- 1Laboratorio Nacional de Ciencias de la Sostenibilidad, Instituto de Ecología, Universidad Nacional Autónoma de México (UNAM), Mexico City, Mexico
- 2Posgrado en Ciencias Biológicas, Universidad Nacional Autónoma de México, Mexico City, Mexico
Mexico City has a population of 9 million inhabitants and was settled on a lakebed with high seismic potential. It is currently embedded in the Mexico City Metropolitan Area, which encompasses 22 million inhabitants and which was self-sufficient in water in the past, but currently extracts 70% from the regional aquifers and imports about 30% of the water required by this megacity. Groundwater represents its main water source but its water quality is increasingly threatened. The purpose of this study was to determine the water quality in areas related to seismic fractures, which may increase the vulnerability of water provision, and to identify specific zones that could be affected. Official water quality data from the period 2002 to 2017 was analyzed and compared to recent data taken in wells in the city after the September 2017 earthquake. Physicochemical parameters were determined and compared to the existing data. Statistical and temporal analyses were performed in order to understand the evolution and spatial distribution of water quality. The results show that free chlorine was below the limits according to the Mexican regulatory framework, while the presence of fecal coliforms, aluminum, ammonia, iron, and manganese exceeded the standards. The presence of arsenic, boron, and chrome was detected in some areas. Clusters show specific parameters that increase with time: turbidity, sulfates, nitrates, arsenic, manganese, lead, and iron. These tendencies could imply the deterioration of groundwater quality and a potential effect on the health of the exposed population. Spatially, vulnerability was observed in Iztapalapa, Tláhuac, Xochimilco, and Coyoacán. Wells coincide spatially with some of the geological damaged areas from the earthquakes in Iztapalapa and Xochimilco. In addition to water quantity, water quality represents a challenge for the urban future, since water disinfection systems are limited to treating the diversity of compounds detected. The water quality-monitoring program must be changed to improve its capacities within a framework of sustainable water management in different regions of the city, and based on the season, considering the potential exposure to the city's population. This represents an opportunity to propose a strategic plan for the groundwater system in order to improve conditions toward a more equitable and sustainable pathway for Mexico City.
Introduction
The Mexico City Metropolitan Area (MCMA) is a megacity of 22 million inhabitants. It is located within the Basin of Mexico, which covers an area of 9,600 km2 and is at an elevation of 2,240 meters above sea level. In order to supply water to the city, groundwater exploitation started in the 1800s, with 1,070 wells drilled by 1899 (Ortega and Farvolden, 1989), and by 1990 a total of 3,537 registered wells were reported (National Research Council (NRC), 1995; Carrera-Hernández and Gaskin, 2007b).
Geological and Geophysical Setting
Seismic zones are related to the origin of the lacustrine plain in which Mexico City was built. According to Auvinet et al. (2017) the lacustrine plain was formed by progressive silting of the basin reservoir, due to the rising of Sierra del Chichinautzin. Silting with volcanic products and river drift propitiated conditions for the formation of a lake system within the Basin of Mexico. The bottom of the basin was silted up with stratification of different materials; the most important being the lacustrine materials including clays and silts, silts and sands, as well as the volcanic ashes that upon weathering generated highly compressible clays. The erosion of the foothills, river drift and deposits of volcanic origin built up a fill that gives the current variation of the sediments and thickness, therefore increasing the vertical extent of the deposits in a north-south direction. Groundwater quality in Mexico City is closely related to these geological formations, which present different hydraulic conductivities, permeabilities, as well as to the type and depth of the extraction wells.
The geotechnical characteristics of Mexico City have been carefully studied and described since the late 1950s. Marsal and Mazari (1959) presented the first zoning of the soils. Reséndiz et al. (1970) and Del Castillo (1978) updated this first description, including new urbanized areas of the city. A more recent map of geotechnical zones was incorporated in 2004, related to the Technical Norms, and the latest version was developed related to the Metro Geotechnical Design (COVITUR) (Santoyo et al., 2005).
As a result of the different geotechnical studies the areas in Mexico City have been described as Lake, Transition and Hill Zones.
The Lake Zone, with soft clays with large thicknesses, has also been divided in three areas:
(a) Virgin Lake, in non-urbanized areas that are considered soft clays, (b) Central Lake I, that includes the urbanized areas in the non-colonial area of the city, with small and medium size constructions, representing an intermediate condition, and (c) Central Lake II, limited by the old colonial trace of the city, with a history of heavy buildings from Aztec to colonial periods, with consolidated clays, soft clays in areas covered by plazas and gardens, and very soft clays in the areas where old canals were located. Additionally, it is important to consider the induced consolidation due to groundwater extraction (Santoyo et al., 2005).
The Transition Zone, located in the border of the lake zone where the lower clay area disappears, includes a hard layer at a depth of 20 m with respect to the average level of the plain. The Transition Zone has been divided in:
(a) High Transition Zone, the one closest to the hills, with stratigraphic irregularities due to the presence of interspersed alluvial deposits, and (b) Low Transition Zone, next to the lake area where the clay layer presents interspersed sandy slime of alluvial origin, deposited during the regression of the ancient lake system, (c) Abrupt Transition in the transition between the lake zones and some isolated hills.
The Hill Zone, located in the surroundings of the city, presents irregular conditions of compactation and cementation.
The described areas were used after the Mexico City 1985 earthquake to generate the Seismic Zonation of the Construction Regulation of the Federal District (now referred as Mexico City), considering all the sources that contribute to seismic risk, and therefore the specific geotechnical conditions in each zone. The map was constructed based on norms of the maps of natural iso-periods of the soil, obtained based on the environmental vibrations, which gives the mean dynamic of the subsoil of Mexico City. Nevertheless, what is considered the most important factor is the local seismic intensity of the subsoil (Santoyo et al., 2005).
Water Extraction and Subsidence
Water is extracted from the regional Quaternary alluvial unit, a pyroclastic and fractured system, overlaid by a compressible lacustrine aquitard (Carrera-Hernández and Gaskin, 2007a; Hernández-Espriú et al., 2014). A regional approach to the groundwater dynamics of the Basin of Mexico has been described by Carrera-Hernández and Gaskin (2007b) and Hernández-Espriú et al. (2014).
Extraction from the aquifer in 1950 was 13.7 m3/s (Mazari and Alberro, 1990), increasing to 52.2 m3/s in the 2000s (Jiménez et al., 2004). Groundwater extraction provides about 72% of the total demand (72.5 m3/s) (Jiménez et al., 2004).
Over the last century, intensive groundwater pumping in this area has led to significant drawdowns and consequentially differential land subsidence, a phenomenon studied since the 1950s (Marsal and Mazari, 1959; Carrillo, 1969; Hiriart and Marsal, 1969), which has effects on urban infrastructure and leads to leakage and potential contaminant propagation to the aquifers (Mazari-Hiriart et al., 2006; Hernández-Espriú et al., 2014). Records show that since the 1900s, some downtown locations have subsided by 9 m (Marsal and Mazari, 1990). Intensive and continuous pumping since the 1940s has caused a differential land subsidence of 6 cm/year in the downtown area to 30 cm/year in the eastern and southern sections of the city (Mazari and Alberro, 1990; Mazari et al., 1992).
The Mexico City Metropolitan Area developed on a lakebed and a highly seismic zone, dependent on groundwater for public supply, a resource that due to its hydrogeological setting is extremely vulnerable (Mazari-Hiriart et al., 2006). This vulnerability not only reflects on water quantity, but also water quality, since often it contains various potential contaminants that are introduced into the aquifer system.
Changes in soil layers due to an earthquake could affect the city's topography; consequently, soil components may come in contact with groundwater, affecting its quality. Important consequences of earthquakes are the fractures in the geologic materials that allow the release of chemicals and may cause other effects, such as change in the groundwater level and the appearance and disappearance of springs (Malakootian and Nouri, 2010). Cities worldwide located in seismic zones have faced changes associated with fractures and variations of soil layers. The natural dynamics of groundwater could be altered in the presence of earthquakes, which has been observed in Santa Barbara County in California (Wood, 2014), the Bam Plains and Baravat in Iran (Malakootian and Nouri, 2010), and in Katmandu, Nepal (Uprety et al., 2017). These changes may be of different magnitudes and may occur at spatially diverse places within the affected areas, which pose complex associated challenges for the city's management authorities. Specifically, attention must be paid on how to prepare to face risks related to water quantity, quality, therefore the availability of water with variations in the water table due to seismic events (Malakootian and Nouri, 2010; Wood, 2014).
