused on the bioavailability of toxic chemical constituents in PM as it is thought to be directly related to their health effects (e.g., Adamson et al., 1999). Bioaccessibility, defined as the solubility of chemicals in simulated lung fluids (SLFs), is used as an in vitro measurement of the bioavailability of chemical constituents associated with inhalation scenario (Stopford et al., 2003). While bioavailability refers to the fraction of total elements that can enter the systemic circulation of the physiological systems and exert toxicity in an organism, bioaccessibility measures the soluble fraction of elements when they are exposed to biologically relevant fluids. Furthermore, the bioavailability test which requires the use of an animal in vivo is expensive, laborious, time-consuming, and often influenced by laboratory conditions. In addition, this test is not suitable to be carried out where a huge quantity of environmental samples is concerned (Lu et al., 2011). Hence, in vitro studies using bioaccessibility to predict bioavailability are becoming increasingly popular as they are affordable, simpler, fast, ethical, and not restricted by number of samples (Patinha et al., 2012). The other reason for adopting the in vitro bioaccessibility method to determine the bioavailability potential of contaminants is due to its high correlation with the bioavailability index (Ruby et al., 1996). However, to the best of our knowledge, there is no information currently available on the bioaccessibility of particulate bound-trace elements in Shanghai and also on the dissolution kinetics of individual trace element in SLFs.
This work was carried out to fill these knowledge gaps in terms of enhancing our understanding of the potential health effects of PM in Shanghai by determining the bioaccessibility of 12 trace elements (Al, Co, Cd, Cr, Cu, Fe, K, Mg, Mn, Ni, Pb, and Zn) in two kinds of SLFs, instead of ultrapure water, and the associated health risk. In addition, dissolution kinetics of individual trace element in SLFs was also investigated to provide insights into their different leaching characteristics. Two typically used SLFs, the Gamble's solution and the artificial lysosomal fluid (ALF), were used to simulate different conditions in the alveolar region. Gamble's solution (pH = 7.4) mimics the neutral interstitial fluid between lung cells, while ALF presents the more acidic cellular condition after the phagocytosis process. The two SLFs are closer to the actual cell conditions inside the human lung after an inhalation scenario than ultrapure water, and provide realistic data for health risk assessment. SLFs have been used to analyze the dissolution of inhaled drug (e.g., for asthma), in order to predict their behavior in vivo (Davies and Feddah, 2003). Several studies have employed SLFs to study the trace elements' bioavailability in certain metal(loid) materials, as well as in field samples (Stopford et al., 2003; Midander et al., 2007; Wiseman and Zereini, 2014; da Silva et al., 2015). However, this is the first study of its kind that addresses both bioaccessibility of trace elements in Shanghai PM and associated inhalation health risk.
Methods and Materials
Sample Collection
Total suspended particle (TSP) samples (n = 12) were collected during August and September 2014 at one of the tall buildings (20 meter above ground) on Minhang campus of Shanghai Jiao Tong University (SJTU), Shanghai, China, using a high volume sampler (Tisch environmental, USA). The sampling site is located in a residential area with a major emission of TSP from on-road vehicles, soil dust etc. It is 20 km far away from the city center, with no industries within 3000 meters radius. There is one expressway and a Shanghai Metro station, 300 meters and 1 km to the west of the sampling site, respectively. The sampling location is shown in Figure 1. PTFE filters (8′ × 10′) were used for collection of TSP, preconditioned (22°C, 33% RH) for at least 24 h in a dry box and weighed before field sampling. Sampling were conducted for 24 h at flowrate of 0.5–0.7 m3/min. Post-sample conditioning of PTFE filters was also conducted in the dry box under the same conditions before weighing the exposed filters laden with TSP samples. After the gravimetric determination of the TSP collected, the filters were kept in a −20°C freezer before chemical analysis.
Bioaccessibility Assessment and Kinetic Study
Concentrations of total trace elements, along with their bioaccessible fractions, in the TSP were determined in this work. A portion (1/64 of the total filter area) of the PTFE filters was cut out using a pair of ceramic scissors and used for quantification of total trace elements. To analyze the total trace elements concentration, a closed vessel microwave digestion system (MLS-1200 mega, Milestone Inc., Italy.) was employed. The cut filter samples were added into the pre-cleaned Teflon vessels, together with 4 mL HNO3, 2 mL H2O2, and 0.2 mL HF, and then processed using a microwave digestion system, operated in three stages (250 W for 5 min, 400 W for 5 min, and 600 W for 2 min). After digestion, the extracts were filtered with 0.45 μm Teflon membrane filters, diluted to 50 mL and stored in 4°C refrigerator before further analysis.
Gamble's solution and ALF were freshly prepared using the same chemical composition as reported in literature (Midander et al., 2007). For the bioaccessibility assessment, another portion of PTFE filter samples was added into falcon tubes containing 20 mL of SLFs to allow the dissolution to occur. A total dissolution time of 48 h was employed. To study the dissolution kinetics, 2 mL sub-samples were taken at each pre-defined time points (within 48 h) after centrifuge. The whole dissolution experiment was conducted in the 34°C incubator. All the sub-samples were filtered with 0.45 μm Teflon membrane filters and stored at 4°C. Lastly, concentrations of trace elements (Al, Co, Cd, Cr, Cu, Fe, K, Mg, Mn, Ni, Pb, and Zn) were quantified using an Inductively Coupled Plasma Mass Spectrometer (ICP-MS) (ELAN 6100 PerkinElmer, Inc., MA, USA).
