- Instituto de Investigaciones en Ecosistemas y Sustentabilidad, Universidad Nacional Autónoma de México, Morelia, Mexico
The nexus approach helps in recognizing the link between water, energy, and food production systems, emphasizing the need to manage them in a more integrated way. The socio-ecosystem (SES) approach, however, goes beyond that, by incorporating the regulation and supporting services in the management equation. Changes in ecosystem integrity affect the delivery of ecosystem services to society, which affects local people's well-being, creating a feedback mechanism regarding management strategies. The SES approach makes explicit the “human-bio-physical” nature of our interaction with ecosystems, highlighting the need for a more integrated and interconnected social-ecological research perspective. In addition, the SES approach makes more explicit the multi-scale character of the ecological processes that structure and maintain social-ecological systems. Water dynamics have an important role in shaping ecosystem's structure and functioning, as well as determining the systems capacity for delivering provisioning services. The tropical dry-deciduous forest (TDF), is particularly useful in studying water-food-energy trade-off interactions. Recently, a category 5 hurricane landed in the study area (Mexico's Pacific coast), triggering various social and ecological problems. This event is challenging the current forest management strategies in the region. The extreme hydrometeorological event created an excellent opportunity to test and promote the SES approach for more integrated food-water-energy research. By using the SES approach within our long-term socio-ecological research project, it was easier to identify opportunities for tackling trade-offs between maintaining the transformation of the system and a more sustainable alternative: promoting the maintenance of the ecosystem's integrity and its capacity to deliver provisioning and regulating services.
Introduction
An international group have been studying the ecosystem implication of biomass extraction for charcoal production in tropical Africa and Latin America (Ghilardi et al., 2013; Mwampamba et al., 2013; Santos et al., 2017). Their main concern is that this extended practice has been a slow, but persistent, pressure on the forest biomass resources. The group has recently adopted the “nexus approach” (sensu lato Hanlon et al., 2013), which seeks a stronger understanding of the interdependencies among food, water, and energy production systems to secure a more sustainable production process. By using simulation models, they have projected the demand for fuel-wood and charcoal for different socio-economic pathways, showing the disproportionate biomass demands that in some regions will require using a large fraction of forest (Santos et al., 2017). By adopting a nexus approach, the group is facilitating the understanding of the socio-economic and ecological interactions of charcoal and agricultural production, especially by highlighting two dimensions of the socio-ecological contexts: charcoal value chains and tenure systems (Iiyama et al., 2017). In addition, the interconnections between sustainable charcoal production in Tanzania, ecosystem services, and trade-offs in the allocation of land, labor, and net primary production have been documented (Doggart and Meshack, 2017).
The aim of this perspective article is to discuss the socio-ecosystem (SES) approach as a conceptual tool for guiding integral food-water-energy research. With the experience gained at the Chamela Mex-LTER Group, which belongs to the International Long-Term Ecological Research (ILTER) network, I will describe ecosystem's water dynamics as an entry point for showing the interconnected nature of the ecological processes. I will then describe the possible effect of management activities on these ecosystems' water dynamics. This analysis helps in recognizing trade-offs between obtaining provisioning ecosystem services (e.g., water, crops, and charcoal) and the conservation of the supporting and regulating ecosystem services. This is also important since the maintenance of an ecosystem's integrity is required to sustain the delivery of such products. Finally, I discuss how the effects an extreme hydrometeorological events is inducing us to define new research questions and hypotheses following a SES approach.
The Socio-Ecosystem (SES) Approach
System thinking has been essential for recognizing the existence of biotic and abiotic components interacting and conforming ecosystems at different and multiple hierarchical scales (systems within systems; Figure 1). Ecosystem ecologists are also helping in identifying the natural processes behind the delivery of provisioning and regulating ecosystem services that sustain human social-economic development. The millennium ecosystem assessment (MA) was successful in documenting the importance of these services and the urgency of conserving and restoring the natural ecosystem behind them. This international initiative (Millennium Ecosystem Assessment, 2005) not only documented the fragility of Earth's life support system, but also the severity of knowledge fragmentation and the difficulties of the scientific system in conducting interdisciplinary research (Norgaard, 2008). System thinking has changed the way we appreciate and understand our world (Ackoff, 1999; Capra and Luisi, 2014), now conceptualizing it as social-ecological systems resulting from humans and ecosystems interacting in time and space at different hierarchical scales (Berkes and Folke, 1998). This SES view is also an attempt to recognize our “human-bio-physical” nature in a completely integrated and interconnected way (Figure 1A; Maass, 2012).
