- 1Museum National d'Histoire Naturelle, PATRINAT, Paris, France
- 2Instituto Español de Oceanografía (IEO), Málaga, Spain
- 3Carl Sagan Center, SETI Institute, Mountain View, CA, United States
- 4NASA Ames Research Center, Moffett Field, CA, United States
- 5Centro de Astrobiología (CSIC-INTA), Madrid, Spain
- 6Department of Astronomy, Cornell University, Ithaca, NY, United States
- 7Centre for Marine Biodiversity and Biotechnology, Heriot-Watt University, Edinburgh, United Kingdom
- 8Faculty of Marine Sciences, King Abdulaziz University, Jeddah, Saudi Arabia
- 9Marine Conservation International, Edinburgh, United Kingdom
- 10Department of Ecology and Geology, University of Málaga, Málaga, Spain
- 11Laboratorio de Química de Productos Naturales, Facultad de Ciencias Naturales y Oceanográficas, Universidad de Concepción, Concepción, Chile
- 12Centro de Biotecnología, Universidad Católica de Norte, Antofagasta, Chile
- 13Campoalto Operaciones SpA, Santiago, Chile
- 14Ocean Technology and Engineering, National Oceanography Centre, Southampton, United Kingdom
On our planet, aqueous environments such as deep sea or high-altitude aphotic lakes, subject to present or past volcanic activity and active deglaciation, may provide analogs to the aqueous environments found on such planetary bodies as Europa, Titan or Enceladus. We report here on the methodologies and technologies tested in Laguna Negra, a high altitude lake in the Central Andes, Chile, for exploring and assessing the presence of life within planetary lakes or interior oceans. We adopted a multi-parametric Rapid Ecological Assessment (REA) approach centered around collecting video imagery (by an Underwater Imaging System) and sampling benthic sediments (for sedimentological, biological and geochemical analysis) to depths of 272 m, to complement physico-chemical sampling of the water column and collection of shallow sediments for microbiological analysis (reported in separate publications). This enabled us to classify and assess the apparent status of benthic habitats, based on substrata and environmental characteristics, together with floral and faunal community characteristics and bioturbation artifacts. Video imagery showed that the lower water column was characterized by a variably intense sestonic flux of particles and debris, among which were planktonic organisms such as ostracods, copepods, and possibly cladocerans. Sediment analysis revealed at all depths abundant diatom frustules, mainly of an acidophile pennade diatom Pinnularia acidicola, amid vegetal debris likely originating from littoral macrophytes. Video imagery showed that the lakebed was partly covered by microbial mats and depositional matter and harbored an unexpectedly rich assortment of macrofauna, including sponges, tubificid worms, flatworms, bivalves and crustaceans. Various forms of bioturbation were also encountered, some with the animals in the tracks. Most notably, at the deepest site, a previously undescribed faunal feature was evident, apparently formed by a mat-like community of several layers of what appeared to be polychaete tubeworms, possibly of the family Siboglinidae. It is hypothesized that the hydrothermal activity observed in the region may supply the compounds able to support the deep-water microrganisms from which such tubeworms typically gain sustenance. Such processes could be present on other deep and aphotic liquid-water-bearing planetary bodies.
Introduction
Interpretation of images taken by the Hubble Space Telescope would suggest that plumes of water erupt from Jupiter's icy moon Europa (Roth et al., 2014) and from Enceladus, one of Saturn's moons (Postberg et al., 2011; Thomas et al., 2016). These plumes are thought to be material ejected from internal oceans or lakes existing beneath an external ice crust as a result of hydrothermal activity, as suggested by Hsu et al. (2015), Postberg et al. (2011) and Thomas et al. (2016) for Enceladus and Vance et al. (2007) for small ocean planets. Consequently other authors have envisioned that Europa's inner oceans might contain a variety of potentially life-supporting environments (Chyba and Phillips, 2002; Pappalardo et al., 2013; Phillips and Pappalardo, 2014), ranging from hydrothermal vents to cold liquid brines (Lowell and DuBose, 2005; Russell et al., 2017). In addition, an internal ocean of salty water is thought to exist on Titan, as revealed by its rotation (Lorenz et al., 2008), while the presence of liquid methane lakes on its surface is confirmed by data received by the Cassini-Huygens spacecraft (Sagan and Dermott, 1982; Lorenz et al., 2008; Lunine and Atreya, 2008; Moriconi et al., 2010).
NASA's Europa Lander Project has as its aim the detection of life or its fingerprints in the internal ocean system of Europa, through exploration and sampling of potential ocean life-forms or of biotic material that might have accumulated through time (Pappalardo et al., 2013; Russell et al., 2017). With this purpose in mind the Planetary Lake Lander (PLL) project (Cabrol et al., 2012, 2014; Pedersen et al., 2015) selected the extreme environment of Laguna Negra, a glacier lake in the southern volcanic zone of the central Chilean Andes, in order to test methods and technologies suitable for work in extreme planetary aqueous environments. The PLL campaign undertaken in 2013 involved a complete investigation of the lake ecosystem, including (i) collection of data on bathymetry (Cabrol et al., 2015), temperature and light distribution levels through the whole water column (Rose et al., 2014; Cabrol et al., 2015), (ii) sampling for water chemistry and the distribution of organics (Sobron et al., 2013), and (iii) investigation of the microbiology of both benthic sediments (Parro et al., 2018) and the water column (Echeverría-Vega et al., 2018). Complementary work involved the analysis, using a Rapid Ecological Assessment (REA) approach, of video imagery collected from all depths, supplemented by the collection of water-sediment samples from the bottom of the lake for sedimentological, biological and geochemical analysis (Tilot, 2014a). The latter supplemented previous microbiological analysis of bottom sediments using (a) DNA extraction, cloning and sequencing, (b) ammonia oxidizing activity and nitrate and sulfate evolution testing in separate mesocosm experiments, and (c) microarray immunoassays with an immunological Life Detector Chip (LDChip) with a 300-antibody microarray biosensor specifically developed for planetary exploration and environmental monitoring (Parro et al., 2018, 2019). Here we present the main results of the REA work directed at to a multi-parametric assessment of the lake ecosystem directed at understanding the structure and functioning of the lake ecosystem.
A separate issue that it was intended for the PLL to address was the concern about the capacity of high-altitude lake systems to buffer the potential effects of nutrient addition anticipated as a consequence of either periodic deglaciation or the deglaciation associated with current climate change CONAMA (2007). Glacier surface areas have diminished by more than 30% in the past 50 years, a trend that has accelerated recently (Rabatel et al., 2011), including for the Echaurren Glacier close to Laguna Negra (Escobar et al., 2000; Carrasco et al., 2005; Rabatel et al., 2011). Increased nutrient input to high altitude lakes has been anticipated as a result of either increased input of water as a result of glacial melt (Roig et al., 2001; Le Quesne et al., 2009), or as a consequence of melting of the upstream permafrost within the catchment (Rogora et al., 2008; Salerno et al., 2016). Such alterations may lead to oligotrophic lakes becoming more eutrophic (Lyons et al., 2006; Modenutti et al., 2013) and to linked changes in trophic chains and benthic habitats (Modenutti et al., 1998, 2013; Uehlinger et al., 2010).
Materials and Methods
General Description of the Lake
Laguna Negra is located at an altitude of 2,700 m on the south slope of the Echaurren Glacier watershed (33.65S/70.13W), where it constitutes part of the complex freshwater resources of the Santiago area (Figures 1, 2A). It has a maximum depth of 272 m, its size (6.1 × 1.7 km) and form being the result of glacial carving of a deep narrow valley combined with local tectonic deformation of bedrock (Farias, 2007) (Figures 2B, 3). During the austral summer, December–May, the lake becomes stratified, with water temperatures in the upper euphotic zone, down to 20 m, reaching 12–20°C (Figure 4), whereas during July–October there is a winter overturn with temperatures falling to 4–5°C throughout the water column (Cabrol et al., 2015). The lake's water is relatively transparent, with turbidity declining from closet to 1 NTU near the surface (0–5 m) to 0.9 NTU in deeper water (>20 m) (Cabrol et al., 2015); thus there is a relatively deep euphotic layer (Figure 4), but below this a large aphotic zone, so that the lake's overall mean primary production is relatively low, resulting in its classification as a cold (4°C), deep, essentially oligotrophic lacustrine ecosystem (Montencino, 1991; Soto, 2002). Laguna Negra is one of at least 84 closed-basin lakes present in this part of Chile and adjacent Bolivia, most of which are saline in nature (Risacher et al., 2001; Alvial et al., 2008), but the water column of Laguna Negra has been recorded has having a low conductivity (130–139 uS/cm) (Echeverría-Vega et al., 2018) indicating that, at least in its upper parts, it is not at all saline. Likewise, as reported in Echeverría-Vega et al. (2018), the pH of the upper portion of the lake appears to be slightly alkaline (pH 7.1–7.4), although, as discussed further below, faunal evidence suggests that the conditions near the lakebed are more acidic.
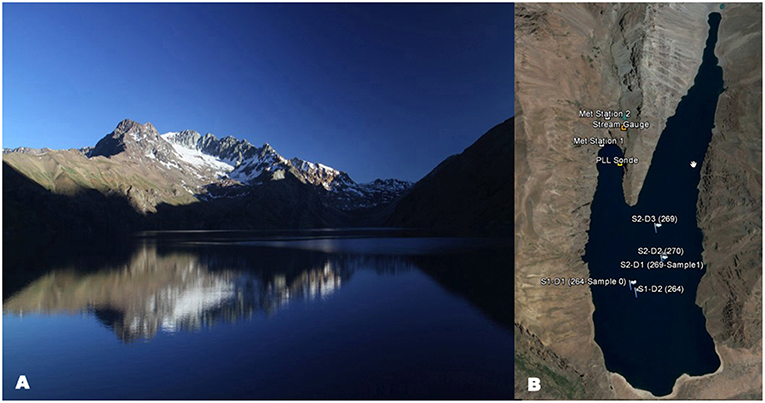
Figure 2. Laguna Negra is located at an altitude of 2,700 m on the south slope of the Echaurren glacier (in background of photo) watershed (33.65S/70.13W), where it constitutes part of the complex freshwater resources of the Santiago area (A). A satellite image of the lake showing the locations of the water sampling sites (B).
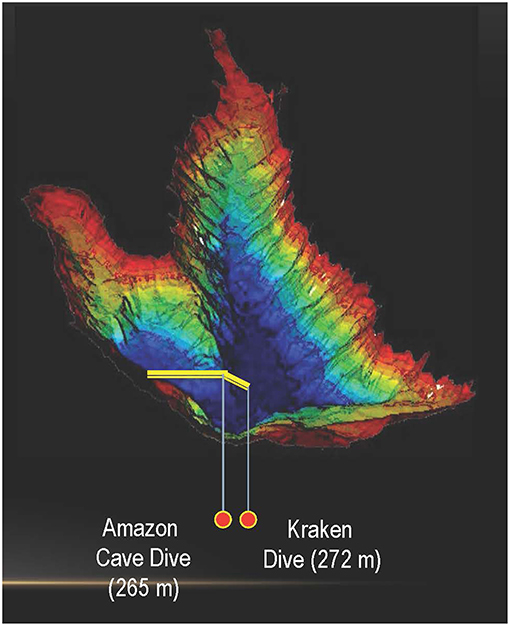
Figure 3. Bathymetric map of Laguna Negra showing locations of the deepest samplings and video points at Amazon Cave Dive (262 m) and Kraken Dive (272 m).
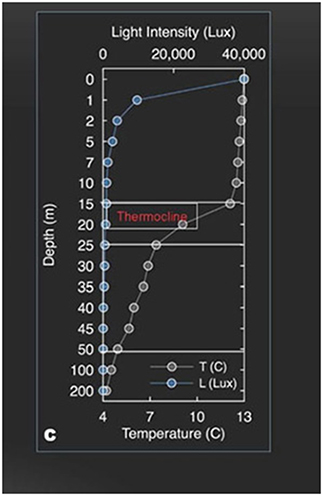
Figure 4. The NASA/ASTEP/SETI Planetary Lake Lander survey data on physico-chemical parameters of the water column from December 2012 to December 2013 (Cabrol et al., 2015): the figure is a sample profile showing variation in light intensity (Lux) and temperature (°C) in relation to depth (to 200 m).
Multi-Parametric Rapid Ecological Assessment (REA)
Ecological assessment of an ecosystem is undertaken to evaluate relationships among organisms and their natural habitat and biophysical environment (Chapman, 1996; Hill and Wilkinson, 2004; Spellerberg, 2005; Hering et al., 2006; Tilot et al., 2008). Rapid Ecological Assessment (REA) is a flexible, accelerated and targeted biodiversity and environmental survey methodology, based on a collection of semi-quantitative field data, or of quantitative data on selected indicator variables. The approach addresses the lack of detailed data that necessarily results when survey time or resources are very limited, or the area to be surveyed is unexplored and/or very extensive. Nevertheless, it is intended to preserve spatially explicit information and facilitate integration of data obtained from different sources and at different scales. Ideally it can be used to generate useful and visually appealing products that can be used to facilitate conservation and management. The REA methodology thus bridges the gap between collection of high volumes of quantitative data and purely qualitative assessments of aquatic or other habitats, while nevertheless allowing statistical comparisons in a manner that makes it a useful ecosystem management and decision-making tool (Tilot, 2014b; Tilot et al., 2018a). The approach serves to provide as close to a quantitative approach as practicable in situations where collection of samples for quantitative analysis is not possible.
In the present case the REA approach was adapted to provide a simple, yet robust, means for obtaining semi-quantitative data from video imagery that would achieve the characterization of distinct habitats or sub-habitats, together with their associated fauna and flora, and an assessment of environmental state. For this purpose, a video camera and sediment sample collection equipment were attached, along with a YSI 6600 multi-parameter probe, to a “lake-lander” platform (Sobron et al., 2013; Rose et al., 2014; Cabrol et al., 2015), which was lowered or towed from a small inflatable boat (Figures 5A–D).
