- 1College of Agriculture and Natural Resources, Delaware State University, Dover, DE, United States
- 2College of Earth, Ocean, and Environment, University of Delaware, Lewes, DE, United States
- 3Biology Department, Whitman College, Walla Walla, WA, United States
- 4Center for Integrated Biological and Environmental Research, Delaware State University, Dover, DE, United States
Diatoms are strongly influenced by water quality and serve as indicators of water quality degradation in freshwater systems. Here, sediment and water samples were collected from four sites in Blackbird Creek, DE, a salt marsh characterized as mostly freshwater to low saline brackish (<8ppt). Recent changes in land use resulted in increased agricultural activity, suggesting the need to develop water quality indicators in this region. To test the hypothesis that diatom community composition changes seasonally with variations in water quality parameters, sediment and water samples were collected in 2009 and 2010 for analysis. Water temperature, salinity, pH, and dissolved oxygen were measured as well as water and sediment dissolved nutrient concentrations (nitrate, ammonia, and total and reactive phosphorous). DNA was extracted from sediments and changes in diatom community composition were evaluated by amplification of 18S rRNA gene using diatom-specific primers, followed by Terminal Restriction Fragment Length Polymorphism (TRFLP) analysis. Shannon (H') index for TRFLP profiles ranged from 2.5 to 3.0 and Simpson (Ds) index was 0.9 which infers moderate levels of diatom species richness and high diversity in these study sites. Although there were no water quality parameters that were significantly correlated with diatom community composition as determined by TRFLP patterns, temperature was the most highly correlated (r = 0.203). Dissolved oxygen, salinity, and pH of water also had moderate but insignificant impacts on the diatom community. Further analysis of cloned 18S rRNA sequences revealed the presence of diatom taxa that tolerate wide salinity ranges, and included Navicula, Cyclotella, Thalassiosira and Skeletonema. Entomoneis sp. were also present in the spring and fall seasons. Overall, results in this study demonstrate significant differences in water qualities among the study years but little change in diatom community composition between study sites and seasons, but may serve as a baseline for future studies.
Introduction
Diatoms are microscopic algae that are ubiquitous in aquatic ecosystems. They have been used to assess the ecosystem health and environmental conditions of rivers and streams since the early 1900's. The United States Environmental Protection Agency (USEPA) and other agencies have widely accepted diatoms as indicators of aquatic stressors (Valentine, 2002). Diatom species in a particular area depend on the environment and its water chemistry (Lee, 2012), also different diatom taxa have distinct ecological requirements and tolerances to water quality parameters (Reavie et al., 2014; Lobo et al., 2016). Even though there are some studies focusing on diatoms in freshwater and coastal habitats, our knowledge is very limited on how environmental conditions in tidal wetlands influence the compositions of diatom communities.
Diatoms are an important part of the aquatic food webs in both marine and fresh water systems. They are key components of the benthic micro algal communities (Byers, 2000; Goldfinch and Carman, 2000; Armitage and Fong, 2004b). As the structure of tidal wetlands differs from rivers and streams, the distribution, and abundance of diatom communities in these ecosystems is largely influenced by their environmental gradients (Weilhoefer et al., 2015). Diatoms play important roles in nutrient cycling and transformation in the water column and sediment (Sundbäck et al., 1991; Bartoli et al., 2003). Nutrient cycling is of great significance as it maintains ecosystem health and influences the survival of other organisms within that ecosystem (Neogi et al., 2014). Aquatic nutrient cycles are vulnerable to human activities and global weather changes that may alter nutrient inputs (Meunier and Boersma, 2017). Changes in aquatic nutrient compositions can shift ecosystems food chain dynamics and may lead to drastic conditions like algal blooms and fish kills (Verspagen et al., 2014). Diatoms have been found to be correlated with changing water quality conditions. Increased abundances of Nitzschia bacillum Hustedt, Nitzchia constricta (Kützing) Ralfs and Stephanodiscus were reported from eutrophic systems of Mongolia, which don't seem to occur in nutrient-poor systems (Shinneman et al., 2009). Moreover, results from a saltmarsh restoration project in North Carolina show that the diatom communities from a pristine marsh and the restored area became similar over the period of time (Zheng and Zhang, 2004). This infers that diatom composition shifts in response to improved water quality conditions. Also, studies in north-west Canada and the European Alpine lakes reflect that climate warming has shifted diatom communities and over represented Cyclotella sp. (Ruhland et al., 2003a,b; Karst-Riddoch et al., 2005).
This research was conducted in Blackbird Creek tidal wetlands of Delaware to monitor the land use impacts (agricultural, residential and recreational activities) and understand diatom community structure within different sites. This watershed was made up of 39% agricultural land, 22% forestland, 25% wetland, 4% open water, and only 10% is residential (DNREC/DNERR, 1999). However, a more recent study by Stone et al. (2016) reported increased agricultural activities in the wetland to 46%. In 1993, Delaware National Estuarine Research Reserve (DNERR) was established as the 22nd National Estuarine Research Reserve System and Blackbird creek became a part of DNERR. Water quality analysis indicated that this creek experienced low dissolved oxygen and high levels of nitrogen, phosphorous and chlorophyll levels and thus proposed total maximum daily load TMDL levels were developed through various models for Blackbird Creek (Delaware Department of Natural Resources Environmental Control, 2006). The development of diatoms as indicators of water quality in this system may provide additional information or verification of improved vs. degraded sites over time.
Terminal Restriction Fragment Length Polymorphism (TRFLP) is a molecular technique for studying microbial diversity, and has been successfully applied for environmental samples in recent decades (Blackwood et al., 2003). This is a rapid and highly reproducible method that often yields larger number of operational taxonomic units (OTUs) (Thies, 2007). The application of TRFLP to evaluate changes in diatom community composition was demonstrated by Lee (2012). The advantage of TRFLP is that it not only detects members of both culturable and non-culturable microbial populations, but also identifies them based on genetic differences, which has been considered superior to the traditional taxonomic identification by microscopic observation of phenotypic traits.
Until now there is limited information on diatom community compositions and their seasonal variations in Delaware salt marshes, particularly in Blackbird creek, which has been a part of the DNERR but subjected to increasing anthropogenic pressures in several locations. The objectives of this study were to observe diatom species diversity, using TRFLP in parallel with traditional genetic (cloning) and taxonomic methods, to evaluate spatio-temporal changes in diatom community composition along a salinity gradient of the Blackbird Creek, Delaware. We tested the hypothesis that composition of diatom communities will vary with location (upstream or downstream) and in response to seasonal or environmental changes in this estuarine ecosystem. The study sites selected were also close to a major highway, suggesting the potential for habitat alteration. An analysis of the diatom community along with water quality will provide a better understanding of habitat health for Blackbird Creek and a baseline for future monitoring efforts in this system.
Materials and Methods
Study Sites
Blackbird Creek is a tidal wetland with minor to moderate levels of anthropogenic activities in Delaware (Biohabitats Inc., 2007). It is one of the National Estuarine Research Reserves (NERR) in the country and one of two in the state. It is located at the Delaware Bay/River Estuary which enters the Delaware River about 9 km upstream and is characterized by tidal rivers or creeks that extend across the wetlands (Coyne, 2010). Selection of study sites in the Blackbird Creek was based on information of water quality from a previous study (Biohabitats Inc., 2007). Four study sites (Figure 1) including both upstream (sites 1, 2, 3) and downstream (site 4) locations were chosen. Site 1 is a Kayak ramp (7.97 km from the bay); Site 2 is the area by the bridge (7.85 km from the bay); site 3 is close to the residential area (7.72 km from the bay) and site 4, is a boat ramp (2.56 km from the bay) which is close to the mouth of the creek where it flows into the bay/river. In estuaries, tides greatly affect the circulation of sediments and mixing of water will affect water quality parameters such as salinity, turbidity, pH, DO, and Chlorophyll-a (Fortune and Mauraud, 2015). At the sample collection sites, water mixing was much higher at sites 1 and 4 than at sites 2 and 3.
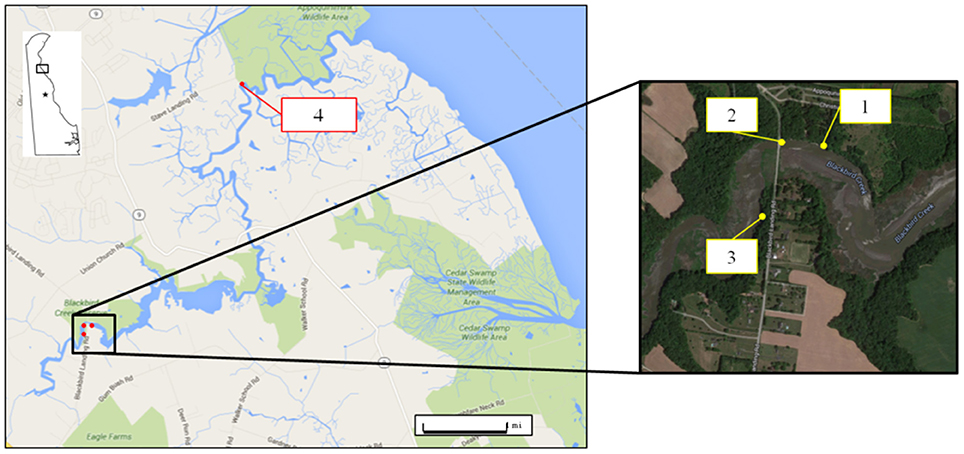
Figure 1. Geographical location of the sample collection points from the upstream (sites 1, 2, 3) and downstream (site 4) sites in Blackbird Creek, Delaware.