For quality control and quality assurance purposes, a standard reference material (SRM) (NIST SRM 1649a, urban particulate matter) and three laboratory blank filters (three each for total elements, Gamble's soluble elements, and ALF soluble elements) were also analyzed. The reported concentrations of trace elements were calculated after subtraction of their concentrations in blank filters from the total concentrations in TSP samples. The recovery of different elements in SRM 1649a ranged from 85 to 111%. Other established quality control and quality assurance protocols as reported elsewhere were also strictly followed (Betha et al., 2014), such as the use of clean stainless steel forceps to handle filter samples and the use of spiked standards after analyzing 20 samples to check the validation of ICPMS calibration.
Risk Assessment
Health risk assessment was conducted for both adults (>20 years old) and children (10–20 years old) in this work. Four steps are involved in the risk assessment: hazard identification, exposure assessment, dose-response assessment and risk characterization. In this study, the trace elements chosen for risk assessment were: (1) carcinogenic Cd, Co, Ni and Cr; and (2) toxic Al, Co, Cr, Cu, Mn, Ni, Pb, and Zn. In second step, an average chronic daily intake (CDI, mg/kg/day) was calculated using Equation (1). C is the trace elements concentration (mg/m3) (bioaccessible amount of trace elements after 48-h dissolution). EF and ED are the exposure frequency and exposure duration, respectively (180 days exposure frequency was assumed). The inhalation rate (IR) and body weight (BW) used in the Equation were 12.3 m3/day and 57 kg for adults, and 13.6 m3/day and 46 kg for children (Wang et al., 2015), respectively. AT is the averaging life time (70 years, 365 days per year).
The cancer risk and toxic health risk were characterized by Excess Lifetime Cancer Risk (ELCR) and health quotient (HQ), respectively, using Equations (2) and (3). The slope factor (SF) and reference dosage (RfD) were derived from the Integrated Risk Information System (IRIS, USEPA; Lu et al., 2014).
Results
PM and Trace Elements Concentrations
The average mass concentration of TSP during the field campaign period was 61.73 ± 14.86 μg/m3. Chan and Yao (2008) reported that there was a declining trend of the annual average TSP concentration in Shanghai (256 μg/m3 in 1996 ~156 μg/m3 in 2000). However, Wang Y. et al. (2006) reported a very high TSP concentration (231 μg/m3) during 2003–2005. The possible reasons of the low TSP concentration reported in the current study include a short sampling duration (12 selected days over a period of 2 months) and the frequent rain events during the sampling period, leading to the washout of the airborne PM through wet deposition. The measured concentrations of trace elements are presented in Table 1. Mg, Al, K and Fe were the most abundant elements (>1000 ng/m3), while Ni, Co, Cd, and Cr were the less abundant elements (<20 ng/m3). Mg, Al, K and Fe in TSP mainly originated from crustal dust and also possibly from resuspension of soil particles (Wang et al., 2013). Local traffic emissions represent another major emission source of particulate-bound trace elements near the sampling site, since an express way was located within 500 meters from the sampling site. This pollution source may explain the presence of Cu, Pb, and Zn in TSP with relatively high concentrations. Enrichment factors were calculated to find out the enrichment of trace elements, using Equation (4).
Where EFx is the enrichment factor of an element x, Cxp and Cxc are the concentrations of the element x in TSP and crustal matters, respectively, and CAlp and CAlc are the concentrations of Al in the TSP samples and crustal materials, respectively. The elemental concentrations in crustal matters used in the above calculation represent the average concentrations of crustral elements reported by Mason (1966) and Balasubramanian and Qian (2004). According to the EF results (Table 2), K, Mn, and Fe had EFs close to 1, suggesting that they mainly originated from crustal materials. Mg, Cr, Co, and Ni were contributed by air pollution sources of mixed origin. Other than natural emission sources, fuel oil combustion and metal production could also emit Co, Cr, and Ni (Hagler et al., 2007; Ye et al., 2014). The large EFs of Cu, Zn, Cd, and Pb (larger than 10) suggested a major anthropogenic contribution to these elements. As mentioned, Cu, Pb, and Zn were mainly released from the local traffic as the sampling site was situated near an express way. Cd can be emitted from high temperature processes, such as metal smelting and fuel combustion.