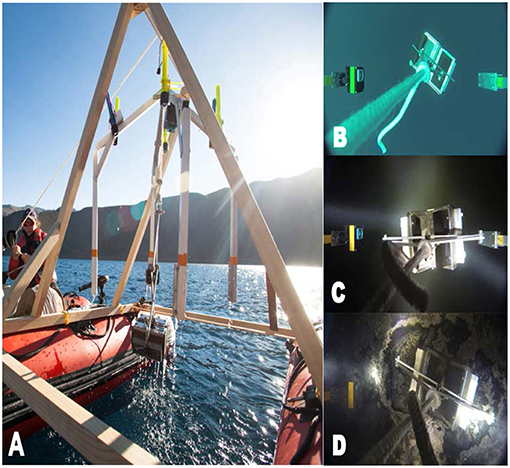
Figure 5. (A) sampling system using two zodiac boats and intervening winch for the collection of sediments, enabling a targeted Rapid Environmental Assessment (REA) based on imagery by means of a GoPro 300 system within a 300-m depth rated casing attached to gear (B) and sampling of sediments with a sampling grab (C). The grab makes contact with the lakebed at Kraken Dive (272 m depth) (D).
Imagery Collection and Analysis
For video imagery collection the lander, fitted with a GoPro Hero3 camera (resolution of 1,920 × 1,080) with a housing and lights rated for 300 m depth, was guided along “video transects” that covered an area extending from the western shoreline to the deepest part of the lake. The lander with camera was deployed for a total of more than 210 h, during which video imagery was recorded on traverses at two sites, from shallow to deeper waters, at Amazon Caves (AC) up to approximately 90–100 m depth and at Peninsula West Shore (PSW) up to approximately 40–50 m depth. In addition, a series of vertical “jump” or “spot” dives (lowering the lander directly to the lake bed and lifting it again) were performed with the lander and video cameras to reach landing sights at target depths of 50, 60, 70, 80, 90 and 100 m. Jump dives were also achieved at the greatest depth obtainable on the lakebed at 4 separate sites, “Amazon Caves” (AC) (2 sites at 262 m), and 2 sites at Kraken Dive (KD) (272 m) (Cabrol et al., 2015) (Figures 2B, 3). Video material was analyzed through visual assessments by an expert observer related to each of a series of timed portions of each bottom transect or jump dive. The observer recorded habitat, and floral and faunal characteristics, along with the presence of evident biota or signs of bioturbation. So far as practicable, features were graded on a semi-quantitative scale of 1–3 or 1–5, with the assistance of written criteria used to aid the recognition of each grade. Where sufficient data was available, scaled values were analyzed statistically to assist recognition of characteristic habitats or biological communities and to assess the apparent status of benthic habitats (Tilot, 2014a).
Sediment Collection and Analysis
Using samplers attached to the Lake Lander, sediments were sampled for sedimentology, biogeochemical, and biological analysis at a depth of 262 m at site AC and a depth of 272 m at KD. The first samples were collected at KD during 2013 (sample S0) (Figure 5D) and two additional samples at AC in April 2015 (samples S1). The sediment samples were collected in 50 mL sterile Falcon tubes, kept refrigerated during shipment, and then stored at 4°C (the temperature measured on the lakebed) pending further treatment (Cabrol et al., 2015).
Grain-size analysis was carried out in the Sedimentology Laboratory of the Spanish Institute of Oceanography (Málaga, Spain). Replicate samples (1 g each) were pre-treated with 1N HCl to remove carbonate particles and 10% H2O2 to oxidize organic matter,1% sodium polyphosphate was added to prevent particle flocculation, and particle size-distribution data obtained using a laser diffraction method in a Malvern Mastersizer 3000 (grain-size range from 0.01 to 3,000 μm). Grain-size statistics (mean particle size, sorting, skewness and kurtosis) were calculated (leptokurtic distributions having more values toward the mean than in a normal mesokurtic, distribution) and samples classified according to the sorting classes of Folk and Ward (1957).
Biological examination of the water-sediment interface samples was carried out at the University of Málaga, Department of Ecology and Geology, using an optical microscope with 200× enlargement. Examination of phytoplankton was undertaken using 1 ml sub-samples within sedimentation chambers viewed with an inverted microscope, Protozoa and copepod nauplii were classified under a binocular microscope with 200× magnification, and larger organisms, such as copepods and cladocerans, determined in 1 ml samples under a binocular microscope with 100× magnification.
Data concerning the physical, biogeochemical and microbiological foundation of this region has been published previously (Montencino, 1991; Catalán et al., 1993; Mermillod-Blondin et al., 2001; Van der Vijver et al., 2004; Alvial et al., 2008; Modenutti et al., 2013; Echeverría-Vega et al., 2018; Parro et al., 2018) and will not be reviewed here, but referred to when appropriate.
Results
Benthic Sediments
The texture of the total and the carbonate-free fractions of sample “Lake S1” (collected at AC) was silt with median grain-size values of 20 and 16 μm, respectively, with the sediment being poorly sorted (sorting of 3.0 and 2.4, respectively) but finely skewed (skewness of ca. −0.1), which indicates a relatively high proportion of fine material, and leptokurtic (kurtosis of ca. 1.1) (Figure 6A) which means less particle scattering and a more homogeneous distribution.
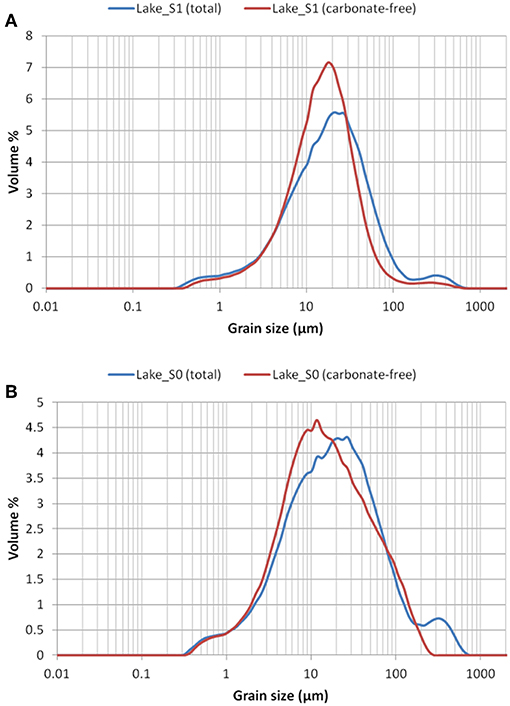
Figure 6. Results of sedimentological analysis of samples collected by the Lake Lander at 265 m depth at Site Amazon Cave Dive (S1) in April 2015 (A) and at 272 m depth at Kraken Dive (S0) in 2013 (B). Variation in % volume of grains of different size is shown both for the total sample and for the carbonate-free portion.
The texture of both total and carbonate-free fractions of sample “Lake S0” (collected at KD) was sandy silt with median grain-size values of 20 and 15 μm, respectively. In general, the sediment was poorly sorted (sorting of 3.8 and 3.4, respectively) and displayed symmetrical (skewness close to 0) and mesokurtic (kurtosis of ca. 1) distributions (Figure 6B), indicating a large admixture of medium to fine particles.
Water-Sediment Interface
The biological analysis of the sediment samples revealed a large quantity of vegetal debris which most likely originated from the littoral macrophytes observed in the videotransects along the shoreline and deeper (at AC and PWS), such as Myriophyllium sp., Chara sp., Nitella sp., Potamogeton sp.
In addition, abundant diatom frustules were present (Rivera, 2006), mainly of the pennades diatom Pinnularia acidicola along with deceased zooplankton (Ostracoda Cladocera sp.) (Villalobos, 2006) and fecal pellets and inorganic particles. Both macrophyte debris and diatom frustules appeared to have drifted down through the water column to settle on the lakebed. There was however no evidence of benthic macroinvertebrates within the sediment samples.
Video Imagery
The imagery recorded an abundance of life in the water column and on the benthic substrate (Figure 7) and also revealed various forms of life and bioturbation associated with the faunal communities down to the maximum depths of 262 m at AC (Figures 8A,B) and 272 m at KD (Figure 9). Details of the observations made on rapid ecological assessment of this imagery are shown for the towed video transects at the shoreline at AC and PWS in Table 1, and for the jump dives in Table 2. These data was compared to produce generalized descriptions of the main sub-habitats observed, for the towed video transects in Table 3 and for the jump-dive imagery in Table 4. The presence of benthic macroinvetebrates was notable on the videos, as was a marked heterogeneity of microhabitats and patchiness of epifaunal communities, together with abundant bioturbation activity. Apart from the biota and the biosignatures, the video imagery indicated significant amounts of organic matter drifting downwards within the water column. For supplementary information justifying the above identifications, as well as for data on depth, substrate, habitat associations, faunal and floral assemblages, and a general assessment of the apparent health or naturalness of the study sites, see Tilot (2014a).
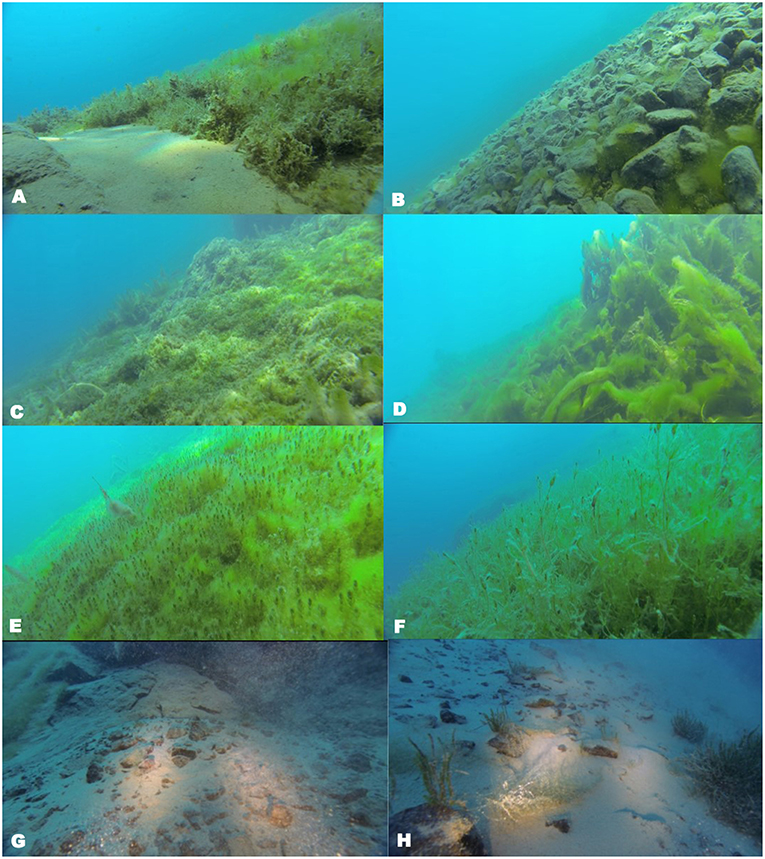
Figure 7. Images from the 2013 video surveys and jump dives illustrating representative habitats and associated biotic communities observed at different depths. (Timings refer to time lapsed on video recordings). (A) Amazon Cave video transect part 1 (08:02): slope 20–40°, 5–10 m depth, rock and sediment, Myriophyllum sp. (M. quitense?) and small Nitella sp. (N. acuminata?, N. flexilis?), Chara sp. (C. globularis?, C. rusbyana?). (B) Dive peninsula west shore profile (11:42): slope 40–55°, 10–20 m depth, stony mass of fallen rocks with a thin layer of filamentous bright green algae Cladophora sp. and oligochaete worm. (C) Dive peninsula west shore profile (13:09): slope 40–55°, 10–20 m depth, 100% coverage of small Chara sp. (C. globularis?, C. rusbyana?) densely covered by bright green algae Cladophora sp., in foreground (and background), Myriophyllum sp. (M. quitenses?) covered by extensive algal mat. (D) Dive peninsula west shore profile (12:04): slope 40–55°, 10–20 m depth, extensive bright green algal mat of Cladophora sp. covering large colonies of Myriophyllum sp. (M. quitenses?). (E) Dive peninsula west shore profile (15:07): slope 40–55°, 10–20 m depth, 100% Charas sp. (C. globularis?, C. rusbyana?) and Nitella sp. (N. acuminata?) covered by a thick layer of bright green algae Cladophora sp., old dead stems of Myriophyllum sp. (M. quitenses?) covered by algal mat and sponges Spongilidae? (F) Amazon Cave (13:40): slope 40–60°, 30–40 m depth, close up of 100% cover of Nitella sp. (N. acuminata?) green algal flocculates on flora, most apex still free. (G) Amazon Caves transect 60 m depth (9:15): slope 40–60°, sediment surface with rocks, some boulders, close to a thick strip of algae Nitella sp. (N. acuminata? N. flexilis?) (H) Jump Dive 60 m (20:00): rocks and thick layer of sediments. One Nitella sp. strip and several bushes of Charophytes Potamogeton sp. (P. pectinatus?).
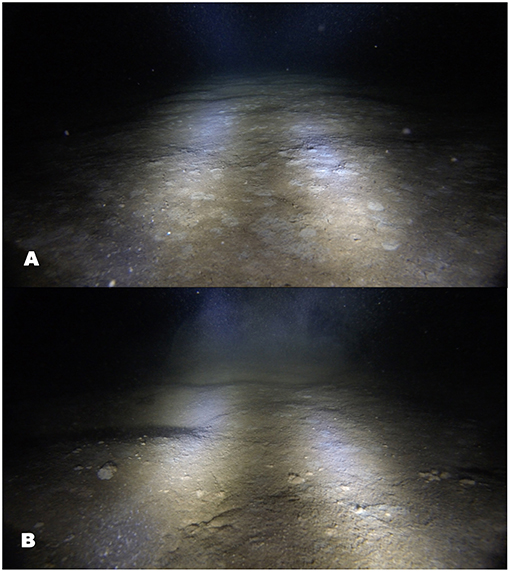
Figure 8. Images from videos taken in 2013 during jump dives to 262 m at the Amazon Caves sites. (A) Amazon Caves Jump dive site 1 (23:24): brownish gray sediment with a layer of deposited particles with numerous (40–50% of area) white circular marks and traces of bioturbation including many small faunal features possibly produced by burrowing bivalves, crustaceans, worms etc. The large white circular marks could also be fluid seeping features (fsf) considering the thermal activity in the area. (B) Amazon Caves Jump dive site 2 (00:20): slightly hummocky lakebed covered by sediment with flocculate detritus matter conglomerated in whitish masses and traces of bioturbation (5–10%) and small grooves. Above the sediment surface, one can see the flow of particles and plankton.