Due to the September 2017 earthquakes, some fractures became evident in the contact zone between hard and soft geologic materials in the southern area of Mexico City, where some of the extraction wells that supply the city are located. The aim of this research was to determine the water quality in areas related to fractures, which may increase the vulnerability of water provision, and to identify any change in the condition of water sources that would be important for decision-making in the city.
Study Site
Based on the information generated during several sampling sessions by the Laboratorio Nacional de Ciencias de la Sostenibilidad (LANCIS) from June to October 2018 and official databases requested from the Water Authority of Mexico City (Sistema de Aguas de la Ciudad de México, SACMEX), we selected sampling sites close to fractures and geologic faults, as well as the frequency of complete datasets, considering water flow (local, intermediate, and regional). Forty-one wells were selected to perform a sampling campaign after the earthquakes (Figure 1).
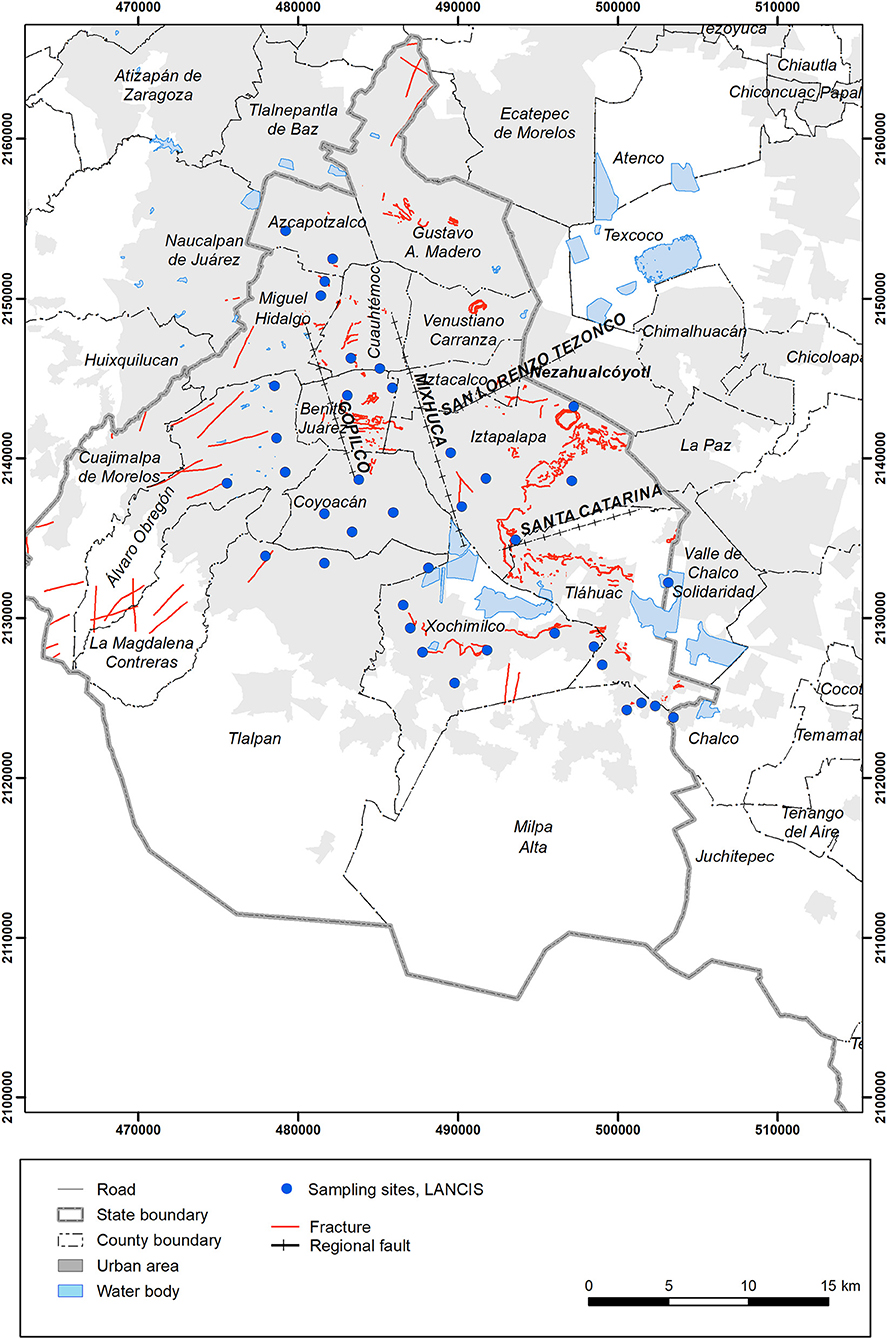
Figure 1. Spatial location of the selected wells (blue dots) to carry out a water quality sampling campaign. Fractures in red and geologic faults in black (based on information in Table 1).
Methods
Information Systematization
We conducted a search and systematization of the available databases and spatial layers of the following physical characteristics: (i) geology; (ii) fractures; (iii) geological faults, (iv) topography; (v) seismic zoning; (vi) infrastructure; (vii) location of wells, and (viii) well flow (Table 1). All spatial layers are in UTM-14 projection and WGS84 datum.
Water Quality Information
Water quality information was obtained from SACMEX, and was selected for wells with complete information that was organized and then associated with the Water Quality Guidelines of water supply for urban public use (Conagua, 2016) and related it to the current Mexican Regulation NOM-127-SSA1-1994 (DOF, 2000). Groundwater quality parameters were selected according to the following criteria: (1) Data reported after the September 2017 earthquakes, and (2) Data sequence reports as complete as possible during the period 2002–2017. The water quality parameters that were selected are shown in Table 2.
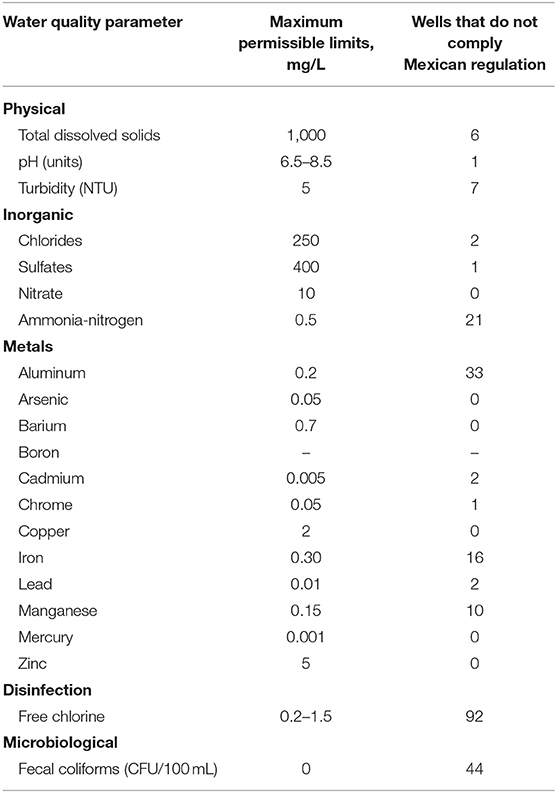
Table 2. Summary of the results of groundwater quality information from wells located in Mexico City according to the Maximum Permissible Limits of NOM-127-SSA1-1994 (DOF, 2000).
Groundwater Sampling Campaign
In order to have recent information and compare it with water quality before and after the seismic event (19 September, 2017), a single sampling campaign was organized to take samples of 41 wells. The city's Water Authority (SACMEX) also accompanied sampling taken throughout the period of June through August 2018.