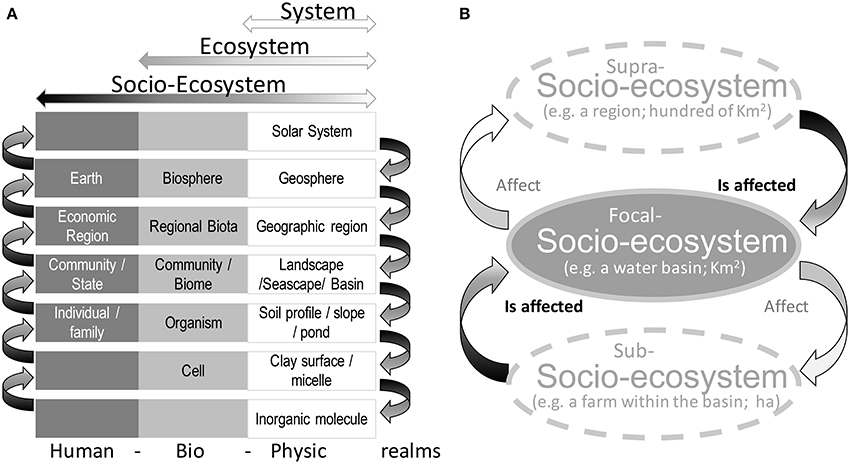
Figure 1. (A) Nested and hierarchical character of socio-ecosystems (SES), with management that always requires a detailed analysis of (B) the factors “affecting” and “being affected” by the supra- and sub-SES.
Socio-ecosystem research requires a shift from viewing humans as external drivers of natural systems to that of agents acting within socio-ecological systems (Grimm et al., 2000; Redman et al., 2004; Haberl et al., 2006). Dealing with SES also requires new epistemic approaches, and the long-term, site-based, bottom-up, and transdisciplinary approach has been suggested as the key ingredient for conducting SES research for sustainability (Carpenter et al., 2012; Fischer et al., 2015; Maass and Equihua, 2015; Balvanera et al., 2017). On these grounds, an “Integrative Science for Society and the Environment” research initiative has been proposed to elevate LTER science to a new level of integration, collaboration, and synthesis necessary for addressing current and emerging environmental research challenges (Collins et al., 2011). This approach has also been the response of some of the ILTER network groups to deal with this endeavor (Maass et al., 2016).
Water as an Integral Component of Ecosystem Processes
Water participates in most energy fluxes and mass recycling ecosystem's processes (Baird and Wilby, 1999; Chaplin, 2001); therefore, water dynamics have an important role in shaping the ecosystem's structure and functioning, as well as determining the system's capacity to deliver provisioning services, such as drinking water, food crops, and fuel-wood biomass. Water availability has been identified as one of the major limiting factors for sustaining terrestrial ecosystem productivity (Chapin et al., 2002). Therefore, maintaining natural water dynamics is a key ecosystem management component and a requirement for reaching sustainable productivity. With this in mind, I will describe the role of water in many ecological processes as an entry point to recognizing the trade-offs between obtaining provisioning ecosystem services and the conservation of the ecological processes that sustain the delivery of such products (also conceived as supporting and regulating services).
Depending on its phase water's presence in the ecosystem highly affects the ecosystem's albedo (the surface short-wave solar reflectivity). For example, while liquid water has an albedo of <20%, a cloud can reach albedos >90%. In addition, significant albedo changes (>25%) can occur within hours when a light-colored soil becomes darker after a rainfall. Albedo is a key ecosystem process since it affects net solar radiation (Q*) entering the ecosystem. Between 80 and 85% of Q* is used either to heat the air through sensible heating fluxes (Qh) or to evaporate water through latent heating fluxes (Qe). The proportion of each flux is known as Bowen's ratio (Qh/Qe). Only a small fraction of Q* (1–3%) is captured through photosynthesis, whereas water evaporation and transpiration processes (Qe fluxes) usually consume >50% of Q* in most forested ecosystems. Transpiration acts as “transportation band,” moving dissolved nutrients in the soil solution to the canopy through a continuous water column flowing from the roots to the stems and branches (Chapin et al., 2002). This high energy consumption process is driven by solar energy, heating the atmosphere, and maintaining the relative humidity gradient (between the stomata and the air) required to sustain transpiration. Water's physical-chemical process (i.e., oxidation, dissolution, evaporation, freezing, etc.) are also the main forces behind rock weathering and nutrient release to the soil solution. Likewise, water moves large quantities of minerals off the land through infiltration, leaching, and erosion. This important “integration character” of water makes its dynamics a key aspect determining ecosystem functioning, as well as a major controlling factor determining ecosystem productivity, including food and biomass production.