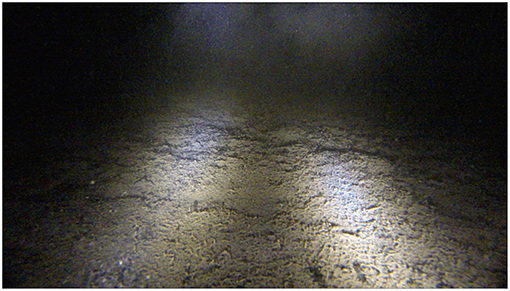
Figure 9. Image from videos taken in 2013 during jump dives to 272 m at Kraken site (14:20). A low mat-like structure is evident that appears to consist of several layers of tubular structures (~10 cm long) with one upright extremity with pinkish moving features. These are possibly polychaete tubeworms, Siboglinidae. Sponges and molluscs are present on top of the tubeworm mat and small planktonic forms, ostracods, shrimps are apparent above the lakebed. The flow of particles seems to move slowly upwards with simmering water.
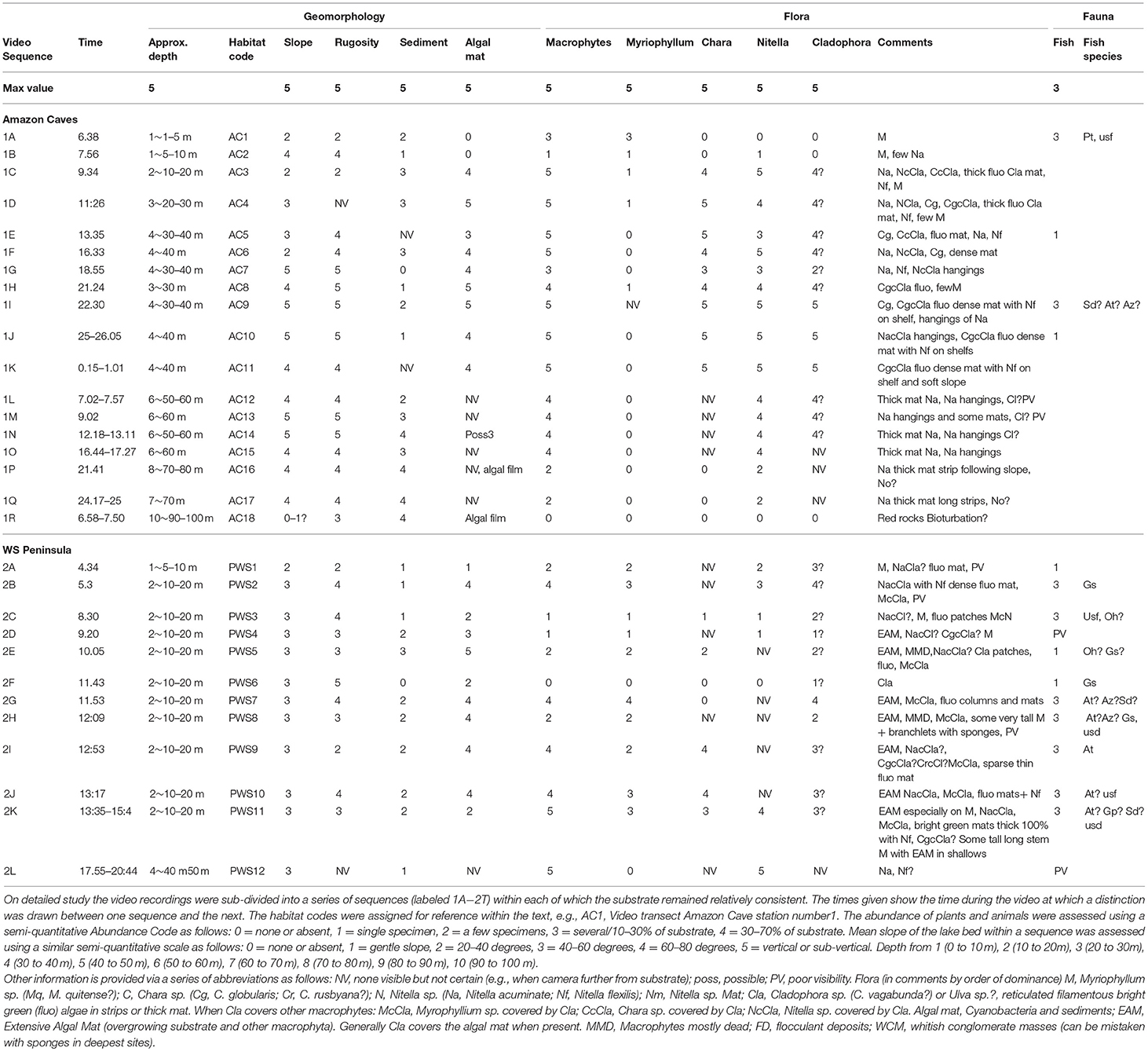
Table 1. Results of analysis of the video sequences taken on bottom transects at two sites, Amazon Caves and Peninsula West Shore, using a GoPro 300 camera system, showing the geomorphological characteristics of the substrate and the most evident flora and fauna.
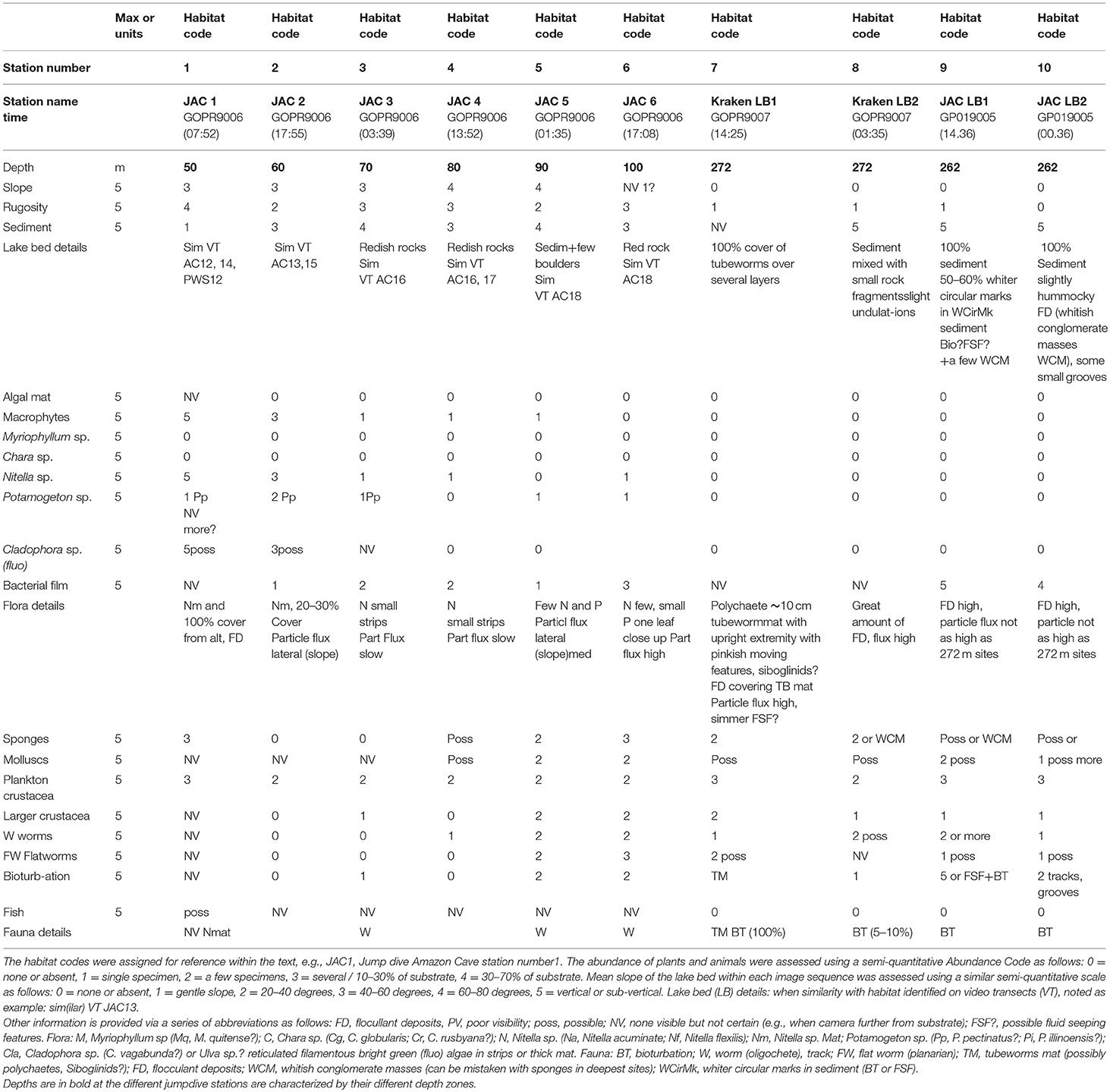
Table 2. Results of analysis of the video sequences on drop-down dives using the deep-water lander, showing the geomorphological characteristics of the substrate and the most evident flora and fauna.
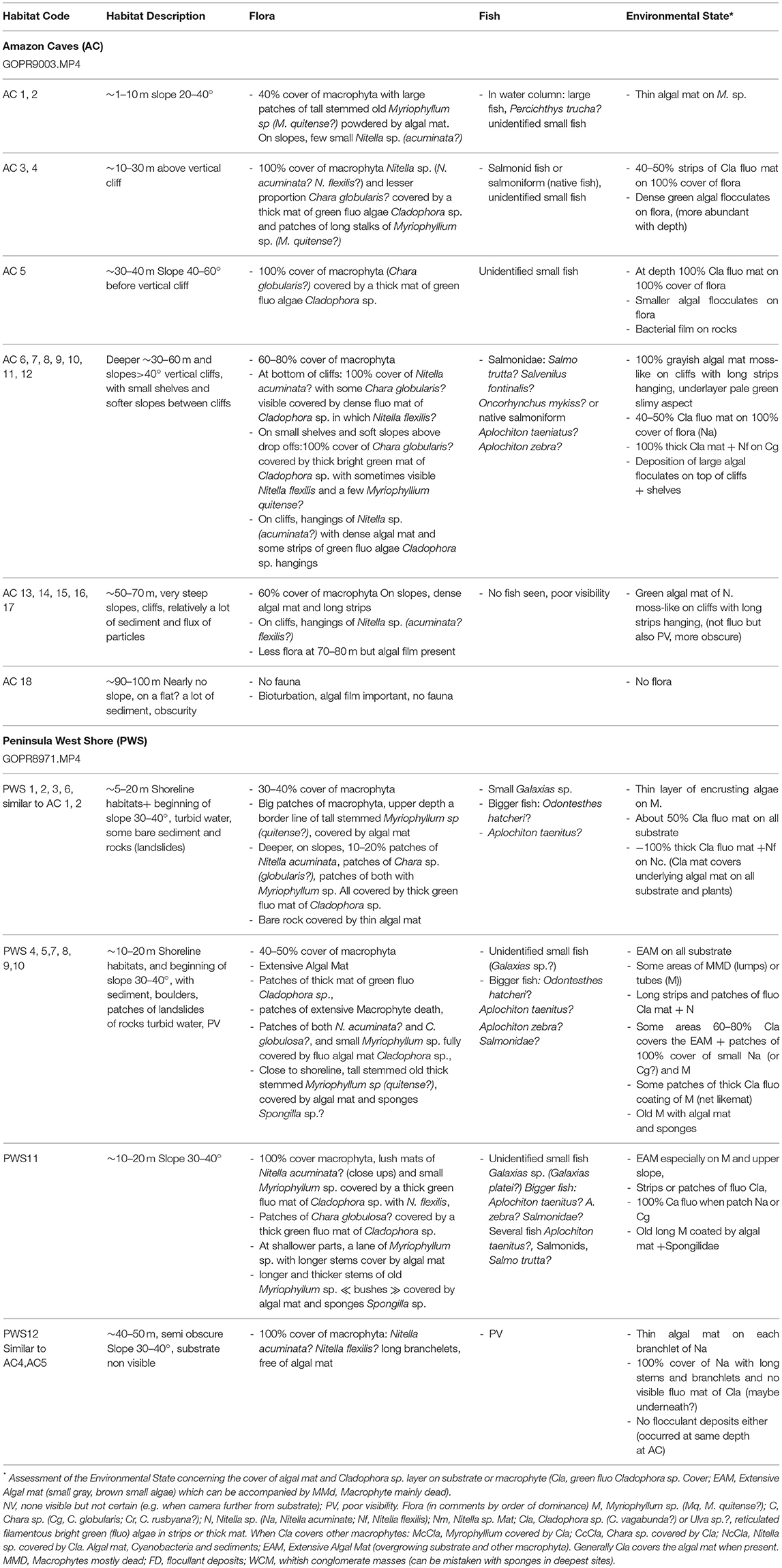
Table 3. Summary of distinct habitats and associated species of macrophytes and fish observed on the video transects at Amazon Caves (5 to 90–100 m) and Peninsula West Shore (5 to 40–50 m).