Sample Collection
Water was collected in 500 mL amber polyethylene sample bottles and sediment samples were collected using a custom-made core sampler (Portune, 2008) in triplicates biweekly from May through November in 2009 and 2010. Temperature (°C), salinity (ppt), dissolved oxygen (mg/L), and pH of water samples were recorded in the field using a handheld YSI 556 Multiprobe meter (YSI Inc., Yellow Springs, OH). Water samples were collected during low tide (<1.5 ft) at depths varying from 0.5 to 1 m. The highest tide recorded in this creek during the sampling period was nearly 1.1 m. Samples were collected mostly when it was a clear day but in October, 2009 there was heavy and steady rain during the sampling time. Although seasonal averages vary from 1 year to another depending on the atmospheric temperature and precipitation, average pattern for Blackbird Creek mimic typical Mid-Atlantic Estuaries. The conditions were normally wet in May and early June, dry in late June, July and early August and start getting wet again in late August and September. It was rainy and very wet in October and November; there were two major hurricanes in June 2010 (NOAA National Centers for Environmental Information State of the Climate, 2018).
Microscopic examination of the diatom communities in the collected water and sediment samples were determined by observing phenotypic traits of plankton under a compound microscope. Processed dry diatom samples were examined by Scanning Electron Microscopy (SEM) (Ewing and Nater, 2003). Soil samples were preserved in ethanol to make a final concentration of 20% (Taylor et al., 2007), and the slides for Light Microscopy (LM) were prepared according to Round et al. (1990) (see Krammer and Lange-Beralot, 1986–1991). For LM, the samples were treated by hot HCl and KMnO4, and a series of digestions were carried out with H2O2 treatment. The samples were cooled, diluted and 500 microliters of the diluted sample were examined under 400 × magnification. Microscopic examination of wet sediments for diatoms was performed in the lab using inverted microscope. For SEM, images of diatom samples were obtained at the University of Delaware after they were prepared from dehydrated samples.
Nutrient Analysis of Water and Sediment Samples
Sediment samples (100–150 g) were centrifuged at 24,000 g for 20 min to separate pore water (water adhered to the soil particles), which was then filtered through a 0.45 μm nylon filter. Pore water samples (50 ml) were transported on ice to University of Delaware Soil Testing Laboratory (Newark, DE) where all analyses were performed. Ammonia (NH3-N) and nitrate () were analyzed by Bran & Luebbe Auto Analyzer 3 (Bran & Luebbe, Buffalo Grove, IL). Total nitrogen (TN), total bound nitrogen (TBN) (ASM International, 2008), and total phosphorous (TP) were measured colorimeterically using a modified Murphy-Riley method (Murphy and Riley, 1962) using Sequoia-Turner Spectrophotometer 340 (Sequoia-Turner Corporation, Mountain View, CA). Total carbon (TC) was measured by direct combustion (APHA, 2005).
Water samples were filtered through a GF/F glass fiber filter (Whatman, Vernon Hills, IL) and analyzed using HACH company (Loveland, CO) protocols and equipment. Total phosphorus (TP), soluble reactive phosphorus (Orthophosphate- ), nitrate () and ammonia (NH3-N) were measured following HACH method nos. 8190, 8048, 8171, and 8155, respectively, with detection ranges of 0.0–3.5, 0.02–2.5, 0.1–10, 0.01–0.5, respectively (https://www.hach.com/dr-4000-u-spectrophotometer-115-vac/product-downloads?id=7640447364). Alkalinity was measured with a YSI 9100 photometer measuring levels between 0 and 500 mg L−1 CaCO3 (Yellow Springs, Ohio).
DNA Extraction and Analysis
Sediment (0.25 g) samples preserved in RNALater (Sigma-Aldrich, St.Louis, MO) were washed with 2% (w/v) marine phosphate buffered saline (0.02% (w/v) NaCl, 0.115% (w/v) KCl, 0.02% (w/v) Na2HPO4∙2H2O) to remove salts. DNA was then extracted using the MO Bio Power Soil DNA Extraction Kit (MO BIO Laboratories, Carlsbad, CA). Primers (STRAM 9F (5′-CTGCCAGTAGTCATACGCTC-3′) labeled at the 5′ end with 6-hexachloro-fluorescein (HEX) and EUK 517R (5′-GACGGGGGACCAGACTTGCCCTC-3′) labeled at the 5′end with 6-fluorescein (FAM) were designed to amplify the 18S rDNA sequence of the diatoms Lee (2012)). PCR was performed according to the methods described by Lee (2012) and a PCR product of 550 bp was obtained.
Terminal Restriction Fragment Length Polymorphism (TRFLP) Analysis
The amplified PCR products were digested with HaeIII and MspI (New England BioLabs, Ipswich, MA) in separate 10 μL reactions (Lee, 2012). Reactions were incubated for 4–6 h at 37°C and 1 μL of the restriction digest was combined with 18 μL HiDi formamide (Applied Biosystems) and 1 μL TAMRA 500 size standard (Applied Biosystems, Foster city, CA) for fragment size analysis. HEX and FAM-labeled terminal restriction fragments were differentiated size-wise (Restriction fragment lengths) on ABI Prism 310 Genetic Analyzer (Applied Biosystems) using Gene Scan v.3.1 software (Applied Biosystems). Results were then analyzed with Peak Scanner v.1.0 software (Applied Biosystems). The fragment peaks between 20 and 500 bases in length were binned at a width of 1 nucleotide, the proportions of individual peak heights within each sample were analyzed statistically (Rees et al., 2004).
Cloning and Sequencing
PCR products of sediment DNA samples were cloned using TOPO TA Cloning Kit (Invitrogen, Carlsbad, CA). About 10 clones from each sample were cultured into Luria broth tubes with 50 μg/mL of kanamycin and incubated overnight at 37°C, with shaking at 200 rpm. Plasmid DNA of the bacterial clones was isolated using the alkaline lysis method (Sambrook and Russell, 2001) and checked for the target sequence by conventional PCR (STRAM 9F and EUK 517R primers). Only positive clones that were amplified by diatom primers were sequenced using Sanger's method at University of Delaware Sequencing and Genotyping Center. Sequences were further analyzed by Zymo Research Inc., using Quantitative insights into microbial ecology (QIIME) (Caporaso et al., 2010) v. 1.8.0 with the 18S rRNA gene database as a reference. Using the QIIME software, Operational Taxonomic Units (OTU) were picked based on the 97% similarity of the 18S rDNA sequences and diatom community abundance was generated. Taxonomic identities were assigned using the Ribosomal Database Project (RDP). The sequences were also searched with BLASTn tool (Altschul et al., 1990; Johnson et al., 2008). OTUs were deposited in GenBank (Accession # KU708273 to KU708312).
Phylogenetic and Diversity Analysis
Clone sequences were aligned with closely related diatom taxa using Geneious v.4.8.5 (Biomatters Ltd., Auckland, NZ) for phylogenetic analysis. Two Raphidophyte sequences from the GenBank with accession numbers DQ191680.1 and DQ191681.1 were used as outgroups. Highly divergent regions in the alignment were then removed using GBlocks (Castresana, 2000; Talavera and Castresana, 2007) to yield 528 informative sites for phylogenetic analysis. Resulting sequences were analyzed using Bayesian inference implemented on MRBAYES, version 3.1.2 (Huelsenbeck and Ronquist, 2001; Ronquist and Huelsenbeck, 2003). Phylogenetic analysis was conducted by running 300,000 generations in four chains, using default temperatures and saving every 10 th tree. Trees were constructed using a GTR+I+G DNA substitution model (Waddell and Steel, 1997), taking into account a gamma-shaped variation in substitution rates across sites. The log-likelihood score of each tree was plotted against the number of generations to determine the point where log-likelihood scores became stationary (burn-in). The first 17,000 sampled trees (the burn-in) were discarded and a consensus tree was constructed from the remaining trees. Diatom species diversity was estimated using the Shannon Diversity Index (H') and Simpson's Diversity Index (SDI) from the TRFLP profiles (Shannon and Weaver, 1949; Simpson, 1949; Pielou, 1966) in Microsoft Excel.
Statistical Analysis
The data for TRFLP standardized peak heights were merged and imported into the multivariate statistical software package, PRIMER 6 (Primer-E Ltd., Plymouth, UK). The data were square root transformed to down weight the contribution of dominant species before calculating the Bray-Curtis similarity matrix (Bray and Curtis, 1957; Clarke, 1993). Non-metric multidimensional scaling (NMDS) (Kruskal, 1964; Kruskal and Wish, 1978) ordination techniques were used to visualize relationships between diatom communities at the sites. The raw data from sediment nutrients and water quality were normalized before performing Analysis of Similarities (ANOSIM) and Principal Component Analysis (PCA). ANOSIM was used to identify statistical differences in water quality and diatom community structure between the study sites and seasons. PCA was used to identify environmental variables that had influenced water and sediment quality of the study sites. NMDS plots based on Euclidean distances were used to visualize the changes in water quality and sediment nutrients at the study sites. A BIO-Env Stepwise Test (BEST) was performed to understand what water quality parameters can best explain the diatom community compositions. In this analysis the Bray-Curtis resemblance matrix of the square-root transformed terminal restriction fragment peak heights (diatom community) and the normalized data of water quality (environmental variables) were used. Spearman's correlation was used to measure the strength of association between the resemblance matrices of the water quality and diatom communities and results with P ≤ 0.05 are considered significant. Correlation values (r) generated will be between 1 and −1 indicating positive and negative relationships. Literature suggests that the responses of diatom communities to environmental changes may be determined using multivariate analyses (Blinn and Herbst, 2003; Potapova and Charles, 2007; Lee, 2012).
