- 1Department of Environmental Science, SBSR, Sharda University, Greater Noida, India
- 2School of Environmental Sciences, Jawaharlal Nehru University, New Delhi, India
Glacier mass balance is the direct and non delayed response against the climate change and hence it is one of the essential variables require to monitor the climate system. This paper presents a brief review of the glacier mass balance status and their analysis under the changing climate in the Indian Himalayan Region (IHR). The reported results of mass balance in the region give the status of continuous negative mass balance with few exceptions for a year or two reflecting the overall negative mass balance with increasing trend in recent decades. This has coordination with continuous increasing pattern of temperature and decreasing (few not significant) pattern of precipitation. The continuous negative mass balance and rising temperature in the region clearly indicate the impact of warming in reducing the storage of snow and ice in the region with the future implication of shortage of fresh water availability in the snow-glacier fed river system. This will have further implication to the downstream communities for their livelihood.
Introduction
In Twenty first century, climate change is one among many challenges to fight with. Rise in average temperature of earth surface is not the only issue to concern about but decreasing trend of precipitation which further affect the water cycle and ultimately affecting it's availability for drinking, agriculture, hydropower, industrial and domestic purposes, also alarms the situation. Several studies show that the increased temperature (Lemke et al., 2007; Kumar et al., 2014) and/or decreased precipitation (Bhutiyani et al., 2010) are responsible for recession of the glaciers worldwide and these glaciers melting have considerable impacts on regional as well as global scales. For example, the rise of 0.20 m during the period 1901–2010 with further increase of 0.26–0.98 m by 2100 has been noticed in global mean sea level (IPCC, 2013). Likewise, in the past, Jacob et al. (2012) estimated the sea-level rise of 1.48 ± 0.26 mm per year during the period 2003–2010 whereas Chen et al. (2013) estimated 1.80 ± 0.47 mm per year for the period 2005–2011.
The global concentration of glaciers is of about 10% of the Earth's land surface and hold ~77% of its fresh water. Major part of the glacier ice (around 96%) lies in the polar region (Dyurgerov and Meier, 2005). Other than Polar Regions, the Himalaya has the largest glacial cover (~116 × 103 km2) and termed as the “third pole” (Vohra, 1996; Dyurgerov, 2001; Scheel et al., 2011). The melt of these snow and ice provides fresh water to billions of population (Casassa et al., 2009). The Indian portion of the Himalaya (21° 57′ to 37° 05′ N and 72° 40′ to 97° 25′ E) covering an area of 0.35 × 106 km2 (Shanker and Srivastava, 2001) makes an important ecosystem in the Indian Territory. These glaciers are important source of fresh water for Indian rivers and water reservoirs. For water resources planning and management in India, it is essential to study and monitor IHR glacier and snow cover.
Glacier mass balance is a linkage between glacier dynamics and climate (Meier and Tangborn, 1961) as well as climate and mountain hydrology. It is a quantitative entity and can be defined as the resultant of net gain and loss in the glacial mass (Knight, 1999). The studies related to mass balance estimation of glacier is in wide consideration as they are useful in identifying global climate change and explaining rising sea level (Meier, 1984; Oerlemans and Fortuin, 1992; Kuhn, 1993; Dyurgerov and Meier, 1997; Gregory and Oerlemans, 1998). The estimation of change in length and area gives an indirect and delayed response to climate change (Oerlemans, 2007). Conversely, the glacier mass balance is an un-delayed and direct method to identify the impact of climate change on the glaciers (Paterson, 1994; Oerlemans, 2001; Braithwaite, 2002; Dyurgerov and Meier, 2005; Zemp et al., 2009; Cogley, 2011). The systematic and long term study of glacier mass balance can make the glaciers as a good indicator of climate variability (Kaser et al., 2006). Glacier mass balance monitoring is an important research area now-a-days as it is of wide recognition that glaciers are shrinking due to global warming and also to predict the melting rates as a response of future climate warming. In addition the understanding about Himalayan glaciers and their response to the climate change is of great importance when there is a need of an assessment of future water availability to the dependent highly dense populated area (Fountain and Tangborn, 1985; Bakalov et al., 1990; Kaser et al., 2010; Shrestha et al., 2015). Several recent ground-based (Koul and Ganjoo, 2010; Vincent et al., 2013) as well as remote-sensing based mass balance studies (Berthier et al., 2007) have been carried out over different parts of Himalaya. There are strong evidences that the climate change is causing the significant glacier mass loss, consequently, rooted the changes in hydrology and water resources of the region so a wide-range analysis of climate change, especially temperature and precipitation variability is necessary for substantiation of the fact. The present work is an attempt to analyze the mass balance of the glaciers of Indian Himalayan Region (IHR) to understand the changes and the impacts of these changes to the downstream population for better water management. In the present work more emphasis has been given to review the mass balance studies carried out through glaciological method. In first part of the study, focus has also been given to the pattern (observed or predicted) of the temperature and precipitation in different parts of the Himalayan Region.
Himalayan Glacier Regime and Influence of Climate Change
Analysis of historical trend of temperature and precipitation data is the main step to study the climate change. These meteorological data for IHR are limited because of the difficulties in the installation of weather stations at such a high altitude and the survival of the installed weather stations in extreme weather conditions. In spite of the complexity in achieving the data many researchers have tried to gather the required meteorological data for different parts of IHR (i.e. western, central and eastern) and analyze them (Table 1). Diodato et al. (2012) have studied whole Himalayan and Tibetan Plateau region and reported that in past few decades the regions have warmed at a higher rate than the last century. They reported warming of 0.5 °C in annual average maximum temperature over a period of 1971–2005 compared to 1901–1960. In western part of Himalayas, Dash et al. (2007) conducted a study for the period of 102 years (1901–2003) and reported the rise of about 0.09 °C/decade in maximum temperature with major increase after 1972. Dimri and Dash (2012) further reported an increase of 0.23–0.43 °C per decade in air temperature across the western Himalaya by taking winter temperature for the period of 1975–2006. Kumar et al. (2014) conducted a temperature trend analysis for the period of 1985–2009 in Naradu glacier basin, western Himalaya by using the reanalysis MERRA 3 h 3-D data from “GIOVANNI” (the acronyms of Geospatial Interactive Online Visualization And aNalysis Infrastructure (Goddard Earth Sciences Data Information Services Center, 2013). The result indicated higher warming in minimum temperature than maximum temperature during analysis period of 1985–2009. This has an impact of increased melting in winter hence reduction of winter snow cover in the basin and ultimately reduction in glacial mass storage. Likewise, temperature record of North-western Indian Himalaya is also showing the warming trend of about 0.16 °C/decade in last century (1901–2000) with winter warming at a faster rate (Bhutiyani et al., 2007). In another analysis for the period of 1876–2006, Bhutiyani et al. (2010) reported an increase of 0.11 °C/decade in Northwest Indian Himalaya. Singh et al. (2008) studied maximum temperature trend in northwest Indian Himalaya and found increasing trend in all seasons except monsoon. For the period of 1901–2003, Jhajharia and Singh (2011) reported a rise of 0.2–0.8 °C/decade in average temperature, 0.1–0.9 °C/decade in maximum temperature and 0.1–0.6 °C/decade in minimum temperature. Immerzeel (2008) also reported an increase of 0.06 °C/decade during the period of 1900–2002 over Brahmaputra basin in the eastern Indian Himalaya and Tibetan Plateau. In spite of the trend analysis of the past, few studies have also concentrated on the future temperature trend over the Himalayas like Sanjay et al. (2017) and Dimri et al. (2018) assess the changes till the end of Twenty-first century. Their results are aligned with the past temperature trend and indicating the warming in ongoing century which certainly will affect negatively to the storage of the glacial mass in the Himalaya.
