- 1Department of Physical Geography, Institute of Geography, University of Goettingen, Göttingen, Germany
- 2Council for Scientific and Industrial Research, Soil Research Institute, Kumasi, Ghana
Tropical soils typically have low cation exchange capacity and nutrient contents. Both are enhanced by soil organic matter (SOM), which is thus particularly important for the fertility of these soils. In this study, we assessed the influence of urbanization on SOM, carbonate contents and pH of arable soils of Kumasi (Ghana, West Africa), since rapid urban sprawl is widespread in West Africa, whereby in many West African cities urban farming is pivotal to the food security of their population. Based on satellite imagery for the year 1986, we defined long-term and short-term urban arable soils, whereby long-term urban soils were urban already in 1986 (thus ≥30 years, as soil sampling took place in 2016), and short-term urban soils became urban afterwards (<30 years). We took 618 undisturbed topsoil samples (0–10 cm) from 206 urban arable fields. The factors land-use (maize fields) and soil (Ferric Acrisols) were kept largely constant. The fine-earth was analyzed for pH (water), carbonate contents, loss on ignition (LOI), total C (TC) and N (TN). SOM contents were obtained from LOI (SOMLOI). All element contents were transformed to bulk soil element stocks per m2. Differences between short-term and long-term urban soils were identified by a set of linear mixed models. Coarse fragments were more abundant in the long-term (16%) compared to the short-term (10%) urban soils, because of solid municipal waste that accumulates over time in urban soils. TC and SOMLOI concentrations of the fine earth, pH and C/N ratios were all significantly higher in the long-term urban soils. SOC concentrations in the fine earth, as well as bulk soil stocks of TC, SOC, and SOMLOI were also higher in the long-term urban soils, but at a lower degree of confidence, due to variable bulk densities and contents of coarse fragments. We conclude that dumping of organic and inorganic waste (including ash, bones, egg shells, concrete and mortar) increases SOM stocks and soil pH, but also leads to accumulation of non-degradable solid materials (including plastic, metals etc.). These findings point to the need of an improved urban waste management system, separating degradable and non-degradable waste.
Introduction
Soil organic matter (SOM) plays a vital role in soil fertility, biogeochemical cycling and mitigation of climate change (Lal, 2004; Lal and Augustin, 2012). For instance, SOM strongly influences soil fertility, because it is a major source of multiple plant nutrients (such as C, N, P, and exchangeable bases) and hence plays a key role for element cycling in the soil-plant system (Blume et al., 2016; Brady and Weil, 2017). SOM also provides energy and habitat for soil fauna, whose activity further enhances soil quality indicators, such as soil structure and nutrient availability amongst others (Magdoff and Weil, 2004; Brock et al., 2017). SOM moreover conditions the soils' texture and porosity, thereby improving water and nutrient retention as well as resilience of soils against mechanical stress (Lal, 2004; Brady and Weil, 2017; Brock et al., 2017). Additionally, dynamics of SOM make soils sources or sinks of atmospheric CO2 (Heitkamp et al., 2012). Hence, the quantity and quality of SOM is a vital indicator of soil quality and soil functioning (Brock et al., 2017).
It is thus of utmost importance to properly manage SOM stocks in soils to ensure crop production, particularly for nutrient-poor tropical soils (Lal, 2004). It is well-recognized that continued cultivation without sufficient fertilizer input leads to decline of SOM and nutrient supply in tropical soils (Bationo et al., 2007; Zingore et al., 2007, 2008). In this regard, inherently low soil fertility and depletion of nutrients are identified as major constraints to agriculture in sub-Sahara Africa (Sanchez et al., 1997). For instance, Stoorvogel and Smaling (1990) and Stoorvogel et al. (1993) calculated negative nutrient balances for arable fields in sub-Sahara Africa in the 1980s. Bationo et al. (2007) estimated a mean annual loss of 2–5% soil organic carbon (SOC) in cultivated soils of West Africa. Application of manure or compost as well as mulching have been identified as pivotal to proper SOM stock management in most tropical arable soils of sub-Sahara Africa (Bationo et al., 2007). Boateng et al. (2006) and Zingore et al. (2008) demonstrated that maize yields increased in response to manure application in Zimbabwe and Ghana. Both studies reiterated the findings of Bationo and Mokwunye (1991) and Bationo et al. (2004), who pointed to the benefit of applying manure to tropical arable fields in sub-Sahara Africa, which enhances multiple soil quality indicators. An increase in SOM stocks thus leads to increased agricultural yields and enhanced functioning of tropical soils as carbon sinks, even at low carbon sequestration rates (Lal, 2004; Lopez-Ulloa, 2006).
There are thresholds of SOC concentrations beyond which tropical soils may fail to provide basic ecosystem functions (Lal, 2004). Aune and Lal (1997) estimated the threshold of SOC concentration for productive maize fields in the tropics at 1.1%. Yet, it has been a major challenge to increase SOM concentrations of tropical soils. This difficulty is partly attributable to tropical climatic conditions that reduce the residence time of SOM (Amundson, 2001). Unlike their temperate counterparts, humid-tropical ecosystems feature temperature and precipitation regimes that enhance the biological, physical and chemical breakdown of SOM (Kirschbaum, 2000; Amundson, 2001). Amundson (2001) calculated a mean SOM residence time of 5 years in moist tropical soils compared to 9–14 years residence time in temperate regions, thus, suggesting a faster SOM turnover in tropical ecosystems that is relatively more sensitive to environmental changes, including land-use changes (Lopez-Ulloa, 2006). As a global phenomenon, urbanization is one of the major types of land-use changes. However, its direct influence on SOM stocks of tropical soils is still largely unknown.
The influences exerted by the agents of urbanization, such as housing, commerce, traffic, construction and waste, amongst others, differentiate soils in cities from soils in natural, semi-natural and agricultural areas (Lehmann and Stahr, 2007; Lorenz and Lal, 2009). For instance, soils in cities have developed from anthropogenic parent material and under the influence of the urban island heat effect (Sauer and Burghardt, 2006; Lorenz and Lal, 2009; Pouyat et al., 2010). Urbanization may moreover lead to compacted, polluted and sealed soils due to construction and infrastructure development (Lehmann and Stahr, 2007). Such extreme anthropogenically altered soils need to be distinguished from soils that even in urbanized areas do not necessarily turn into artificialized soils, which may e.g., underlie urban green infrastructure, such as parks, forests and arable fields (Edmondson et al., 2014; Joimel et al., 2016). With more than half of the global human population already living in urban environments, functions of urban soils that underlie the provision of various ecosystem services have been investigated in several studies (Baveye et al., 2016). Ecosystem services, such as microorganism habitat and carbon sequestration services may be enhanced in urban soils (Pouyat et al., 2002; Lorenz and Kandeler, 2005; Lorenz et al., 2006; Liu et al., 2018). Conversely, contamination of urban soils by heavy metals may endanger their potential for the provision of some ecosystem services (Anikwe, 2002; Imperato et al., 2003; Cardoso, 2014). Waste from households and industry is regarded as one of the key agents of urbanization that may alter soil properties (Bouma, 2016), including enhancement of ecosystem services provision and contamination of urban soils as mentioned above. Against this background, it has been proposed to harness the potentials of organic household waste as compost, particularly for cities in the global south (Drechsel and Kunze, 2001). However, many of the interrelations between urbanization and soil functioning remain unclear (Byrne, 2007; Lorenz and Lal, 2009), especially for cities in West-Africa.
Urbanization in Ghana like elsewhere in Africa has been associated with unsustainable features, such as rapid population growth, extensive land assimilation, environmental degradation and inadequate waste disposal systems (Pacione, 2009; Cobbinah et al., 2015). In particular, rapid urbanization in Africa has been demonstrated to proceed at the expense of agricultural land (Vermeiren et al., 2013). Still, urban agriculture has persisted and continues to provide food and employment for about 20 million people in West-African cities alone (Cofie et al., 2003; Drechsel and Dongus, 2010). These urbanization features eventually affect stocks of SOM and nutrients directly or indirectly through mechanical and/or chemical disturbance, changed management, environmental changes and pollution (Lorenz and Lal, 2009; Pouyat et al., 2010). The increased demand on land due to rapid urbanization has intensified urban agriculture in West-African cities (McGregor et al., 2011; Vermeiren et al., 2013). This situation directly affects the dynamics of SOM and nutrients, as fallow periods that are typically needed to balance nutrients in tropical soils drastically decreased (Lal, 2004; Bationo et al., 2007). Some urban farmers have responded by irrigation and higher fertilizer inputs, while others continue to depend on rain-fed agriculture with little or no fertilizer inputs, as reported for the city of Kumasi in Ghana (Drechsel, 2002; McGregor et al., 2011). Such different management schemes may result in different SOM stocks and nutrient dynamics, especially under a rapidly increasing urban influence. Yet, there is very limited knowledge on how urbanization is changing the properties of tropical arable soils in West-Africa.
Few studies have analyzed the nutrient levels of arable soils in some sub-Sahara African cities (Drechsel, 2002; Bellwood-Howard et al., 2015). However, they are largely inconclusive on the exact role of urbanization for these soils. For instance, Bellwood-Howard et al. (2015) attributed the observed different soil fertility trends of arable soils of Ouagadougou (Burkina-Faso) and Tamale (Ghana) to their differences in climatic conditions and fertilizer application, which may be independent of the urbanization process in the respective cities. To our knowledge, no study has yet specifically analyzed the role of urbanization for SOM stocks in tropical arable soils of West Africa. Therefore, in this study we systematically investigated SOM contents and stocks of arable soils of Kumasi in Ghana, West Africa. The aim of this work was to understand the potential of tropical urban soils to support food production, which is an important provisioning ecosystem service of urban soils in Ghana, as well as in West-Africa as a whole. We analyzed pH, carbonate and SOM concentrations of arable soils that had been in an urbanized environment for different periods in time (<30 years vs. ≥30 years). Contents of heavy metals and exchangeable cations were analyzed as well but are not in the focus of this article. This systematic approach considerably advances the knowledge on the potential of tropical soils for the provision of ecosystem services in West-African cities.
Materials and Methods
Study Area
Kumasi (Figure 1) is the second largest city in Ghana (after Accra, the capital) in terms of population and socioeconomic functionality. Unlike Accra, Kumasi is known for the production of considerable amounts of staples and vegetables in its core and peripheral areas (Drechsel et al., 2007). There are estimates that two out of three households in Kumasi have urban gardens for food production (Drechsel et al., 2007). This situation makes Kumasi particularly suitable for this study, as soils used for urban farming can be sampled in all parts of the city. Like most West-African cities that are undergoing rapid urbanization, Kumasi's population doubled from ~1 to ~2 million people between 2000 and 2010, exhibiting a rate of 5.6% population growth per year (Ghana Statistical Service, 2014). This rate has made Kumasi the fastest growing urban agglomeration in Ghana (Acheampong et al., 2017). Consistent with (Drechsel, 2002), field reconnaissance revealed that the crops that are mainly grown in the city of Kumasi include maize, cassava, plantain and local vegetables. These crops are grown in rain-fed agriculture, either in monoculture or in mixed cropping systems (Figure 2). In addition, intensively irrigated vegetables (e.g., spring onions and lettuce) are grown in monoculture to some extent.