Physicochemical parameters of water quality assessment were performed in situ by multiparametric probe EXO2 (YSI). Physicochemical parameters were flow (L/s), temperature (°C), electrical conductivity (μS/cm), total dissolved solids (mg/L), salinity (psu), dissolved oxygen (mg/L), pH, ORP (mV), and turbidity (NTU). Inorganic compounds were analyzed by spectrophotometry with the HACH method: ammonia (mg/L, Nessler Method, 8038); nitrates (mg/L); cadmium (mg/L, Reduction Method, 8039); organic phosphorus (mg/L, PhosphoVer3®, 8048); sulfate (mg/L; SulfaVer®, 8051), and free chlorine (mg/L, DPD, 10245). Microbiological analysis was performed by Membrane Filtration Technique (American Public Health Association, 2005), and microbiological indicators were Fecal Coliform (CFU/100 mL) and Escherichia coli (CFU/100 mL).
Statistical and Spatial Analysis
Cluster Analysis
A cluster analysis was performed in order to identify groups of wells with a similar pattern. Previously, water quality data was transformed to values between 0 and 1, to allow comparison and data analysis. Data transformation was done using the R version 3.5.1 statistical package. Clusters were identified and analyzed applying Normal Mixture Modeling for Model-Based Clustering, Classification, and Density Estimation (Fraley et al., 2014). Bayesian Information Criteria (BIC) was used and graphic visualization to determine the model and appropriate number of clusters. BIC allows the comparison of the different models with several parameterizations or different number of clusters. In general, the larger the BIC value, the stronger the evidence for the model and number of clusters (Fraley and Raftery, 2002). The characteristics of each cluster were identified and visualized with the construction of star graphics (Figure 2).
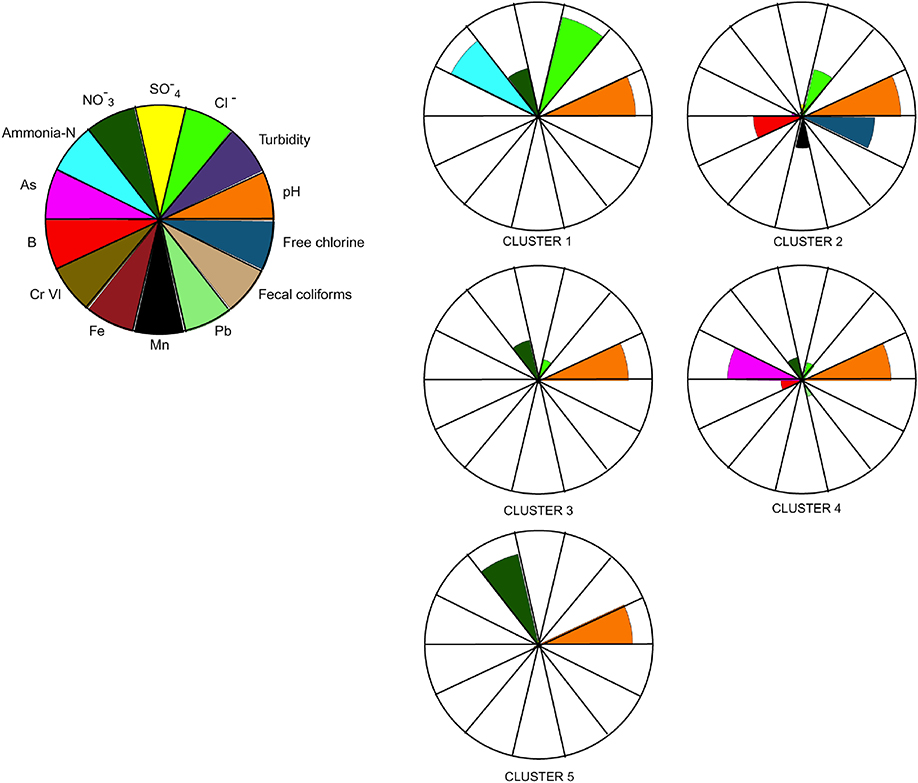
Figure 2. Cluster models generated with groundwater quality information, figure shows number of data included to generate clusters. Number of data by cluster 231, 149, 347, 561, and 254 for cluster 1, 2, 3, 4, and 5, respectively.
Temporal Trend Analysis
Mann-Kendal and Thiel-Shien tendency statistical tests were performed to detect changes in the groundwater quality information obtained from SACMEX.
The tendency statistical tests of Mann-Kendall and Thiel-Sen were applied for the detection and estimation of tendency and magnitude, respectively, in the temporal annual series. Therefore, the presence of an increasing tendency or decreasing monotonic was demonstrated by the non-parametric Mann-Kendall non-parametric test; then, the slope of the linear tendency was estimated based under the Thiel-Sen non-parametric method (Gilbert, 1987). These tests have no distribution assumptions and allow for atypical or missing data or atypical data (Gibbons et al., 2009). The Mann-Kendall and Thiel-Sen graphic visualizations were performed with ProUCL (USEPA, 2010).
Spatial Analysis
Based on information reported by the Institute of Geology (Olea, 2017, unpublished report) regarding underground flux types of the Mexico City extraction wells, we located each well in order to visualize and compare spatial patterns. Each well with the corresponding cluster was mapped and compared spatially. Similarly, we overlapped the well cluster with the geophysical and geological features (regional faults, fractures, reliefs, and seismic zones), as well as zones damaged as a result of the seismic events of September 19, 2017, in order to identify vulnerable areas for groundwater quality. The analysis was performed using ArcGIS 10.2.2 (ESRI, 2014).
Results
Water Quality Information
According to the results obtained from the water quality information provided by SACMEX during 2002–2017, we found 107 wells that had the most complete information during this period. From these wells, we observed that free chlorine is the parameter that was frequently found below the recommended limit (Table 2) by NOM-127-SSA1-1994 (DOF, 2000). This parameter is relevant given its importance as a disinfectant, and it is one of the parameters of water quality that can be regulated, unlike other parameters (OMS, 2006). A low concentration of chlorine can be related to the presence of fecal coliform bacteria in water, since disinfection is not carried out properly. These results are confirmed by the presence of CF in 44 of the 107 wells. Aluminum, ammonia nitrogen, iron, and manganese were the parameters that mostly exceeded the limits established by the Mexican Regulation in 33, 21, 16, and 10 wells, respectively (Table 2, column 3).
Groundwater Sampling Campaign vs. Water Quality Information
One of the main purposes of this research was to exploit the possible differences in water quality parameters that are monitored by the local water authority (SACMEX), which have been generating data for many years, as well as data registered after the September 2017 earthquakes.
The analyses performed with pre-earthquake and post-earthquake data only allowed us to identify a significant difference for the pH data. Nevertheless, it is important to note that this research was conducted 9 months after the 2017 earthquakes, due to paperwork and permit requirements. In other reports elaborated in different parts of the world, differences have been reported for oxygen isotopes (Onda et al., 2018), electric conductivity, total dissolved solids, cations, and anions (Malakootian and Nouri, 2010), as well as in microbiological community composition (Uprety et al., 2017). In such cases, water quality monitoring was performed continuously, and when the earthquake occurred, it was possible to have a more solid database.
In this study, even though we did not have access to water quality data of dates close to previous earthquakes, it was possible to observe some tendencies in the variation of parameters registered for 15 years, a topic discussed in section Temporal Trend.
With respect to water quality regulations, it is important to point out that in Mexico, there are specific regulations, such as NOM-127-SSA1 (DOF, 2000), which establish the permissible limits of chemical contaminants and microbiological parameters, as well as the water organoleptic conditions required for human use and consumption. Additionally, regulation NOM-179-SSA1 (DOF, 2010) outlines the monitoring frequencies, considering variation in the parameters, and the measuring methods for each parameter. Both regulations are applicable for water that is to be used and consumed, that is, water that has received some type of disinfection to be able to comply with both regulations (DOF, 2000, 2010) and that is distributed to the population.
The water analyzed in this study during the 2018 campaign (June-August) was groundwater without any treatment; therefore, we reiterate that water regulations NOM-127-SSA1 and NOM-179-SSA1 were only used as reference and not as a standard for compliance.