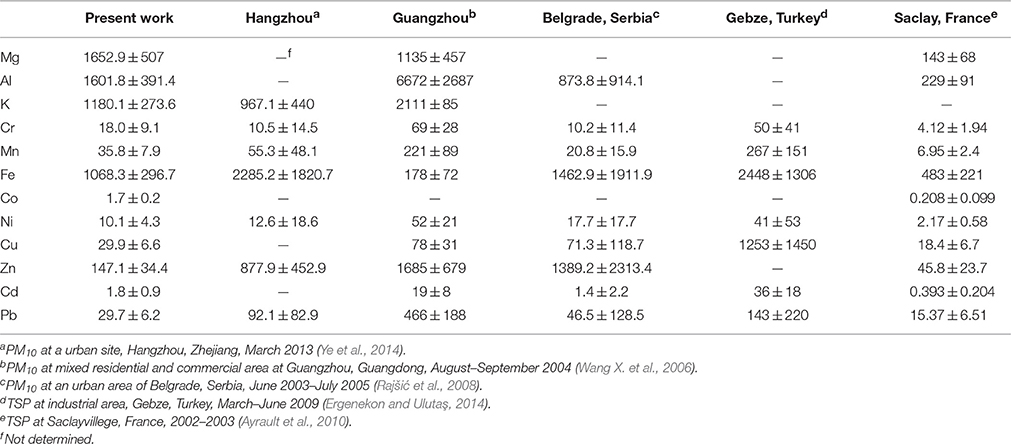
Table 1. Concentration of airborne particulate-bound trace elements in TSP of Shanghai, and other cities (mean ± SD, ng/m3).
Bioaccessibility
Different particulate-bound chemical species behave differently in the human body after their inhalation and deposition, depending on their solubility in lung fluids and toxicity. In general, highly soluble chemical species are easily taken up by lung fluids and then get introduced to the body circulation system through absorption. Thus, bioaccessibility of trace elements is a very important factor to be considered for the assessment of the overall health risk associated with inhalation of PM. Bioaccessibility (%) of the 12 trace elements was estimated as the ratio of the soluble fraction of trace elements in SLFs to the total concentration. The results are shown in Figure 2. Bioaccessibility was then categorized as very high (>50%), high (31–50%), intermediate (16–30%) and low mobility (<15%). The resultant bioaccessibility ranged from 7% (Al) to 47% (K) in the Gamble's solution and 32% (Mn) to 67% (K) in ALF. All the trace elements showed higher solubility in ALF than in the Gamble's solution (p < 0.05), which can be attributed to the more acidic condition of ALF (Colombo et al., 2008). Mg, K and Cu were the most soluble elements in both the Gamble's solution and ALF. No trace elements showed very high solubility in the Gamble's solution. On the contrary, all the trace elements presented a high or very high solubility in ALF. Zn was highly soluble in both the Gamble's solution and ALF. Pb showed a very high bioaccessbility in ALF, but low solubility in the Gamble's solution. Cr, Mn, Co, Ni, and Cd presented high bioaccessibility in ALF, and intermediate solubility in the Gamble's solution. The relatively high accessibility of four carcinogenic trace elements (Cr, Co, Ni, and Cd) may enhance the potential of their health effects. The difference in the bioaccessibility in ALF and the Gamble's solution ranged from 13% (Zn) to 50% (Pb). There was no correlation between bioaccessibility and the total concentrations of trace elements present in PM, suggesting that the bioaccessible fraction of trace elements is not directly related to their abundance in PM. The high bioaccessibility of Cu observed in this study is of concern. According to Fuchs and Packer (2001), chronic copper poisoning can cause damage to eye, liver, and kidney. Though the copper concentration measured in Shanghai is much lower than the guideline value (0.54 μg/m3), the concentration of Cu should still be closely monitored to make sure it is within the safe range. Cu could be released to the atmosphere from sources such as brake pads, rubber tires, concrete, antifouling paints, metal industry, and roofing materials (Midander et al., 2007).
Dissolution Kinetics
Figure 3 shows the representative kinetic results of dissolution of trace elements in the Gamble's solution and ALF. It was observed that the dissolution processes of all the trace elements were fast during the first 2 h and gradually reached an equilibrium afterwards. It appears that a duration of total 48 h of dissolution is sufficient for a complete transfer of trace elements from the particulate phase to the liquid phase. Julien et al. (2011) reported similar dissolution profiles when analyzing the dissolution of SRM s in the Gamble's solution. In general, the trend in the concentration (mg/L) of dissolved trace elements (Mg > K > Fe > Zn > Cu > Ni) is similar to that of total concentrations of particulate-bound trace elements in ambient air (Mg > K > Fe > Zn > Cu > Ni). This observation implied that higher concentrations of trace elements in TSP would also result in their higher dissolved concentrations in lung fluids after inhalation and deposition. However, this trend was not observed for all the trace elements (such as Mn), suggesting that the concentration of total trace elements was not the only factor that governs the leaching process. For instance, Al had a higher total concentration than those of K and Fe, but lower dissolved concentrations in Gamble's solution. All the trace elements had higher dissolved concentrations in ALF than in the Gamble's solution, as well as higher bioaccessibility in ALF. Many studies reported that a lower pH of the liquid media would enhance the dissolution of trace elements (Colombo et al., 2008; Joshi et al., 2009; Wiseman and Zereini, 2014). The higher dissolution of trace elements in ALF suggests that the inhaled PM is likely to undergo phagocytosis before significant amounts of trace elements are dissolved. Phagocytosis is one of the major clearance pathways of particles in the lung (Gehr and Heyder, 2000). It is also suspected that the dissolved metals may hamper the immune function of macrophages (Jalava et al., 2008), and thus lower down the clearance rate of particles through phagocytosis. From Figure 3, it can be observed that different trace elements dissolved in the lung fluids at different rates. For example, it was observed that the dissolution of Cr was slower than that of Cd and Ni. Moreover, the slope of the dissolution profiles of trace elements in ALF was not always larger than that of trace elements in the Gamble's solution. The dissolution rate is determined by the specific chemical speciation forms of trace elements, for example, As (III) vs. As(V) and salts vs. oxidates and origins of the trace elements (crustal or combustion sources; Midander et al., 2007).