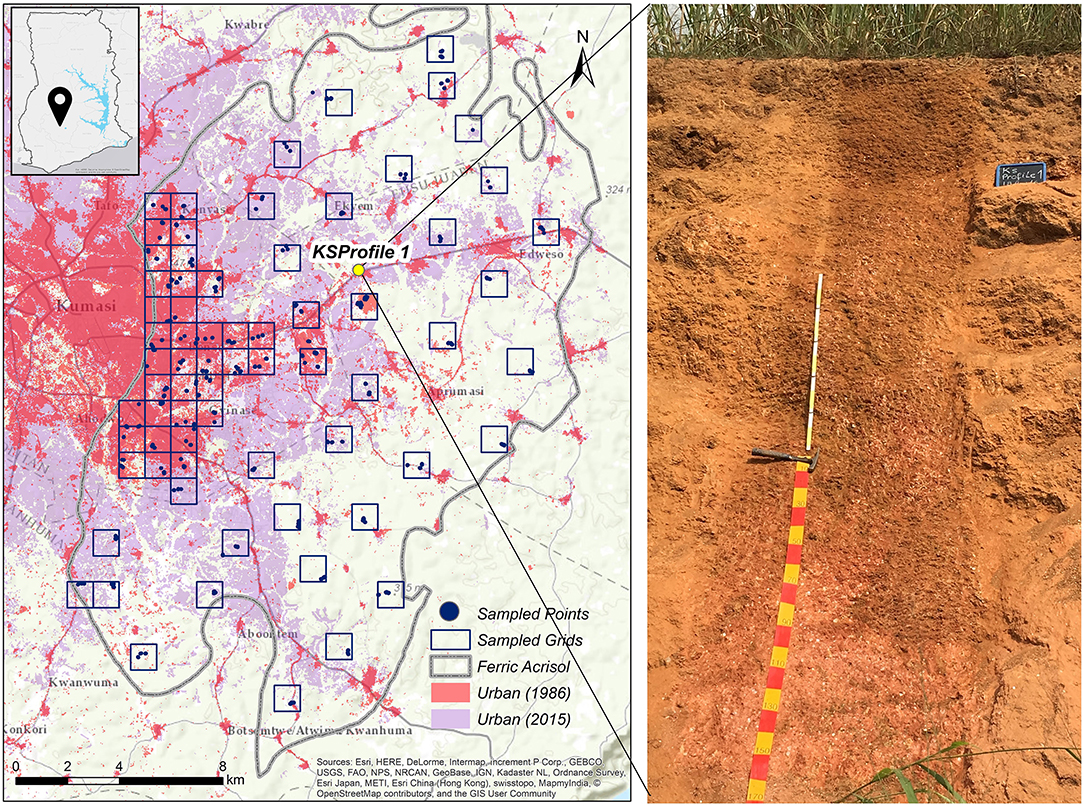
Figure 1. Kumasi agglomeration built-up area in 1986 showing the stratified grid-based sampling locations of the Ferric Acrisols. Sampling grids (1 × 1 km) closer to the core of the city that were already urban in 1986, were denoted as long-term urban soils (≥30 years with respect to the sampling year 2016), and those located in the periphery that were not yet urban in 1986, were denoted short-term urban soils. Three fields per grid were sampled, whereby three replicates per field were taken.
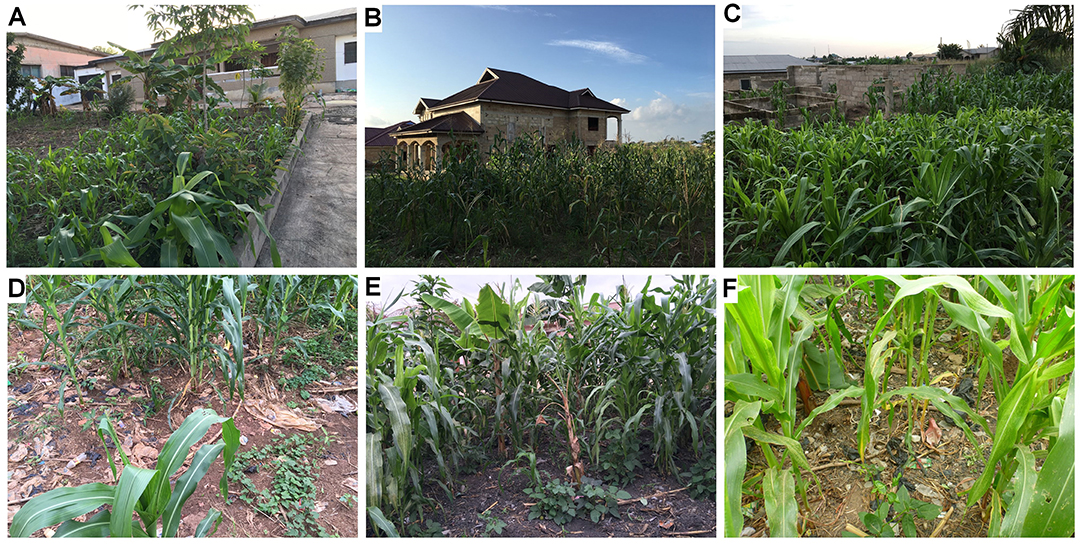
Figure 2. Some urban farms in Kumasi and their soil conditions. (A) shows a typical urban farm in the front yard of a residential home inside the city, (B,C) show urban farms in and around uncompleted residential houses in the outskirts of the city. (D–F) show plastic, charcoal, as well as other organic and inorganic wastes that are mostly disposed in these farms. Pictures taken in August to September 2016 by Stephen Asabere and Daniela Sauer.
The city is located in the deciduous forest agro-ecological zone of Ghana with a mean annual precipitation of 1,500 mm and a bimodal rainfall pattern. The peaks of the rainy season occur from May to June and from September to October (Dickson et al., 1988). Monthly average temperatures range between 26 and 27°C. The relief is undulating at an altitude of 250–300 m above sea level, and the soils are dominated by Ferric Acrisols (IUSS Working Group WRB, 2014). Their parent rock is the Cape Coast granite (Adu, 1992). The soils are generally strongly acidic with low base saturation and moderate soil organic matter contents (Adu, 1992).
Field Sampling Design and Approach
To investigate the influence of urbanization on arable soils, a stratified sampling design was employed. In a first step, we defined short-term and long-term strata, which were distinguished based on the built-up class of a 1986 Landsat satellite image of the Greater Kumasi metropolitan area that was classified by Asabere et al. (in preparation; Figure 1). Field sampling was conducted from August to September 2016. The soils of the inner built-up area that were in an urban environment already in 1986, thus having experienced ≥30 years of continuous urbanization, were denoted as long-term urban soils (Figure 1). The soils of the peripheral areas that became urban after 1986, thus having experienced < 30 years of continuous urbanization, were denoted as short-term urban soils (Figure 1). To keep the factor soil type largely constant, a Ferric Acrisol sub-group referenced in the local soil classification as the Bomso-Asuansi/Nta-offin compound association (Adu, 1992) was selected, and the boundaries of this mapping unit were used to define the study area (Figure 1). This particular soil unit was selected because it has a wide spatial coverage in both the core and peripheral area of Kumasi. Despite the widespread practice of dumping mixed organic/inorganic household waste on the urban fields and the resulting omnipresence of artifacts, the percentage of artifacts in the soils was still very low, and none of the soils had turned into a Technosol or Anthrosol. A 1 × 1 km grid was overlaid within the boundaries of the selected soil unit as shown in Figure 1.
To keep the factor land use/crop constant, the soil sampling focused only on fields where maize was grown, either in monoculture or mixed cultivation. Three maize fields per 1 × 1 km grid cell were sampled as illustrated in Figure 1. In this way, soils were sampled on altogether 206 maize fields, whereby three replicates per maize field were taken. Thus, 618 soil samples were taken from urban maize fields, including 357 samples from long-term urban fields and 261 samples from short-term urban fields. In addition, top soils of three rural arable maize fields (9 samples) and a forest within the city (9 samples) were sampled for comparison. All samples were taken in steel cylinders (length = 10 cm, volume = 255.17 cm3) that were vertically inserted from the soil surface, in order to obtain undisturbed top-soil samples from 0 to 10 cm soil depth. The fields were mostly located in the backyards or frontyards of houses, on public land adjacent to roads, open spaces and corners, and building construction sites (Figure 2). They all appeared to be under rain-fed agriculture without fertilizer inputs. Thus, the factors parent material, soil unit, climate and cultivated crop were all largely kept constant.
In addition to the 636 undisturbed top-soil samples, samples were taken from a soil profile in the central part of the study area. This soil profile, KS Profile 1 (Figure 1; Table 1), a Ferric Acrisol, served as a reference profile for the Bomso-Asuansi/Nta-offin compound association, since all 636 samples of this study were taken from this soil unit. The profile was exposed in a road cut located along the regional highway N6. The soil has formed in a granitic colluvial deposit, overlying the Cape Coast granite. It was described according to FAO (2006) and classified according to IUSS Working Group WRB (2014).
Laboratory Analysis
The soil samples were dried at 40°C and passed through a 2 mm sieve to separate the coarse fragments from the fine earth. Both were weighed. The coarse fragments included rock fragments and anthropogenic materials, such as metal, wood, plastic, leather, textile, charcoal, egg shells, animal bones etc. All analyses were carried out on the fine earth fraction. Fine earth subsamples were pulverized with a planetary ball mill for C and N analysis. Total C (TC) and N (TN) contents were analyzed by dry combustion with a CHN analyzer (LecoTruSpec). Soil pH was measured in water at a soil:solution ratio of 1:5 (ISO 10390, 2005). Samples with pH ≥ 6.0 were analyzed for carbonate contents using the Scheibler method (Blume et al., 2011; Vuong et al., 2013). The carbonate contents were also used for calculating soil inorganic carbon (SIC) contents. Soil organic carbon (SOC) contents were obtained by subtracting SIC from TC. To correct all results for residual water contents of the 40°C-dried samples, ~10 g fine earth was dried at 105°C to gravimetrically determine the water contents. The samples were then further incinerated at 430°C in a muffle furnace for 16 h to determine SOM by loss on ignition (SOMLOI) (Davies, 1974). All element contents were transformed to element stocks per 1 m2, considering bulk density and contents of coarse fragments.
Statistical Analysis
In order to identify effects of urbanization on soil properties, we tested all soil variables (analytical data) obtained for the long-term urban soils vs. the short-term urban soils with a set of linear mixed models (LMM) (Bates et al., 2015). In each model, a soil variable constituted the response, which was explained by a categorical variable indicating whether the sample belonged to a long-term or short-term urban soil. The model estimated a fixed effect, which described the relative difference between the long-term urban soils and the short-term urban soils with respect to the selected soil variable. To account for spatial correlation of data from the field triplicates, a field-level random effect was added to the model. After fitting the models, the residuals were tested for normality prior to further interpretation. 95% confidence intervals (CI) were estimated around each of the fixed effects by use of 500 parametric bootstrap simulations. Soil variables with a CI excluding zero indicated significant influence of urbanization (i.e., either long-term or short-term). Estimates of the fixed effect above zero indicated that the value of the respective variable was higher in long-term urban soils, whereas estimates below zero indicated that the value of the variable was lower in long-term compared to short-term urban soils. Beyond this inference, the upper and lower limits of the CI were used to further characterize the degree of confidence for the models.
A similar modeling approach was used to assess how bulk density was affected by SOM content in these soils in the context of urbanization, by analyzing the relationship between soil bulk density and its predictor SOM content in the fine earth. In the same way, the relationship between SOC concentration (calculated as SOC = TC–TIC) and its predictor SOMLOI concentration was tested, in order to check, whether the commonly used SOC-SOM conversion factors are applicable to these soils. The goodness of fit for the models was evaluated in both cases by the coefficient of determination (r2).