Statistical and Spatial Trend
Cluster Analysis
Five clusters were obtained during the 2002–2017 period (Figure 2). The cluster analysis consisted of 14 groundwater quality parameters from 107 wells. Clusters 4 and 3 group the majority of the data/clusters with 561 and 347, respectively; while clusters 5, 1, and 2 group 254, 231, and 149 data/clusters, respectively. Cluster 4 was characterized based on highest arsenic concentrations and low concentrations of boron, lead, nitrates, chlorides, and high pH. Cluster 3 characterizes low concentrations of arsenic and boron, under low concentrations of nitrates and chlorides, with high pH values. In contrast, cluster 5 was characterized by high concentrations of nitrates and high pH values. Cluster 1 was characterized by high concentrations of ammonia and chlorides, as well as low concentrations of nitrates. Cluster 2 was characterized by a moderate concentration of boron and manganese, chlorides, and residual chlorine, and high pH values.
Temporal Trend
Based on the water quality analyses, we observed a great heterogeneity of water quality characteristics in the Mexico City wells. An important aspect of the analysis are the potential health risks associated with water extraction from these wells, since there is a tendency for the concentration of metals, such as arsenic, boron, lead, and manganese, to increase over time (Table 3). Only chrome VI showed a significant decrease, which was observed in 74 wells. Chlorides, sulfates, and nitrates showed a significant increase over time in 32, 32, and 67 wells, respectively. Both free chlorine and fecal coliforms did not follow a significant temporal trend.
Spatial Trend
The spatial analysis of 107 wells classified by flow type -local, intermediate, and regional- as depicted in Figure 3. The majority of the wells are of intermediate flow, distributed in the central zone of Mexico City, running from north to south. The local flow wells are mainly distributed in the southern and southwestern counties (alcaldías), including Milpa Alta, Xochimilco, Tlalpan, Magdalena Contreras, Álvaro Obregón, and Benito Juárez. The regional flow well area is mainly located in the eastern area of Mexico City, principally in Iztapalapa and Tláhuac counties. These wells are characterized by high ammonium concentrations, chloride, and high pH values, as well as low concentrations of nitrates.
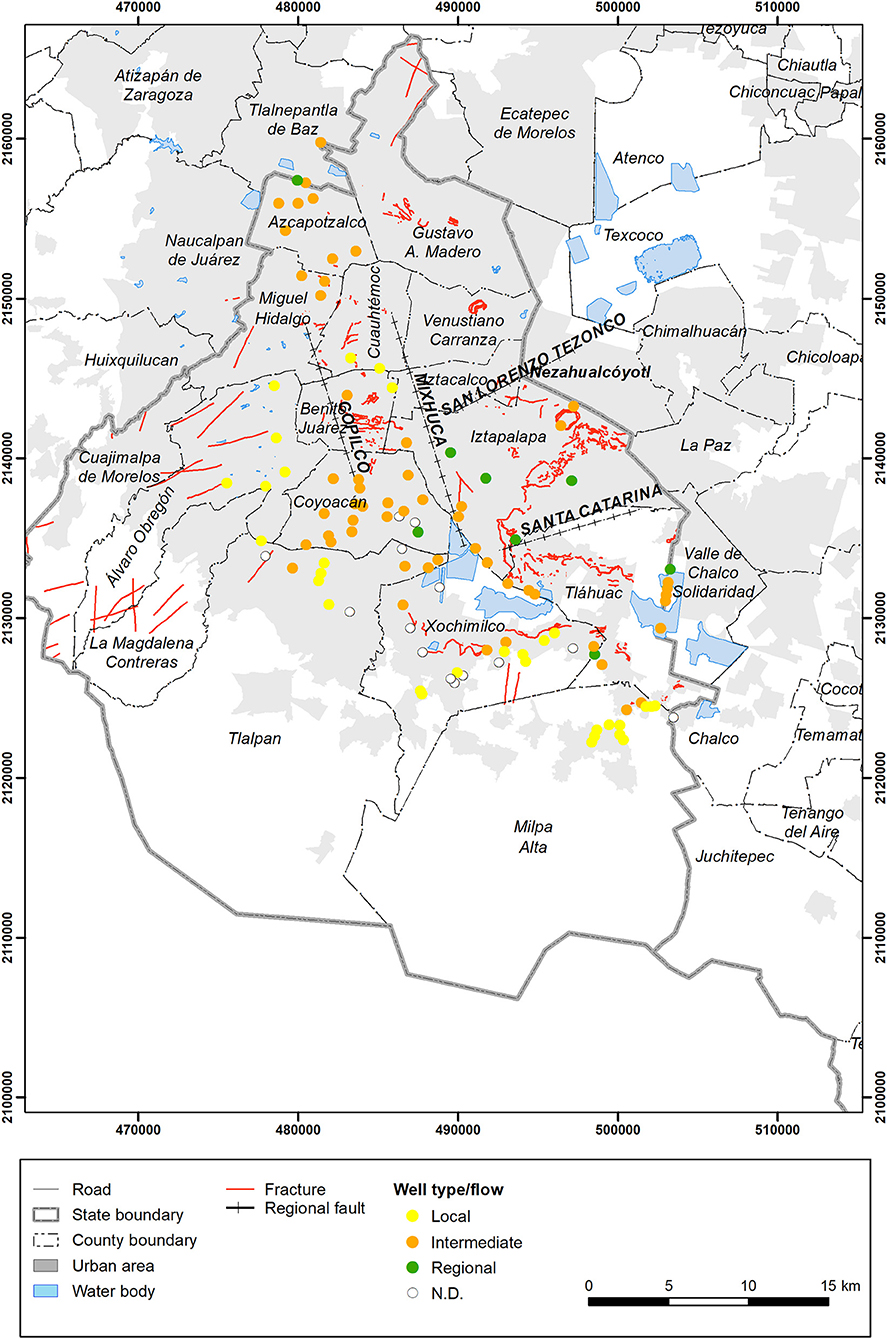
Figure 3. Representation of wells with different flows, local (yellow), intermediate (orange), and regional (green).
The seismic zones and clusters on water quality are shown in Figure 4, note fractures mainly on zones iiia and iiib of the Lake Zone, and ii of the Transition Zone, as well as most damaged zones in the 2017 earthquake (purple delimitation). The geological characteristics represented by the permeability (%) and different well types according to flow are depicted in Figure 5. In order to show spatially the integration of this information and to understand this complex system, an overlap of the two previous maps was performed and presented in Figure 6. It is possible to observe the lower permeability zones match with the seismic zones, and the location of the fractures (red lines).