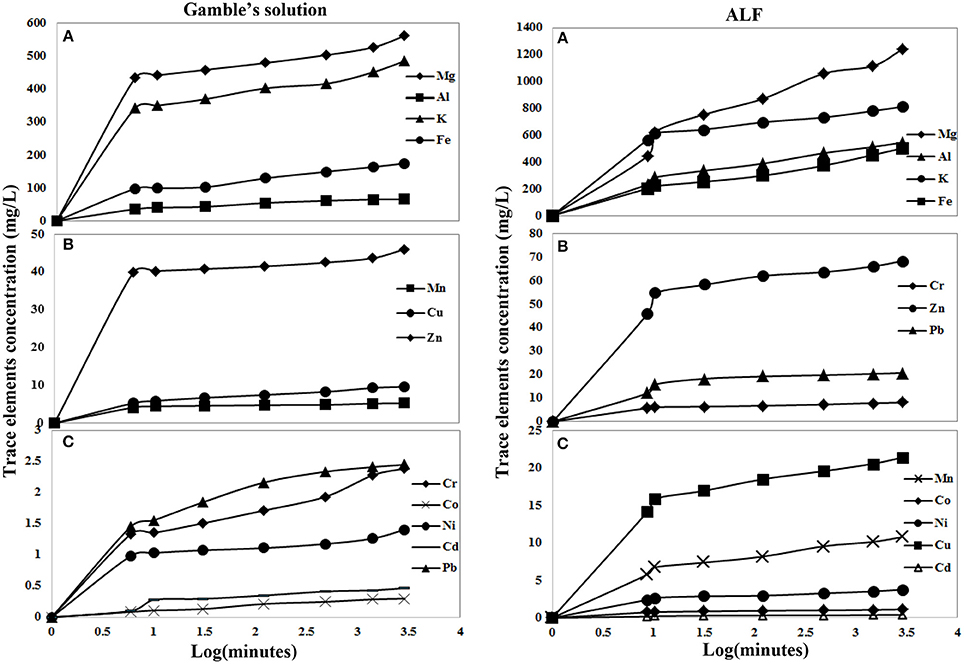
Figure 3. Dissolution kinetics of different trace elements in the Gamble's solution and ALF. Left: (A) for Mg, Al, K, Fe; (B) for Mn, Cu, Zn; and (C) for Cr, Co, Ni, Cd, Pb. Right: (A) for Mg, Al, K, Fe; (B) for Cr, Zn, Pb; and (C) for Mn, Co, Ni, Cu, Cd.
The dissolution of nearly all elements proceeds in a curvilinear trend, when exposed to ALF and the Gamble's solution, with their rapid release occurring at the beginning of a protracted period of incubation (48 h). However, there are variations in the solubility patterns of individual trace elements which are not unexpected because of differences in their origins, chemical states, physical and chemical aging as a function of time during the sampling period, consequently leading to high heterogeneity nature of PM samples collected.
Risk Assessment
The bioaccessible concentrations used in this study provide the upper limit of risk assessment estimation since they represent the availability of toxic elements for absorption when dissolved in SLFs (Mukhtar and Limbeck, 2013). The purpose of using bioaccessible concentrations instead of the total elemental contents in PM samples for health risk calculations is to make a realistic estimate of the actual health risks due to inhalation of PM and to prevent overestimation as the particulate-bound elements are not fully bioaccessible (Luo et al., 2012).
Health risks were conducted for both adults and children. The resultant ELCR and HQ values are presented in Tables 3, 4, respectively. ELCR higher than 10−6 (1 in a million) indicates a significant level of cancer risk, whereas HQ higher than 1 implies significant toxic health effects on exposed human population. As can be seen from Table 4, the HQ values calculated from the bioaccessible fraction of trace elements in both the Gamble's solution and ALF were far <1 (0.084–0.253), suggesting that no significant toxic effects on both adults and children could be expected with the measured concentrations of toxic trace elements. However, only trace elements were considered in the risk assessment. Other toxic compounds presenting in TSP such as PAHs were not considered in this study. Consequently, the HQ may be underestimated. The HQ values estimated for children using both bioaccessible fractions in the Gamble's solution and in ALF were higher than those for adults (p < 0.05). A similar trend was found in the estimated cumulative ELCR (Gamble's solution: 13.4 × 10−6 for adults and 20.4 × 10−6 for children; ALF: 31.1 × 10−6 for adults and 47.4 × 106 for children) (p < 0.05). The results suggested that children that are exposed to outdoor particulate air pollution in Shanghai may experience higher cancer risk and non-cancer risk than adults. All the resultant cumulative ELCRs were higher than 10−6, indicating that adults and children are likely to experience significant cancer risk due to inhalation of particulate-bound trace elements. Mn was the key contributor to HQ while Cr made a significant contribution to the cumulative ELCR. The concentration of Mn was not the highest among all the trace elements considered in the risk assessment. However, it should be of concern because of its high toxicity. It was assumed all the Cr dissolved was Cr(VI), which may overestimate the ELCR for Cr. Both HQ and ELCR calculated using the bioaccessible fraction in ALF were about two times of that calculated using the Gamble's solution (p < 0.05). This was due to the high solubility of trace elements in ALF (pH = 4.5) under more acidic conditions compared to the Gamble's solution (pH = 7.4).