Human needs for energy, food, and water have promoted ecosystems' transformations. In fact, at least in tropical areas, regionally distinct modes of agricultural expansion, wood extraction, and infrastructure extension have been identified as the prevailing proximate causes of deforestation (Geist and Lambin, 2002). Deforestation entails nearly total destruction of forest structure and composition, as well as disruption of key ecosystem functions, including its water dynamics (Maass, 1995). Changes in forest cover induce a Bowen's ratio increase (through Qe reduction) and a change in the ecosystem energy balance (through albedo modification). In fact, albedo and Bowen's ratio modification have been identified as a major drivers of climate change, along with green-gas emissions which are also promoted by land use change (Eltahir, 1998). Further land degradation and water dynamic disruption occurs when management practices, such as induced fire or tilling, expose bare soil to direct impact of raindrops. Although small, raindrops are strong enough to break soil clods into small particles that clog soil pores, creating a surface crust which significantly reduces soil infiltration. The soil crusting process has been identified as a major cause of soil erosion, not only inducing land degradation through fertility reduction, but also as the main source of water pollution and siltation in river beds, lakes, and dams (Pimentel, 2006). Infiltration reduction also changes the main route water takes to reach the valley bottomlands, promoting faster overland runoff and floods, and reducing underground water recharge and stream flow during the dry season (Bruijnzeel, 2004). All these water-related trade-offs emerge when natural ecosystems are transformed and should be at the core of any ecosystem management discussion.
Charcoal is an important cooking energy source in rural areas (Ghilardi et al., 2013; Iiyama et al., 2017). Its production promotes forest degradation and, in the long run, can produce a complete deforestation process (Santos et al., 2017) and its consequences in terms of albedo changes, soil crusting, water and wind erosion, floods and droughts. Even biodiversity loss has been detected as a result of the selective harvest of indigenous hardwood species (Naughton-Treves et al., 2007). Charcoal, however, can be produced in more sustainable ways by avoiding deforestation or a permanently degrading process, as well as by protecting harvested areas from cultivation, intensive grazing, and fire, thus enabling natural regeneration (Doggart and Meshack, 2017). This has been the case in Mozambique areas where even under long-term charcoal production they continued to provide ecosystem services (Woollen et al., 2016).
Ecosystem Services and Ecosystem Integrity
Ecosystem transformation to obtain water, energy, and food production not only generates a trade-off between these provisioning ecosystem services, but also with cultural, regulating and, most importantly, supporting services (the basic ecological processes behind the maintenance of all services; Daily et al., 1997). Dealing with these trade-offs, and with the delayed effects of ecosystem manipulation, is a complicated task. One way to do it is by recognizing that natural ecosystems are our best reference of sustainability. Working with nature, understanding and respecting the natural processes behind the ecosystem services is becoming a better strategy than transforming nature at will (Jordan, 1998). Through the maintenance of the “ecosystem integrity,” we can reduce the possibility of unsuspected and long-term effects. Therefore, it is important to link food-water-energy provisioning services with the particular configurations of supporting ecosystem processes that provide those services, using natural ecosystems as sustainable references (Garcia-Alaniz et al., 2017). Equihua et al. (2014) define ecosystem integrity as “the condition where its structure and functions are not impaired and auto-organization dynamics alone are driving the system” and can be measured by how different an actual ecosystem is from some original and desired condition. Changes in integrity take place through ecosystem degradation, and one is the mathematical complement of the other. Since the specific setting of abiotic environment in a given area establishes the context for the compositional, structural, and functional ecosystem attributes, these settings can be measured to infer “ecosystem integrity” status (Garcia-Alaniz et al., 2017). At ILTER, we suggest doing this by using ecosystem integrity and human well-being as key response variables in the analyses of how these variables change under different ecosystem management regimes and in diverse socio-ecological settings (Maass et al., 2016).
Climate Change, LTER, and Research Opportunities
The study of SES responses to intense hydrometeorological phenomena (e.g., drought, flood, frost, etc.) is becoming extremely important under the current climate change scenarios, which are forecasting an increase in their intensity (IPCC's, 2014; Knutson et al., 2015). Extreme hydrometeorological events generate complex management issues such as insect pests, plant mortality, fuel load and fire increase, and CO2 emissions (Shaver et al., 2000; Held and Soden, 2006; Álvarez-Yépiz and Martínez-Yrízar, 2015). In turn, these problems also generate indirect social-economic effects (e.g., tree mortality reduces wood supply) (Walker et al., 1999). Species of TDF have evolved under these highly variable conditions and are adapted to extreme droughts (Holbrook et al., 1995). Land use change, however, increases SES vulnerability and lowers the resilience capacity to these extreme hydrometeorological events (Gavito et al., 2014). Under perturbed conditions, exotic, and invader species resistant to drought and fire (like buffelgrass) also increase (Búrquez-Montijo et al., 2002).
LTER is helping to evaluate the effect of hydrometeorological events by analyzing the risk with more precision (Gavito et al., 2014). LTER also brings information useful to better designing management policies under climate change scenarios. As Collins et al. (2011) have pointed out, pulses and pressures (natural and human-induced) drive ecosystem dynamics, which affects the structure and functioning of natural ecosystems. In turn, the delivery of ecosystem services decreases depending on how much ecosystem integrity has changed. Variation in ecosystem service delivery has an impact on local peoples' well-being, creating a feedback mechanism on management strategies, resulting into pulses or pressure on the ecosystem. In other words, to properly manage this adaptive management cycle, identifying, and understand trade-off among different management alternatives is crucial. Those alternatives that better maintain an ecosystem's integrity will produce higher ecosystem services.