The precipitation data of the Himalayan region are more scarce than the availability of the temperature data and do not show any statistically significant trend. Due to the limitation of the observational data in Himalayas, many studies have been conducted to model the precipitation data over the Himalayan region (Sanjay et al., 2017). Himalayan glaciers have its precipitation due to two major weather systems (i) Indian Summer Monsoon (ISM) system (June-September) and (ii) the winter westerly disturbances (WD) (December to March) (Finkel et al., 2003; Yang et al., 2008; Bookhagen and Burbank, 2010; Wulf et al., 2010; Tiwaril et al., 2017). The dynamics of ISM is entrenched with the dynamics of the large-scale ocean–atmosphere interaction called as Inter-Tropical Convergence Zone (ITCZ) (Held and Hou, 1980; Goswami et al., 1984; Neelin and Held, 1987; Chou and Neelin, 2003; Schneider et al., 2014). The ITCZ occurs because of the difference in seasonal precipitation and seasonal temperature in the northern and southern hemisphere (Webster et al., 1998; Loschnigg and Webster, 2000). The input by WD snow fall plays a vital role to sustain regional snowpack, replenishing regional water resources (Pisharoty and Desai, 1956; Chitlangia, 1976; Dimri et al., 2015). The moisture of the western disturbance originates over the Mediterranean Sea (Hatwar et al., 2005) and crosses the country Iran, Afghanistan, and Pakistan before its arrival in India during the winter season. In winter months, western disturbance go to their lowest latitudes and carries moisture from Arabian Sea during its path (Mohanty et al., 1998; Kar and Rana, 2014). During its way it crosses the northern and central part of the India in a phased manner (Yadav et al., 2009) which causes the solid precipitation in the higher elevations of North-Western India and winter liquid precipitation in the plains of northern and central India. Eastern Himalayan glaciers have their precipitation during the summer season with little precipitation in winter month caused by the North-east monsoon. The amount of winter precipitation has large impact in the survival of the glacier and consequently in water management. Bhutiyani et al. (2010) analyzed three stations (northwest Indian Himalaya) precipitation data and reported a statistically significant downward trend in monsoon season during 1866–2006. The similar trend but not significant has been found by Sontakke et al. (2009) for the period of 1960–2006 over the western Indian Himalayan region. Winter precipitation which has large impact on glacier basins and associated population shows a significant decreasing trend in western Himalayan region during 1975–2006 (Dimri and Dash, 2012). Another study in Jammu and Kashmir and Uttarakhand of Himalayan region also shows the statistically significant downward trend in winter precipitation during 1901–2003 (Guhathakurta and Rajeevan, 2008).
Glacier Mass Balance
Mountain glaciers are essentially connected to the population of nearby surroundings, thus changes to mountain glaciers have quicker and considerable impact on huge population compared to the variations of polar ice. Hence to know about the glacial changes in terms of length, area and volume becomes important to address the concerns of the downstream communities as well as the biodiversity of the region. There are several methods to measure the glacier mass balance that include glaciological, hydrological, geodetic, and remote sensing based methods. Among all methods, glaciological method is preferred to be the most accurate method to study the glacier mass although this method is comparatively difficult because it requires visit to tough terrain in the inclement weather condition and is costly as well. This makes the less study of the mass balance in such a large segment of the available snow and glaciers. Glaciological method is based on in-situ measurements. The change in mass between the two different dates of any particular hydrological year is measured at several positions at the glacier surface. The resultant mass gives the mass balance of that particular point when multiplied with the surface density. Glacier mass changes can be measured by measuring the exposed stakes at two different dates and depth of snow relative to a recognized stratigraphic surface (e.g., previous summer/winter surfaces). Snow density is measured by digging of snow pits down to a reference surface in accumulation area.
The study compiled by World Glacier Monitoring Service (2017) provides the most recent summary of 6,500 in situ mass balance from 450 glaciers over the globe. In Alaska, a long-term (~50 years) mass balance measurements have been reported from Gulkana and Wolverine. Another, long term observed Muir glacier, shows a mass balance of –ve 0.5 m w.e. a−1 during the 1990s and 2000s, with 4 years of positive mean balances in 1999–2000, 2000–01, 2007-08, and 2011-12. In the year 2013-14, the maritime glaciers showed a negative mass balance with −962 mm w.e. a−1 followed by more negative balance of −1,337 mm w.e. a−1 in 2014-15. The glaciers of Western North America, showed a mean annual mass balance rates of 400 mm w.e. a−1, 450 mm w.e. a−1 and almost −1000 mm w.e. a−1 during the years 1980s, 1990s, and in the 2000s respectively. In Greenland, Mittivakkat and Freya are the few glaciers for which observations are available after 2010. Both the glaciers show –ve mass balance during 1990–2000. In 2013-14, Mittivakkat showed again a negative balance (−1,200 mm w.e.), while Freya glacier showed a +ve mass balance (394 mm w.e.). In 2014-15 both glaciers had positive balances, averaging 171 mm w.e. Asia South West and East comprise the world's largest mountain range known as Himalaya, which covered a wide region from Nanga Parbat (8,126 m a.s.l.) from NW to Mancha Barwa (7,782 m a.s.l.) from SE. The variable glacier sample of the region shows the balance of −828 and −680 mm w.e. for 2013-14 and 2014-15 respectively (World Glacier Monitoring Service, 2017). Recent study of other Asian Himalayan glaciers, like Pokalde and West Changri Nup glaciers of Everest region, Nepal Himalaya also shows the negative mass balance for the year 2009-2015 (Sherpa et al., 2017). Likewise, extensive mass balance measurement record of 22 glaciers of China shows a decrease of −0.015 m w.e. per year during the period 1959–2015 (Che et al., 2017).
For Indian Himalayan glaciers, the detailed mass balance study using glaciological method has started in 1974 by Geological Survey of India (GSI). Gara glacier in Himachal Pradesh was taken for detailed studies with a view to understand the importance of mass balance inputs in various fields of developments and their interaction with climate and hydrological system.
