- 1Department of Biological and Environmental Sciences, College of Arts Sciences, Qatar University, Doha, Qatar
- 2ETH Zurich, Department of Earth Sciences, Zurich, Switzerland
- 3Central Laboratories Unit, Office of Research & Graduate Studies, Qatar University, Doha, Qatar
- 4Center for Sustainable Development, College of Arts and Sciences, Qatar University, Doha, Qatar
Dolomite (MgCa(CO3)2) is an important petroleum reservoir rock mineral common in ancient sedimentary rocks which is infrequently found in modern environments. The mechanism of dolomite formation remains poorly understood, although recent research has focused on the contribution of microbial processes. Sabkha is the Arabic term for saline mudflats occurring in regions characterized by extreme environmental conditions (high temperature, salinity, light intensity, and aridity), where diverse halophilic and extremophilic microorganisms are found. The dynamic evaporitic systems characteristic of sabkhas are crucial for the precipitation of minerals and a role for microorganisms in sabkhas in the process of mineralization has been proposed. In this study the Dohat Faishakh Sabkha in Qatar was investigated for evidence of the role for aerobic bacteria in mediating the formation of high magnesium carbonates and dolomite, two minerals that commonly occur in the sabkha sediments. Twenty-nine strains of aerobic microbes were obtained through inoculation on agar plates from two different cores sampled from the sabkha and identified by 16S rRNA gene sequencing as belonging to the genera Bacillus, Salinivibrio, Staphylococcus and, primarily, Virgibacillus. All strains examined caused the pH of an artificial growth medium to increase from 7 to 8.5; however, not all were capable of mediating mineral formation. Only Salinivibrio and Virgibacillus spp. isolates mediated the formation of detectable solid phases within the agar plates. Light microscopy, scanning electron microscopy energy dispersive X-ray (SEM/EDX), and X-ray diffraction (XRD) analyses indicate that the solid phase produced in the presence of these bacterial strains is MgCa(CO3)2 with a MgCO3 mol% varying from 0 to 40%. The results of these laboratory experiments suggested that, in the Dohat Faishakh Sabkha, aerobic bacteria may contribute in the formation of very high Mg calcite, a mineral that is considered the precursor of ordered dolomite.
Introduction
The processes by which organisms form minerals are known as biomineralization (Weiner and Dove, 2003). Biominerals are of great importance due to their huge impact on the global biogeochemical cycle (Bindschedler et al., 2016). The most abundant biogenic minerals are the calcium carbonates (Mukherjee, 2011). Carbonates such as limestone and dolomite are important carbon reservoirs (Zhu and Dittrich, 2016). Calcium carbonate formation and its burial in marine sediments account for approximately 80% of total carbon removal from the Earth's surface by abiotic and biotic precipitation (Sun and Turchyn, 2014). The biotic precipitation of calcium carbonates, defined as that which is biologically induced or influenced, is performed by various organisms, including bacteria, and has been widely reported and discussed in the literature (Dhami et al., 2013; Anbu et al., 2016; Zhu and Dittrich, 2016). In contrast, the formation of high-magnesium calcites is extremely challenging, due to the high level of hydration of Mg2+ ions, which promotes the formation of Mg-free aragonite, rather than calcite (Lenders et al., 2013). High-magnesium calcite (HMC), containing approximately 50 mol% MgCO3 with a near-dolomite stoichiometry is present in natural settings and can be produced in the laboratory either microbially at low temperature or by chemical synthesis at high temperatures (Long et al., 2014). HMC is commonly referred to in the literature as ‘proto-dolomite’ or sometimes ‘disordered dolomite’ (Gregg et al., 2015), and is proposed as a precursor of ordered dolomite (Kelleher and Redfern, 2002; Rodriguez-Blanco et al., 2015). However, it remains to be determined whether the generation of very HMC represents the initial step of ordered dolomite formation.
Sabkha is the Arabic term for a coastal or inland flat saline mud area occurring in regions characterized by extreme environmental conditions, with high temperature, salinity, light intensity, and aridity, where diverse halophilic and extremophilic microorganisms are found (Dong and Yu, 2007). The dynamic evaporitic systems characteristic of sabkhas are crucial for the precipitation of minerals (Edwards et al., 2010). According to Warren (2000), a poorly ordered non-stoichiometric dolomite, referred to as proto-dolomite, generally precipitates in evaporitic environments. Subsequent diageneses processes, such as inorganic multiple recrystallization, are proposed to lead to the formation of more ordered dolomite (Burns et al., 2000; Baldermann et al., 2015).
Microbial mats, usually within sabkhas, are dynamic ecosystems with variable nutrient resources that support a high diversity of species and a wide range of metabolic processes (Wong et al., 2016). The limitations in mass transfer associated with the activities of various microorganisms, particularly photosynthetic, aerobic, heterotrophic, and sulfate reducing bacteria, lead to the formation of specific microenvironments. These microenvironments are characterized by high temporal and spatial variation, resulting in heterogeneous environments with diverse community structures (Al-Thani et al., 2014).
Dolomite (MgCa(CO3)2) is a common carbonate mineral in ancient sedimentary rocks that is not often found in modern environments (Burns et al., 2000). It is an important petroleum reservoir rock, representing up to 50% of hydrocarbon basins worldwide (Mazzullo, 2004). The Permo-Triassic Khuff Formation of the Middle East, is estimated to contain approximately 15–20% of the world's gas reserves (Adam et al., 2014), and is of fundamental importance for the economies of Qatar, Gulf countries, and the world. It is considered a prominent example of a gas and oil reservoir formed in an arid and evaporitic environment. However, the mechanism of dolomite formation remains poorly understood; in particular, a method to synthesize it in the laboratory at low temperatures has yet to be developed, an issue frequently highlighted in the literature as “the dolomite problem” (Fairbridge, 1957; Land, 1988; Mckenzie and Vasconcelos, 2009; Bontognali et al., 2014a).