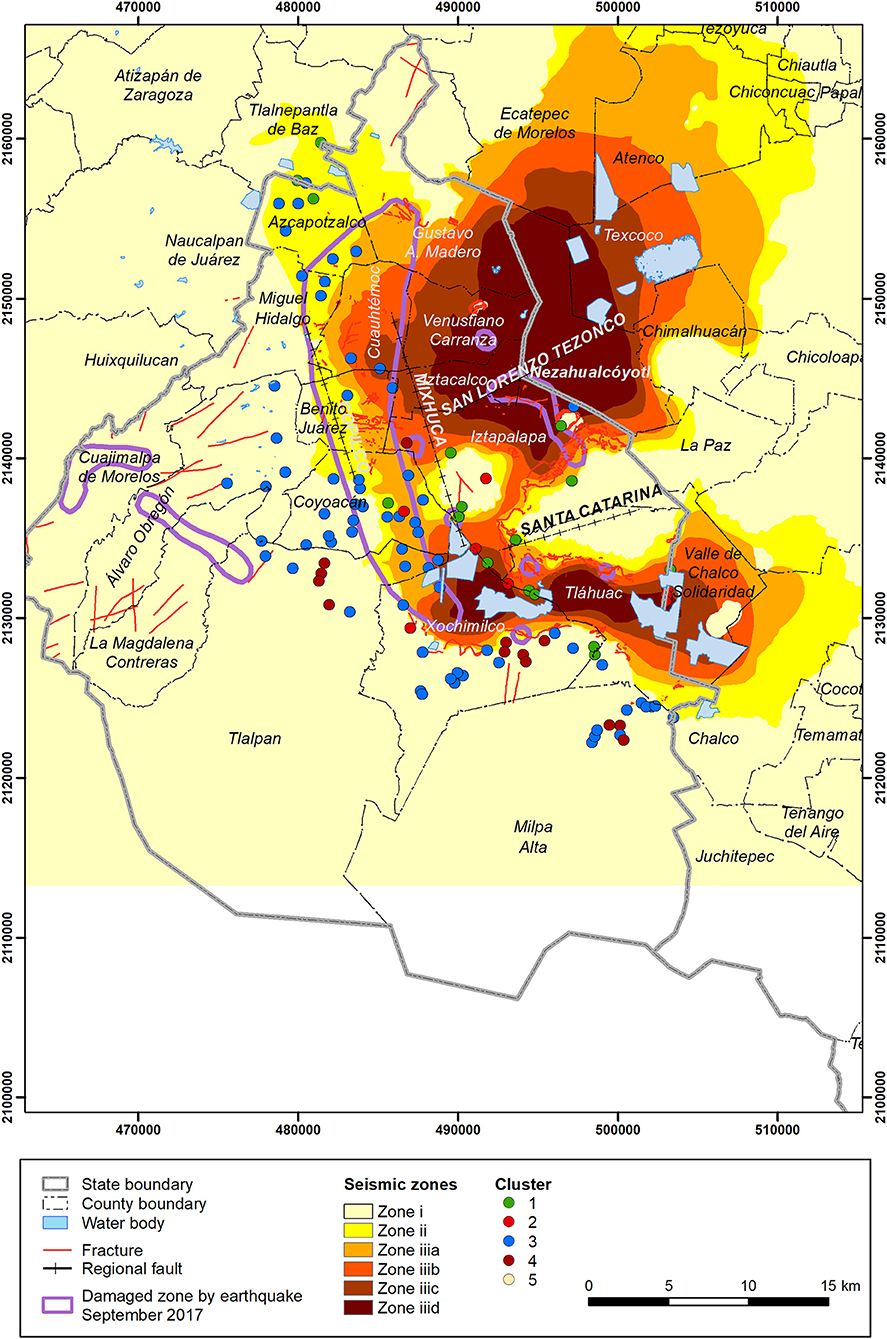
Figure 4. Spatial representation of groundwater quality clusters, related to seismic zones, damaged zones in the 2017 earthquake (pink delimitation).
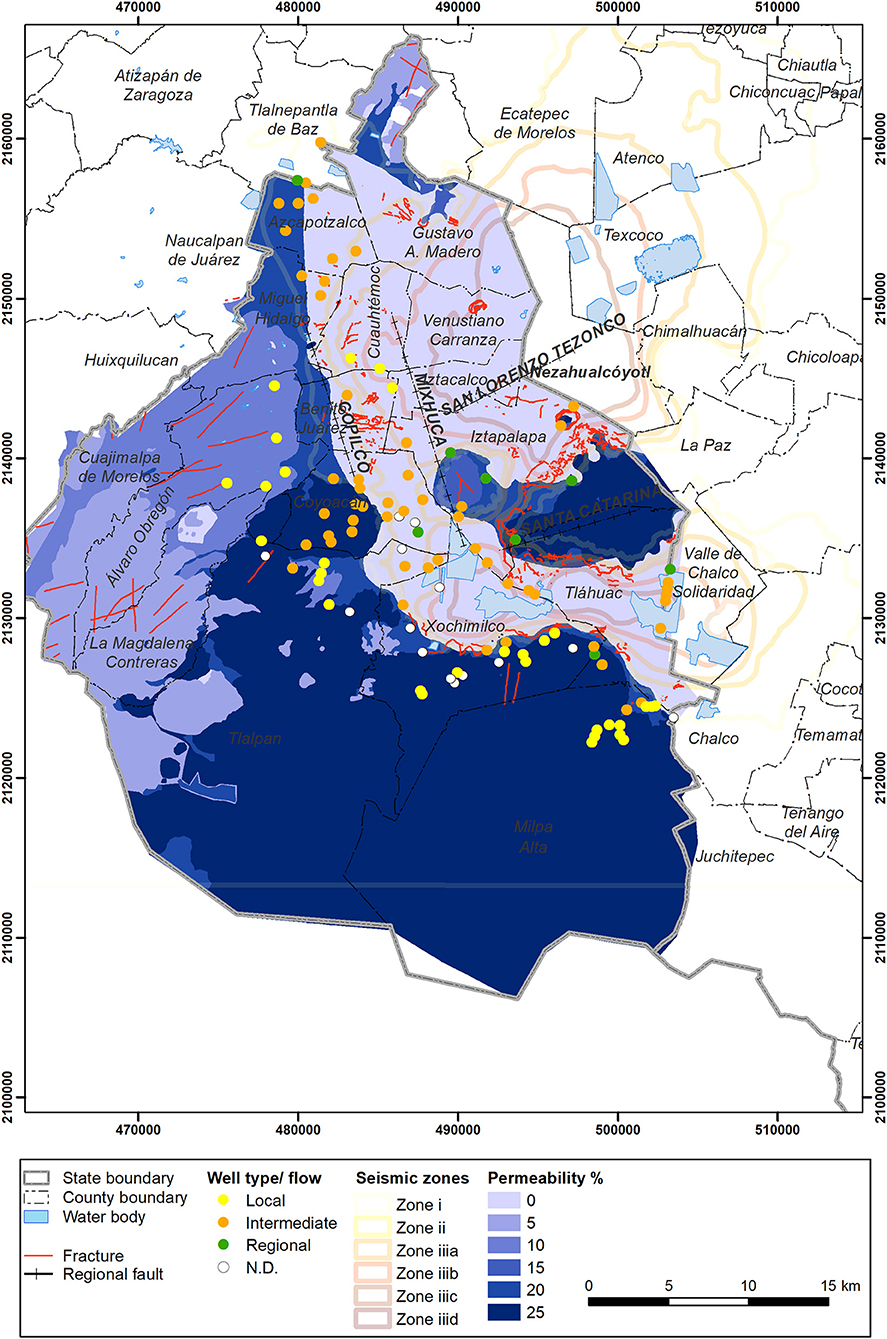
Figure 5. Spatial representation of permeabilities and wells with different flows local (yellow), intermediate (orange), and regional (green).