The Chamela Mex-LTER Research Site
At Chamela's Mex-LTER site, in the Mexican Pacific Coast (105°W, 20°N), we have been studying the structure and functioning of the tropical dry-deciduous forest (TDF) within the Chamela-Cuixmala Biosphere Reserve (Maass et al., 2002). The ecosystem's water dynamics, energy fluxes and nutrient cycling have been studied for decades (>35 years). TDF has a strong seasonal character in which 65% of the yearly rain falls in 3 months, creating a strong dry-wet ecosystem dynamic. Inter-annual rainfall is also highly variable in the study region (from 340 to 1,329 mm year−1). Extended droughts alternate with heavy rainstorms creating highly unpredictable climate conditions. Dry periods of 8 consecutive months without any rain are common in the area. Native species are adapted to these extreme conditions. Introduced species under a highly transformed ecosystem, however, become highly vulnerable to these extreme hydrometeorological events. Subsistence agriculture and cattle ranching are the main productive activities in the area. Most stakeholders are not native farmers; they recently colonized the region (in 1960s), arriving from areas other than TDF (Castillo et al., 2005). “Traditional” land management consists of clear-cutting the forest, growing corn for 1–2 years and then converting the agricultural land into induced pasture fields (De Ita, 1983). Soil erosion, compaction, and infiltration reduction are the result of poor management practices, creating a vulnerable environment for the local settlers who suffer from recurrent crop failures because of the lack of sufficient rain during critical moments in the production cycle (Maass, 1995).
In addition, excess rainfall for short periods creates occasional floods with harmful consequences for the settlers at the lower section of the basin. During the last 15 years, a more socio-ecological approach has been conducted (Castillo et al., 2005, 2007; Maass et al., 2005), and currently, a transdisciplinary approach (Spangenberg, 2011) is in the process of being established, promoting appropriate conditions for different stakeholders' participation, not only in our research activities but, most importantly, in the definition of our research program.
In October 2011, Hurricane Jova hit the region and, recently (October 2015), Hurricane Patricia (category 4–5) crossed the Chamela-Cuixmala Reserve, seriously disrupting its forest structure and functioning. This has created an opportunity for triggering a transdisciplinary research under the SES approach. Workshops with local stakeholders allowed us to identify their major concerns after the hurricane landfall in their village and croplands. By consulting with local settlers' views, concerns and interests, our research agenda deviated from the traditional approach, in which the scientific hypotheses are defined strictly on either ecological or social aspects as a separate issue. The exercise helped us link social-ecological process with two possible response scenarios: the “business as usual” response and the “conservation” alternative. The latter pushes forward a more sustainable SES approach. Inspired by the stakeholders input, I identified those social-ecological processes we must evaluate and monitored them after the disturbance (see column one in Table 1). In addition, I identified the most likely response of local settlers to hurricane effects (column two in the same table). Finally, an effort was undertaken to define the type of actions we may need to implement for preventing or mitigating those problematic and likely responses, as a way toward finding of a more hypothetical socio-ecological alternative (last column).
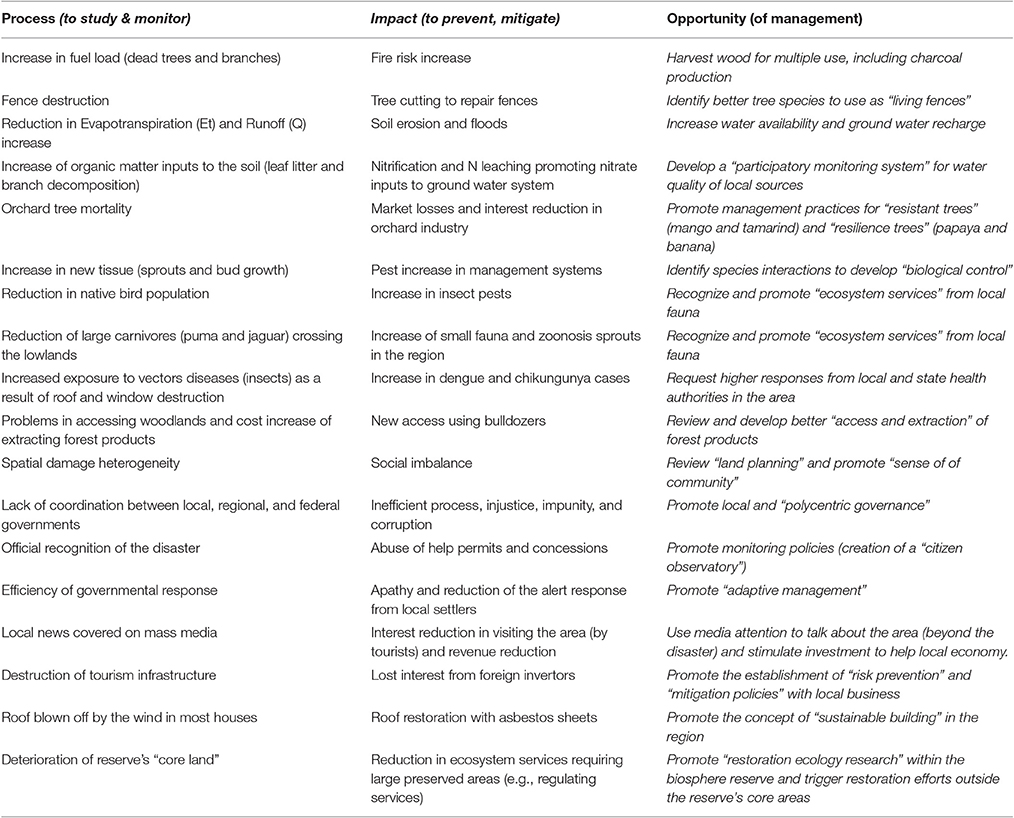
Table 1. Processes, impacts, and management opportunities as a result of Hurricane Patricia affecting the tropical dry-deciduous forest at the Chamela-Cuixmala Biosphere Reserve.