The Geological Survey of India estimated total 9,575 glaciers in IHR (Sangewar and Shukla, 2009), out of which only 15 glaciers have been studied for glacier mass balance till now. Details of the studied glaciers of IHR have been summarized in Table 2 and their specific mean annual mass balance has been shown through Figure 1. The Gara glacier (1974/75–1981/82) and Nehnar glacier (1975/76–1983/84) have been studied for continuously 8 years. The Gara glacier showed positive mass balance for first 2 years and last 1 year and for rest 5 years it showed negative balance. Seasonal study has shown the positive mass balance in summer consecutively makes the annual mass balance positive (Raina et al., 1977; Raina, 2009). The historic data of temperature and precipitation of the same year may give clear picture behind the findings. Nehnar glacier showed negative mass balance for entire study period and ranges from −1.23 to −0.02 m w.e. (Srivastava et al., 1999). Shaune Garang is well-studied glacier and have long series (10 years) of mass balance. During the study period Shaune Garang showed positive balance in 2 years (1982–83 and 1988–89) whereas the ablation was more in comparison to accumulation in rest 8 years (Singh and Sangewar, 1989; Mukherjee and Sangewar, 1996). Another group of researchers are working on different aspects of Shaune Garang glacier including the mass balance since 2013 under the project entitled Contribution to High Asia Runoff from Ice and Snow (CHARIS) and their findings are also showing the negative mass balance (to be published). The ongoing mass balance study on Naradu glacier of Himachal Himalaya under the funding of Department of Science and Technology (DST), Govt. of India is also showing the negative mass balance in 6 (2011–17) years (under communication). In 1982–83, Gor Garang showed positive mass balance (Shankar, 2001) and three other glaciers (Nehnar, Tipra, and Changme Khangpu) showed less negative mass balance compared to the previous year (1982–83) (Gautam and Mukherjee, 1989; Sharma, 1999; Srivastava et al., 1999). Such phenomenon has been attributed by heavy winter snow fall in IHR (Raina and Srivastava, 2008). Three glaciers (Rulung, Kolahoi II, Shishram) of Ladak and Kashmir region (Dyurgerov and Meier, 2005) have only been studied for 1 year and shows negative mass balance. The measurements of mass balance of these regions are too short to infer the wide perspective of impact of climate change but there is clear evidences of influence of warming on the glacial health of the Himalayan region. In the Uttarakhand Himalaya three glaciers (Dunagiri, Dokriani and Chorabari) have been studied for 6 years and more (Geological Survey of India, 1992; Dobhal et al., 2008, 2013) and showed the negative mass balance as reported in other parts of IHR. Hamtah and Naradu glacier of western Himalayan region have been studied for 11 and 3 years respectively. Both the glaciers show negative mass balance (Koul and Ganjoo, 2010; Mishra et al., 2014; Shukla et al., 2015). The mass balance of Dokriyani glacier for the period of 6 years showed a negative trend. The observed reported reason was the less winter precipitation causes longer period exposure of the glacier surface ice for melting. The less precipitation during the winter season leads to the less input to the accumulation zone of the glacier. The mass balance data for Chhota Shigri glacier is one of the longest series available in the IHR (Wagnon et al., 2007). In IHR, till now Chhota Shigri is the only glacier for which reconstruction of the annual mass balance has been done. This reconstructed longer series mass balance data helped to create a better understanding about the glacier-climate relationship. The 43 years (1969–2012) of mass balance have been reconstructed by Azam et al. (2014) using required meteorological data from nearby Bhuntar observatory. The study showed that the specific annual mass balance is negative by 60% of the time and positive for rest of the time. The mass balance of Chhota Shigri glacier by using glaciological method (2002–2010) showed negative mass balance except for the years 2004/2005; 2008/2009; and 2009/2010 (Azam et al., 2012) with a marginal positive balance. Contrary to this, the Hamtah glacier (Chandra basin), exists in the same climatic zone, governed by the same climatic conditions and have almost same orientation (Table 2) showed negative mass balance for those years. The lower maximum elevation and the smaller accumulation area of Hamtah glacier, in comparison to the Chhota Shigri glacier, are the reported probable reason behind the difference in nature of the mass balances of the glaciers (Singh et al., 2016). In addition, thickness of the debris layer of ablation area of Hamtah glacier may play a major role in more melting of the glacier (Pratap et al., 2015). The orographic changes and the prevailing wind condition could also influence the behavior of the mass balance in the mountain region. In later year, Pandey et al. (2017) studied the topographic characteristics of both the glaciers to understand the role of topography in controlling the glacier response by using multi sensor and multi temporal remote sensing data. They reported that glacier hypsometry along with slope and aspect play an important role in glacier response. Like, more sensitivity toward the changes in the snowline due to the existence of the maximum area above the median elevation (Jiskoot et al., 2009), steeper slopes of the upper reaches of the glacier and more incoming solar radiation at the ablation zone due to very shallow slope make Hamtah glacier to melt more. In view of difficulty to access the glacier site to gather the mass balance data, Agrawal et al. (2017) used modeled data (TRMM and REMO) for the estimation of mass balance of Gangotri glacier for the period of 1985–2014. The estimated mass balance was −0.92 ± 0.36, −0.82 m w.e., and −0.98 ± 0.23 m w.e. a−1 for the period 2001–2014, 2006–2007, and 1985–2005, respectively.
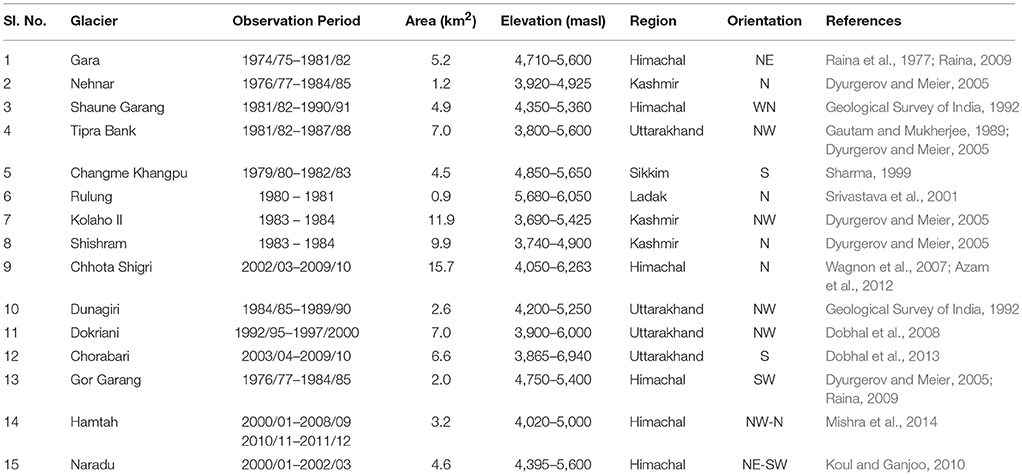
Table 2. Topographic details of the glaciers of IHR having the mass balance observation through glaciological method.
There is a need to estimate the mass balance of large number of glaciers to analyze the effect of climate change on the run-off of Himalayan Rivers. The glaciological method can provide the mass balance of only few glaciers as this method needs wide field investigations, and the field visits of rugged terrain of the Himalayas. For this purpose, Kulkarni et al. (2004), suggested the use of accumulation area ratio (AAR) method and developed a regression equation between AAR and mass balance.