To complement the model-based inferences, an unbiased partitioning (Hothorn et al., 2006) for the binomial response of the short-term and long-term urban soils was carried out. The employed algorithm uses the complete dataset and, step by step, splits it into homogenous sub-groups according to the analyzed soil properties, thereby identifying the hierarchical levels at which the various soil properties discriminate the dataset. The hierarchical outcome, a dendrogram, finally also shows the proportions of short-term and long-term urban soils that correspond to a certain combination of soil properties. This approach helps to evaluate, which soil variables do most clearly discriminate short-term and long-term urban soils in Kumasi. The dendrogram also showed, at which significant values the classification into sub-groups happened. All statistical analyses were conducted in R. Core Team (2017), using its extensions “party” (Hothorn et al., 2006), “lme4” (Bates et al., 2015), and “MuMIn” (Barton, 2018).
Results
Bulk Density, Coarse and Fine Fractions of the Soils
The bulk densities of the long-term and the short-term urban soils were both 1.39 ± 0.01 g cm−3 (mean ± standard error). The model estimates of the response coefficients and associated confidence intervals were centered around zero, supporting this result (Figure 3). The mean bulk density of the rural arable soils (1.33 ± 0.04 g cm−3) was slightly lower, and that of the forest soils was again somewhat lower (mean = 1.28 ± 0.05 g cm−3; Figure 4). However, bulk densities of urban soils showed greater variability than those of rural soils, including several outliers (Figure 4). Some of the urban soils showed very low (< 1 g cm−3) or high (>1.6 g cm−3) bulk densities. Variability was greater in the long-term (range = 1.1 g cm−3) compared to the short-term (range = 0.72 g cm−3) urban soils.
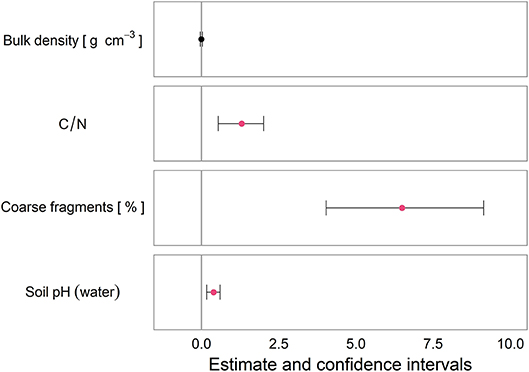
Figure 3. Estimated fixed effects of model coefficients and 95% confidence intervals (CI) of the linear mixed models for bulk density, C/N ratio, coarse fragment content and soil pH (water), which compares the two urban soil strata. Error bars that do not cross the zero line indicate statistical significance.
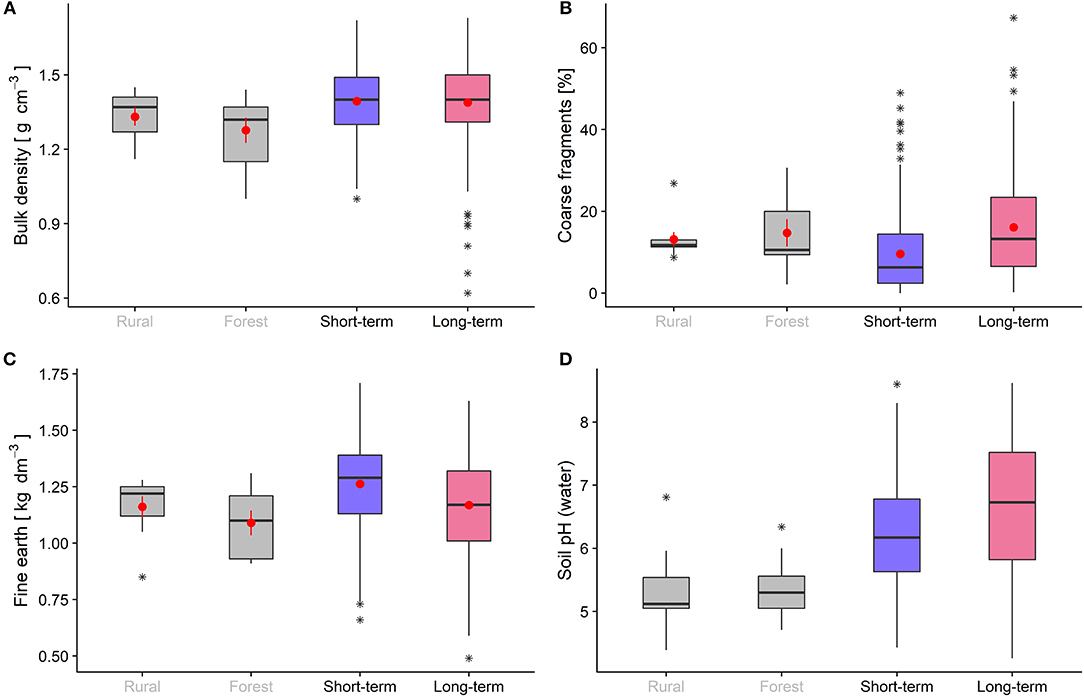
Figure 4. Boxplots for (A) bulk density, (B) coarse fragment content, (C) fine earth content, and (D) soil pH (water), of rural, forest and urban soils. Statistical analysis focused on relative differences between short-term and long-term urban soils. Arithmetic means (red points), standard errors (red whiskers), medians (bars), ranges (whiskers) and outliers (asterisks) are shown.
The long-term urban soils had moreover considerably higher contents of coarse fragments (mean = 16.1 ± 0.6%) compared to the short-term urban soils (mean = 9.6 ± 0.6%; Figure 4), whereby the model indicated a strong positive effect (Figure 3), reconfirming that the long-term urban soils had significantly more coarse fragments (~6% more), compared to the short-term urban soils. Conversely, the amounts of fine earth per dm−3 (providing SOM, CEC, and nutrients) were lower in the long-term compared to the short-term urban soils (Figure 3). Generally, the constituents of the coarse fragments included a mixture of rock fragments and anthropogenic materials, such as metal, wood, plastic, leather, textile, charcoal, egg shells, animal bones etc, which corresponded with field observations in Figure 2.
Soil pH
Mean soil pH values for the forest soils and the rural arable soils were pH 5.7 ± 0.3 and pH 6.0 ± 0.1, respectively (Figure 4). The urban soils exhibited substantially higher pH, with means of pH 7.4 ± 0.1 for the long-term and pH 7.0 ± 0.1 for short-term urban soils (Figure 4). Within the urban soils, the pH of the long-term urban soils was significantly higher (0.4 pH units) compared to their short-term counterparts (Figure 3). In 197 urban soils, including 155 long-term urban soils, pH was higher than pH 7, reaching up to a maximum of pH 8.6 (Figure 4).
Contents, Stocks and Quality of Soil Organic Matter
SOMLOI contents in the fine earth of the soils were significantly higher (7.0 g kg−1 difference) in the long-term compared to the short-term urban soils (Figures 5, 6). SOMLOI bulk soil contents and stocks of the long-term urban soils exceeded those of the short-term urban soils, too (Figures 7, 8), however with less confidence, as indicated by inclusion of zero in the output confidence interval of the model (Figure 5). Further inspection of the descriptive statistics generally supported this decreased confidence, as the mean SOMLOI fine earth contents of 50.8 ± 1.3 g kg−1 for the long-term and 43.9 ± 1.1 g kg−1 for the short-term urban soils were more clearly differentiated, compared to their SOMLOI stocks of 5.6 ± 0.1 and 5.3 ± 0.1 kg m−2, respectively (Table 2). The reason for the reduced confidence regarding the differences of SOM stocks compared to SOM contents is that the transformation of element contents to stocks considered the coarse fraction (>2 mm) of the soils, whereby the soil volume occupied by the coarse fraction was regarded as not contributing to SOM. The proportion of the coarse fraction was increased in the urbanized soils due to artifacts, thus counteracting the general increase of SOM contents in the urbanized soils. The mean SOMLOI stock of the forest soils (5.7 ± 0.5 kg m2) was comparable to that of the long-term urban soils, whereas the mean SOMLOI stock of the rural arable soils (4.3 ± 0.2 kg m−2) was the lowest of all subsets of soil samples. Thus, the urban arable soils, particularly those under long-term urbanization, exhibited similar SOM stocks as those of soils under tropical forest conditions in Kumasi.
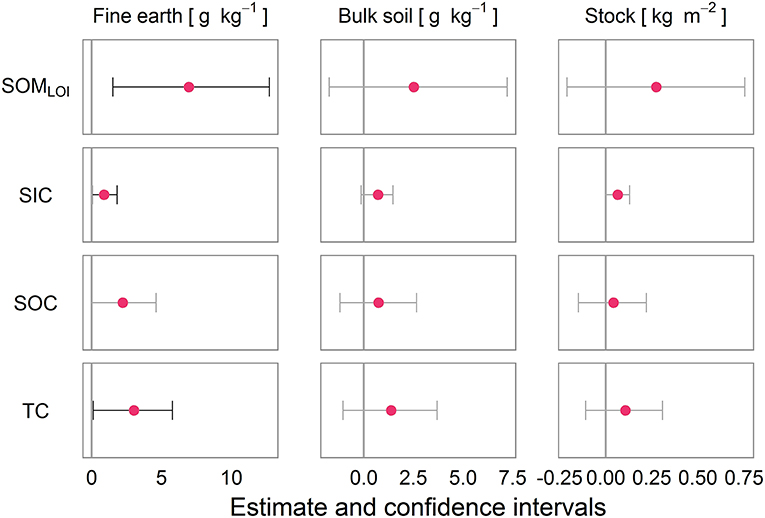
Figure 5. Comparison of long-term vs. short-term urban soils, estimating fixed effects of model coefficients and 95% confidence intervals (CI) of the linear mixed models for SOMLOI, soil organic matter obtained from loss on ignition; SIC, soil inorganic carbon; SOC, soil organic carbon; and TC, total carbon. Positive values indicate an increase of the respective soil variable in long-term urban soils, whereas negative values indicate a decrease in long-term urban soils compared to short-term urban soils. Error bars that do not cross the zero line indicate statistical significance.
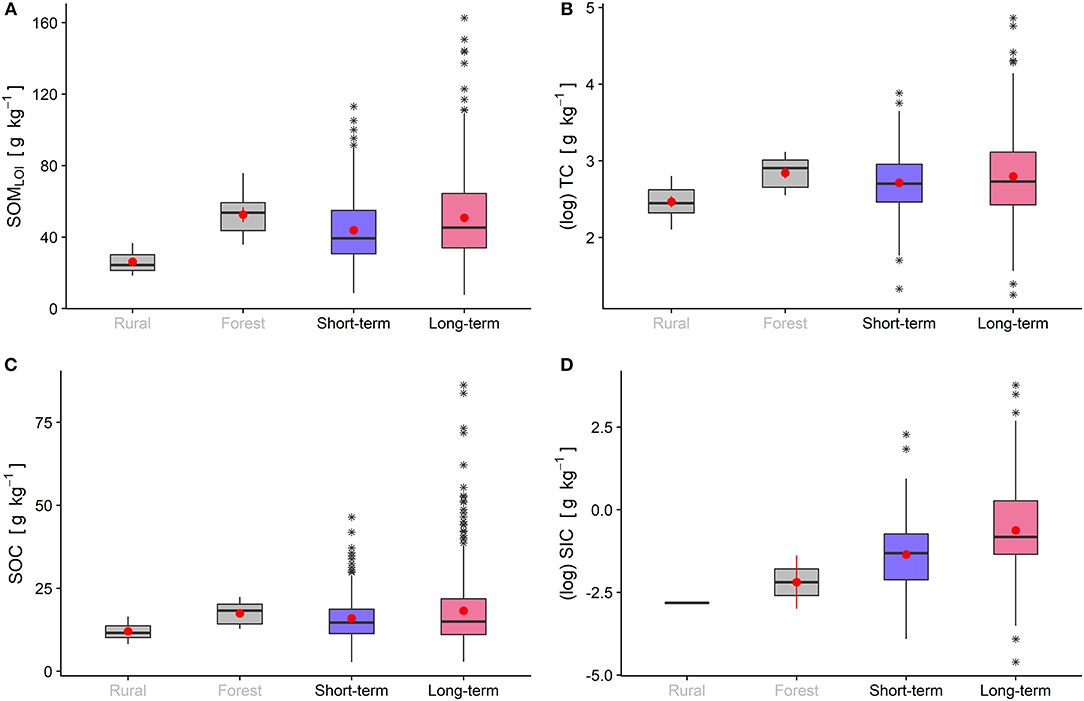
Figure 6. Boxplots comparing rural, forest and urban soils with respect to fine earth contents of (A) SOMLOI, soil organic matter obtained from loss on ignition; (B) TC, total carbon; (C) SOC, soil organic carbon; (D) SIC, soil inorganic carbon. Arithmetic means (red points), standard errors (red whiskers), medians (bars), ranges (whiskers), and outliers (asterisks) are shown.
