- 1Urat Desert-Grassland Research Station, Naiman Desertification Research Station, Northwest Institute of Eco-Environment and Resources, Chinese Academy of Sciences, Lanzhou, China
- 2Sustainable Development Institute for Western Region of Mongolia, Ulaanbaatar, Mongolia
Slow organic material and nutrient turnover is one of the limiting processes in arid and semiarid ecosystems, and cellulose decomposers play an important role in straw turnover and nutrient return in cropland ecosystem in drylands. In order to moderate the limiting effect of material turnover, a highly efficient cellulose decomposing fungus was screened from 85 cellulose decomposing fungi and we named the isolated fungus as NMCel-crop1 in the sandy cropland of Horqin Sandy Land in a semiarid agro-pasture ecotone in northern China. This fungal decomposer was identified as Rhizomucor variabilis by using morphological and rDNA-ITS molecular methods. The optimized temperature for expressing its carboxymethyl cellulose (CMC) enzyme activity ranges from 40 to 55°C. The CMC enzyme activity was significantly and highly produced by the NMCel-crop1 than that in situ soil in the cropland, and the filter paper decomposition rate was 82% in 7 days. The field straw decomposition experiment showed that the decomposition rate of maize straw infected by NMCel-crop1 reached at 92.5% in 1 year, which was 26% higher than that without infection. Straw turnover accelerated by NMCel-crop1 significantly increased soil organic carbon (SOC) and total nitrogen (TN) by 34.08 and 14.26%, respectively, indicating that the selected highly efficient decomposing fungus could accelerate straw turnover rate and increase SOC and nitrogen content and promote soil fertility and soil health in the sandy cropland, as well as potentially improve crop productivity and quality in the sustainable agriculture management of the arid and semiarid sandy cropland.
Introduction
The boundary line of the agricultural and pastoral area is approximately consistent with the 400-mm isohyet in China. The land use in the southeast part of the isohyet is cropping dominant, and the northwest part is pasture dominant. The agro-pasture transitional ecotone is in between the agricultural and pastoral area, in which both cropping is expanding and grazing intensified spatially and temporally. The agro-pasture transitional ecotone of northern China starts from Hulun Buir of western Great Khingan, southward to Tongliao and Chifeng of Inner Mongolia, then goes to northern Hebei, Shanxi, and Shaanxi provinces, and ends up at eastern Gansu province (Zhao et al., 2002). The annual precipitation of the transitional ecotone is ranging from 300 to 450 mm. Precipitation, which fluctuates significantly, is the main limiting factor for both cropping and grazing in this area. The vegetation changes variably in consequence with the precipitation, which makes this transitional area a very vulnerable ecotone (Zhao et al., 2003).
Horqin Sandy Land is located in the southeast part of Inner Mongolia, which is one of the most vulnerable and most typical areas for ecological and environmental study in semiarid agro-pasture transitional ecotone. During the recent few decades, a large area of grassland has been converted into farmland year by year in this region, mostly due to the rapid growth of population and their aspiration for better life. Therefore, maize (Zea mays L.) became the best choice for cropping, because of its higher productivity and higher price. However, maize consumes much more water than the local plants, and the overuse of water for irrigation resulted in desertification of this vulnerable sandy land ecosystem (Zhao et al., 2015; Wang et al., 2016a).
Straw turnover is a conservational management to enhance the soil fertility in agricultural sustainable maintenance (Kassam et al., 2009). However, the low precipitation and temperature makes the straw decompose much slower in winter and spring, retaining a large amount of undecomposed cellulose in and/or upon the surface soil of the cropland in Horqin sandy cropland. The undecomposed straw residual will lead to pest and virus breakouts in this area. So many farmers prefer burning the maize straw after harvest, which causes heavy air pollution, consequently causing respiratory infections and also waste of organic resource in the semiarid area (Qu et al., 2012; Zhang et al., 2014). Cellulose is one of the most renewable bioenergy materials, producing alcohol, carbohydrate, single-cell protein, and organic fertilizer during its enzymatic decomposition procedure (Lynd et al., 2002). Therefore, the efficient treatment and suitable utilization of cellulose could not only supplement bioenergy shortage but also relieve environmental pollution from agricultural waste, especially in dryland areas. It is an efficient and environmentally friendly approach to decompose cellulose by cellulolytic decomposers (Panagiotou et al., 2003). Cellulose decomposers play a very important role in sandy farmland ecosystems. They participate actively in straw turnover, nutrient uptake, and pest control (Jin, 2004; Wang et al., 2016b; Jaiswal et al., 2017). The cellulose decomposers isolated from terrestrial ecosystems are mostly fungi, and most of them could be categorized into Trichoderma, Penicillium, Aspergillus, and Fusarium (Panagiotou et al., 2003; Wen et al., 2005; Lu et al., 2011; Wang et al., 2015a). The study on the functional cellulose decomposers and their ecological service are fundamental and essential in sustainable agricultural management in drylands (Qin and Wei, 2007). However, it is seldom reported on the isolation of efficient cellulosed decomposers in semiarid sandy cropland. The purpose of this study is to isolate several highly efficient cellulose decomposers in Horqin sandy cropland. We hypothesized that isolation of highly efficient cellulose decomposers in Horqin sandy cropland soil could not only enrich soil functional microbial bank and accelerate maize straw turnover but also promote sustainable agricultural development in the agro-pasture ecotone of northern China.
Materials and Methods
Study Area
This study was conducted in Naiman Desertification Research Station of the Chinese Academy of Sciences (NDRS), which is located in Naiman County in the southwestern part of the Horqin Sandy Land, Inner Mongolia, northern China (120°55′E, 42°41′N; 360 m a.s.l.) (Figure 1). The climate in this area is characterized as a temperate, semiarid continental monsoon, with a hot summer and cold winter. The mean annual precipitation is 366 mm, with 70–80% falls during the general plant growing season from June to September. The annual mean open-pan evaporation is around 1935 mm, five times greater than annual precipitation. The annual mean temperature is around 6.4°C, ranging from a monthly maximum of 23.5°C in July to a monthly minimum of −16.8°C in January. The annual mean wind velocity ranges from 3.6 to 4.1 m/s, and the dominant wind is southwest in summer and autumn and northwest in winter and spring. The zonal soil is classified as sandy chestnut, which is sandy in texture, light yellow in color, and loose in structure and is vulnerable to wind erosion (Zhao et al., 2003; Wang et al., 2016b). The original landscape was dominated by sandy grassland with scattered trees (mostly elms, Ulmus spp.). However, the grassland has been replaced by farmland, due to the increase in population and development of irrigation. Maize (Z. mays L.) monoculture dominates the cultivated land because of its higher productivity and easier management (Wang et al., 2016a).