Where, Y is the specific mass balance in m w.e. and X is the AAR.
The relationship was developed by using field mass balance data of the two glaciers of Baspa basin (Shaune Garang and Gor Garang). These field based mass balance data were taken from different reports of Geological Survey of India (Singh and Sangewar, 1989) whereas the glacier area was estimated by using LISS-III of Indian Remote Sensing Satellite (IRS). The developed model shows 0.50 AAR for zero mass balance in comparison to the AAR 0.70 of the Alps and Rocky Mountains. By using above regression equation, Kulkarni et al. (2004) studied the mass balance of 19 glaciers of the same Baspa basin during 2001 and 2002. The study suggested that all the studied glaciers of Baspa basin have negative balance because they are losing more snow and ice mass due to melting than the accumulation of seasonal snow. By using the above equation, 43 and 38 glaciers of Warwan and Bhut basins respectively have been studied by Brahmbhatt et al. (2012). The mean specific mass balance of Warwan basin was −0.19 m w.e., −0.27 m w.e. and −0.2 m w.e. for the years 2005, 2006, and 2007 respectively while the mean mass balance of Bhut basin was 0.05 m w.e., −0.11 m w.e. and −0.19 m w.e. for the years 2005, 2006 and 2007 respectively. During the monitoring period of 3 years, the total mass loss in Warwan and Bhut basins of western Himalaya was 4.3 and 0.83 km3 respectively. Similarly, Puri and Thakur (2015) studied the Chhota Shigri glacier for the period of 1997–2010. They developed a regional model by mapping the satellite images using two different techniques i.e., NDSI and band ration. They found that the AAR ranges from 0.3 to 0.7 and mass balance ranges in between −1.38 m w.e. and 0.284 m w.e. The field based and satellite derived mass balance showed good correlation. Some other glaciers were monitored by using geodetic method which enables the monitoring of more glaciers in a region at a single time and consequently avoids costly and time consuming field studies. Geodetic method is the best method to monitor those glaciers which are large in size, and difficult to visit, hence do not permit the estimation of mass through glaciological method. The geodetic method is based on the topographic change and the elevation of glacier. In this method, estimation of volume is defined as the difference of surface elevation of glacier at two different times (Meier and Tangborn, 1965; Krimmel, 1989; Bamber and Rivera, 2007; Cogley, 2009). This method achieves mass change through volume change when the surface density at the different parts of the glacier is known (Hagen and Reeh, 2003; Kaser et al., 2003; Hubbard and Glasser, 2005). This method fails to produce annual mass balance and is only useful when there is a need to estimate region-wide long-term mass balance (Berthier et al., 2007; Gardelle et al., 2013; Vincent et al., 2013; Vijay and Braun, 2016). Several studies strongly recommended the use of geodetic method so that the method-dependent uncertainties and associated systematic errors of glaciological mass balance measurement can be reduced (Zemp et al., 2009, 2013). By using geodetic method, Kumar et al. (2017), have estimated the mass balance of the glaciers of Baspa basin for 11 years (2000–2011). Out of total 89 glaciers present in the basin, 42 glaciers (area >1 km2) were chosen for the study. An average thinning of ~50 ± 11 m and average accumulation of ~35 ± 11 m were estimated between the periods of 2000–2011. In the span of 11 years the cumulative mass balance varies between −36.9 ± 1.98 m w.e. and 6.47 ± 1.98 m w.e. An average annual mass loss of −1.09 ± 0.32 m w.e. a−1 was estimated for the entire basin. The study reported that the glaciers of Baspa basin are losing mass at higher rate compared to the glaciers of eastern and central Himalayas. Another mass balance study over Chhota Shigri glacier has been conducted by Berthier et al. (2007). They found a good agreement between the mass balance estimated through field data (−1.13 m w.e. a−1 for 2002–2004) and by using satellite data (−1 to −1.1 m w.e. a−1 for 1999–2004). In this study a comparison of digital elevation model (DEM) of 2004 to the SRTM (Shuttle Radar Topographic Mission) topography of 2000 has been done to obtain the measurements. The used 2004 DEM was derived from two SPOT5 satellite optical images which did not have any ground control points. Kääb et al. (2012) conducted a study to show the mass wastage in the eastern, central and south-western parts of the Hindu Kush and Karakoram Himalayas during the period of 2003–2008. For the study, they use satellite laser altimetry and a global elevation model. The rate of the thinning of glacial mass was maximum (0.66 ± 0.09 m a−1) in the Jammu–Kashmir region. In contrast, the Karakoram glaciers showed only a few centimeters thinning per year. During the study period specific mass balance for entire study region was −0.21 ± 0.05 m w.e. a−1 (Kääb et al., 2012).
Conclusion
The mass balance of Indian Himalayan glacier is showing the influence of weather parameters especially temperature and precipitation. The regional summer temperature and winter precipitation are the major glacier mass balance governing factors whereas the role of other features like slope and aspect in glacier mass change cannot be ruled out. All the reported studies indicate about the warming trend in IHR at different rate depending on the region and season but there is a need of more studies to elaborate the reason behind the warming of IHR. A small number of temperature trend related studies of different parts of IHR arises restriction to give any comment with high confidence about the highest warming part of the region. The seasonal analysis shows that the warming was consistently higher in winter over different parts of Indian Himalayas. The higher winter warming has also been noted by several researchers in Tibetan Plateau. It is difficult to find any particular precipitation trend in IHR as few studies show the statistically not significant trend. The importance of temperature and precipitation in the analysis of impacts of climate change on glaciers tempted the researchers to find the future change in the parameters through modeling however these reported projections were found to be uncertain over the Himalayan Region because of its complex orography dependent climate regime.
The ice mass of the whole world—from polar to sub-polar region are decreasing and the mass balance studies are sparsely distributed. In general, the mass balance of IHR shows the negative trend except few positive balances for a year or two on Gara, Gor Garang and Shaune Garang of Baspa Basin and Chhota Shigri glacier of Chandra Basin. The positive values of observed mass balance are not very promising in comparison to the observed negative values. The study of glacier mass balance is of great importance because of its direct relation with the changing climate but the number of glaciers studied for mass balance is very few as compared to the total number of glaciers present in IHR. Hence, there is an urgent need to increase the number of regional mass balance study for better understanding about the impacts of climate change on glaciers. The Dokriani glacier of Garhwal Himalaya has the higher correlation coefficient with the precipitation evidenced by the less amount of precipitation causing negative mass balance. During the review we found that there are only few studies which correlate the precipitation with mass balance so it is advisable to pay more attention to these kind of studies. The study about the debris cover area and its role in glacier melting has been poorly covered in IHR whereas these probable reasons of mass change is receiving wide attention world-wide. Several researchers found that the model which does not include the impact of debris cover has higher uncertainty in mass loss prediction. These studies are essential for the glaciers of IHR to understand the different behavior of two nearby glaciers (like Hamtah and Chhota Shigri) of the same region. The use of other methods to estimate the mass balance are in pace as they give competitive results to the glaciological mass balance method.