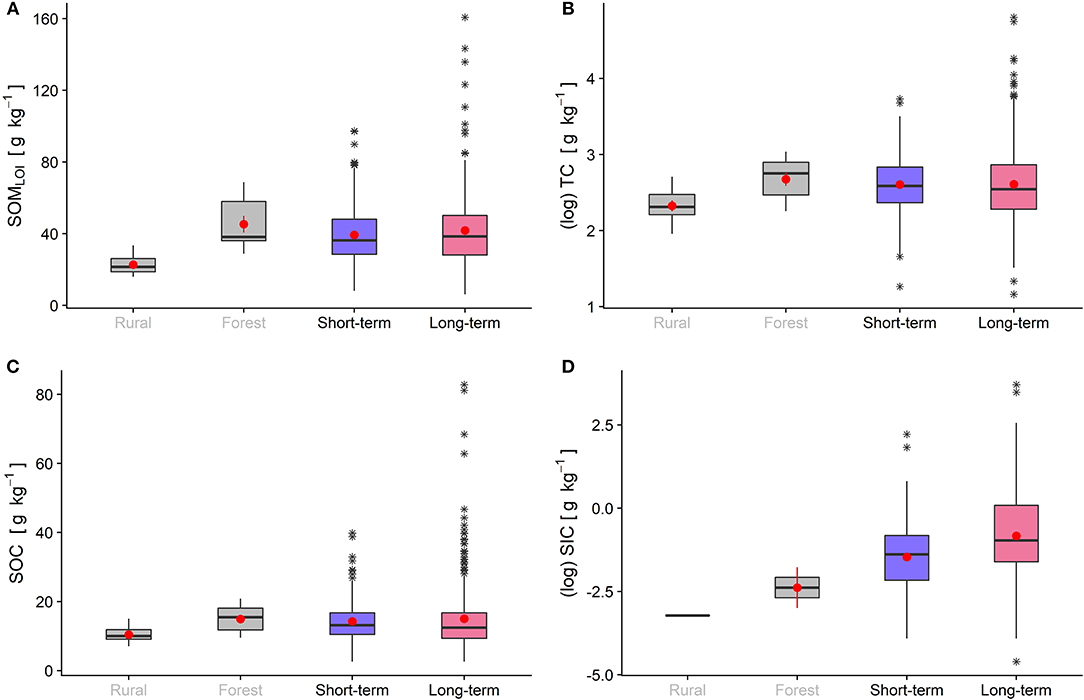
Figure 7. Boxplots comparing rural, forest and urban soils with respect to bulk soil contents of (A) SOMLOI, soil organic matter obtained from loss on ignition; (B) TC, total carbon; (C) SOC, soil organic carbon; (D) SIC, soil inorganic carbon. Arithmetic means (red points), standard errors (red whiskers), medians (bars), ranges (whiskers), and outliers (asterisks) are shown.
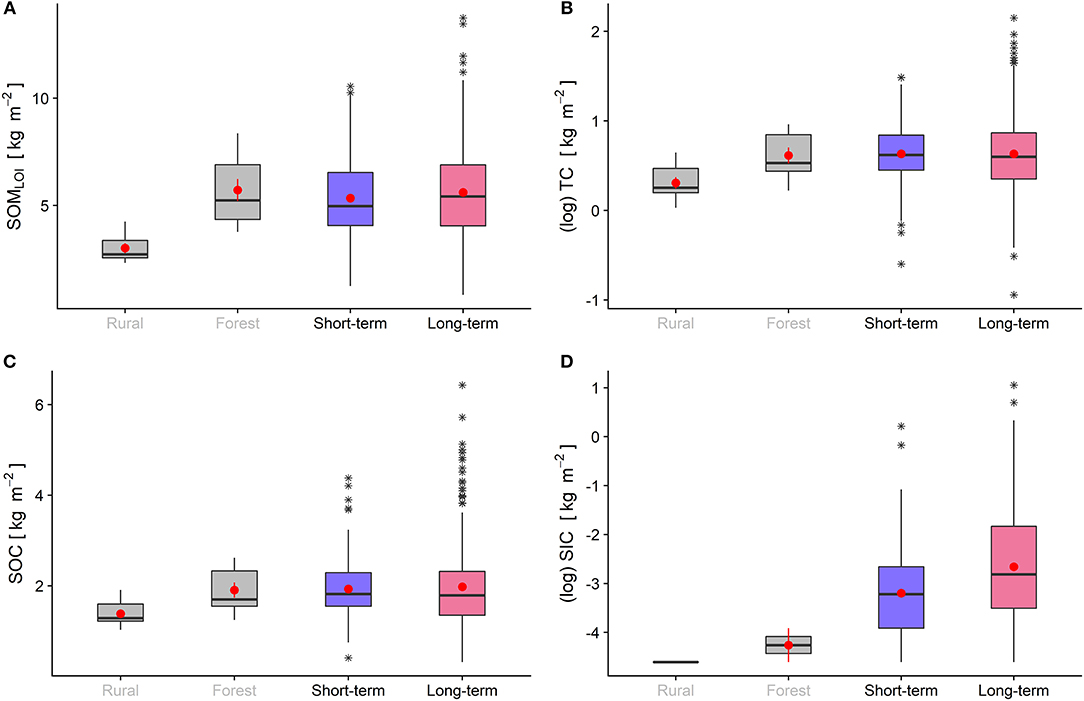
Figure 8. Boxplots comparing rural, forest and urban soils with respect to their amounts per m2 (in the upper 10 cm) of (A) SOMLOI, SOM obtained from loss on ignition; (B) TC, total carbon; (C) SOC, soil organic carbon; (D) SIC, soil inorganic carbon. Arithmetic means (red points), standard errors (red whiskers), medians (bars), ranges (whiskers), and outliers (asterisks) are shown.
Total carbon (TC) followed the same trend as SOMLOI (Figures 5–8), with the long-term urban soils having significantly higher TC contents in their fine earth than the short-term urban soils. Consistently, the contents and stocks of the bulk soils of the long-term urban soils also exceeded those of the short-term urban soils, but with less confidence.
The contents and stocks of soil inorganic carbon (SIC) in both fine earth and bulk soils of the long-term urban soils significantly exceeded those of the short-term urban soils (Figure 5; Table 2). The upper limit of the model estimates might include zero (~0.1), particularly for the bulk soil contents and stocks (Figure 5). However, the descriptive statistics, such as the means (Table 2) showed that SIC bulk soil contents and stocks in the long-term urban soils were at least twice as high as in the short-term urban soils.
Also, the soil organic carbon (SOC) contents and stocks of the long-term urban soils exceeded those of the short-term urban soils (Figures 5–8; Table 2). Inspection of the model estimates, however, resulted in a low confidence for both fine earth and bulk soil SOC data (Figure 5). Mean SOC content and stock of the urban soils (Table 2) were comparable to those of the forest soils (content = 17.4 ± 1.2 g kg−1, stock = 1.9 ± 0.2 kg m2), whereas the rural arable soils had much lower mean SOC content and stock (content = 12.0 ± 1.0 g kg−1, stock = 1.4 ± 0.1 kg m2). Thus, over all, the SOMLOI, TC and SOC fine earth and bulk soil concentrations, and the corresponding stocks showed a gradual decrease in confidence of the model estimates.
SOC data are commonly used to calculate SOM contents of soils by multiplication with the factor 2.0 or 1.72 (Pribyl, 2010). These factors would correspond to SOC/SOM ratios of 0.5 and 0.58, respectively. However, the SOC/SOMLOI ratios of the 636 topsoil soil samples of Kumasi exhibited a very wide range, from 0.03 to 0.72. Their mean SOC/SOMLOI ratio was only 0.36 ± 0.1, which corresponds to a SOC-SOM conversion factor of 2.78. One sample showed a SOC/SOMLOI ratio of 4.7, which is impossible. For this particular sample, concentrations of the SOC and TC in the fine earth was higher relative to its SOM concentration, and thus the value was attributed to analytical error from the LOI. This value was therefore not included in the calculations. The SOC/SOM ratios of the short-term and long-term urban soils were similar (Figure 9A). The rural arable soils exhibited the highest mean SOC/SOM ratio of 0.46, whereas the forest soils showed a mean SOC/SOM ratio of 0.34 (Figure 9A). The relationship between SOC and SOMLOI of all samples from Kumasi (Figure 10A) can be expressed by the equation SOC = 0.37 SOMLOI (r2 = 0.89).
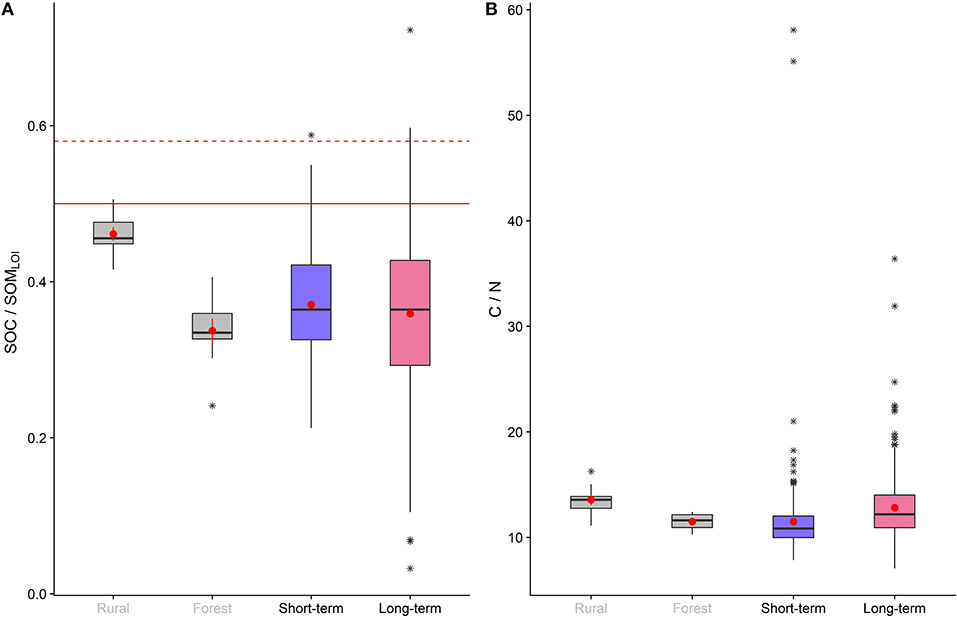
Figure 9. Boxplots comparing rural, forest and urban soils with respect to their (A) SOC/SOMLOI ratios, and (B) C/N ratios. Arithmetic means (red points), standard errors (red whiskers), medians (bars), ranges (whiskers), and outliers (asterisks) are shown. In (A), the thick red line represents the ratio of 0.5, and the dash red line represents the ratio of 0.58, which are both commonly used in literature for SOC/SOM conversions.
