- 1Department of Land, Air and Water Resources, University of California, Davis, Davis, CA, United States
- 2Natural Resources Management and Environmental Sciences, College of Food, Agriculture and Environmental Sciences, California Polytechnic State University, San Luis Obispo, CA, United States
The use of sustainable soil management practices is becoming common in wine growing regions around the world in response to an increased awareness of the value of soil health to maintain environmental quality, crop yield, and grape quality. In spite of this, little information is available on the meaning of soil health within a viticultural context, the effects of soil management practices on soil health and the consequences for grape quality and the expression of the terroir. In this review we discuss interrelated dynamic physical, chemical, and biological properties associated with soil health and how they could be important in the expression of the terroir. We focus on the use of cover crops and compost application, two practices commonly used in vineyard soils, and how they affect these physical, chemical and biological aspects of soil health, grape quality and the expression of the terroir. Finally, we discuss research gaps, and best management practices to reduce possible tradeoffs associated with these practices such as the emission of greenhouse gasses.
Introduction
Increasing awareness that soils are a non-renewable resource and the prevailing role of soils in climate change mitigation have spurred worldwide efforts to protect and improve soil health. Recent estimates indicate that 36 billion tons of soil are lost annually due to water and wind erosion alone (Borrelli et al., 2017). Besides erosion, soils are threatened by soil organic matter (SOM) loss, soil nutrient imbalances, salinization and sodification, soil sealing and land intake, loss of soil biodiversity, contamination, acidification, compaction, and waterlogging (FAO and ITPS, 2015). The term “Soil Health” has been co-opted by governmental and non-governmental organizations, academic institutions, industry and grassroot organizations alike in an effort to raise awareness of soil conservation and protect soils from degradation (Karlen et al., 2019). This urgency to protect soils has been further strengthened by their potential to store carbon (C) that would otherwise be emitted into the atmosphere as carbon dioxide (CO2), thereby offering an appealing pathway for climate change mitigation (Lal, 2004; Paustian et al., 2016). France announced the four Permille Initiative at the Climate Summit in Paris in 2015, aimed at reducing greenhouse gas (GHG) emissions and increasing food security by increasing soil organic carbon (SOC) concentrations by four permille worldwide (Minasny et al., 2017). Similar initiatives have followed, including California’s Healthy Soils Program which was launched in 2017 (Ross, 2016). Conservation of soils and soil ecosystem services has been proposed as a key strategy to attain the United Nations Sustainable Development Goals by 2030 (Keesstra et al., 2016, 2018), and implemented in the European Union through payment for ecosystem services in rural development programs (Galati et al., 2016).
Under these initiatives, management practices that increase sequestration of SOC are promoted or incentivized. Much of the research on soil health and C sequestration have focused on cereal cropping systems in temperate climate zones (Fine et al., 2017). Given important differences between vineyards and cereal cropping systems, it is pertinent to evaluate soil health in the context of vineyard soils in particular and discuss the implications for the winemaking industry.
Vineyards in the old world were often established on marginal land, reserving the most fertile soils for cultivation of cereals, and other food crops (Martínez-Casasnovas and Ramos, 2006; Blavet et al., 2009), while extensive availability of land in the new world offered deep, alluvial, fertile soils for vineyard establishment (White, 2015). As a result, vineyards arguably occupy a broader range of soil types than any other crop. Due to microclimatic effects on winegrape quality, vineyards are often established on slopes, taking advantage of the hill’s aspect to optimize solar radiation for the crop (White, 2015). Vineyards on slopes and marginal lands especially are at elevated risk for soil degradation, causing declines in soil quality and fertility, reduced water infiltration and storage, impaired quality of ground and surface water, diminished air quality, and risks associated with climate change mitigation and adaptation. Meanwhile, vineyards have a relatively low nitrogen (N) requirement, implying a low risk for N pollution associated with N fertilization compared to other crops (Williams, 1999). Moreover, as perennial crops with a relatively deep root system, vineyard soils were suggested to have great potential to sequester C (Suddick et al., 2013). Viticulture practices often aim to optimize wine grape quality rather than yield, as winemakers seek to bring out the flavors of a specific terroir, while consumers increasingly value sustainable practices in their decision process when buying wines (Schäufele and Hamm, 2017). As such, many questions remain on how to balance soil management to optimize soil health and the expression of terroir. Therefore, the objectives of this review are to (1) explore where soil characteristics for soil health and fine wine terroir intersect; (2) synthesize the literature on how cover crops and compost application affect soil health and terroir in vineyards; and (3) identify knowledge gaps in the management of cover crops, compost application and other novel practices to improve soil health and terroir.
Where Soil Health Management and the Expression of Terroir Intersect
The concept of soil health or soil quality has been of concern to soil scientists and agronomists for decades. Various attempts have been made to define soil health and identify metrics and frameworks to quantify the health status of a soil (Karlen and Rice, 2015; Bünemann et al., 2018; Stewart et al., 2018). The United States Natural Resources Conservation Services (NRCS) defined soil health as “the continued capacity of soil to function as a vital living ecosystem that sustains plants, animals, and humans,” an adaptation of the earlier definition of soil quality by Doran (1994). A key component in this definition is the importance of soil functions; a healthy soil does not only show certain properties, these properties link to the capacity of the soil to perform functions or deliver ecosystem services that benefit humankind (Doran, 1994; Haygarth and Ritz, 2009). In this review, we adopted previously proposed concepts of soil functions (Schulte et al., 2014; Vogel et al., 2019), and envision a healthy soil to (1) support high yield and crop quality, (2) have good water infiltration and the capacity to store water, (3) retain and recycle nutrients efficiently, while supplying nutrients in correspondence with crop demand, (4) store C and reduce GHG emissions, and (5) support diverse microorganisms and high biological activity (Figure 1). The capacity of a soil to perform these soil functions can be assessed by a suite of chemical, physical, and biological soil health indicators; however, a standardized approach to assess soil health remains elusive (Fine et al., 2017; Stewart et al., 2018; Vogel et al., 2019).
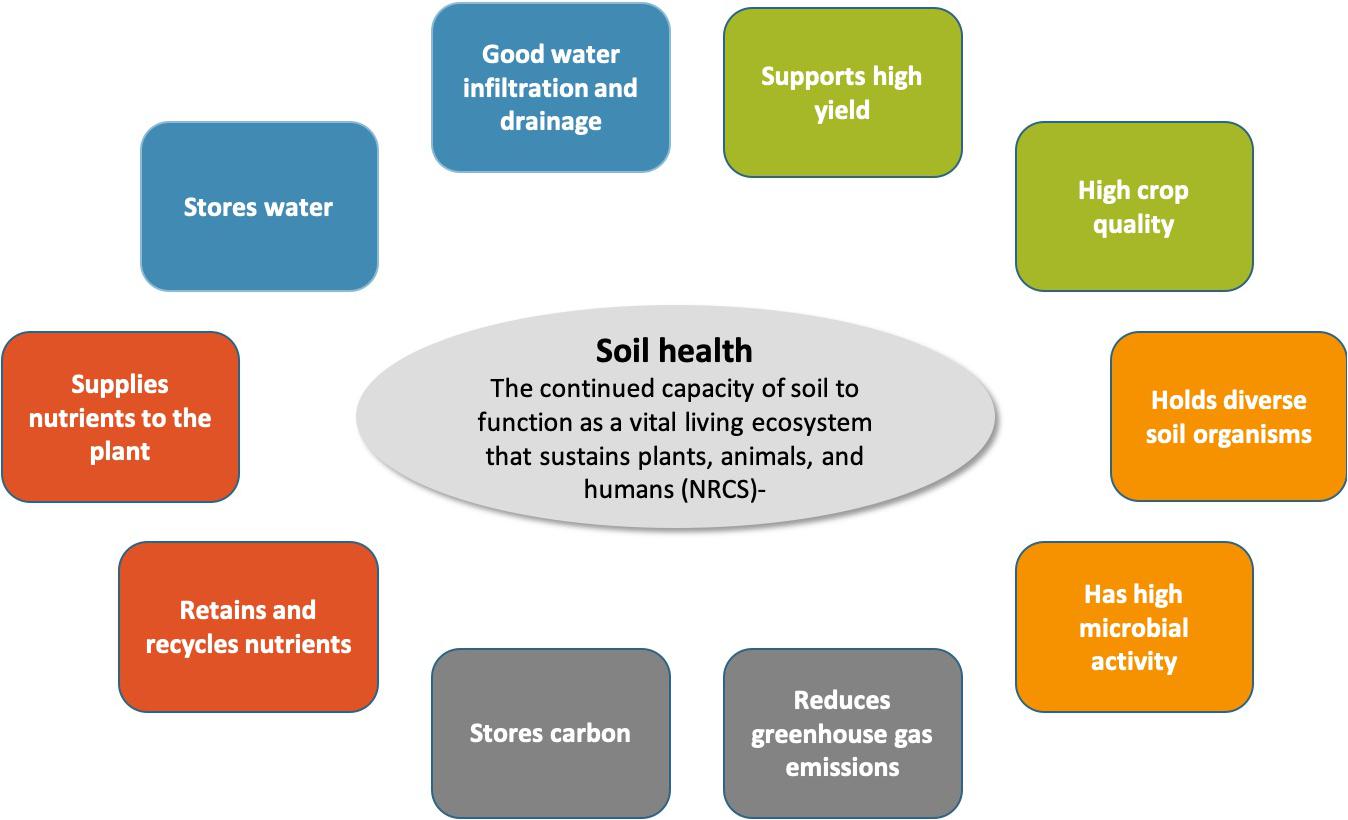
Figure 1. Definition of soil health with representation of soil functions performed by a healthy soil.
For the objective of winemaking, the most important soil function to be optimized is support of high crop quality. It is well known that soils influence the quality of winegrapes; in fact, the impact of the soil, when combined with climate, topography and grapevine variety, is referred to as the terroir effect (Van Leeuwen et al., 2018). When we consider the soil component of the terroir effect in relation to soil health, it is helpful to distinguish (i) inherent (or use-invariant) soil properties from (ii) dynamic or manageable soil properties (Wienhold et al., 2004; Karlen et al., 2019). The soil component of terroir has been linked to factors such as soil depth and minerology (White, 2015), which would be considered inherent soil properties, while other studies have found effects of soil nutrient supply, soil moisture, and soil temperature on winegrape quality (Van Leeuwen et al., 2018), which are considered dynamic soil properties. In this review, we deem that soil health is reigned by dynamic soil properties, and that a healthy soil is a soil that performs soil functions to its maximum capacity. This is in line with Fine et al. (2017), who proposed scoring schemes for soil health indicators based on soil texture groups, as implemented in Cornell’s comprehensive soil health assessment. It also resonates with Vogel et al. (2019), who considered that each soil has a limited potential to deliver a certain soil function, and that soil health assessment should reflect the status of a soil relative to its potential. As such, soil health and terroir are expected to intersect with respect to the dynamic soil components of terroir (Figure 2). Because crop quality is one of the functions performed by a healthy soil, we postulate that a healthy vineyard soil must optimally express its terroir. While the concept of terroir and its relationship with crop quality is well understood and deeply engrained in the context of wine production, the concept of soil health has recently gained acceptance by growers worldwide (Brevik et al., 2019) and there is an urgent need to reconcile these two concepts. Here, we discuss how dynamic soil properties of terroir intersect with soil health.
Soil Temperature
Van Leeuwen et al. (2018) proposed that cool soils may be an advantage in warm climates because they can slightly delay ripeness, thereby enhancing certain flavors. Soil temperature is greatly affected by soil color; light colors have a high albedo effect which keeps the soil relatively cool. Soil color can be described as an inherent soil property. However, light colors on the soil surface can be achieved by management practices such as mulching and leaving cover crop residue on the surface (Teasdale and Mohler, 1993). While soil temperature appears relevant to terroir, it has not been commonly associated with soil health assessment. Management practices that decrease soil temperature, however, are greatly promoted in regard to other soil health functions.
Soil Chemical Fertility
It can be argued that soils of the best terroirs should be characterized by a stable and well balanced nutrient supply, able to assure the target qualitative result without massive integration of fertilizers (Costantini and Bucelli, 2014). Experience shows that these soils are often characterized by only moderate chemical fertility. Correlations between the mineral composition in soils and wine has been used with mixed success to identify the geographic origin of wines (Versari et al., 2014; Pepi et al., 2017). Yet, scientific evidence linking soil chemical properties to grape and wine quality is scarce. Maltman (2013) argues that the minerals in wine are typically metallic cations at minuscule concentrations that lack flavor, and only distantly relate to vineyard geological minerals. Meanwhile, plant nutrients play an important role in plant physiology and metabolism. Mackenzie and Christy (2005) found that grape juice properties such as Baumé and titratable acidity (TA) were correlated with several plant-available trace elements in the soil, most notably Ca, Sr, Ba, Pb, and Si. Potassium content of soil has been found to have an effect on must acidity (Costantini and Bucelli, 2014), and manganese has been correlated with phenolics in grape berries (Bramley and Janik, 2005). While mechanisms underlying these correlations are lacking, the results suggest that soil cation chemistry does have an influence on wine grape composition. We increasingly understand how soil biological processes govern the availability of plant essential and beneficial nutrients and acknowledge that soil management has the potential to modify these processes. In particular, increased popularity of organic amendments such as composts in vineyards could greatly affect the availability of essential and beneficial plant nutrients. Hence, it likely behooves viticulturalists to better understand how managing soil nutrient supply can optimize the soil chemistry related expression of terroir.