By using this SES approach, it was easier to identify opportunities for tackling trade-offs between continuing to transform the system and a more preserving alternative, which imply the protection of the ecosystem's integrity. For example, it has been suggested the identification of resilient native species to be used as living fences (instead of the traditional use of dead trunks or artificial poles). Likewise, there is a proposal to grant authorization of the removal of dead boles to produce charcoal (traditionally forbidden in the protected areas) as a management strategy to reduce the fire risk that resulted from the the increase of fuel load after the hurricane. In addition, we identify an opportunity for launching a community-based water monitoring system, to promote a better understanding by local people about the importance of conserving their forest land to maintain a good quality of their water sources. See more examples in Table 1.
Final Thoughts
The SES approach not only aids in linking energy, nutrient, and water processes in a natural ecosystem, but it also connects these supporting services with provisioning services, such as food, charcoal, and clean water. The SES approach also helps to recognize the importance of preserving ecosystem integrity and its link with local people's well-being. With this connection in mind, it is easier to identify and deal with the trade-offs between preserving and transforming natural ecosystems. Furthermore, the SES approach highlights the multi-scale (nested and hierarchical) character of the social-ecological processes that structure and maintain SES (Figure 1A), which permits recognizing the high uncertainty that large-scale processes generate in the management practice. To deal with such multi-scale complexity, the manager should focus on one particular scale and analyze its link with the immediate upper (supra system) and lower (sub system) scales; see Figure 1B. This multi-scale character of SES also shows the importance of focusing on local processes as a strategy for facilitating the adaptive management cycle. Finally, the need for promoting long-term and site-based research (i.e., academic groups anchoring their research on specific sites for many years) has become evident for developing not only a better understanding of the local ecosystem, but also the necessary trust between researchers and the local community for efficient transdisciplinary research.
Ethics Statement
Table 1 was prepared using statements from different stakeholders affected by the hurricane's impact on their homes and agricultural lands. No particular names are included in the table. All workshop participants attended by invitation and knew its objectives and the intention of using the obtained information in the publication of scientific papers.
Author Contributions
MM is the sole author of this article. It was prepared under the invitation of Dr. Tuyeni Heita Mwampamba, editor of the special research topic “Charcoal, Food, and Water Production in the Tropics: Applying Nexus Thinking to Improve Research and Policy Approaches in Complex Landscapes” to be included in Frontiers in Environmental Science, in the section Agroecology and Land Use Systems.
Funding
Funding for this publication came from CONACYT (Project No. 179045) and the UNAM-PAPIIT Program (Project No. IN209117).
Conflict of Interest Statement
The author declares that the research was conducted in the absence of any commercial or financial relationships that could be construed as a potential conflict of interest.
Acknowledgments
The author thanks Tuyeni Heita Mwampamba for her invitation to participate in this volume, and two reviewers who provided excellent input to improve the clarity of the document. The author also thanks Raúl Ahedo, who helped with the preparation of the manuscript. The Chamela Long-Term Ecological Research Project (Chamela Mex-LTER) has been the effort of many people during more than 3 decades. This perspective article benefited from the discussions at the “Stakeholders” workshop conducted after the Hurricane Patricia organized by the Chamela Mex-LTER Group, in particular with the help of Drs. Patricia Balvanera, Alicia Castillo, Ek del Val, Elena Lazos, and Angelina Martínez-Yrízar.
References
Ackoff, R. L. (1999). Ackoff's Best: His Classic Writings on Management. New York, NY: John Wiley and Son.
Álvarez-Yépiz, J. C., and Martínez-Yrízar, A. (2015). Huracanes, Sequías y Heladas: Eventos Climáticos Extremos en México. Available online at: http://web.ecologia.unam.mx/oikos3.0/index.php/articulos/fracturacion-hidraulica/8-articulos/165-eventos-climaticos-extremos-y-sus-impactos-en-los-socio-ecosistemas-de-mexico.