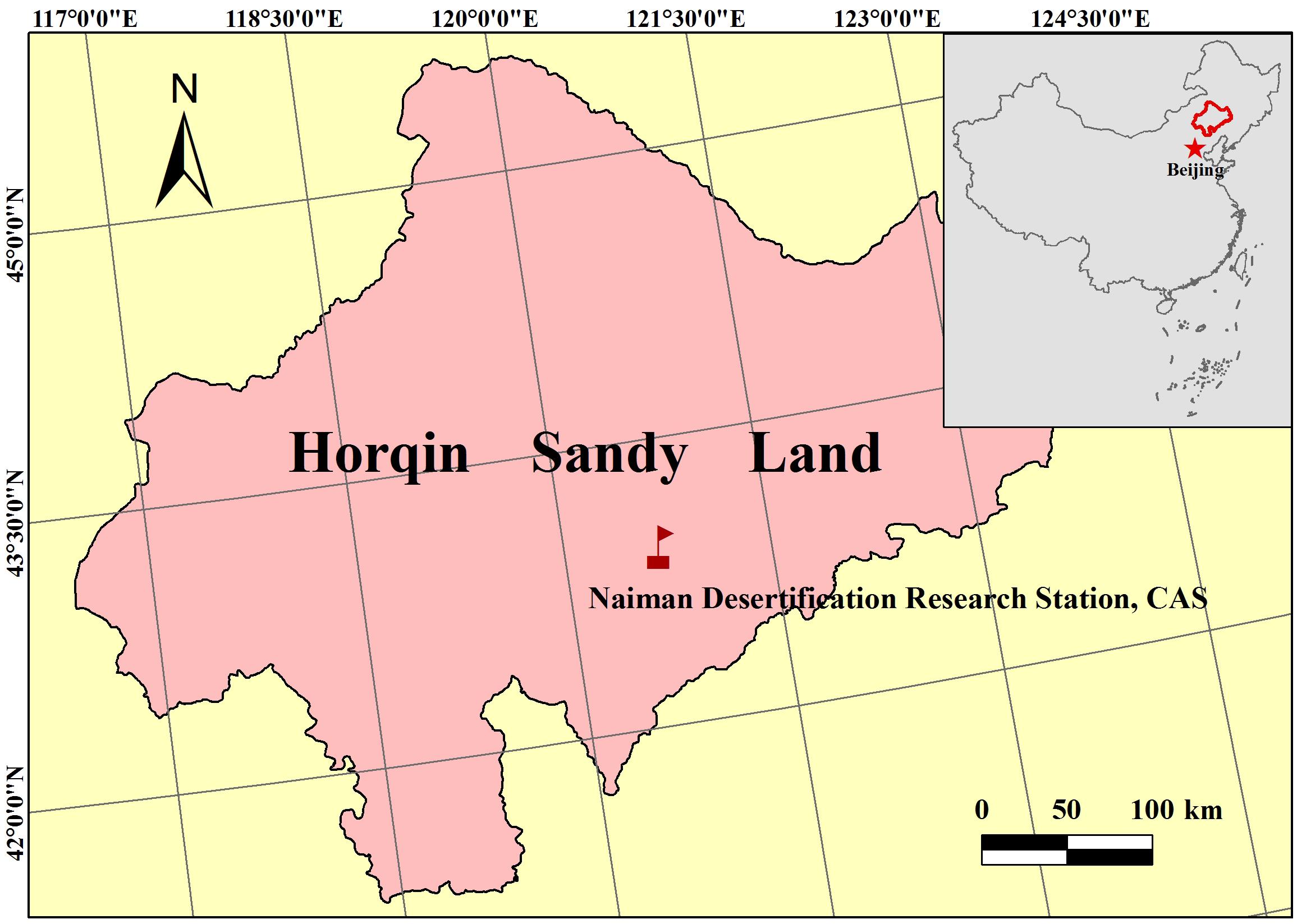
Figure 1. Study area of Naiman Desertification Research Station (NDRS), in the southeastern part of Inner Mongolia, typical area in agro-pasture ecotone of northern China.
Experimental Design
Soil Collection
We collected soil samples for isolating cellulose decomposers in the comprehensive observation cropland field in NDRS. This long-term observation field was established in 1997, covering 20,000 m2 and planting maize since then. This field is a typical irrigated sandy cropland in Horqin sandy cropland. We randomly set up 10 quadrats (1 m × 1 m) in the observation field, took five replications of soil cores at a depth of 0–20 cm in each quadrat and mixed as a pooled sample. Every pooled sample was sieved (<2 mm) to remove rocks and plant material and stored separately in sterilized ziplock bags at 4°C, prepared for laboratory isolation of cellulose decomposers. The soil collection was conducted in early August. The basic characteristics of the observation field are shown in Table 1.
Culturable Medium for Cellulose Decomposing Fungi Isolation
The medium used for isolating and selecting efficient cellulose-decomposing fungi includes carboxymethyl cellulose (CMC) medium, PDA medium, Congo Red CMC medium, litter medium, and liquid medium (peptone: 2.6 g; yeast extract: 1.3 g; litter powder: 5 g; MgSO4⋅7H2O: 0.8 g; KH2PO4: 2 g; NaCl: 0.1 g; H2O: 1,000 mL). The detailed recipe for each medium was shown in Wang et al. (2015b).
Procedures for Isolation and Screening
Isolation: 10 g fresh soil was mixed into 90 ml sterilized water until the soil was totally suspended. 1 ml supernate was added into 9 ml sterilized water and shaken to produce 1 and 10% soil microbial suspensions. 1-ml suspensions were transferred into CMC medium plates with five replicates for both 1 and 10% suspensions, separately. The plates were incubated at 30°C for 10–15 days until different colonies grew big enough to be picked for purification.