Soil Nitrogen Supply and Retention
Nitrogen is one of the most limiting nutrients for plant growth, and by far the dominant nutrient applied as fertilizer to cropping systems worldwide. As ensuring high N supply is considered a type of insurance for high crop production, it is not surprising that average N use efficiencies (i.e., the amount of applied N taken up by the crop) are as low as 50% (Ladha et al., 2005). N not taken up by the plant is commonly lost from the soil-plant system through leaching or gaseous emission (Figure 3A). While an adequate supply of N is crucial for good crop productivity, the overapplication of N fertilizer over the last decades has caused large losses of N to the environment with alarming consequences for air and water resources (Rockström et al., 2009).
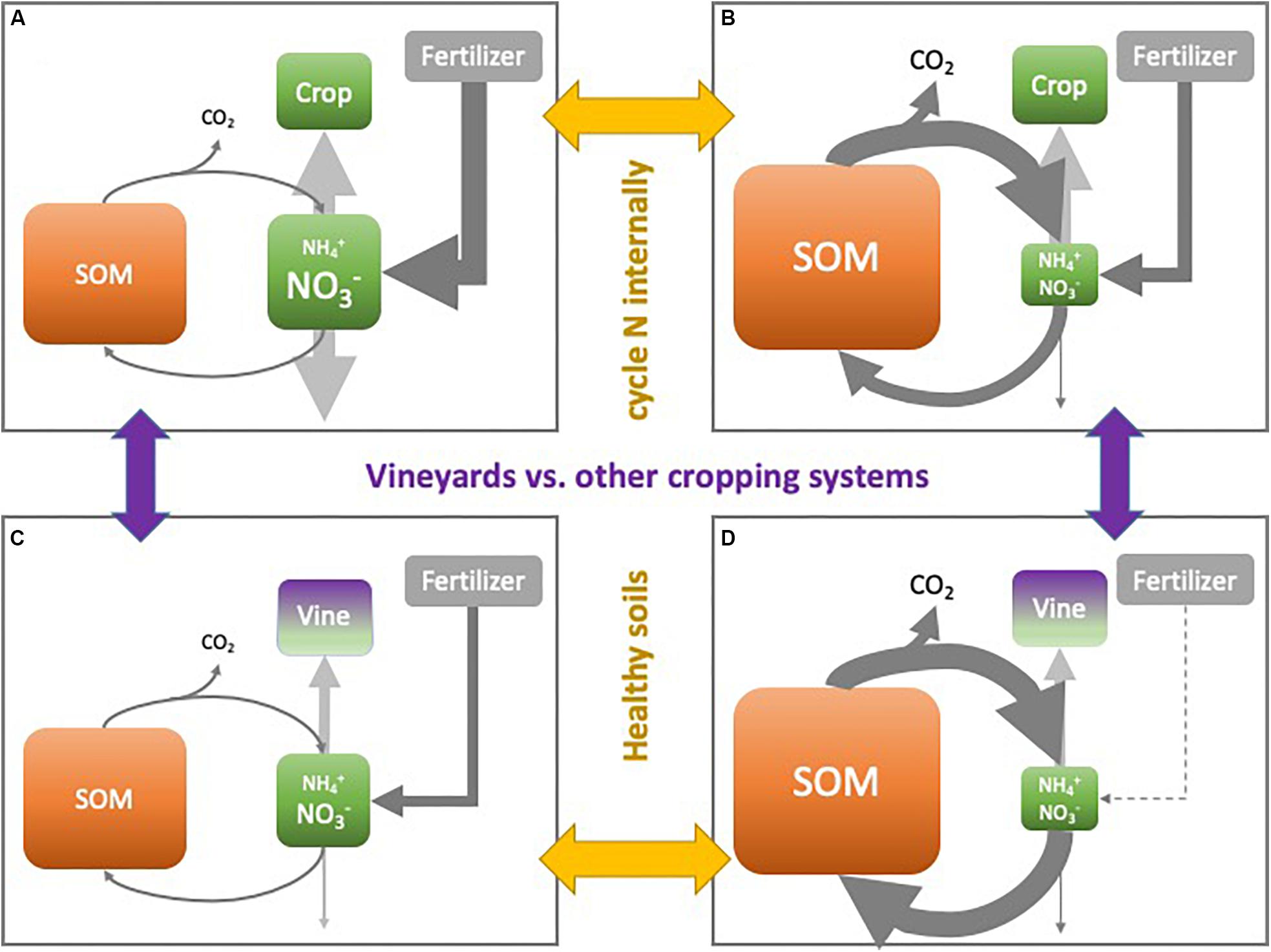
Figure 3. Illustration of the N cycle for (A) conventional agricultural systems for crops with high N demand, (B) agricultural systems for crops with high N demand under soil health management, (C) conventional vineyard system, and (D) vineyards with soil health management.
Soil NO3– concentrations are typically shown on soil test reports and have been used to monitor the availability of N for the plant. Good nutrient stewardship keeps soil NO3– concentrations high enough to meet crop N demand, and low enough to reduce the risk of N loss to the environment. However, improved understanding of the N cycle increasingly demonstrates that NO3– is not a static pool waiting to be taken up by the plant. Instead, soil NH4+ and NO3– pools can be very dynamic, reliant on rapid mineralization, nitrification and immobilization rates that continuously produce and consume new plant available N (Drinkwater and Snapp, 2007; Bowles et al., 2015). In this scenario, plants successfully compete with microorganisms for the use of newly mineralized plant available N, and soil NO3– concentrations can likely be kept much lower than previously thought, further reducing N losses to the environment (Figure 3B). Following this paradigm shift, a healthy soil supplies N when the plant needs it and retains N during periods of low plant demand by cycling it efficiently through a thriving soil food web.
In most cropping systems, the success of this new paradigm is subject to the ability of the plant to compete with microorganisms for soil available N. In wine grape production, however, the story is even more complicated. As for most crops, N plays a major role in many biological functions and processes and is therefore a highly abundant and often limiting nutrient in grapevine production. Winegrapes remove about 22–56 kg of N ha–1 through harvest (Williams, 1999), and this value serves as an indication of the minimum amount of N that should be replenished annually. In addition, a great amount of N is invested in the growth of trunk, roots, leaves and shoots. Although a fraction of this N is reabsorbed into permanent structures when plants go dormant or recycled in the soil-plant system with leaf fall and pruning, vegetative growth does imply additional N demand. Meanwhile, excessive N availability can boost vigor, negatively impacting sink-source relationships in the vine and the canopy microclimate, all of which can lead to loss of grape yield and quality (Wheeler and Pickering, 2003; White et al., 2007). Moreover, N nutrition also directly affects the form and concentration of yeast assimilable nitrogen (YAN) in the grapes, with further implications for wine quality (Bell and Henschke, 2005; Reynard et al., 2011). It is clear that to balance vine health and grape quality, vineyard N status must be managed very carefully.
Traditionally, excessive availability of N in vineyards is avoided by delivering N in small doses when vine N demand is greatest (Figure 3C). When building SOM and soil health, however, care must be taken that this does not inadvertently lead to an increase in the soil’s capacity to supply N, thereby promoting vine vigor and loss of wine grape quality. As the balance between N mineralization and immobilization is governed to a great extent by the availability of C relative to N (Booth et al., 2005), C-rich organic inputs such as straw amendments have been proposed as one strategy to manage excessive N supply in healthy soils (Wheeler and Pickering, 2003) (Figure 3D). Alternatively, non-legume cover crops have been used to remove excessive soil NO3–, by increasing competition with the vines for available N (Wheeler and Pickering, 2003; Van Leeuwen et al., 2018).
Water Availability and Drainage
In wine grape cultivation, it is important to carefully manage water availability and drainage in order to ensure vine health as well as wine grape quality. Excessive water availability can promote vigor and cause direct and indirect negative effects on wine grape quality (Wheeler and Pickering, 2003; White et al., 2007). Therefore, high wine grape quality is often expressed under mild water stress, as found on well-drained soils or as a result of deficit irrigation. If water stress becomes too severe, however, vine health, yield as well as wine grape quality are jeopardized. This is particularly relevant for vineyards in Mediterranean climates where water scarcity can become an issue, especially in the light of climate change (Medrano et al., 2015; Mirás-Avalos et al., 2017).
Whether a vineyard experiences excessive water supply or severe water stress depends on the climate, the cultivar, the rootstock, irrigation management, canopy management, soil type, as well as soil health management (Medrano et al., 2015). Various soil properties affect soil water regulation, most notoriously water holding capacity, plant available water, and hydraulic conductivity. Water holding capacity is the water the soil can hold on to against gravitational forces. The water holding capacity reflects the amount of water in the soil at a matric potential of approximately –33 kPa. Permanent wilting point is reached at a matric potential of approximately –1,500 kPa. At this point, water left in the soil is strongly held by capillary forces and unavailable to the plant. Therefore, plant available water is defined as the amount of water observed between field capacity and permanent wilting point. Finer textured soils have a greater water holding capacity compared to coarser textured soils (Figure 4). Meanwhile, the greatest plant available water is typically observed in silt loams and silty clay loams. In finer soil texture classes, plant available water decreases as the water left in the soil at the permanent wilting point increases. Even though water holding capacity and plant available water are predominately determined by soil texture class, building soil health by increasing SOM and improving soil structure has the capacity to increase water holding capacity and plant available water (Kern, 1995; Olness and Archer, 2005). While water holding capacity and plant available water are important metrics for water storage, hydraulic conductivity describes the ease with which water can move through the soil. Hydraulic conductivity is greatly affected by texture and soil moisture. Hydraulic conductivity is typically greater in coarse textured soils under very wet conditions but as the soil becomes dryer it decreases sharply and, at low soil moisture conditions, hydraulic conductivity is lower than in fine textured soils (Figure 4). In addition to the inherent property soil texture, manageable soil properties including SOM content and soil structure have been shown to affect hydraulic conductivity (Vereecken, 1995).
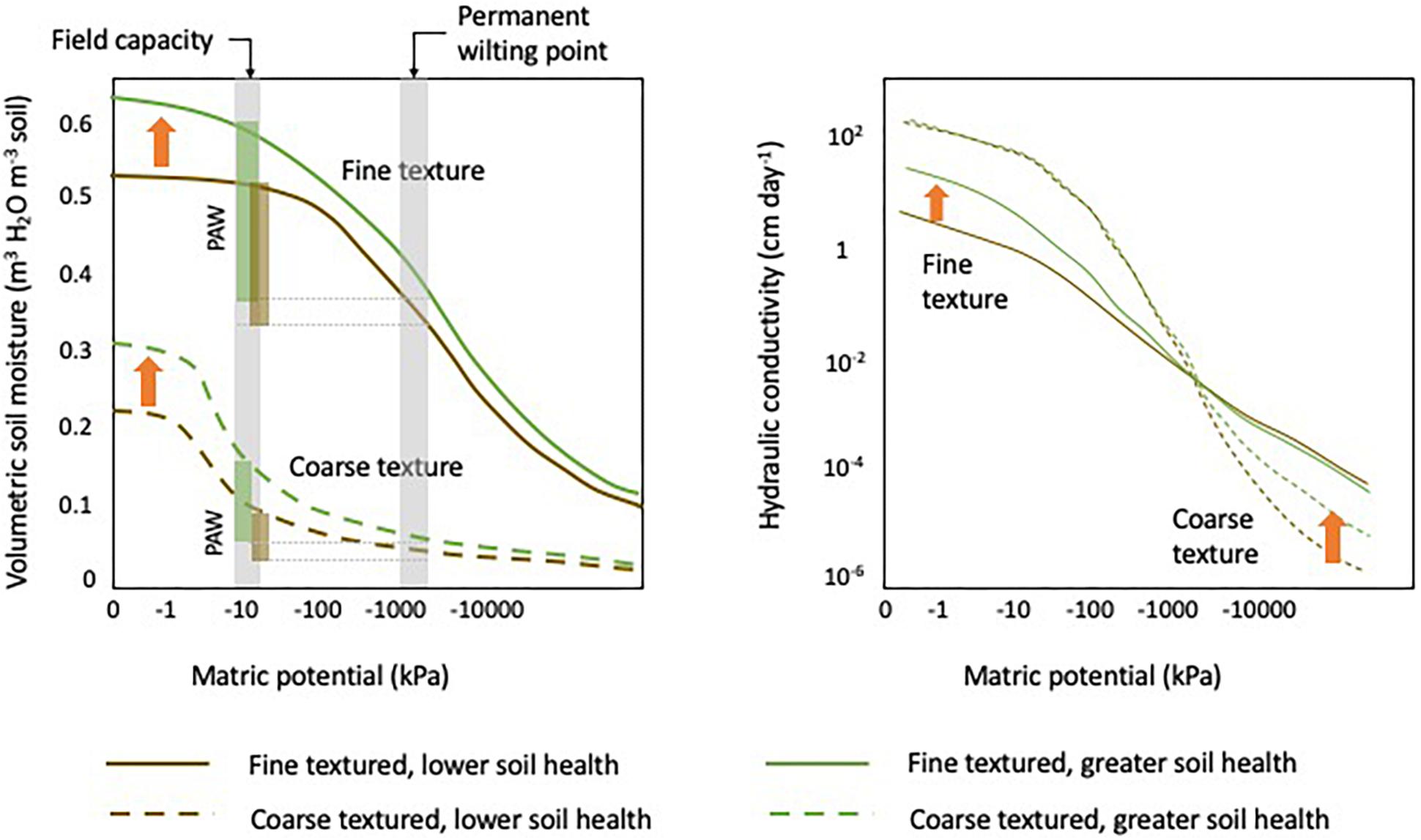
Figure 4. Left: Conceptual representation of soil moisture retention curves for coarse textured soils (dashed line) and fine textured soils (solid lines) soils with lower soil health (brown lines) compared to soils with greater soil health (green lines). Columns represent the amount of plant available water (PAW). Right: Conceptual representation of unsaturated hydraulic conductivity in function of soil matric potential for coarse textured soils (dashed line) and fine textured soils (solid lines) soils with lower soil health (brown lines) compared to soils with greater soil health (green lines). Orange arrows in both figures indicate the direction of improved soil health. Charts modified from Brady and Weil (2016).