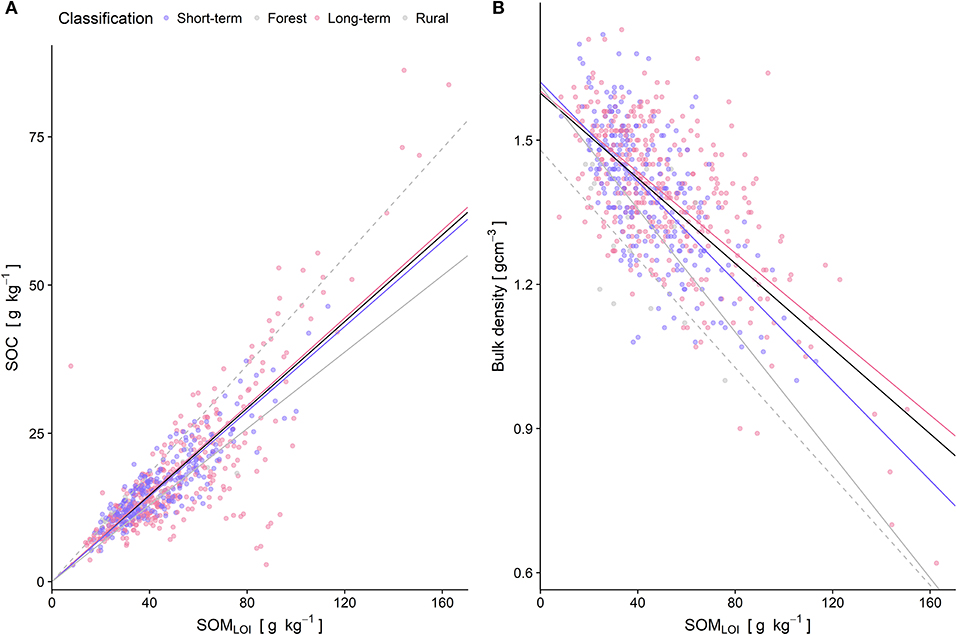
Figure 10. Relationships between (A) the concentrations of SOC, soil organic carbon; and SOMLOI, soil organic matter obtained from loss on ignition in the fine earth, (B) Bulk density and concentration of SOMLOI in the fine earth. The black line represents the linear relationship for forest, rural and the urban soils, the pink line represents the long-term urban soil, the violet line represents the short-term urban soil, the gray dash line represents rural arable soils, and the gray thick line represents forest soils.
C/N ratios, commonly used to characterize SOM quality, were calculated as SOC/TN. They ranged from 7.1 to 58.1, with four samples showing C/N ratios above 25. Out of the four samples, two with extreme C/N ratios of 55.1 and 58.1 belonged to the short-term urban soils, and the other two, with C/N ratios of 36.4 and 31.2, belonged to the long-term urban soils. The four maize fields from where these samples were obtained were all characterized by massive household waste dumping. In general, C/N ratios were significantly wider (by 1.3 units) in the long-term urban soils, which showed a mean C/N ratio of 12.8 ± 0.2, compared to the short-term urban soils that exhibited a mean C/N ratio of 11.5 ± 0.2 (Figures 3, 9B). The mean C/N ratio of the rural arable soils was 13.6 ± 0.5, and that of the forest soils was 11.5 ± 0.3. Thus, the rural arable soils showed the highest C/N ratios, whereas the forest soils had C/N ratios similar to the short-term urban soils.
Relationships Between Bulk Density, SOM and Other Soil Variables
As to be expected, bulk density showed a strong negative relationship with SOMLOI concentration in the fine earth (Figure 10B), which was supported by the model estimates and confidence intervals (−88.6 to −71.8). A linear regression equation BD = 1.6–0.004 SOMLOI, was used to express this relationship with r2 = 0.74. Similar model estimates and coefficients were also derived for the long-term and the short-term urban soils (Figure 10B).
The dendrogram that was obtained from the recursive partitioning (Figure 11) revealed that out of all variables that were investigated, only six clearly discriminated long-term from short-term urban soils. These variables included (1) contents of coarse fragments, (2) soil pH, (3) C/N ratios, (4) SOC stocks, (5) SOMLOI contents in the fine earth, and (6) SIC contents in the bulk soils. At the highest hierarchical level, the samples were split by the model into samples with ≤ 5.42 and >5.42% coarse fragments. A combination of >5.42% coarse fragments and soil pH > 7 included predominantly (~87%) long-term urban soils. Also, the combination of >5.42% coarse fragments, soil pH ≤ 7, C/N > 10.09, and SOMLOI > 59.54 g kg−1 included predominantly long-term urban soils. Conversely, the two following combinations included predominantly short-term urban soils: (A) ≤ 5.4% coarse fragments and C/N ratio ≤ 10.2, (B) >5.4% coarse fragments, soil pH ≤ 7, C/N ratio ≤ 10.09, and SOC stocks >1.71 kg m2.
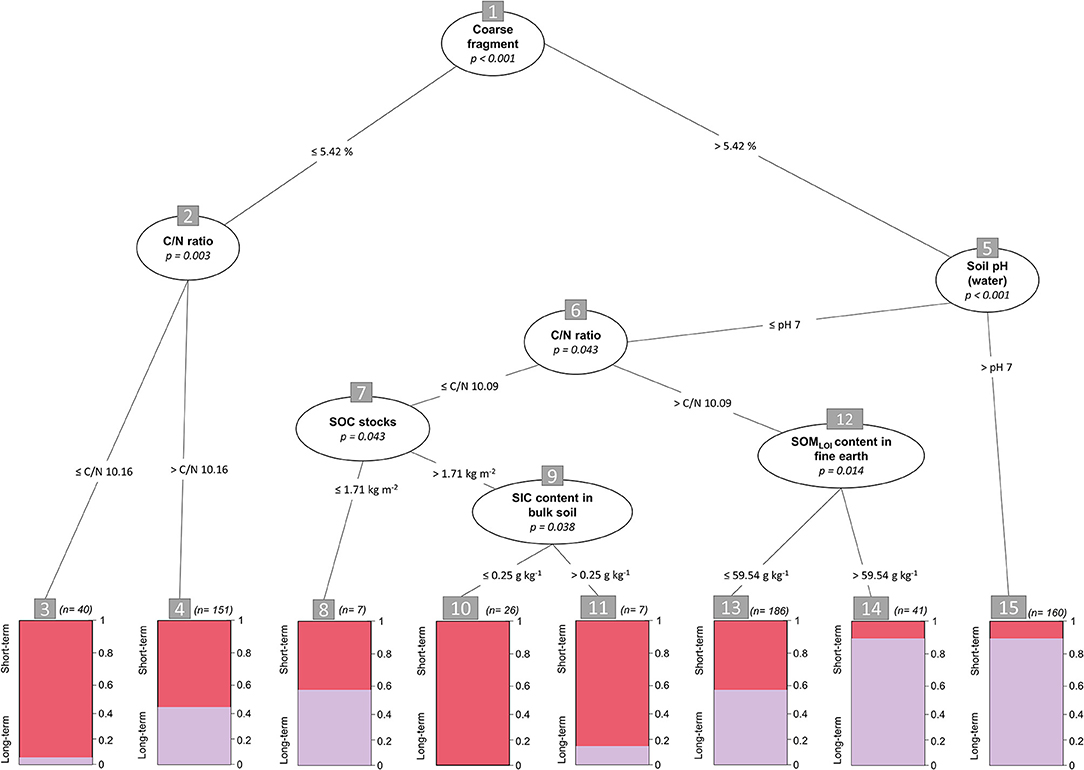
Figure 11. Dendrogram showing the hierarchical alignment of the six variables that can most clearly discriminate short-term and long-term urban soils. Branches show threshold values for the partitioning referring to the variable shown in the node above together with the test statistics for variable selection. Numbers on top of the nodes indicate the hierarchical level.
Discussion
Effects of Urbanization on Bulk Density and Coarse Fractions of Arable Soils
Soil bulk density in both short-term and long-term urban soils was conducive to plant growth (Hazelton and Murphy, 2016) and was only slightly higher than in forest and rural arable soils. Thus, the physical conditions of the urban arable soils were still intact, even under prolonged urban influence. The range of bulk density data reported for maize fields in humid tropical regions of Ghana and Zimbabwe were within a similar range as that of the soils under maize in Kumasi (Minta, 1998; Zingore et al., 2008). This range is also consistent with bulk density values reported for soils in other cities around the world (Lorenz and Kandeler, 2005; Smetak et al., 2007; Edmondson et al., 2011). Thus, contrary to soils investigated e.g., by Jim (1998), Lehmann and Stahr (2007), and Lorenz and Lal (2009), the bulk densities of urban soils of Kumasi are conducive to plant growth.
Bulk density variability was greater in the long-term compared to the short-term urban soils. This finding can be explained by the types of urban activities in the direct vicinity of some of the long-term urban maize fields. For instance, the field, where the highest bulk density (1.73 g cm−3) was measured, was located close to Kumasi airport, a bus station, a concrete block production factory, and a major road, all of which involve human activities that may lead to soil compaction. The field, where the lowest bulk density (0.62 g cm−3) was measured, was located within the confines of an abandoned wood processing factory, near Kaase industrial area. These two situations, though extreme, may serve as examples for the possible influence of urban and industrial activities on soil physical properties in the Kumasi area. Thus, the considerable variability in bulk density that was found particularly for the long-term urban soils, can be attributed to the exposure of some of these arable fields to various urban influences, including anthropogenic compaction and deposition, which may also increase surface run-off and erosion, and thus enhance soil degradation (Labrière et al., 2015). Such variability is reminiscent of the general pattern of soil properties in urban soils, as also observed in other cities around the world (Jim, 1998; Schleuß et al., 1998; Pouyat et al., 2010).
Anthropogenic activities are also reflected in the coarse fraction of the soils, which, in addition to rock fragments, contained also various anthropogenic materials. Whereas, some studies highlighted the nutrient contribution of coarse fragments to soils (Corti et al., 2002), it is usually assumed that a high rock fragment content rather has a diluting effect on the fine earth nutrient stocks available to plants (Augustin et al., 1998), as the nutrients are released rather slowly from weathering rock fragments. In the case of the urban maize fields of Kumasi, the nature of the coarse fragments, and their potential to release elements to the soils, is very diverse. In addition to rock fragments, the coarse fraction comprises household and construction waste, including metal, plastics, textiles, etc. The abundance of these materials is the most obvious direct consequence of rapid urbanization for the arable soils of Kumasi.
It is well-recognized that one third to one half of solid waste in most cities in the global south is not collected (Pacione, 2009). Likewise, the inadequacy of waste disposal systems in Kumasi is ubiquitous in Ghana (Fuseini and Kemp, 2016). This situation was clearly reflected in the on-field observations made during this study, as most of the arable fields also served as household waste disposal sites. Hence, we conclude that the identified and significantly higher coarse fragment contents of the long-term urban soils compared to the short-term urban soils of Kumasi are due to long-term continuous dumping on the fields.