Purification: Separated colonies were picked and transferred into PDA medium plates and incubated at 30°C for 3–5 days. Colonies without any infection were selected as purified fungi for advanced screening.
Screening for efficient cellulose-decomposing fungi: Pure colonies were transferred into litter medium plates at 30°C for 5–10 days. Pure colonies with long mycelium and abundant spore were chosen as cellulose-decomposing fungi. Separated colonies were scraped by vaccinating lancet, placed into Congo Red CMC medium plates, and incubated at 30°C for 10 days to select efficient cellulose decomposing fungi. Colonies with faster growth and larger transparent rings in the Congo Red CMC medium plates were screened as highly efficient cellulose decomposing fungi. The selected fungal strains were stored in Congo Red CMC medium tubes for morphological and molecular identification and further experiment.
Morphological and Molecular Identification
Morphology
The selected fungal strains were transferred into PDA medium plates and incubated at 30°C for 3–5 days. Microscopic examination was performed to observe the characteristics of the mycelium and spore for each strain. “Manual of Fungi Taxa Identification” (Wei, 1979) and “Illustrated Genera of Imperfect Fungi” (Barnett and Hunter, 1998) were used for the fungi taxa identification.
Molecular Identification
DNA was extracted from a small portion of the selected fungi mycelium (Zhang et al., 2008). Universal primers of ITS1 (5′-TCCGTAGGTGAACCTGCGG-3′) and ITS 4 (5′-TCCTCCGCTTATTGATATGC-3′) were used for PCR. PCR reaction system (50 μL): dd H2O 30 μL, PCR buffer 5 μL, dNTP 5 μL, MgCl2 2 μL, formamide 2 μL, ITS1 1.5 μL, rTaq enzyme 1 μL, template DNA 2 μL. PCR reaction condition: 94°C for 5 min, 94°C for 40 s, 55°C for 40 s, 72°C for 1 min, 72°C for 10 min, 38 cycles. The target PCR products with a clear strip were sequenced in Nuosai Gene. The DNA sequences were aligned with the GenBank database in National Center for Biotechnology Information (NCBI)1 to determine the taxa of the selected fungus. Molecular Evolutionary Genetics Analysis 7 (MEGA 7) was used to build a phylogenetic Minimum Evolution (ME) tree based on the DNA sequences that are similar with the target DNA sequence in NCBI, and the evolutionary distances were computed using the p-distance method (Nei and Kumar, 2000).
CMC Enzyme Activity
Carboxymethyl cellulose enzyme activity from the supernate was determined at different temperature gradient (20, 25, 30, 70°C).
A small amount of the final selected fungus was taken and dipped into the conical flasks with the liquid medium. The flasks were shaken (150 r/min) at 30°C for 7 days and then centrifuged at 4,000 r/min for 15 min. The supernate was used for determining the CMC enzyme activity. 0.5 ml of supernate was placed into a tube with 1.5 ml citrate buffer (0.05 mol/l, pH 5.0, containing 0.5% CMC-Na). The tube was water-bathed at different temperature gradients (20, 25, 30, 35, 40, 45, 50, 55, 60, 65, 70°C) for 30 min. Then, 1 ml dinitrosalicylic acid (DNS) was added into the tube which was boiled at 100°C for 5 min. The volume was remained constant at 5 ml when the tube cooled at room temperature. The absorbance was determined at 540 nm in a spectrophotometer. The glucose content was calculated based on the standard curve (Fang et al., 2007; Bayer et al., 2013; Sun et al., 2017).
CMC enzyme activity X = m/(V.t).n (Equation 1)
where m represents glucose content, V represents supernate volume (0.5 mL), t represents reaction time (30 min), and n represents dilution ratio (5/0.5).
A 10 g fresh soil was diluted into 90 ml sterilized water, shaken for 15 min at 150 r/min, and 0.5 ml of the soil supernate was used to determine soil CMC enzyme activity (Guan, 1986).
Decomposition Ability
A small piece of the strain was taken and incubated in the liquid medium conical flask for 24 h. Then, five pieces of filter paper were put into the flask, shaken (150 r/min), and incubated at 30°C for 10 days. Weight loss was determined every day until the weight did not change significantly (Yao and Huang, 2006).
Decomposition rate D = ((m0-mi)/m0) × 100% (Equation 2)
where m0 is the original weight of the filter paper, while mi is the weight at the ith day.
Straw Decomposition in Field Experiment
The maize straw was cut into small pieces (<5 cm) after harvest, and the straw pieces were oven dried at 70°C for 24 h to a constant weight. The maize straw carbon and nitrogen contents were 451.6 ± 6.6 and 7.73 ± 0.26, respectively, and its C:N ratio was 58.42 ± 1.64. 10-g straw pieces were sealed in a 20 cm × 25 cm nylon net bag (net hole are 2 mm × 2 mm). The net bags were soaked with straw in the liquid medium and incubated with the selected cellulose-decomposing fungi for 30 min. Meanwhile, net bags of straw without decomposer infection were set as control. The control net bags were soaked in the liquid medium without any microbes for 30 min to minimize the influence of the liquid medium for straw decomposition. Then, 20 net bags with and without the decomposing fungi were buried at 10 cm deep in the cropland soil, respectively. The net bags were set randomly 20 m away from each other in the comprehensive observation cropland field in NDRS in early October. We collected five net bags with the impact of the selected cellulose decomposing fungi, as well as five control net bags in early December (frozen season, 60th day), next early May (seeding season, the 200th day), next mid August (growing season, 300th day), and next early October (harvest season, 360th day), respectively. The straws in the net bags were cleaned and then oven-dried at 70°C for 24 h. The mycelia from NMCel-crop1 were observed in all of the net bags added with the selected decomposing fungi, suggesting that NMCel-crop1 fungi were present in all the decomposing stages. The maize straw mass loss rate is calculated as 1-D (D refers to the decomposition rate in Eq. 2) (Qu et al., 2011). A modified “Olson” litter mass loss model (Eq. 3) was used to predict straw turnover.