From a soils water balance perspective, soil water supply is determined by infiltration rate, storage, drainage, runoff, and evapotranspiration (Figure 5). In a fine-textured soil, the amount of water stored in the soil may be compromised if runoff is high relative to infiltration. In contrast, water supply may be excessive in fine textured soils in wetter climates, due to the high water holding capacity of fine textured soils (Van Leeuwen et al., 2018). Soil health management can be tailored to improve infiltration and water storage in dry climates; or mitigate excessive water storage in fine textured soils by improving hydraulic conductivity and drainage or promoting evapotranspiration in wetter climates. Meanwhile, the low water holding capacity of coarse textured soils causes increased risk for severe water stress in dry climates. Here, soil health management can increase water holding capacity and help mitigate severe water stress (Medrano et al., 2015). As such, soil health management regarding water regulation can greatly impact the terroir effect.
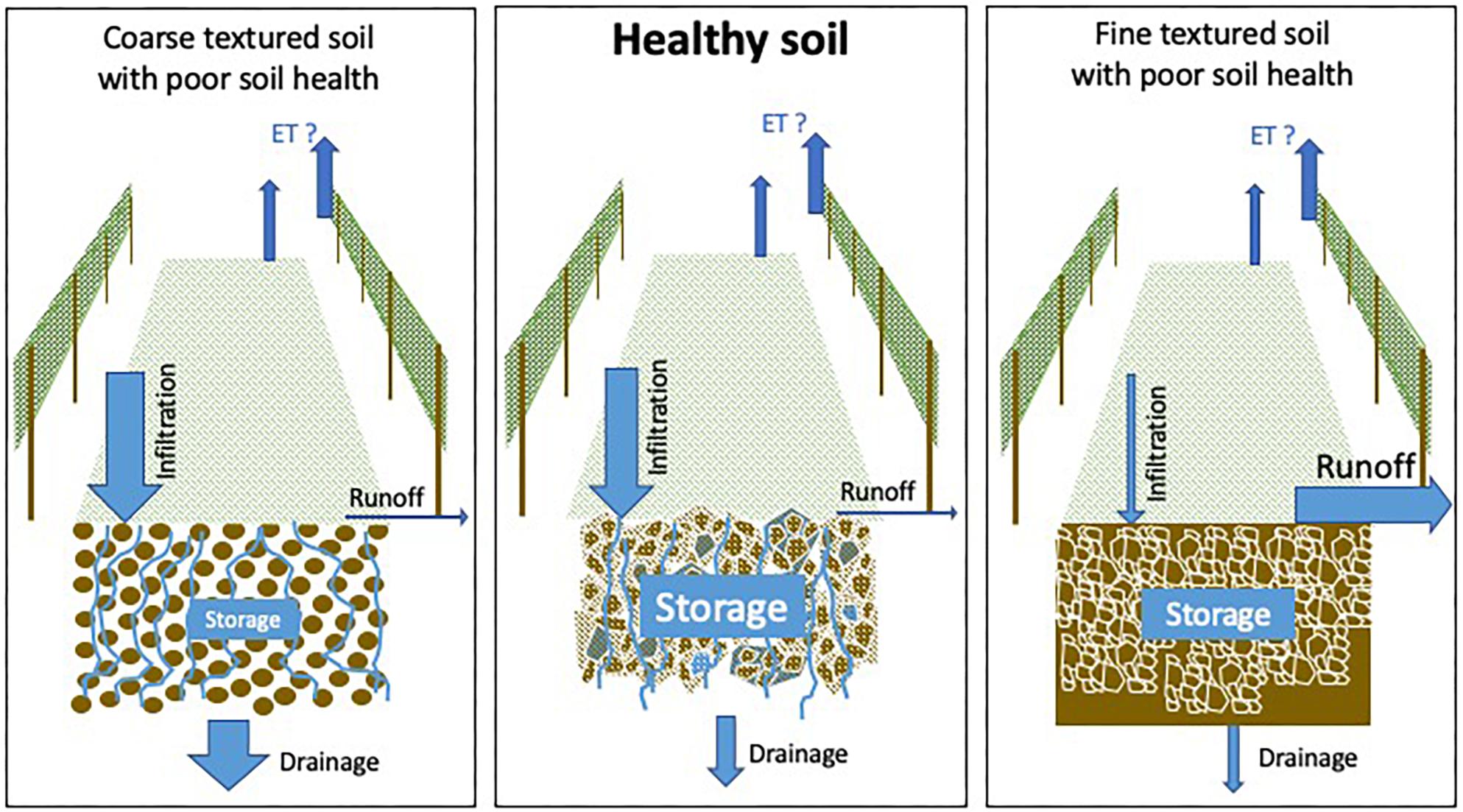
Figure 5. Vineyard water fluxes from a soil perspective in coarse textured soil with poor soil health (left), a healthy soil (middle), and a fine textured soil with poor soil health (right).
Soil Biodiversity
There is a growing interest in the role of the soil microbiome not only for grapevine health and nutrition but also for wine quality. The soil microbiome includes all microorganisms that can be found in soil, including archaea, bacteria, viruses, fungi, protists, and other microbial eukaryotes (Fierer, 2017). For most of the history of viticulture, and agriculture in general, soil microbes were mostly seen as something negative (i.e., pathogens), and efforts to improve wine quality were mostly focused on grapevine genetics and soil cultural practices, with little consideration for the soil and plant microbiome. The increasing availability, affordability and use of molecular techniques is revealing the existence of a great amount of biodiversity in the soil, the root–soil interface (the rhizosphere) and the plant itself with beneficial functions for plants (Vandenkoornhuyse et al., 2015). Soil microbial communities support key ecological processes and are directly responsible for the provision of the most important soil ecosystem services such as decomposition, mineralization of plant nutrients, atmospheric N fixation and C sequestration, all of them of high relevance for agricultural production (Bardgett and van der Putten, 2014; Fierer, 2017). Plants with adequate nutrition may be more resistant to biotic stress; in addition, soil microorganisms are known to support plant health by competing with pathogenic microorganisms for soil resources, production of antibiotic substances, or triggering of plant defenses, the so-called induced systemic resistance (Berendsen et al., 2012). But the role of the soil microbiome in wine production goes beyond supporting the typical functions of a healthy soil, wine making is essentially a microbial process in which the whole grape microbiome in early fermentation stage, including both bacteria and fungi, drives fermentation performance and the chemical characteristics of the finished wine (Bokulich et al., 2013; Belda et al., 2017). The microbial community of the winegrapes is known to share several microbial taxa with the soil, which suggests that soil may serve as a reservoir for grape microorganisms that are carried over into the fermentation stage (Zarraonaindia et al., 2015). Soil microorganisms may colonize the grapes through different pathways, such as the migration of root endophytes, dust, rain splashes, or people during harvesting and other management events (Gilbert et al., 2014). Therefore both the soil and grape microbiome are as important as the grape varietal, the soil type or the climate in regulating the organoleptic properties of wine, and show a strong biogeographical pattern that allows to differentiate between wine growing regions and even vineyards (Bokulich et al., 2016; Belda et al., 2017). This has led several scientists to propose the concept of the microbial terroir (Belda et al., 2017) opening a new and promising research field in which the soil and plant microbiome could be manipulated to support plant health, fermentation and produce optimal organoleptic wine properties. This would be achieved through management for a healthy soil microbiome as an integral part of the soil ecosystem to achieve the maximum expression of the terroir. Recent evidence shows that practices such as cover cropping results in significant changes in the soil microbiome, potentially altering the grape microbiome and wine quality (Burns et al., 2016). Yet, Chou et al. (2018) found little change in the grape microbiome with soil management practices such as herbicide application or cultivation for vegetation removal under the vine.
Soil Carbon Sequestration and Greenhouse Gas Emissions
Soil C sequestration has been promoted as a powerful strategy to mitigate climate change (Lal, 2004). In most soils, the predominant form of C is organic C. Soils serve as a sink for atmospheric CO2 when management practices that increase SOC stocks are adopted. Soil C sequestration hinges on the balance between C inputs and losses from decomposition. Soil C inputs can be increased through organic amendments or cover crops, but the net effect on SOC stocks depends on how much of the C that enters the soil is ultimately retained. Relatively stable SOC pools are typically thought to be chemically protected (binding with clay and silt minerals), physical protected (occlusion into soil aggregates), or biochemically stabilized (condensation of recalcitrant C compounds) (Six et al., 2000). Moreover, rather than a pool of inherently stable and chemically unique compounds, evidence has mounted that SOM is a continuum of progressively decomposing organic compounds processed through the soil food web (Lehmann and Kleber, 2015; Kögel-Knabner, 2017). Therefore, C sequestration requires continuous management of the turnover and volume of organic compounds and warrants further research into balancing both stocks and flows of organic matter (Lehmann and Kleber, 2015).
When building SOC stocks as a means to mitigate climate change, one must also consider tradeoffs from GHG emissions, most notably the potent GHG nitrous oxide (N2O). N2O is produced predominately by nitrifying and denitrifying microorganisms in the soil and stimulated by anthropogenic and natural disturbances such as fertilizer and organic amendment inputs, rain events, irrigation, and tillage (Butterbach-Bahl et al., 2013; Verhoeven et al., 2017). While building SOC stocks is a slow process that may take 5–10 years before a measurable management impacts are observed, responses of N2O emissions to management changes are much more instantaneous. The net effect of management on GHG emission reductions takes the balance between changes in SOC stocks and changes in N2O emissions over multiple years.
Several studies assessing the C balance of vineyards have found that vineyards have great potential for storing C (Kroodsma and Field, 2006; Suddick et al., 2013; Brunori et al., 2016; Scandellari et al., 2016). Kroodsma and Field (2006) estimated that 68 g C m–2 year–1 could be sequestered by converting annual cropping systems to vineyard agroecosystems in California. Nevertheless, the potential of vineyards to sequester C depends on soil physical characteristics, the grapevine’s biological properties, as well as management practices in the vineyard (Brunori et al., 2016; Vicente-Vicente et al., 2016). Most measurements of N2O emissions in vineyards have taken place in Mediterranean climates. Under these environmental conditions, N2O emissions in vineyards are relatively small compared to potential to sequester SOC (Garland et al., 2011; Longbottom and Petrie, 2015). Nevertheless, N2O emissions from vineyards can be further reduced by modifying the timing of N fertilizer application, offsetting soil and cover-cropping activities in the tractor row in relation to forecasted precipitation and minimizing floor management activity in the fall is also recommended (Longbottom and Petrie, 2015; Verhoeven et al., 2017).
What does this all mean for terroir? Soil C sequestration and GHG emissions will unlikely have a direct effect on terroir. However, it is well-known that SOC is essential for maintaining a good soil structure, retaining nutrients, and supporting an active soil food web, all of which have been linked to terroir. Moreover, market research shows that wine consumers increasingly value sustainability in wine production, especially among higher spenders and wine experts (Pomarici, 2016; Schäufele and Hamm, 2017). In a recent review of articles published between 2000 and March 2016 (Schäufele and Hamm, 2017) found that a considerable number of consumers across different countries reported a willingness to pay a premium for wine with characteristics of sustainable production and that sustainability cues were often perceived as quality indicators.
As a next step, efforts should be focused on establishing the spatial variability and mapping of soil C and soil biodiversity as components of soil and the terroir (Vaudour et al., 2015). Furthermore, it is important to understand how soil management commonly used to support soil health in wine grape production, will affect the expression of the terroir.
Cover Crops and Cover Crop Management for Improved Vineyard Soil Health and Expression of Terroir
Cover crops are traditionally used in the vineyard interrow to prevent soil degradation and erosion (Battany and Grismer, 2000; Ruiz-Colmenero et al., 2013; Novara et al., 2018), a practice that is being increasingly incentivized in most of the wine growing regions of the world such as California or the Mediterranean countries of Europe, where soil degradation is a pressing issue (Rodrigo-Comino, 2018; Rodrigo-Comino et al., 2018). Moreover, cover crops provide multiple other services, depending on the plant species used, such as acting as catch crops, fodder or green manure (Ramírez-García et al., 2015). Additionally, several recent meta-analyses show that cover crops support ecosystem services such as above- and below-ground biodiversity, pest control, C sequestration and soil fertility (Vicente-Vicente et al., 2016; Bowles et al., 2017; Winter et al., 2018; Shackelford et al., 2019). Many of the ecosystem services provided by cover crops are mediated through effects on soil physical, chemical and biological properties that support soil health and that may affect the expression of the terroir.
Even though the use of cover crops to manage soil fertility dates back to the Roman empire, this practice was mostly abandoned after the green revolution and widespread availability of synthetic fertilizers (Dunn et al., 2016). Today, in spite of the increasing evidence of the benefits for soil health, adoption rates are still very low due to the contrasting perceptions of producers on the actual benefits provided by cover crops, concerns over water and nutrient usage (especially in arid regions), and lack of adequate management strategies (Dunn et al., 2016; Schütte and Bergmann, 2019). Furthermore, cover crops can act as vectors or increase susceptibility of vines to plant diseases (Forte et al., 2010; Muscas et al., 2017). These issues limit the adoption of this practice and drive the active removal of vegetation in the vineyard interrow through the use of tillage and/or herbicides with negative consequences for soil health. As consumers demand sustainable products and are willing to pay the premium of sustainably grown wine, air and water quality regulations are imposed, growers are forced to consider the use of cover crops. Thus, there is a strong need for a careful synthesis of the potential benefits of this practice and how management decisions can reduce potential drawbacks.