Discussion
The concentrations of trace elements measured in this study were compared to those from other studies in other cities of China, as well as other countries (Table 1). The trace elements concentrations observed at our sampling location were higher than those in Hangzhou (Ye et al., 2014), except for Zn and Pb. Hangzhou is a famous tourist city in Zhejiang, China. Heavy traffic could be the reason for the higher Zn and Pb concentrations present in the PM of Hangzhou. However, Guangzhou, another highly urbanized, and fast-grown city in China, had much higher concentrations of trace elements than the results of the present work (Wang X. et al., 2006), as was the case with the industrial city, Gebze, in Turkey (Ergenekon and Ulutaş, 2014). Compared to Saclay, France (Ayrault et al., 2010), the trace elements concentrations in Shanghai were still quite high. However, the current study did not account for the temporal variations over 1 year. According to Wang et al. (2013), there was a clear difference between the concentrations of trace elements observed in different seasons in Shanghai with their concentrations being lower during the summer and autumn period. In conclusion, even though the concentrations of trace elements in the current sampling location of Shanghai were lower than some heavy polluted industrial cities, they may still be higher than those in some European countries.
da Silva et al. (2015) studied the bioaccessibility of 10 trace elements of PM10 from 4 different locations in Janeiro city, Brazil, in Gamble's solution. Compared to their results, the bioaccessibilities of Mn and Cd measured in Gamble's solution in the present work were lower, whereas Cr had higher bioaccessibility (Table 5). Compared to the bioaccessibility of trace elements reported by Wiseman and Zereini (2014) in Frankfurt Germany, Cr, and Cd had similar solubility in the Gamble's solution. It should be noted that the bioaccessibilities reported by da Silva et al. (2015) varied considerably among the four locations they investigated. The bioaccessibility of trace elements is a function of multi factors such as PM's chemical composition, the affinity between the trace elements and solid matrix, chemical speciation and the size and structure of the particles (Desboeufs et al., 2005; Midander et al., 2007; Fu et al., 2012; Oakes et al., 2012). Desboeufs et al. (2005) revealed that trace elements associated with carbonaceous aerosols were more mobile than those associated with alumina-silicated aerosols. They postulated that the elemental carbon concentration was the main factor that governs the solubility of trace elements. Oakes et al. (2012) reported an increase in the solubility of Fe with an increase in the sulfur content in PM released from combustion sources, because iron sulfate was more soluble in water than iron oxides. These factors varied substantially for different trace elements and for different PM samples, which led to the variations in bioaccessibility results. Compared with the water solubility of trace elements in coarse particles at a suburban area of Shanghai reported by Tong et al. (2002) (67% for K and 46% for Cr), K and Cr showed a similar solubility in ALF, but lower solubility in the Gamble's solution (by 20% for K and 27% for Cr). Mn, Fe and Zn had much higher solubility in both the Gamble's solution and ALF than the water solubility reported by Tong et al. (2002). Thus, using the solubility of trace elements in the water medium as an indicator of bioavailability, as done in many studies, may result in a bias in the assessment of health risk associated with particulate-bound trace elements. Instead, the use of bioaccessible concentrations of trace elements in SLFs which closely mimic the conditions in the human lung is highly recommended for conducting health risk assessment associated with inhalation of PM.
It should be noted that, in the present study, TSP was collected. However, only fine and ultrafine particle can reach the lower part of respiratory system. Thus, the health risk estimated in the current study may be overestimated. For future studies, fine particles should be collected to get a more realistic health risk. With the use of total elemental concentrations in the health risk assessment, the resulting ELCR was 65.1 × 10−6 for adults and 99.0 × 10−6 for children. As per this calculation, the ELCR was overestimated by a factor of 5 compared to the soluble fraction of trace elements in the Gamble's solution and a factor of about 2 compared to that in ALF.
Conclusion
Airborne particles in the urban atmosphere of Shanghai were collected during August and September 2014 for the measurement of bioaccessibility of particulate-bound trace elements using SLFs and for health risk assessment. The results showed that the crustal elements (Al, Fe, K and Mg) had higher concentrations at the sampling location, while anthropogenic elements (Cu, Cd, Pb, etc) had relatively lower concentrations. However, Mg, K and Cu had the highest bioaccessibility in both the Gamble's solution and ALF. Al was the least soluble element. Because of the increased acidity of ALF together with its high ionic strength, all the trace elements were more soluble in ALF than in the Gamble's solution. The difference in the solubility ranged from 13% (Zn) to 50% (Pb). No elements showed very high (>50%) bioaccessibility in the Gamble's solution. However, all the trace elements presented high or very high bioaccessibility (>30%) in ALF. This suggested that more trace elements would further dissolve after the phagocytosis process of the body defense system. The dissolution kinetics of the 12 trace elements was also studied. The dissolution processes were quite fast and almost reached equilibrium after 48 h. The concentration of the dissolved trace elements in SLFs followed the trend of their total concentrations in TSP, implying that trace elements with higher total concentration would result in higher dissolved concentrations in lung fluid after inhalation and deposition. Risk assessment for adults and children conducted using the bioaccessible fraction of trace elements showed that exposure to the outdoor particulate-bound trace elements would not cause any toxic effects (HQ < 1). However, the excess lifetime cancer risk (ELCR: 13.4 × 10−6 to 47.4 × 10−6) was significant and higher to children than adults.
