- 1Departamento De Ciencias Biológicas, Universidad Icesi, Valle del Cauca, Colombia
- 2Centre for Bioinformatics and Photonics-CIBioFi, Universidad del Valle, Cali, Colombia
Plant endophytic bacteria have received special attention in recent decades for their ability to improve plant response to multiple stresses. A positive effect of endophytes on plant’s ability to cope with drought, salinity, nitrogen deficiency, and pathogens have already been demonstrated in numerous studies, and recently this evidence was consolidated in a meta-analysis of published data. Endophytic bacteria have also been implicated in increasing resistance to heavy metals in plants; despite the important biotechnological applications of such effect in heavy metal bioremediation and agriculture, efforts to systematically analyze studies in this field have been limited. In this study, we address this task with the objective of establishing whether the findings made for other types of stresses extend to the response to heavy metals. Specifically, we seek to establish if plant inoculation with plant-growth promoting endophytic bacteria have an impact on their tolerance to heavy metal stress? We carried out a meta-analysis of the effect size of inoculation with endophytic bacteria on the host plant biomass in response to heavy metal stress (aluminum, arsenic, cadmium, copper, chromium, manganese, nickel, lead, and zinc), which included 27 (from 76 published in the last 10 years) studies under controlled conditions that evaluated 19 host plants and 20 bacterial genera. Our results suggest that endophytic bacteria increase the biomass production of host plants subjected to different heavy metals, indicating their effectiveness in protecting plants from a wide range of metal toxicities. Stress mitigation by the bacteria was similar among the different plant groups with the exception of non-accumulating plants that benefit most from the symbiotic association. Host identity and heavy metal concentration seem to influence the effect of the bacteria. Our analysis revealed that bacterial consortia provide the greatest benefit although the most common biotechnological applications are not directed towards them, and support the value of endophytic bacteria as an alternative to mitigate heavy metal stress in a wide variety of hosts.
Introduction
Plants are constantly affected by different types of stress, both biotic and abiotic, which induce an alteration in their metabolism and functioning, and consequently reduce their productivity, generating large losses in crop yields (Rejeb et al., 2014). Abiotic stress refers to the pressures exerted by the environment that alter plant physiology, and negatively impact the development and productivity of plants. It is estimated that reductions in growth and yield can exceed 50 and 70%, respectively, in most plant species, severely affecting all crops (Wang et al., 2003). Major types of abiotic stress include drought, salinity, and heavy metal toxicity.
Heavy metals such as zinc, copper, molybdenum, manganese, cobalt, and nickel are naturally found in soils and are essential for the functioning of important biological processes. However, these elements in combination with more toxic heavy metals (arsenic, lead, cadmium, mercury, chromium, aluminum, and beryllium) can reduce crop productivity when their concentrations exceed optimal values for plant functioning, causing morphological abnormalities and metabolic disorders that increase the production of reactive oxygen species (Tiwari and Lata, 2018). Both essential and non-essential metals, when present at phytotoxic concentrations, generate common adverse effects such as chlorosis, growth inhibition, reduced photosynthesis, low biomass accumulation, altered water balance, senescence and ultimately plant death (Singh et al., 2016). Although the availability of heavy metals depends largely on soil characteristics, their accumulation is favored by the use of agrochemicals (Mustafa and Komatsu, 2016), hence the need for a transition to more sustainable agricultural practices. Besides, human activities and industrialization in recent decades have led to an excessive release of these elements into the environment, so rapidly that plants face pressure to develop mechanisms to cope with their phytotoxicity.