Early models of dolomite formation focused on physiochemical processes (Lippmann, 1973; Baker and Kastner, 1981; Lumsden et al., 1995; Brady et al., 1996), while more recent research has emphasized the role of microbial processes in the mineralization process (Sánchez-Román et al., 2008; Sadooni et al., 2010; Bontognali et al., 2012, 2014a; Brauchli et al., 2016). Earlier models of microbial involvement discussed the role of sulfate reducing bacteria in dolomite formation by increasing pH and alkalinity and removing sulfate (Vasconcelos et al., 1995; Vasconcelos and McKenzie, 1997; Wright, 1999). However, dolomites can still form in the presence of high concentrations of dissolved sulfate (Sánchez-Román et al., 2009a). There is increasing evidence for the involvement of aerobic microorganisms in mediating dolomite formation (Sánchez-Román et al., 2007, 2008, 2011). Bacterial activity causes local supersaturation in the microenvironment surrounding the cell, and can overcome low-temperature kinetic barriers to dolomite precipitation (Van Lith et al., 2003; Sánchez-Román et al., 2009b). In fact, microorganisms exhibit the ability to create suitable microenvironments for microbially-mediated mineralization and recycling of metabolites, which has enabled them to adapt to a wide range of environmental conditions throughout the history of Earth, as widely discussed in the literature (Dupraz et al., 2009; Vu et al., 2009; De Carvalho and Fernandes, 2010; Zhang et al., 2013). For example, the production of exopolymeric substances (EPS) may function to provide templates and/or the nucleation sites for mineral formation (Bontognali et al., 2014a).
Recent studies in sabkhas in Abu Dhabi and Qatar carried out by Bontognali et al. (2010, 2012, 2014b) and Brauchli et al. (2016), respectively, provided evidence of a close association between dolomite formation and microbial mats, and the authors propose a role for microorganisms in the mineralization process.
Dohat Faishakh, a hypersaline coastal sabkha in Qatar, has received much attention since the 1960s as a rare modern geological environment where dolomite formation occurs (Illing et al., 1965). The most recent work in Dohat Faishakh, confirmed the importance of living and non-living microbial mats in the formation of authigenic dolomite (Brauchli et al., 2016). Thus, the sabkha represents a unique environment to investigate the involvement of aerobic bacteria in mineral formation.
The aim of this study was to determine whether halophilic, heterotrophic, aerobic bacteria, exhibiting biological activities that could potentially result in dolomite formation, occur in the evaporitic Dohat Faishakh in Qatar and the diversity of such organisms. Aerobic bacteria were isolated from layers of sediments of the Dohat Faishakh sabkha, and their involvement in mineral formation investigated.
Materials and Methods
Description of the Sampling Site
Dohat Faishakh sabkha is a coastal sabkha located in north west of Qatar. Sampling sites were selected based on previous studies confirming the presence of many forms of precipitates and predicting potential dolomite formation (Illing et al., 1965; Brauchli et al., 2016).
Two core samples were collected in two different seasonal periods. Core 1 (24 cm in length) was collected in March 2015 at 25°C. Core 2 (52 cm in length) was collected in October 2015 at 41°C. Samples from layers were immediately harvested aseptically, preventing any possible contamination of layers by one another. Both cores were properly sealed, labeled, and wrapped with foil to prevent any further exposure to light; they were temporally stored in an icebox at 4°C and then transferred to the laboratory for further analysis.
Culture Media
Liquid MEC2 medium (Sánchez-Román et al., 2007) was used to isolate halophilic, heterotrophic, aerobic bacteria, and is composed of 1% (w/v) yeast extract, peptone 0.5% (w/v), glucose 0.1% (w/v), NaCl 7.5% (w/v), and supplemented with 8 g/l calcium acetate monohydrate, and 1 g/l magnesium acetate tetrahydrate (corresponding to Mg: Ca molar ratio of 6.0). MEC2 solid medium was obtained by adding 18 g/l agarose to liquid medium. All media were sterilized by autoclaving at 121°C for 20 min.
Isolation and Purification of Aerobic, Halophilic, and Heterotrophic Bacteria
From both cores, 1 g samples of sediment from equally spaced layers, starting from the surface layer, were suspended in 20 ml liquid MEC2 medium and incubated for 48 h in a shaker at 200 rpm and 30°C. Aliquots of each culture (2 ml) were used to inoculate 20 ml fresh MEC2 medium and incubated similarly to initial cultures. This enrichment step was repeated twice. Next, One hunderd microliter aliquots of each culture were spread on solid MEC2 medium, incubated at 30°C, and colonies formed after 4 days were isolated. Purification of bacterial isolates was carried out using the streak plate method until pure isolates were obtained. Colonies from each plate were observed under the light microscope with 40x and 100x magnification, allowing differentiation of isolates based on colony shapes and colors. Finally, isolates from each layer were selected and given specific codes, referring to core, layer, and colony numbers.
Molecular Identification of Bacterial Isolates
DNA was obtained from cells after overnight growth at 30°C on LB plates. The cells were suspended in 0.5 ml of distilled water, boiled for 10 min in a water bath and then centrifuged for 10 min at 12,300 g. The supernatant (containing total DNA) was placed in a new PCR amplification tube. The 16S rRNA gene fragments (1500 bp) of the bacterial strains were amplified using universal primers; RibS73sp 5′-AGAGTTTGATCCTGGCTCA-3′ and RibS74sp 5′-AAGGAGGTGATCCAGCCGCA-3′ (Weisburg et al., 1991). Each PCR reaction was carried out in a total volume of 25 μl PCR buffer containing 1.5 μM MgCl2, 0.8 μM dNTP, 1.35 μM of both forward and reverse primers, 10–20 ng genomic DNA of the isolates used as template for PCR reactions and 0.5 IU Taq DNA polymerase.
The thermocycler program for each PCR reaction began with an initial denaturation step at 94°C for 3 min followed by 35 cycles of a 45 s denaturation step at 94°C, a 45 s annealing step at 50°C, and a 45 s elongation step at 72°C, then one final 2-min extension step at 72°C. The amplicons were purified using QIAquick PCR Purification Kit–Qiagen.
A secondary PCR of the purified amplicons was carried out, followed by final purification steps using the Applied Biosystems BigDye X-Terminator Purification Kit. Sequencing was carried out using an Applied Biosystems 3500 Series Genetic Analyzer. The obtained 16S rRNA gene sequences from each isolate were used to determine the most closely related sequence among those available in the GenBank database using the NCBI Blast server.
Screening of Mineral Precipitating Isolates
MEC2 plates inoculated with each isolate by surface streaking were incubated for 30 days at 30°C and observed under an optical microscope at 40 and 100x magnification to identify the formation of mineral crystals. Crystals were harvested from each plate and examined under 100x magnification, using the “Acid test” method (King, 2016); one drop of 1 M hydrochloric acid was placed near the edge of the cover slide and allowed to seep under the cover slide. Carbonate minerals were dissolved, causing visible bubbles of carbon dioxide gas, resulting from an acid/carbonate reaction. Carbonate precipitates disappeared after the acid reaction.
Experiments were carried-out in triplicate and four controls were used. One of the isolates which was unable to form mineral crystals was used as a negative control. The second control consisted of uninoculated plates, the third control was performed using modified MEC medium supplemented with minimum concentrations of acetate salts (18 mM magnesium acetate and 3 mM calcium acetate), and the fourth control was performed by spreading 0.1 ml of 1 M NaCO3 on the surface of MEC2 plates, and inducing mineral formation by reaching supersaturation. The latter two controls were performed to check for the abiotic formation of minerals on MEC2 plates.