(Equation 3) (Olson, 1963; Liu et al., 2006)
where R is the percentage of remaining mass; x0 is the initial litter mass, and x is the litter mass at the time of t; k represents the litter turnover rate (higher k means faster decomposition rate); α is a correction factor.
Meanwhile, soils were carefully collected under net bags (≤1 cm both with and without selected decomposers) and bare soil at a depth of 0–10 cm was collected as control at the end of the straw decomposition experiment. The soil organic carbon (SOC) and total nitrogen (TN) were analyzed to calculate the nutrient return in the field. SOC was measured by the dichromate oxidation method of Walkley and Black (Nelson and Sommers, 1982), and TN was determined by the Kjeldahl procedure (Institute of Soil Sciences CAS, 1978).
Data Analysis
Origin 8.0 (OriginLab, United States), SPSS 17.0 (IBM SPSS Statistics, United States), and Microsoft excel 2016 (Microsoft, United States) were used to analyze the descriptive statistical data and significance tests. Significant differences were assessed by one-way ANOVA and LSD tests at p < 0.05. All the descriptive data were expressed as means ± SE.
Results
Isolation
The results showed that 85 cellulose-decomposing fungi were detected in the CMC medium plates, 11 of which were purified from the isolation due to the different colors and shapes of the colony. Five strains grew much faster after prescreening in the litter medium plates. These five screened strains were numbered as NMCel-crop1, NMCel-crop2, NMCel-crop3, NMCel-crop4, and NMCel-crop5. Advanced screening showed that NMCel-crop1 grew much faster, and the diameter of hydrolytic ring was significantly larger than the other four strains in the Congo Red CMC medium plates (Table 2). Therefore, NMCel-crop1 was selected as the highly efficient cellulose decomposing fungus.
Identification
From the microscopic images (Figure 2), we could clearly see that there are no separation in the hypha, and single sporangiophores emerge at the end of each hypha. Spherical sporangia, with a great number of spores, appear at the top of sporangiophores. The morphological characteristics illustrated that NMCel-crop1 could be assigned in the Mucoraceae family. DNA sequencing analysis showed that the length of the extracted DNA is 1048 bp, with 38.26% of the G + C base. Blast alignment of the DNA sequence in the NCBI GenBank database indicated that the strain is mostly associated with the known fungus sequence (FJ227892.1: Rhizomucor variabilis var. regularior isolate 80, genetic similarity = 99.49%) (Figure 3). The NMCel-crop1 fungus was identified as the species of R. variabilis in the genus of Rhizomucor and family of Mucoraceae.
CMC Enzyme Activity
Carboxymethyl cellulose enzyme activity from the supernate was determined at different temperature gradients (20, 25, 30, 70°C). The CMC enzyme activity increased gradually from 20 to 40°C, then increased smoothly, and got to its peak at 50°C. It decreased sharply from 55°C (Figure 4). Therefore, the optimized temperature for the NMCel-crop1 fungus to fulfill its decomposing ability is 50°C. The CMC enzyme activity produced by the NMCel-crop1 fungus (0.43 ± 0.03 mg/ml⋅min) was significantly higher than that from the sandy cropland soil (0.05 ± 0.01 mg/ml⋅min) (p < 0.001).
Decomposition Ability
The change in filter paper mass loss, decomposed by the NMCel-crop1 fungus, showed that it decomposed very slowly in the first 3 days (D < 20%). From the 3rd to 6th days, the filter paper weight lost very fast from 20 to 80% and almost lost at a rate of 20% per day. Then, after the 7th day (D = 82.03 ± 2.46%), the mass did not change significantly (Figure 5).
Straw Decomposition in the Field Experiment
The result from the field experiment (Figure 6) showed that straw was decomposed very fast at the first decomposing stage (harvest to frozen seasons, 0–60 day) by 42.27 ± 3.91% infected by NMCel-crop1 fungus, which was 11.47% higher than bare soil, and then became much slower in the frozen season from early December to early May (60–200 day), 9.03% infected by the NMCel-crop1 fungus, and 4.47% in bare soil. When it became warmer, the straw decomposition rate was much faster again. The decomposition rate reached 83.69 ± 2.50% infected by the NMCel-crop1 fungus, 20% higher than bare soil at the end of the growing season in mid August. The daily decomposition rate did not show a statistical difference between NMCel-crop1 fungus (0.28%/day) and bare soil (0.26%/day) at the 3rd decomposing stage (seeding season to growing season, 200–300 day). After the growing season (300–400 day), the straw mass loss was still going on when infected by the NMCel-crop1 fungus, but it slowed down in bare soil. The straw mass loss reached 92.49% infected by the NMCel-crop1 fungus, 26% higher than that in bare soil after 1 year buried in the sandy cropland soil. The residual of the straw remained less than 10% infected by the NMCel-crop1 fungus, which significantly accelerated the straw turnover in the semiarid sandy cropland. The modified “Olson” mass loss model was made to predict maize straw turnover both in bare soil and that affected by the NMCel-crop1 fungus in the field. It clearly showed that the observed mass loss rate could be well fitted in the model (R2 > 0.9) and the litter turnover rate k was much higher in the model affected by NMCel-crop1 than that in bare soil (Table 3), indicating that the maize straw turnover rate was much fast with the help of the NMCel-crop1 fungus than that in natural bare soil.
To evaluate the quantitative nutrient return in the cropland soil, SOC and TN were collected and analyzed at the end of the field experiment. The result showed that maize straw turnover increased SOC significantly. SOC was 20.16 and 34.08% significantly higher in soils under the net bag with (WD) the selected decomposer compared with that in soils without the selected decomposer (ND) and the control soil (CK), respectively. Soil TN did not show a statistical difference between ND and CK. However, TN was significantly higher in WD than that in ND and CK. SOC and nitrogen ratio (C:N) increased significantly by 13.06 and 16.80% in ND and WD than that in CK. The C:N ratio did not show a significant difference between ND and WD. The results indicated that our selected decomposing fungus could not only accelerate the straw turnover rate but also could increase SOC and nitrogen content and potentially promote soil fertility and soil health in the sandy cropland.