Cover crops protect the soil from the eroding action of raindrops preventing soil erosion and runoff (Battany and Grismer, 2000), but they also improve infiltration rates (Gulick et al., 1994; Biddoccu et al., 2017) which is particularly important for vineyards established on steep slopes with higher erosion rates (Ruiz-Colmenero et al., 2013; Rodrigo-Comino, 2018). Higher infiltration, lower runoff and erosion rates in cover cropped vineyards lead to lower nitrate runoff and therefore the increase in nutrient retention (García-Díaz et al., 2017). Higher infiltration has been attributed to the increase in SOM, improvement of structure, including aggregate stability and pore connectivity (Aljibury and Christensen, 1972; Ruiz-Colmenero et al., 2013). Cover crop termination by mowing increases infiltration rates by 45% as compared to tillage (Ruiz-Colmenero et al., 2013). It is also well known that vineyards under cover crops and with a better soil structure store more water than soils without permanent vegetation cover or tilled (García-Díaz et al., 2017; López-Vicente and Álvarez, 2018).
Improvements in soil structure, pore connectivity and water holding capacity are interrelated soil properties which are directly affected by the growth of plant roots and input of organic matter not only through aboveground biomass incorporation but mostly through root turnover and root exudates released from living roots (Sokol et al., 2019). More than half of the plant biomass is belowground, which constitutes a large input of C directly into the soil; the release of root exudates constitutes a constant drip of C that feeds the rhizosphere microbial community leading to the formation of stable C-mineral associations, which contribute to further aggregate formation (Sokol and Bradford, 2019). In Mediterranean climates cover crop growth occurs during the wet season (fall and winter) and afterward, during the dry season, annual cover crops die down whereas perennial plants go into dormancy. Even though this stops root exudation, the role of roots in maintaining root structure during the dry season is still highly relevant, as dead roots hold together and preserve microbially created macroaggregates (Blankinship et al., 2016). As a result, total soil organic C and aggregate stability increases in vineyard soils with cover crops as compared to bare soil (Guzmán et al., 2019); these increases are more pronounced in the upper centimeters of soil (Wolff et al., 2018) and seem to depend on cover crop management; for instance, certain cover crop mixes result in higher C sequestration rates and increases in soil C and improvements in soil structure are higher if cover crop is not tilled (Winter et al., 2018; Novara et al., 2019).
Above and belowground C inputs by cover crops influence the structure of the soil microbial community, increasing microbial biomass and changing the species composition. Burns et al. (2016) observed that cover crop presence and species drove soil microbial community composition and consistently resulted in distinctive bacterial and archaeal soil communities throughout 19 vineyards in Napa Valley, California. As mentioned earlier, the soil microbiome has important implications, not only for nutrient cycling and soil health in general, but also for the health, yield and quality of the grapevine, and it is currently regarded as an important component of the terroir (Belda et al., 2017).
In addition to changes in the structure of the soil microbial community, cover crop C inputs increase microbial activity, soil respiration and CO2 efflux as compared to non-cover cropped and tilled vineyard soils (Steenwerth and Belina, 2008b). N inputs with leguminous cover crops could increase the amount of available N in the soil and therefore nitrification and denitrification rates that lead to the production and release of N2O, a potent GHG (Garland et al., 2011). Increase in CO2 and N2O emissions to the atmosphere could offset the potential environmental benefits of cover crops. Nevertheless, the amount of total C sequestered in plant biomass and the increase in soil C usually exceeds the increased CO2 emissions under cover crops (Wolff et al., 2018). Emissions of N2O from vineyard soils are usually small but can be increased by soil management practices that affect soil nutrient content, labile C and moisture (Verhoeven et al., 2019). Reductions in N2O emissions from cover cropped soils could be potentially achieved through the selection of the cover crop mix to reduce the presence of legumes or the choice of cover crop termination method (tilling, mowing, or grazing), however, no clear differences have been observed yet in this respect (Garland et al., 2011; Wolff et al., 2018).
Changes in soil microbiome, physical and chemical properties could have significant impacts to vine vegetative growth, yields, grape and must quality, by changing water and nutrient availability. Cover crops can reduce N uptake during grapevine vegetative growth and reduce vigor and N nutritional status, increasing the anthocyanin and polyphenols contents in the grapes and therefore having a positive impact in must quality (Pérez-Álvarez et al., 2013).
Nevertheless, cover crops can also be large sources of plant-available N if the mix contains legumes (Novara et al., 2019). In addition to improving soil nutrient status, potential nitrification, N mineralization and denitrification can be up to 2–4-fold greater in cover crop soils than in bare soils under tillage showing increased nutrient turnover and availability (Steenwerth and Belina, 2008a). Higher N availability under legume cover crops results in larger vine vegetative growth and reduced polyphenol in grapes as compared to non-legume cover crops (Muscas et al., 2017).
Finally, high transpiration rates in the cover crop can reduce water availability for grapevines; while this may be convenient in humid regions to regulate growth, reduce vigor and avoid negative impacts to must quality, in Mediterranean arid climates it may induce water stress in the grapevine (Celette et al., 2008; Celette and Gary, 2013). Water stress can have beneficial effects for grapevine health and must quality when it is not excessive, as it reduces vegetative growth and increases anthocyanins and polyphenol contents in the grapes (Monteiro and Lopes, 2007).
In spite of the above, several studies have seen little differences between cover cropped and non-cover cropped grapevines, suggesting that this practice does not seem to negatively affect crop vine yield and quality overall (Sweet and Schreiner, 2010; Steenwerth et al., 2013; Pérez-Bermúdez et al., 2016; Winter et al., 2018; Wolff et al., 2018), and that negative effects can be reduced by management decisions. For instance, no tilling the cover crops can reduce grapevine N uptake as compared to tilling (Steenwerth et al., 2013). Fertilizer and irrigation management, mowing the cover crop at budbreak or reducing the sowing density can reduce competition between grapevines and cover crops in dryer and warmer growing regions (Tesic et al., 2007; Delpuech and Metay, 2018). Younger vines may also be more sensitive to water and nutrient stress in the presence of cover crops, as they don’t have a sufficiently developed root system to explore different parts of the soil profile or store nutrients (Celette et al., 2008).
Compost Use and Management for Improved Vineyard Soil Health and Expression of Terroir
One widely adopted method to improve soil health in vineyards is through the application of composts or other organic input materials (Morlat and Chaussod, 2008; Brown and Cotton, 2011; Gaiotti et al., 2017). Increases in SOC and SOM from compost addition underlie most of the biological, chemical, and physical impacts to vineyard soils we will review in the following sections. SOC or SOM are universally increased from compost additions in vineyard soils (Pinamonti, 1998; Korboulewsky et al., 2002; Ramos and López-Acevedo, 2004; Morlat and Chaussod, 2008; Brown and Cotton, 2011; Bustamante et al., 2011; Peregrina et al., 2012; Rubio et al., 2013; Calleja-Cervantes et al., 2015a,b; Gaiotti et al., 2017; Mondini et al., 2018). Higher rates of compost addition typically result in greater treatment effects between compost treated soils and controls or grower standard NPK fertilizer practices (Korboulewsky et al., 2002; Morlat and Chaussod, 2008; Peregrina et al., 2012; Mondini et al., 2018). Both short term applications (Larchevêque et al., 2006; Rubio et al., 2013) and long-term applications (Morlat and Chaussod, 2008; Calleja-Cervantes et al., 2015a,b) result in increases in SOC/SOM in compost treated plots. The amount of SOM increases linearly with the amount of C applied in composts (Mondini et al., 2018). The longer the continued application, the stronger the treatment effect with time (Morlat and Chaussod, 2008). Soil C is the basis of the soil food web and increases in SOC from compost addition typically increases the size of the microbial biomass (Bustamante et al., 2011; Rubio et al., 2013; Wilson et al., 2016), although not always (Gaiotti et al., 2017). Increases in SOM lead to increases in total N, absolute amounts of organic N as well as inorganic N from mineralization (Larchevêque et al., 2006; Morlat and Chaussod, 2008; Calleja-Cervantes et al., 2015a; Gaiotti et al., 2017). Additions of compost derived C can influence P dynamics through competitive inhibition of P sorption sites by organic acid anions, as well as providing a source of mineral and organic P (Hue, 1992; Korboulewsky et al., 2002; Hunt et al., 2007; Wilson et al., 2016). Increases in SOC lead to better soil aggregation, infiltration and water holding capacity and reduced bulk density (Celik et al., 2004; Morlat and Chaussod, 2008; Brown and Cotton, 2011; Salomé et al., 2016; Ramos, 2017). Further, compost additions have been suggested as a potential C sequestration practice (Calleja-Cervantes et al., 2015a; Longbottom and Petrie, 2015), although N2O (denitrification) and CO2 (respiration) emissions may be increased in compost treated plots as compared to controls or grower standard NPK practices (Calleja-Cervantes et al., 2015a). Thus, many of the improvements to soil health and the manifestation of terroir from compost additions to be discussed here are rooted in the addition of C and increases to SOC/SOM from compost addition.
Addition of composts routinely increases soil microbial biomass carbon (MBC) and respiration as measured by CO2 evolution (Bustamante et al., 2011; Rubio et al., 2013; Wilson et al., 2016; Gaiotti et al., 2017). An increase in the size and activity of the microbial biomass can have beneficial effects to nutrient cycling, especially in degraded soils (Ros, 2003; Lazcano et al., 2013). Compost application can also change the structure of the soil microbial community; for instance, in a calcareous vineyard sheep compost enhanced soil microbial activity and shifted bacterial composition from oligotrophic to copiotrophic, as shown by differences in 16S rRNA gene sequences (Calleja-Cervantes et al., 2015a). A robust and diverse microbial community is a hallmark of healthy soils. However, changes in the soil microbial community resulting from application of composts can be different for different soil types. For example, organic inputs increased MBC in fine textured non-calcareous soils, but not in calcareous soils in France (Salomé et al., 2016). Increases in MBC are tied to the rate of C applied, but less to the source of C. Inputs from diverse composts (mushroom compost, farmyard manure, and vine pruning waste compost) had a similar response in MBC. Increases in the rate of C applied from composts applied lead to incremental increases in MBC (Morlat and Chaussod, 2008; Peregrina et al., 2012). Both single doses, and long-term applications increases MBC (Morlat and Chaussod, 2008; Rubio et al., 2013). Other investigations have reported no response or a modest non-significant responses of compost addition to MBC (Nendel and Reuter, 2007; Gaiotti et al., 2017). Differences in the magnitude of the MBC response from compost addition is tied to the antecedent soil health, particularly the physical and biological conditions, as well as the source and rate of compost additions (Gaiotti et al., 2017). More research is needed to connect compost addition rates, soil types and soil health objectives and potential benefits from increased MBC.
Compost application routinely increases soil N, and this increase is incremental with compost application rate (Korboulewsky et al., 2002; Peregrina et al., 2012). Peregrina et al. (2012) reported increases in N and SOC with increases in the rate of fresh and composted mushroom substrate applied. Application of various sources of organic materials, including composted sewage sludges, mushroom composts, composted cattle manure, winery wastes and pruning wastes routinely increase soil total N (Larchevêque et al., 2006; Morlat and Chaussod, 2008; Calleja-Cervantes et al., 2015a; Gaiotti et al., 2017). Mugnai et al. (2012) reported an increase in total N over a positive control (NPK) following 9 years of compost grape waste application, with NH4–N favored over NO3–N in compost treated plots, with the opposite true in the mineral nutrient positive control (grower standard NPK practice). Long term and continuous applications of cattle manure in excess of crop demand, may result in N build up, N leaching and potential suppressive effects on yield and quality (Morlat and Chaussod, 2008; Morlat and Symoneaux, 2008). While application of composts and other organic materials generally increases soil total N, differences exist in the amounts of organic N versus inorganic N, as well as the timing of release soluble N from mineralization of added organic inputs.
Initial compost applications result in a quick pulse of inorganic N followed by a prolonged period of elevated organic N. Synchronizing mineralization of compost N and the timing of high N demand in winegrapes is an area of ongoing investigation. In a 3-year study applying a range of compost types to a calcareous soil, Bustamante et al. (2011) reported that diverse sources of compost result in an initial spike in soluble N that is tempered with time, while organic N tends to remain elevated. They concluded that excess N was not observed in the soil, suggesting that the pulse of inorganic N following mineralization was utilized by the vines. Similar results were reported by Rubio et al. (2013), after application of a variety of compost sources including pruning wastes, winery wastes (pumice), sheep manure, cattle manure and mixes of manures and pumice. Similarly, application of vermicompost and vine shoot compost led to an initial large increases in extractable N, with subsequent applications leading to progressively smaller increases in extractable N, but higher values of microbial biomass N (Mondini et al., 2018). Potentially, initial applications of compost lead to initial increases in inorganic N before the additional C increased the size of the microbial biomass, and additional N resources are either assimilated into the microbial biomass, taken up by vines or lost through denitrification (Korboulewsky et al., 2002) applied three rates of sewage sludge to a French vineyard and reported an initial spike in inorganic N (NO3 and NH4), which subsided with increased application. Broadly, amounts of N remain elevated in compost treated plots compared to controls, and inorganic and organic N increased incrementally with compost application rate.