Scanning Electron Microscopy, Energy Dispersive X-Ray (SEM/EDX) and X-Ray Diffraction (XRD) Analyses
Representative 1 g samples of sediments from each layer of cores 1 and 2 were manually ground using a mortar and pestle, homogenized, and air dried overnight at 37°C. The dried samples were used for SEM and XRD analyses.
For analysis of crystal formation mediated by bacteria, bacterial biomass was removed from the surface of agar plates by gently scrapping the upper layer using a scalpel. MEC2 solid media containing crystals was cut into small pieces, transferred into a 15 ml centrifuge tube and washed three times with distilled water to remove saline and impurities by centrifugation at 5000 × g for 15 min. The minerals were collected from the bottom of the tubes, washed three times with distilled water, and air-dried at 37°C. This procedure did not change the morphology of the crystals, as observed by optical microscopy before and after their recovery. Dried samples were used for SEM/EDS and XRD analyses.
SEM images were obtained using a FEI Quanta 200 Environmental Scanning Electron Microscope (ESEM), with a resolution of 5 nm, and a magnification of x200,000 equipped with an energy dispersive X-ray microanalysis system (EDX, model 2011). Targeted particles were selected and allocated for discrete X-ray analysis to determine their mineralogical composition. Bulk mineralogical composition was determined using a Rigaku model Miniflex II Desktop X-ray Diffractometer with a Scintillator NaI (TI) detector.
Results
Occurrence of HMC and Dolomite in the Evaporitic Environment of Dohat Faishakh Sabkha
Before studying the potential of bacteria to mediate mineral formation in the evaporitic environment of the Dohat Faishakh sabkha in Qatar, evidence for the occurrence of HMC and dolomite was determined in the sediments along the two cores used for isolating the microbial strains, using SEM/EDX and XRD analyses. Figure 1 shows representative SEM/EDX images of bulk sediments from cores 1 and 2, indicating the presence of carbonate minerals with various Mg:Ca ratios. The results of XRD analysis of representative sediments, demonstrating the presence of dolomite, along with gypsum, halite, and a mixture of carbonate minerals comprising calcite and high-Mg calcite in varying proportions, are presented in Figure 2. The main d104 dolomite peaks are broad and slightly shifted to the right compared with the reference dolomite peak, indicating a Mg-rich, non-stoichiometric composition.
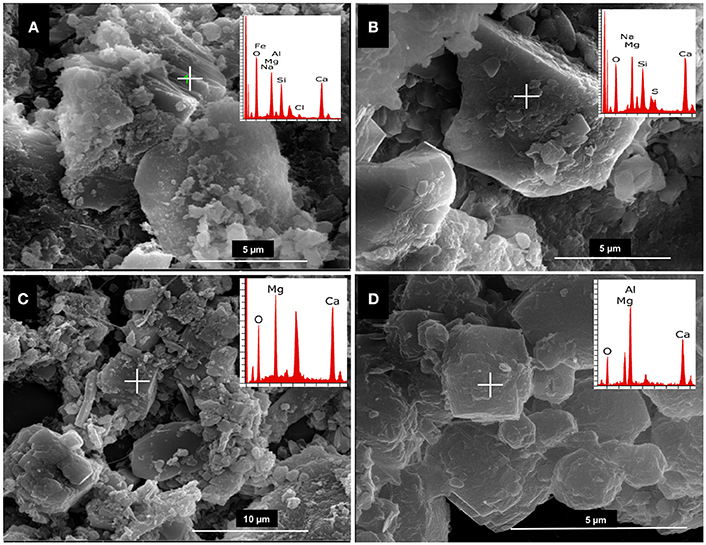
Figure 1. Representative SEM/EDX images of sediment core 1 at −2.5 cm (A), and core 2 at −12.5 cm (B), −22.5 cm (C), and −37.5 cm (D) depth. EDX analysis (top right panels) indicate the presence of high magnesium carbonates and dolomite.
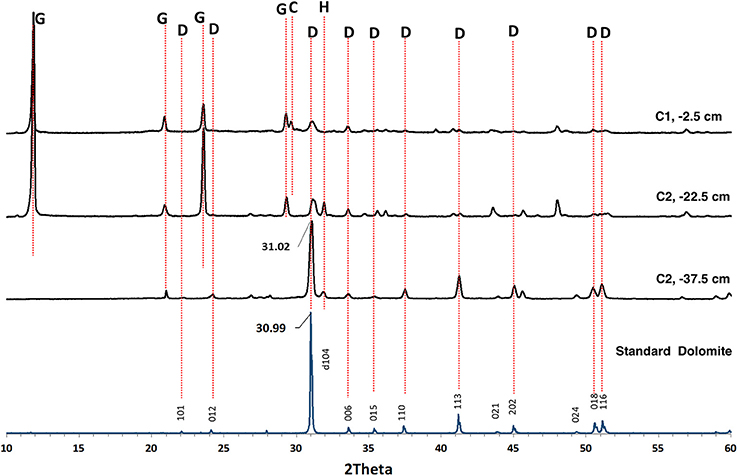
Figure 2. Representative X-ray diffraction patterns obtained by analyzing bulk sediments sampled from cores 1 (C1) and 2 (C2) at selected depths below the surface. The d104 dolomite peaks are slightly shifted to the right with respect to the dolomite standard indicating a composition that contains more mole% MgCO3 than a stoichiometric dolomite. The other peaks correspond to gypsum, calcite, and halite. C, Calcite; D, Dolomite; G, Gypsum; H, Halite.
Isolation and Identification of Aerobic, Halophilic, Heterotrophic Bacteria from Dohat Faishakh Sabkha in Qatar
The 16S rRNA gene sequence of each pure isolate was determined and analyzed using the NCBI BLAST server to find the most closely related sequence available in the GenBank database. A list of isolated strains is presented in Table 1. From core 1, four different genera were isolated from all layers, including three Bacillus species (B. tequilensis, B. licheniformis, and B. subtilis), two Virgibacillus species, two Staphylococcus species (S. sp. and S. epidermidis) and Salinivibrio costicola. From core 2, three genera were identified from all layers with two B. licheniformis isolates, one Staphylococcus sp., and 21 strains of Virgibacillus spp. with two species identified (Virgibacillus marismortui and Virgibacillus salarius). Although colonies were selected based on morphological distinction by light microscopy examination of the corresponding cells, it is possible that some isolates belonged to the same species. These genera provide an initial assessment, and a starting point for the subsequent bacterial mineral formation analyses in the studied Sabkha, obtained independently of their individual potentiality to mediate mineral precipitation.