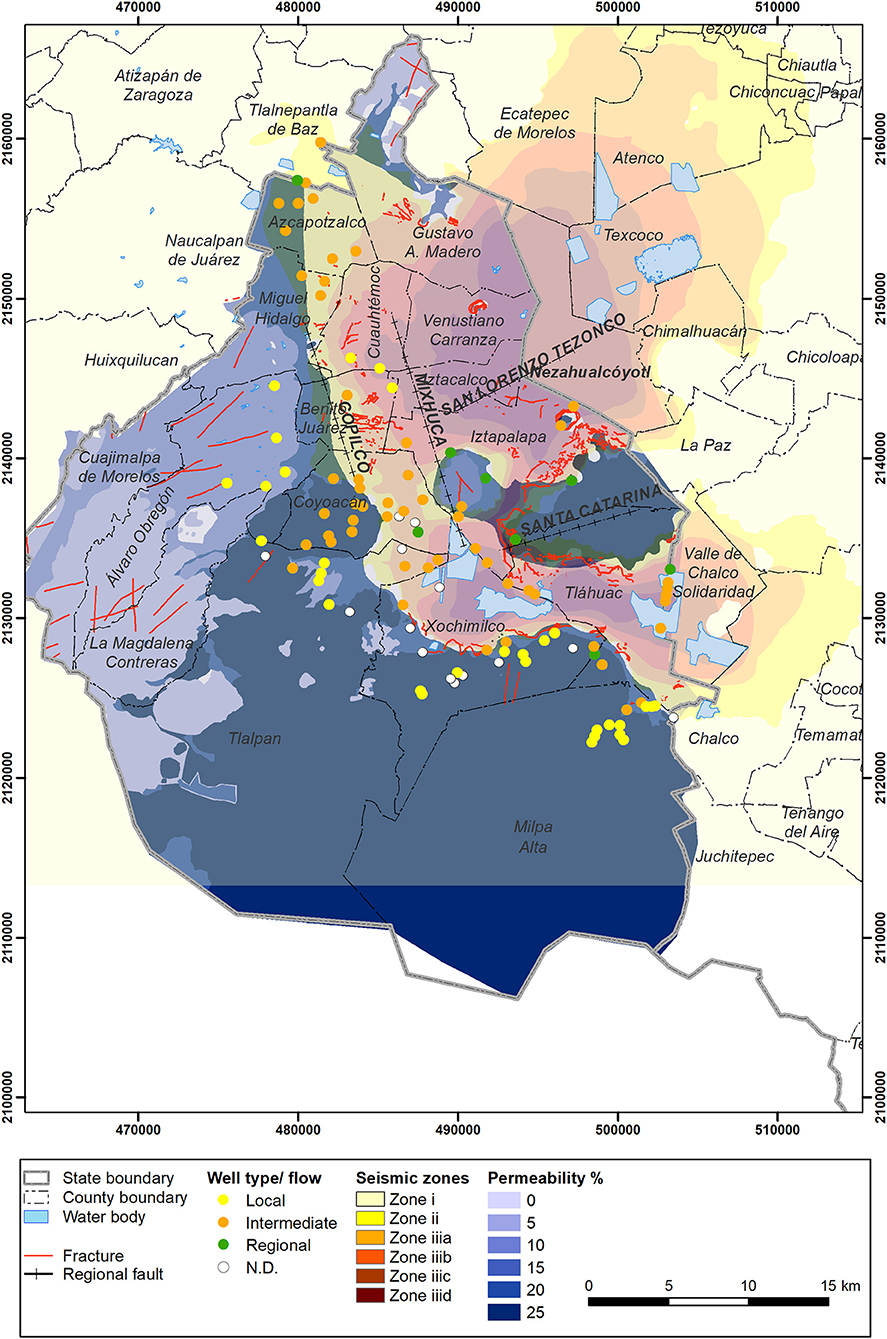
Figure 6. Overlap of seismic zones with permeabilities and wells of different flows local (yellow), intermediate (orange), and regional (green).
It is possible to observe that cluster 2 is located in Tláhuac. Additionally, the well in the Cerro de la Estrella piedmont, with a permeability of 20%, which is within the limits of Xochimilco and Tláhuac, has a moderate concentration of manganese, boron, and chloride, and relatively high values of pH, as well as higher free chlorine concentrations. It is important to note that this is located in seismic zone iiib, iiic, and iiid, but in the lake area with low permeability, such as clay, with high water content, which favors seismic wave transport.
In the central area of Xochimilco, north of Tlalpan and the northeastern area of Milpa Alta, it is possible to observe cluster 4, which presents high arsenic concentrations, low boron and lead values, as well as high nitrates and chloride. In Xochimilco, this condition coincides with the spatial location of fractures and interspersed materials of the Lake and Transition Zone with permeabilities of 20–25%.
Wells in cluster 3 are distributed mainly in the Lake and Transition seismic zone ii, iiia, and iiib, presenting low concentrations of nitrates and chlorine, as well as relatively high pH values. These wells are mainly of intermediate flow and coincide with areas of major damage during the September 2017 earthquakes.
Discussion
In an earthquake in Mexico City the lacustrine clays produce an amplification effect of the seismic waves, therefore it is important to describe the area based on its geophysical and geological characteristics. In this case we relate the earthquake impacts to the presence of fractures in contact areas of different materials, and explore the water quality data in order to assess if seismic zones may have an effect on water quality.
Considering seismic zones also related to the areas with relatively high sinking velocity, which may affect infiltration, that flooding events can occur, and that the well structure could be damaged, there is a need to evaluate all these phenomena and the potential influence on the water quality.
The clay layer with very low permeability protects to some extent the aquifer in the Lake Zone, which corresponds to the area marked as the seismic zones colored in orange in Figure 4 iiid, iiic, iiib, iiia, but that it is also prone to fracture formation in the areas in contact with different materials such as Transition and Hill zones, fact that needs to be taken into account.
In a monitoring campaign of 41 wells between June and August of 2018, after the September 2017 earthquake there was no evidence of recent alteration; these based on a single monitoring campaign. The evidence found by analyzing data of 107 wells during 15 years indicated a temporal tendency for the concentration of certain compounds in specific areas of the city, mainly located in the Lake Zone iii in the Southern area of the city including Xochimilco, North of Tlalpan, Milpa Alta, and Tláhuac. These areas coincide with the most affected area since seismic waves entered the city in this particular earthquake in a southeast-northwest direction, therefore showing this was already a problematic area and we don't know if this was exacerbated after the earthquake. No evidence was found from the only sampling effort that took place close to the event.
The water quality of the wells located in the Western area of the city also corresponds to the zone where the aquitard presents the largest drawdown percentages (>60%), as reported in areas with clay thickness smaller than 25 m (Auvinet et al., 2017), a condition that may also influence water quality.
Local, intermediate and regional flux type wells were used in order to assess if there was a difference in water quality according to flow regime. According to Tóth (1963) and Olea (2017) there would be an effect of the depth of the flux system, if deeper the water flux may be slower, and therefore could present a different quality. The local, intermediate and regional flows would allow us to get different information since water is flowing in the local flux from a recharge area with a high topography and discharging in the lower topographic area, an intermediate flow in which recharge and discharge areas do not occupy higher or lower places, respectively, and a regional flow where the recharge area is in the main watershed limit and the discharge zone is at the bottom of the basin. In order to understand if fractures affect wells with a different flux system, the wells analyzed were divided in these categories.
Indeed the cluster statistical analysis showed five patterns of water quality for the 107 extraction wells analyzed. In general terms, intermediate flow wells correspond to cluster 4, in which arsenic, lead and nitrates are relevant; local flow wells correspond to cluster 3, in which the most relevant parameters were nitrates and arsenic; and regional flow was represented by cluster 1, with the presence of ammonia and nitrates as relevant parameters. Clusters show, besides specific parameters types, wells with variable water quality. A more detailed study along a larger period of time is needed in order to get a better understanding of the water quality dynamics, including recent water quality data.
Of what is possible to say based on the available information, there is a trend of fracture formation in the contact areas of different materials and seismic zones (Figures 4–6), where wells are present and therefore this may correspond to overexploited areas with different stratigraphy and geologic characteristics, which in some specific areas is already affecting water quality. The more worrisome is that this trend will not stop and groundwater quality will continue to be contaminated and most likely the areas will increase.
At present the requirements for better environmental information are useful, not only for keeping track of water quality through monitoring programs, but also are useful for the implementation of actions relevant from the public health perspective and for the well being of the population. This is the case in water planning distribution, prioritizing investments for water management, facing environmental variations (climatic), responding to sanitary crises, and facing extreme dry events, flooding, and earthquakes. Adequate information is critical to face any problem related with water. Therefore, it is necessary to design and maintain a system that would be transparent, of reliable quality, and efficient, these latter currently a necessity anywhere worldwide (Horne, 2015; Plana et al., 2019).
Mexico City faces challenges to comply with constitutional mandates related to adequate quantity and quality of water for its inhabitants. There is a vast amount of information generated by the water authorities that has not been analyzed temporally and spatially, and that would aid in decision making for assuring minimal quality standards for the water distributed in the city.
It would be very useful to analyze databases of prolonged time periods, longer than 10 years, in order to understand tendencies of parameters such as fecal coliforms associated with free chlorine, as well as iron and manganese in certain areas of the city, and to monitor chemical contaminants strictly in industrial areas.
Water quality may be affected by extreme events such as earthquakes. Therefore, a baseline analysis of the regional water quality would be very useful to compare regular risks and potential contamination during extreme events such as earthquakes and climate extremes (drought and floods).
The main method of water disinfection in Mexico is chlorination. Our data show that it is evident that this process is not adequately performed, since the analysis shows that the majority of the wells do not comply with the minimum standard. In extreme events, this has been a problem, considering that the first obstacle evident following an event is the inadequate water quality due to lack of disinfection.