Therefore, the responses and tolerance mechanisms of plants to this type of stress have been extensively studied. Using tools such as omic technologies (proteomics, transcriptomics, metabolomics, and iolomics) it has been possible to identify several genes involved in the response to heavy metals in several plant species (Singh et al., 2016). The presence of heavy metals activates several signaling pathways, such as the reactive oxygen species (ROS) pathway and hormone signaling pathways that enhance the expression of stress response genes (Kumar and Trivedi, 2015). These findings have been used to develop heavy metal resistant genotypes through classical genetic improvement or genetic engineering; however, these processes are labor and time intensive (Tiwari and Lata, 2018). New strategies need to be developed to increase tolerance to heavy metals in crops so that their performance is not strongly affected. The association of plants with various microorganisms (fungi, yeasts, and bacteria) represents a sustainable strategy to cope with this type of stress. Plant-bacteria interaction is a natural and complex phenomenon involving different biotic, abiotic, and genetic factors that have been studied for decades. Understanding the effect of this association is of great interest due to applications at the agricultural level. In recent years, a diversity of efficient microorganisms have been identified, including endophytic bacteria, rhizobacteria, fungi, and others, capable of promoting the growth of the plants with which they are associated, even under conditions of abiotic and biotic stress (Tanya Morocho and Leiva-Mora, 2019).
Endophytic bacteria are then proposed as a sustainable and effective alternative to promote the growth of crop species and increase stress tolerance. This type of bacteria is characterized by residing most of its life cycle inside the plant tissues in an asymptomatic way without causing damage to its host; although variable among bacterial species, endophytic bacteria tend to form small aggregates dispersed in the tissues/organs of the plant where the exchange of nutrients occurs, promoting the metabolic activity of the microorganism and, in turn, the physiological activities of the host plant (Perez et al., 2013). These microorganisms can be associated with a wide range of plants in a very specific (specialist) or general (generalist) way and transmitted to the next generations (Johnston and Raizada, 2011). Endophytic bacteria can be diazotrophic organisms, that is, biological fixers of atmospheric nitrogen (N2) that convert this element into more available forms for the host plant (ammonia), thus benefiting its productivity (Hernández-Rodríguez et al., 2017). In addition to nitrogen fixation, endophyte bacteria can promote plant growth and improve stress response and disease resistance through various mechanisms including mineral solubilization, phytohormone synthesis, and production of siderophores (metal-chelating substances) (Ahemad and Khan, 2012).
Previous studies have confirmed the plant growth-promoting ability of endophytic bacteria (termed PGPB) in various crop species (Yan et al., 2018; Zhu and She, 2018). These bacteria have been found to increase plant growth under salinity or heavy metals stress and can maintain their metabolic activity (Tirry et al., 2018; Soldan et al., 2019). In Arabidopsis thaliana, it was determined that the association with PGBPs induced the overexpression of genes related to aluminum stress, so the plant-bacteria association improves the tolerance mechanisms exhibited by the plant (Farh et al., 2017). Due to this, there is a growing interest in the use of these microorganisms in agriculture and bioremediation. Currently, a great deal of information has been generated concerning the positive effects that endophytic bacteria have on plant tolerance to various types of stress and their biomass.
Stress tolerance mediated by endophytic bacteria is considered the most effective because it is environmentally sustainable, low cost, and socially well accepted (Singh et al., 2016). Bacteria have adapted well to environments polluted with heavy metals and the resistance mechanisms they exhibit include bioaccumulation and enzymatic reduction or oxidation to non-toxic forms (Tiwari and Lata, 2018). In addition to these mechanisms, some microorganisms have been described as promoting the metal accumulation in plants and enhancing the expression of stress-related genes (Srivastava et al., 2013). Also, the alteration of the levels of 1-aminocyclopropane-1-carboxylate (ACC) by various bacteria can affect heavy metal tolerance directly through the manipulation of ethylene levels in plants (Zhang et al., 2011).
Similar conclusions have been reached for other types of stress such as drought and salinity (Sukweenadhi et al., 2015). Rho et al. (2018) through a meta-analysis of effect size, found that several endophytes (bacteria, fungi, and yeasts) do indeed have a positive effect on a wide range of plant species under different types of stress, particularly drought, salinity, and nitrogen deficiency. This same type of analysis has been previously carried out for specific endophyte species, such as the dark septum fungi (DSE) as promoters of tolerance to various types of stress (Newsham, 2011). These meta-analyses, which have considered a great diversity of variables and types of stress, take data from different experimental studies to be analyzed collectively with statistical methods and allow determining the magnitude of response to a treatment (Rosenberg et al., 2000). However, the efficacy of endophytic bacteria to mitigate the adverse effects of heavy metals has not been studied at this level, nor has its specificity, so there is a gap in knowledge in this area. Therefore, this study sought to determine if the findings made by Rho et al. (2018) extend to heavy metal tolerance, specifically our goal was to determine if endophytic bacteria are a real and robust alternative for increasing heavy metal stress tolerance. To this end, research data related to heavy metal tolerance mediated by endophytic bacteria from the last 10 years were collected and an effect size analysis was performed. The results obtained show an overall positive effect of inoculation with endophyte bacteria on the growth and tolerance to heavy metals in different plant species, although some influence of the type of host plant (hyperaccumulator vs. non-hyperaccumulator) and the heavy metal identity was evidenced.