In winegrapes N uptake has been observed in two major periods, one from budbreak to veraison, and the second post-harvest, with as much as 34% of the total seasonal N uptake in this period (Conradie, 2017). N pulses following compost application are likely to be utilized by the vine, especially if N deficiencies exits. Winegrapes benefit from an extensive established root system such that the soluble N pulse following application can be utilized by vines at budbreak into flowering, whereas spring N pulses may be lost in shallow rooted row crop systems. This is particularly true if a restorative effect is desired from application of compost to established vines, where roots are eager for fresh nutrient inputs. For example, in an investigation of compost application rates to a degraded vineyard soil with established, underperforming vines, compost application in the dormant season resulted in a significant increase petiole N in the following growing season, with this increase incremental with application rate (significant dose response). Application of composts to established vines will result in uptake of the initial pulse of soluble N. Conversely large applications of high N composts (composted manures) at preplant may result in losses of N to the environment.
Soil phosphorus (P) is typically increased as the result of compost application (Korboulewsky et al., 2002; Morlat and Chaussod, 2008; Bustamante et al., 2011; Wilson et al., 2016), due to many factors including the additional P applied in the compost, the chelation of active Al or Ca by organic acids and other decomposition products, and competitive inhibition of P-sorption sites by organic acid anions (Hue, 1992; Delgado et al., 2002). Winegrapes have relatively low P demands (Schreiner and Osborne, 2018). The combined effect of relatively low P demand, and increased P availability due to P sorption inhibition, can lead to excess P following compost application (Korboulewsky et al., 2002; Wilson et al., 2016). Care should be taken, especially when composts or other organic materials are applied to meet N demands, that excess P is not applied (Korboulewsky et al., 2002). Nonetheless, P deficiency in winegrapes has been reported in certain soil types with less than 10 mg P per kg soil (Olsen-P) (Skinner et al., 1988). P deficiency can lead to reduced fruit set, yields, and vegetative growth (Skinner et al., 1988). Yield reduction from P deficiency is likely due to deleterious effects on the initiation and differentiation of bud primordia. P deficiency is manifest in blotchy red interveins, which can be mistaken for leafroll disease or red blotch disease. Soil P dynamics are directly tied to the soil component of terroir, with certain soil types, particularly red, clay rich soils from high Fe/Al parent materials (basalts and andesites), and volcanic ash soils, exhibiting P deficiency and higher P sorption capacities. In these instances, compost application can be a favorable management strategy to relieve P deficiency, due to the combined beneficial effect of applied SOM and applied P. Management of P deficiency in winegrapes is largely driven by the terroir of the soil, as influenced by pedogensis. For example, weathered soils derived from low P lithologies, such as granite, may be P deficient, but also have low P fixation capacities, and hence would respond well to P applications (Wilson et al., 2016, 2017). Conversely, P deficient soils with high P sorption capacities, such as volcanic ash soils, will require higher applications of compost to overcome the P fixation capacity. In most instances applying composts to meet P demand will result in lower P sorption capacities, and higher P availability, than mineral fertilizers alone due to competitive inhibition of anion sorption sites (Hue, 1992; Hunt et al., 2007; Wilson et al., 2016). The management of P, both with and without composts, is dependent on the terroir of the site, as manifested by soil genesis, particularly soil mineralogy and acidity/alkalinity.
In winegrapes, reduced K supply can lead to premature leaf drop, and negative effects on yield and vegetative growth (Christensen and Peacock, 2000). Potassium is the major cation in grape juice and musts and has a significant effect on juice pH (Mpelasoka et al., 2003). Excess K has been connected to undesirably high wine pH, reduced wine stability and declines in color quality (Mpelasoka et al., 2003). Excessive K in berries may lead to lower levels of the more desirable tartaric acid, altering the perception of flavor (Mpelasoka et al., 2003). In a wide range of studies with many different sources, compost application usually increases soil extractable K. Many different types of composts, cattle manures, winery wastes, sheep manures, municipal solid wastes, garden green waste mulches, mushroom composts, and mixes of these materials result in elevated K status in soils (Pinamonti, 1998; Larchevêque et al., 2006; Morlat and Chaussod, 2008; Bustamante et al., 2011; Chan and Fahey, 2011; Calleja-Cervantes et al., 2015a,b). This is particularly true for composts with a significant proportion of winery wastes which are higher in soluble K, and composts derived from manures (Bustamante et al., 2008; Rubio et al., 2013). Compost application leads to incremental increases in soil K, with very high levels of soil K corresponding to higher application rates (Morlat and Chaussod, 2008; Chan and Fahey, 2011).
When available, vines tend to uptake excess K, such that when soil K was increased from compost addition, plant tissue K also increases (Pinamonti, 1998; Morlat and Chaussod, 2008; Chan and Fahey, 2011). Given that berries are strong sinks for K, excess K supplied to vines results in larger amounts of K in berries (Pinamonti, 1998; Mpelasoka et al., 2003; Morlat and Symoneaux, 2008; Chan and Fahey, 2011). The strongest effect of compost applications to vineyard soils on wine quality may be from K supply, with increased K observed in grapes and musts as the result of compost addition (Pinamonti, 1998; Morlat and Symoneaux, 2008). With respect to terroir, Chan and Fahey (2011) noted that the treatment effect of composts on berry K was much less than the site effect on berry K. This suggest that, especially with respect to K, the inherent terroir, related to soil mineralogy and parent material, may be a greater factor in soil, vine and berry K status than the K applied in composts. However, further research is required to understand the variability in soil and vine K status attributable to variability in site characteristics and terroir. While several studies report increased berry K from increased extractable soil K following compost addition (Rubio et al., 2013) observed significant increases in soil K, but not in berry K. Conversely, lower rates of vermicompost and compost additions did not result in increased soil K status nor vine K status (Martinez et al., 2018). Mpelasoka et al. (2003) notes that the relationship between K supply in soils and K in berries and tissues is not always absolute. Differences in application rate, source, timing, irrigation, as well as vine parameters such as root architecture and the initial plant nutrient status will influence the uptake and availability of K. Given the significant variability in K status of soils due to differences in their terroir and pedogenic environment (mineralogy and parent material) as well as the effect of composts on soil K status, significant knowledge gaps exits connecting antecedent soil K, K supplied in composts, and the connection between soil K, berry K, must K, and wine quality.
In vineyard soils, increases in organic C from compost addition improve many soil physical properties. For example, in Mediterranean-type soils, compost additions increase aggregate stability (Celik et al., 2004; Kong et al., 2005; Goulet et al., 2006). The organic C fraction of soils is lighter than the mineral fraction and compost additions to vineyard soils typically reduce the soil bulk density (Celik et al., 2004; Morlat and Chaussod, 2008; Brown and Cotton, 2011; Salomé et al., 2016; Ramos, 2017). Both reductions in bulk density and improved aggregation lead to improved soil porosity, with improved porosity reported following compost addition (Pérès et al., 1998; Pinamonti, 1998). Soil temperature fluctuations are also reduced from application of compost mulches, especially compared to plastic row covers (Pinamonti, 1998). Improvements to soil structure and aggregation as well as improvements to porosity, lead to improvements in soil water holding capacity. Improved water holding capacity following compost addition is widely reported (Pinamonti, 1998; Morlat and Chaussod, 2008; Brown and Cotton, 2011; Ramos, 2017; Mondini et al., 2018). Of all soil properties, soil water has the most significant effect on vine growth, vigor, berry formation and subsequent effects on wine quality (Keller, 2005, 2010). Excess water, which leads to excess shoot growth, has been linked to declines in wine quality (Hepner et al., 1985; Wheeler and Pickering, 2003). However, concerns over climate change, drought and water use efficiency suggest that improvements to soil water holding capacity following compost addition are likely to be beneficial dependent on soil type and edaphic conditions. For example, Ramos (2017) reported significant improvements to soil water holding capacity and infiltration rate following compost addition to degraded vineyard soils, with the positive effects of compost addition on per vine yield stronger in drier years. These data suggest that, in degraded soils low in organic matter, increasing SOC from composts addition may provide some resilience to drought conditions. Similarly, in degraded vineyard soils application of vine shoot composts, but not vermicompost, lead to improved soil water holding capacity (Mondini et al., 2018). Dependent on the rate and amount of compost, compost application can be expected to improve soil water holding capacity, especially with repeated applications of high C sources. Increases in soil water holding capacity following compost addition can have significant effects on vine performance. Care should be taken by viticulturalists to mediate irrigation practices following sustained application of composts. Nonetheless, compost addition to vineyard soils is promising to mitigate soil drought and improve water use efficiency. Changes and improvements to vine water use efficiency following application of diverse sources of compost requires further investigation.
Improvements to vineyard soil health are generally consistent following compost application, improving soil biological, chemical, and physical characteristics. However, there is a view among viticulturalists that compost applications may have a negative effect on quality due to excess vigor and an imbalance between shoot growth and yield, and that the clear benefits to vineyard soil health from compost application may be outweighed by the negative effects on vine balance and grape quality. Yet, this is not borne out of the available data. For example, several studies have shown no significant changes to vine balance or juice quality following compost application (Pinamonti, 1998; Bartoli and Dousset, 2011; Mugnai et al., 2012; Mondini et al., 2018). Mugnai et al. (2012) applied municipal green waste compost to a chardonnay vineyard in the Tuscany region of Italy and conclude that, using measures of both leaf area and leaf chlorophyll, excess vigor, yields, and grape quality were not significantly affected by compost addition. Similarly, Pinamonti (1998) report no negative effects to vine balance (yields/pruning weights), total soluble solids (TSS) or pH following compost addition. Where improved water status is implicated in yield or shoot growth increases in some studies (Pinamonti, 1998; Ramos, 2017), soil N status drives increased yields and shoot growth in others. (Gaiotti et al., 2017) applied composted cattle manure and composted vine pruning wastes over a 5-year period and reported significant increases in vine growth and yield, without significant changes to vine balance (per vine yield/pruning weights). They note increased vigor in treatments containing composted cattle manure, a result they ascribe to faster mineralization rates, and more available N. In a similar, but single season study Rubio et al. (2013) applied diverse composts (citrus waste, winery waste, composted cattle manure, vine pruning wastes, and combinations of materials) and reported increases to yields, especially for mixes containing composted cattle manure, a response they also attribute to the elevated soil N status. With respect to juice chemistry, TSS was unaffected and the total polyphenol index was influenced by compost treatment, but trends were unclear. While increased N status led to increased yield in some studies, excess N was implicated in yield decline in others (Morlat, 2008). There, sustained applications of high rates (20 t ha–1 for 28 years) of relatively high N composted manures had a negative effect on yields and pruning weights, potentially due to N toxicity. Conversely, lower application rates of vine pruning compost (with higher C:N ratios) had a positive effect on yields and pruning weights. Nonetheless, no changes were reported to vine balance after 28 years of continued compost application from any source trialed. With respect to juice chemistry, Morlat (2008) report that total anthocyanins and TSS were decreased, while pH and K+ were increased in berries following addition of high rates of composted manures. In contrast, in a 3-year investigation of irrigation strategies with and without compost addition, (Cirigliano et al., 2017) found improved anthocyanin and other polyphenol contents in berries following compost addition compared to treatments without compost. Their data suggest that in drip irrigated Mediterranean-type climates, compost application can improve grape quality over irrigation alone, perhaps due to better infiltration and water holding capacity in compost treated vines. Contrasting results on the impact of composts on vine growth and yield, as well as on grape quality, especially polyphenol compositions, requires further investigation.
Compost applications can lead to improved yields and pruning weights, without detrimental effects to grape quality (pH, TSS, and TA), although effects to anthocyanins and other polyphenols are unclear. Increases in yields and pruning weights are tied to increases in soil water status and soil N status. Evaluating the existing soil conditions, as well as available water for irrigation and yield and quality objectives, ultimately informs the compost approach adopted. For example, in established normally performing vineyards, success has been accomplished with lower N inputs, such as vine pruning waste composts (Morlat, 2008; Morlat and Chaussod, 2008; Gaiotti et al., 2017). If a significant yield response is desired, or if soils are degraded, composts richer in plant available nutrients can be applied with success (Ramos, 2017; Mondini et al., 2018). The beneficial effect of compost application on yields in drought years noted by Mondini et al. (2018) and Ramos (2017) highlights a potentially significant benefit from compost addition in the face of water shortages and requires further investigation. Broadly, more research is needed to quantify yield, pruning weight, and grape quality responses from application of diverse composts in a variety of soil types, to solidify our understanding of the effect of composts in viticulture.
Conclusion
As we increase our understanding of the fundamental role of soils and soil health in long term climate regulation, crop yields and quality, there is an urgent need to understand how the dynamic and inherent aspects of soil health may overlap with the concept of terroir in wine grape production. In this review we show that, because of their impact on vine health and grape quality, the physical, chemical, and biological aspects of soil health overlap clearly with the soil related aspects of the terroir. Furthermore, we find potential for the expansion of the terroir concept by incorporating dynamic aspects of soil health such as SOM, soil C and soil biota which influence vine performance, potentially affecting wine quality. In spite of this, there are no crop specific guidelines or reference values that would help growers manage soil health for an optimum expression of the terroir. These guidelines need to be established by defining the meaning and main functions of a healthy soil in grapevines as compared to other crops and incorporating regional variability and site-specific needs.