Study of the Potential of the Isolated Bacteria to Mediate Mineral Formation
The potential of the isolated bacteria to mediate mineral formation was studied using solid MEC2 medium. Isolated strains were cultured by streaking each pure isolate on to the surface of solid MEC2 medium and they were then incubated at 30°C for 30 days. Example results obtained from isolate DF251 (a mineral forming bacterium) are illustrated in Figure 3. The occurrence of solid phase mineral crystals was monitored daily by light microscopy (Figure 3B). All mineral forming strains were able to form crystals within 1 week (Table 1). For the majority of isolates, the earliest mineral formation was recorded after 3 days of incubation. A further examination of the crystals formed (Figures 3C,D) revealed three main morphologically distinct types: dark brown, spherical, large size (Figure 3D1), light brown, spherical, medium size (Figure 3D2), and white, very small, dumbbell shape (Figure 3D3).
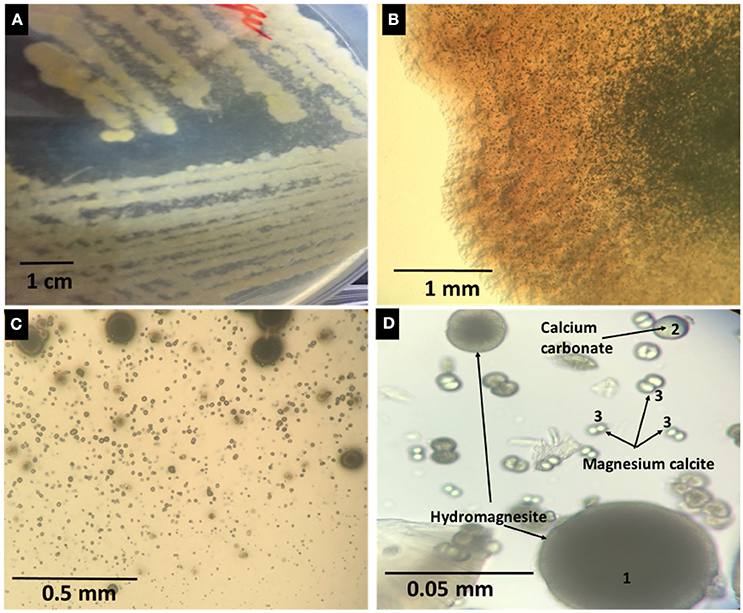
Figure 3. (A) Plates showing visible mineral precipitation in association with the growing biomass of DF251. (B) Minerals observed in culture plates by optical microscopy (40x). (C,D) Microscopic images (100x) showing different shapes and sizes of minerals forming in DF251 cultures.
To confirm the ability of the bacterial isolates to form the observed categories of minerals, four sets of controls were performed. In control experiments using uninoculated solid medium, neither mineral precipitation nor changes in pH were detected. A significant increase of pH (from 7.0 to approximately 8.5) was measured when solid or liquid media was inoculated with the non-mineral forming isolate DF2101; however, there was no mineral formation. Thus, DF2101 is considered a non-crystal forming isolate under the conditions used in this study. All the mineral forming isolates also increased the pH of medium to 8.5. None of the isolates were able to form minerals on the modified MEC medium (18 mM magnesium acetate and 3 mM calcium acetate). Addition of 100 μl 1 M sodium carbonate to the solid media plates led to an increase in pH to approximately 9, but was not associated with mineral precipitation. In liquid cultures, all isolates clearly formed extensive biofilms. An SEM photogram and EDX analysis of the dried biofilm from DF2101 liquid culture are presented in Figure 4.
Involvement of Aerobic Bacteria in HMC Formation
Next, we determined the relationship between the growth of isolated bacteria and the composition of formed crystals in solid media. Analyses of the minerals recovered from MEC2 agar plates were performed by SEM/EDX. Four isolates of Virgibacillus bacteria were selected for this study: DF112 (V. marismortui strain GSP17) from core 1, and DF251, DF291, and DF2141 (all Virgibacillus sp.) from core 2 and the isolate DF153 of S. costicola. The Virgibacillus and Salinivibrio strains from the two cores and different layers were able to increase the pH from 7.0 to approximately 8.5 and mediate mineral formation. The observed crystals had similar spherical morphology; however, they varied in diameter and magnesium: calcium ratios (Figure 5). The largest spheres (diameter, approximately 100 μm) were hydrated magnesium carbonate (hydromagnesite), the smaller spheres (diameter, approximately 50 μm) were calcium carbonate spheres, while the smallest dumbbell shaped crystals (approximately 10 μm) were very high magnesium calcite (HMC).
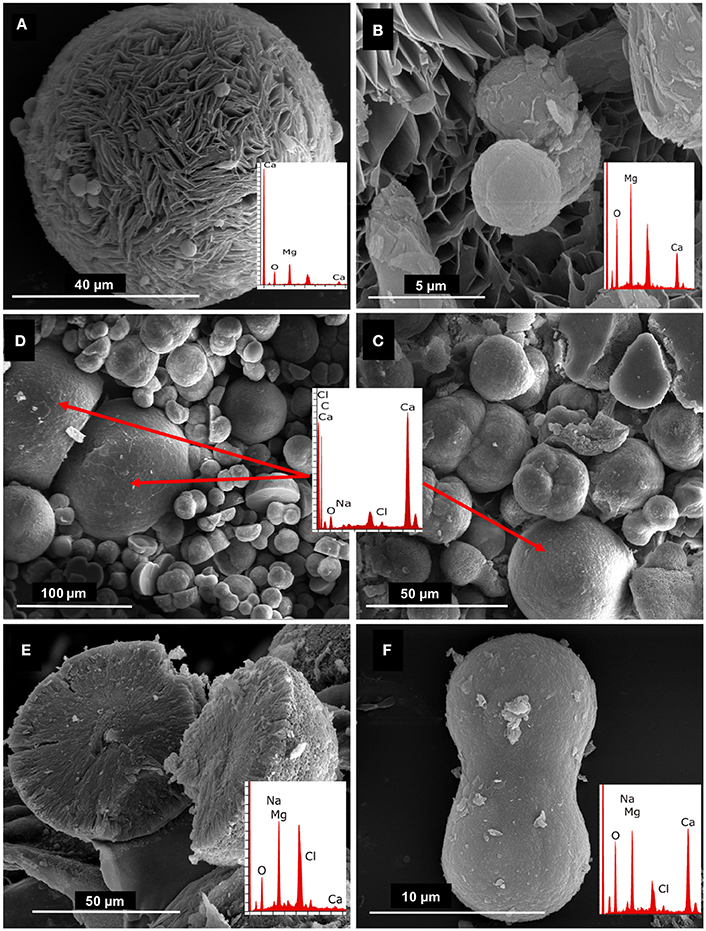
Figure 5. SEM/EDX of (A) Crystal recovered from DF112 culture with elemental composition and morphology of huntite; (B) small HMC crystals embedded into the huntite crystal (close up); (C,D) mixture of crystals recovered from DF291 and DF2141 cultures, respectively, showing calcium carbonate (large crystals) and magnesium calcium carbonates (small, dumbbell shaped crystals), the EDX data shown (overlaid panels) relates to the large calcium carbonate crystals; (E) representative hydromagnesite crystal recovered from all studied cultures; (F) representative close up of dumbbell shaped crystal (HMC) recovered from cultures of isolates DF291 and DF2141.