Author Contributions
SS: conception of the article, in drafting of the article, updation of the article as per the suggestions; RK: make substantial contributions towards genesis and design of the article, review the draft; AD: review the draft of the article and suggestion for further updation of the article.
Conflict of Interest Statement
The authors declare that the research was conducted in the absence of any commercial or financial relationships that could be construed as a potential conflict of interest.
Acknowledgments
Authors would like to acknowledge the financial support in the form of research projects from Department of Science and Technology (DST), Govt. of India (grant no. SB/DGH-92/2014 dated 19.02.2015); Ministry of Earth Sciences (MoES) Govt. of India (grant no. MoES/PAMC/H&C/61/2015-PC-II dated 29.03.2016) United States Agency for International Development (USAID) for their funding through research projects. We would also like to thank to the researchers/authors who have immensely contributed to the topic through their findings. A special thanks to World Glacier Monitoring Services for the compilation of the huge data from all over the world and make them available for the researchers which gave detail overview about the global status of glacier mass balance. The criticism and suggestions by the reviewers and editor are greatly acknowledged that helped in making a better version of the paper.
References
Agrawal, A., Thayyen, R. J., and Dimri, A. P. (2017). Mass-balance modelling of Gangotri glacier. Geol. Soc. Lond. 462:SP462.1. doi: 10.1144/SP462.1
Azam, M. F., Wagnon, P., Ramanathan, A., Vincent, C., Sharma, P., Arnaud, Y., et al. (2012). From balance to imbalance: a shift in the dynamic behaviour of Chhota Shigri Glacier (Western Himalaya, India). J. Glaciol. 58, 315–324. doi: 10.3189/2012JoG11J123
Azam, M. F., Wagnon, P., Vincent, C., Ramanathan, A., Linda, A., and Singh, V. B. (2014). Reconstruction of the annual mass balance of Chhota Shigri glacier, Western Himalaya, India, since 1969. Ann. Glaciol. 55, 69–80. doi: 10.3189/2014AoG66A104
Bakalov, V. D., Groman, D. S., Zalikhanov, M., and Panov, V. D. (1990). Upravlenierezhimomgornikhlednikov I stokomrek. (Regime Control of Mountain Glaciers and Glacier Rivers Flow-Off). Leningrad: Hydrometeoizdat.
Bamber, J. L., and Rivera, A. (2007). A review of remote sensing methods for glacier mass balance determination. Glob. Planet Change 59, 138–148. doi: 10.1016/j.gloplacha.2006.11.031
Berthier, E., Arnaud, Y., Kumar, R., Ahmad, S., Wagnon, P., and Chevallier, P. (2007). Remote sensing estimates of glacier mass balances in the Himachal Pradesh (Western Himalaya, India). Remote Sens. Environ. 108, 327–338. doi: 10.1016/j.rse.2006.11.017
Bhutiyani, M. R., Kale, V. S., and Pawar, N. J. (2007). Long-term trends in maximum, minimum and mean annual air temperatures across the north western Himalaya during the twentieth century. Clim. Change 85, 159–177. doi: 10.1007/s10584-006-9196-1
Bhutiyani, M. R., Kale, V. S., and Pawar, N. J. (2010). Climate change and the precipitation variations in the northwestern himalaya: 1866–2006. Int. J. Climatol. 30, 535–548. doi: 10.1002/joc.1920
Bookhagen, B., and Burbank, D. W. (2010). Toward a complete Himalayan hydrological budget: spatiotemporal distribution of snowmelt and rainfall and their impact on river discharge. J. Geophys. Res. 115:F03019. doi: 10.1029/2009JF001426
Brahmbhatt, R. M., Bahuguna, I., Rathore, B. P., Kulkarni, A. V., Shah, R. D., Nainwal, H. C., et al. (2012). Variation of snowline and mass balance of glaciers of Warwan and Bhut Basins of Western Himalaya using remote sensing technique. J. Ind. Soc. Rem. Sens. 40, 629–637. doi: 10.1007/s12524-011-0186-z
Braithwaite, R. J. (2002). Glacier mass balance: the first 50 years of international monitoring. Prog. Phys. Geogr. 26, 76–95. doi: 10.1191/0309133302pp326ra
Casassa, G., Lopez, P., Pouyaud, B., and Escobar, F. (2009). Detection of changes in glacial run-off in alpine basins: examples from North America, the Alps, central Asia and the Andes. Hydrol. Process. 23, 31–41. doi: 10.1002/hyp.7194
Che, Y., Zhang, M., Li, Z., Li, H., Wang, S., Sun, M., et al. (2017). Glacier mass-balance and length variation observed in China during the periods 1959–2015 and 1930–2014. Quat. Int. 454, 68–84. doi: 10.1016/j.quaint.2017.07.003
Chen, J. L., Wilson, C. R., and Tapley, B. D. (2013). Contribution of ice sheet and mountain glacier melt to recent sea level rise. Nat. Geosci. 6, 549–552. doi: 10.1038/ngeo1829
Chitlangia, P. R. (1976). Mean model of western depression. Ind. J. Meteorol. Hydrol. Geophys. 7, 157–162.
Chou, C., and Neelin, J. D. (2003). Mechanisms limiting the northward extent of the northern summer monsoons over North America, Asia, and Africa. J. Clim. 16, 406–425. doi: 10.1175/1520-0442(2003)016 < 0406:MLTNEO>2.0.CO;2
Cogley, J. G. (2009). Geodetic and direct mass-balance measurements: comparison and joint analysis. Ann. Glaciol. 50, 96–100. doi: 10.3189/172756409787769744
Cogley, J. G. (2011). Present and future states of Himalaya and Karakoram glaciers. Ann. Glaciol. 52, 68–73. doi: 10.3189/172756411799096277
Dash, S. K., Jenamani, R. K., Kalsi, S. R., and Panda, S. K. (2007). Some evidence of climate change in twentieth-century india. Clim. Change J. 85, 299–321. doi: 10.1007/s10584-007-9305-9
Dimri, A. P., and Dash, S. K. (2012). Wintertime climatic trends in the western himalayas. Clim. Change J. 111, 775–800. doi: 10.1007/s10584-011-0201-y
Dimri, A. P., Kumar, D., Choudhary, A., and Maharana, P. (2018). Future changes over the Himalayas: mean temperature. Glob. Planet. Change. 162, 235–251. doi: 10.1016/j.gloplacha.2018.01.014
Dimri, A. P., Niyogi, D., Barros, A. P., Ridley, J., Mohanty, U. C., Yasunari, T., et al. (2015). Western disturbances: a review. Rev. Geophys. 53, 225–246. doi: 10.1002/2014RG000460
Diodato, N., Bellocchi, G., and Tartari, G. (2012). How do Himalayan areas respond to global warming? Int. J. Climatol. 32, 975–982. doi: 10.1002/joc.2340
Dobhal, D. P., Gergan, J. T., and Thayyen, R. J. (2008). Mass balance studies of the Dokriani Glacier from 1992 to 2000, Garhwal Himalaya, India. Bull. Glaciol. Res. 25, 9–17.