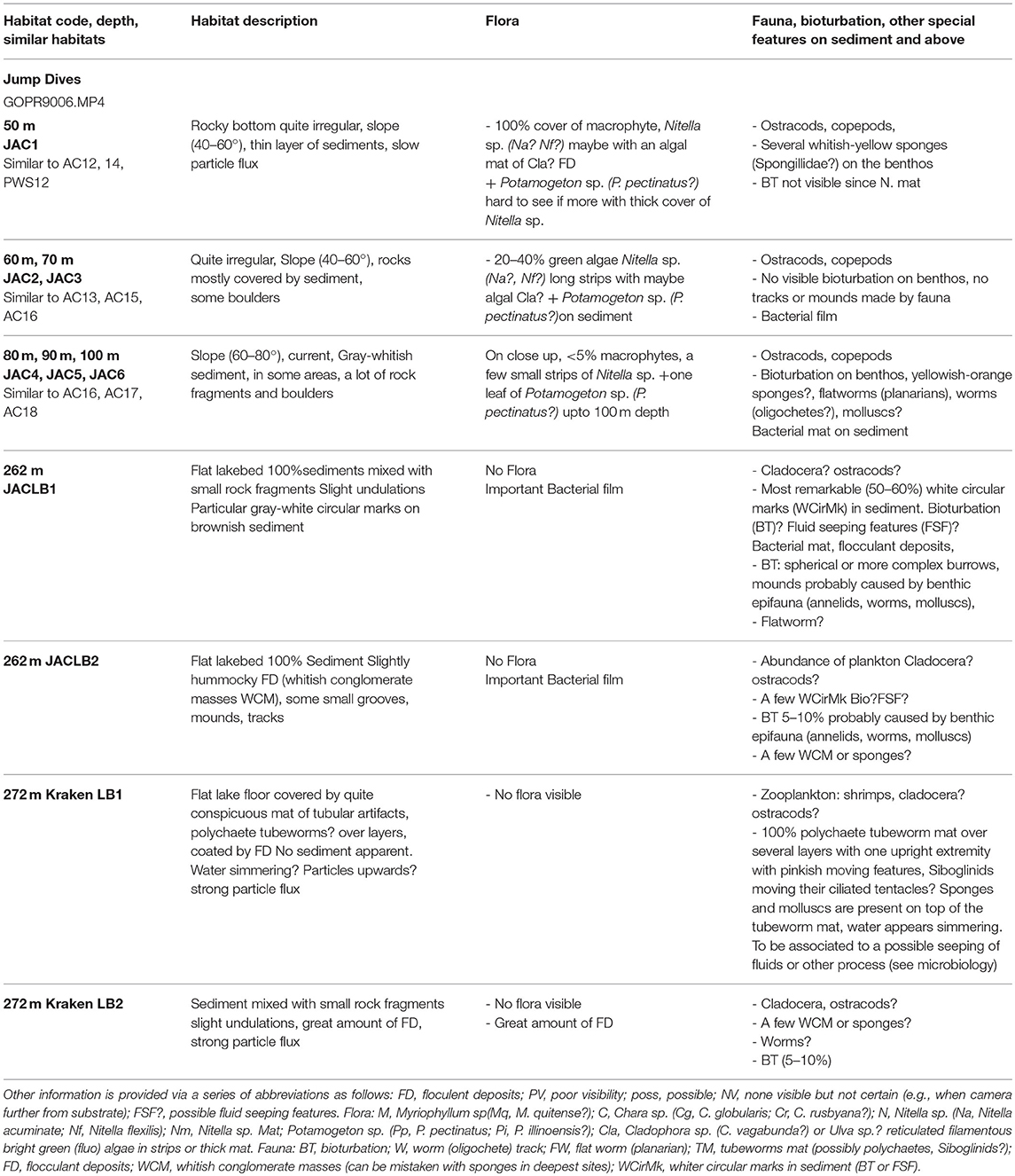
Table 4. Summary of the main habitats and associated species of macrophytes and macrofauna observed on the videos taken during the jump dives.
Macrophyta and Algae
The evident macrophyte communities appeared surprisingly lush and green, especially in shallow waters where both macrophyta and algae were present (Table 1). This can be related to the high illuminance recorded in shallow water down 10–20 m (see Figure 4). The dominant species of macrophyta were Myriophyllum sp. (M. quitense?) (Figures 7A,D), Chara sp. (globularis? rusbyana?) (Figures 7C,E), Nitella sp. (N. acuminata?) (Figure 7E). Some macrophyta extended to great depth such as Nitella sp. (N. acuminata? N. flexilis?) (Figures 7G,H at 60 m), and Potamogeton sp, (P. pectinatus?), both species were still seen at 100 m. Bright green filamentous algae Cladophora sp. (C. vagabunda?) were also quite abundant, often covering in thick layers the substrate and large communities of Chara sp. (Ch. rusbyana?, Ch. globularis?) and Nitella sp. (N. acuminata?, N. flexilis?) (Figures 7B–E). On sequences of macro-imagery video, charophytes could easily be identified, especially at blooming season in spring, such as Chara globularis by its corticated branchlets and stems, its needle shaped stipulodes and elongate black oospores, and Nitella acuminata by its crown oospores. The smooth stoneworth, Nitella flexilis had very long stems (more than 100 cm) and often formed dense mats, frequently hanging over cliffs in deeper darker waters (Figures 7F,G). It appeared on the imagery that once maturity is reached, the oldest parts of these algae die and decompose. Another observation was that charophytes appeared morphologically different with increasing depth and reducing light availability, their stems appearing more elongated, leaves larger and pigments lighter.
Macrophytes were present in abundance (100% cover) in shallower water, although quite often their growth appeared constrained by the presence of the green algal mats described previously. Colonies of Myriophyllum sp. (most probably Myriophillium quitenses) were generally found in more sheltered places such as behind rocks, in small grooves, within gulleys, and along the sides of ridges (Figure 7A). These macrophytes often trapped particles of sediment and planktonic debris and appeared to serve as nurseries for some faunal species (such as fish larvae seen on close-up in videos).
On the basis of the assessments of the video-transects recorded at Amazon Caves and Peninsula West Shore, a series of communities could be distinguished, characterized by the following macrophytes at different levels of abundance (Tables 1, 3):
- At shallow depths (1–10 m), the general coverage of macrophytes was about 30–40%. There were large patches of tall stemmed old Myriophyllum sp (M. quitense?), which at very shallow depths bordering the shoreline was partly covered by small colonies of algal mat. On slopes there were also a few small Nitella sp. (acuminata?),
- At 10–30 m depth, on slopes of 20–40°, there was mostly 100% coverage of macrophyta Nitella sp. (N. acuminata? N. flexilis?) and lesser amounts of Chara globularis? covered by a thick mat of bright green filamentous algae Cladophora sp., with patches of long stalked Myriophyllium sp. (M. quitense?). On the video transects at Peninsula West Shore the total coverage at this depth was only 30–40%, since there were some areas covered by an extensive algal mat with abundant but mostly dead macrophytes.
- At 30–40 m depth, there was about 60–80% coverage of macrophyta on the steep slopes and vertical cliffs, interspersed with small shelves and gentler slopes. At the bottom of the cliffs there was typically 100% cover of Nitella acuminata?, with some Chara globularis?, covered by a dense bright green mat of Cladophora sp., among which Nitella flexilis? could also be seen in macro-images. On the small shelves and gentler slopes there was often 100% coverage of Chara globularis? under a thick bright green layer of filamentous Cladophora sp., with Nitella flexilis and Myriophyllium quitense? sometimes also visible. On the cliffs themselves, there were hangings of Nitella sp. (N. acuminata? N. flexilis?), with a dense algal mat and occasional strips of bright green filamentous algae Cladophora sp.
- At 50–70 m, there was about 50% coverage of macrophyta on very steep slopes and cliffs, with dense algal mat and long strips of Nitella sp. (acuminata? obscura? flexilis?) also present.
- At more than 70 m, there was only a small coverage of macrophyte, the precise amounts being difficult to assess due to the limited visibility. Nitella sp. and Potamogeton sp. were still present down to 100 m. Potamogeton sp. (P. pectinatus?, P. illinoensis?) was conspicuous between 50 and 100 m; it may have been present in shallower waters, but escaped detection because of the dense cover of macrophytes.
On the basis of the video sequences from jump dives, the main communities were characterized by the following macrophytes at different levels of abundance (Tables 2, 4):
- at 50 m, on slopes of 40–60°, there was 100% cover of macrophytes, Nitella sp. (N. acuminata? N. flexilis?), possibly with filamentous algae Cladophora sp.? and Potamogeton sp. (P. pectinatus?).
- at 60 and 70 m depth, on slopes of 40–60°, there was 20–40% cover of the green algae Nitella sp. (N. acuminata? N. flexilis?) forming mats and long strips following the slope. There were also some small bushes of Potamogeton sp. (P. pectinatus?) anchored in the sediment.
- at 80, 90 and 100 m depth, on slopes of 60–80°, there was <5% cover of macrophytes, only a few small strips of Nitella sp. and Potamogeton sp. (P. pectinatus?) both present in the twilight zone.
Benthic Invertebrates
A wide variety of benthic and epibenthic invertebrates were also visible in the video sequences, especially in bottom transects, including: crustaceans (amphipods), molluscs (bivalves, Pisidiidae? Pisidium sp.?, Sphaeriidae? Hyriidae? Diplodon chilensis?), gastropods (Ampullariidae?, Tateidae?, Lymnaeidae?, Melanopsidae?, Physidae?), limpets (Planorbidae?, Thiaridae?), segmented annelid worms (Naididae), oligochaete worms (Tubificidae? Branchiura?), flatworms or platyhelminths (Dendrocoelidae? Turbellaria?), hydroids, sponges (Spongillidae?), Planariidae (Turbellaria) and Oligochaeta, although because of the limited lightning and resolution, rarely could invertebrates be identified to genus level. Also visible were animal tracks, mounds, burrows, and other forms of bioturbation (see below).
Sponges belonging to the family Spongillidae, were recorded, peaking at about 50 m depth. They were typically observed emerging from the dense algal mats, where they were often attached to the dead stems of Myriophyllium sp., generally in shallow areas that might have been affected by eutrophication. There were indications that here, as in other extreme environments, they adapt to seasonal change by forming “gemmules,” resistant buds that can survive dormant after the mother sponge has died until conditions improve.
Bioturbation
Besides direct observations of living animals, signs of their activity on or within the bottom sediment (bioturbation) were also visible at the bottom of the lake, especially in its deepest parts on the 2 jump dives sites at Kraken (272 m) and the 2 sites at Amazon caves (262 m). Bioturbation was evident not only where there was physical disturbance, but where nutrient cycling has been accelerated as a result of bivalves, crustaceans or worms mixing the sediment. Otherwise, a large number of bioturbation features were identified and described, readily recognizable tracks and characteristically shaped burrows such as rosette-shaped ones, usually found to be caused by worms (Figures 8A,B). Vermiform animals (principally annelids) typically create vertical burrows, while crustaceans primarily excavate more open burrow systems. Horizontal traces were also present, including a range of branching and variably sinuous to meandering burrows made by worms. Based on such signs, bioturbation was observed that was ascribed to sponges, annelids (naidid oligichaetes and tentaculate polychaetes), nematodes, arthropods including crustaceans, isopods, amphipods, pericarids and decapods, molluscs (sphaericean and unionid bivalves) and also fish.
In places, the benthos was covered with white marks on the brownish gray mat of deposited organic particles and sediment (Figures 8A,B). Elsewhere were other features characterized by a central burrow with a polychaete tube, or a mound or series of mounds. In particular, a “rosette shaped mound” was recorded, probably created by a benthic worm dwelling under the tumulus, the “rosette” markings being formed where the worm's proboscis is “licking” the surface sediment in search of nutritive particles. Other markings noted resembled those created by pelecipod molluscs, taking the form of a series of small burrows, some with part of a shell apparent. There was also a remarkable funnel-shaped burrow that had an unidentified type of worm protruding from the center. At 90 and 100 m on videos of the jump dives at Amazon caves, animals that were probably relatively big flatworms of around 5–7 cm (Dendrocoelidae?) were also observed. Generally planarians measure only a few mm in length, but some giant species (up to 40 cm long) have been found in greater depths in Lake Baikal). In Laguna negra their tracks resembled the long furrows made by sipunculids on the deep sea benthos (Tilot, 2006).
At both deepest sites, REA of jump dive imagery identified zooplankton (crustacean Ostracods, and possibly Cladocera spp.) and, in the lower water column, copepods, amid an intense sestonic flux of plankton, fecal pellets, inorganic particles and debris, which on descent and ascent appeared present through the entire water column. At AC the benthos was covered by a microbial mat and deposited matter. Several indications of biological activity were recorded, such as different artifacts, mounds, funnel-shaped burrows and rosette-shaped mounds, probably caused by benthic fauna such as annelids, crustaceans, fish (Figures 8A,B). Other biological activity covering 30–40% of the benthos was probably produced by burrowing bivalves, crustaceans, tubificid worms, or lophophorate bryozoans, and probably again large flatworms (planarians), like those described above. On one video sequence from the Amazon Cave jump dive site (Figure 8A), the brownish gray sediment showed numerous (40–50% of area) white circular marks (about 5 cm in diameter) which could be bioturbation artifacts or fluid seeping features considering the thermal activity in the area.