Balvanera, P., Daw, T. M., Gardner, T. A., Martín-López, B., Norström, A. V., Ifejika Speranza, C., et al. (2017). Key features for more successful place-based sustainability research on social-ecological systems: a programme on ecosystem change and society (PECS) perspective. Ecol. Soc. 22:14. doi: 10.5751/ES-08826-220114
Berkes, F., and Folke, C. (1998). Linking Social and Ecological Systems: Management Practices and Social Mechanisms for Building Resilience. Cambridge: Cambridge University Press.
Bruijnzeel, L. A. (2004). Hydrological functions of tropical forests: not seeing the soil for the trees? Agric. Ecosyst. Environ. 104, 185–228. doi: 10.1016/j.agee.2004.01.015
Búrquez-Montijo, A., Miller, M., and Martínez-Yrízar, A. (2002). “Mexican grasslands, thornshrub, and the transformation of the Sonoran Desert by invasive exotic buffelgrass (Pennisetum ciliare),” in Invasive Exotic Species in the Sonoran Region, ed B. Tellman (Tucson, AZ: University of Arizona Press), 126–146.
Capra, F., and Luisi, P. L. (2014). The System View of Life: A Unifying Vision. New York, NY: Cambridge University Press.
Carpenter, S. R., Folke, C., Norström, A., Olsson, O., Schultz, L., Agarwal, B., et al. (2012). Program on ecosystem change and society: an international research strategy for integrated social–ecological systems. Curr. Opin. Environ. Sustain. 4, 134–138. doi: 10.1016/j.cosust.2012.01.001
Castillo, A., Magaña, A., Pujadas, A., Martínez, L., and Godínez, C. (2005). Understanding the interaction of rural people with ecosystems: a case study in a tropical dry forest of mexico. Ecosystems 8, 630–643. doi: 10.1007/s10021-005-0127-1
Castillo, A., Pujadas, A., and Schroeder, N. (2007). “La reserva de la biosfera Chamela-Cuixmala, México: perspectivas de los pobladores rurales sobre el bosque tropical seco y la conservación de ecosistemas,” in Hacia una Cultura de Conservación de la Diversidad Biológica, Vol. 6, eds G. Halffter, S. Guevara, and A. Melic (Zaragoza: Monografías Tercer Milenio), 245–254.
Chapin, T. S. III., Matson, P. A., and Mooney, H. A. (2002). Principles of Terrestrial Ecosystem Ecology. New York, NY: Springer.
Chaplin, M. F. (2001). Water: its importance to life. Biochem. Mol. Biol. Educ. 29, 54–59. doi: 10.1111/j.1539-3429.2001.tb00070.x
Collins, S. L., Carpenter, S. R., Swinton, S. M., Orenstein, D. E., Childers, D. L., Gragson, T. L., et al. (2011). An integrated conceptual framework for long-term social–ecological research. Front. Ecol. Environ. 9, 351–357. doi: 10.1890/100068
Daily, G. C., Alexander, S., Ehrlich, P., Goulder, L., Lubchenco, J., Matson, P. A., et al. (1997). Ecosystem services: benefits supplied to human societies by natural ecosystems. Issues Ecol. 2, 1–16.
De Ita, C. (1983). Patrones de producción agrícola en un ecosistema tropical en la costa de Jalisco, Tesis de Licenciatura, Facultad de Ciencias; Universidad Nacional Autónoma de México, 183.
Doggart, N., and Meshack, C. (2017). The marginalization of sustainable charcoal production in the policies of a modernizing african nation. Front. Environ. Sci. 5:27. doi: 10.3389/fenvs.2017.00027
Eltahir, E. A. B. (1998). A soil moisture–rainfall feedback mechanism 1. Theory and observations. Water Resour. Res. 34, 765–776. doi: 10.1029/97WR03499
Equihua, M., García-Alaniz, N., Pérez-Maqueo, O., Benítez, G., Kolb, M., Schmidt, M., et al. (2014). “Integridad ecológica como indicador de la calidad ambiental,” in Bioindicadores: Guardianes de Nuestro Futuro Ambiental. Instituto Nacional de Ecología y Cambio Climático -El Colegio de la Frontera Sur, eds C. A. González Zuarth, A. Vallarino, J. C. Pérez-Jiménez, and A. M. Low Pfeng. (México: El Colegio de la Frontera Sur, Instituto Nacional de Ecología y Cambio Climático), 779.