Conservation of soil health needs to be prioritized rather than restoration of degraded soils. The use of cover crops and compost, supports the physical, chemical, and biological aspects of soil health, therefore contributing to the expression of the soil related aspects of the terroir. However, there are outstanding knowledge gaps that need to be addressed in order to implement grapevine specific best management practices. In particular, more information is needed on different cover crop species and mixes, their effects on vine nutrient and water uptake, the suitability of to boost different aspects of a healthy soil, and the consequences for grape and wine quality. In regard to the use of cover crops, we need a better understanding of the effects of different termination strategies (mowing, tilling, and grazing) and timing on soil health, crop yield and quality. Compost application clearly improves soil health, yet there is large variability in the observed effects potentially associated with the use of different feedstocks, placement, application rate and timing. The role of these factors needs to be understood in order to manage soil health for the maximum expression of the terroir.
Author Contributions
CL and CD developed the idea for this review manuscript. CL, SW, and CD performed the literature review, and discussed and collected the data.
Funding
This work was done within the framework of two projects supported by the California Department of Food and Agriculture (CDFA) Healthy Soils Initiative (Grant 17-0624-000-HS) and the Agricultural Research Institute (ARI) of the California State University (Grant 19-03-104), respectively.
Conflict of Interest
The authors declare that the research was conducted in the absence of any commercial or financial relationships that could be construed as a potential conflict of interest.
References
Aljibury, F. K., and Christensen, L. P. (1972). Water penetration of vineyard soils as modified by cultural practices. Am. J. Enol. Vitic. 23, 35–39.
Bardgett, R. D., and van der Putten, W. H. (2014). Belowground biodiversity and ecosystem functioning. Nature 515, 505–511. doi: 10.1038/nature13855
Bartoli, F., and Dousset, S. (2011). Impact of organic inputs on wettability characteristics and structural stability in silty vineyard topsoil. Eur. J. Soil Sci. 62, 183–194. doi: 10.1111/j.1365-2389.2010.01337.x
Battany, M. C., and Grismer, M. E. (2000). Rainfall runoff and erosion in Napa Valley vineyards: effects of slope, cover and surface roughness. Hydrol. Process. 14, 1289–1304. doi: 10.1002/(sici)1099-1085(200005)14:7<1289::aid-hyp43>3.0.co;2-r
Belda, I., Zarraonaindia, I., Perisin, M., Palacios, A., and Acedo, A. (2017). From vineyard soil to wine fermentation: microbiome approximations to explain the “terroir” Concept. Front. Microbiol. 8:821. doi: 10.3389/fmicb.2017.00821
Bell, S.-J., and Henschke, P. A. (2005). Implications of nitrogen nutrition for grapes, fermentation and wine. Aust. J. Grape Wine Res. 11, 242–295. doi: 10.1111/j.1755-0238.2005.tb00028.x
Berendsen, R. L., Pieterse, C. M. J., and Bakker, P. A. H. M. (2012). The rhizosphere microbiome and plant health. Trends Plant Sci. 17, 478–486. doi: 10.1016/j.tplants.2012.04.001
Biddoccu, M., Ferraris, S., Pitacco, A., and Cavallo, E. (2017). Temporal variability of soil management effects on soil hydrological properties, runoff and erosion at the field scale in a hillslope vineyard, North-West Italy. Soil Tillage Res. 165, 46–58. doi: 10.1016/j.still.2016.07.017
Blankinship, J. C., Fonte, S. J., Six, J., and Schimel, J. P. (2016). Plant versus microbial controls on soil aggregate stability in a seasonally dry ecosystem. Geoderma 272, 39–50. doi: 10.1016/j.geoderma.2016.03.008
Blavet, D., De Noni, G., Le Bissonnais, Y., Leonard, M., Maillo, L., Laurent, J. Y., et al. (2009). Effect of land use and management on the early stages of soil water erosion in French Mediterranean vineyards. Soil Tillage Res. 106, 124–136. doi: 10.1016/j.still.2009.04.010
Bokulich, N. A., Collins, T. S., Masarweh, C., Allen, G., Heymann, H., Ebeler, S. E., et al. (2016). Associations among wine grape microbiome, metabolome, and fermentation behavior suggest microbial contribution to regional wine characteristics. mBio 7:e00631-16. doi: 10.1128/mBio.00631-16
Bokulich, N. A., Thorngate, J. H., Richardson, P. M., and Mills, D. A. (2013). Microbial biogeography of wine grapes is conditioned by cultivar, vintage, and climate. Proc. Natl. Acad. Sci. U.S.A. 111, 139–148. doi: 10.1073/pnas.1317377110
Booth, M. S., Stark, J. M., and Rastetter, E. (2005). Controls on nitrogen cycling in terrestrial ecosystems: a synthetic analysis of literature data. Ecol. Monogr. 75, 139–157. doi: 10.1890/04-0988
Borrelli, P., Robinson, D. A., Fleischer, L. R., Lugato, E., Ballabio, C., Alewell, C., et al. (2017). An assessment of the global impact of 21st century land use change on soil erosion. Nat. Commun. 8:2013. doi: 10.1038/s41467-017-02142-7
Bowles, T. M., Hollander, A. D., Steenwerth, K., and Jackson, L. E. (2015). Tightly-coupled plant-soil nitrogen cycling: comparison of organic farms across an agricultural landscape. PLoS One 10:e0131888. doi: 10.1371/journal.pone.0131888
Bowles, T. M., Jackson, L. E., Loeher, M., and Cavagnaro, T. R. (2017). Ecological intensification and arbuscular mycorrhizas: a meta-analysis of tillage and cover crop effects. J. Appl. Ecol. 54, 1785–1793. doi: 10.1111/1365-2664.12815
Brady, N. C., and Weil, R. R. (2016). The Nature and Properties of Soils, 15th Edn. Columbus, OH: Pearson.
Bramley, R. G. V., and Janik, L. J. (2005). Precision agriculture demands a new approach to soil and plant sampling and analysis—examples from Australia. Commun. Soil Sci. Plant Anal. 36, 9–22. doi: 10.1081/CSS-200042958
Brevik, E. C., Steffan, J. J., Rodrigo-Comino, J., Neubert, D., Burgess, L. C., and Cerdà, A. (2019). Connecting the public with soil to improve human health: connect public with soil to improve human health. Eur. J. Soil Sci. 70, 898–910. doi: 10.1111/ejss.12764
Brown, S., and Cotton, M. (2011). Changes in soil properties and carbon content following compost application: results of on-farm sampling. Compost Sci. Util. 19, 87–96. doi: 10.1080/1065657X.2011.10736983
Brunori, E., Farina, R., and Biasi, R. (2016). Sustainable viticulture: the carbon-sink function of the vineyard agro-ecosystem. Agric. Ecosyst. Environ. 223, 10–21. doi: 10.1016/j.agee.2016.02.012
Bünemann, E. K., Bongiorno, G., Bai, Z., Creamer, R. E., De Deyn, G., de Goede, R., et al. (2018). Soil quality – A critical review. Soil Biol. Biochem. 120, 105–125. doi: 10.1016/j.soilbio.2018.01.030
Burns, K. N., Bokulich, N. A., Cantu, D., Greenhut, R. F., Kluepfel, D. A., O’Geen, A. T., et al. (2016). Vineyard soil bacterial diversity and composition revealed by 16S rRNA genes: differentiation by vineyard management. Soil Biol. Biochem. 103, 337–348. doi: 10.1016/j.soilbio.2016.09.007
Bustamante, M. A., Moral, R., Paredes, C., Pérez-Espinosa, A., Moreno-Caselles, J., and Pérez-Murcia, M. D. (2008). Agrochemical characterisation of the solid by-products and residues from the winery and distillery industry. Waste Manag. 28, 372–380. doi: 10.1016/j.wasman.2007.01.013
Bustamante, M. A., Said-Pullicino, D., Agulló, E., Andreu, J., Paredes, C., and Moral, R. (2011). Application of winery and distillery waste composts to a Jumilla (SE Spain) vineyard: effects on the characteristics of a calcareous sandy-loam soil. Agric. Ecosyst. Environ. 140, 80–87. doi: 10.1016/j.agee.2010.11.014
Butterbach-Bahl, K., Baggs, E. M., Dannenmann, M., Kiese, R., and Zechmeister-Boltenstern, S. (2013). Nitrous oxide emissions from soils: how well do we understand the processes and their controls? Philos. Trans. R. Soc. B Biol. Sci. 368:20130122. doi: 10.1098/rstb.2013.0122
Calleja-Cervantes, M. E., Fernández-González, A. J., Irigoyen, I., Fernández-López, M., Aparicio-Tejo, P. M., and Menéndez, S. (2015a). Thirteen years of continued application of composted organic wastes in a vineyard modify soil quality characteristics. Soil Biol. Biochem. 90, 241–254. doi: 10.1016/j.soilbio.2015.07.002
Calleja-Cervantes, M. E., Menéndez, S., Fernández-González, A. J., Irigoyen, I., Cibriáin-Sabalza, J. F., Toro, N., et al. (2015b). Changes in soil nutrient content and bacterial community after 12 years of organic amendment application to a vineyard: compost changes soil’s mineral content and microbial community. Eur. J. Soil Sci. 66, 802–812. doi: 10.1111/ejss.12261
Celette, F., and Gary, C. (2013). Dynamics of water and nitrogen stress along the grapevine cycle as affected by cover cropping. Eur. J. Agron. 45, 142–152. doi: 10.1016/j.eja.2012.10.001
Celette, F., Gaudin, R., and Gary, C. (2008). Spatial and temporal changes to the water regime of a Mediterranean vineyard due to the adoption of cover cropping. Eur. J. Agron. 29, 153–162. doi: 10.1016/j.eja.2008.04.007
Celik, I., Ortas, I., and Kilic, S. (2004). Effects of compost, mycorrhiza, manure and fertilizer on some physical properties of a Chromoxerert soil. Soil Tillage Res. 78, 59–67. doi: 10.1016/j.still.2004.02.012
Chan, K. Y., and Fahey, D. J. (2011). Effect of composted mulch application on soil and wine grape potassium status. Soil Res. 49, 455–461. doi: 10.1071/SR11084
Chou, M.-Y., Vanden Heuvel, J., Bell, T. H., Panke-Buisse, K., and Kao-Kniffin, J. (2018). Vineyard under-vine floor management alters soil microbial composition, while the fruit microbiome shows no corresponding shifts. Sci. Rep. 8:11039. doi: 10.1038/s41598-018-29346-1
Christensen, L. P., and Peacock, W. L. (2000). “Mineral nutrition and fertilization,” in Raisin Production Manual, ed. L. P. Christensen (Oakland, CA: University of California Agriculture and Natural Resources), 102–114.
Cirigliano, P., Vincenza Chiriacò, M., Nunez, A., Dal Monte, G., and Labagnara, T. (2017). Efecto combinado de la aplicación de riego y compost sobre la composición de la baya Montepulciano en un entorno volcánico de la región de Lacio (Italia central). Cienc. E Investig. Agrar. 44, 195–206. doi: 10.7764/rcia.v44i2.1691
Conradie, W. J. (2017). Seasonal uptake of nutrients by Chenin Blanc in sand culture: II. Phosphorus, potassium, calcium and magnesium. S. Afr. J. Enol. Vitic. 2, 7–13. doi: 10.21548/2-1-2403
Costantini, E. A. C., and Bucelli, P. (2014). “Soil and Terroir,” in Soil Security for Ecosystem Management, eds S. Kapur and S. Erşahin (Cham: Springer), 97–133. doi: 10.1007/978-3-319-00699-4_6
Delgado, A., Madrid, A., Kassem, S., Andreu, L., and del Campillo, M. D. C. (2002). Phosphorus fertilizer recovery from calcareous soils amended with humic and fulvic acids. Plant Soil 245, 277–286. doi: 10.1023/A:1020445710584
Delpuech, X., and Metay, A. (2018). Adapting cover crop soil coverage to soil depth to limit competition for water in a Mediterranean vineyard. Eur. J. Agron. 97, 60–69. doi: 10.1016/j.eja.2018.04.013
Drinkwater, L. E., and Snapp, S. S. (2007). Nutrients in agroecosystems: rethinking the management paradigm. Adv. Agron. 92, 163–186. doi: 10.1016/S0065-2113(04)92003-2
Dunn, M., Ulrich-Schad, J. D., Prokopy, L. S., Myers, R. L., Watts, C. R., and Scanlon, K. (2016). Perceptions and use of cover crops among early adopters: findings from a national survey. J. Soil Water Conserv. 71, 29–40. doi: 10.2489/jswc.71.1.29
Fierer, N. (2017). Embracing the unknown: disentangling the complexities of the soil microbiome. Nat. Rev. Microbiol. 15, 579–590. doi: 10.1038/nrmicro.2017.87
Fine, A. K., van Es, H. M., and Schindelbeck, R. R. (2017). Statistics, scoring functions, and regional analysis of a comprehensive soil health database. Soil Sci. Soc. Am. J. 81, 589–601. doi: 10.2136/sssaj2016.09.0286
Forte, V., Angelini, E., Maixner, M., and Borgo, M. (2010). Preliminary results on population dynamics and host plants of Hyalesthes obsoletus in North-Eastern Italy. Vitis 49, 39–42.