Author Contributions
XH carried out the laboratory work, interpreted data and contributed to the manuscript writing. JC worked with RBa to make research plans and design experiments. DB carried out the field work. RBe and RBa supervised the work and contributed to the manuscript writing.
Conflict of Interest Statement
The authors declare that the research was conducted in the absence of any commercial or financial relationships that could be construed as a potential conflict of interest.
Acknowledgments
This work represents a collaborative research project that was conducted by the respective teams of National University of Singapore (NUS) and Shanghai Jiao Tong University (SJTU). This research program is funded by the National Research Foundation (NRF), Prime Minister's Office, Singapore, under its Campus for Research Excellence and Technological Enterprise (CREATE) program. The authors are grateful to NRF for the financial support from Grant No. R–706–002–101–281, Chinese National Natural Science Foundation (21577090) and National Program on Key Basic Research Project of China (973 Program) (No. 2013CB430005).
References
Adamson, I. Y., Prieditis, H., and Vincent, R. (1999). Pulmonary toxicity of an atmospheric particulate sample is due to the soluble fraction. Toxicol. Appl. Pharmacol. 157, 43–50. doi: 10.1006/taap.1999.8658
Andersson, A., Deng, J., Du, K., Yan, C., Zheng, M., Sköld, M., et al. (2015). Regionally-varying combustion sources of the January 2013 severe haze events over eastern China. Environ. Sci. Technol. 49, 2038–2043. doi: 10.1021/es503855e
Ayrault, S., Senhou, A., Moskura, M., and Gaudry, A. (2010). Atmospheric trace element concentrations in total suspended particles near Paris, France. Atmos. Environ. 44, 3700–3707. doi: 10.1016/j.atmosenv.2010.06.035
Balasubramanian, R., and Qian, W. B. (2004). Characterization and source identification of airborne trace metals in Singapore. J. Environ. Monit. 6, 813–818. doi: 10.1039/b407523d
Betha, R., Behera, S. N., and Balasubramanian, R. (2014). 2013 Southeast Asian smoke haze: fractionation of particulate-bound elements and associated health risk. Environ. Sci. Technol. 48, 4327–4335. doi: 10.1021/es405533d
Bi, X., Feng, Y., Wu, J., Wang, Y., and Zhu, T. (2007). Source apportionment of PM 10 in six cities of northern China. Atmos. Environ. 41, 903–912. doi: 10.1016/j.atmosenv.2006.09.033
Chan, C. K., and Yao, X. (2008). Air pollution in mega cities in China. Atmos. Environ. 42, 1–42. doi: 10.1016/j.atmosenv.2007.09.003
Colombo, C., Monhemius, A. J., and Plant, J. A. (2008). Platinum, palladium and rhodium release from vehicle exhaust catalysts and road dust exposed to simulated lung fluids. Ecotoxicol. Environ. Saf. 71, 722–730. doi: 10.1016/j.ecoenv.2007.11.011
da Silva, L. I. D., Yokoyama, L., Maia, L. B., Monteiro, M. I. C., Pontes, F. V. M., Carneiro, M. C., et al. (2015). Evaluation of bioaccessible heavy metal fractions in PM10 from the metropolitan region of Rio de Janeiro city, Brazil, using a simulated lung fluid. Microchem. J. 118, 266–271. doi: 10.1016/j.microc.2014.08.004
Davies, N. M., and Feddah, M. R. (2003). A novel method for assessing dissolution of aerosol inhaler products. Int. J. Pharm. 255, 175–187. doi: 10.1016/S0378-5173(03)00091-7
Desboeufs, K. V., Sofikitis, A., Losno, R., Colin, J. L., and Ausset, P. (2005). Dissolution and solubility of trace metals from natural and anthropogenic aerosol particulate matter. Chemosphere 58, 195–203. doi: 10.1016/j.chemosphere.2004.02.025
Ergenekon, P., and Ulutaş, K. (2014). Heavy metal content of total suspended air particles in the heavily industrialized town of gebze, Turkey. Bull. Environ. Contam. Toxicol. 92, 90–95. doi: 10.1007/s00128-013-1148-7
Fu, H., Lin, J., Shang, G., Dong, W., Grassian, V. H., Carmichael, G. R., et al. (2012). Solubility of iron from combustion source particles in acidic media linked to iron speciation. Environ. Sci. Technol. 46, 11119–11127. doi: 10.1021/es302558m
Fuchs, J., and Packer, L. (2001). Environmental Stressors in Health And Disease, Vol. 7. New York, NY: CRC Press.