XRD analysis was carried out for crystals collected from each culture of the four strains after 1 month of incubation (Figure 6). The XRD patterns indicate the presence of very HMC peaks, located between the dolomite and calcite peaks. The Mg contents of the samples were calculated from the (104) peak shift in XRD patterns (Goldsmith et al., 1955). The calculated values of percentage molecules of magnesium ranged from 30 to 40%. Three Virgibacillus isolates from different layers of core 2 and Salinivibrio from core 1 showed similar XRD patterns. The Virgibacillus from the top layer of core 1 exhibited a different pattern, with an HMC peak intensity much higher than that of the other three strains. Overall, these results demonstrate the potential of the Virgibacillus isolated from Dohat Faishakh sabkha in Qatar for mediation of HMC formation.
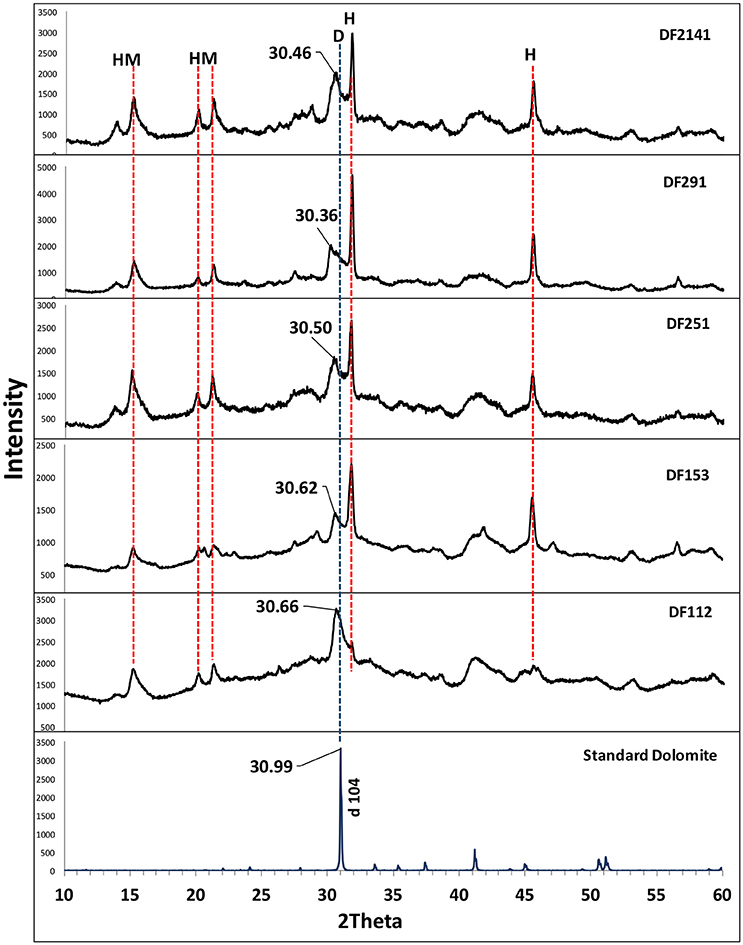
Figure 6. X-ray diffraction pattern of minerals recovered from (DF112, DF153, DF251, DF291, and DF2141) cultures, compared with a standard dolomite XRD pattern. D, Dolomite; HM, Hydromagnesite; H, Halite.
Discussion
A collection of aerobic, halophilic, heterotrophic bacteria was isolated from Dohat Faishakh sabkha in Qatar. It is established that the standard culture techniques used to isolate target microorganisms are highly selective and may not represent the entire microbial community (Rastogi and Sani, 2011). Hence, the bacteria isolated may represent a small population of the microbial species present (Hugenholtz, 2002). In our core samples, the majority of cultured isolates were from four main phyla; Proteobacteria, Firmicutes, Bacteroidetes, and Actinobacteria, which are easily cultivated under laboratory conditions (Rastogi and Sani, 2011). The genus Virgibacillus was the most abundant, followed by Bacillus. The bacterial genera and species found in Dohat Faishakh are reported for the first time in Qatar; however, they have previously been reported in other hypersaline areas. The occurrence of these moderately halophilic species in saline environments is established in the literature. In a review study carried out by Moreno et al. (2013), bacteria belonging to the genera Bacillus, Staphylococcus, and Salinivibrio were isolated from different saline settings across the world, and examined for the production of extracellular enzymes. The long term survivability of Virgibacillus sp. was demonstrated by isolation and growth of these spore forming bacteria from a primary salt crystal aged 250 million years from the Permian Salado formation (Vreeland et al., 2000; Satterfield et al., 2005).
The majority of isolated bacteria were demonstrated by SEM/EDX and XRD analyses to mediate mineral formation in MEC2 solid medium. All the Virgibacillus isolates mediated biological crystal formation, in addition to a Salinivibrio isolate.
The dumbbell and spherical morphologies of the minerals formed have been recognized as microbially induced carbonates in previous reports (Sánchez-Román et al., 2008; Mckenzie and Vasconcelos, 2009; Bahniuk et al., 2015; Mettraux et al., 2015). The co-existence of hydromagnesite and spheroidal dolomite suggests that both may be indicators of microbial dolomite, as previously suggested by Sánchez-Román (2006). In natural environments, the co-precipitation of dolomite and hydromagnesite has been reported, suggesting that hydrous calcium magnesium carbonate may be a precursor of dolomite formation (Kelleher and Redfern, 2002). Here, we confirmed that different aerobic Virgibacillus strains can mediate the formation of carbonate minerals with distinctive mol% of MgCO3. Moreover, individual isolates could also mediate the formation of carbonate minerals with different mol% of MgCO3 during the same experiment. In addition, we observed that all isolated strains were able to increase the pH of the medium, although not all could induce mineral precipitation. This suggests that the role of aerobic bacteria for mineral formation is not limited to increasing pH and alkalinity through respiration. Rather, it is likely that also the chemistry of their cell walls or that of their extracellular polymeric substances (EPS) play and important role for the mineralization process (Bontognali et al., 2008, 2010, 2014a; Roberts et al., 2013). The mechanism by which magnesium is incorporated in the crystal lattice remains a topic of debate in the literature. The role of microbial cell walls, and/or the organic molecules produced by microorganisms was recently discussed (Kenward et al., 2013). Extensive production of EPS associated with the identified species of the aerobic bacteria may play a crucial role in providing the| templates and/or the nucleation sites for mineral formation and the incorporation of Mg in the mineral lattice (Bontognali et al., 2014a).