Dobhal, D. P., Mehta, M., and Srivastava, D. (2013). Influence of debris cover on terminus retreat and mass changes of Chorabari Glacier, Garhwal region, central Himalaya, India. J. Glaciol. 59, 961–971. doi: 10.3189/2013JoG12J180
Dyurgerov, M. B. (2001). Mountain glaciers at the end of the twentieth century: global analysis in relation to climate and water cycle. Polar Geogr. 25:241e338. doi: 10.1080/10889370109377717
Dyurgerov, M. B., and Meier, M. F. (1997). Mass balance of mountain and subpolar glaciers: a new global assessment for 1961–1990. Arctic Alpine Res. 29, 379–391. doi: 10.2307/1551986
Dyurgerov, M. B., and Meier, M. F. (2005). “Glaciers and the Changing Earth System: a 2004 snapshot,” in Colorado, Institute of Arctic and Alpine Research. University of Colorado, Occasional Paper, (Boulder, CO), 117.
Finkel, R. C., Owen, L. A., Barnard, P. L., and Caffee, M. W. (2003). Beryllium-10 dating of Mount Everest moraines indicates a strong monsoon influence and glacial synchroneity throughout the Himalaya. Geology 31, 561–564. doi: 10.1130/0091-7613(2003)031 < 0561:BDOMEM>2.0.CO;2
Fountain, A. G., and Tangborn, W. V. (1985). The effect of glaciers on stream flow variations. Water Resour. Res. 21, 579–586. doi: 10.1029/WR021i004p00579
Gardelle, J., Berthier, E., Arnaud, Y., and Kääb, A. (2013). Region-wide glacier mass balances over the Pamir-Karakoram-Himalaya during 1999–2011. Cryosphere 7, 1263–1286. doi: 10.5194/tc-7-1263-2013
Gautam, C. K., and Mukherjee, B. P. (1989). “Mass balance vis-a-vis snout position of Tiparabank glacier District Chamoli, Uttar Pradesh,” in Proceedings of National Meet on Himalayan Glaciology, 141–148.
Goswami, B. N., Shukla, J., Schneider, E. K., and Sud, Y. (1984). Study of the dynamics of the inter-tropical convergence zone with a symmetric version of the GLAS climatic model. J. Atmos. Sci. 41, 5–19. doi: 10.1175/1520-0469(1984)041 < 0005:SOTDOT>2.0.CO;2
Gregory, J. M., and Oerlemans, J. (1998). Simulated future sea-level rise due to glacier melt based on regionally and seasonally resolved temperature changes. Nature 391, 474–476. doi: 10.1038/35119
Geological Survey of India (1992). Geological Survey of India(GSI): Glaciological studies on Dunagiri Glacier, district Chamoli (Field Seasons 1983-84 to 1991-92), Final Report 1992 (Geological Survey of India), 5–9.
Guhathakurta, P., and Rajeevan, M. (2008). Trends in the rainfall pattern over India. Int. J. Climatol. 28, 1453–1469. doi: 10.1002/joc.1640
Hagen, J. O., and Reeh, N. (2003). “In situ measurement techniques: land ice,” in Mass Balance of the Cryosphere: Observation and Modelling of Contemporary and Future Changes, eds J. L. Bamber and A. J. Payne (Cambridge: Cambridge University Press), 11–42.
Hatwar, H. R., Yadav, B. P., and Rama Rao, Y. V. (2005). Prediction of western disturbances over Western Himalaya. Curr. Sci. 88, 913–920.
Held, I. M., and Hou, A. Y. (1980). Nonlinear axially symmetric circulations in a nearly inviscid atmosphere. J. Atmos. Sci. 37, 515–533. doi: 10.1175/1520-0469(1980)037 < 0515:NASCIA>2.0.CO;2
Hubbard, B., and Glasser, N. (2005). Field Techniques in Glaciology and Glacial Geomorphology. Chichester: Wiley.
Immerzeel, W. (2008). Historical trends and future predictions of climate variability in the brahmaputra basin. Int. J. Climatol. 28, 243–254. doi: 10.1002/joc.1528
IPCC (2013). “Summary for Policymakers,” in Climate Change 2013: The Physical Science Basis. Contribution of Working Group I to the Fifth Assessment Report of the Intergovernmental Panel on Climate Change, eds T. F. Stocker, D. Qin, G. K. Plattner, M. Tignor, S. K. Allen, J. Boschung, A. Nauels, Y. Xia, V. Bex, and P. M. Midgley (Cambridge; New York, NY: Cambridge University Press).
Jacob, T., Wahr, J., Pfeffer, W. T., and Swenson, S. (2012). Recent contributions of glaciers and ice caps to sea level rise. Nature 482, 514–518. doi: 10.1038/nature10847
Jhajharia, D., and and, Singh, V. P. (2011). Trends in temperature, diurnal temperature range and sunshine duration in Northeast India. Int. J. Climatol. 31, 1353–1367. doi: 10.1002/joc.2164
Jiskoot, H., Curran, C. J., Tessler, D. L., and Shenton, L. R. (2009). Changes in clemenceau icefield and chaba group glaciers, Canada, related to hypsometry, tributary detachment, length slope and area–aspect relations. Ann. Glaciol. 50, 133–143. doi: 10.3189/172756410790595796
Kääb, A., Berthier, E., Nuth, C., Gardelle, J., and Arnaud, Y. (2012). Contrasting patterns of early twenty-first-century glacier mass change in the Himalayas. Nature 488, 495–498. doi: 10.1038/nature11324
Kar, S. C., and Rana, S. (2014). Interannual variability of winter precipitation over northwest India and adjoining region: impact of global forcing's. Theor. Appl. Climatol. 116, 609–623. doi: 10.1007/s00704-013-0968-z
Kaser, G., Cogley, J. G., Dyurgerov, M. B., Meier, M. F., and Ohmura, A. (2006). Mass balance of glaciers and ice caps: consensus estimates for 1961–2004. Geopyhs. Res. Lett. 33:L19501. doi: 10.1029/2006GL027511
Kaser, G., and Fountain, A. (2003). A Manual for Monitoring the Mass Balance of Mountain Glaciers, International Hydrological Programme Technical Documents in Hydrology, No. 59. Paris: UNESCO.
Kaser, G., Grosshauser, M., and Marzeion, B. (2010). Contribution potential of glaciers to water availability in different climate regimes. Proc. Natl Acad. Sci. U.S.A. 107, 20223–20227. doi: 10.1073/pnas.1008162107
Koul, M. N., and Ganjoo, R. K. (2010). Impact of inter- and intra-annual variation in weather parameters on mass balance and equilibrium line altitude of Naradu Glacier (Himachal Pradesh), NW Himalaya, India. Clim. Change 99, 119–139. doi: 10.1007/s10584-009-9660-9
Krimmel, R. M. (1989). “Mass balance and volume of South Cascade Glacier, Washington, 1958-1985,” in Glacier Fluctuations and Climatic Change, ed J. Oerlemans (Boston, MA: Kluwer Academic Publishers), 193–206.