Results
Water Quality
Water quality at the study sites during the years 2009 and 2010 was determined by measuring physicochemical parameters as shown in Table 1.
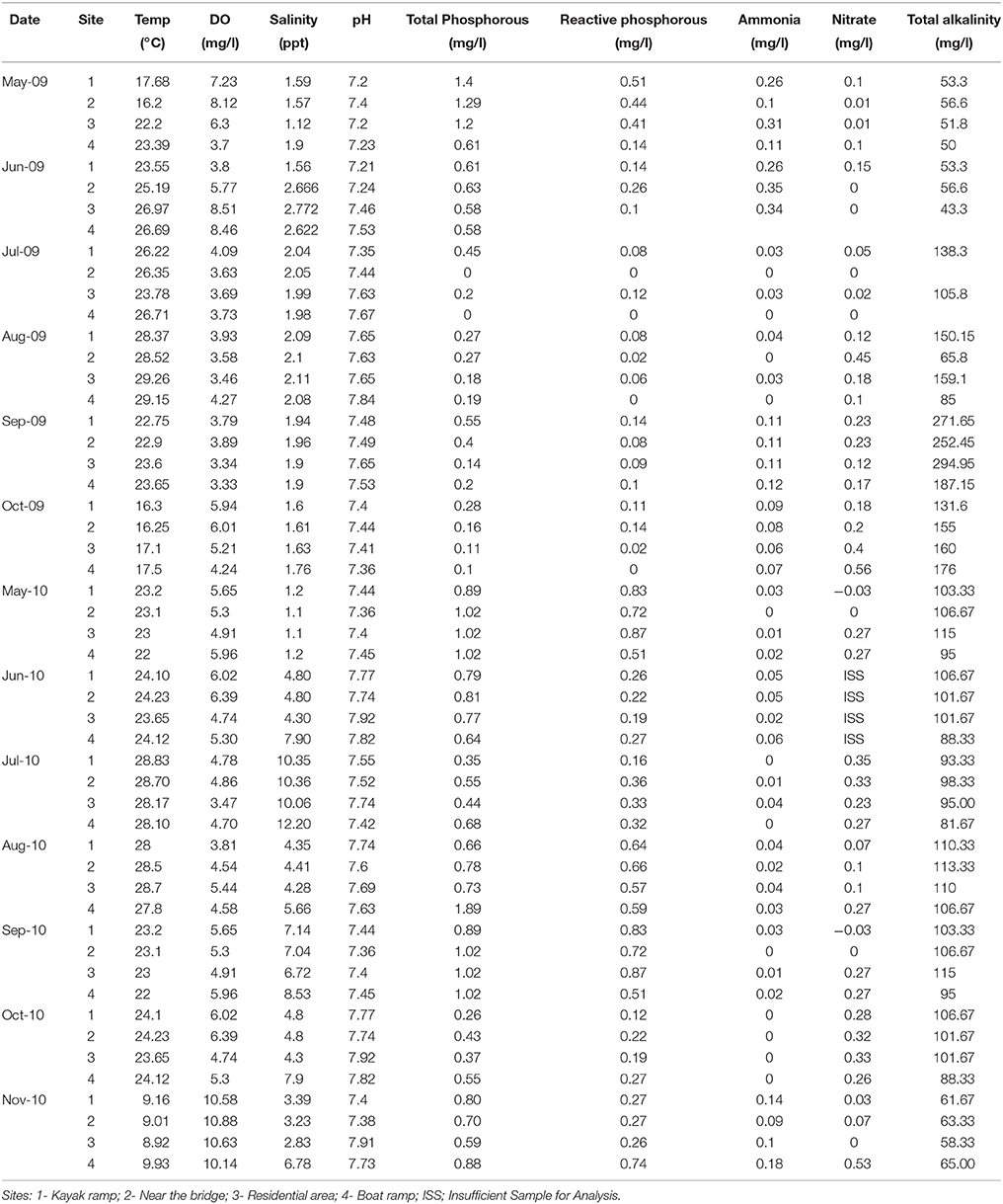
Table 1. Physicochemical parameters of the water samples at the study sites during different sampling periods of the years 2009 and 2010.
Box plots (Figures 2, 3) were generated to provide a clear representation of the water quality for the 2009 vs. 2010 at each site. Temperatures in 2010 were more highly varied than 2009 as 25% of the samples ranged from 9.1 to 20°C (Figure 2). Dissolved Oxygen (DO) levels were higher in 2010, especially for the upstream sites. Low DO levels (3.4–6.39 mg/L) were observed during the warmer months such as May, June and July (Table 1). Salinity levels were lower (1.2–2.7 ppt) in 2009 when compared to 2010 (1.1–12.2 ppt). Alkalinity levels varied widely during 2009, reaching 294 mg/L, with less variability and generally lower alkalinity during 2010. Soluble reactive phosphorous and total phosphorous levels ranged from 0.1 to 0.87 and 0.1 to 1.4 mg/L, respectively, and were higher in 2010 compared to 2009. Total phosphorous levels at site 4 during August 2010 were higher (~1.9 mg/L) than all other sampling times in year 2009 (<1.4 mg/L). Ammonia levels in the waters from both study years were less than 0.35 mg/L (Figure 3). Even though ammonia levels were within the expected range for aquatic systems, they were, on average, higher in 2009 than 2010. Nitrate levels in water samples varied widely at all the sites for 2009 and 2010, and ranged from 0 to 0.56 mg/L.
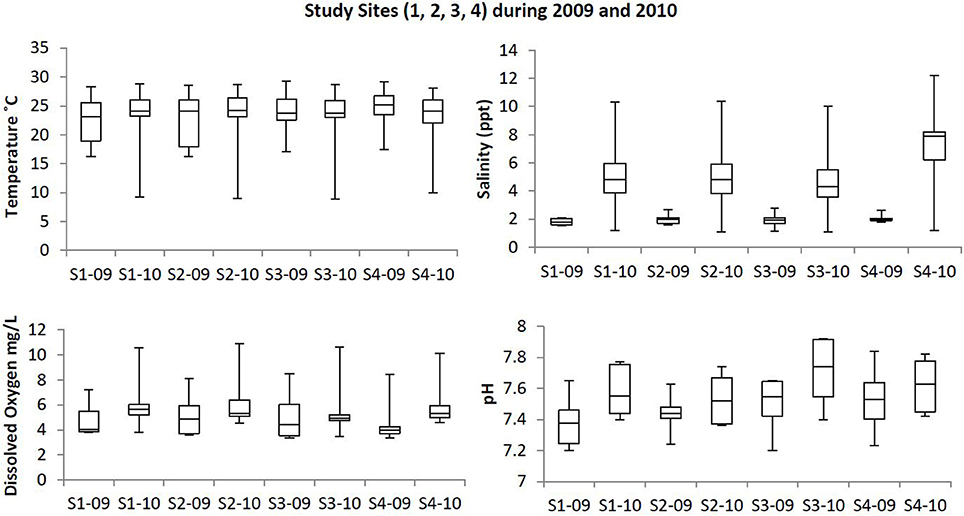
Figure 2. Box plots showing the trends at the study sites for temperature, dissolved oxygen, salinity, and pH in the upstream and downstream sites during 2009 and 2010. Study sites within the images are labeled as S1-09 (Site 1, 2009); S1-10 (Site 1, 2010); S2-09 (Site 2, 2009); S2-10 (Site 2, 2010); S3-09 (Site 3, 2009); S3-10 (Site 3, 2010); S4-09 (Site 4, 2009); S4-10 (Site 4, 2010).
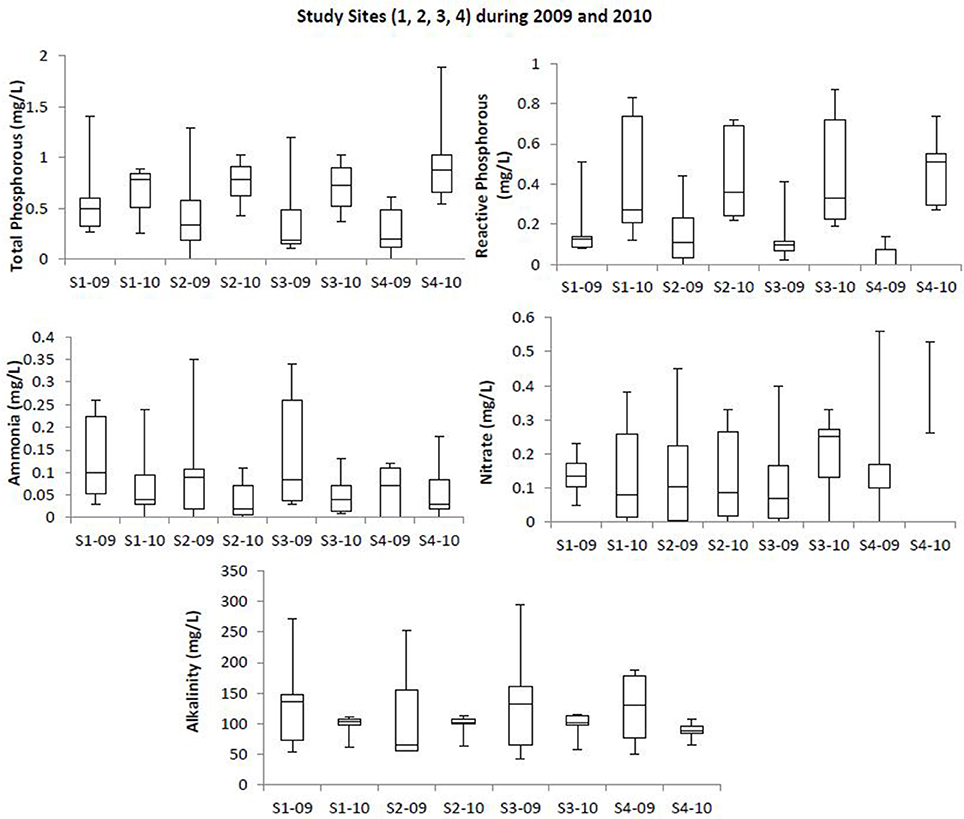
Figure 3. Box plot showing trends at the study sites for total phosphorous, reactive phosphorous, ammonia, and alkalinity profiles at the upstream and downstream sites of Blackbird Creek watershed during 2009 and 2010. Study sites within the images are labeled as S1-09 (Site 1, 2009); S1-10 (Site 1, 2010); S2-09 (Site 2, 2009); S2-10 (Site 2, 2010); S3-09 (Site 3, 2009); S3-10 (Site 3, 2010); S4-09 (Site 4, 2009); S4-10 (Site 4, 2010).