The results of the current study of the Dohat Faishakh sabkha environment, a unique site where dolomite is being formed under the prevailing weather conditions, demonstrate that pure cultures of aerobic bacteria isolated from the site are able to mediate the formation of very high Mg-calcite, which has been suggested as precursor to ordered dolomite. Four bacterial genera were shown to exist in two cores sampled in different seasons, and several Virgibacillus and Salinivibrio isolates were capable of mediating magnesium calcite formation under aerobic conditions. Our study demonstrates that the Dohat Faishakh sabkha environment in Qatar is an appropriate site in which to investigate the involvement of aerobic heterotrophic bacteria in the formation of carbonate minerals.
Author Contributions
ZA, NZ, SJ, and TB: Substantial contributions to the conception and design of the work, acquisition, analysis, and interpretation of data for the work, drafting the work or revising it critically for important intellectual content, final approval of the version to be published, agreement to be accountable for all aspects of the work in ensuring that questions related to the accuracy or integrity of any part of the work are appropriately investigated and resolved. EA: Acquisition and analysis of data. HA: Analysis of data, and final approval of the version to be published.
Funding
This publication was made possible by NPRP grant 7-443-1-083 from the Qatar National Research Fund (a member of Qatar Foundation). The statements made herein are solely the responsibility of the authors.
Conflict of Interest Statement
The authors declare that the research was conducted in the absence of any commercial or financial relationships that could be construed as a potential conflict of interest.
Acknowledgments
We would like to thank Dr. Saeed Almeer, Director of Central Laboratories Unit (CLU-QU) for his very kind support in SEM/EDX analysis, and Dr. Fadhil Saadoni, Dr. Judith McKenzie, and Dr. Maria Dittrich for providing insight and expertise that greatly assisted the research.
References
Adam, A. M., Abdullatif, O., Yassin, M. A., Eltom, H. A., Babalola, L. O., Abdelraziq, A., et al. (2014). “Enhanced reservoir heterogeneity description; khartam member of the permo-triassic khuff carbonate: outcrop reservoir analog approach from Central Saudi Arabia,” in International Conference and Exhibition (Istanbul: AAPG Datapages).
Al-Thani, R., Al-Najjar, M. A., Al-Raei, A. M., Ferdelman, T., Thang, N. M., Shaikh, et al. (2014). Community structure and activity of a highly dynamic and nutrient-limited hypersaline microbial mat in Um Alhool Sabkha, Qatar. PLoS ONE 9:e92405. doi: 10.1371/journal.pone.0092405
Anbu, P., Kang, C.-H., Shin, Y.-J., and So, J.-S. (2016). Formations of calcium carbonate minerals by bacteria and its multiple applications. Springerplus. 5:250. doi: 10.1186/s40064-016-1869-2
Bahniuk, A. J. A., Perri, E., Bontognali, T. R., Vögeli, N., Rezende, C. E., and Vasconcelos, C. (2015). Characterization of environmental conditions during microbial Mg-carbonate precipitation and early diagenetic dolomite crust formation: Brejo do Espinho, Rio de Janeiro. Brazil 418, 243–259. doi: 10.1144/sp418.11
Baker, P. A., and Kastner, M. (1981). Constraints on the formation of sedimentary dolomite. Science 213, 214–216. doi: 10.1126/science.213.4504.214
Baldermann, A., Deditius, A. P., Dietzel, M., Fichtner, V., Fischer, C., Hippler, D., et al. (2015). The role of bacterial sulfate reduction during dolomite precipitation: implications from upper Jurassic platform carbonates. Chem. Geol. 412, 1–14. doi: 10.1016/j.chemgeo.2015.07.020
Bindschedler, S., Cailleau, G., and Verrecchia, E. (2016). Role of fungi in the biomineralization of Calcite. Minerals 6, 1–19. doi: 10.3390/min6020041
Bontognali, T. R., McKenzie, J. A., Warthmann, R. J., and Vasconcelos, C. (2014a). Microbially influenced formation of Mg-calcite and Ca-dolomite in the presence of exopolymeric substances produced by sulphate-reducing bacteria. Terra Nova 26, 72–77. doi: 10.1111/ter.12072
Bontognali, T. R., Mckenzie, J., Strohmenger, C., Sadooni, F., and Al-kuwari, H. A. (2014b). “The sabkhas of Qatar: an ideal place to study the origin of dolomitic hydrocarbon reservoir rocks,” in Qatar Foundation Annual Research Conference 2014 (ARC'14) (Doha).
Bontognali, T. R., Vasconcelos, C. G., Warthmann, R. J., Bernasconi, S. M., Dupraz, C., Strohmenger, C. J., et al. (2010). Dolomite formation within microbial mats in the coastal sabkha of Abu Dhabi (United Arab Emirates). Sedimentology 57, 824–844. doi: 10.1111/j.1365-3091.2009.01121.x
Bontognali, T. R., Vasconcelos, C., Warthmann, R., Dupraz, C., Bernasconi, S. M., and McKenzie, J. A. (2008). Microbes produce nanobacteria-like structures, avoiding cell entombment. Geology 36, 663–666. doi: 10.1130/G24755A.1
Bontognali, T. R., Vasconcelos, C., Warthmann, R. J., Lundberg, R., and McKenzie, J. A. (2012). Dolomite-mediating bacterium isolated from the sabkha of Abu Dhabi (UAE). Terra Nova 24, 248–254. doi: 10.1111/j.1365-3121.2012.01065.x
Brady, P. V., Krumhansl, J. L., and Papenguth, H. W. (1996). Surface complexation clues to dolomite growth. Geochim. Cosmochim. Acta 60, 727–731. doi: 10.1016/0016-7037(95)00436-X
Brauchli, M., McKenzie, J. A., Strohmenger, C. J., Sadooni, F., Vasconcelos, C., and Bontognali, T. R. (2016). The importance of microbial mats for dolomite formation in the Dohat Faishakh sabkha, Qatar. Carbonates Evaporites 31, 339–345. doi: 10.1007/s13146-015-0275-0
Burns, S. J., Mckenzie, J. A., and Vasconcelos, C. (2000). Dolomite formation and biogeochemical cycles in the Phanerozoic. Sedimentology 47 49–61. doi: 10.1046/j.1365-3091.2000.00004.x
De Carvalho, C. C., and Fernandes, P. (2010). Production of metabolites as bacterial responses to the marine environment. Mar. Drugs 8, 705–727. doi: 10.3390/md8030705
Dhami, N. K., Reddy, M. S., and Mukherjee, A. (2013). Biomineralization of calcium carbonates and their engineered applications: a review. Front. Microbiol. 4:314. doi: 10.3389/fmicb.2013.00314
Dong, H., and Yu, B. (2007). Geomicrobiological processes in extreme environments: a review. Episodes 30, 202–216.