Materials and Methods
Methodology implemented in this study is an adaption from Rho et al. (2018).
Data Collection
We carried out a search of scientific literature related with plant growth-promoting endophytic bacteria that increase tolerance to heavy metal stress, following PICO’s framework (Supplementary Table S1; Miller and Forrest (2001) and PRISMA checklist (Supplementary Table S2; Moher et al., 2009). The keywords used in the search were “endophytic bacteria,” “heavy metal stress” and “plant growth promotion.” The different heavy metals (cadmium, lead, zinc, aluminum, etc.) were also added as independent terms, while the words “fungi” and “fungal” were used as exclusion terms. In addition, selection filters were applied to publication period time (2010–2020) and article type (Research article). The time period was selected considering that it includes the largest production of relevant articles in this topic, as shown by robust reviews that have addressed the topic in a descriptive way (Zaets and Kozyrovska, 2012; Ma et al., 2016; White et al., 2019). We retrieved a total of 76 articles from the specialized databases of International Acknowledgment Springer Link (www.link.springer.com/), SCOPUS (www.scopus.com), Science Direct (www.sciencedirect.com), and PubMed (www.ncbi.nlm.nih.gov/pubmed/).
Figure 1 depicts the flow of articles, exclusions, and the reasons for exclusion (PRISMA Flowchart, Liberati et al., 2009). The following inclusion criteria were used to further screen the collected articles:
i. Studies had to report the standard deviations (SD) or standard errors (SE), as those values were required to perform the meta-analysis.
ii. Studies had to report experiments under controlled environments—a lab, growth chamber, or greenhouse environment, to be sure that the responses of the plants are due to the inoculation without the influence of other factors present in the uncontrolled environments.
iii. Studies had to report an experimental design that included control and inoculated groups grown under stress and non-stress conditions. This to make the results comparable, and estimate the effect size.
iv. Studies had to report biomass data, particularly dry weight (DW). Biomass was considered proxy of plant performance.
v. Studies had to be published as full research articles in indexed journals (grey literature was not considered).
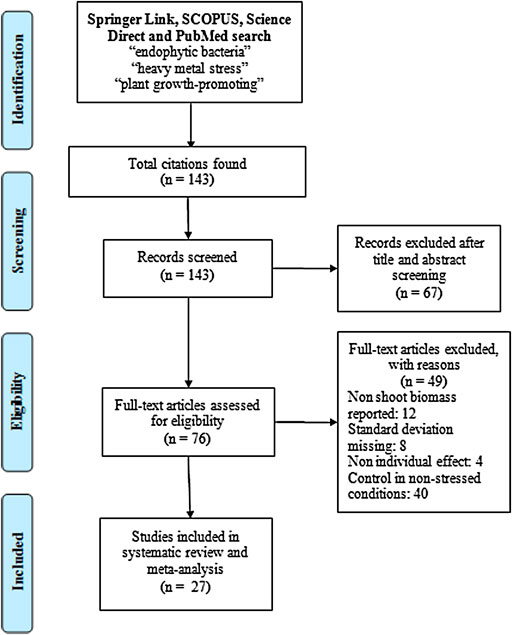
FIGURE 1. PRISMA (Preferred Reporting Items for Systematic Reviews and Meta-Analyses) flowchart showing the selection of studies eligible for a meta-analysis of the effect size of inoculation with endophytic bacteria on the host plant biomass in response to heavy metal stress (aluminum, arsenic, cadmium, copper, chromium, manganese, nickel, lead, and zinc).