Kuhn, M. (1993). “Possible future contributions to sea level change from small glaciers”–Chapter 8 in Climate and Sea Level Change Observations, Projections and Implications, eds R. A. Warrick, E. M. Barrow, and T. M. L. Wigley (Cambridge: Cambridge University Press), 134–143.
Kulkarni, A. V., Rathore, B. P., and Suja, A. (2004). Monitoring of glacial mass balance in the Baspa basin using accumulation-area ratio method. Curr. Sci. 86, 101–106.
Kumar, G. V., Kulkarni, A. V., Gupta, A. K., and Sharma, P. (2017). Mass balance estimation using geodetic method for glaciers in Baspa basin, Western Himalaya. Curr. Sci. 113, 486–492. doi: 10.18520/cs/v113/i03/486-492
Kumar, R., Singh, S., Randhawa, S. S., Singh, K. K., and Rana, J. C. (2014). Temperature trend analysis in the glacier region of Naradu Valley, Himachal Himalaya, India. Comptes Rendus Geosci. 346, 213–222. doi: 10.1016/j.crte.2014.09.001
Lemke, P., Ren, J., Alley, R. B., Allison, I., Carrasco, J., Flato, G., et al. (2007). “Observations: changes in snow, ice and frozen ground,” in Climate Change 2007: The Physical Science Basis. Contribution of Working Group I to the Fourth Assessment Report of the Intergovernmental Panel on Climate Change, eds S. Solomon, D. Qin, M. Manning, Z. Chen, M. Marquis, K. B. Averyt, M. Tignor, and H. L. Miller (Cambridge; New York, NY: Cambridge University Press).
Loschnigg, J., and Webster, P. J. (2000). A coupled ocean-atmosphere system of SST regulation for the Indian Ocean. J. Climatol. 13, 3342–3360. doi: 10.1175/1520-0442(2000)013 < 3342:ACOASO>2.0.CO;2
Meier, M. F. (1984). Contribution of small glaciers to global sea level. Science 226, 1418–1421. doi: 10.1126/science.226.4681.1418
Meier, M. F., and Tangborn, W. V. (1961). “Distinctive characteristics of glacier runoff,” in U.S. Geological Survey Professional Paper 424-B, P14–B16.
Meier, M. F., and Tangborn, W. V. (1965). Net budget and flow of South cascade glacier, Washington. J. Glaciol. 5, 547–566. doi: 10.1017/S0022143000018608
Mishra, R., Kumar, A., and Singh, D. (2014). Long term monitoring of mass balance of Hamtah Glacier, Lahaul and Spiti district, Himachal Pradesh. Geol. Surv. India 147(Pt 8), 230–231.
Mohanty, U. C., Madan, O. P., and Raju, P. V. S. (1998). Meteorological Fields Associated With Western Disturbances in Relation to Glacier Basins of Western Himalayas During Winter Season. Technical Report, Centre for Atmospheric Science, IIT Delhi, India.
Mukherjee, B. P., and Sangewar, C. V. (1996). “Correlation of accumulation area ratio and equilibrium-line altitude with the mass balance of Gara, Gor Garang and Shaune Garang Glaciers of Himachal Pradesh,” in Proceeding of the Symposium NW Himalaya and Fore Deep GSI Special Publication, 303–305.
Neelin, J. D., and Held, I. M. (1987). Modeling tropical convergence based on the moist static energy budget. Monthly Weather Rev. 115, 3–12. doi: 10.1175/1520-0493(1987)115 < 0003:MTCBOT>2.0.CO;2
Oerlemans, J. (2007). Estimating response times of Vadret da Morteratsch, Vadret da Palu, Briksdalsbreen and Nigardsbreen from their length records. J. Glaciol. 53, 357–362. doi: 10.3189/002214307783258387
Oerlemans, J., and Fortuin, J. P. (1992). Sensitivity of glaciers and small ice caps to greenhouse warming. Science 258, 115–117. doi: 10.1126/science.258.5079.115
Pandey, P., Nawaz Ali, S., Ramanathan, A. L., Champati raya, P. K., and Venkataram, G. (2017). Regional representation of glaciers in Chandra Basin region, western Himalaya, India. Geosci. Front. 8, 841–850. doi: 10.1016/j.gsf.2016.06.006
Pisharoty, P., and Desai, B. N. (1956). Western disturbance and indian weather. Ind. J. Meteor. Hydrol. Geophys. 7, 333–338.
Pratap, B., Dobhal, D. P., Mehta, M., and Bhambri, R. (2015). Influence of debris cover and altitude on glacier surface melting: a case study on Dokriani Glacier, central Himalaya, India. Ann. Glaciol. 56, 9–16. doi: 10.3189/2015AoG70A971
Puri, M., and Thakur, P. K. (2015). Remote sensing based accumulation area ratio method for glacier mass balance of Chhota Shigri Glacier. Int. J. Adv. Res. Sci. Eng. 4, 171–184.
Raina, V. K. (2009). A State-of-Art Review of Glacial Studies, Glacial Retreat and Climate Change. Discussion Paper, MOEF, New Delhi. Available online at: http://www.moef.nic.in/sites/default/files/MoEF%20Discussion%20Paper%20_him.pdf
Raina, V. K., Kaul, M. K., and Singh, S. (1977). Mass-balance studies of Gara glacier. J Glaciol. 18, 415–423. doi: 10.1017/S0022143000021092
Raina, V. K., and Srivastava, D. (2008). Glacier Atlas of India. Bangalore: Geological Society of India.
Sangewar, C. V., and Shukla, S.P. (eds). (2009). Inventory of the Himalayan Glaciers-A Contribution to International Hydrological Programme. Special Publication No. 34, Geological Survey of India.
Sanjay, J., Krishnan, R., Shrestha, A. B., Rajbhandari, R., and Yu Ren, G. (2017). Downscaled climate change projections for the Hindu Kush Himalayan region using CORDEX South Asia regional climate models. Adv. Clim. Change Res. 8, 185–198. doi: 10.1016/j.accre.2017.08.003
Scheel, M., Frey, H., and Bolch, T. (2011). Report on the Current Distribution of Glaciers in the Hindu Kush–Himalayan Region. Highnoon Seventh Framework Programme, Technical Report. No. 1.9.
Schneider, T., Bischoff, T., and Haug, G. H. (2014). Migrations and dynamics of the intertropical convergence zone. Nature 513, 45–53. doi: 10.1038/nature13636
Shankar, R. (2001). “Glaciological studies in India; contribution from Geological survey of India,” in Proceeding of Symposium on Snow, Ice and Glacier, March 1999 (Geological Survey of India Special), 11–15.
Shanker, R., and Srivastava, D. (2001). “Glaciated Regime Environmental Interaction and Himalayan Ecosystem,” in Proceedings on Snow Ice (Lucknow: Geological Survey of India), 17–21.
Sharma, S. (1999). Mass Balance Studies of Changme Khangpu Glacier, Mangan District, Sikkim. Symposium on Snow, Ice and Glacier.