Dupraz, C., Reid, R. P., Braissant, O., Decho, A. W., Sean Norman, R., and Visscher, P. T. (2009). Processes of carbonate precipitation in modern microbial mats. Earth Sci. Rev. 96, 141–162. doi: 10.1016/j.earscirev.2008.10.005
Edwards, H. G., Sadooni, F., Vítek, P., and Jehlička, J. (2010). Raman spectroscopy of the Dukhan sabkha: identification of geological and biogeological molecules in an extreme environment. Phil. Trans. Royal Soc. A. 368, 3099–3107. doi: 10.1098/rsta.2010.0101
Goldsmith, J. R., Graf, D. L., and Joensuu, O. I. (1955). The occurrence of magnesian calcites in nature. Geochim. Cosmochim. Acta 7, 212–230. doi: 10.1016/0016-7037(55)90033-8
Gregg, J. M., Bish, D. L., Kaczmarek, S. E., and Machel, H. G. (2015). Mineralogy, nucleation and growth of dolomite in the laboratory and sedimentary environment: a review. Sedimentology 62, 1749–1769. doi: 10.1111/sed.12202
Hugenholtz, P. (2002). Exploring prokaryotic diversity in the genomic era. Genome Biol. 3:REVIEWS0003. doi: 10.1186/gb-2002-3-2-reviews0003
Illing, L. V., Wells, A. J., and Taylor, J. C. M. (1965). “Penecontemporary dolomite in the Persian Gulf.” Soc. Sediment. Geol. Special Publications (SPEM). 13, 89–111. doi: 10.2110/pec.65.07.0089
Kelleher, I. J., and Redfern, S. (2002). Hydrous calcium magnesium carbonate, a possible precursor to the formation of sedimentary dolomite. Mol. Simulat. 28, 557–572. doi: 10.1080/08927020290030134
Kenward, P. A., Fowle, D. A., Goldstein, R. H. M., Ueshima, M., Gonzalez, L. A., and Roberts, J.A. (2013). Ordered low-temperature dolomite mediated by carboxyl-group density of microbial cell walls. AAPG Bull. 97, 2113–2125. doi: 10.1306/05171312168
King, H. (2016). Geology.com. from The “Acid Test” for Carbonate Minerals and Carbonate Rocks. Available online at: http://geology.com/minerals/acid-test.shtml (Accessed April 13, 2016).
Land, L. S. (1988). Failure to precipitate dolomite at 25°C from solution despite 1000-fold oversaturation after 32 Years. Aquat. Geochem. 4, 361–368. doi: 10.1023/A:1009688315854
Lenders, J. J., Dey, A., Bomans, P. H., Spielmann, J., Hendrix, M. M., With, G. D., et al. (2013). High-magnesian calcite mesocrystals: a coordination chemistry approach. J. Am. Chem. Soc. 134, 1367–1373. doi: 10.1021/ja210791p
Lippmann, D. F. (1973). “Crystal chemistry of sedimentary carbonate minerals,” Sedimentary Carbonate Minerals, Vol. 6 (Berlin; Heidelberg: Springer), 5–96. doi: 10.1007/978-3-642-65474-9_2
Long, X., Ma, Y., and Qi, L. (2014). Biogenic and synthetic high magnesium calcite – a review. J. Struct. Biol. 185, 1–14. doi: 10.1016/j.jsb.2013.11.004
Lumsden, D. N., Morrison, J. W., and Lloyd, R. V. (1995). The role of iron and Mg/Ca ratio in dolomite synthesis at 192°C. J. Geol. 103, 51–61. doi: 10.1086/629721
Mazzullo, S. J. (2004). Overview of porosity evolution in carbonate reservoirs. Kansas Geol. Soc. Bull. 49, 22–28.
Mckenzie, J. A., and Vasconcelos, C. (2009). Dolomite mountains and the origin of the dolomite rock of which they mainly consist: historical developments and new perspectives. Sedimentology 56, 205–219. doi: 10.1111/j.1365-3091.2008.01027.x
Mettraux, M., Homewood, P., Anjos, C. D., Erthal, M., Lima, R., Matsuda, N., et al. (2015). Microbial communities and their primary to early diagenetic mineral phases; the record from Neoproterozoic microbialites of Qarn Alam, Oman. Geol. Soc. Lond. Spec. Publ. 418, 123–154.
Moreno, M. D., Pérez, D., García, M. T., and Mellado, E. (2013). Halophilic bacteria as a source of novel hydrolytic enzymes. Life 3, 38–51. doi: 10.3390/life3010038
Mukherjee, S. (2011). “Minerals and their chemical composition,” in Applied Mineralogy: Applications in Industry and Environment, ed S. Mukherjee (Kolkata: Springer), 19–22.
Rastogi, G., and Sani, R. K. (2011). “Molecular techniques to assess microbial community structure, function, and dynamics in the environment,” in I. Microbes and Microbial Technology, eds F. Ahmad, J. Ahmad, and Pichtel (New York, NY: Springer), 29–57.
Roberts, J. A., Kenward, P. A., Fowle, D. A., Goldstein, R. H., González, L. A., and Moore, D. S. (2013). Surface chemistry allows for abiotic precipitation of dolomite at low temperature. Proc. Natl. Acad. Sci. U.S.A. 110, 14540–14545. doi: 10.1073/pnas.1305403110
Rodriguez-Blanco, J. D., Shaw, S., and Benning, L. G. (2015). A route for the direct crystallization of dolomite. Am. Mineralog. 100, 1172–1181. doi: 10.2138/am-2015-4963
Sadooni, F. N., Howari, F., and El-Saiy, A. (2010). Microbial Dolomites from carbonate-evaporite sediments of the coastal sabkha of abu dhabi and their exploration implications. J. Petrol. Geol. 33, 289–298. doi: 10.1111/j.1747-5457.2010.00480.x
Sánchez-Román, M. (2006). Calibration of Microbial and Geochemical Signals Related to Dolomite Formation by Moderately Halophilic Aerobic Bacteria: Significance and Implication of Dolomite in the Geologic Record. Zurich: Swiss Federal Institute of Technology in Zurich (ETHZ).