Discussion
We have isolated and selected abundant and highly efficient cellulose decomposers, some of which contributed to litter decomposition by 50% in 30 days in the former work in Horqin sandy grassland (Wang et al., 2015a). However, the cellulose decomposers detected in the sandy cropland was much less (Wang et al., 2016a) and could not isolate highly efficient cellulose decomposers for a long time. That is why we optimized the isolation, purification, and screening procedures to obtain this highly efficient cellulose-decomposing fungus (R. variabilis) for promoting straw turnover and nutrient cycling in semiarid sandy cropland maintenance in Horqin sandy cropland.
Soil organic carbon is an important component to soil fertility and water holding capacity. Soil nitrogen is a major nutrient element that influences plant growth and biogeochemical cycle in terrestrial ecosystems. The SOC and nitrogen ratio (C:N) has been used as a key factor to evaluate soil health in agriculture ecosystem (Al-Kaisi et al., 2005; Kibblewhite et al., 2008; Swangjang, 2015; Liu et al., 2018). Our result showed that straw turnover accelerated by the selected efficient decomposing fungus significantly increased SOC, TN, and C:N ratio in the sandy cropland (Figure 7), indicating that our selected decomposer (NMCel-crop1) could be magnified and demonstrated to accelerate straw turnover and increase soil nutrient in Horqin sandy cropland and other semiarid regions.
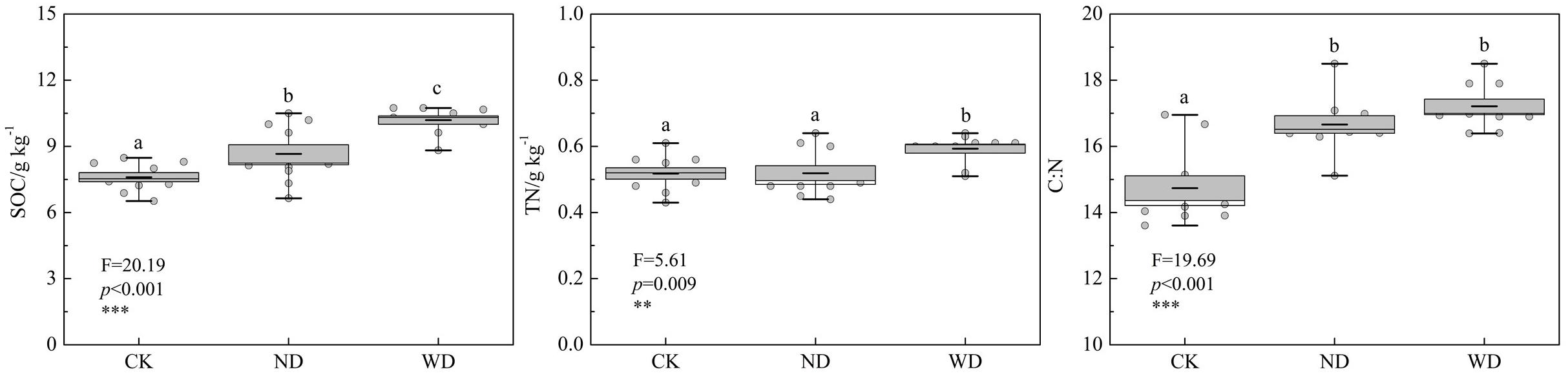
Figure 7. Influence of straw turnover (with and without efficient cellulose decomposer) on soil organic carbon (SOC), total nitrogen (TN), and carbon and nitrogen ratio (C:N) in the field decomposition experiment. CK: bare soil without straw; ND: soil under straw net bags without selected decomposing fungi; WD: soil under straw net bags with selected decomposing fungi. Different letters represent significant difference among the treatments at p < 0.05.
Generally speaking, the soil C:N ratio ranging from 15 to 25% indicates that the soil is in good health. The higher soil C:N ratio indicates that the soil has greater ability to immobilize additional nitrogen input (Livesley et al., 2016). In our experimental site, the C:N ratio in the bare soil was 14.74, mostly due to low SOC in sandy soil, as well as high chemical fertilizer input of agricultural management. Straw turnover significantly increased soil C:N ratio to 16.66 because of increased carbon input. Highly efficient cellulose-decomposing fungus (NMCel-crop1) addition promoted the soil C:N ratio at 17.21, indicating that maize absorbed more immobilized nitrogen during the growing season.
The selected highly efficient cellulose-decomposing fungus could produce CMC enzyme at 50°C and even higher (Figure 4), which is almost the maximum soil temperature recorded in Naiman Desertification Research Station2, as well as in most of other deserts in Asia and in Africa (Zhao, 2012). The result indicated that the selected cellulose-decomposing fungus could also be potentially magnified and used in the arid and semiarid areas to accelerate litter decomposition, where the litter decomposed rate is slow.
Carboxymethyl cellulose enzyme activity from the supernate was determined at different temperature gradient (20, 25, 30, 70°C). The CMC enzyme activity increased gradually from 20 to 40°C, then increased smoothly, and got to its peak at 50°C. It decreased sharply from 55°C (Figure 4). Therefore, the optimized temperature for the NMCel-crop1 fungus to fulfill its decomposing ability is 50°C, and the optimized range for litter decomposition should be 40–55°C. The CMC enzyme activity produced by the NMCel-crop1 fungus (0.43 ± 0.03 mg/ml⋅min) was significantly higher than that from the sandy cropland soil (0.05 ± 0.01 mg/ml⋅min) (p < 0.001).