The existence and use of databases with hard data generated by an efficient water quality monitoring system would be imperative to support decision making related to water quality. Specifically, there is a need to examine the microbiological and chemical components in water, which can have impacts on acute-infectious and chronic-degenerative diseases. These databases would also contribute to our understanding of water quality issues related to seismic activity, as observed in Nepal, Iran, and Japan (Banjara and Paudel, 2017; Onda et al., 2018).
Therefore, it is necessary to consider technical, social, and financial feasibility for the implementation of a water quality monitoring system for Mexico City, as well as the Metropolitan Area. This means that it should integrate the water supply to the megacity, its intensely exploited aquifer, heterogeneous infiltration (Mazari-Hiriart et al., 2006), and high leakage into the pipeline (40%) and drainage system. All these issues should be considered for Mexico City, which is one of the 20 most vulnerable cities to earthquakes worldwide, as well as being the economic center of Mexico.
Vulnerable areas of Mexico City, such as Iztapalapa, Tláhuac, Xochimilco, and Coyoacán, represent 36% of the city's population (INEGI, 2015). These areas have a particularly young population, being one of the more sensitive sectors of the population, a fact that the health and environmental authorities should take into account.
Specifically, for this change in paradigm of groundwater management, it is fundamental to have databases of solid data based on an efficient water quality monitoring system with defined indicators. The idea is that the databases should be the main source of information for the analyses and decision-making process for the city.
Conclusion
The data analysis from SACMEX (2002–2017) shows high heterogeneity in the water quality parameters. Due to the health risk, the concentrations of heavy metals (arsenic, mercury, boron, iron, manganese. and lead) stand out because they increase over time. This observation is of concern because if the aquifer is altered in an extreme event, this would be irreversible, with serious consequences for the water supply of several areas of Mexico City.
The water sampling that took place during 2018 allowed us to acquire a basic understanding of the groundwater conditions several months after the earthquake. The information obtained was consistent with the tendencies identified with the SACMEX database analysis.
Although it was not possible with such a small amount of data to relate groundwater quality parameters to the effects of the 2017 earthquake, it was possible to identify zones that were affected to a larger extent, specifically in the Southern, Northern, and Southwestern areas of the city, and these areas coincide with the increasing concentration of very important contaminants. Monitoring should continue to observe the evolution of specific parameters in order to reduce or control the potential risks related to the exposure of the city's inhabitants, especially in particular areas. We also recommend comparing this data to existing health reports in the more vulnerable parts of the city.
The trend observed for groundwater quality is extremely alarming in Mexico City, particularly for parameters such as arsenic and lead in the water that is being distributed throughout the water system. This trend is likely to continue and even expand to larger areas with low-income residents, generating greater social inequities in densely populated areas of the city. The environmental authority should strictly control contaminant sources in order to reduce the presence of these dangerous chemicals in Mexico City's intensely exploited water resources. Additionally, groundwater exploitation should be reduced, if possible, to stop the release of some of these contaminants from the geologic formations.
The activities of a new administration open opportunities for innovation in this water system, on which a large number of people depend as inhabitants of this complex megacity.
Data Availability Statement
The datasets for this study will not be made publicly available because the databases are confidential, data generated by Sistema de Aguas de la Ciudad de México (SACMEX). We were allowed to analyze it and present the spatial results and conclusions.
Author Contributions
MM-H, AE-G, and MT-P conceived the study. MT-P and AE-G performed the field and analytical work. AE-G supervised the laboratory tests. AZ-A, MT-P, and MM-H performed the statistical and spatial analyses. MM-H, AE-G, and MT-P wrote the manuscript. AZ-A elaborated all maps. All authors read and approved the final manuscript.
Funding
This research was carried out in 2018 with financial aid from the Comité Científico de Reconstrucción y Futuro de la CDMX, at Laboratorio Nacional de Ciencias de la Sostenibilidad, Instituto de Ecología, Universidad Nacional Autónoma de México, México. Project Eco-IE487, to carry out the study: Estudio sobre el estado actual de mantos acuíferos, la explotación de pozos, el abasto real y la demanda actual y potencial del líquido en la Ciudad de México.
Conflict of Interest
The authors declare that the research was conducted in the absence of any commercial or financial relationships that could be construed as a potential conflict of interest.
Acknowledgments
The authors would like to acknowledge Sistema de Aguas de la Ciudad de México (SACMEX) for providing the database for the period 2002–2017 and logistic support during fieldwork. We are grateful to Erick R. Hjort-Colunga, Rosa Solano-Ortiz, Jesús Sotomayor-Bonilla, and Blanca E. Hernández-Bautista, students and technicians of the Laboratorio Nacional de Ciencias de la Sostenibilidad, Instituto de Ecología, Universidad Nacional Autónoma de México, for their valuable help during field and lab work, as well as to Amy Lerner for reading the final English version of this manuscript. We are grateful for the SNI-CONACYT grant assigned to MT-P as Research Assistant (2018).
References
American Public Health Association (2005). Standard Methods for the Examination of Water and Wastewater. Washington, DC: APHA.
Auvinet, G., Méndez, E., and Juárez, M. (2017). El Subsuelo de la Ciudad de México/The Subsoil of Mexico City. Vol. III. Mexico, DF: Instituto de Ingenieria, UNAM.
Banjara, S., and Paudel, S. R. (2017). Earthquake-science in himalayas: ground water quality change and its implications. J. Inst. Eng. 13, 201–205. doi: 10.3126/jie.v13i1.20367
Carreón-Freyre, D., Cerca, M., Gutiérrez-Calderón, R., López-Quiroz, P., Alcántara-Durán, C., González-Hernández, M., et al. (2017). “Posible influencia de la subsidencia y fracturamiento en la Ciudad de México en las construcciones dañadas por el sismo del 19 de Septiembre de 2017,” in Geociencias. Available online at: http://www.geociencias.unam.mx/geociencias/desarrollo/fracturas_sismo2017.pdf
Carrera-Hernández, J. J., and Gaskin, S. J. (2007a). Spatio temporal analysis of daily precipitation and temperature in the Basin of Mexico. J. Hydrol. 336, 231–249. doi: 10.1016/j.jhydrol.2006.12.021
Carrera-Hernández, J. J., and Gaskin, S. J. (2007b). The Basin of Mexico aquifer system: regional groundwater level dynamics and database development. Hydrogeol. J. 15, 1577–1590. doi: 10.1007/s10040-007-0194-9
Carrillo, N. (1969). “Influencia de los pozos artesianos en el hundimiento de la Ciudad de México. 1948,” in El Hundimiento de la Ciudad de México. Proyecto Texcoco, ed N. Carrillo (Mexico, DF: Secretaría de Hacienda y Crédito Público, 7–14.
Conagua, C. N. (2016). Ley Federal de Derechos Aguas Nacionales 2016. Mexico City: Federal Law. Available online at: www.gob.mx/semarnat
Del Castillo, R. (1978). El Subsuelo y la Ingeniería de Cimentaciones en el Área Urbana del Valle de México. Mexico, DF: Simposio Sociedad Mexicana de Mecánica de Suelos.
DOF (2000). Norma Oficial Mexicana NOM-127-SSA1-1994, Salud Ambiental, Agua para uso y Consumo Humano-Límites Permisibles de Calidad y Tratamientos a que debe Someterse el agua para su Potabilización. Cambridge: Cambridge University Press.
DOF (2010). Norma Oficial Mexicana NOM-179-SSA-1998, Vigilancia y Evaluación del Control y Calidad del Agua para uso y Consumo Humano, Distribuida por Sistemas de Abastecimiento Público. Cambridge: Cambridge University Press. Available online at: https://www.ucol.mx/content/cms/13/file/NOM/NOM-179-SSA1-1998.pdf
Fraley, C., Raftery, A., and Scrucca, L. (2014). mclust: Normal Mixture Modeling for Model-based Clustering, Classification, and Density Estimation. R Package Version 4.