The most notable observation however was made on the jump dive at site KD, where, at the deepest point in the lake, there was evidence of a low mat-like structure, covering 100% of the substrate. The mat consisted of several layers of tubular structures, each with one upright extremity showing pinkish moving features. These appear to be polychaete tubeworms of the family Siboglinidae moving their ciliated tentacles (Figure 9). Sponges and molluscs were present on top of this potential polychaete tubeworm reef, apparently having colonized the structure (see also Cabrol et al., 2015; Tilot et al., 2018b). The sediment plume caused by the landing of the camera indicated the texture of these tubular structures; they were not agglomerated cohering structures since it was noted that large amounts of soft tubes and independent elements were lifted into the water column by the lander before subsequently redepositing. These artifacts thus responded quite differently than the very fine benthic particles that formed finer sediment plumes when it was disturbed by the lander. It appeared that many of the tubes were hollow, suggesting that the worms that formed them were no longer alive, while those worms that were alive appeared to inhabit tubes that were more erect than the others, possibly with the living worms extending out of the tube to catch food with tentacle-like antennae. Interestingly, the water column close to the lakebed appeared to be simmering, with particles moving upwards. Unfortunately however, given the aims and scope of the study, gear was not available with which these organisms could be sampled.
Vertebrates
Fish were clearly present on the video recordings and it was anticipated that the common native fish, Galaxias platei, which inhabits a wide range of lake habitats might be present (Dyer, 2000; Arismendi and Penaluna, 2009). However, its presence could not be confirmed while other species present proved very hard to determine under the prevailing conditions. A particular salmonid (Salmo trutta or Salvenilus fontinalis or Oncorhynchus mykiss) could be distinguished by a characteristic silhouette. In addition, a native salmoniform (Aplochiton taeniatus or A. zebra), usually reported (Habit et al., 2006, 2012; Arismendi and Penaluna, 2009) from more southern latitudes (37–56°C) than Laguna Negra, could be recognized by its smaller size, thinner body, and smaller head with relatively larger eyes, and on some videos was relatively common. Other native fish species, tentatively identified, were Odontesthes hatcheri, mostly recorded in shallow water, and a larger species with a characteristically rounded back, determined as the perch Percichthys trucha. One or more small benthic fish species also appeared to be present close to the lake bed in its deepest parts.
Assessment of Environmental Status
Based on the species present and their relative abundances, estimated by REA from the recorded imagery, and on the apparent naturalness of each area based on the principal observer's experience, the environmental status of each area was assessed. In comparison with other oligotrophic deep water lakes (Hoffmann et al., 1997; Hauenstein, 2006; Ramírez and San Martín, 2006; Zuloaga et al., 2008), the biota appeared somewhat abnormal, with large areas of the shallow parts of the lake bed overgrown by macroalgae.
In particular, a large part of the substrate at 5–20 m depth at Peninsula West Shore was covered by a thick layer of extensive brownish gray algal mat, with any macrophytes mainly dead (PWS habitats 4, 5, 7, 8, 9, 10, 11, Table 3). Also many areas, especially between 10 and 40 m depth, were covered by a thick mat of filamentous green algae Cladophora sp., often mixed with Nitella fragilis and extending over thick patches of Chara sp. (C. globularis?) and Nitella sp. (N. accuminata?) and on Myriophyllium sp. stems. In deeper parts of the lake, a grayish algal moss-like mat covered the submerged cliffs.
The excessive overgrowth by green algae and the abundance of macrophytes suggested higher than usual amounts of inorganic nutrients.
Discussion
Water Physico-Chemistry and Microbiology of Sediments
Previously, the water of Laguna Negra had been described as transparent, with a deep euphotic layer and low primary productivity (Montencino, 1991). The microbial diversity of water samples was considered consistent with the oligotrophic and photic characteristics of the upper water column (Echeverría-Vega et al., 2018). Taken together, the studies were thought to confirm that Laguna Negra is an oligotrophic, cold water (4°C) lake, typical of the region (Modenutti et al., 2013). However, the water near the lake bed differed from the water column in containing relatively higher but variable levels of ammonium (up to 2.8 ppm) and nitrate (up to 1.7 ppm) (Parro et al., 2019), while as described above, the large amounts of macrophyte and algae in many shallower areas suggest parts of the lake may be mesotrophic, rather than oligotrophic.
Other observations from the PPL study of the water temperature of the lake down to 40 m depth (under the thermocline) over 2 years showed that the melting season and resulting fluctuations in discharge influenced the physico-chemical parameters of the environment, its pattern of productivity and the depth distribution of flora and fauna. The thermocline depth varied according to season; during the austral summer it varied from −15 to −35 m with the water temperature beneath the thermocline being at 4.3–6°C. Then, at the beginning of the austral winter, the lake overturned bringing nutrients from the lake bed toward the surface, resulting a period of high productivity. Subsequent to this overturn there was little variation in temperature with depth.
Top sediments from the deep lake bed of Laguna Negra contained diatom species indicative of a low-pH environment, including Pinnularia acidicola which has been found previously in Andean lakes when associated with a pyritic environment (Alvial et al., 2008). On the video sequences of the lakebed, freshwater tubificids oligochaete worms were present. These macroinvertebrates are also acid-tolerant (Ilyashunk, 1999). Such species feed mainly within the sediment selectively ingesting fine organic particles with attached microflora and are able to survive in low oxygen environments (Chekanovskaya, 1962; Brinkhurst et al., 1972; Ilyashunk, 1999; Mermillod-Blondin et al., 2001).
In addition, the microbiological composition of the sediments based on an immunological life detection chip (LDChip) revealed the presence of iron and sulfur oxidizing acidophiles similar to Leptospirillum spp., Acidothiobacillus spp. or Halothiobacillus spp. (Parro et al., 2019), which support the idea of acidic niches with certain amounts of iron-sulfur minerals. While we did not sequence the sediments from the current study, here we provide a brief summary of the microbiology of Laguna Negra sediments (272 m depth, but at a different site and year), which is fully described in Parro et al. (2019). Diverse Proteobacteria, dominated by Epsilonproteobacteria, comprised ca. 30% of recovered 16S rRNA sequence data. While groups commonly associated with sediments and soils were recovered (Bacteriodetes and Verrucomicrobia), the nitrite-oxidizing Bacteria Nitrospirae comprised >5% of the recovered sequences. These organisms are commonly associated with marine environments, though they have also been found in freshwaters (Beaton et al., 2016). The potential for sulfide oxidation in these sediments is indicated by the presence of sulfide-oxidizing Sulfurimonas and Sulfuricurvum species.
The Archaeal 16S rRNA data recovered in the sediments indicated links to carbon and nitrogen cycling (Parro et al., 2018, 2019), while > 30% of the recovered Archaeal 16S rRNA data was affiliated with the Marine Group I Archaea (Nitrosoarchaeum), suggesting active ammonia oxidation in this region. Methanomicrobia, a methanogen, comprised most of the Archaeal sequence data. The remaining classified groups of organisms were also both extremophiles. Halobacteriales are typically halophilic, while Thermoplasmatales are often acidophilic and thermophilic.
Flora and Fauna
The benthic plant species observed may also be indicative of an alternating oligotrophic / eutrophic regime, since Chara rusbyana, Chara globularis, and Nitella acuminatus are usually described as indicative of oligotrophic conditions, while Ceratophyllum demersum and Myriophyllum quitense are considered indicative of eutrophic conditions, based on observations of similar high-altitude lakes in Ecuador (Kiersch et al., 2004; Aranguren-Riaño et al., 2018). The native Nitella sp. (acuminata or flexilis) reported here has also been found in other of the region's high altitude lakes (Parra et al., 2003).
The impression that the charophytes in the video sequences varied morphologically according to depth and light availability is substantiated by the literature (Andrews et al., 1984a; Casanova, 1994; Casanova and Brock, 1999; Blindow and Schütte, 2007; Wang et al., 2008, 2015). The squatter form is considered an adaptation to protect the gametangia from damage by high light intensity and low water levels (Schneider et al., 2006, 2015; Wang et al., 2008). Conversely, charophytes adapt to depth and low light availability by developing elongated stems and branchlets as seen on the videotransects of Laguna Negra.
Our observations of shifting abundances and species specific zonation in relation to depth and light availability are supported by the literature (Schwarz et al., 1996, 2002). To this picture we would add topography and nature of substrate as additional parameters structuring macrophytic communities. These distribution patterns can be explained by inter-specific differences in photosynthetic responses and intracellular partitioning patterns of newly fixed organic carbon in response to light (Sorrell et al., 2001). Concerning our observation that pigments appear to change according to depth, this can be explained by the fact that charophytes photosynthetically acclimate to different amounts of light by regulating pigment content and pattern (Andrews et al., 1984b; Czeczuga, 1986; Howard-Williams et al., 1995; Küster et al., 2000, 2004, 2005; Schagerl and Pichler, 2000; Wang et al., 2008).
Although there was no evidence of benthic macroinvertebrates in the sediment samples, this was probably due to the low sediment volumes collected in the samples. In contrast, macro-invertebrates were conspicuous on the video imagery and the REA methodology enabled both determination to an acceptable level of most of the taxa observed and characterization of the separable habitats encountered. Broadly speaking, almost all the macro-invertebrates identified on screen appeared compatible with previous ecological studies of the region's lakes and freshwater systems (Kong Urbina and Castro Fuentes, 2002; Figueroa et al., 2003; Valdovinos, 2006; Prat et al., 2009; Thorp and Rogers, 2015).
One question that may arise in connection with the distribution of these invertebrates is how they manage to colonize these remote locations. However, it is now widely accepted that besides being distributed downstream within watersheds most invertebrates may be dispersed by occasional accidental transport within the beaks or plumage of wildfowl and other birdlife (Green and Figuerola, 2005; Frisch et al., 2007). In addition, the possible salmonid fish species observed on videotransects have been introduced from the northerm hemisphere to many South American lakes to provide improved fishing opportunities (Arismendi et al., 2009; Cussac et al., 2009; Penaluna et al., 2009; Escobar et al., 2017). However, besides presenting a serious challenge to the survival of native fish species (Macchi et al., 1999; Soto et al., 2006, 2007; Pascual and Cianco, 2007; Habit et al., 2010, 2012), they also effect the abundance of planktonic and benthic invertebrates (Mouillet et al., 2018), and, on liberation into lakes may have with them other species.
Bioturbation
As indicated by the data, the video camera recordings revealed a great variety of different forms of bioturbation evident on the lakebed. Besides indicating the presence of a wide range of macro-invertebrates, many forms of bioturbation contribute to mixing of sediment and so increase the rates of recycling of macro- and micronutrients (Mermillod-Blondin et al., 2001).
Polychaete Tubeworm Mats
The most remarkable faunal community feature revealed by the video recordings was the low mat-like structure consisting of several layers of tubular structures (~10 cm long), provisionally identified as a polychaete tubeworm mat discovered at one site at Kraken in the deepest part of the lake (272 m depth). The visual identification of the mat as composed of clusters of polychaete tubeworms was very convincing to those authors with experience of encountering similar species elsewhere (VT, JR, pers.com.), since in a few places there appeared on the videos to be pinkish moving features on upright tubular structures which could be interpreted as polychaete tubeworms moving their ciliated tentacles (Figure 9). Alternatively, the structure could represent some sort of periphyton configuration resulting from the degradation of organic matter that, in turn, may support the reported microbial ammonium oxidation and nitrification processes, but this would not be possible at that depth in an aphotic zone. It is more plausible that it is a mat of chitinous tubular structures supporting a large colony of tubeworms.
This tubeworm community mat, living in what can be identified on videos as an organic-rich sediment habitat, presents many similarities to deep seafloor counterparts found living in vent and seep habitats (Tilot et al., 2010) and mud volcanoes (Paul et al., 2015). The worms observed in the present study appear to be polychaetes belonging to the family Siboglinidae. Their presence in freshwater systems is unrecorded in the literature. However, although different morphologically and ecologically, communities of a biomineralizing tube-building polychaete, identified as a serpulids, Marifugia cavatica, have been described from freshwater cave systems distributed through ground waters of the Dinaric Karst of the northeastern part of the Adriatic sea (Kupriyanova et al., 2009). Interestingly, phylogenetic analyses of the freshwater serpulids revealed that they are most closely related to brackish water tubeworms (Ficopomatus spp.), it being hypothesized that the freshwater species evolved from this ancestral estuarine species after it penetrated into freshwater lakes (Kupriyanova et al., 2009). Communities of serpulids have also been identified during surveys performed in 2008–2010 by the deep-water manned submersible Mir in Lake Baikal, a unique freshwater lake of tectonic origin, characterized by strikingly heterogeneous physico-chemical conditions (increased heat fluxes, discharges of mineralised and oil-and gas-bearing liquids, methane seeps and mud volcanoes). In the literature there is evidence of similarities between the vent/seep ecosystems of Lake Baikal and its counterparts in the marine realm (Zemskaya et al., 2012). However, no siboglinid tubeworm communities have been recorded in Lake Baikal.
Sulfide is the most common electron donor for known siboglinid symbionts and can support high carbon fixation activities (Girguis and Childress, 2006). Active hydrothermal venting may lead to sulfide emissions in the study area, as previously described within the Andean volcanic belt (Vergara et al., 2004). The benthic diatom taxa observed in the lake point to the presence of sulphuric acid (Alvial et al., 2008), while the benthic microbiology associated in Laguna Negra suggests an active sulfur cycle. The high organic carbon load supplied by macroalgae and related phytodetritus supports sulfate reducing communities in the sediments (Parro et al., 2019), and the active sulfate production measured in incubated waters points to high rates of sulfide oxidation in the Laguna Negra benthos (Parro et al., 2018, 2019).
Thus, our PLL observations suggest the presence within Laguna Negra of a novel ecosystem centered upon a possible siboglinid tubeworm community and associated symbiotic microbes. Its presence could be associated with past or present hydrothermal activity or gas-emissions described as occurring within the Andean volcanic belt (Vergara et al., 2004), with, as in other cases, the siboglinids supported by microbial communities that are dependent on compounds released through the vents and other outlets. In fact in some of the video imagery the water just above the mat appeared to be simmering, with some suspended particles being carried upwards, an effect not noted elsewhere on the lake bed. Also, the white circular marks observed in video sequences of the lakebed near Amazon Caves (Figure 8A), while possibly some form of bioturbation, could also be fluid seeping features, a sign of hydrothermal activity.