Effects of Urbanization on Soil pH
Soil pH has great influence on soil chemical processes that determine the behavior of nutrients and contaminants (Blume et al., 2016). Hence, it is also an important influencing factor for nutrient availability and plant growth. Whereas, acidification is a natural process in most humid tropical soils, anthropogenic activities may lead to alkanization (Blume et al., 2016). Soil pH in the long-term urban soils of Kumasi was significantly higher, compared to the short-term urban soils. Both, long-term and short-term urban soils, had higher pH (mean pH > 7) compared to the rural arable soils and the forest soils. Consistent with Adu (1992), the pH values of the rural arable soils and the forest soils were in the usual range for these soils. Boateng et al. (2006) and Quansah (2010) reported pH (water) 4.3–4.6 and pH (water) 6.1–6.3, respectively, for arable maize fields on similar soils in Ghana. Soil pH obtained for the urban arable soils in Kumasi in this study were significantly higher than both (i.e., pH data from literature and our own pH data of rural and forest soils). This clear difference indicates that arable soils in Kumasi which become included in the urbanization process, are influenced by anthropogenic activities that have a similar effect as liming (Blume et al., 2016; Hazelton and Murphy, 2016), although intentional liming as part of the management of the fields is usually not done, as confirmed by field observations and farmer interviews.
Possible reasons for the high pH (>7) in the urban soils include in particular the release of alkaline leachates from calcareous materials (Jim, 1998) and decomposing organic waste (Boateng et al., 2006; Cofie et al., 2009). Both field and lab experiments confirmed that decomposition of organic matter may increase the soil pH of tropical soils (Boateng et al., 2006; Zingore et al., 2008; Cofie et al., 2009). In addition, the formation of alkaline leachate from construction materials, such as building sand and cement is very likely under the conditions of this study. Similar to the situation in Kumasi, Jim (1998) reported higher soil pH in urban soils of Hong Kong, compared to adjacent natural soils, and attributed the increased pH to construction waste. Such influence is very likely in Kumasi, too, as most of the sampled arable fields in Kumasi were located within active construction sites. This is a common situation in Ghana, as the construction of a building usually takes at least 10 years (Yeboah and Obeng-Odoom, 2010; Stow et al., 2016). Thus, many of the urban arable soils were most likely under the influence of a nearby construction site for at least 10 years and were possibly used as repositories of construction materials for some time. Carbonates (as contained in various construction materials) in equilibrium with natural systems, such as soils are known to exhibit a theoretical pH (water) ~8.5 (Brady and Weil, 2017), and hence the presence of carbonate compounds in the urban soils is another potential source of the high pH. In this regard, various components of household waste that were identified in the urban arable soils during fieldwork provide sources of such carbonate solid phases, including e.g., eggshells, animal bones, batteries, charcoal and ashes. Also, the detection and measurement of carbonates in most of the urban soils with >pH 6 emphasizes this point. For instance, two samples that had the highest SIC stock of >2.0 kg m−2, were also amongst the three samples with a ≥pH 8.6. Like the abundance of coarse fragments, the high pH was another very clear consequence of rapid urbanization that was identified in this study for the arable soils of Kumasi. However, such high soil pH as observed in the urban arable soils of Kumasi may induce phosphorus and micronutrient deficiency (Jim, 1998). It remains, therefore, to be confirmed by further studies, if this is the case for these soils.
Effects of Urbanization on SOM
SOMLOI contents and stocks of the long-term urban soils generally exceeded those of the short-term urban soils, although at various degrees of confidence. Considering the nature of the coarse fragments and the observed anthropogenic activities on and near the fields, it was evident that the measured LOI and TC contents included not only typical SOM, but also artificial combustible materials, such as fragments of textiles, plastics, and charcoal amongst others. The abundance of SIC in the urban soils strongly supported this assumption. The trends of SIC contents and stocks, which were consistent with the trends of SOMLOI and TC contents and stocks, as well as those of soil pH and coarse fragment contents, stressed the prominent role of household waste on the urban arable soils.
These findings point to two main mechanisms related to rapid urbanization. The first mechanism is the common practice of dumping of household waste on urban arable fields, and more specifically, the high proportion of organic materials in the household waste of Kumasi, which substantially contributes to SOM stocks of the urban arable soils of Kumasi. The range of SOM contents of urban soils of Kumasi was comparable to the range found for soils under various urban land-use types in the city of Baltimore, USA (Pouyat et al., 2002). Windmeijer and Andriesse (1993) reported 24.5 g kg−1 SOC for West African equatorial forests, which is also within the range of the SOC contents of the urban arable soils of Kumasi. Prudencio (1993) reported a range of 11–22 g kg−1 SOC for home gardens in Burkina Faso, which is slightly less, compared to the arable soils of Kumasi. Dicko (2016) reported SOC stocks of 1.02 kg m−2 for maize fields in the Upper East region of Ghana, which is markedly lower compared to the mean SOC stocks of the urban arable fields in Kumasi.
The share of SOC in the SOMLOI of the arable soils in Kumasi was about 37%, which is markedly lower compared to the estimated 50 or 58% that are usually assumed in the common conversion equations from SOC into SOM data (Pribyl, 2010). Further studies are needed to confirm this finding, because in this study, we calculated SOC contents as SOC = TC – SIC, whereby SIC contents were determined by the Scheibler method, which is less precise than the TC analysis by CN analyzer. Moreover, SOM contents were calculated based on LOI, and in the case of Kumasi, it is very likely that not only SOM but also the combustion of fine material of disintegrated artificial materials contributed to SOMLOI (Chatterjee et al., 2009; Vuong et al., 2013). Despite these uncertainties, the substantial difference in the proportion of SOC in SOM (37% for Kumasi) suggests that the SOM of the urban arable soils of Kumasi has a considerable lower proportion of SOC than usually assumed for SOM (50 and 58%, respectively).
The potential contribution of artificial materials to the soil carbon contents does apparently not lead to an increase in C/N ratio, as the rural arable soils showed a mean C/N ratio of 13.6, whereas the short-term urban soils exhibited a mean C/N ratio of 11.5, and the long-term urban soils had a mean C/N ratio of 12.8. Thus, the urban soils exhibited even somewhat lower C/N ratios than the rural soils. A possible explanation for this trend might be higher C/N ratios in the organic household waste, as the latter is an important source of SOM in the urban soils, in addition to crop residues of the maize plants. In contrast, the SOM of the rural soils under maize has no such source in addition to the maize plant residues. Only four out of the 618 urban soils exhibited C/N ratios above 25, indicating either a lower quality of the SOM (Hazelton and Murphy, 2016), or a contribution of charcoal. In general, the C/N ratio suggested favorable characteristics of the SOM (Schipper et al., 2004) in all arable soils (urban, rural, and forest) of Kumasi. The mean C/N ratios of the soils analyzed in this study were similar to the C/N ratios of 10.1–11.5 obtained for forest soils of Ghana by Duah-Yentumi et al. (1998), and 14.9 ± 8.6 (mean ± standard deviation) for many humid-tropical soils in general, as reported by Post et al. (1985). The even slightly lower mean C/N ratio of the long-term relative to the short-term urban soils indicates that the quality of SOM in the arable soils of Kumasi is maintained even under the prolonged influence of rapid urbanization.
Thus, by disposing household waste on their fields (through mostly unplanned efforts), urban farmers in Kumasi cannot only keep the SOM stocks of their soils at higher levels compared to rural arable soils, but they may also maintain suitable C/N ratios. Consistent to the explanation of increased SOM stocks and decreased C/N ratios in urban soils by dumping of household waste with low C/N ratio, Miezah et al. (2015) reported that 67% of municipal solid waste in Ghana is biodegradable. More specifically, in Kumasi the proportion of biodegradable materials was estimated to be 64%, in addition to 22% inert materials, which are described to include e.g., ashes, sand and charcoal (Asase et al., 2009). These estimates, therefore, suggest that at least 86% of urban solid waste in Kumasi, which may be uncollected due to inadequate waste management systems, thus contributes significantly to SOM stocks.
The second main mechanism related to rapid urbanization, is the accumulation of non-biodegradable household wastes and construction materials, which dilute SOM and nutrient concentrations measured in the fine earth fraction to varying degree. This effect may explain why the differences between short-term and long-term urban arable soils with respect to SOMLOI and SOC stocks were less significant than those with respect to the SOMLOI and SOC contents in the fine earth fraction. In a related study, Asabere et al. (in preparation) identified the nucleated urban expansion nature of Kumasi, underscoring that areas that are closer to the core of the city have been under longer urbanization with denser population. Such progressively denser population however leads to enhanced environmental degradation (Cobbinah and Erdiaw-Kwasie, 2016), including e.g., enhanced accumulation of municipal solid waste and rock fragments. Thus, we suggest that the long-term urban soils that have been under continuous urbanization for over 30 years and experienced an increase in population density over this period, accumulated greater amounts of uncollected waste and rock fragments than the short-term urban soils, whereby the accumulation proceeded in a non-linear way but was enhanced as the population density increased. The outcome of the recursive partitioning strongly confirmed the importance of coarse fragments in discriminating short-term and long-term urban soils.
The findings of this study strongly suggest that Kumasi would benefit immensely from an integrated waste management system (Asase et al., 2009). As demonstrated by Miezah et al. (2015), 92% of urban residents in Ghana are willing to separate their waste in order to benefit from the associated environmental benefits. Thus, a promising way could be that city authorities actively engage urban residents in municipal solid waste management, provide the necessary infrastructure for collecting non-biodegradable waste, allow the urban citizens to separate biodegradable waste from other waste, and to use their biodegradable waste, preferably after composting, as organic fertilizer on their urban arable fields (Drechsel and Kunze, 2001; Adamtey et al., 2009; McGregor et al., 2011). Such separation of waste would moreover allow the city of Kumasi to expand the lifespan of their single landfill site for a few more decades (Asase et al., 2009).
Conclusion
The outcomes of this study demonstrate that SOM contents and stocks of long-term urban arable soils in Kumasi have been maintained and even increased, compared to short-term urban arable soils. At the same time, coarse fragments (including various kinds of artificial materials) that are related to rapid urbanization have considerably accumulated in the long-term urban arable soils. The study suggests that a general notion that rapid urbanization eventually enhances soil degradation (McGregor et al., 2011) needs to be differentiated. Urban arable soils of Kumasi are different from urban soils in some other cities, which have been described as highly modified, compacted, and of poor quality that compromises ecosystem services provision (Jim, 1998; Lehmann and Stahr, 2007; Lorenz and Lal, 2009). Nevertheless, the findings in this study are in agreement with recent studies, such as those of Edmondson et al. (2014), Joimel et al. (2016), Tresch et al. (2018), which demonstrated that urban arable soils may adequately support ecosystem provision in some European cities.
In this study, direct interrelations between the SOM stocks in urban arable soils and urban household wastes in the rapidly urbanizing city of Kumasi have been identified. The obtained knowledge on these interrelations may strengthen the theoretical link between waste and soil sustainability, which is within the broader soil-waste-water nexus framework for sustainable resource management (Lal, 2013, 2014; Bouma, 2016). As illustrated by Drechsel et al. (2007), Ghanaian cities have become nutrient hubs due to the net import of crop produce from their hinterlands. The study also reconfirms conclusions of other studies, e.g., of Miezah et al. (2015) and Bouma (2016), proposing an integrated waste management system that reincorporates the imported nutrients and organic matter into the urban arable soils as a sustainable pathway to improve food security. In a broader sense, the outcomes of this research call for greater attention to urban arable soils, especially in tropical regions, where their provision of multiple ecosystem services is of utmost importance. As Kumasi is a typical rapidly urbanizing West-African city, we conclude that the outcomes of this research are also relevant to most other cities in the West-African sub-region.
Data Availability Statement
The data that is generated for this study is part of the ongoing interdisciplinary Urban-RESS project, hosted at the Physical Geography—University of Goettingen. Data supporting the conclusions of this manuscript will be made available by the corresponding author, upon request to any qualified researcher. Also, all data will be publicly available upon completion of the project.