Fraley, C., and Raftery, A. E. (2002). Model-based clustering, discriminant analysis, and density estimation. J. Am. Stat. Assoc. 97, 611–631. doi: 10.1198/016214502760047131
Gibbons, R. D., Bhaumik, D. K., and Aryal, S. (2009). Statistical Methods for Groundwater Monitoring. Hoboken, NJ: John Wiley & Sons.
Gilbert, R. O. (1987). Statistical Methods for Environmental Pollution Monitoring. Hoboken, NJ: John Wiley & Sons.
Hernández-Espriú, A., Reyna-Gutiérrez, J. A., Sánchez-León, E., Cabral-Cano, E., Carrera-Hernández, J., Martínez-Santos, P., et al. (2014). The DRASTIC-Sg model: an extension to the DRASTIC approach for mapping groundwater vulnerability in aquifers subject to differential land subsidence, with application to Mexico City. Hydrogeol. J. 22, 1469–1485. doi: 10.1007/s10040-014-1130-4
Horne, J. (2015). Water information as a tool to enhance sustainable water management-The Australian experience. Water 7, 2161–2183. doi: 10.3390/w7052161
INEGI (2015). Encuesta Intercensal 2015. México: Instituto Nacional de Estadística y Geografía. Available online at: https://www.inegi.org.mx/programas/intercensal/2015/
Jiménez, C. B., Mazari-Hiriart, M., Domínguez, R., and Cifuentes, E. (2004). “El agua en el Valle de México,” in El Agua en México vista desde la Academia, eds B. Jiménez and L. Marín (Academia Mexicana de Ciencias), 15–32.
Malakootian, M., and Nouri, J. (2010). Chemical variations of ground water affected by the earthquake in bam region Malakootian, M. Int. J. Environ. Res. 4, 443–454.
Marsal, R., and Mazari, M. (1990). “Desarrollo de la mecánica de suelos en la Ciudad de México,” in En Anon. El Subsuelo la Cuenca del Val. México y su Relación con la Ing. Cimentaciones a Cinco Anos del Sismo (Ciudad de México: Soc. Mex. Mecánica Suelos), 3–25.
Marsal, R. J., and Mazari, M. (1959). El Subsuelo de la Ciudad de México. Vol. I. Congreso Panamericano de Mecánica de Suelos y Cimentaciones. Mexico, DF: Facultad de Ingenieria, UNAM.
Mazari, M., and Alberro, J. (1990). “Hundimiento de la Ciudad de México,” in Problemas de la Cuenca de México, México, eds M. Mazari and J. Kumate (Ciudad de México), 83–114. Available online at: http://biblio.upmx.mx/library/index.php?title=284156&query=@title=Special:GSMSearchPage@process=@autor=KUMATE, JESUS @mode = &recnum = 12
Mazari, M., Ramírez, C., and Alberro, J. (1992). Efectos de la extracción de agua en la zona de lacustre del valle de México: México DF, Sociedad Mexicana de Mecánica de Suelos, AC. Raul J. Marsal 1992, 37–48.
Mazari-Hiriart, M., Cruz-Bello, G., Bojórquez-Tapia, L. A., Juárez-Marusich, L., Alcantar-lópez, G., Marín, L. E., et al. (2006). Groundwater vulnerability assessment for organic compounds: fuzzy multicriteria approach for Mexico City. Environ. Manag. 37, 410–421. doi: 10.1007/s00267-005-0059-8
Mazari-Hiriart, M., Zarco-Arista, A. E., Tapia-Palacios, M. A., Hernández-Bautista, B., Solano-Ortiz, R., and Hjort-Colunga, E. R. (2018). Estudio sobre el Estado Actual de Mantos Acuíferos, la Explotación de Pozos, el Abasto real y la Demanda Actual y Potencial del Líquido en la Ciudad de México. Ciudad de México: CFE.
Mooser, F., Montiel, A., and Zuñiga, Á. (1996). Nuevo Mapa Geológico de las Cuencas de México, Toluca y Puebla: Estratigrafía, Tectónica Regional y Aspectos Geotérmicos. México, DF: CFE, Comisión Federal de Electricidad.
National Research Council (NRC) (1995). Mexico City's Water Supply: Improving the Outlook for Sustainability. Washington, DC: National Academy of Sciences
Olea, S. (2017). Los Manantiales Como Manifestaciones de los Sistemas de Flujo del Agua Subterranea. Unpublished Report. UNAM. Ciudad de México: CFE.
OMS (2006). Guías para la Calidad del agua Potable. 3rd Edn. Available online at: https://www.who.int/water_sanitation_health/dwq/gdwq3_es_fulll_lowsres.pdf
Onda, S., Sano, Y., Takahata, N., Kagoshima, T., Miyajima, T., Shibata, T., et al. (2018). Groundwater oxygen isotope anomaly before the M6. 6 Tottori earthquake in Southwest Japan. Sci. Rep. 8:4800. doi: 10.1038/s41598-018-23303-8
Ortega, A., and Farvolden, R. N. (1989). Computer analysis of regional groundwater flow and boundary conditions in the basin of Mexico. J. Hydrol. 110, 271–294. doi: 10.1016/0022-1694(89)90192-3
Plana, Q., Alferes, J., Fuks, K., Kraft, T., Maruéjouls, T., Torfs, E., et al. (2019). Towards a water quality database for raw and validated data with emphasis on structured metadata. Water Qual. Res. J. 54, 1–9. doi: 10.2166/wqrj.2018.013
Reséndiz, D., Springall, G., Rodríguez, J. M., and Esquivel, R. (1970). Nformación Reciente sobre las Características del Subsuelo y la Práctica de la Ingeniería de Cimentaciones en la Ciudad de México. Memorias de la V Reunión Nacional de Mecánica de Suelos. Mexico, DF: Sociedad de Mecánica de Suelos.
Santoyo, E., Ovando-Shelly, E., Mooser, F., and León, E. (2005). Síntesis Geotécnica de la Cuenca del Valle de México. TGC 20 Años. Mexico, DF: TGC Geotécnia S.A. de C.V.
Tóth, J. (1963). A theoretical analysis of groundwater flow in small drainage basins. J. Geophys. Res. 68, 4795–4812. doi: 10.1029/JZ068i016p04795
Uprety, S., Hong, P. Y., Sadik, N., Dangol, B., Adhikari, R., Jutla, A., et al. (2017). The effect of the 2015 earthquake on the bacterial community compositions in water in Nepal. Front. Microbiol. 8:2380. doi: 10.3389/fmicb.2017.02380
USEPA (2010). ProUCL Software Documentation. Available online at: https://www.epa.gov/land-research/proucl-software (accessed September 14, 2018).
Wood, H. (2014). The Effects of Earthquakes on Groundwater in Santa Clara County. Available online at: https://www.csus.edu/envs/Documents/Theses/Fall 2014/829.The Effects of Earthquakes on Groundwater in Santa Clara County.pdf
Keywords: water quality, groundwater, Mexico City, urban, earthquake
Citation: Mazari-Hiriart M, Tapia-Palacios MA, Zarco-Arista AE and Espinosa-García AC (2019) Challenges and Opportunities on Urban Water Quality in Mexico City. Front. Environ. Sci. 7:169. doi: 10.3389/fenvs.2019.00169
Received: 23 March 2019; Accepted: 09 October 2019;
Published: 29 October 2019.
Edited by:
Yang Liu, Emory University, United StatesReviewed by:
Gulnihal Ozbay, Delaware State University, United StatesJose Alfredo Ramos Leal, Instituto Potosino de Investigación Científica y Tecnológica (IPICYT), Mexico
Copyright © 2019 Mazari-Hiriart, Tapia-Palacios, Zarco-Arista and Espinosa-García. This is an open-access article distributed under the terms of the Creative Commons Attribution License (CC BY). The use, distribution or reproduction in other forums is permitted, provided the original author(s) and the copyright owner(s) are credited and that the original publication in this journal is cited, in accordance with accepted academic practice. No use, distribution or reproduction is permitted which does not comply with these terms.
*Correspondence: Marisa Mazari-Hiriart, bWF6YXJpQHVuYW0ubXg=