The primary nutrition of known siboglinids associated with hydrothermal vents is derived from the sulfide-rich fluids emanating from the proximate vents. These sulfides are metabolized by symbiotic hydrogen sulfide-oxidizing bacteria living in an internal organ, the trophosome (adult siboglonids lack the complete digestive track).
The possibility of active chemoautotrophy in the Laguna Negra sediments was indirectly supported by the microbiological work undertaken in association with the present work (Parro et al., 2018, 2019). Specifically, DNA sequencing revealed a relatively large proportion of ammonia-oxidizing archaea (AOA), very similar (97%) both to Nitrosopumilus maritimus (Thaumarchaeota), a species associated with deep marine environments, hydrothermal vents, and in particular with sponges (Lazar et al., 2010; Kennedy et al., 2014), and also to (99% similarity) a Yellowstone Lake vent archaea. Also detected were nitrite oxidizing bacteria (NOB) from the Nitrospira group at relevant proportions, suggesting a synthropic relationship between ammonia and nitrite oxidation. A similar co-occurrence of AOA and NOB has been described in the literature for marine environments (Ward, 2008), but not for the benthos of a freshwater oligotrophic system. However, the few eukaryotic sequences retrieved from the PLL microbiology analysis corresponded to golden algae, diatoms, some amoeba, various fungi and yeasts as well as copepods and worms, but not tubeworms. Additionally, some sequences corresponded with >98% similarity to methanogenic archaea (97% identity to Methanosaeta concilii) (Parro et al., 2018, 2019).
The lack of definitive evidence of tubeworms and sponges in the massive 16/18 S rRNA gene sequencing can be explained by the extreme difficulty of accurate sampling at 272 m depth without a remotely operated vehicle (ROV), or autonomous underwater vehicle (AUV). Thus, for the time-being the identification of the mat as composed of siboglinid polychaetes must be regarded as provisional. Hence further investigations with dedicated technology will be necessary to confirm the presence of tubeworm communities that is suggested by the video and microbiological data.
There is now a scientific consensus that the family of Siboglinidae has Mesozoic or Cenozoic origins and that they include four distinct clades: vestimentiferans, Sclerolinum, frenulates, and Osedax, which represent four evolutionary tracks and different habitat associations. Vestimentiferans live in vent and seep habitats and Sclerolinum is a monogeneric clade living on organic-rich remains. Frenulates live in often oxic organic-rich sediments habitats and Osedax is a monogeneric clade specialized in living on whale and fish bones. Hydrothermal vent communities have survived in diverse sulphidic refugia in the ocean (McArthur and Tunnicliffe, 1998), and it may be that ancestral communities colonized Laguna Negra or neighboring saline lakes and were able to persist there due to the availability of sulfide to support their chemoautotrophic symbionts.
Effect of Deglaciation and Climate Change
The excessive overgrowth by green algae and the abundance of macrophytes suggested that the ecology of shallower areas had been impacted by the input of higher than usual amounts of inorganic nutrient. However, while this might reflect long-term anthropogenic influences (Adams, 2002; Hauenstein et al., 2011), it might only be a seasonal phenomenon. The signs of eutrophication evident in the upper portion of the lake, including notably extensive overgrowth by green algal mats and in particular the spread of the stonewort Nitella sp. at depths down to 100 m, suggest recent input of nutrients. Increased input of nutrients could be a consequence of slow deglaciation and/or thawing of the thermafrost occurring as a consequence of climate change (see Introduction). The present survey took place during the earlier part of the austral summer (i.e., November 26–December 19, 2013), shortly after the period when a Spring algal bloom might have been anticipated, following the return of warmth and light to the photic zone after a winter overturn. Thus, some increase in nutrient availability and plant growth might occur naturally at that time of year. However, algal blooms are uncommon in predominantly oligotrophic high-altitude lakes (Acha et al., 2018).
In addition to seasonal and pluri-annual patterns (El Niño, la Niña and general climate change), human impacts could increase the phenomenon of eutrophisation. Glacier surface area changes over the last decades, in particular of the Echaurren Glacier close to Laguna Negra, have been primarily driven by the observed decreasing trend in precipitation (Santibanez, 1997; Quintana, 2004; Carrasco et al., 2005; CONAMA, 2007). An additional threat lies with the continuing increase of Persistent Organic Pollutants (POPs) and Poly Chlorinated Biphenyls (PCBs) inputs that may reach remote Chilean lakes by Long Range Atmospheric Transport (LRAT) and deposition (Wania and Mackay, 1995; Poster and Baker, 1996; Schulz-Bull et al., 1998; Pozo et al., 2007). Remote altitudinal lakes seem also to be more vulnerable to this kind of pollution because climatic conditions (cool environments) may enhance the residence time of POPs in these abiotic compartments (Grimalt et al., 2004). Moreover, most of the urban settlements are located on the western side of the Andes (Laguna Negra is not too distant to Santiago di Chile), which might support the regional background concentrations or influence atmospheric transport of contaminants toward remote zones (Pozo et al., 2007). The orographic effect (mountain range acting as barrier) impedes atmospheric transport of contaminants from the western to the eastern region. Contaminants absorbed on particles and dissolved in rain droplets are washed out of the atmosphere during rainfall mainly onto the western side of the mountain, enhancing deposition, and most likely accounts for the significantly higher levels of PCBs found in the western and eastern region of Chili (Pozo et al., 2007).
Methods and Instrumentation for Planetary Exploration
A Rapid Environmental Assessment (REA) approach, based on semi-quantitative but statistically valid assessment of video imagery obtained by a latest generation high resolution miniature video has been successful here when combined with monitoring of basic physico-chemical parameters of the water column, biological and ecological sediment analysis and in depth advanced life detection technologies in geochemistry, immunological (with Life detector LDChip) and 16S rRNA gene sequencing. It has proved effective in overviewing the biota and ecosystem elements of an extreme aquatic environment represented by a high altitude oligotrophic lake within the Andean volcanic belt. In the present study this approach permitted analysis of over 200 h of video recording and resulted both in the documentation of life forms and signs of bioturbation, and in the classification of distinct habitats, community associations, environmental variations and indicators over very much larger areas than would be possible using fully quantitative methods. Such a multi-layered methodology can be successful in exploration of very large terrestrial and ocean areas, such as the deep slopes of coral reefs or continental slopes or the deep ocean floor (Tilot, 2006; Tilot et al., 2010). This same approach should prove of value in the exploration of alien non-liquid and liquid environments such as seas and lakes elsewhere in the solar system.
Author Contributions
NC, VP, and AF designed the project. VT conceived an REA methodology to analyse the video imagery and assess environmental parameters, identify biological species, associated communities, and habitats. VT worked on the ecological analysis with RO. NL-G performed sedimentological analysis. EM-O and FF achieved the limnological analysis of the sediments. CT, ES, and PS performed sampling (sediments and imagery) campaign and in situ measurements. VP, IG-C, AF, and CD undertook the microbiological work and considered the astrobiological implcations. JR made the interpretation of the microbiology in reference to the possible discovery of a tubeworm community. VT wrote the manuscript. RO and JR helped with the editing. All authors read and approved the final manuscript.
Conflict of Interest Statement
CT was employed by company Campo Alto Operaciones SpA (Chile).
The remaining authors declare that the research was conducted in the absence of any commercial or financial relationships that could be construed as a potential conflict of interest.
Acknowledgments
We thank the Aguas Andinas Company (Santiago, Chile) for providing access to Laguna Negra. The NASA ASTEP Planetary Lake Lander (PLL) project was under Grants No. NNX09AE80A & NNX13AO07A. VT was part-supported by the CNRS AFB PATRINAT department of the Museum National d'Histoire Naturelle, Paris. RO was part-supported through the chair in marine and coastal conservation at King Abdulaziz University, Jeddah, funded by HRH Prince Khaled bin Sultan. VP and AF were supported by the Spanish Agencia Estatal de Investigación (AEI) and FEDER projects no. ESP2015-69540-R, and AF was supported by the Project icyMARS, European Research Council Starting Grant no 307496.
References
Acha, D., Guedron, S., Amouroux, D., Point, D., KLazzaro, X., Fernandez, P. E., et al. (2018). Algal bloom exacerbates hydrogen sulphide and methylmercury contamination in the emblematic high-altitude lake Titicaca. Geosciences 9:438. doi: 10.3390/geosciences8120438
Adams, S. M. (2002). Biological Indicators of Aquatic Ecosystem Stress. Bethesda, MD: American Fisheries Society.
Alvial, I. E., Cruces, F. J., Araneda, A. E., Grosjean, M., and Urrutia, R. E. (2008). Estructura comunitaria de diatomeas presentes en los sedimentos superficiales de ocho lagos andinos del Chile central. Rev. Chil. Hist. Nat. 81, 83–94. doi: 10.4067/S0716-078X2008000100007
Andrews, M., Box, R., McInroy, S., and Raven, J. A. (1984a). Growth of Chara hispida II. Shade adaptation. J. Ecol. 72, 885–895.
Andrews, M., Davison, I. R., Andrews, M. E., and Raven, J. A. (1984b). Growth of Chara hispida: I. Apical growth and basal decay. J. Ecol. 72, 873–884.
Aranguren-Riaño, N. J., Shurin, J. B., Pedroza-Ramos, A., Muñoz-López, C. L., López, R., and Cely, O. (2018). Sources of nutrients behind recent eutrophication of Lago de Tota, a high mountain Andean lake. Aquat. Sci. 80:39. doi: 10.1007/s00027-018-0588-x
Arismendi, I., and Penaluna, B. (2009). Peces nativos en aguas continentales del Sur de Chile (Native inland fishes of Southern Chile). Field guide has been funded by the Millenium Scientific Initiate through the FORECOS Nucleus Millenium P04-065-F of Mideplan, Imprenta América Ltd.
Arismendi, I., Soto, D., Penaluna, B., Jara, C., Leal, C., and León-Muñoz, J. (2009). Aquaculture, non-native salmonid invasions and associated declines of native fishes in northern Patagonian lakes. Freshw. Biol. 54, 1154–1147. doi: 10.1111/j.1365-2427.2008.02157.x
Beaton, E. D., Stevenson, B. S., King-Sharp, K. J., Stamps, B. W., Nunn, H. S., and Stuart, M. (2016). Local and regional diversity reveals dispersal limitation and drift as drivers for groundwater bacterial communities from a fractured granite formation. Front. Microbiol. 7:1933. doi: 10.3389/fmicb.2016.01933
Blindow, I., and Schütte, M. (2007). Elongation and mat formation of Chara aspera under different light and salinity conditions. Hydrobiologia 584, 69–76. doi: 10.1007/978-1-4020-6399-2_7
Brinkhurst, R. O., Chua, K. E., and Kaushik, N. K. (1972). Interspecific interactions and selective feeding of tubificid oligochaetes. Limnol. Oceanograph. 17, 122–133. doi: 10.4319/lo.1972.17.1.0122
Cabrol, N., Smith, T. M., Grin, E. A., Lee, S., Lorenz, R., Moersch, J., et al. (2012). “Planetary lake lander: year 3 science overview,” in AGU Fall Meeting (San Francisco, CA).
Cabrol, N., Smith, T. M., Grin, E. A., Lee, S., Lorenz, R., Moersch, J., et al. (2014). “Planetary lake lander: year 3 science overview,” in 45th Lunar Planetary Science Conference (Houston).
Cabrol, N., Tilot, V., Parro, V. A., Sobron, P., Smith, E. W., Tambley, C., et al. (2015). “The Planetary Lake lander (PLL) Project—A novel lake ecosystem in the chilean andes,” in Astrobiology Science Conference (Chicago, IL).
Carrasco, J. F., Casassa, G., and Quintana, J. (2005). Changes of the 0°C isotherm in central Chile during the last Quarter of the 20th Century. Hydrol. Sci. J. 50, 933–948. doi: 10.1623/hysj.2005.50.6.933
Casanova, M. T. (1994). Vegetative and reproductive responses of charophytes to water-level fluctuations in permanent and temporary wetlands in Australia. Aust. J. Mar. Freshw. Res. 45, 1409–1419.
Casanova, M. T., and Brock, M. A. (1999). Charophyte occurrence, seed banks and establishment in farm dams in New South Wales. Aust. J. Bot. 47, 437–444.
Catalán, J., Ballesteros, E., García, E., Palau, A., and Camarero, L. (1993). Chemical composition of disturbed and undisturbed high mountain lakes in the Pyrenees: a reference for acidified sites. Water Res. 27, 133–141.
Chapman, D. (1996). Water Quality Assessments. A Guide to Use of Biota, Sediments and Water in Environmental Monitoring, 2nd edn. Cambridge: Chapman and Hall.
Chyba, C. F., and Phillips, C. B. (2002). Europa as an abode of life. Origins Life Evol. Biosphere 32, 47–68. doi: 10.1073/pnas.98.3.801
CONAMA (2007). Resultados Proyecto Estudio de la Variabilidad Climatica en Chile para el Siglo XXI. DGF/UCH for CONAMA, CONAMA Report.
Cussac, V. E., Fernandez, D. A., Gomez, S. E., and Lopez, H. I. (2009). Fishes of southern South America: a story driven by temperature. Fish Physiol. Biochem. 35, 29–42. doi: 10.1007/s10695-008-9217-2
Czeczuga, B. (1986). The effect of light on the content of photosynthetically active pigments in species of the genus Chara. Aquat. Bot. 24, 397–401.
Dyer, B. (2000). Systematic review and biogeographyof the freshwater fishes of Chile. Revision sistematica y biogeografica de los peces dulceacuicola de chile. Estud. Oceanol. 19, 77–98.
Echeverría-Vega, A., Chong, G., Serrano, A. E., Guajardo, M., Encalada, O., Parro, V., et al. (2018). Watershed-induced limnological and microbial status in two oligotrophic andean lakes exposed to the same climatic scenario. Front Microbiol. 9:357. doi: 10.3389/fmicb.2018.00357
Escobar, F., Casassa, G., and Garin, C. (2000). “25 yr record of mass balance of Echaurren glacier, central Chile, and its relation with ENSO events,” in Proceedings, Sixth International Conference on Southern Hemisphere Meteorology and Oceanography (Santiago de Chile: American Meteorological Society), 118–119.
