- 1Villanova University, Villanova, PA, United States
- 2Department of Biogeochemistry, Plant and Microbial Ecology, Instituto de Recursos Naturales y Agrobiologia de Sevilla, IRNAS-CSIC, Seville, Spain
Halogenated compounds are environmental pollutants toxic to humans and wildlife. Certain microorganisms degrade these halogenated compounds. However, little is known about the potential of microorganisms in bioremediation under extreme conditions, specifically in arid and semi-arid soils frequently exposed to high temperatures and desiccation periods. Arid and semi-arid environments and deserts make up vast areas of Earth's landmass. To investigate the degradation of 2-chlorophenol (2-CP) in soils as a function of temperature and water availability, three bacterial species were tested, two soil mesophiles of the genus Rhodococcus, R. opacus and R. erythropolis, and a soil thermophilic isolate, Parageobacillus thermoglucosidasius. Degradation trials in soil samples with these species were performed over a range of water activity from 1 to 0.4. At their optimum growth temperature, R. opacus showed maximum 2-CP degradation at water activity 0.9 sharply decreasing when lowering water activity. Nevertheless, the Parageobacillus isolate (optimum growth temperature 60°C) showed maximum 2-CP degradation rates at water activity 0.5 which represented highly desiccating conditions. Parageobacillus degradation of 2-CP was very low at water activity above 0.9. Thus, biodegradation of 2-CP in soils is possible even under arid conditions although different microbial species might be involved in this task depending on the interactions of abiotic factors and the diversity of microbial communities in soils. These results contribute to understand the potential biodegradation of specific halogenated compounds in the environment which is of great relevance to comprehend the fate of halogenated pollutants (i.e., 2-CP) in deserts, arid and semi-arid soils.
Introduction
Halogenated compounds, such as chlorophenols, represent important environmental pollutants. Chlorophenols are released into the environment through anthropogenic activities, for instance by their use in pesticides, herbicides, their presence in industrial wastes (Arora and Bae, 2014) and also as a result of organic matter combustion and biological chlorination (Ahling and Lindskog, 1982). Chlorophenols can adhere onto particulate organic matter persisting in the environments for extended periods of time and leading to accumulation in the organisms through different trophic levels. Understanding the fate of chlorophenols in nature is complex and it is certainly the result of an interplay of biotic and abiotic processes.
The biological degradation of chlorophenols has been studied (Arora and Bae, 2014) and several microorganisms have been reported to be able to mineralize chlorophenols (Arora and Bae, 2014). The extent to what these microorganisms could perform chlorophenol degradation in the natural environment remains to be fully understood. In addition, the influence and effect of multiple abiotic factors needs to be added to our comprehension of chlorophenol degradation to be able to provide a knowledgeable management of bioremediation procedures and models on the environmental fate of chlorophenol.
Soils are highly heterogeneous environments (Or et al., 2007) holding a huge microbial diversity (Curtis et al., 2002). As a consequence, following the fate of chlorophenols in these environments is a complex task. Environmental factors, such as temperature and water content, are important contributors to soil heterogeneity and changes in microbial activity (Manzoni et al., 2012) which must directly influence soil biodegradation of chlorophenols. Nevertheless, the influence of these factors and their interplay with specific chlorophenol-degrading microorganisms remains to be understood.
Meteorological factors can influence the functioning and mechanisms occurring at upper soil layers. For instance, high temperature and sun exposure can result in elevated temperature measurements at upper soil layers (Portillo et al., 2012), which implies the activation of soil thermophilic bacteria (Portillo et al., 2012), and, consequently, cause a switch on the functionally dominant bacteria and metabolic processes (Portillo et al., 2012; Santana and Gonzalez, 2015). This scenario can have important consequences both at local and global scales (Santana and Gonzalez, 2015). In addition, high temperature events imply increased evaporation leading to soils with low water availability, desiccation and aridity. Temperature and water content are intimately related at upper soil layers and could be implicated in important consequences on the behavior of soil microbial communities (Manzoni et al., 2012; Biederman et al., 2016), including their potential for biodehalogenation. There is a current gap in our knowledge on the influence of these factors (temperature and water availability) on the microbial potential to decompose chlorophenols.
The extension of landmass assigned to deserts, arid and semi-arid ecosystems represents a major area of terrestrial Earth surface located mostly at medium and low latitudes (Portillo et al., 2012; Santana and Gonzalez, 2015). Many of the arid-classified sites are potential targets for halogenated compound disposal in the field because these lands frequently belong to under-developed countries or present relatively low inhabiting human population. In this scenario, understanding the fate of chlorophenols in dry terrestrial environments is an important concern to envision if these pollutants are to be decomposed or will long persist in these environments.