ANOSIM analysis between the study sites across the sampling seasons showed that there were no significant differences in the water quality between the study sites across seasons in 2009 and 2010 (R = −0.067; P = 0.97). Pairwise tests between the study sites as shown in Table 2 also indicate that there were no significant differences between them as P-values were greater than 0.05. But overall, the water quality between the years, 2009 and 2010 has some differences which can be considered as statistically significant (R = 0.232, P = 0.001).
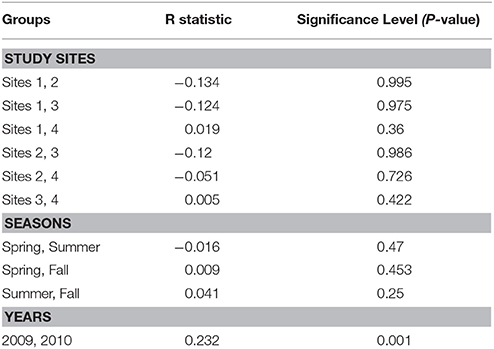
Table 2. Pairwise tests for differences in water quality between the study sites, seasons, and years.
Principal Component Analysis (PCA) plot (Figure 4) explains 50% of the variation in water quality between sites where total alkalinity, temperature, nitrate, pH, salinity (explained by PC1), total phosphorous and reactive phosphorous, (explained by PC2) were the variables positively impacting the water quality. Within the PCA plot (Figure 4), study sites 1 through 4 were shown as the water quality data was plotted for the study years 2009 and 2010. Similarities were observed in the total alkalinity levels for the months July, August, September and October of 2009. In 2010, differences were observed in salinity, total alkalinity and ammonia levels; salinity was high in July and September whereas total alkalinity was high in June and October. Ammonia was high in November 2010 when compared to May.
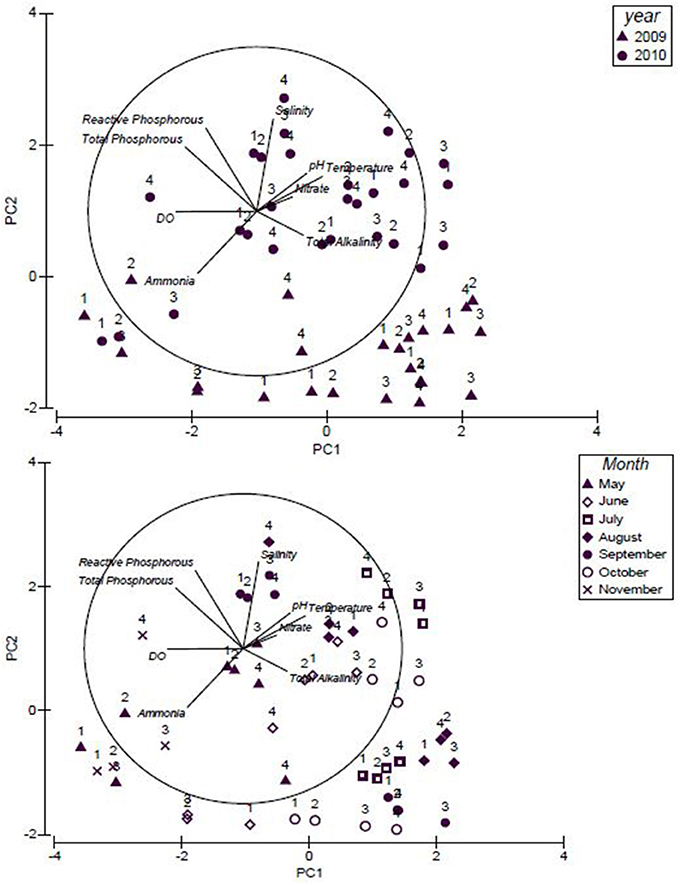
Figure 4. Principal Component Analysis (PCA) plot for the water quality of the upstream and downstream sites for the study years, 2009 and 2010.
Sediment nutrient qualities were strongly influenced by total nitrogen and ammonia, while total phosphorous, total carbon and total bound nitrogen were the weak contributors (Figure 5). Upstream sites displayed greater levels of ammonia and total bound nitrogen than the downstream sites of this tidal wetland. Contrastingly, the levels of nitrogen species in water samples were low. These analyses predict that water and soil nutrient quality were greatly impacted by the seasons and extreme weather events like heavy rainfall.
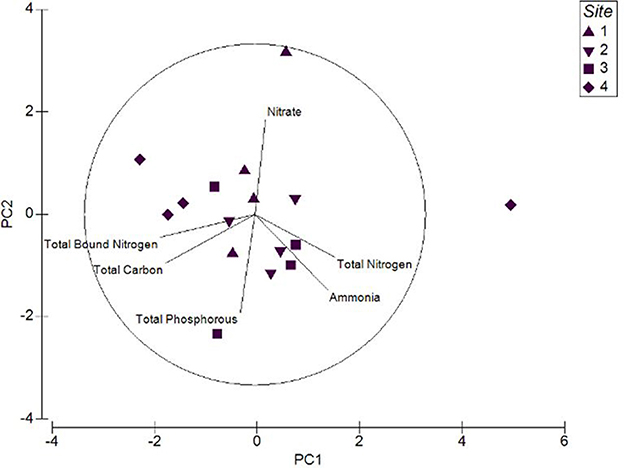
Figure 5. Principal Component Analysis (PCA) plot for the sediment nutrient quality of the upstream and downstream sites.
Diatom Community Analysis Using TRFLP Profiles
TRFLP analysis is based on the differences in the position of restriction sites and the length of the TRFs (Liu et al., 1997). In TRFLP analysis, the height and area of the TRF peaks represent the relative abundances of groups of microorganisms; similar microorganisms are shared by similar TRF peaks. These TRFLP profiles were used to differentiate and compare the diatom communities present in the soils of upstream and downstream sites. TRF peaks with fluorescence units of 72 and greater (~2000) were considered for statistical analysis. The strength of this method includes speed and high sample throughput, on which statistical analysis can be performed to differentiate the microbial communities (Blackwood et al., 2003). Multivariate analysis (NMDS) of the TRFLP profiles generates the relative occurrence of each diatom species (TRFs) which was performed based on Bray-Curtis (Blinn and Herbst, 2003) values. The diatom TRFs were clustered into three groups based on the differences in their TRFLP profiles (Figure 6), of the 44 samples analyzed 38 samples were clustered as one big group which consists of samples from all four sites and all seasons. This can mean that a higher percentage of the diatom TRFs analyzed share a greater level of similarity at their genus level. But the remaining 6 samples might be dissimilar to some extent as they were clustered into 2 different groups. All these 6 TRF samples belong to fall and spring seasons collected from the study sites boat ramp, residential site and the site near the bridge. Cluster analysis did not show any site or month-specific domination of particular diatom groups or species. The differences in TRF patterns were not specific to the study sites and the study months, while these differences might be caused due to other environmental conditions.
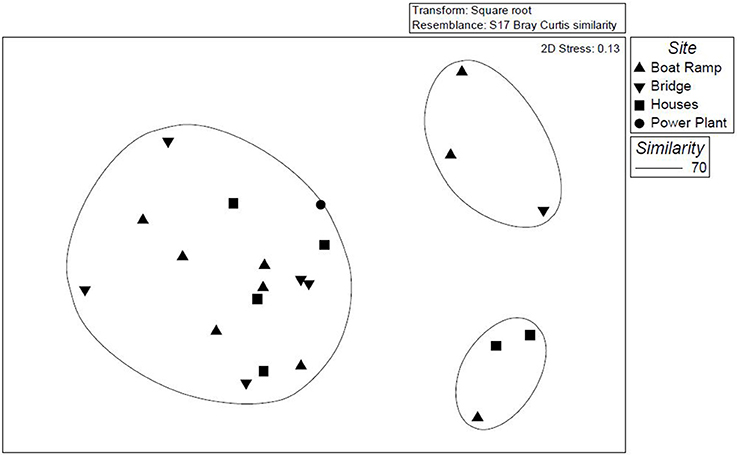
Figure 6. NMDS plot showing relationships between diatom community compositions for each sampling month during 2009 and 2010.