Hagler, G. S. W., Bergin, M. H., Salmon, L. G., Yu, J. Z., Wan, E. C. H., Zheng, M., et al. (2007). Local and regional anthropogenic influence on PM 2.5 elements in Hong Kong. Atmos. Environ. 41, 5994–6004. doi: 10.1016/j.atmosenv.2007.03.012
Han, L., Zhou, W., Li, W., and Li, L. (2014). Impact of urbanization level on urban air quality: a case of fine particles (PM2.5) in Chinese cities. Environ. Pollut. 194, 163–170. doi: 10.1016/j.envpol.2014.07.022
Health Effects Institute (2013). 2010 “Global Burden of Disease,” in Conference on Air Pollutionand Health Effect, School of Environment, Tsinghua University.
Hua, J., Yin, Y., Peng, L., Du, L., Geng, F., and Zhu, L. (2014). Acute effects of black carbon and PM2.5 on children asthma admissions: a time-series study in a Chinese city. Sci. Total Environ. 481, 433–438. doi: 10.1016/j.scitotenv.2014.02.070
IARC. (17 October 2013). Iarc: Outdoor Air Pollution a Leading Environmental Cause Of Cancer Deaths. Available online at: http://www.iarc.fr/en/mediacentre/iarcnews/pdf/pr221_E.pdf
Jalava, P. I., Salonen, R. O., Pennanen, A. S., Happo, M. S., Penttinen, P., Hälinen, A. I., et al. (2008). Effects of solubility of urban air fine and coarse particles on cytotoxic and inflammatory responses in RAW 264.7 macrophage cell line. Toxicol. Appl. Pharmacol. 229, 146–160. doi: 10.1016/j.taap.2008.01.006
Joshi, U. M., Vijayaraghavan, K., and Balasubramanian, R. (2009). Elemental composition of urban street dusts and their dissolution characteristics in various aqueous media. Chemosphere 77, 526–533. doi: 10.1016/j.chemosphere.2009.07.043
Julien, C., Esperanza, P., Bruno, M., and Alleman, L. Y. (2011). Development of an in vitro method to estimate lung bioaccessibility of metals from atmospheric particles. J. Environ. Monit. 13, 621–630. doi: 10.1039/c0em00439a
Luo, X., Yu, S., and Li, X. (2012). The mobility, bioavailability, and human bioaccessibility of trace metals in urban soils of Hong Kong. Appl. Geochem. 27, 995–1004. doi: 10.1016/j.apgeochem.2011.07.001
Lü, S., Zhang, R., Yao, Z., Yi, F., Ren, J., Wu, M., et al. (2012). Size distribution of chemical elements and their source apportionment in ambient coarse, fine, and ultrafine particles in Shanghai urban summer atmosphere. J. Environ. Sci. 24, 882–890. doi: 10.1016/S1001-0742(11)60870-X
Lu, X., Wu, X., Wang, Y., Chen, H., Gao, P., and Fu, Y. (2014). Risk assessment of toxic metals in street dust from a medium-sized industrial city of China. Ecotoxicol. Environ. Saf. 106, 154–163. doi: 10.1016/j.ecoenv.2014.04.022
Lu, Y., Yin, W., Huang, L., Zhang, G., and Zhao, Y. (2011). Assessment of bioaccessibility and exposure risk of arsenic and lead in urban soils of Guangzhou City, China. Environ. Geochem. Health 33, 93–102. doi: 10.1007/s10653-010-9324-8
Midander, K., Wallinder, I. O., and Leygraf, C. (2007). In vitro studies of copper release from powder particles in synthetic biological media. Environ. Pollut. 145, 51–59. doi: 10.1016/j.envpol.2006.03.041
Mukhtar, A., and Limbeck, A. (2013). Recent developments in assessment of bio-accessible trace metal fractions in airborne particulate matter: a review. Anal. Chim. Acta 774, 11–25. doi: 10.1016/j.aca.2013.02.008
Oakes, M., Ingall, E. D., Lai, B., Shafer, M. M., Hays, M. D., Liu, Z. G., et al. (2012). Iron solubility related to particle sulfur content in source emission and ambient fine particles. Environ. Sci. Technol. 46, 6637–6644. doi: 10.1021/es300701c
Patinha, C., Reis, A. P., Dias, C., Cachada, A., Adao, R., Martins, H., et al. (2012). Lead availability in soils from Portugal's Centre Region with special reference to bioaccessibility. Environ. Geochem. Health 34, 213–227. doi: 10.1007/s10653-011-9431-1
Pavagadhi, S., Betha, R., Venkatesan, S., Balasubramanian, R., and Hande, M. (2013). Physicochemical and toxicological characteristics of urban aerosols during a recent Indonesian biomass burning episode. Environ. Sci. Pollut. Res. 20, 2569–2578. doi: 10.1007/s11356-012-1157-9
Qiao, L., Cai, J., Wang, H., Wang, W., Zhou, M., Lou, S., et al. (2014). PM2.5 Constituents and hospital emergency-room visits in Shanghai, China. Environ. Sci. Technol. 48, 10406–10414. doi: 10.1021/es501305k
Rajšić, S., Mijić, Z., Tasić, M., Radenković, M., and Joksić, J. (2008). Evaluation of the levels and sources of trace elements in urban particulate matter. Environ. Chem. Lett. 6, 95–100. doi: 10.1007/s10311-007-0115-0