This study will focus on 2-chlorophenol (2-CP) as a case study of biodehalogenation to better understand the fate of this pollutant in soils. We directed special attention to the effect of temperature and desiccating conditions on the fate of 2-CP in the environment. The aim of this study is to assess the influence of high temperature and low water content on the differential removal of 2-CP by distinct soil bacteria as a first approach to understand the potential bioremediation of halogenated pollutants, above all, in arid and dry soils.
Materials and Methods
Bacterial Strains and Soil Samples
Two mesophilic (optimum growth temperature 28°C), 2-CP-degrading strains of the genus Rhodococcus, R. opacus IG (DSM 43205) (Klatte et al., 1994) and R. erythropolis DSM 43066 (Goodfellow and Anderson, 1977), were used in this study as soil isolates previously evaluated for their ability to degrade 2-CP (Bondar et al., 1999; Goswami et al., 2002; Arora and Bae, 2014). These strains were grown in medium 535 (Klatte et al., 1994) as recommended by the DSMZ (German Collection of Microorganisms and Cell Cultures) which composition (per liter) included: pancreatic digest of casein (17.0 g), enzymatic digest of soy bean (3.0 g), sodium chloride (5.0 g), dipotasium hydrogen phosphate (2.5 g), and glucose (2.5 g). Medium 535 was adjusted to pH 7.3. In addition, a soil thermophilic isolate (optimum growth temperature 60°C), P. thermoglucosidasius strain 23.6 (previous Geobacillus) (Aliyu et al., 2016) was a representative of the ubiquitous thermophilic bacterial community detected in soils (Marchant et al., 2002; Portillo et al., 2012) and grown in Nutrient Broth which contains (per liter): beef extract (5.0 g), peptone (10.0 g), and sodium chloride (5.0 g). Nutrient Broth was adjusted to pH 7.2 at 60°C. Strain 23.6 was confirmed to decompose 2-CP in liquid medium.
Soil samples were collected at Coria del Rio (Sevilla, Spain) at the experimental agricultural area “La Hampa” (IRNAS-CSIC) which soil characteristics have been previously reported (Cardoso de Barros, 1996).
Biodegradation of 2-Chlorophenol
Most studies have performed estimates of dehalogenation in aqueous solution. Unlike them, herein, we present a more realistic analysis by quantifying decomposition of a halogenated compound (i.e., 2-CP) in soils considering its high physical and chemical heterogeneity. The procedure allows to evaluate decomposition of 2-CP (and similarly other halogenated pollutants) at different water availabilities (i.e., water activity). To evaluate the decomposition of 2-CP over time by the studied strains under realistic soil conditions, 400 mg of autoclaved soil (3 consecutive days each at 121°C for 20 min) were supplemented with 2-CP (50 μl, 0.5 g/l) and 70 μl of a culture at its exponential phase of growth. Controls lack the addition of cells which were replaced by the same volume of sterile distilled water. The soil plus cell mixtures were desiccated in a vacuum concentrator (Micro-Cenvac, N-Biotek, Gyeonggi-do, South Korea) to obtain the desired water activity (aw). Once the desired aw was obtained, the samples (in triplicates) were placed in an incubator to the required growth temperature (either 20 or 60°C for mesophiles or thermophiles, respectively). Over time, samples were collected at 0, 4, 8, 24, and 72 h incubation to determine the concentration of 2-CP in the soil mixtures.
Water activity represents the water available for microorganisms and it is defined as the partial vapor pressure of water in a sample divided by the partial vapor pressure of pure water, and it ranges from 0 (no water) to 1 (water). The aw was determined using a water activity probe (Rotronic AG, Bassersdorf, Switzerland). The soil sample to be determined was placed in the temperature controlled probe chamber to perform the measurement following the manufacturers' recommendations. As a reference, the minimum aw that allows the growth of a microorganism (the fungus Xeromyces bisporus) is >0.63 (Stevenson et al., 2015).
Quantification of 2-CP was carried out by HPLC (Agilent Infinity 1260; Agilent, Santa Clara, California, USA) using a mobile phase consisting on Acetonitrile:Water (70:30), and an injection volume of 10 μl through a column Agilent Zorbax Eclipse Plus C18 (Agilent, USA) at 35°C. Soil aliquots collected over time were supplemented with 1 ml acetonitrile to dissolve 2-CP, sonicated at 40°C for 15 min and vortex at room temperature for additional 15 min. The suspension was centrifuged at 8,000 × g for 4 min. The supernatant was collected and filtered through a 0.2 μm-pore-diameter filter. The extracted solution was injected in the described HPLC system and 2-CP quantified by peak integration after absorbance measurement at 273 nm (Goswami et al., 2002). 2-CP quantifications over time were used to estimate the decomposition rate (per hour) through the linear portion of the decay curve (Figure 1). These decomposition rates were estimated by linear regression (Sokal and Rohlf, 1995). All experiments were carried out in independent triplicates and the averages and standard deviations are presented.