Gaiotti, F., Marcuzzo, P., Belfiore, N., Lovat, L., Fornasier, F., and Tomasi, D. (2017). Influence of compost addition on soil properties, root growth and vine performances of Vitis vinifera cv Cabernet sauvignon. Sci. Hortic. 225, 88–95. doi: 10.1016/j.scienta.2017.06.052
Galati, A., Crescimanno, M., Gristina, L., Keesstra, S., and Novara, A. (2016). Actual provision as an alternative criterion to improve the efficiency of payments for ecosystem services for C sequestration in semiarid vineyards. Agric. Syst. 144, 58–64. doi: 10.1016/j.agsy.2016.02.004
García-Díaz, A., Bienes, R., Sastre, B., Novara, A., Gristina, L., and Cerdà, A. (2017). Nitrogen losses in vineyards under different types of soil groundcover. A field runoff simulator approach in central Spain. Agric. Ecosyst. Environ. 236, 256–267. doi: 10.1016/j.agee.2016.12.013
Garland, G. M., Suddick, E., Burger, M., Horwath, W. R., and Six, J. (2011). Direct N2O emissions following transition from conventional till to no-till in a cover cropped Mediterranean vineyard (Vitis vinifera). Agric. Ecosyst. Environ. 144, 423–428. doi: 10.1016/j.agee.2011.11.001
Gilbert, J. A., van der Lelie, D., and Zarraonaindia, I. (2014). Microbial terroir for wine grapes. Proc. Natl. Acad. Sci. U.S.A. 111, 5–6. doi: 10.1073/pnas.1320471110
Goulet, E., Dousset, S., Chaussod, R., Bartoli, F., Doledec, A. F., and Andreux, F. (2006). Water-stable aggregates and organic matter pools in a calcareous vineyard soil under four soil-surface management systems. Soil Use Manag. 20, 318–324. doi: 10.1111/j.1475-2743.2004.tb00376.x
Gulick, S. H., Grimes, D. W., Goldhamer, D. A., and Munk, D. S. (1994). Cover-crop-enhanced water infiltration of a slowly permeable fine sandy loam. Soil Sci. Soc. Am. J. 58, 1539–1546. doi: 10.2136/sssaj1994.03615995005800050038x
Guzmán, G., Cabezas, J. M., Sánchez-Cuesta, R., Lora, Á., Bauer, T., Strauss, P., et al. (2019). A field evaluation of the impact of temporary cover crops on soil properties and vegetation communities in southern Spain vineyards. Agric. Ecosyst. Environ. 272, 135–145. doi: 10.1016/j.agee.2018.11.010
Haygarth, P. M., and Ritz, K. (2009). The future of soils and land use in the UK: soil systems for the provision of land-based ecosystem services. Land Use Policy 26, S187–S197. doi: 10.1016/j.landusepol.2009.09.016
Hepner, Y., Bravdo, B., Loinger, C., Cohen, S., and Tabacman, H. (1985). Effect of drip irrigation schedules on growth, yield, must composition and wine quality of cabernet sauvignon. Am. J. Enol. Vitic. 36, 77–85.
Hue, N. V. (1992). Correcting soil acidity of a highly weathered Ultisol with chicken manure and sewage sludge. Commun. Soil Sci. Plant Anal. 23, 241–264. doi: 10.1080/00103629209368586
Hunt, J. F., Ohno, T., He, Z., Honeycutt, C. W., and Dail, D. B. (2007). Inhibition of phosphorus sorption to goethite, gibbsite, and kaolin by fresh and decomposed organic matter. Biol. Fertil. Soils 44, 277–288. doi: 10.1007/s00374-007-0202-1
Karlen, D. L., Veum, K. S., Sudduth, K. A., Obrycki, J. F., and Nunes, M. R. (2019). Soil health assessment: past accomplishments, current activities, and future opportunities. Soil Tillage Res. 195:104365. doi: 10.1016/j.still.2019.104365
Karlen, D., and Rice, C. (2015). Soil degradation: Will humankind ever learn? Sustainability 7, 12490–12501. doi: 10.3390/su70912490
Keesstra, S. D., Bouma, J., Wallinga, J., Tittonell, P., Smith, P., Cerdà, A., et al. (2016). The significance of soils and soil science towards realization of the United Nations Sustainable Development Goals. Soil 2, 111–128. doi: 10.5194/soil-2-111-2016
Keesstra, S., Mol, G., de Leeuw, J., Okx, J., Molenaar, C., de Cleen, M., et al. (2018). Soil-related sustainable development goals: four concepts to make land degradation neutrality and restoration work. Land 7, 1–20. doi: 10.3390/land7040133
Keller, M. (2010). The Science of Grapevines: Anatomy and Physiology. Burlington, MA: Academic Press.
Kern, J. S. (1995). Evaluation of soil water retention models based on basic soil physical properties. Soil Sci. Soc. Am. J. 59, 1134–1141. doi: 10.2136/sssaj1995.03615995005900040027x
Kögel-Knabner, I. (2017). The macromolecular organic composition of plant and microbial residues as inputs to soil organic matter: fourteen years on. Soil Biol. Biochem. 105, A3–A8. doi: 10.1016/j.soilbio.2016.08.011
Kong, A. Y. Y., Six, J., Bryant, D. C., Denison, R. F., and van Kessel, C. (2005). The relationship between carbon input, aggregation, and soil organic carbon stabilization in sustainable cropping systems. Soil Sci. Soc. Am. J. 69, 1078–1085. doi: 10.2136/sssaj2004.0215
Korboulewsky, N., Dupouyet, S., and Bonin, G. (2002). Environmental risks of applying sewage sludge compost to vineyards: carbon, heavy metals, nitrogen, and phosphorus accumulation. J. Environ. Qual. 31, 1522–1527. doi: 10.2134/jeq2002.1522
Kroodsma, D. A., and Field, C. B. (2006). Carbon sequestration in california agriculture, 1980–2000. Ecol. Appl. 16, 1975–1985. doi: 10.1890/1051-0761(2006)016[1975:csica]2.0.co;2
Ladha, J. K., Pathak, H. J., Krupnik, T., Six, J., and van Kessel, C. (2005). Efficiency of fertilizer nitrogen in cereal production: retrospects and prospects. Adv. Agron. 87, 85–156. doi: 10.1016/S0065-2113(05)87003-8
Lal, R. (2004). Soil carbon sequestration to mitigate climate change. Geoderma 123, 1–22. doi: 10.1016/j.geoderma.2004.01.032
Larchevêque, M., Baldy, V., Montès, N., Fernandez, C., Bonin, G., and Ballini, C. (2006). Short-term effects of sewage-sludge compost on a degraded mediterranean soil. Soil Sci. Soc. Am. J. 70, 1178–1188. doi: 10.2136/sssaj2005.0115
Lazcano, C., Gómez-Brandón, M., Revilla, P., and Domínguez, J. (2013). Short-term effects of organic and inorganic fertilizers on soil microbial community structure and function: a field study with sweet corn. Biol. Fertil. Soils 49, 723–733. doi: 10.1007/s00374-012-0761-7
Lehmann, J., and Kleber, M. (2015). The contentious nature of soil organic matter. Nature 528, 60–68. doi: 10.1038/nature16069
Longbottom, M. L., and Petrie, P. R. (2015). Role of vineyard practices in generating and mitigating greenhouse gas emissions: greenhouse gas emissions in vineyards. Aust. J. Grape Wine Res. 21, 522–536. doi: 10.1111/ajgw.12197
López-Vicente, M., and Álvarez, S. (2018). Stability and patterns of topsoil water content in rainfed vineyards, olive groves, and cereal fields under different soil and tillage conditions. Agric. Water Manag. 201, 167–176. doi: 10.1016/j.agwat.2018.02.004
Mackenzie, D. E., and Christy, A. G. (2005). The role of soil chemistry in wine grape quality and sustainable soil management in vineyards. Water Sci. Technol. 51, 27–37. doi: 10.2166/wst.2005.0004
Maltman, A. (2013). Minerality in wine: a geological perspective. J. Wine Res. 24, 169–181. doi: 10.1080/09571264.2013.793176
Martinez, L. E., Vallone, R. C., Piccoli, P. N., and Ratto, S. E. (2018). Assessment of soil properties, plant yield and composition, after different type and applications mode of organic amendment in a vineyard of Mendoza, Argentina. Rev. Fac. Cienc. Agrar. 50, 17–32.
Martínez-Casasnovas, J. A., and Ramos, M. C. (2006). The cost of soil erosion in vineyard fields in the Penedès–Anoia Region (NE Spain). Catena 68, 194–199. doi: 10.1016/j.catena.2006.04.007
Medrano, H., Tomás, M., Martorell, S., Escalona, J.-M., Pou, A., Fuentes, S., et al. (2015). Improving water use efficiency of vineyards in semi-arid regions. A review. Agron. Sustain. Dev. 35, 499–517. doi: 10.1007/s13593-014-0280-z
Minasny, B., Malone, B. P., McBratney, A. B., Angers, D. A., Arrouays, D., Chambers, A., et al. (2017). Soil carbon 4 per mille. Geoderma 292, 59–86. doi: 10.1016/j.geoderma.2017.01.002
Mirás-Avalos, J. M., Buesa, I., Llacer, E., Jiménez-Bello, M. A., Risco, D., Castel, J. R., et al. (2017). Water versus source–sink relationships in a semiarid tempranillo vineyard: vine performance and fruit composition. Am. J. Enol. Vitic. 68, 11–22. doi: 10.5344/ajev.2016.16026
Mondini, C., Fornasier, F., Sinicco, T., Sivilotti, P., Gaiotti, F., and Mosetti, D. (2018). Organic amendment effectively recovers soil functionality in degraded vineyards. Eur. J. Agron. 101, 210–221. doi: 10.1016/j.eja.2018.10.002
Monteiro, A., and Lopes, C. M. (2007). Influence of cover crop on water use and performance of vineyard in Mediterranean Portugal. Agric. Ecosyst. Environ. 121, 336–342. doi: 10.1016/j.agee.2006.11.016
Morlat, R. (2008). Long-term additions of organic amendments in a loire valley vineyard on a calcareous sandy soil. II. Effects on root system, growth, grape yield, and foliar nutrient status of a cabernet franc vine. Am. J. Enol. Vitic. 59, 364–374.
Morlat, R., and Chaussod, R. (2008). Long-term additions of organic amendments in a loire valley vineyard. I. Effects on properties of a calcareous sandy soil. Am. J. Enol. Vitic. 59, 353–363.
Morlat, R., and Symoneaux, R. (2008). Long-term additions of organic amendments in a loire valley vineyard on a calcareous sandy soil. III. Effects on fruit composition and chemical and sensory characteristics of cabernet franc wine. Am. J. Enol. Vitic. 59, 375–386.