It has been reported that the number of different-sized TRFs provide an estimate of the minimum number of species present in the diatom community (Dunbar et al., 2001). Increased numbers of TRFs were observed in the sediment samples at upstream sites (Table 2) than the downstream site. This may imply that diatom diversity was greater in the upstream sites specifically at site 1. Eleven common TRF patterns (70, 71, 72, 75, 76, 312, 325, 326, 470, 471, and 473 bp) were consistently present in all study sites and all months throughout the study period, suggesting that a core diatom community was present in all soil samples. We also observed some TRF peaks (56, 272, 357, 458, 468 bp and so on) that were only specific to some samples within the study sites, and are referred as unique TRF fragments (Table 3). Shannon index (H') values for the diatom community based on the number of TRF peaks at the study sites were greater than 2.5 and less than 3.0, which represents diverse diatom communities within this creek. In TRFLP analysis 2 peaks were generated for each species or OTU (one end is HEX labeled and the other is FAM labeled) and for 2 restriction digests (Hae III and MspI). This means technically there were 4 TRFs for each species or OTU and since each TRF may represent more than 1 species the true diversity may differ from the Shannon diversity index.
Simpson Diversity index (Ds) was calculated to test if two randomly sampled individuals belong to different species. The Simpson diversity values (Ds) usually range from 0 and 1, if Ds values are higher the diversity will be high and vice versa. At all the study sites, Simpson indices for the TRFLP profiles were greater than or equal to 0.9.
Diatom Community Analysis Using Cloning and Sequencing
Sequences with ≥98% match to the reference sequences from the NCBI database were used for phylogenetic analysis. Non-diatom sequences were removed from the analysis. Phylogenetic tree was constructed (Figure 7); two Raphidophytes [Chattonella antiqua (Y.Hada) C.Ono and Heterosigma akashiwo (Y.Hada)] were used as outgroups. Highly similar sequences were related to the diatom taxa Cyclotella, Thalassiosira, Skeletonema, and Navicula. The presence and absence of the OTUs from each site were provided in the Table 4 along with NCBI accession numbers for their nearest matches.
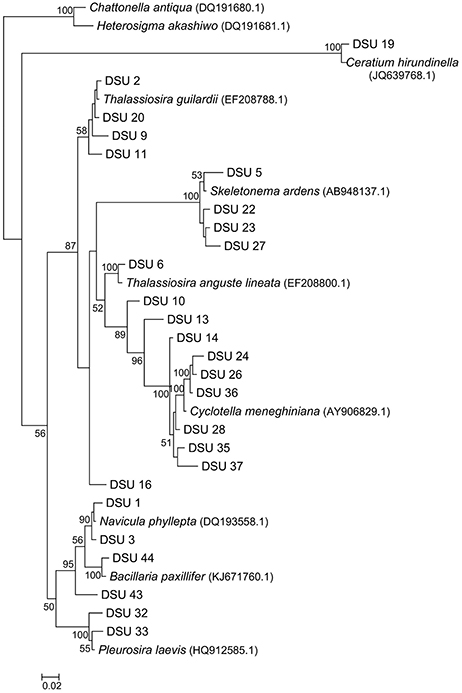
Figure 7. Neighbor joining tree inferred from 18S rDNA diatom sequences. Boot strap values are only shown for nodes on the main trunk of the tree; the bar represents 0.02 nucleotide substitution per position.
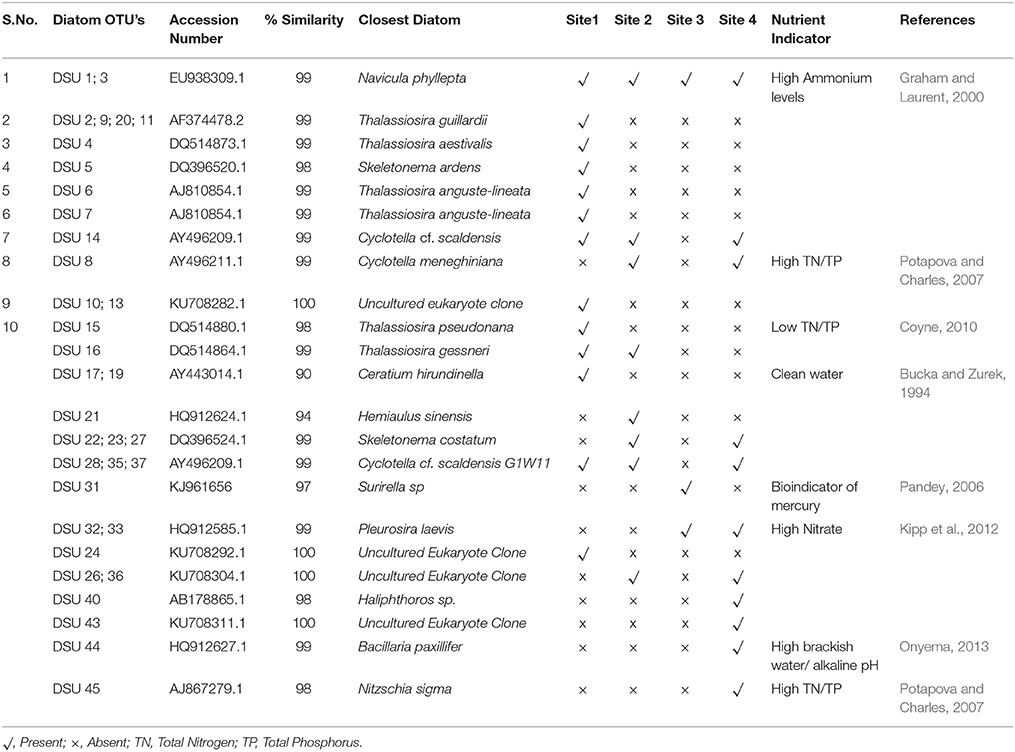
Table 4. Identification of diatom Operational Taxonomic Units (OTU'S) from the study sites, accession numbers, and similarity percentages of closely matched diatom species from the Genbank database has been displayed next to the OTU's.
Sixty two percent of the diatom sequences isolated from this tidal creek belong to Cyclotella menighiniana Kützing, Thalassiosira guilardii Hasle and Skeletonema ardens Sarno & Zingone, these are centric diatoms from the family Coscindiscophyceae (Stoermer and Julius, 2003). Microscopic images of diatoms from the sediment samples of this tidal creek were shown in Figure 8.
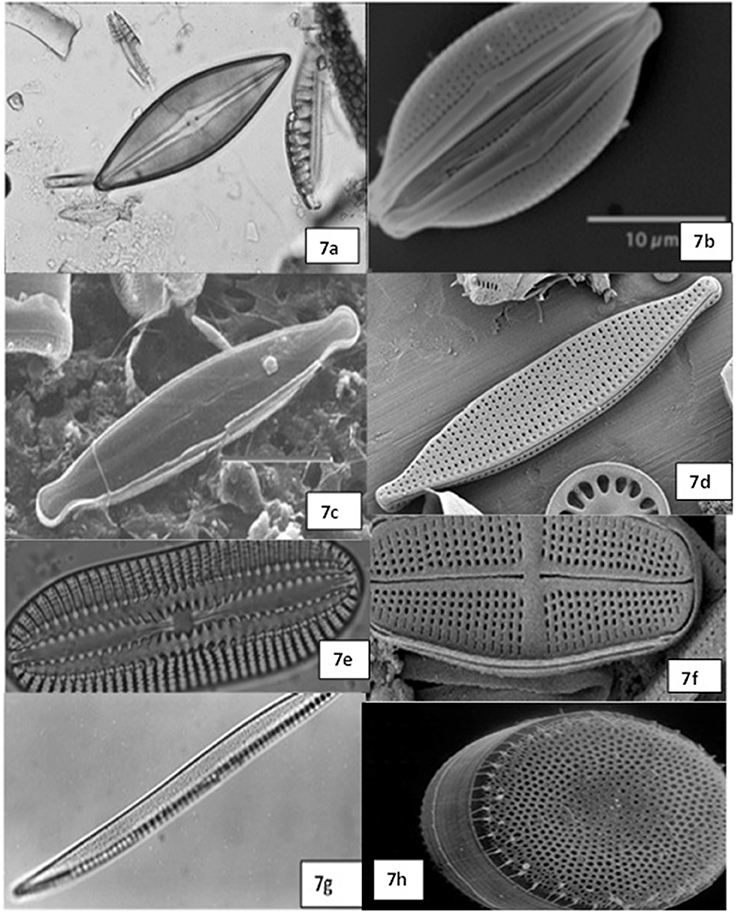
Figure 8. Microscopic images of the diatoms observed in the Blackbird Creek soil samples. 7a–6f, Navicula sp.; 7d, Portion of Cyclotella sp.; 7e,g, Nitzschia sp.; 7h, Thalassiosira sp.
Skeletonema species are known as one of the most widely occurring genera of coastal marine diatoms (Zingone et al., 2005; Liu et al., 2012). This genus was found primarily in estuaries (Spaulding and Edlund, 2008) with a large number of species (Sarno et al., 2005). Sequences from sites 2 and 3 were high in diatom matches pertaining to Cyclotella meneghiniana Kützing and Pleurosira laevis (Ehrenberg) Compère. Ceratium, a dinoflagellate was also observed at the study sites and was mostly in high numbers at site 1.
Relationship of Water Quality With Diatom Community Analysis
BEST analysis results showed that temperature was correlated with the diatom community composition; on diatom community composition; we saw shifts in the diatom composition during the study months (especially May vs. October). The Spearman correlation (r) for temperature was 0.203 with a P-value of 0.71 and df = 2, which shows a weak positive relation between temperature and the diatom community. Other variables, such as dissolved oxygen, pH, soluble reactive phosphorous and total alkalinity also resulted in weak positive relations with an r-value of 0.202; df = 2 and a P-value of 0.61. Similarly, nutrient variables like ammonia (r = 0.084; df = 1), total phosphorous (r = 0.040; df = 1), and soluble reactive phosphorous (r = 0.040; df = 1), did not have significant positive correlations on the diatom community compositions (P > 0.05). None of these water quality variables showed significant relationships as their P-values were greater than 0.05, but it can be interpreted that temperature is the only variable that was highly correlated with the diatom community composition in this tidal creek.