Fischer, J., Gardner, T. A., Bennett, E. M., Balvanera, P., Biggs, R., Carpenter, S., et al. (2015). Advancing sustainability through mainstreaming a social–ecological systems perspective. Curr. Opin. Environ. Sustain. 14, 144–149. doi: 10.1016/j.cosust.2015.06.002
Garcia-Alaniz, N., Equihua, M., Pérez-Maqueo, O., Equihua Benítez, J., Maeda, P., Pardo Urrutia, F., et al. (2017). The mexican national biodiversity and ecosystem degradation monitoring system. Curr. Opin. Environ. Sustain. 26–27, 62–68. doi: 10.1016/j.cosust.2017.01.001
Gavito, M. E., Martínez-Yrízar, A., Ahedo, R., Araiza, S., Ayala, B., Ayala, R., et al. (2014). La vulnerabilidad del socio-ecosistema de bosque tropical seco de Chamela, Jalisco, al cambio global: un análisis de sus componentes ecológicos y sociales. Invest. Ambiental Ciencia y Política Pública 6, 109–126. Available online at: http://www.revista.inecc.gob.mx/article/view/236#.WX4aBtM1-Rs
Geist, H. J., and Lambin, E. F. (2002). Proximate causes and underlying driving forces of tropical deforestation tropical forests are disappearing as the result of many pressures, both local and regional, acting in various combinations in different geographical locations. Bioscience 52, 143–150. doi: 10.1641/0006-3568(2002)052[0143:PCAUDF]2.0.CO;2
Ghilardi, A., Mwampamba, T., and Dutt, G. (2013). What role will charcoal play in the coming decades? Insights from up-to-date findings and reviews. Energy Sustain. Dev. 17, 73–74. doi: 10.1016/j.esd.2013.02.007
Grimm, N. B., Grove, J. G., Pickett, S. T. A., and Redman, C. L. (2000). Integrated approaches to long-term studies of urban ecological systemsurban ecological systems present multiple challenges to ecologists—pervasive human impact and extreme heterogeneity of cities, and the need to integrate social and ecological approaches, concepts, and theory. Bioscience 50, 571–584. doi: 10.1641/0006-3568(2000)050[0571:IATLTO]2.0.CO;2
Haberl, H., Winiwarter, V., Andersson, K., Ayres, R. U., Boone, C., Castillo, A., et al. (2006). From LTER to LTSER: conceptualizing the socioeconomic dimension of long-term socioecological research. Ecol. Soc. 11, 13. doi: 10.5751/ES-01786-110213
Hanlon, P., Madel, R., Olson-Sawyer, K., Rabin, K., and Rose, J. (2013). Food, Water and Energy: Know the Nexus. GRACE Communications Foundation Water and Energy Programs, New York, NY. Available online at: http://www.gracelinks.org/media/pdf/knowthenexus_final_051513.pdf.
Held, I. M., and Soden, B. J. (2006). Robust responses of the hydrological cycle to global warming. J. Clim. 19, 5686–5699. doi: 10.1175/JCLI3990.1
Holbrook, N. M., Whitbeck, J. L., and Mooney, H. A. (1995). “Drought responses of Neotropical dry forest trees,” in Seasonally Dry Tropical Forests, eds S. H. Bullock, H. A. Mooney, and E. Medina (New York, NY: Cambridge University Press), 243–276.
Iiyama, M., Neufeldt, H., Njenga, M., Derero, A., Ndegwa, G. M., Mukuralinda, A., et al. (2017). Conceptual analysis: the charcoal-agriculture Nexus to understand the socio-ecological contexts underlying varied sustainability outcomes in african landscapes. Front. Environ. Sci. 5:31. doi: 10.3389/fenvs.2017.00031
IPCC (2014). Climate Change 2014. Synthesis Report Summary for Policymakers, Available online at: https://www.ipcc.ch/pdf/assessment-report/ar5/syr/AR5_SYR_FINAL_SPM.pdf
Jordan, C. F. (1998). Working with Nature: Resource Management for Sustainability. Amsterdam: Taylor and Francis.
Knutson, T. R., Sirutis, J. J., Zhao, M., Tuleya, R. E., Bender, M., Vecchi, G. A., et al. (2015). Global projections of intense tropical cyclone activity for the late twenty-first century from dynamical downscaling of CMIP5/RCP4.5 scenarios. J. Clim. 28, 7203–7224. doi: 10.1175/JCLI-D-15-0129.1
Maass, J. M. (1995). “Tropical deciduous forest conversion to pasture and agriculture,” in Seasonally Dry Tropical Forests, eds S. H. Bullock, H. A. Mooney, and E. Medina (New York, NY: Cambridge University Press), 399–422.
Maass, J. M. (2012). “El manejo sustentable de socio-ecosistemas,” in Cambio Climático y Políticas de Desarrollo Sustentable, Tomo 14 de la Colección Análisis Estratégico Para el Desarrollo, ed J. L. Calva(Coord) (México: Editorial Casa Juan Pablos-Consejo Nacional de Universitarios), 267–290.