Many species in Rhizomucor genus are key fungi for fermentation in the food industry, and some of them are pathogenic fungi for skin disease. They play a very important role in alcoholic fermentation, sugar production, and lipid enzymolysis. Research showed that Rhizomucor pusillus could catalyze different carbon source substrates into small particles, such as glycerinum, lactic acid, and xylitol, which are very important products in the food industry (Millati et al., 2005). Chadha et al. (2004) isolated a fungus (R. pusillus) with high phosphatase activity from the compost, and it could be used to regulate the composition of livestock fodder. Several species of Rhizomucor was isolated from soils in a semiarid area of southern Africa. These fungal species had high tolerance in excess nitrite and could survive under extreme drought stress (Seabi et al., 1999). Another species of Rhizomucor (Rhizomucor miehei) could produce lipase, which could be used in dairy products (Soltani et al., 2019) and also in biofuel industry (Rodrigues and Fernandez-Lafuente, 2010; Adnan et al., 2018). Some of the R. miehei could also produce mannose enzyme in strong acid and high-temperature environments (Li et al., 2017). Reports about R. variabilis were mostly focused on medical science. They are pathogenic fungi for many skin diseases (Lu et al., 2009; Tomita et al., 2011; Patil et al., 2013). Recently, a few reports on food production indicated that R. variabilis showed highly alkali resistance to produce lipase (Bancerz et al., 2015, 2018). Researchers isolated R. variabilis from mushroom (Ke et al., 2016) and corncob (Xu et al., 2017). These fungi were sensitive to nitrogen variations and beneficial for protecting crop from rot disease. Our research firstly recruited new fungal species into soil functional microbe banks in semiarid sandy cropland and tested their highly efficient litter decomposition function.
Soil microorganisms work collaboratively to regulate soil health and maintain the sustainable production in agricultural cropland (Chapin et al., 2002; Acostamartínez et al., 2010; Jaiswal et al., 2017). Cellulose decomposers are mainly responsible for crop straw decomposition and acceleration of its turnover in the agricultural ecosystem. Crop straw turnover could promote soil microbial activity and increase soil fertility, so that less chemical fertilizer would be applied and high-quality agricultural products could be produced in green agricultural development. Therefore, straw turnover became an effective practice in sustainable agricultural management (Roper and Gupta, 1995; Wei et al., 2017). However, in the cold and semiarid agro-pasture ecotone, such as Horqin Sandy Land, the precipitation is low and so is the temperature, especially in winter and spring. The straw decomposed very slowly after harvest, and the organic material could not return to the soil rapidly, leading to a waste of organic resource and even pest disease in the coming year. In the natural field condition of Horqin sandy cropland, the maize straw decomposition rate is only 35% from its harvest to the next seeding season, and it reaches 66% in a whole year, and 1/3 of the maize straw could not be decomposed. The straw residual is mostly lignin and/or polycellulose, and the residual could hardly be decomposed. We isolated and selected this highly efficient cellulose decomposing fungus (R. variabilis) from soils in situ in Horqin sandy cropland and magnified and accelerated straw turnover. The application of the decomposing microbial agents significantly increased the straw decomposition rate by 50% from its harvest to the next seeding season, and reached to >90% in 1 year. The maize straw could mainly return into soil as organic matter in a year. The application of this fungus could reduce the chemical fertilizer application and produce healthy crop products in a sustainable agricultural management system in the arid and semiarid agro-pasture area.
Conclusion
Five cellulose-decomposing fungi were isolated, and one of them was selected as a highly efficient cellulose decomposer in sandy cropland of the agro-pasture ecotone in northern China. This selected cellulose-decomposing fungus was identified as R. variabilis. The optimized temperature for expressing its CMC enzyme activity ranges from 40 to 55°C. The CMC enzyme activity produced by the selected fungus was 0.43 mg/ml⋅min, which was significantly higher than that from sandy cropland soil. It has high ability to decompose cellulose material, not only in laboratory test but also in the field experiment. Straw turnover accelerated by NMCel-crop1 could significantly increase SOC and TN input, indicating that the selected highly efficient decomposing fungi could potentially promote soil fertility and soil health in sandy cropland.
Data Availability Statement
The datasets generated for this study are available on request to the corresponding author.
Author Contributions
SW and XZ conceived and planned the experiment. SW and JL participated in the field and laboratory work. SW performed the experiment and wrote the original manuscript. BS contributed the data analysis and manuscript editing. All authors contributed to the final manuscript.
Funding
This work was financially supported by the National Natural Science Foundation of China (41771117), China National Key Research and Development Plan (2016YFC0500506) from the Northwest Institute of Eco-Environment and Resources, CAS, and collaborative support from CRRP2017-04MY-Balt project by Asia-Pacific Network for Global Change Research (APN).
Disclaimer
Frontiers Media SA remains neutral with regard to jurisdictional claims in published maps and institutional affiliations.
Conflict of Interest
The authors declare that the research was conducted in the absence of any commercial or financial relationships that could be construed as a potential conflict of interest.
Acknowledgments
We thank all the members in Naiman Desertification Research Station and Urat Desert-Grassland Research Station of CAS for their help in field and laboratory work.
Footnotes
References
Acostamartínez, V., Burow, G., Zobeck, T. M., and Allen, V. G. (2010). Soil microbial communities and function in alternative systems to continuous cotton. Soil Sci. Soc. Am. J. 74, 1181–1192. doi: 10.2136/sssaj2008.0065
Adnan, M., Li, K., Xu, L., and Yan, Y. (2018). X-Shaped ZIF-8 for immobilization Rhizomucor miehei lipase via encapsulation and its application toward biodiesel production. Catalysts 8:96. doi: 10.3390/catal8030096
Al-Kaisi, M. M., Yin, X., and Licht, M. A. (2005). Soil carbon and nitrogen changes as influenced by tillage and cropping systems in some Iowa soils. Agric. Ecosyst. Environ. 105, 635–647. doi: 10.1016/j.agee.2004.08.002
Bancerz, R., Jaroszuk, M. O., Jaszek, M., Janusz, G., Stefaniuk, D., Sulej, J., et al. (2015). New alkaline lipase from Rhizomucor variabilis: biochemical properties and stability in the presence of microbial EPS. Biotechnol. Appl. Biochem. 63, 67–76. doi: 10.1002/bab.1351
Bancerz, R., Osińska-Jaroszuk, M., Jaszek, M., Sulej, J., Wiater, A., Matuszewska, A., et al. (2018). Fungal polysaccharides as a water-adsorbing material in esters production with the use of lipase from Rhizomucor variabilis. Int. J. Biol. Macromol. 118, 957–964. doi: 10.1016/j.ijbiomac.2018.06.162
Barnett, H. L., and Hunter, B. B. (1998). Illustrated Genera of Imperfect Fungi, 4th Edn. St. Paul: APS Press.