Discussion
There have been very few studies comparing diatoms in Delaware tidal wetlands. TRFLP profiles of site 3 (area close to a residential site) had considerably fewer peaks and were consistent between the months and years. The six diatom TRF'S that formed a separate group in Figure 5 matched with the diatom OTU's such as Entomoneis ornate (Bailey) Reimer from site 1, Entomoneis cf. alata. from site 3, and Entomoneis sp. and other unidentified sequences from site 2. Blackbird Creek is a low saline brackish to freshwater tidal creek in Delaware, and the diatom groups observed in this study were mostly freshwater species or species that tolerate wide salinity ranges (Cyclotella, Navicula, Hemialus, Skeletonema, Thalassiosira, and Pleurosira). Of all the diatom taxa observed in this creek, Thalassiosira, Cyclotella, and Skeletonema are the most abundant groups. Marshall et al. (2005) reported that the diatom taxa Cyclotella, Thalassiosira, Skeletonema, and Navicula were dominant in the tidal estuaries of Chesapeake Bay. Previous studies reported species like Cyclotella, Skeletonema, and Thalassiosira act as indicators of high eutrophication and high salinity (Weckstrom and Juggins, 2005). Cyclotella group contains species that survive in both marine and freshwaters (Alverson et al., 2011) while Pleurosira laevis (Ehrenberg) Compère was recorded to thrive in high nitrogen conditions and act as an indicator of poor water quality (Smucker and Vis, 2011; Kipp et al., 2012). Lee (2012) reported that diatom communities in the Delaware Inland Bays were influenced by some environmental variables such as DO, total organic carbon and sediment chlorophyll. The occurrences of diatom communities in this wetland were partly influenced by sites rather than seasons and years in our study. Not only species identification and confirmation microscopically, but also detailed and quantitative microscopic analysis would have further confirm our molecular findings. Diatom communities were diverse and abundant from May through October in Blackbird Creek. Previous research showed that the number of diatom species and their diversity decreased with increased nutrient concentrations and salinity levels (Blinn and Bailey, 2001; Lionard et al., 2005; Putland and Iverson, 2007). It has been reported that diatoms have strong correlations to environmental characteristics and can also respond rapidly to environmental changes (McCorkle, 2000; Potapova and Charles, 2007; Martin and Fernandez, 2012). Desianti et al. (2017), reported that the nutrients from the water column and sediment did not impact the diatom community composition but the organic matter in sediments greatly influences the diatom species in New Jersey coastal lagoons.
Water quality in Blackbird Creek was consistent throughout the study sites, most of the parameters followed predictable seasonal patterns. Dissolved oxygen is also an important variable in estuaries and decreased levels will impact the ecological balance of estuaries (Zheng and Zhang, 2004). Low DO levels can be observed in the summer months of 2009 due to increased water temperatures and turbidity (Wetzel, 2001). According to the state of Delaware surface water quality standards (Delaware Department of Natural Resources Environmental Control, 2004), DO in marine waters must not be less than 5 mg/L with a minimum of 4 mg/L for short exposure as it becomes detrimental to aquatic life. The pH levels at all the sites were mostly within their ranges, and these results are in agreement with HydroQual Inc. (2006). Study sites closer to the residential areas had slightly higher pH values. Studies indicate that shifts in pH levels in the aquatic systems can impact the diatom species number and diversity (Patrick et al., 1968). A pH range of 6.0–9.0 is optimal for living organisms in the aquatic systems (Oram, 2014b). According to the Blackbird Creek TMDL Analysis (Delaware Department of Natural Resources Environmental Control, 2006), the threshold value for total phosphorous is 0.2 mg/L. Concentrations for total phosphorous in water samples ranged between 0.1 and 0.63 mg/L during 2009 with exceptions in the month of May 2009, and between 0.26 and 1.02 mg/L during 2010, exceeding the threshold value in both years. Increased nutrient levels indicate that the ecosystem conditions are being altered and can enhance the growth of tolerant diatoms that are being used as indicators of pollution (Coyne, 2010; Lee, 2012; Martin and Fernandez, 2012). Concentrations ranging from 0.53 to 22.8 mg/L were considered toxic for freshwater species (Oram, 2014a). Total phosphorous, soluble reactive phosphorous, and nitrate levels were high in September and October, possibly due to the frequent rainfalls in the fall. Reports indicated that the total precipitation levels in October 2009 for Delaware were above normal (NOAA National Centers for Environmental Information, 2016) while National Weather Service Report (2010) indicates heavy rain fall with 15.24–20.32 cm rain causing floods in Delaware from September 30th to October 1st in 2010. Technical report by the state of Delaware (2010) stated that the source of contamination in Blackbird Creek is non-point source mainly and an average DO is 4.5 mg/L in the creek (Rogerson et al., 2010).
Based on the correlation analysis between diatom community composition and water quality, temperature was highly correlated with the diatom community composition than other parameters measured. Studies by Weilhoefer et al. (2015), reported that the occurrences of diatom communities in tidal wetlands are sensitive to the environmental changes in the wetland and its topography. According to Blinn and Herbst (2003), the environmental quality of an ecosystem determines the growth of specific groups of diatoms occurring in that ecosystem. Sullivan (1975) conducted studies on diatom communities in Delaware salt marsh and reported that differences between the communities were closely related to differences in temperature and elevation between the habitats. Further microscopic and molecular analysis with increased number of diatom sequences will help to identify water quality parameters that may have an effect on diatom community composition. Inclusion of more downstream study sites is also needed to get a better picture of how diatom communities are distributed among the sites.
Conclusion
There are not many studies on diatom community compositions in Delaware salt marshes. Results from our study indicated that diatom communities in this tidal wetland were mostly influenced by temperature. In addition, there were no major differences in the diatom community (TRF) compositions among the study sites, but temporal variations in their species diversity were observed for the study sites. Water nutrient quality data suggests that increased nutrient loads, especially under extreme weather events along with increased anthropogenic runoff, may be related to higher abundance of particular diatom species that can tolerate nutrient-rich conditions at locations close to residential areas. Overall, our findings of insignificant differences in the environmental quality and diatom community compositions of the study sites during most of the sampling periods, indicates that these benthic communities are relatively tolerant to environmental changes. Although indicator species for environmental quality were not identified, our study provides important baseline data on the diatom community composition in Delaware salt marshes. Future research may focus on land use impacts by comparing agricultural, residential and control sites (marsh grass) using studies designed to address the limitations identified here.
Author Contributions
LC was involved with planning, designing, performing the research, data analysis, training students with molecular analyses and preparation of the manuscript. KC was responsible for planning the research and training the students and staff on molecular analyses. AP initiated this study and was responsible for sample collection, water quality analysis, and soil DNA extraction. KL trained the students on DNA extraction and designed the TRFLP assay. CD was involved with cloning and sequence analysis. VK provided assistance with molecular analysis. GO is the project director and was responsible for planning and directing the research activities, site selection, field sample collection, microscopic examination, and training students with sampling and analyses.
Conflict of Interest Statement
The authors declare that the research was conducted in the absence of any commercial or financial relationships that could be construed as a potential conflict of interest.
Acknowledgments
We would like to thank the following individuals for their review of the manuscript: Dr. Gary P. Richards and Mrs. Mollee Dworkin. We would like to thank the DNERR scientists, Dr. Robert Scarborough, Mrs. Kimberly Cole, and Mr. Michael Mensinger for their support with study site selection and sample collection. This work was supported by NOAA ECSC under Grant Award NA11SEC4810001; USDA-NIFA under Grant Award 2010-38821-21456; Delaware Sea Grant Program (NOAA Grant NA09OAR4170070, project R/BT2 to KC) and by NSF-EPSCoR under Grant Award EPS-1301765.
References
Altschul, S. F., Gish, W., Miller, W., Myers, E. W., and Lipman, D. J. (1990). Basic local alignment search tool. J. Mol. Biol. 215, 403–410. doi: 10.1016/S0022-2836(05)80360-2
Alverson, A. J., Beszteri, B., Juius, M. L., and Theriot, E. C. E. (2011). The model marine diatom Thalassiosira pseudonana likely descended from a freshwater ancestor in the genus Cyclotella. BMC Evol. Biol. 11:125. doi: 10.1186/1471-2148-11-125
APHA (2005). “Total organic carbon (TOC): high temperature combustion method (5310 A and 5310 B),” in Standard Methods for the Examination of Water and Wastewater, 21st Edn, eds A. D. Eaton, L. S. Clesceri, E. W. Rice, A. E. Greenberg, and M. A. H. Franson (Maryland, NY: American Public Health Association).
Armitage, A. R., and Fong, P. (2004b). Upward cascading effects of nutrients: shifts in a benthic micro algal community and a negative herbivore response. Oecologia 139, 560–567. doi: 10.1007/s00442-004-1530-6
ASM International (2008). Designation: D5176-08, Standard Test Method for the Total Chemically Bound Nitrogen in Water by Pyrolysis and Chemiluminescence Detection.
Bartoli, M., Nizzoli, D., and Viaroli, P. (2003). Microphytobenthos activity and fluxes at the sediment–water interface: interactions and spatial variability. Aqu. Ecol. 37, 341–349. doi: 10.1023/B:AECO.0000007040.43077.5f
Biohabitats Inc. (2007). Blackbird Creek Reserve, Ecological Restoration Master Plan. Baltimore, MD.