Maass, J. M., Balvanera, P., Castillo, A., Daily, G. C., Mooney, H. A., Ehrlich, P., et al. (2005). Ecosystem services of tropical dry forests: insights from long-term ecological and social research on the Pacific coast of Mexico. Ecol. Soc. 10:17. doi: 10.5751/ES-01219-100117
Maass, M., Balvanera, P., Bourgeron, P., Equihua, M., Baudry, J., Dick, J., et al. (2016). Changes in biodiversity and trade-offs among ecosystem services, stakeholders, and components of well-being: the contribution of the international long-term ecological research network (ILTER) to programme on ecosystem change and society (PECS). Ecol. Soc. 21:31. doi: 10.5751/ES-08587-210331
Maass, M., and Equihua, M. (2015). “Earth stewardship, socio-ecosystems, the need for a transdisciplinary approach and the role of the international long term ecological research network (ILTER),” in Earth Stewardship: Linking Ecology and Ethics in Theory and Practice, eds R. Rozzi, F. S. Chapin, J. B. Callicott III, S. T. A. Pickett, M. E. Power, J. J. Armesto, and R. H. May Jr. (Dordrecht: Springer). 217–233.
Maass, M., Jaramillo, V., Martínez-Yrízar, A., García-Oliva, F., Pérez-Jiménez, A., and Sarukhán, Y. J. (2002). “Aspectos funcionales del ecosistema de selva baja caducifolia en Chamela, Jalisco,” in Historia Natural de Chamela, eds F. A. Noguera, J. H. Vega-Rivera, A. N. García-Aldrete, and M. Quesada-Avendaño (México: UNAM), 525–542.
Millennium Ecosystem Assessment (2005). Ecosystems and Human Well-Being: Synthesis. Washington, DC, Island Press.
Mwampamba, T. H., Ghilardi, A., Sander, K., and Chaix, K. J. (2013). Dispelling common misconceptions to improve attitudes and policy outlook in developing countries. Energy Sustain. Dev. 17, 75–85. doi: 10.1016/j.esd.2013.01.001
Naughton-Treves, L., Kammen, D. M., and Chapman, C. (2007). Burning biodiversity: woody biomass use by commercial and subsistence groups in western Uganda's forests. Biol. Conserv. 134, 232–241. doi: 10.1016/j.biocon.2006.08.020
Norgaard, R. B. (2008). Finding hope in the millennium ecosystem assessment: an essay. Conserv. Biol. 22, 862–869. doi: 10.1111/j.1523-1739.2008.00922.x
Pimentel, D. (2006). Soil erosion: a food and environmental threat. Environ. Dev. Sustain. 8, 119–137. doi: 10.1007/s10668-005-1262-8
Redman, C. L., Grove, J. M., and Kuby, L. H. (2004). Integrating social science into the long-term ecological research (lter) network: social dimensions of ecological change and ecological dimensions of social change. Ecosystems 7, 161–171. doi: 10.1007/s10021-003-0215-z
Santos, M. J., Dekker, S. C., Daioglou, V., Braakhekke, M. C., and van Vuuren, D. P. (2017). Modeling the effects of future growing demand for charcoal in the tropics. Front. Environ. Sci. 5:28. doi: 10.3389/fenvs.2017.00028
Shaver, G. R., Canadell, J., Chapin, I. F. S., Gurevitch, J., Harte, J., Henry, G., et al. (2000). Global warming and terrestrial ecosystems: a conceptual framework for analysis ecosystem responses to global warming will be complex and varied. Ecosystem warming experiments hold great potential for providing insights on ways terrestrial ecosystems will respond to upcoming decades of climate change. documentation of initial conditions provides the context for understanding and predicting ecosystem responses. Bioscience 50, 871–882. doi: 10.1641/0006-3568(2000)050[0871:GWATEA]2.0.CO;2
Spangenberg, J. (2011). Sustainability science: a review, an analysis and some empirical lessons. Environ. Conserv. 38, 275–287. doi: 10.1017/S0376892911000270
Walker, B. H., Steffen, W. L., Canadell, J., and Ingram, J. S. I. (eds.). (1999). The Terrestrial Biosphere and Global Change. Implications for Natural and Managed Ecosystems. Cambridge: Cambridge University Press.
Keywords: nexus, social-ecological systems, transdisciplinary research, trade-offs, LTER, LTSER, Chamela
Citation: Maass M (2017) Integrating Food-Water-Energy Research through a Socio-Ecosystem Approach. Front. Environ. Sci. 5:48. doi: 10.3389/fenvs.2017.00048
Received: 07 March 2017; Accepted: 24 July 2017;
Published: 08 August 2017.
Edited by:
Rob Bailis, Stockholm Environment Institute, SwedenReviewed by:
Martin Zimmer, Leibniz Centre for Tropical Marine Research (LG), GermanyRajan Ghimire, New Mexico State University, United States
Copyright © 2017 Maass. This is an open-access article distributed under the terms of the Creative Commons Attribution License (CC BY). The use, distribution or reproduction in other forums is permitted, provided the original author(s) or licensor are credited and that the original publication in this journal is cited, in accordance with accepted academic practice. No use, distribution or reproduction is permitted which does not comply with these terms.
*Correspondence: Manuel Maass, bWFudWVsLm1hYXNzQGdtYWlsLmNvbQ==