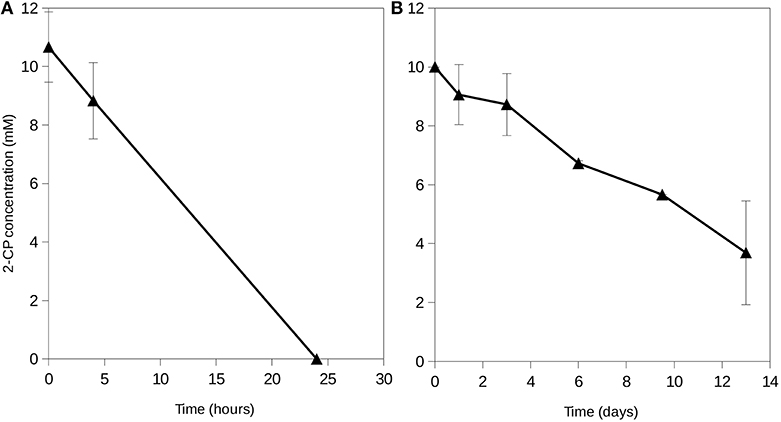
Figure 1. Examples of the decay curves of 2-CP decomposition over time by Rhodococcus opacus (A; at aw 0.93) and Parageobacillus thermoglucosidasius (B; at aw 0.96) in soil samples.
Results and Discussion
Understanding the functioning of soil ecosystems under a variety of environmental conditions is a requirement for a proper management of soils. Soils can be used as a source for economical benefit and food products as well as a potential site for the biodecomposition of human activity pollutants. Soils, and specifically, semi-arid and arid soils, are potential targets for dumping halogenated pollutants. Their environmental risks are high and it is of most importance to understand when and how the biodecomposition of 2-CP (as well as other halogenated pollutants) occurs in these soils.
Environmental conditions, such as high temperature and dry periods, affect soil upper layers. Besides, these upper layers are the most influenced by pollutants. However, the range of environmental conditions allowing bacteria to biodegrade halogenated compounds remains to be studied. Herein, we approach the biodegradation of 2-CP in a soil by different soil bacteria under a variety of temperature and water availability conditions commonly observed in natural soils.
The analysis of the capability of Rhodococcus species to decompose 2-CP in natural soils revealed that these soil mesophiles are only able to carry out efficient biodegradation of 2-CP under soggy and temperate conditions (Figure 2). Nevertheless, soil upper layers frequently reach high temperatures (e.g., up to 75°C during summer in Southern Spain and over 90°C at desserts) (McCalley and Sparks, 2009; Portillo et al., 2012) and consequently, their water availability is highly reduced, reaching highly desiccating values. Under these frequently encountered soil conditions, it is unknown if bacteria can carry out the process of decomposition of halogenated pollutants in soils and which bacteria and under which conditions this could be processed. Common 2-CP decomposing soil bacteria, such as R. opacus and R. erythropolis, are unable to carry out efficient decomposition of 2-CP in dry soils and at high soil temperatures (Figure 2).
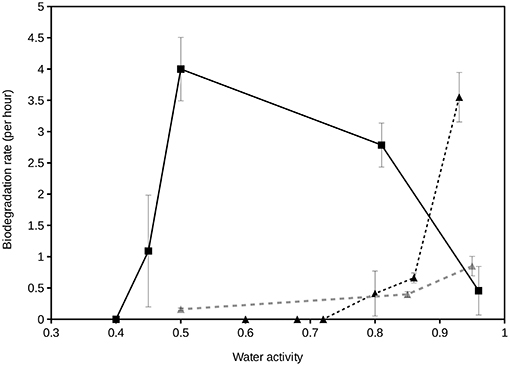
Figure 2. Biodegradation of 2-CP in soil as a function of water activity for three bacterial species: a thermophilic Parageobacillus thermoglucosidasius (black continuous line and squares), and two mesophiles, Rhodococcus opacus (black discontinuous line and triangles) and R. erythropolis (gray discontinuous line and triangles). Note that the optimum 2-CP decomposition rate for the thermophilic species, P. thermoglucosidasius, was observed at water activity 0.5 (corresponding to a quite dry soil). For Rhodococcus, the optimum 2-CP decomposition rate occurred under soggy conditions, at water activity >0.9. Error bars represent one standard deviation.