Blackwood, C. B., Marsh, T., Kim, S. H., and Paul, E. A. (2003). Terminal restriction fragment length polymorphism data analysis for quantitative comparison of microbial communities. Appl. Environ. Microbiol. 69, 926–932. doi: 10.1128/AEM.69.2.926-932.2003
Blinn, D. W., and Bailey, P. C. E. (2001). Land-use influence on stream water quality and diatom communities in Victoria, Australia: a response to secondary salinization. Hydrobiologia 466, 231–244. doi: 10.1023/A:1014541029984
Blinn, D. W., and Herbst, D. B. (2003). Use of Diatoms and Soft Algae as Indicators of Environ Determinants in the Lahontan Basin, USA. Annual Report For California State Water Resources Board Contract Agreement.
Bray, R. J., and Curtis, J. T. (1957). An ordination of the upland forest communities of southern Wisconsin. Ecol. Monogr. 27, 325–349. doi: 10.2307/1942268
Bucka, H., and Zurek, R. (1994). Trophic relations between phyto- and zooplankton in a field experiment in the aspect of the formation and decline of water blooms. Acta Hydrobiol. 34, 139–155.
Byers, J. E. (2000). Competition between two estuarine snails: implications for invasions of exotic species. Ecology 81, 1225–1239. doi: 10.1890/0012-9658(2000)081[1225:CBTESI]2.0.CO;2
Caporaso, J. G., Kuczynski, J., Stombaugh, J., Bittinger, K., Bushman, F. D., and Costello, E. K. (2010). QIIME allows analysis of high-throughput community sequencing data. Nat. Methods 7, 335–336. doi: 10.1038/nmeth.f.303
Castresana, J. (2000). Selection of conserved blocks from multiple alignments for their use in phylogenetic analysis. Mol. Biol. Evol. 17, 540–552. doi: 10.1093/oxfordjournals.molbev.a026334
Clarke, K. R. (1993). Non-parametric multivariate analyses of changes in community structure. Aust. J. Ecol. 18, 117–143. doi: 10.1111/j.1442-9993.1993.tb00438.x
Coyne, K. J. (2010). Benthic diatoms as indicators of water quality in Delaware's inland bays. Cent. for Inland Bays Final Proj. Rep. 1–33.
Desianti, N., Potapova, M., Enache, M., Belton, T. J., Velinsky, D. J., Thomas, R., et al. (2017). Sediment diatoms as environmental indicators in new jersey coastal lagoons. J. Cost. Res. 78, 127–140. doi: 10.2112/SI78-011
Delaware Department of Natural Resources and Environmental Control, DNREC. (2004). The State of Delaware promulgated the Delaware Coastal Zone Act.
Delaware Department of Natural Resources and Environmental Control, DNREC. (2006). Blackbird Creek Proposed Watershed TMDL'S.
DNREC/DNERR (1999). Estuarine Profiles. Delaware Department of Natural Resources and Environmental Control, Delaware National Estuarine Research Reserve (Dover, DE).
Dunbar, J., Ticknor, L. O., and Kuske, C. R. (2001). Phylogenetic specificity and reproducibility and new method for analysis of terminal restriction fragment profiles of 16S rRNA genes from bacterial communities. Appl. Environ. Microbiol. 67, 190–197. doi: 10.1128/AEM.67.1.190-197.2001
Ewing, H. A., and Nater, E. A. (2003). Use of scanning electron microscopy to investigate records of soil weathering preserved in lake sediment. Holocene. 13, 51–60. doi: 10.1191/0950683603hl594rp
Fortune, J., and Mauraud, N. (2015). Effect of tide on water water quality of Jones Creek, Darwin Harbour. Department of Land and Resource Management, Aquatic Health Unit. Palmerston, NT. Available online at www.nt.gov.au/lrm/water/aquatic/index.html
Goldfinch, A. C., and Carman, K. R. (2000). Chironomid grazing on benthic microalgae in a Louisiana salt marsh. Estuaries 23, 536–547. doi: 10.2307/1353144
Graham, J. C. U., and Laurent, P. (2000). Determining the environmental preferences of four estuarine epipelic diatom taxa: growth across a range of salinity, nitrate and ammonium conditions. Eur. J. Phycol. 35, 173–182. doi: 10.1080/09670260010001735761
Huelsenbeck, J. P., and Ronquist, F. (2001). MRBAYES: Bayesian inference of phylogenetic trees. Bioinformatics 17, 754–755. doi: 10.1093/bioinformatics/17.8.754
HydroQual Inc. (2006). Blackbird Creek Watershed Tmdl Model Development. Rep. No.: DNRE0070. Updated by Del. Dep. of Nat. Resources and Environ. Control, 78.
Johnson, M., Zaretskaya, I., Raytselis, Y., Merezhuk, Y., McGinnis, S., and Madden, T. L. (2008). NCBI BLAST: a better web interface. Nucleic Acids Res. 36, 5–9. doi: 10.1093/nar/gkn201
Karst-Riddoch, T. L., Pisaric, M. F. J., and Smol, J. P (2005). Diatom responses to 20th century climate related environmental changes in high elevation mountain lakes of the northern Canadian Cordillera. J. Paleolimnol. 33, 265–282. doi: 10.1007/s10933-004-5334-9
Kipp, R. M., McCarthy, M., and Fusaro, A. (2012). Pleurosira laevis. USGS Nonindigenous. Aquat. Species Database, Gainesville (FL) and Noaa Great Lakes Aquatic Nonindigenous Species Information System. Ann Arbor, MI. (Accessed June 2016).
Krammer, K., and Lange-Beralot, H. (1986–1991). “Bacillariophyceae,” in Süβwasserflora von Mitteleuropa Band 2/1-4, eds H. Ettl, J. Gerloff, H. Heynig, D. Mollenhauer (Stuttgart: Gustav Fischer Verlag), 1–437.
Kruskal, J. B. (1964). Nonmetric multidimensional scaling: a numerical method. Psychometrika 29, 115–129.
Kruskal, J. B., and Wish, M. (1978). Multidimensional Scaling. Sage University paper Series on Quantitative Applications in the Social Sciences, Series no. 07-001. Beverly Hills; London: Sage Publication.
Lee, K. (2012). Molecular Assessment if Benthic Diatom Assemblages in Delaware's Inland Bays. dissertation, University of Delaware, Newark, NJ.
Lionard, M., Muylaert, K., Gansbeke, D. V., and Vyverman, W. (2005). Influence of changes in salinity and light intensity on growth of phytoplankton communities from the Schelde River and estuary (Belgium/The Netherlands). Hydrobiologia 540, 105–115. doi: 10.1007/s10750-004-7123-x
Liu, D., Jiang, J., Wang, Y., Zhang, Y., and Di, B. (2012). Large scale northward expansion of warm water species Skeletonema tropicum (Bacillariophyceae) in China seas. Chin. J. Oceanol. Limnol. 30, 519–527. doi: 10.1007/s00343-012-1249-x
Liu, W., Marsh, T. L., Cheng, H., and Forney, L. J. (1997). Characterization of microbial diversity by determining terminal restriction fragment length polymorphisms of genes encoding 16S rRNA. Appl. Environ. Microbiology 63, 4516–4522.
Lobo, E. A., Heinrich, C. G., Schuch, M., Wetzel, C. E., and Ector, L. (2016). Diatoms as Bio Indicators in Rivers, in River Algae, ed Necchi J. R O. (Cham: Springer).
Marshall, H. G., Burchardt, L., and Lacouture, L. (2005). A review of phytoplankton within Chesapeake Bay and its tidal estuaries. J. Plankton Res.. 27, 1083–1102. doi: 10.1093/plankt/fbi079
Martin, G., and Fernandez, M. R. (2012). “Ecological Water Quality,” Water Treatment and Reuse, ed Voudouris (London: In Tech). doi: 10.5772/33831
McCorkle, R. C. (2000). Land Use/ Land Cover Changes and Ecological Health Indicators in the Blackbird Creek Watershed: With Recommendations for Restoration. master's thesis, University of Delaware, Newark, NJ.
Meunier, C. L., and Boersma, M. (2017). Aquatic Nutrient Cycling. North Carolina, NC: Oxford bibliography.
Murphy, J., and Riley, J. P. (1962). A modified single solution method for the determination of phosphate in natural waters. Anal. Chim. Acta 27, 31–36.
NOAA National Centers for Environmental Information (2016). National Overview- October 2009. Available online at https://www.ncdc.noaa.gov/sotc/national/200910.
National Weather Service Report (2010). Heavy Rainfall September 30- October 01, 2010. Binghamton (NY): National Weather Service (Accessed 2016 June). Available online at: http://www.weather.gov/bgm/pastFloodSeptember302010.
Neogi, S. B., Yamasaki, S., Alam, M., and Lara, R. J. (2014). The role of wetland microinvertebrates in spreading human diseases. Wetlands Ecol. Manage. 22, 469–491. doi: 10.1007/s11273-014-9373-3
NOAA National Centers for Environmental Information State of the Climate (2018). Hurricanes and Tropical Storms for Annual 2010, Published Online January 2011, (Retrieved April 18, 2018). Available online at: https://www.ncdc.noaa.gov/sotc/tropical-cyclones/201013.