Bayer, E. A., Shoham, Y., and Lamed, R. (2013). “Lignocellulose-decomposing bacteria and their enzyme systems,” in The Prokaryotes: Prokaryotic Physiology and Biochemistry, eds E. Rosenberg, E. DeLong, S. Lory, E. Stackebrandt, and F. Thompson (Berlin: Springer), 215–266. doi: 10.1007/978-3-642-30141-4_67
Chadha, B. S., Harmeet, G., Mandeep, M., Saini, H. S., and Singh, N. (2004). Phytase production by the thermophilic fungus Rhizomucor pusillus. World J. Microbiol. Biotechnol. 20, 105–109. doi: 10.1023/b:wibi.0000013319.13348.0a
Chapin, F. S. III, Matson, P. A., and Vitousek, P. M. (2002). Principles of Terrestrial Ecosystem Ecology. Berlin: Springer.
Fang, X., Chen, H., Zhao, X., Tao, P., and Xu, H. (2007). Determination of enzyme activity of straw cellulose-decomposing microorganisms. Lett. Biotechnol. 18, 628–630.
Institute of Soil Sciences CAS (1978). Physical and Chemical Analysis Methods of Soils. Shanghai: Shanghai Science Technology Press.
Jaiswal, D. K., Verma, J. P., and Yadav, J. (2017). Microbe Induced Degradation of Pesticides in Agricultural Soils. Berlin: Springer.
Jin, H. (2004). Effect of applying cellulose-decomposing microbes on rice straw decomposition. Acta Agric. Shanghai 20, 83–85.
Kassam, A., Friedrich, T., Shaxson, F., and Pretty, J. (2009). The spread of conservation agriculture: justification, sustainability and uptake. Int. J. Agric. Sustainabil. 7, 292–320. doi: 10.3763/ijas.2009.0477
Ke, L. N., Hu, Y. H., Gao, H. J., Pan, Z. Z., and Wang, Q. (2016). Antimicrobial activities of cinnamic acid and its derivatives to microorganisms isolated from rotten Agaricus bisporus. J. Xiamen Univ. 55, 330–335.
Kibblewhite, M. G., Ritz, K., and Swift, M. J. (2008). Soil health in agricultural systems. Philos. Trans. R. Soc. B Biol. Sci. 363, 685–701.
Li, Y., Yi, P., Yan, Q., Qin, Z., Liu, X., and Jiang, Z. (2017). Directed evolution of a β-mannanase from Rhizomucor miehei to improve catalytic activity in acidic and thermophilic conditions. Biotechnol. Biofuels 10:143.
Liu, X., Li, L., Wang, Q., and Mu, S. (2018). Land-use change affects stocks and stoichiometric ratios of soil carbon, nitrogen, and phosphorus in a typical agro-pastoral region of northwest China. J. Soils Sediments 18, 3167–3176. doi: 10.1007/s11368-018-1984-5
Liu, Z. W., Gao, W. J., Pan, K. W., Du, H. X., and Zhang, L. P. (2006). Discussion on the study methods and models of litter decomposition. Acta Ecol. Sin. 26, 1993–2000.
Livesley, S. J., Ossola, A., Threlfall, C. G., Hahs, A. K., and Williams, N. S. G. (2016). Soil carbon and carbon/nitrogen ratio change under tree canopy, tall grass, and turf grass areas of urban green space. J. Environ. Qual. 45, 215–223. doi: 10.2134/jeq2015.03.0121
Lu, G. X., Liu, W., Bian, J., Fu, M. T., and Cheng, X. R. (2011). Study on cultural characteristic of one cellulose-decomposing strains of sungus from alpine grassland soil in eastern Qilian Mountains. Grassland Turf 31, 50–55.
Lu, X., Liu, Z., Shen, Y., She, X., Lu, G., Ping, Z., et al. (2009). Primary cutaneous zygomycosis caused by Rhizomucor variabilis: a new endemic zygomycosis? A case report and review of 6 cases reported from China. Clin. Infect. Dis. 49, e39–e43.
Lynd, L. R., Weimer, P. J., Van Zyl, W. H., and Pretorius, I. S. (2002). Microbial cellulose utilization: fundamentals and biotechnology. Microbiol. Mol. Biol. Rev. 66, 506–577. doi: 10.1128/mmbr.66.3.506-577.2002
Millati, R., Edebo, L., and Taherzadeh, M. J. (2005). Performance of Rhizopus, Rhizomucor, and Mucor in ethanol production from glucose, xylose, and wood hydrolyzates. Enzyme Microb. Technol. 36, 294–300. doi: 10.1016/j.enzmictec.2004.09.007
Nei, M., and Kumar, S. (2000). Molecular Evolution and Phylogenetics. Oxford: Oxford University Press.
Nelson, D. W., and Sommers, L. E. (1982). Total carbon, organic carbon and organic matter. Methods Soil Anal. 9, 961–1010.
Olson, J. S. (1963). Energy storage and the balance of producers and decomposers in ecological systems. Ecology 44, 322–331. doi: 10.2307/1932179
Panagiotou, G., Kekos, D., Macris, B. J., and Christakopoulos, P. (2003). Production of cellulolytic and xylanolytic enzymes by Fusarium oxysporum grown on corn stover in solid state fermentation. Ind. Crops Prod. 18, 37–45. doi: 10.1016/s0926-6690(03)00018-9
Patil, A. B., Chandramohan, K., Shivaprakash, M. R., Nadgir, S. D., and Lakshminarayana, S. A. (2013). Rhizomucor variabilis: a rare causative agent of primary cutaneous zygomycosis. Indian J. Med. Microbiol. 31, 302–305. doi: 10.4103/0255-0857.115662
Qin, H. L., and Wei, W. X. (2007). Ecological significance of microbial biotechnology in modern agroecosystem. Chinese Agric. Sci. Bull. 23, 249–253.
Qu, C., Li, B., Wu, H., and Giesy, J. P. (2012). Controlling air pollution from straw burning in china calls for efficient recycling. Environ. Sci. Technol. 46, 7934–7936. doi: 10.1021/es302666s
Qu, H., Zhao, X., Zhao, H., Zuo, X., Wang, S., Wang, X., et al. (2011). Litter decomposition rates in Horqin Sandy Land, Northern China: effects of habitat and litter quality. Fresenius Environ. Bull. 20, 3304–3312.