Sherpa, S. F., Wagnon, P., Brun, F., Berthier, E., Vincent, C., Lejeune, Y., et al. (2017). Contrasted surface mass balances of debris-free glaciers observed between the southern and the inner parts of the Everest region (2007–15). J. Glaciol. 63, 637–651. doi: 10.1017/jog.2017.30
Shrestha, A. B., Agrawal, N. K., Alfthan, B., Bajracharya, S. R., Maréchal, J., and van Oort, B. (2015). The Himalayan Climate and Water Atlas: Impact of Climate Change on Water Resources in Five of Asia's Major River Basins. ICIMOD, GRID-Arendal and CICERO.
Shukla, S. P., Mishra, R., and Chitranshi, A. (2015). Dynamics of Hamtah Glacier, Lahaul & Spiti district, Himachal Pradesh. Ind. Goephys. Union 19, 414–421.
Singh, P., Kumar, V., Thomas, T., and Arora, M. (2008). Basin-wide assessment of temperature trends in northwest and central India / Estimation par bassin versant de tendances de température au nord-ouest et au centre de l'Inde. Hydrol. Sci. J. 53, 421–433. doi: 10.1623/hysj.53.2.421
Singh, R. K., and Sangewar, C. V. (1989). “Mass balance variation and its impact on glacier flow movement at Shaune Garang Glacier, Kinnaur, Himachal Pradesh,” in Proceedings National Meet on Himalayan Glaciology (New Delhi: Department of Science and Technology), 149–152.
Singh, S., Kumar, R., Bhardwaj, A., Sam, L., Shekhar, M., Singh, A., et al. (2016).Changing climate and glacio-hydrology in Indian Himalayan Region: a review. WIREs Clim Change 7, 393–410. doi: 10.1002/wcc.393
Sontakke, N. A., Singh, H. N., and Singh, N. (2009). Monitoring physiographic rainfall variation for sustainable management of water bodies in India,” in Natural and Anthropogenic Disasters: Vulnerability, Preparedness and Mitigation, ed M. K. Jha (Springer), 293–331.
Srivastava, D., Sangewar, C. V., Kaul, M. K., and Jamwal, K. S. (2001). “Mass balance of Rulung glacier – A Trans-Himalayan glacier, Indus basin, Ladakh, of Symposium on snow, ice and glacier, March 1999, Abstract,” in Geological Survey of India Special, 41–46.
Srivastava, D., Singh, R. K., Bajpai, I. P., and Roy, A. J. K. (1999). “Mass balance of Neh Nar Glacier, District Anantang, J&K,” in Proceedings of the Symposium on Snow, Ice and Glaciers–a Himalayan perspective.
Tiwaril, P. R., Kar, S. C., Mohanty, U. C., Dey, S., Sinha, P., and Shekhar, M. S. (2017). Sensitivity of the Himalayan orography representation in simulation of winter precipitation using Regional Climate Model (RegCM) nested in a GCM. Clim. Dyn. 49, 4157–4170.
Vijay, S., and Braun, M. (2016). Elevation change rates of glaciers in the Lahaul–Spiti (western Himalaya, India) during 2000–2012 and 2012–2013. Remote Sens. 8, 1–16. doi: 10.3390/rs8121038
Vincent, C., Ramanathan, A. L., Wagnon, P. Dobhal, D. P., Linda, A., Berthier, E., et al. (2013). Balanced conditions or slight mass gain of glaciers in the Lahaul and Spiti region (northern India, Himalaya) during the nineties preceded recent mass loss. Cryosphere 7, 569–582. doi: 10.5194/tc-7-569-2013
Vohra, C. P. (1996). “Himalayan glacier research in India,” in Proceedings 1st Working Group Meeting of Himalayan Glaciology (Kathmandu).
Wagnon, P., Linda, A., Arnaud, Y., Kumar, R., Sharma, P., Vincent, C., et al. (2007). Four years of mass balance on Chhota Shigri Glacier, Himachal Pradesh, India, a new benchmark glacier in the western Himalaya. J. Glaciol. 53, 603–611. doi: 10.3189/002214307784409306
Webster, P. J., Magana, V. O., Palmer, T. N., Shukla, J., Tomas, R. A., Yanai, M., et al. (1998). Monsoons: processes, predictability, and the prospects for prediction. J. Geophys. Res. 103, 14451–14510. doi: 10.1029/97JC02719
World Glacier Monitoring Service (2017). Global Glacier Change Bulletin No., 2 (2014–2015), eds M. Zemp, S. U. Nussbaumer, I. GärtnerRoer, J. Huber, H. Machguth, F. Paul, and M. Hoelzle (Zurich: ICSU(WDS)/IUGG(IACS)/UNEP/ UNESCO/WMO, World Glacier Monitoring Service).
Wulf, H., Bookhagen, B., and Scherler, D. (2010). Seasonal precipitation gradients and their impact on fluvial sediment flux in the Northwest Himalaya. Geomorphology 118, 13–21. doi: 10.1016/j.geomorph.2009.12.003
Yadav, R. K., Kumar, K. R., and Rajeevan, M. (2009). Out of phase relationship between convection over northwest India and warm pool region during winter seasons. Int. J. Climatol. 29, 1330–1338. doi: 10.1002/joc.1783
Yang, S., Zhang, Z., Kousky, V. E., Higgins, R. W., Yoo, S. H., Liang, J., et al. (2008). Simulations and seasonal prediction of the Asian summer monsoon in the NCEP climate forecast system. J. Clim. 21, 3755–3775. doi: 10.1175/2008JCLI1961.1
Zemp, M., Hoelzle, M., and Haeberli, W. (2009). Six decades of glacier mass balance observations: a review of the worldwide monitoring network. Ann. Glaciol. 50, 101–111. doi: 10.3189/172756409787769591
Keywords: glacier mass balance, temperature trend, precipitation trend, Indian Himalayan Region, climate change
Citation: Singh S, Kumar R and Dimri AP (2018) Mass Balance Status of Indian Himalayan Glaciers: A Brief Review. Front. Environ. Sci. 6:30. doi: 10.3389/fenvs.2018.00030
Received: 28 February 2018; Accepted: 03 May 2018;
Published: 21 August 2018.
Edited by:
Yuhua Duan, National Energy Technology Laboratory (DOE), United StatesReviewed by:
Brenda Cecilia Alcántar-Vázquez, Universidad Nacional Autónoma de México, MexicoXander Wang, University of Louisiana at Lafayette, United States
Keling Zhang, Cristal, United States
Copyright © 2018 Singh, Kumar and Dimri. This is an open-access article distributed under the terms of the Creative Commons Attribution License (CC BY). The use, distribution or reproduction in other forums is permitted, provided the original author(s) and the copyright owner(s) are credited and that the original publication in this journal is cited, in accordance with accepted academic practice. No use, distribution or reproduction is permitted which does not comply with these terms.
*Correspondence: Rajesh Kumar, cmFqZXNoa3JzaC5pbkBnbWFpbC5jb20=