Sánchez-Román, M., McKenzie, J. A., Wagener, A. D., Rivadeneyra, M. A., and Vasconcelos, C. (2009a). Presence of sulfate does not inhibit low-temperature dolomite precipitation. Earth Planet. Sci. Lett. 285, 131–139. doi: 10.1016/j.epsl.2009.06.003
Sánchez-Román, M., Rivadeneyra, M., Vasconcelos, C., and McKenzie, J. (2007). Biomineralization of carbonate and phosphate by moderately halophilic bacteria. FEMS Microbiol. Ecol. 61, 273–284. doi: 10.1111/j.1574-6941.2007.00336.x
Sánchez-Román, M., Romanek, C. S., Fernández-Remolar, D. C., Sánchez-Navas, A., McKenzie, J. A., Pibernat, R. A., et al. (2011). Aerobic biomineralization of Mg-rich carbonates: implications for natural environments. Chem. Geol. 28, 143–150. doi: 10.1016/j.chemgeo.2010.11.020
Sánchez-Román, M., Vasconcelos, C., Schmid, T., Dittrich, M., McKenzie, J., and Zenobi, R. (2008). Aerobic microbial dolomite at the nanometer scale: implications for the geologic record. Geology 36, 879–882. doi: 10.1130/G25013A.1
Sánchez-Román, M., Vasconcelos, C., Warthmann, R., Rivadeneyra, M., and McKenzie, J. A. (2009b). Microbial dolomite precipitation under aerobic conditions: results from Brejo do Espinho Lagoon (Brazil) and culture experiments. Int. Assoc. Sedimentol. Spec. Publ. 41, 167–178. doi: 10.1002/9781444312065.ch11
Satterfield, C. L., Lowenstein, T. K., Vreeland, R. H., Rosenzweig, W. D., and Powers, D. W. (2005). New evidence for 250 Ma age of halotolerant bacterium from a Permian salt crystal. Geol. Soc. Am. 33, 265–268. doi: 10.1130/g21106.1
Sun, X., and Turchyn, A. V. (2014). Significant contribution of authigenic carbonate to marine carbon burial. Nat. Geosci. 7, 201–204. doi: 10.1038/ngeo2070
Van Lith, Y., Warthmann, R., Vasconcelos, C., and McKenzie, J. A. (2003). Sulphate-reducing bacteria induce low temperature dolomite and high Mg-calcite formation. Geobiology 1, 71–79. doi: 10.1046/j.1472-4669.2003.00003.x
Vasconcelos, C., and McKenzie, J. A. (1997). Microbial mediation of modern dolomite precipitation and diagenesis under anoxic conditions (Lagoa Vermelha, Rio de Janeiro, Brazil). J. Sediment. Res, 67, 378–390. doi: 10.1306/D4268577-2B26-11D7-8648000102C1865D
Vasconcelos, C., McKenzie, J. A., Bernasconi, S., Grujic, D., and Tien, A. J. (1995). Microbial mediation as a possible mechanism for natural dolomite formation at low temperatures. Nature 377, 220–222. doi: 10.1038/377220a0
Vreeland, R. H., Rosenzweig, W. D., and Powers, D. W. (2000). Isolation of a 250 million-year-old halotolerant bacterium from a primary salt crystal. Nature. 407, 897–900. doi: 10.1038/35038060
Vu, B., Chen, M., Crawford, R. J., and Ivanova, E. P. (2009). Bacterial Extracellular Polysaccharides Involved in Biofilm Formation. Molecules. 14, 2535–2554. doi: 10.3390/molecules14072535
Warren, J. K. (2000). Dolomite: occurrence, evolution and economically important associations. Earth Sci. Rev. 52, 1–81. doi: 10.1016/S0012-8252(00)00022-2
Weiner, S., and Dove, P. M. (2003). An overview of biomineralization processes and the problem of the vital effect. Rev. Mineral. Geochem. 54, 1–30. doi: 10.2113/0540001
Weisburg, W. G., Barns, S. M., Pelletier, D. A., and Lane, A. D. (1991). 16S Ribosomal DNA amplification for phylogenetic study. J. Bacteriol. 173, 697–703. doi: 10.1128/jb.173.2.697-703.1991
Wright, D. T. (1999). The role of sulphate-reducing bacteria and cyanobacteria in dolomite formation in distal ephemeral lakes of the Coorong region, South Australia. Sediment. Geol. 126, 147–157. doi: 10.1016/S0037-0738(99)00037-8
Wong, H. L., Ahmed-Cox, A., and Burns, B. P. (2016). Molecular ecology of hypersaline microbial mats: current insights and new directions - review. Microorganisms 4:6. doi: 10.3390/microorganisms4010006
Zhang, W., Wang, Y., Lee, O. O., Tian, R., Cao, H., Gao, Z., et al. (2013). Adaptation of intertidal biofilm communities is driven by metal ion and oxidative stresses. Sci, Rep. 3:3180. doi: 10.1038/srep03180
Keywords: Biominerals, carbonates, high magnesium calcite, dolomite, Evaporites, aerobic bacteria
Citation: Al Disi ZA, Jaoua S, Bontognali TRR, Attia ESM, Al-Kuwari HAAS and Zouari N (2017) Evidence of a Role for Aerobic Bacteria in High Magnesium Carbonate Formation in the Evaporitic Environment of Dohat Faishakh Sabkha in Qatar. Front. Environ. Sci. 5:1. doi: 10.3389/fenvs.2017.00001
Received: 19 October 2016; Accepted: 09 January 2017;
Published: 03 February 2017.
Edited by:
Jason Raymond, Arizona State University, USAReviewed by:
Doug Bartlett, Scripps Institution of Oceanography, USAJames F. Holden, University of Massachusetts Amherst, USA
Copyright © 2017 Al Disi, Jaoua, Bontognali, Attia, Al-Kuwari and Zouari. This is an open-access article distributed under the terms of the Creative Commons Attribution License (CC BY). The use, distribution or reproduction in other forums is permitted, provided the original author(s) or licensor are credited and that the original publication in this journal is cited, in accordance with accepted academic practice. No use, distribution or reproduction is permitted which does not comply with these terms.
*Correspondence: Nabil Zouari, bmFiaWwuem91YXJpQHF1LmVkdS5xYQ==