Mpelasoka, B. S., Schachtman, D. P., Treeby, M. T., and Thomas, M. R. (2003). A review of potassium nutrition in grapevines with special emphasis on berry accumulation. Aust. J. Grape Wine Res. 9, 154–168. doi: 10.1111/j.1755-0238.2003.tb00265.x
Mugnai, S., Masi, E., Azzarello, E., and Mancuso, S. (2012). Influence of long-term application of green waste compost on soil characteristics and growth, yield and quality of grape (Vitis vinifera L.). Compost Sci. Util. 20, 29–33. doi: 10.1080/1065657X.2012.10737019
Muscas, E., Cocco, A., Mercenaro, L., Cabras, M., Lentini, A., Porqueddu, C., et al. (2017). Effects of vineyard floor cover crops on grapevine vigor, yield, and fruit quality, and the development of the vine mealybug under a Mediterranean climate. Agric. Ecosyst. Environ. 237, 203–212. doi: 10.1016/j.agee.2016.12.035
Nendel, C., and Reuter, S. (2007). Soil biology and nitrogen dynamics of vineyard soils as affected by a mature biowaste compost application. Compost Sci. Util. 15, 70–77. doi: 10.1080/1065657X.2007.10702315
Novara, A., Cerdà, A., and Gristina, L. (2018). Sustainable vineyard floor management: an equilibrium between water consumption and soil conservation. Curr. Opin. Environ. Sci. Health 5, 33–37. doi: 10.1016/j.coesh.2018.04.005
Novara, A., Minacapilli, M., Santoro, A., Rodrigo-Comino, J., Carrubba, A., Sarno, M., et al. (2019). Real cover crops contribution to soil organic carbon sequestration in sloping vineyard. Sci. Total Environ. 652, 300–306. doi: 10.1016/j.scitotenv.2018.10.247
Olness, A., and Archer, D. (2005). Effect of organic carbon on available water in soil. Soil Sci. 170, 90–101. doi: 10.1097/00010694-200502000-00002
Paustian, K., Lehmann, J., Ogle, S., Reay, D., Robertson, G. P., and Smith, P. (2016). Climate-smart soils. Nature 532, 49–57. doi: 10.1038/nature17174
Pepi, S., Sansone, L., Chicca, M., and Vaccaro, C. (2017). Relationship among geochemical elements in soil and grapes as terroir fingerprintings in Vitis vinifera L. cv. “Glera.”. Geochemistry 77, 121–130. doi: 10.1016/j.chemer.2017.01.003
Peregrina, F., Larrieta, C., Colina, M., Mariscal-Sancho, I., Martín, I., Martínez-Vidaurre, J. M., et al. (2012). Spent mushroom substrates influence soil quality and nitrogen availability in a semiarid vineyard soil. Soil Sci. Soc. Am. J. 76, 1655–1666. doi: 10.2136/sssaj2012.0018
Pérès, G., Cluzeau, D., Curmi, P., and Hallaire, V. (1998). Earthworm activity and soil structure changes due to organic enrichments in vineyard systems. Biol. Fertil. Soils 27, 417–424. doi: 10.1007/s003740050452
Pérez-Álvarez, E. P., Pérez-Sotés, J. L., García-Escudero, E., and Peregrina, F. (2013). Cover crop short-term effects on soil no 3 - -n availability, nitrogen nutritional status, yield, and must quality in a calcareous vineyard of the AOC Rioja, Spain. Commun. Soil Sci. Plant Anal. 44, 711–721. doi: 10.1080/00103624.2013.748122
Pérez-Bermúdez, P., Olmo, M., Gil, J., García-Férriz, L., Olmo, C., Boluda, R., et al. (2016). Cover crops and pruning in Bobal and Tempranillo vineyards have little influence on grapevine nutrition. Sci. Agric. 73, 260–265. doi: 10.1590/0103-9016-2015-0027
Pinamonti, F. (1998). Compost mulch effects on soil fertility, nutritional status and performance of grapevine. Nutr. Cycl. Agroecosyst. 51, 239–248. doi: 10.1023/A:1009701323580
Pomarici, E. (2016). Recent trends in the international wine market and arising research questions. Wine Econ. Policy 5, 1–3. doi: 10.1016/j.wep.2016.06.001
Ramírez-García, J., Carrillo, J. M., Ruiz, M., Alonso-Ayuso, M., and Quemada, M. (2015). Multicriteria decision analysis applied to cover crop species and cultivars selection. Field Crops Res. 175, 106–115. doi: 10.1016/j.fcr.2015.02.008
Ramos, M. C. (2017). Effects of compost amendment on the available soil water and grape yield in vineyards planted after land levelling. Agric. Water Manag. 191, 67–76. doi: 10.1016/j.agwat.2017.05.013
Ramos, M. C., and López-Acevedo, M. (2004). Zinc levels in vineyard soils from the Alt Penedès-Anoia region (NE Spain) after compost application. Adv. Environ. Res. 8, 687–696. doi: 10.1016/S1093-0191(03)00041-8
Reynard, J.-S., Zufferey, V., Nicol, G.-C., and Murisier, F. (2011). Soil parameters impact the vine-fruit-wine continuum by altering vine nitrogen status. OENO One 45, 211–221. doi: 10.20870/oeno-one.2011.45.4.1502
Rockström, J., Steffen, W., Noone, K., Persson, Å., Chapin, F. S., Lambin, E. F., et al. (2009). A safe operating space for humanity. Nature 461, 472–475. doi: 10.1038/461472a
Rodrigo-Comino, J. (2018). Five decades of soil erosion research in “terroir”. The State-of-the-Art. Earth Sci. Rev. 179, 436–447. doi: 10.1016/j.earscirev.2018.02.014
Rodrigo-Comino, J., Keesstra, S., and Cerdà, A. (2018). Soil erosion as an environmental concern in vineyards. The case study of Celler del Roure, Eastern Spain, by Means of Rainfall Simulation Experiments. Beverages 4:31. doi: 10.3390/beverages4020031
Ros, M. (2003). Soil microbial activity after restoration of a semiarid soil by organic amendments. Soil Biol. Biochem. 35, 463–469. doi: 10.1016/S0038-0717(02)00298-5
Ross, K. (2016). Preparing for an uncertain future with climate smart agriculture. Calif. Agric. 70, 4–5. doi: 10.3733/ca.v070n01p4
Rubio, R., Pérez-Murcia, M. D., Agulló, E., Bustamante, M. A., Sánchez, C., Paredes, C., et al. (2013). Recycling of agro-food wastes into vineyards by composting: agronomic validation in field conditions. Commun. Soil Sci. Plant Anal. 44, 502–516. doi: 10.1080/00103624.2013.744152
Ruiz-Colmenero, M., Bienes, R., Eldridge, D. J., and Marques, M. J. (2013). Vegetation cover reduces erosion and enhances soil organic carbon in a vineyard in the central Spain. Catena 104, 153–160. doi: 10.1016/j.catena.2012.11.007
Salomé, C., Coll, P., Lardo, E., Metay, A., Villenave, C., Marsden, C., et al. (2016). The soil quality concept as a framework to assess management practices in vulnerable agroecosystems: a case study in Mediterranean vineyards. Ecol. Indic. 61, 456–465. doi: 10.1016/j.ecolind.2015.09.047
Scandellari, F., Caruso, G., Liguori, G., Meggio, F., Palese, A. M., Zanotelli, D., et al. (2016). A survey of carbon sequestration potential of orchards and vineyards in Italy. Eur. J. Hortic. Sci. 81, 106–114. doi: 10.17660/eJHS.2016/81.2.4
Schäufele, I., and Hamm, U. (2017). Consumers’ perceptions, preferences and willingness-to-pay for wine with sustainability characteristics: a review. J. Clean. Prod. 147, 379–394. doi: 10.1016/j.jclepro.2017.01.118
Schreiner, R. P., and Osborne, J. (2018). Defining phosphorus requirements for pinot noir grapevines. Am. J. Enol. Vitic. 69, 351–359. doi: 10.5344/ajev.2018.18016
Schulte, R. P. O., Creamer, R. E., Donnellan, T., Farrelly, N., Fealy, R., O’Donoghue, C., et al. (2014). Functional land management: a framework for managing soil-based ecosystem services for the sustainable intensification of agriculture. Environ. Sci. Policy 38, 45–58. doi: 10.1016/j.envsci.2013.10.002
Schütte, R., and Bergmann, H. (2019). The attitudes of French and Spanish winegrowers towards the use of cover crops in vineyards. J. Wine Res. 30, 107–121. doi: 10.1080/09571264.2019.1568975
Shackelford, G. E., Kelsey, R., and Dicks, L. V. (2019). Effects of cover crops on multiple ecosystem services: ten meta-analyses of data from arable farmland in California and the Mediterranean. Land Use Policy 88, 1–10. doi: 10.1016/j.landusepol.2019.104204
Six, J., Elliott, E. T., and Paustian, K. (2000). Soil macroaggregate turnover and microaggregate formation: a mechanism for C sequestration under no-tillage agriculture. Soil Biol. Biochem. 32, 2099–2103. doi: 10.1016/S0038-0717(00)00179-6
Skinner, P. W., Cook, J. A., and Matthews, M. A. (1988). Phosphorus fertilizer applications under phosphorus-limited. Vitis 27, 95–109.
Sokol, N. W., and Bradford, M. A. (2019). Microbial formation of stable soil carbon is more efficient from belowground than aboveground input. Nat. Geosci. 12, 46–53. doi: 10.1038/s41561-018-0258-6
Sokol, N. W., Kuebbing, S. E., Karlsen-Ayala, E., and Bradford, M. A. (2019). Evidence for the primacy of living root inputs, not root or shoot litter, in forming soil organic carbon. New Phytol. 221, 233–246. doi: 10.1111/nph.15361
Steenwerth, K. L., McElrone, A. J., Calderon-Orellana, A., Hanifin, R. C., Storm, C., Collatz, W., et al. (2013). Cover crops and tillage in a mature merlot vineyard show few effects on grapevines. Am. J. Enol. Vitic. 64, 515–521. doi: 10.5344/ajev.2013.12119
Steenwerth, K., and Belina, K. M. (2008a). Cover crops and cultivation: impacts on soil N dynamics and microbiological function in a Mediterranean vineyard agroecosystem. Appl. Soil Ecol. 40, 370–380. doi: 10.1016/j.apsoil.2008.06.004
Steenwerth, K., and Belina, K. M. (2008b). Cover crops enhance soil organic matter, carbon dynamics and microbiological function in a vineyard agroecosystem. Appl. Soil Ecol. 40, 359–369. doi: 10.1016/j.apsoil.2008.06.006
Stewart, R. D., Jian, J., Gyawali, A. J., Thomason, W. E., Badgley, B. D., Reiter, M. S., et al. (2018). What we talk about when we talk about soil health. Agric. Environ. Lett. 3, 1–5. doi: 10.2134/ael2018.06.0033
Suddick, E. C., Ngugi, M. K., Paustian, K., and Six, J. (2013). Monitoring soil carbon will prepare growers for a carbon trading system. Calif. Agric. 67, 162–171. doi: 10.3733/ca.v067n03p162
Sweet, R. M., and Schreiner, R. P. (2010). Alleyway cover crops have little influence on pinot noir grapevines (Vitis vinifera L.) in Two Western Oregon Vineyards. Am. J. Enol. Vitic. 61, 240–252.
Teasdale, J. R., and Mohler, C. L. (1993). Light transmittance, soil temperature, and soil moisture under residue of hairy vetch and rye. Agron. J. 85, 673–680. doi: 10.2134/agronj1993.00021962008500030029x
Tesic, D., Keller, M., and Hutton, R. J. (2007). Influence of vineyard floor management practices on grapevine vegetative growth, yield, and fruit composition. Am. J. Enol. Vitic. 58, 1–11.
Van Leeuwen, C., Roby, J.-P., and De Rességuier, L. (2018). Soil-related terroir factors: a review. OENO One 52, 173–188. doi: 10.20870/oeno-one.2018.52.2.2208
Vandenkoornhuyse, P., Quaiser, A., Duhamel, M., Le Van, A., and Dufresne, A. (2015). The importance of the microbiome of the plant holobiont. New Phytol. 206, 1196–1206. doi: 10.1111/nph.13312
Vaudour, E., Costantini, E., Jones, G. V., and Mocali, S. (2015). An overview of the recent approaches to terroir functional modelling, footprinting and zoning. Soil 1, 287–312. doi: 10.5194/soil-1-287-2015
Vereecken, H. (1995). Estimating the unsaturated hydraulic conductivity from theoretical models using simple soil properties. Geoderma 65, 81–92. doi: 10.1016/0016-7061(95)92543-X
Verhoeven, E., Decock, C., Garland, G., and Lazcano, C. (2019). Vineyard nitrous oxide (N2O) emissions. Wine Business Monthly, 196–205.
Verhoeven, E., Pereira, E., Decock, C., Garland, G., Kennedy, T., Suddick, E., et al. (2017). N 2 O emissions from California farmlands: a review. Calif. Agric. 71, 148–159. doi: 10.3733/ca.2017a0026
Versari, A., Laurie, V. F., Ricci, A., Laghi, L., and Parpinello, G. P. (2014). Progress in authentication, typification and traceability of grapes and wines by chemometric approaches. Food Res. Int. 60, 2–18. doi: 10.1016/j.foodres.2014.02.007
Vicente-Vicente, J. L., García-Ruiz, R., Francaviglia, R., Aguilera, E., and Smith, P. (2016). Soil carbon sequestration rates under Mediterranean woody crops using recommended management practices: a meta-analysis. Agric. Ecosyst. Environ. 235, 204–214. doi: 10.1016/j.agee.2016.10.024
Vogel, H.-J., Wollschläger, U., Helming, K., Heinrich, U., Willms, M., Wiesmeier, M., et al. (2019). “Assessment of soil functions affected by soil management,” in Atlas of Ecosystem Services, eds M. Schröter, A. Bonn, S. Klotz, R. Seppelt, and C. Baessler (Cham: Springer), 77–82. doi: 10.1007/978-3-319-96229-0_13
Wheeler, S. J., and Pickering, G. J. (2003). Optimizing grape quality through soil management practices. Food Agric. Environ. 1, 190–197.
White, R. E., Balachandra, L., Edis, R., and Chen, D. (2007). The soil component of terroir. OENO One 41, 9–18. doi: 10.20870/oeno-one.2007.41.1.860
Wienhold, B. J., Andrews, S. S., and Karlen, D. L. (2004). Soil quality: a review of the science and experiences in the USA. Environ. Geochem. Health 26, 89–95. doi: 10.1023/B:EGAH.0000039571.59640.3c
Williams, L. E. (1999). Fertilizer Use Efficiency and Influence of Rootstocks on Uptake and Accumulation of Nutrients in Wine Grapes Grown in the Coastal Valleys of California. Davis, CA: UC Davis Department of Viticulture and Enology.
Wilson, S. G., Lambert, J.-J., and Dahlgren, R. A. (2016). Seasonal phosphorus dynamics in a volcanic soil of Northern California. Soil Sci. Soc. Am. J. 80, 1222–1230. doi: 10.2136/sssaj2016.02.0028
Wilson, S. G., Lambert, J.-J., Nanzyo, M., and Dahlgren, R. A. (2017). Soil genesis and mineralogy across a volcanic lithosequence. Geoderma 285, 301–312. doi: 10.1016/j.geoderma.2016.09.013
Winter, S., Bauer, T., Strauss, P., Kratschmer, S., Paredes, D., Popescu, D., et al. (2018). Effects of vegetation management intensity on biodiversity and ecosystem services in vineyards: a meta-analysis. J. Appl. Ecol. 55, 2484–2495. doi: 10.1111/1365-2664.13124
Wolff, M. W., Alsina, M. M., Stockert, C. M., Khalsa, S. D. S., and Smart, D. R. (2018). Minimum tillage of a cover crop lowers net GWP and sequesters soil carbon in a California vineyard. Soil Tillage Res. 175, 244–254. doi: 10.1016/j.still.2017.06.003
Keywords: sustainable soil management, viticulture, C sequestration, compost, cover crops, soil quality
Citation: Lazcano C, Decock C and Wilson SG (2020) Defining and Managing for Healthy Vineyard Soils, Intersections With the Concept of Terroir. Front. Environ. Sci. 8:68. doi: 10.3389/fenvs.2020.00068
Received: 18 January 2020; Accepted: 08 May 2020;
Published: 18 June 2020.
Edited by:
Silvia Winter, University of Natural Resources and Life Sciences Vienna, AustriaReviewed by:
Daniela Businelli, University of Perugia, ItalyArtemi Cerdà, University of Valencia, Spain
Copyright © 2020 Lazcano, Decock and Wilson. This is an open-access article distributed under the terms of the Creative Commons Attribution License (CC BY). The use, distribution or reproduction in other forums is permitted, provided the original author(s) and the copyright owner(s) are credited and that the original publication in this journal is cited, in accordance with accepted academic practice. No use, distribution or reproduction is permitted which does not comply with these terms.
*Correspondence: Cristina Lazcano, Y2xhemNhbm9AdWNkYXZpcy5lZHU=