Escobar, F., Mallez, S., McCartney, M., Lee, C., Zielinski, P., Gjosal, R., et al. (2017). Aquatic invasive species in the great lakes región: an overview. Rev. Fish. Sci. Aquacult. 26, 121–138. doi: 10.1080/23308249.2017.1363715
Farias, M. (2007). Tectonique, Erosion et Evolution du Relief dans les Andes du Chili Central au cours du Néogène. PhD Thesis. Toulouse, University of Chile. Available online at: https://tel.archives-ouvertes.fr/tel-00256817
Figueroa, R., Valdovinos, C., Araya, E., and Parra, O. (2003). Macroinvertebrados bentónicos como indicadores de calidad de agua del sur de Chile. Rev. Chil. Hist. Nat. 76, 275–285. doi: 10.4067/S0716-078X2003000200012
Folk, R. L., and Ward, W. C. (1957). A study in the significance of gran-size parameters. J. Sedimentary Petrol. 27, 3–26.
Frisch, D., Green, A. J., and Figuerola, J. (2007). High dispersal capacity of a broad spectrum of aquatic invertebrates via waterbirds. Aquat. Sci. 69, 568–574. doi: 10.1007/s00027-007-0915-0
Girguis, P. R., and Childress, J. J. (2006). Metabolite uptake, stoichiometry and chemoautotrophic function of the hydrothermal vent tubeworm Riftia pachyptila: responses to environmental variations in substrate concentrations and temperature. J. Exp. Biol. 209, 3516–3528. doi: 10.1242/jeb.02404
Green, A. J., and Figuerola, J. (2005). Recent advances in the study of long-distance dispersal of aquatic invertebrates via birds. Divers. Distributions 11, 149–156. doi: 10.1111/j.1366-9516.2005.00147.x
Grimalt, J., Borghini, F., Sanchez-Hernandez, J. C., Barra, R., Torres Garcia, C. J., and Focardi, S. (2004). Temperature dependence of the distribution of organochlorine compounds in the Mosses of the Andean Mountains. Environ. Sci. Technol. 38, 5386–5392. doi: 10.1021/es040051m
Habit, E., Dyer, B., and Vila, I. (2006). Estado de conocimiento de los peces dulceacuicolas de Chile (current state of knowledge of freshwater fishes of Chile). Gayana 70, 100–113. doi: 10.4067/s0717-65382006000100016
Habit, E., Gonzalez, J., Ruzzante, D., and Walde, S. (2012). Native and introduced fish species richness in Chilean Patagonian lakes: inferences on invasión mechanisms using salmonid-free lakes. J. Conserv. Biogeography Diversity Distributions 18, 1153–1165. doi: 10.1111/j.1472-4642.2012.00906.x
Habit, E., Piedra, P., Ruzzante, D. E., Walde, S. J., Belk, M. C., Cussac, V. E., et al. (2010). Changes in the distribution of native fishes in response to introduced species and other anthropogenic effects. Global Ecol. Biogeography 19, 697–710. doi: 10.1111/j.1466-8238.2010.00541.x
Hauenstein, E. (2006). Visión sinóptica de los macrófitos dulceacuícolas de Chile. Gayana 70, 16–23. doi: 10.4067/s0717-65382006000100004
Hauenstein, E., Barriga, F., and De los Rios-Escalante, P. (2011). Macrophytes assemblages in mountain lakes of Huerquehue National Park (39°S, Araucania Region, Chile). Lat. Am. J. Aquat. Res. 39, 593–599. doi: 10.3856/vol39-issue3-fulltext-19
Hering, D., Feld, C. K., Moog, O., and Ofenböck, T. (2006). Cook book for the development of a Multimetric Index for biological condition of aquatic ecosystems: experiences from the European AQEM and STAR projects and related initiatives. Hydrobiologia 566, 311–324. doi: 10.1007/978-1-4020-5493-8_22
Hill, J., and Wilkinson, C. R. (2004). Methods for Ecological Monitoring of Coral Reefs. Townsville: Australian Institute of Marine Science.
Hoffmann, A., Kalin, M., Liberona, F., Muñoz, M., and Watson, J. (1997). Plantas Altoandinas en la Flora Silvestre de Chile. Santiago: Edición Fundación Claudio Gay.
Howard-Williams, C., Schwarz, A. M., and Vincent, W. F. (1995). Deepwater aquatic plant communities in an oligotrophic lake: physiological responses to variable light. Freshw. Biol. 33, 91–102.
Hsu, H. W., Postberg, F., Sekine, Y., Shibuya, T., Kempf, S., Horányi, M., et al. (2015). Ongoing hydrothermal activities within Enceladus. Nature 519, 207–210. doi: 10.1038/nature14262
Ilyashunk, B. P. (1999). Littoral oligochaete (Annelida: Oligochaeta) communities in neutral and acidic lakes in the Republic of Karelia, Russia. Boreal Environ. Res. 4, 277–284.
Kennedy, J., Flemer, B., Jackson, S. A., and Dobson, A. (2014). Evidence of a putative deep sea specific microbiome in marine sponges. PLoS ONE 9:e91092. doi: 10.1371/journal.pone.0091092
Kiersch, B., Muhleck, R., and Gunkel, G. (2004). Las macrofitas de algunos lagos alto-andinos del Ecuador y su bajo potencial como bioindicadores de eutrofizacion. Rev. Biol. Trop. 52:4. Available online at: http://www.scielo.sa.cr/scielo.php?script=sci_arttext&pid=S0034-77442004000400001&lng=en&nrm=iso
Kong Urbina, I., and Castro Fuentes, H. (2002). Guía de Biodiversidad N°3. Vol 1 Macrofauna y Algas Marinas. Antofagasta: Centro Regional de Estudios y Educacion Ambiental II Region de Antofagasta Chile (CREA).
Kupriyanova, E. K., Ten Hove, H. A., Sket, B., Zakšek, V., Trontelj, P., and Rouse, G. W. (2009). Evolution of the unique freshwater cave-dwelling tube worm Marifugia cavatica (Annelida: Serpulidae). Syst. Biodivers. 7, 389–401. doi: 10.1017/s1477200009990168
Küster, A., Schaible, R., and Schubert, H. (2000). Light acclimation of the charophyte Lamprothamnium papulosum. Aquat. Bot. 68, 205–216. doi: 10.1016/s0304-3770(00)00122-4
Küster, A., Schaible, R., and Schubert, H. (2004). Light acclimation of photosynthesis in three charophyte species. Aquat. Bot. 79, 111–124. doi: 10.1016/j.aquabot.2004.01.010
Küster, A., Schaible, R., and Schubert, H. (2005). Sex-specific light acclimation of Chara canescens (Charophyta). Aquat Bot. 83, 129–140. doi: 10.1016/j.aquabot.2005.05.009
Lazar, C. S., Dinasquet, J., Pignet, P., Prieur, D., and Toffin, L. (2010). Active archaeal communities at cold seep sediments populated by siboglinidae tubeworms from the storegga slide. Microb. Ecol. 60, 516–527. doi: 10.1007/s00248-010-9654-1
Le Quesne, C., Acuña, C., Boninsegna, J. A., Rivera, A., and Barichivich, J. (2009). Long-term glacier variations in the Central Andes of Argentina and Chile, inferred from historical records and tree-ring reconstructed precipitation. Palaeogeogr. Palaeocl. 281, 334–344. doi: 10.1016/j.palaeo.2008.01.039
Lorenz, R. D., Stiles, B. W., Kirk, R. L., Allison, M. D., Del Marmo, P. P., Iess, L., et al. (2008). Titan's rotation reveals an internal ocean and changing zonal winds. Science 319, 1649–1651. doi: 10.1126/science.1151639
Lowell, R. P., and DuBose, M. (2005). Hydrothermal sytems on Europa. Geophys. Res. Lett. 32, 1–4. doi: 10.1029/2005gl022375
Lunine, J., and Atreya, S. (2008). The methane cycle on Titan. Nat. Geosci. 1:335. doi: 10.1038/ngeo187
Lyons, W. B., Laybourn-Parry, J., Welch, K. A., and Priscu, J. C. (2006). “Antarctic lake systems and climate change,” in Trends in Antarctic Terrestrial and Limnetic Ecosystems: Antarctica as a Global Indicator, eds D. M. Bergstrom, P. Convey, and A. H. L. Huiskes (Dordrecht: Springer), 273–295.
Macchi, P. J., Cussac, V. E., Alonso, M. F., and Denegri, M. A. (1999). Predation relationships between introduced salmonids and the native fish fauna in lakes and reservoirs in northern Patagonia. Ecol. Freshw. Fish. 8, 227–236.
McArthur, A. G., and Tunnicliffe, A. (1998). Relics and antiquity revisited in the modern vent fauna. Geol. Soc. Lond. Spec. Publ. 148:271. doi: 10.1144/GSL.SP.1998.148.01.15
Mermillod-Blondin, F., Gérino, M., Degrange, V., Lensi, R., Chass,é, JL, Rards, M., and Creuzé des Châtelliers, M. (2001). Testing the functional redundancy of Limnodrilus and Tubifex (Oligochaeta, Tubificidae) in hyporheic sediments: an experimental study in microcosms. Can. J. Fish. Aquat. Sci. 58:1747–1759. doi: 10.1139/f01-119
Modenutti, B., Balseiro, E., Bastidas Navarro, M., Laspoumaderes, C., Souza, M. S., and Cuassolo, F. (2013). Environmental changes affecting light climate in oligotrophic mountain lakes: the deep chlorophyll maxima as a sensitive variable. Aquat. Sci. 75, 361–371. doi: 10.1007/s00027-012-0282-3
Modenutti, B. E., Balseiro, E. G., Queimaliños, C. P., Añón Suárez, D. A., Diéguez, M., and Albariño, R. J. (1998). Structure and dynamics of food webs in Andean lakes. Lake Reserv. Res. Manage 3, 179–186.
Montencino, V. (1991). Primary productivity in South American temperate lakes and reservoirs. Rev. Chilena Hist. Nat. 64, 555–567.
Moriconi, M. L., Lunine, J. I., Adriani, A., D'Aversa, E., Negrão, A., and Filacchione, G. (2010). Characterization of Titan's Ontario Lacus region from Cassini/VIMS observations. Icarus 210, 823–831. doi: 10.1016/j.icarus.2010.07.023
Mouillet, C., Barta, B., Espinosa, R., Andino, P., Seestern Christoffersen, K., and Jacobsen, D. (2018). Ecological effects of introduced rainbow trout (Oncorhynchus mykiss) in pristine Ecuadorian high Andean lakes. Fundam. Appl. Limnol. 191, 323–337. doi: 10.1127/fal/2018/1154
Pappalardo, R. T., Vance, S., Bagenal, F., Bills, B. G., Blaney, D. L., Blankenship, D. D., et al. (2013). Science potential from a Europa Lander. Astrobiology 13, 740–773. doi: 10.1089/ast.2013.1003
Parra, O., Valdovinos, C., Urrutia, R., Cisternas, M., Habit, E., and Mardone, M. (2003). Caracterización y tendencias tróficas de cinco lago costeros de Chile Central. Limnetica 22, 51–83. Available online at: https://www.limnetica.com/documentos/limnetica/limnetica-22-1-p-51.pdf
Parro, V., Blanco, Y., Puente-Sánchez, F., Rivas, L. A., Moreno-Paz, M., Echeverria, A., et al. (2018). Biomarkers and metabolic patterns in the sediments of evolving glacial lakes as a proxy for planetary lake exploration. Astrobiology 17:1342. doi: 10.1089/ast.2015.1342
Parro, V., Puente-Sánchez, F., Cabrol, N. A., Gallardo-Carreño, I., Moreno-Paz, M., Blanco, Y., et al. (2019). Microbiology and Nitrogen Cycle in the Benthic Sediments of a Glacial Oligotrophic Deep Andean Lake as Analog of Ancient Martian Lake-Beds. Front. Microbiol. 10:929. doi: 10.3389/fmicb.2019.00929
Pascual, M. A., and Cianco, J. E. (2007). “Introduced anadromous salmonids in Patagonia: risks, uses, and a conservation paradox,” Ecological and Genetic Implications of Aquaculture Activities, ed T. M. Bert (New York, NY: Springer), 1–18.
Paul, C. K., Dallimore, S. R., Caress, D. W., Gwiazda, R., Melling, H., Riedel, M., et al. (2015). Active mud volcanoes on the continental slope of the Canadian Beaufort Sea. Geochem. Geophys. Geosyst. 16, 3160–3181. doi: 10.1002/2015GC005928
Pedersen, L., Smith, T., Lee, S. Y., and Cabrol, N. (2015). Planetary LakeLander—A robotic sentinel to monitor remote lakes. J. Field Robot. 32, 860–879. doi: 10.1002/rob.21545
Penaluna, B., Arismendi, I., and Soto, D. (2009). Evidence of interactive segregation between introduced trout and native fishes in Northern Patagonian Rivers, Chile. Trans. Am. Fish. Soc. 138, 839–845. doi: 10.1577/t08-134.1
Phillips, C. B., and Pappalardo, R. T. (2014). Europa clipper mission concept: exploring jupiter's ocean moon. Eos Transac. Am. Geophys. Union 95, 165–167. doi: 10.1002/2014eo200002
Postberg, F., Schmidt, J., Hillier, J., Kempf, S., and Srama, R. (2011). A salt-water reservoir as the source of a compositionally stratified plume on Enceladus. Nature 474, 620–622. doi: 10.1038/nature10175
Poster, D. L., and Baker, J. E. (1996). Influence of submicron particles on hydrophobic organic contaminants in precipitation. 1. Concentrations and distributions of polycyclic aromatic hydrocarbons and polychlorinated biphenyls in rainwater. Environ. Sci. Technol. 30, 341–348.