Ruby, M., Davis, A., Schoof, R., Eberle, S., and Sellstone, C. (1996). Estimation of lead and arsenic bioavailability using a physiologically based extraction test. Environ. Sci. Technol. 30, 422–430. doi: 10.1021/es950057z
Stopford, W., Turner, J., Cappellini, D., and Brock, T. (2003). Bioaccessibility testing of cobalt compounds. J. Environ. Monit. 5, 675–680. doi: 10.1039/b302257a
Tan, J., and Zhao, J. (2014). Aerosol pollution in China: present and future impact on environment. Front. Econ. China 9, 109–137. doi: 10.1016/j.partic.2009.09.003
Tie, X., and Cao, J. (2009). Aerosol pollution in China: present and future impact on environment. Particuology 7, 426–431. doi: 10.1016/j.partic.2009.09.003
Tong, Y., Ni, X., Zhang, Y., Chen, F., Zhang, G., and Ye, S. (2002). Study of the toxicological mechanism of acidified aerosols. Biol. Trace Elem. Res. 85, 149–156. doi: 10.1385/BTER:85:2:149
Wang, D., Hu, J., Xu, Y., Lv, D., Xie, X., Kleeman, M., et al. (2014). Source contributions to primary and secondary inorganic particulate matter during a severe wintertime PM 2.5 pollution episode in Xi'an, China. Atmos. Environ. 97, 182–194. doi: 10.1016/j.atmosenv.2014.08.020
Wang, H., Zhuang, Y., Wang, Y., Sun, Y., Yuan, H., Zhuang, G., et al. (2008). Long-term monitoring and source apportionment of PM2.5/PM10 in Beijing, China. J. Environ. Sci. 20, 1323–1327. doi: 10.1016/S1001-0742(08)62228-7
Wang, J., Hu, Z., Chen, Y., Chen, Z., and Xu, S. (2013). Contamination characteristics and possible sources of PM10 and PM2.5 in different functional areas of Shanghai, China. Atmos. Environ. 68, 221–229. doi: 10.1016/j.atmosenv.2012.10.070
Wang, X., Bi, X., Sheng, G., and Fu, J. (2006). Chemical composition and sources of PM10 and PM2.5 aerosols in Guangzhou, China. Environ. Monit. Assess. 119, 425–439. doi: 10.1007/s10661-005-9034-3
Wang, X., Chen, J., Cheng, T., Zhang, R., and Wang, X. (2014). Particle number concentration, size distribution and chemical composition during haze and photochemical smog episodes in Shanghai. J. Environ. Sci. 26, 1894–1902. doi: 10.1016/j.jes.2014.07.003
Wang, Y., Hu, L., and Lu, G. (2015). Health risk assessments based on existing data of arsenic, chromium, lead, and zinc in china's air. Hum. Ecol. Risk Assess. Int. J. 21, 560–573. doi: 10.1080/10807039.2014.934602
Wang, Y., Zhuang, G. S., Zhang, X. Y., Huang, K., Xu, C., Tang, A., et al. (2006). The ion chemistry, seasonal cycle, and sources of PM2.5 and TSP aerosol in Shanghai. Atmos. Environ. 40, 2935–2952. doi: 10.1016/j.atmosenv.2005.12.051
Wiseman, C. L. S., and Zereini, F. (2014). Characterizing metal(loid) solubility in airborne PM10, PM2.5 und PM1 in Frankfurt, Germany using simulated lung fluids. Atmos. Environ. 89, 282–289. doi: 10.1016/j.atmosenv.2014.02.055
Ye, H., Yang, K., Jiang, X., Niu, L., Hua, D., Li, D., et al. (2014). Hourly variations and potential sources of airborne trace elements in pm10 in four representative regions of southeastern china. Aerosol Air Qual. Res. 14, 1986–1997. doi: 10.4209/aaqr.2014.07.0125
Keywords: trace elements, simulated lung fluids, gamble's solution, artificial lysosomal fluid, risk assessment
Citation: Huang X, Cheng J, Bo D, Betha R and Balasubramanian R (2016) Bioaccessibility of Airborne Particulate-Bound Trace Elements in Shanghai and Health Risk Assessment. Front. Environ. Sci. 4:76. doi: 10.3389/fenvs.2016.00076
Received: 30 May 2016; Accepted: 08 November 2016;
Published: 22 November 2016.
Edited by:
Philip C. W. Kwong, University of Adelaide, AustraliaReviewed by:
Marita Kosmadaki, Andreas Sygros Hospital, GreecePınar Ergenekon, Gebze Technical University, Turkey
Copyright © 2016 Huang, Cheng, Bo, Betha and Balasubramanian. This is an open-access article distributed under the terms of the Creative Commons Attribution License (CC BY). The use, distribution or reproduction in other forums is permitted, provided the original author(s) or licensor are credited and that the original publication in this journal is cited, in accordance with accepted academic practice. No use, distribution or reproduction is permitted which does not comply with these terms.
*Correspondence: Rajasekhar Balasubramanian, Y2VlcmJhbGFAbnVzLmVkdS5zZw==