Author Contributions
SA and DS contributed to the conception and design of the study. SA, DS, and KN organized the field campaign and sampled the soils. SA analyzed samples and organized the database. SA and TZ performed the statistical analysis. SA wrote the first draft of the manuscript. TZ, KN, and DS wrote sections of the manuscript. All authors contributed to manuscript revision, read and approved the submitted version.
Conflict of Interest Statement
The authors declare that the research was conducted in the absence of any commercial or financial relationships that could be construed as a potential conflict of interest.
Acknowledgments
We thank George Ashiagbor and Martin Manu (Faculty of Renewable Natural Resources, Kwame Nkrumah University of Science and Technology), Eric Asamoah and Alexander Owus-Ansah (Soil Research Institute, Council for Scientific and Industrial Research) for the immense support and assistance during sampling in Kumasi. We also thank Dr. Jürgen Grotheer, Petra Voigt, Anja Södje, Raffael Hearth, Johanna Diederich, and Julia Sindermann (Dept. of Physical Geography, University of Göttingen) for their assistance in the lab analysis. The corresponding author thanks the Graduate Academy of Technische Universität Dresden and the Association of Friends and Sponsors of Technische Universität Dresden for providing initial grants for this research.
References
Acheampong, R. A., Agyemang, F. S. K., and Abdul-Fatawu, M. (2017). Quantifying the spatio-temporal patterns of settlement growth in a metropolitan region of Ghana. Geo J. 82, 823–840. doi: 10.1007/s10708-016-9719-x
Adamtey, N., Cofie, O., Ofosu-Budu, G. K., Danso, S. K. A., and Forster, D. (2009). Production and storage of N-enriched co-compost. Waste Manag. 29, 2429–2436. doi: 10.1016/j.wasman.2009.04.014
Adu, S. V. (1992). Soils of the Kumasi Region, Ashanti Region, Ghana. Kwadaso-Kumasi: Soil Research Institute.
Amundson, R. (2001). The carbon budget in soils. Annu. Rev. Earth Planet. Sci. 29, 535–562. doi: 10.1146/annurev.earth.29.1.535
Anikwe, M. (2002). Long term effect of municipal waste disposal on soil properties and productivity of sites used for urban agriculture in Abakaliki, Nigeria. Bioresour. Technol. 83, 241–250. doi: 10.1016/S0960-8524(01)00154-7
Asase, M., Yanful, E. K. Mensah, M., Stanford, J., and Amponsah, S. (2009). Comparison of municipal solid waste management systems in Canada and Ghana. A case study of the cities of London, Ontario, and Kumasi, Ghana. Waste Manag. 29, 2779–2786. doi: 10.1016/j.wasman.2009.06.019
Augustin, S., Schall, P., and Schmieden, U. (1998). Modelling aspects of forest decline in Germany. I. Theoretical aspects and cause-effect relationships. Chemosphere 36, 965–970. doi: 10.1016/S0045-6535(97)10156-4
Aune, J. B., and Lal, R. (1997). Agricultural productivity in the tropics and critical limits of properties of Oxisols, Ultisols, Alfisols. Trop. Agric. 74, 96–103.
Barton, K. (2018)MuMIn: Multi-Model Inference. R package version 1.40.4. Available online at: https://CRAN.R-project.org/package=MuMIn.
Bates, D., Mächler, M., Bolker, B., and Walker, S. (2015). Fitting linear mixed-effects models using lme4. J. Stat. Softw. 67, 1–51. doi: 10.18637/jss.v067.i01
Bationo, A., Kihara, J., Vanlauwe, B., Waswa, B., and Kimetu, J. (2007). Soil organic carbon dynamics, functions and management in West African agro-ecosystems. Agric. Syst. 94, 13–25. doi: 10.1016/j.agsy.2005.08.011
Bationo, A., and Mokwunye, A. U. (1991). Role of manures and crop residue in alleviating soil fertility constraints to crop production. With special reference to the Sahelian and Sudanian zones of West Africa. Fertil. Res. 29, 117–125. doi: 10.1007/BF01048993
Bationo, A., Nandwa, S. M., Kimetu, J. M., Kinyangi, J., Bado, B. V., Lompo, F., et al. (2004). “Sustainable intensification of crop-livestock system through manure management in eastern and western Africa,” in Lessons Learned and Emerging Research Approaches. International Livestock Research Institute (ILRI). (Wageningen, NL: Technical Centre for Agricultural and Rural Cooperation (CTA)). Available online at: https://cgspace.cgiar.org/bitstream/10568/56088/1/Pg173_198%20Batiano.pdf
Baveye, P. C., Baveye, J., and Gowdy, J. (2016). Soil “ecosystem” services and natural capital: critical appraisal of research on uncertain ground. Front. Environ. Sci. 4:609. doi: 10.3389/fenvs.2016.00041
Bellwood-Howard, I., Haring, V., Karg, H., Roessler, R., Schlesinger, J., and Shakya, M. (2015). Characteristics of Urban and Peri-Urban Agriculture in West Africa: Results of an Exploratory Survey Conducted in Tamale (Ghana) and Ouagadougou (Burkina Faso) (Colombo: International Water Management Institute).
Blume, H.-P., Brümmer, G. W., Fleige, H., Horn, R., Kandeler, E., Kögel-Knabner, I., et al. (2016). Scheffer/Schachtschabel Soil Science. Heidelberg: Springer.
Blume, H.-P., Stahr, K., and Leinweber, P. (2011). “Bodenkundliches Praktikum,” in Eine Einführung in Pedologisches Arbeiten Für Ökologen, Insbesondere Land-Und Forstwirte, Und Für Geowissenschaftler, Heidelberg: Spektrum Akademischer Verlag).
Boateng, S. A., Zickermann, J., and Kornahrens, M. (2006). Poultry Manure effect on growth and yield of maize. West Afr. J. Appl. Ecol. 9, 1–11. doi: 10.4314/wajae.v9i1.45682
Bouma, J. (2016). “Implications of the nexus approach when assessing water and soil quality as a function of solid and liquid waste management,” in Environmental Resource Management and the Nexus Approach. Managing Water, Soil, and Waste in the Context of Global Change. ed H. Hettiarachchi (New York NY: Springer Berlin Heidelberg), 179–209.
Brock, C., Oberholzer, H.-R., and Franko, U. (2017). Soil organic matter balance as a practical tool for environmental impact assessment and management support in arable farming. Eur. J. Soil Sci. 68, 951–952. doi: 10.1111/ejss.12495
Byrne, L. B. (2007). Habitat structure. A fundamental concept and framework for urban soil ecology. Urban Ecosyst. 10, 255–274. doi: 10.1007/s11252-007-0027-6
Cardoso, R. B. S. M. (2014). The Fate of Contaminants in Urban Soils and Sediments. Novel Assessments and Implications for Risks. Ph.D. thesis, Manchester.
Chatterjee, A., Lal, R., Wielopolski, L., Martin, M. Z., and Ebinger, M. H. (2009). Evaluation of different soil carbon determination methods. CRC. Crit. Rev. Plant Sci. 28, 164–178. doi: 10.1080/07352680902776556
Cobbinah, P. B., and Erdiaw-Kwasie, M. O. (2016). “Urbanization in Ghana: insights and implications for urban governance,” in Population Growth and Rapid Urbanization in the Developing World, eds U. G. Benna, and S. B. Garba (Hershey, PA: IGI Global), 82–104.
Cobbinah, P. B., Erdiaw-Kwasie, M. O., and Amoateng, P. (2015). Africa's urbanisation. Implications for sustainable development. Cities 47, 62–72. doi: 10.1016/j.cities.2015.03.013
Cofie, O., Kone, D., Rothenberger, S., Moser, D., and Zubruegg, C. (2009). Co-composting of faecal sludge and organic solid waste for agriculture. Process dynamics. Water Res. 43, 4665–4675. doi: 10.1016/j.watres.2009.07.021
Cofie, O. O., Van Veenhuizen, R., and Drechsel, P. (2003). “Contribution of urban and peri-urban agriculture to food security in sub-Saharan Africa,” Africa Day of the 3rd WWF in Kyoto, 17–3. Available online at: https://pdfs.semanticscholar.org/4933/b466f8b661890d422622ade396361d0e7289.pdf
Corti, G., Ugolini, F. C., Agnelli, A., Certini, G., Cuniglio, R., Berna, F., et al. (2002). The soil skeleton, a forgotten pool of carbon and nitrogen in soil. Eur. J. Soil Sci. 53, 283–298. doi: 10.1046/j.1365-2389.2002.00442.x
Davies, B. E. (1974). Loss-on-ignition as an estimate of soil organic matter1. Soil Sci. Soc. Am. J. 38:150. doi: 10.2136/sssaj1974.03615995003800010046x
Dicko, G. (2016). Soil Carbon Change and CO2 Fluxes Under Different Agricultural Land Use in the Vea Catchment, Upper East Region of Ghana. Doctoral, Kumasi, Ghana.
Drechsel, P., and Dongus, S. (2010). Dynamics and sustainability of urban agriculture. Examples from sub-Saharan Africa. Sustain. Sci. 5, 69–78. doi: 10.1007/s11625-009-0097-x
Drechsel, P. editor, (2002). Comparing Soil Nutrient Depletion in Typical Urban, Peri-Urban and 783 Rural Farming Systems in Ghana. Bangkok: International Water Management Institute.
Drechsel, P., Graefe, S., and Fink, M. (2007). Rural-urban food, nutrient and virtual water flows in selected West African cities. Water Manag. 115, 1–39. doi: 10.3910/2009.115
Drechsel, P., and Kunze, D. (2001). “Waste composting for urban and peri-urban agriculture,” in Closing the Rural-Urban Nutrient Cycle in Sub-Saharan Africa eds P. Drechsel, D. Kunze (Wallingford; Columbo: CABI Pub; International Water Management Institute).
Duah-Yentumi, S., Rønn, R., and Christensen, S. (1998). Nutrients limiting microbial growth in a tropical forest soil of Ghana under different management. Appl. Soil Ecol. 8, 19–24. doi: 10.1016/S0929-1393(97)00070-X
Edmondson, J. L., Davies, Z. G., Gaston, K. J., and Leake, J. R. (2014). Urban cultivation in allotments maintains soil qualities adversely affected by conventional agriculture. J. Appl. Ecol. 51, 880–889. doi: 10.1111/1365-2664.12254
Edmondson, J. L., Davies, Z. G., McCormack, S. A., Gaston, K.J, and Leake, J. R. (2011). Are soils in urban ecosystems compacted? A citywide analysis. Biol. Lett. 7, 771–774. doi: 10.1098/rsbl.2011.0260
FAO. Guidelines for Soil Description 4th Edn. Rome: Food and Agriculture Organization of the United Nations. (2006).
Fuseini, I., and Kemp, J. (2016). Characterising urban growth in Tamale, Ghana. An analysis of urban governance response in infrastructure and service provision. Habit. Int. 56, 109–123. doi: 10.1016/j.habitatint.2016.05.002
Ghana Statistical Service (2014). 2010 Population and Housing Census. District Analytical Report. Accra: Kumasi metropolitan.
Hazelton, P. A., and Murphy, B. W. (2016). Interpreting Soil Test Results. What Do All the Numbers Mean? South VIC, Australia: CSIRO Publishing, Clayton.