Nevertheless, our results have shown that common soil thermophilic bacteria (i.e., Parageobacillus) (Portillo et al., 2012; Santana and Gonzalez, 2015; Aliyu et al., 2016) are capable of efficiently biodecompose 2-CP under high temperature and dry conditions commonly observed at upper soil layers (Figure 2). This suggests that thermophilic bacteria could replace mesophiles during hot soil periods. In fact, soils can get frequently hot at medium and low latitudes which is coincident with those land areas on Earth most exposed to high temperatures (Gonzalez et al., 2015; Santana and Gonzalez, 2015). These results confirm that under arid conditions, some soil bacteria can continue the biodegradation process of halogenated pollutants (i.e., 2-CP) and so these polluted soils can be bioremediated even under extreme temperature and water scarcity conditions.
Previous reports have indicated that thermophilic bacteria are ubiquous in soils (Marchant et al., 2002; Portillo et al., 2012). This presence of thermophilic bacteria in soils has explained the observation that maximum enzymatic hydrolytic activities in soils always occur at high temperatures (Gonzalez et al., 2015) and this has been related to soils with reduced organic matter content (Santana and Gonzalez, 2015). Those results represented previous evidence on the great potential of soil thermophiles in soils functioning and, definitively, in soil health (Marchant et al., 2002; Portillo et al., 2012; Santana and Gonzalez, 2015; Wong et al., 2015), even on their potential for dechlorination (Larsen et al., 1991) and the decomposition of recalcitrant hydrocarbons (Wong et al., 2015) in soils. Consequently, soil microbial communities present a great dynamism so that different species can be temporarily replaced depending on the environmental conditions that soils experience. That dynamism and high diversity of microbial soil communities represent the basis to maintain healthy and well-functioning soil ecosystems. These are important aspects to be considered when analyzing local and global consequences of natural microbial communities in response to the variable environmental conditions existing in our planet. Consequently, the above represent critical factors to understand soil bioremediation processes as a function of environmental parameters and under a current climate change scenario.
Author Contributions
EM executed the experiment in collaboration with EP-F and EG. JG proposed the project and, in collaboration with EP-F and EG, designed and worked out the new methodology. JG prepared the manuscript and provided funding.
Conflict of Interest Statement
The authors declare that the research was conducted in the absence of any commercial or financial relationships that could be construed as a potential conflict of interest.
Acknowledgments
This study was supported through funding from the Spanish Ministry of Economy and Competitiveness (CGL2014-58762-P; PCIN2016-129) and the Regional Government of Andalusia (RNM2529). These projects have been cofunded by FEDER funds. EM acknowledges funding through a Fullbright fellowship. We acknowledge support of the publication fee by the CSIC Open Access Publication Support Initiative through its Unit of Information Resources for Research (URICI).
References
Ahling, B., and Lindskog, A. (1982). “Emissions of chlorinated organic substances from combustion,” in Chlorinated dioxins and Related Compounds. Impact on the Environment, eds O. Hutzinger, R.W. Frei, E. Merian, and F. Pocchiari (Oxford: Pergamon Press), 215–225.
Aliyu, H., Lebre, P., Blom, J., Cowan, D., and De Maayer, P. (2016). Phylogenomic re-assessment of the thermophilic genus Geobacillus. Syst. Appl. Microbiol. 39, 527–533. doi: 10.1016/j.syapm.2016.09.004
Arora, P. K., and Bae, H. (2014). Bacterial degradation of chlorophenols and their derivatives. Microb. Cell Factor. 13, 1–17. doi: 10.1186/1475-2859-13-31
Biederman, J. A., Scott, R. L., Goulden, M. L., Vargas, R., Litvak, M. E., Kolb, T. E., et al. (2016). Terrestrial carbon balance in a drier world: the effects of water availability in southwestern North America. Global Change Biol. 22, 1867–1879 doi: 10.1111/gcb.13222
Bondar, V. S., Boersma, M. G., van Berkel, W. J., Finkelstein, Z. I., Golovlev, E. L., Baskunov, B. P., et al. (1999). Preferential oxidative dehalogentation upon conversion of 2-halophenols by Rhodococcus opacus 1G. FEMS Microbiol. Lett. 181, 73–82. doi: 10.1111/j.1574-6968.1999.tb08828.x
Cardoso de Barros, J. M. (1996). Quantitative Analysis of Selected Land-Use Systems With Sunflower. Ph.D. thesis, Wageningen Agricultural University, Wageningen.