Pozo, K., Urrutia, R., Barra, R., Mariottini, M., Treutler, H. C., Araneda, A., et al. (2007). Records of polychlorinated biphenyls (PCBs) in sediments of four remote Chilean Andean Lakes. Chemosphere 66, 1911–1921. doi: 10.1016/j.chemosphere.2006.07.080
Prat, N., Rios, B., Acosta, R., and Rieradevall, M. (2009). “Los macroinvertebrados como indicadores de calidad de las aguas,” in Macroinvertebrados Bentonicos Sudamericanos, eds E. Dominguez and H. R. Fernandez (San Miguel de Tucuman: Publicaciones Especiales. Fundacion Miguel Lillo), 631–654.
Quintana, J. M. (2004). Factors Affecting Central Chile Rainfall Variations at Interdecadal Scales. Master's thesis. Santiago: University of Chile.
Rabatel, A., Castebrunet, H., Favier, V., Nicholson, L., and Kinnard, C. (2011). Glacier changes in the Pascua-Lama region, Chilean Andes (29°S): recent mass balance and 50 yr surface area variations. Cryosphere 5, 1029–1041. doi: 10.5194/tc-5-1029-2011
Ramírez, C., and San Martín, C. (2006). “Diversidad de macrófitos chilenos,” in Macrófitos y Vertebrados de Los Sistemas Límnicos de Chile, eds I. Vila, A. Veloso, R. Schlatter, and Ramírez C (Santiago: Editorial. Universitaria), 21–72.
Risacher, F., Alonso, H., and Salazar, C. (2001). Hydrochemistry of two adjacent acid saline lakes in the Andes of northern Chile. Chem. Geol. 187, 39–57. doi: 10.1016/s0009-2541(02)00021-9
Rivera, P. (2006). Estado de conocimiento de las diatomeas dulceacuícolas de Chile. Gayana 70, 1–7. doi: 10.4067/s0717-65382006000100002
Rogora, M., Massaferro, J., Marchetto, A., Tartari, G., and Mosello, R. (2008). The water chemistry of some shallow lakes in Northern Patagonia and their nitrogen status in comparison with remote lakes in different regions of the globe. J. Limnol. 67, 75–86. doi: 10.4081/jlimnol.2008.75
Roig, F. A., Le-Quesne, C., Boninsegna, J. A., Briffa, K. R., Lara, A., Grudd, H., et al. (2001). Climate variability 50,000 years ago in mid-latitude Chile as reconstructed from tree rings. Nature 410, 567–570. doi: 10.1038/35069040
Rose, K., Hamilton, C., Williamson, C., McBride, C., Fischer, J., Olson, M., et al. (2014). Light attenuation characteristics of glacially-fed lakes. JGR Biosci. 119, 1446–1457. doi: 10.1002/2014jg002674
Roth, L., Saur, J., Retherford, K. D., Strobel, D. F., Feldman, P. D., and McGrath, M. A. (2014). Transient water vapor at Europa's south pole. Science 343, 171–174. doi: 10.1126/science.1247051
Russell, M. J., Murray, A. E., and Hand, K. P. (2017). The possible emergence of life and differentiation of a shallow biosphere on irradiated icy worlds: the example of Europa. Astrobiology 17, 1265–1273. doi: 10.1089/ast.2016.1600
Salerno, F., Rogora, M., Balestrini, R., Lami, A., Tartari, G. A., and Thakuri, S. (2016). Glacier melting increases the solute concentrations of himalayan glacial lakes. Environ. Sci. Technol. 50, 9150–9160. doi: 10.1021/acs.est.6b02735
Santibanez, F. (1997). “Tendencias seculares de la precipitacion en Chile,” in Diagnostico Climatico de la Desertificacion en Chile, eds G. Soto and F. Ulloa (La Serena: CONAF), 24–31.
Schagerl, M., and Pichler, C. (2000). Pigment composition of freshwater Charophyceae. Aquat. Bot. 67, 117–129. doi: 10.1016/s0304-3770(99)00095-9
Schneider, S., Ziegler, C., and Melzer, A. (2006). Growth towards light as an adaptation to high light conditions in Chara branches. New Phytol. 172, 83–91. doi: 10.1111/j.1469-8137.2006.01812.x
Schneider, S. C., Pichler, D. E., Andersen, T., and Melzer, A. (2015). Light acclimation in submerged macrophytes: the role of plant elongation, pigmentation and branch orientation differ among Chara species. Aquat. Bot. 120, 121–128. doi: 10.1016/j.aquabot.2014.05.002
Schulz-Bull, D. E., Bruhn, P. R., and Duinker, J. C. (1998). Chlorobiphenyls (PCB) and PAHs in water masses of the northern North Atlantic. Mar. Chem. 61, 101–114.
Schwarz, A. M., de Winton, M., and Hawes, I. (2002). Species-specific depth zonation in New Zealand charophytes as a function of light availability. Aquat. Bot. 72, 209–217. doi: 10.1016/s0304-3770(01)00201-7
Schwarz, A. M., Hawes, I. I., and Howard-Williams, C (1996). The role of photosynthesis/ light relationship in determining lower depth limits of Characeae in South Island, New Zealand lakes. Freshw. Biol. 35, 69–80.
Sobron, P. C., Lefebvre, R., Leveille, A., Koujelev, T., Haltigin, H., Du, A., et al. (2013). Geochemical profile of a layered outcrop in the Atacama analogue using laser-induced breakdown spectroscopy; Implications for Curiosity investigations in Gale. Geophys. Res. Lett. 40, 1965–1970. doi: 10.1002/grl.50261
Sorrell, B. K., Hawes, I., Schwarz, A. M., and Sutherland, D. (2001). Inter-specific differences in photosynthetic carbon uptake, photosynthate partitioning and extracellular organic carbon release by deep-water characean algae. Freshw. Biol. 46, 453–464. doi: 10.1046/j.1365-2427.2001.00686.x
Soto, D. (2002). Oligotrophic patterns in southern Chilean lakes: the relevance of nutrients and mixing depth. Rev. Chil. Hist. Nat. 75, 377–393. doi: 10.4067/s0716-078x2002000200009
Soto, D., Arismendi, I., Di Prinzio, C., and Jara, F. (2007). Establishment of Chinook salmon (Oncorhynchus tshawytscha) in Pacific basins of southern South America and its potential ecosystem implications. Rev. Chil. Hist. Nat. 80, 81–98. doi: 10.4067/S0716-078X2007000100007
Soto, D., Arismendi, I., González, J., Sanzana, J., Jara, F., Jara, C., et al. (2006). Sur de Chile, país de truchas y salmones: patrones de invasión y amenazas para las especies nativas. Rev. Chil. Hist. Nat. 79, 97–117. doi: 10.4067/S0716-078X2006000100009
Thomas, P. C., Tajeddine, R., Tiscareno, M. S., Burns, J. A., Joseph, J., and Loredo, T. J. (2016). Enceladus's measured physical libration requires a global subsurface ocean. Icarus 264, 37–47. doi: 10.1016/j.icarus.2015.08.037
Thorp, J., and Rogers, C. (2015). “Ecology and general biology,” in Thorp and Covich's Freshwater Invertebrates, eds J. H. Thorp and D. C. Rogers (Cambridge: Elsevier Academic Press).
Tilot, V. (2006). “Biodiversity and distribution of the megafauna Vol 1/3 The polymetallic nodule ecosystem of the Eastern Equatorial Pacific Ocean,” in Intergovernmental Oceanographic Commission. Technical Series 69, Project Unesco COI/Min Vlanderen, Belgium. Available online at: http://unesdoc.unesco.org/images/0014/001495/149556f.pdf
Tilot, V. (2014a). Assessment of Aquatic Biodiversity and Habitats of Laguna Negra, Central Andes, Chili by Analysis of Videos Taken by the Planetary Lake Lander (PLL project of the NASA/ASTEP/SETI Institute). MNHN/IEO Report to NASA.
Tilot, V. (2014b). “An innovative Multilayer Rapid Ecological Assessment adapted to monitoring and management of Deep Sea Ecosystems,” in Proceedings Deep Sea Mining Summit (London), 17–19.
Tilot, V., Cabrol, N., Parro, V., Sobron, P., Smith, W., Tambley, C., et al. (2018b). “A novel benthic ecosystem possibly associated to hydrothermal processes or gas-emissions discovered in a high altitude Chilean lake by the NASA Planetary Lake Lander (PLL) Project,” in 15th Deep sea Biology Symposium (Monterey Bay).
Tilot, V., Gente, P., and The Scientific Team. (2010). “Discovery of new faunal sites on the EPR (16°N); Abyssal, bathyal and hydrothermal communities,” in Proceedings of the 12th Deep Sea Symposium (Reykjavik).
Tilot, V., Leujak, W., and Ormond, R. (2008). Monitoring of South Sinai coral reefs: influence of natural and anthropogenic factors. Aquat. Conserv. Marine Freshw. Ecosyst. 18, 1109–1126. doi: 10.1002/aqc.942
Tilot, V., Ormond, R., Moreno-Navas, J., and Catala, T. (2018a). The benthic megafaunal assemblages of the CCZ (Eastern Pacific) and an approach to their management in the face of threatened anthropogenic impacts. Front. Mar. Sci. 5:7. doi: 10.3389/fmars.2018.00007
Uehlinger, U., Robinson, C. T., Hieber, M., and Zah, R. (2010). The physico-chemical habitat template for periphyton in alpine galcial strems under a changing climate. Hydrobiologia 657, 107–121. doi: 10.1007/s10750-009-9963-x
Valdovinos, C. (2006). Diversidad de Especias Invertebrados Dulceacuicolas. Biodiversidad de Chile: Nuestra Diversidad Biologica.
Van der Vijver, B., Beyens, L., Vincke, S., and Gremmen, N. (2004). Moss-inhabiting diatom communities from Heard Island, sub-Antartic. Polar Biol. 27:532–543. doi: 10.1007/s00300-004-0629-x
Vance, S., Harnmeijer, J., Kimura, J., Hussmann, H., Demartin, B., and Brown, J. M. (2007). Hydrothermal Systems in Small Ocean planets. Astrobiology 7:6. doi: 10.1089/ast.2007.0075
Vergara, M., López-Escobar, L., Palma, J. L., Hickey-Vargas, R., and Roeschmann, C. (2004). Late tertiary volcanic episodes in the area of the city of Santiago de Chile: new geochronological and geochemical data. J. South Am. Earth Sci. 17, 227–238. doi: 10.1016/j.jsames.2004.06.003
Villalobos, L. (2006). Estado de conocimiento de los crustáceos zooplanctónicos dulceacuícolas de Chile. Gayana 70, 31–39. doi: 10.4067/s0717-65382006000100007
Wang, H., Liu, C., and Yu, D. (2015). Morphological and reproductive differences among three charophyte species in response to variation in water depth. Aquat. Biol. 24, 91–100. doi: 10.3354/ab00638
Wang, H., Yu, D., and Xiao, K. (2008). The interactive effects of irradiance and photoperiod on Chara vulgaris L.: concerted responses in morphology, physiology, and reproduction. Hydrobiologia 610, 33–41. doi: 10.1007/s10750-008-9420-2
Wania, F., and Mackay, D. (1995). A global distribution model for persistent organic chemicals. Sci. Total Environ. 160/161, 211–232.
Ward, B. B. (2008). “Chapter:5 Nitrification in marine systems,” in Nitrogen in the Marine Environment, 2nd Edn. eds D. G. Capone, D. A. Bronk, M. R. Mulholland, E. J. Carpenter (Amsterdam: Elsevier Academy Press), 199–261.
Zemskaya, T., Sitnikova, T., Kiyashko, S., Kalmychkov, G., Pogodaeva, T., Mekhanikova, I., et al. (2012). Faunal communities at sites of gas- and oil-bearing fluids in Lake Baikal. Geo Mar Lett. 32, 437–451. doi: 10.1007/s00367-012-0297-8
Zuloaga, F., Morrone, O., and Belgrano, M. (2008). Catálogo de las plantas vasculares del Cono Sur (Sur de Brasil). Available online at: http://www.darwin.edu.ar/Proyectos/FloraArgentina/Especies.asp
Keywords: planetary exploration, rapid environmental assessment, life detection, macrophytes, diatoms, high altitude lakes, andes environment, tubeworm communities
Citation: Tilot VC, Cabrol NA, Parro V, Fairén AG, Ormond RFG, Moreno-Ostos E, Lopez-Gonzalez N, Figueroa FA, Gallardo-Carreño I, Smith EW, Sobrón P, Demergasso C, Tambley C and Robidart J (2019) A Test in a High Altitude Lake of a Multi-Parametric Rapid Methodology for Assessing Life in Liquid Environments on Planetary Bodies: A Potential New Freshwater Polychaete Tubeworm Community. Front. Environ. Sci. 7:70. doi: 10.3389/fenvs.2019.00070
Received: 06 December 2018; Accepted: 09 May 2019;
Published: 18 June 2019.
Edited by:
Henderson James Cleaves, Tokyo Institute of Technology, JapanReviewed by:
Dirk De Beer, Max-Planck-Gesellschaft (MPG), GermanyAmy Michele Grunden, North Carolina State University, United States
Copyright © 2019 Tilot, Cabrol, Parro, Fairén, Ormond, Moreno-Ostos, Lopez-Gonzalez, Figueroa, Gallardo-Carreño, Smith, Sobrón, Demergasso, Tambley and Robidart. This is an open-access article distributed under the terms of the Creative Commons Attribution License (CC BY). The use, distribution or reproduction in other forums is permitted, provided the original author(s) and the copyright owner(s) are credited and that the original publication in this journal is cited, in accordance with accepted academic practice. No use, distribution or reproduction is permitted which does not comply with these terms.
*Correspondence: Virginie C. Tilot, virginie.tilot@mnhn.fr; v.tilot@wanadoo.fr