Heitkamp, F., Jacobs, A., Jungkunst, H. F., Heinze, S., Wendland, M., and Kuzyakov, Y. (2012). “Processes of soil carbon dynamics and ecosystem carbon cycling in a changing world,” in Recarbonization of the Biosphere. Ecosystems and the Global Carbon Cycle, eds R. Lal, K. Lorenz, R F. Hüttl, B. U. Schneider, and J. von Braun (Dordrecht: Springer), 395–428
Hothorn, T., Hornik, K., and Zeileis, A. (2006). Unbiased recursive partitioning. A conditional inference framework. J. Comput. Graph. Stat. 15, 651–674. doi: 10.1198/106186006X133933
Imperato, M., Adamo, P., Naimo, D., Arienzo, M., Stanzione, D., and Violante, P. (2003). Spatial distribution of heavy metals in urban soils of Naples city (Italy). Environ. Pollut. 124, 247–256. doi: 10.1016/S0269-7491(02)00478-5
ISO 10390 (2005). Soil Quality – Determination of pH. Geneva: International Organization for Standardization.
IUSS Working Group WRB (2014). World Reference Base for Soil Resources 2014. International Soil Classification System for Naming Soils and Creating Legends for Soil Maps. Rome: FAO.
Jim, C. Y. (1998). Urban soil characteristics and limitations for landscape planting in Hong Kong. Landsc. Urban Plan. 40, 235–249. doi: 10.1016/S0169-2046(97)00117-5
Joimel, S., Cortet, J., Jolivet, C. C., Saby, N. P. A., Chenot, E. D., Branchu, P., et al. (2016). Physico-chemical characteristics of topsoil for contrasted forest, agricultural, urban and industrial land uses in France. Sci. Total Environ. 545–546, 40–47. doi: 10.1016/j.scitotenv.2015.12.035
Kirschbaum, M. U. F. (2000). Will changes in soil organic carbon act as a positive or negative feedback on global warming? Biogeochemistry 48, 21–51. doi: 10.1023/A:1006238902976
Labrière, N., Locatelli, B., Laumonier, Y., Freycon, V., and Bernoux, M. (2015). Soil erosion in the humid tropics. A systematic quantitative review. Agric. Ecosyst. Environ. 203, 127–139. doi: 10.1016/j.agee.2015.01.027
Lal, R. (2004). Soil carbon sequestration impacts on global climate change and food security. Science 304, 1623–1627. doi: 10.1126/science.1097396
Lal, R. (2013). Climate-strategic agriculture and the water-soil-waste nexus. J. Plant Nutr. Soil Sci. 176, 479–493. doi: 10.1002/jpln.201300189
Lal, R. (2014). “The nexus approach to managing water, soil and waste under changing climate and growing demands on natural resources,” in Governing the Nexus, eds M. Kurian, and R. Ardakanian (New York NY: Springer), 39–60
Lehmann, A., and Stahr, K. (2007). Nature and significance of anthropogenic urban soils. J. Soils Sediments 7, 247–260. doi: 10.1065/jss2007.06.235
Liu, R., Wang, M., and Chen, W. (2018). The influence of urbanization on organic carbon sequestration and cycling in soils of Beijing. Landsc. Urban Plan. 169, 241–249. doi: 10.1016/j.landu
Lopez-Ulloa, R. M. (2006). Soil Ecosystem Services in Different Land Use Types in Coastal Ecuador. Ph.D. thesis, Göttingen.
Lorenz, K., and Kandeler, E. (2005). Biochemical characterization of urban soil profiles from Stuttgart, Germany. Soil Biol. Biochem. 37, 1373–1385. doi: 10.1016/j.soilbio.2004.12.009
Lorenz, K., and Lal, R. (2009). Biogeochemical C and N cycles in urban soils. Environ. Int. 35, 1–8. doi: 10.1016/j.envint.2008.05.006
Lorenz, K., Preston, C. M., and Kandeler, E. (2006). Soil organic matter in urban soils. Estimation of elemental carbon by thermal oxidation and characterization of organic matter by solid-state 13C nuclear magnetic resonance (NMR) spectroscopy. Geoderma 130, 312–323. doi: 10.1016/j.geoderma.2005.02.004
Magdoff, F., and Weil, R. R. (2004). Soil Organic Matter in Sustainable Agriculture. Boca Raton, FL: CRC Press.
McGregor, D. F. M., Adam-Bradford, A., Thompson, D., and Simon, D. (2011). Resource management and agriculture in the periurban interface of Kumasi, Ghana. Problems and prospects. Singap. J. Trop. Geogr. 32, 382–398. doi: 10.1111/j.1467-9493.2011.00438.x
Miezah, K., Obiri-Danso, K., Kádár, Z., Fei-Baffoe, B., and Mensah, M. Y. (2015). Municipal solid waste characterization and quantification as a measure towards effective waste management in Ghana. Waste Manag. 46, 15–27. doi: 10.1016/j.wasman.2015.09.009
Minta, P. (1998). Effect of Mucuna Pruriens Mulch on the Growth and Yield of Maise (Zea mays L.). Master thesis, Accra, Ghana.
Post, W. M., Pastor, J., Zinke, P. J., and Stangenberger, A. G. (1985). Global patterns of soil nitrogen storage. Nature 317, 613–616. doi: 10.1038/317613a0
Pouyat, R., Groffman, P., Yesilonis, I., and Hernandez, L. (2002). Soil carbon pools and fluxes in urban ecosystems. Environ. Pollut. 116(Suppl. 1), S107–S118. doi: 10.1016/S0269-7491(01)00263-9
Pouyat, R. V., Szlavecz, K., Yesilonis, I. D., Groffman, P. M., and Schwarz, K. (2010). “Chemical, physical and biological characteristics of urban soils,” in Urban Ecosystem Ecology eds J. Aitkenhead-Peterson, A. Volder (Madison WI: American Society of Agronomy; Crop Science Society of America; Soil Science Society of America), 119–152. doi: 10.2134/agronmonogr55.c7
Pribyl, D. W. (2010). A critical review of the conventional SOC to SOM conversion factor. Geoderma 156, 75–83. doi: 10.1016/j.geoderma.2010.02.003
Prudencio, C. Y. (1993). Ring management of soils and crops in the West Ring management of soils and crops in the West African semi-arid tropics: the case of the Mossi farming system in Burkina Faso. Agric. Ecosyst. Environ. 47, 237–264. doi: 10.1016/0167-8809(93)90125-9
Quansah, G. W. (2010). Effect of Organic and Inorganic Fertilisers and Their Combinations on the Growth and Yield of Maize in the Semi-Deciduous Forest Zone of Ghana. Master thesis, Kumasi, Ghana.
R. Core Team (2017). R: A Language and Environment for Statistical Computing. Vienna: R Foundation for Statistical Computing.
Sanchez, P. A., Shepherd, K. D., Soule, M. J., Place, F. M., Buresh, R. J., Izac, A-MN, et al. (1997). “Soil fertility replenishment in Africa: an investment in natural resource capital,” in Replenishing Soil Fertility in Africa, 51st edn, eds P. A. S. Roland, J. Buresh, and F. Calhoun (Wisconsin, WI: Soil Science Society of America and American Society of Agronomy), 1–46.
Sauer, D., and Burghardt, W. (2006). The occurrence and distribution of various forms of silica and zeolites in soils developed from wastes of iron production. Catena 65, 247–257. doi: 10.1016/j.catena.2005.11.017
Schipper, L. A., Percival, H. J., and Sparling, G. P. (2004). An approach for estimating when soils will reach maximum nitrogen storage. Soil Use Manag. 20, 281–286. doi: 10.1079/SUM2004255
Schleuß, U., Wu, Q., and Blume, H.-P. (1998). Variability of soils in urban and periurban areas in Northern Germany. Catena 33, 255–270. doi: 10.1016/S0341-8162(98)00070-8
Smetak, K. M., Johnson-Maynard, J. L., and Lloyd, J. E. (2007). Earthworm population density and diversity in different-aged urban systems. Appl. Soil Ecol. 37, 161–168. doi: 10.1016/j.apsoil.2007.06.004
Stoorvogel, J. J., and Smaling, E. M. A. (1990). Assessment of soil nutrient depletion in Sub-Saharan Africa, 1983–2000. Report 28. The Winand Staring Centre for Integrated Land, Soil and Water Research. Wageningen.
Stoorvogel, J. J., Smaling, E. M. A., and Janssen, B. H. (1993). Calculating soil nutrient balances in Africa at different scales. Fertil. Res. 35, 227–235. doi: 10.1007/BF00750641
Stow, D. A., Weeks, J. R., Shih, H.-C., Coulter, L. L., Johnson, H., Tsai, H.-Y., et al. (2016). Inter-regional pattern of urbanization in southern Ghana in the first decade of the new millennium. Appl. Geogr. 71, 32–43. doi: 10.1016/j.apgeog.2016.04.006
Tresch, S., Moretti, M., Le Bayon, R.-C., Mäder, P., Zanetta, A., Frey, D., et al. (2018). A Gardener's influence on urban soil quality. Front. Environ. Sci. 6:605. doi: 10.3389/fenvs.2018.00025
Vermeiren, K., Adiyia, B., Loopmans, M., Tumwine, F. R., and van Rompaey, A. (2013). Will urban farming survive the growth of African cities. A case-study in Kampala (Uganda)? Land Use Policy 35, 40–49. doi: 10.1016/j.landusepol.2013.04.012
Vuong, T. X., Heitkamp, F., Jungkunst, H. F., Reimer, A., and Gerold, G. (2013). Simultaneous measurement of soil organic and inorganic carbon. Evaluation of a thermal gradient analysis. J. Soils Sediments 13, 1133–1140. doi: 10.1007/s11368-013-0715-1
Windmeijer, P. N., and Andriesse, W. (1993). Inland Valleys in West Africa: An Agro-Ecological Characterization of Rice-Growing Environments. Wageningen: International Institute for Land Reclamation and Improvement.
Yeboah, E., and Obeng-Odoom, F. (2010). We are not the only ones to blame. District Assemblies' perspectives on the state of planning in Ghana. Commonw. J. Local Govern. 1996, 78–98. doi: 10.5130/cjlg.v0i7.1893
Zingore, S., Delve, R. J., Nyamangara, J., and Giller, K. E. (2008). Multiple benefits of manure. The key to maintenance of soil fertility and restoration of depleted sandy soils on African smallholder farms. Nutr. Cycl. Agroecosyst. 80, 267–282. doi: 10.1007/s10705-007-9142-2
Keywords: urbanization, Ghana, West Africa, tropical soils, urban farming, soil organic matter, soil pH, household waste
Citation: Asabere SB, Zeppenfeld T, Nketia KA and Sauer D (2018) Urbanization Leads to Increases in pH, Carbonate, and Soil Organic Matter Stocks of Arable Soils of Kumasi, Ghana (West Africa). Front. Environ. Sci. 6:119. doi: 10.3389/fenvs.2018.00119
Received: 08 May 2018; Accepted: 24 September 2018;
Published: 12 October 2018.
Edited by:
Sophie Cornu, INRA Centre Provence-Alpes-Côte d'Azur, FranceReviewed by:
Beatrice Bechet, Institut Français des Sciences et Technologies des Transports, de l'Aménagement et des Réseaux (IFSTTAR), FranceAna Teresa Lima, Universidade Federal do Espírito Santo, Brazil
Copyright © 2018 Asabere, Zeppenfeld, Nketia and Sauer. This is an open-access article distributed under the terms of the Creative Commons Attribution License (CC BY). The use, distribution or reproduction in other forums is permitted, provided the original author(s) and the copyright owner(s) are credited and that the original publication in this journal is cited, in accordance with accepted academic practice. No use, distribution or reproduction is permitted which does not comply with these terms.
*Correspondence: Stephen Boahen Asabere, c2FzYWJlckB1bmktZ29ldHRpbmdlbi5kZQ==