Rodrigues, R. C., and Fernandez-Lafuente, R. (2010). Lipase from Rhizomucor miehei as an industrial biocatalyst in chemical process. J. Mol. Catal. B Enzym. 64, 1–22. doi: 10.1016/j.molcatb.2010.02.003
Roper, M. M., and Gupta, V. (1995). Management-practices and soil biota. Austral. J. Soil Res. 33, 321–339. doi: 10.1071/sr9950321
Seabi, B. O., Viljoen, B. C., Roux, C., and Botha, A. (1999). Nitrogen utilization and growth at reduced water activity by mucoralean fungi present in soil. S. Afr. J. Bot. 65, 407–413. doi: 10.1016/s0254-6299(15)31031-0
Soltani, M., Sahingil, D., Gokce, Y., and Hayaloglu, A. A. (2019). Effect of blends of camel chymosin and microbial rennet (Rhizomucor miehei) on chemical composition, proteolysis and residual coagulant activity in Iranian Ultrafiltered White cheese. J. Food Sci. Technol. 56, 589–598. doi: 10.1007/s13197-018-3513-3
Sun, S. C., Nan, H. Y., Wang, Y. G., Leng, F. F., Hu, W., Li, W. X., et al. (2017). Screening and enzymatic productivity of a strain of efficient cellulose hydrolyzing bacteria. Chinese J. Microbiol. 29, 1131–1135.
Swangjang, K. (2015). “Soil carbon and nitrogen ratio in different land use,” in Proceedings of the 2015 International Conference on Advances in Environment Research, Hazratbal, 36–40.
Tomita, H., Muroi, E., Takenaka, M., Nishimoto, K., Kakeya, H., Ohno, H., et al. (2011). Rhizomucor variabilis infection in human cutaneous mucormycosis. Clin. Exp. Dermatol. 36, 312–314. doi: 10.1111/j.1365-2230.2010.03956.x
Wang, S. K., Zhao, X. Y., Huang, W. D., Li, Y. Q., Yue, X. F., and Zhang, L. M. (2015a). Isolation and identification of cellulose decomposing fungi and their decomposition ability in the Horqin Sandy Grassland. J. Desert Res. 35, 1584–1591.
Wang, S. K., Zhao, X. Y., Zhao, H. L., Lian, J., Luo, Y., and Yun, J. (2016a). Impact of sand burial on maize (Zea mays L.) productivity and soil quality in Horqin sandy cropland, Inner Mongolia, China. J. Arid Land 8, 569–578. doi: 10.1007/s40333-016-0011-1
Wang, S. K., Zhao, X. Y., Zuo, X. A., Liu, X. P., Qu, H., Mao, W., et al. (2015b). Screening of cellulose decomposing fungi in sandy dune soil of Horqin Sandy Land. Sci. Cold Arid Regions 7, 74–80.
Wang, S. K., Zuo, X. A., Zhao, X. Y., Li, Y. Q., Zhou, X., Lv, P., et al. (2016b). Responses of soil fungal community to the sandy grassland restoration in Horqin Sandy Land, northern China. Environ. Monit. Assess 188, 1–13.
Wei, B., An, J., Zhang, L., Pang, H., Sun, Z., Niu, S., et al. (2017). Improving of soil physical and chemical properties and increasing spring maize yield by straw turnover plus nitrogen fertilizer. Trans. Chinese Soc. Agric. Eng. 33, 168–176.
Wei, J. C. (1979). Manual of Fungi Taxa Identification. Shanghai: Shanghai Science and Technology Press.
Wen, Z., Liao, W., and Chen, S. (2005). Production of cellulase by Trichoderma reesei from dairy manure. Bioresour. Technol. 96, 491–499. doi: 10.1016/j.biortech.2004.05.021
Xu, D. F., Zhang, H. S., Li, T. C., Qi, Y. C., Jiang, C. Q., and Zhou, B. G. (2017). Isolation and identification of the pathogen of maize ear rot in fengyang,anhui province. J. Anhui Agric. Sci. 45, 145–150.
Yao, H. Y., and Huang, C. Y. (2006). Soil Microbial Ecology and Their Research Approaches. Beijing: Science Press.
Zhang, Y., Wei, D., Xing, L., and Li, M. (2008). A modified method for isolating DNA from fungus. Microbiology 35, 466–469.
Zhang, Y., Zang, G. Q., Tang, Z. H., Chen, X. H., and Yu, Y. S. (2014). Burning straw, air pollution, and respiratory infections in China. Am. J. Infect. Control 42, 815–815. doi: 10.1016/j.ajic.2014.03.015
Zhao, H. L., Zhao, X. Y., and Zhang, T. H. (2002). Boundary line on agro-pasture zigzag zone in north China and its problems on eco-environment. Adv. Earth Sci. 17, 739–747.
Zhao, H. L., Zhao, X. Y., and Zhang, T. H. (2003). Desertification processes and its restoration mechanisms in the Horqin Sand Land. Beijing: Ocean Press.
Keywords: cellulose decomposer, CMC enzyme activity, sandy cropland, straw turnover, agro-pasture ecotone
Citation: Wang S, Zhao X, Suvdantsetseg B and Lian J (2020) Isolation of Efficient Cellulose Decomposer in Sandy Cropland and Its Application in Straw Turnover in Agro-Pasture Ecotone of Northern China. Front. Environ. Sci. 8:528732. doi: 10.3389/fenvs.2020.528732
Received: 13 February 2020; Accepted: 17 August 2020;
Published: 11 September 2020.
Edited by:
Atsushi Tsunekawa, Tottori University, JapanCopyright © 2020 Wang, Zhao, Suvdantsetseg and Lian. This is an open-access article distributed under the terms of the Creative Commons Attribution License (CC BY). The use, distribution or reproduction in other forums is permitted, provided the original author(s) and the copyright owner(s) are credited and that the original publication in this journal is cited, in accordance with accepted academic practice. No use, distribution or reproduction is permitted which does not comply with these terms.
*Correspondence: Shaokun Wang, wangsk@lzb.ac.cn; Xueyong Zhao, zhaoxy@lzb.ac.cn