Curtis, T. P., Sloan, W. T., and Scannell, J. W. (2002). Estimating prokaryotic diversity and its limits. Proc. Natl. Acad. Sci. U.S.A. 99, 10494–10499 doi: 10.1073/pnas.142680199
Gonzalez, J. M., Portillo, M. C., and Piñeiro-Vidal, M. (2015). Latitude-dependent underestimation of microbial extracellular enzyme activity in soils. Int. J. Environ. Sci. Technol. 12, 2427–2434. doi: 10.1007/s13762-014-0635-7
Goodfellow, M., and Anderson, G. (1977). The actinomycete genus Rhodococcus: a home for the ‘rhodochrous' complex. J. General Microbiol. 100, 99–122.
Goswami, M., Shivaraman, N., and Singh, R. P. (2002). Kinetics of chlorophenol degradation by benzoate-induced culture of Rhodococcus erythropolis M1. World J. Microbiol. Biotechnol. 18, 779–783. doi: 10.1023/A:1020429109524
Klatte, S., Kroppenstedt, R. M., and Rainey, F. A. (1994). Rhodococcus opacus sp.nov., an unusual nutritionally versatile Rhodococcus-species. Syst. Appl. Microbiol. 17, 355–360. doi: 10.1016/S0723-2020(11)80051-2
Larsen, S., Hendriksen, H. V., and Ahring, B. K. (1991). Potential for thermophilic (50°C) anaerobic dechlorination of pentachlorophenol in different ecosystems. Appl. Environ. Microbiol. 57, 2085–2090.
Manzoni, S., Schimel, J. P., and Porporato, A. (2012). Responses of soil microbial communities to water stress:results from meta-analysis. Ecology 93, 930–938 doi: 10.1890/11-0026.1
Marchant, R., Banat, I. M., Rahman, T. J., and Berzano, M. (2002). The frequency and characteristics of highly thermophilic bacteria in cool soil environments. Environ. Microbiol. 4, 595–602. doi: 10.1046/j.1462-2920.2002.00344.x
McCalley, C. K., and Sparks, J. P. (2009). Abiotic gas formation drives nitrogen loss from a desert ecosystem. Science 326, 837–840. doi: 10.1126/science.1178984
Or, D., Smets, B. F., Wraith, J. M., Dechesn, A., and Friedman, S. P. (2007). Physical constraints affecting bacterial habitats and activity in unsaturated porous media – a review. Adv. Water Resourc. 30, 1505–1527. doi: 10.1016/j.advwatres.2006.05.025
Portillo, M. C., Santana, M., and Gonzalez, J. M. (2012). Presence and potential role of thermophilic bacteria in temperate terrestrial environments. Naturwissenschaften 99, 43–53. doi: 10.1007/s00114-011-0867-z
Santana, M. M., and Gonzalez, J. M. (2015). High temperature microbial activity in upper soil layers. FEMS Microbiol. Lett. 362:fnv182. doi: 10.1093/femsle/fnv182
Stevenson, A., Cray, J. A., Williams, J. P., Santos, R., Sahay, R., Neuenkirchen, N., et al. (2015). Is there a common water-activity limit for the three domains of life? ISME J. 9, 1333–1351. doi: 10.1038/ismej.2014.219
Keywords: 2-chlorophenol, temperature, water availability, desiccation, soil, biodegradation Rhodococcus, Parageobacillus, bioremediation
Citation: Moxley E, Puerta-Fernández E, Gómez EJ and Gonzalez JM (2019) Influence of Abiotic Factors Temperature and Water Content on Bacterial 2-Chlorophenol Biodegradation in Soils. Front. Environ. Sci. 7:41. doi: 10.3389/fenvs.2019.00041
Received: 17 January 2019; Accepted: 14 March 2019;
Published: 04 April 2019.
Edited by:
Ethel Eljarrat, Institute of Environmental Assessment and Water Research (CSIC), SpainReviewed by:
Gulnihal Ozbay, Delaware State University, United StatesJean Challacombe, Colorado State University, United States
Copyright © 2019 Moxley, Puerta-Fernández, Gómez and Gonzalez. This is an open-access article distributed under the terms of the Creative Commons Attribution License (CC BY). The use, distribution or reproduction in other forums is permitted, provided the original author(s) and the copyright owner(s) are credited and that the original publication in this journal is cited, in accordance with accepted academic practice. No use, distribution or reproduction is permitted which does not comply with these terms.
*Correspondence: Juan M. Gonzalez, am1ncmF1QGlybmFzZS5jc2ljLmVz