Onyema, I. C. (2013). Phytoplankton bioindicators of water quality situations in Iyagbe lagoon, South-Western Nigeria. Res. J. Pharm., Biol. Chem. Sci. 4, 639–652. Available Online at: http://www.actasatech.com/index.php?q=journal.view.141
Oram, B. (2014a). Ammonia in Groundwater, Runoff and Streams. Dallas, PA: Water Research Center (Accessed June, 2016). Available online at: http://www.water-research.net/index.php/ammonia-in-groundwater-runoff-and-streams
Oram, B. (2014b). The Role Of Alkalinity In Citizen Monitoring. Dallas, PA: Water Research Center (Accessed June, 2016). Available online at: https://www.water-research.net/index.php/the-role-ofalkalinity-citizen-monitoring
Pandey, B. N. (2006). Animal Sciences and Environmental Issues: Agriculture, 106. Avaliable onlilne at: https://books.google.com/books?isbn=8176489883.
Patrick, R., Roberts, N. A., and Davis, B. (1968). The effect of changes in pH on the structure of diatom communities. Not Naturae. Acad. Nat. Sci. Philadelphia 416:16.
Pielou, E. C. (1966). The measurement of diversity in different types of biological collections. J. Theor. Biol. 13, 131–144 doi: 10.1016/0022-5193(66)90013-0
Portune, K. (2008). Examinations on Harmful Algal Cyst Distribution, Germination, and Reactive Oxygen Species Production Within Delaware's Inland Bays, USA. master's thesis, University of Delaware, Lewes.
Potapova, M., and Charles, F. D. (2007). Diatom metrics for monitoring eutrophication in rivers of the United States. Ecol. Indic. 7, 48–70. doi: 10.1016/j.ecolind.2005.10.001
Putland, J. N., and Iverson, R. (2007). Phytoplankton biomass in a subtropical estuary: distribution, size composition, and carbon: chlorophyll ratios. Estuar Coasts 30, 878–885. doi: 10.1007/BF02841341
Reavie, E. D., Heathcote, A. J., and Shaw Chraibi, V. L. (2014). Laurentian great lakes phytoplankton and their water quality characteristics, including a diatom based mdel for paleoreconstruction of phosphorous. PLoS ONE 8:e104705. doi: 10.1371/journal.pone.0104705
Rees, G. N., Baldwin, D. S., Watson, G. O., Perryman, S., and Nielsen, D. L. (2004). Ordination and significance testing of microbial community composition derived from terminal restriction fragment length polymorphisms: application of multivariate statistics. Antonie van Leeuwenhoe 86, 339–347. doi: 10.1007/s10482-004-0498-x
Rogerson, A. B., Jacobs, A. D., and Howard, A. M. (2010). Wetland Condition of the St.Jones River Watershed. Delawre Department of Natural Resources and Environmental Control, Watershed Assessment Section, Dover, USA. Available online at: https://www.researchgate.net/profile/Amy_Jacobs7/publication/242598754_Condition_of_Wetlands_in_the_St_Jones_River_Watershed/links/554d10400cf29f836c9cd66a/Condition-of-Wetlands-in-the-St-Jones-River-Watershed.pdf.
Ronquist, F., and Huelsenbeck, J. P. (2003). MRBAYES 3: Bayesian phylogenetic inference under mixed models. Bioinformatics 19, 1572–1574. doi: 10.1093/bioinformatics/btg180
Round, F. E., Crawford, R. M., and Mann, D. G. (1990). The diatoms: Biology and Morphology of the Genera. Cambridge: Cambridge University Press.
Ruhland, K. M., Priesnitz, A., and Smol, J. P. (2003a). Paleolimnological evidence from diatoms for recent environmental changes in 50 lakes across Canadaian Arctic treeline. Arct. Antarct. Alp. Res. 35, 111–124. doi: 10.1657/1523-0430(2003)035[0110:PEFDFR]2.0.CO;2
Ruhland, K. M., Smol, J. P., and Pienitz, R. (2003b). Ecology and spatial distributions of surface-sediment diatoms from 77 lakes in the subarctic Canadian tree line region. Can. J. Bot. 81, 57–73. doi: 10.1139/B03-005
Sambrook, J. F., and Russell, D. W. (2001). Molecular cloning: A Laboratory Manual, 3rd Edn. New York, NY: Cold Spring Harbor Laboratory Press.
Sarno, D., Kooistra, W. C. H. F., Medlin, L. K., Percopo, I., and Zingone, A. (2005). Diversity in the genus Skeletonema (Bacillariophyceae): III an assessment of the taxonomy S. costatum-like species, with the description of four new species. J. Phycol. 41, 151–176. doi: 10.1111/j.1529-8817.2005.04067.x
Shannon, C. E., and Weaver, W. (1949). The Mathematical Theory of Communication. Urbana: University of Ilinois Press,
Shinneman, A. L., Edlund, M. B., Almendinger, J. E., and Soninkhishig, N. (2009). Diatoms as indicators of water quality in western Mongolian lakes: A 54-site calibration set. J. Paleolimnol. 42, 373–389. doi: 10.1007/s10933-008-9282-7
Smucker, N. J., and Vis, M. L. (2011). Contributions of habitat sampling and alkalinity to diatom diversity and distributional patterns in streams: implications for conservation. Biodivers.Conserv. 20:643. doi: 10.1007/s10531-010-9972-0
Spaulding, S., and Edlund, M. (2008). Skeletonema in diatoms of the United States. Boulder (CO): US Geological Survey Institute of Arctic and Alpine Research. (Accessed 2016 Jun). available online at: http://westerndiatoms.colorado.edu/taxa/genus/skeletonema
Stoermer, E. F., and Julius, M. L. (2003). Centric Diatoms Freshwater Algae Of North America: Ecology And Classification.
Stone, M. L., Roseke, K., Chintapenta, L. K., Phalen, L., Kalavacharla, V., and Ozbay, G. (2016). A Water Quality Investigation of Blackbird Creek, Delaware. Fron. Environ. Sci. 4:70. doi: 10.3389/fenvs.2016.00070
Sundbäck, K., Enoksson, V., Graneli, W., and Petterson, K. (1991). Influence of sublittoral microphytobenthos on the oxygen and nutrient fluxes between sediment and water: a laboratory continuous flow study. Mar. Ecol. Prog. Ser. 74, 263–279. doi: 10.3354/meps074263
Talavera, G., and Castresana, J. (2007). Improvement of phylogenies after removing divergent and ambiguously aligned blocks from protein sequence alignments. Syst. Biol. 56, 546–577. doi: 10.1080/10635150701472164
Taylor, J. C., Archibald, C. G. M., and Harding, W. R. (2007). A Methods Manual for the Collection, Preparation and Analysis of Diatom Samples. WRC report no. TT 281/07, Water Research Commission, Pretoria, South Africa.
Thies, J. E. (2007). Soil microbial community analysis using terminal restriction fragment length polymorphisms. Soil Sci. Soc. Am. J. 71, 579–591. doi: 10.2136/sssaj2006.031
Valentine, V. J. (2002). Scrub-shrub/Emergent Wetland Ecotone Migration Along Delaware tidal Rivers in Response to Relative Sea-Level Change, Natural Impacts, and Human Modifications. dissertation, University of Delaware, Newark, NJ.
Verspagen, J. M. H., Van de Waal, D. B., Finke, J. F., Visser, P. M., Van Donk, E., and Huisman, J. (2014). Rising CO2 levels will intensify phytoplankton blooms in eutrophic and hypertrophic lakes. PLoS ONE 9:e104325. doi: 10.1371/journal.pone.0104325
Waddell, P. J., and Steel, M. A. (1997). General time-reversible distances with unequal rates across sites: mixing gamma and inverse Gaussian distributions with invariant sites. Mol. Phylogenet Evol. 8, 398–414.
Weckstrom, K., and Juggins, S. (2005). Coastal diatom-environment relationships from the Gulf of Finland, Baltic Sea. J. Phycol. 42, 21–35. doi: 10.1111/j.1529-8817.2006.00166.x
Weilhoefer, C. L., Nelson, W. G., and Clinton, P. (2015). Tidal channel diatom assemblages reflect within wetland environmental conditions and land use at multiple scales. Estuar. Coast. 38, 534–545. doi: 10.1007/s12237-014-9826-1
Zheng, L. C., and Zhang, F. Y. (2004). Development of water quality model in Satilla Estuary, Georgia. Ecol. Model. 178, 457–482. doi: 10.1016/j.ecolmodel.2004.01.016
Keywords: diatoms, tidal wetland, TRFLP, water quality, Delaware
Citation: Chintapenta LK, Coyne KJ, Pappas A, Lee K, Dixon C, Kalavacharla V and Ozbay G (2018) Diversity of Diatom Communities in Delaware Tidal Wetland and Their Relationship to Water Quality. Front. Environ. Sci. 6:57. doi: 10.3389/fenvs.2018.00057
Received: 27 November 2017; Accepted: 30 May 2018;
Published: 02 July 2018.
Edited by:
Khwaja M. Sultanul Aziz, Bangladesh Academy of Sciences (BAS), BangladeshReviewed by:
Sucharit Basu Neogi, International Centre for Diarrhoeal Disease Research, BangladeshAsli Aslan, Georgia Southern University, United States
Moniruzzaman Khondker, University of Dhaka, Bangladesh
Copyright © 2018 Chintapenta, Coyne, Pappas, Lee, Dixon, Kalavacharla and Ozbay. This is an open-access article distributed under the terms of the Creative Commons Attribution License (CC BY). The use, distribution or reproduction in other forums is permitted, provided the original author(s) and the copyright owner(s) are credited and that the original publication in this journal is cited, in accordance with accepted academic practice. No use, distribution or reproduction is permitted which does not comply with these terms.
*Correspondence: Gulnihal Ozbay, gozbay@desu.edu