Each combination of an endophytic bacteria strain, a plant species and a heavy metal in one article was regarded as one data set to be analyzed. Graphical data in figures were extracted using ImageJ v.1.52 with the “Figure Calibration” plugin package (Schneider et al., 2012).
Conversions of concentration and biomass units were realized for make results from different articles comparable. Metal concentration unit were fixed in millimoles (mM) and biomass unit were grams (g). All information (data from tables and figures) was collected and arranged in an Excel spreadsheet.
Estimation of Effect Size
Inoculation with endophytic bacteria was counted as a fixed effect in different environmental and experimental conditions; thus, a fixed-effect model was implemented for analyzing the data. The mean values, standard deviations (SDs), standard errors (SEs) and the number of replicates were additionally processed to be imported to the R platform v. 3.6.3 (R Core Team, 2020) to execute the statistical analysis. Shoot biomass of host plants was set as the response variable. The following formula was used to calculate Hedges (d)—non-biased and scaled differences addressing sample sizes of datasets (Crawley, 2007):
where
The variance of d (Vd) was calculated with the next Eq. 3:
The bias-corrected versions of Hedge’s mean differences and their variances—g and Vg—were calculated by simply multiplying J and J2 to d and Vd. Calculated Vg was used in the estimation of 95% confidence intervals (CIs) of each g (Eq. 4). These weighted measures correct the bias that could affect the effect size estimates derived from the different sample sizes in individual studies.
The reciprocals of Vds were used as the weights (W = 1/Vg) for determining the summary fixed effects. The sum of the products of the weights and the effects (WY = W × g) was divided by the sum of the weights to finally determine the summary effect (M) as follows:
The variance of the summary effect (VM) is just the reciprocal of ∑W.
Standard errors of M (SEM) is,
Finally, the sum of W was used to calculate SE of the mean summary effects to further compute the z-test statistic (z = M/SEM). In the cases where the effect size was found to be significant at α = 0.05, we calculated the fail-safe number (nfs) to estimate the publication bias using the “metafor” package in R (Viechtbauer, 2010). If nfs is above 5n + 10, is safe ignore publication bias like is described in Rosenberg et al. (2000), where n is the number of studies analyzed.
The effects were divided in sub-categories according to the type of host plant used in the experiments. Group 1 compared the effect on accumulating vs. non-accumulating species, following by group 2 that compared the effect on host vs. non-host plants. Group 3 compared the effect on crop vs. non-crop plants. The effect sizes without stress and vs. stress were also compared using a paired t-test procedure in the R platform. To investigate the effect of endophytic bacteria on host plants under stress for high toxicity heavy metals, a heat map showing the combined measures of the selected three most toxic heavy metals cadmium, arsenic and lead was constructed.
Results
General Information
Because our study was limited to the last 10 years, we cannot infer the year in which the trend of investigating the effect of endophytic bacteria on plant tolerance to heavy metal stress began. The trend presented in Figure 2 shows that the number of studies focused on this area has increased in the last 4 years; the decrease observed in 2020 is explained by the fact that it is the current year. According to this trend, by the end of this year we would expect to have a greater number of articles than those reported for 2019.
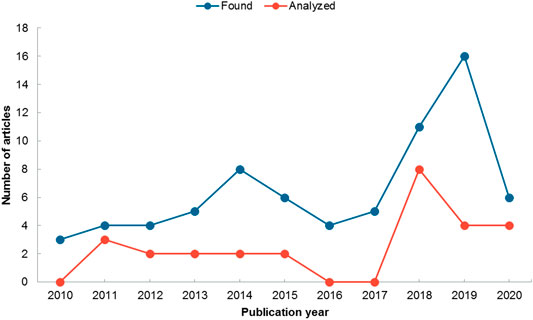
FIGURE 2. Number of articles published per year on endophytic bacteria and heavy metal tolerance. Blue line shows articles found in the databases (n = 76) and red line articles that were included in the analysis (n = 27).
Of the 76 articles found in the databases, only 27 were used to carry out the meta-analysis (Supplementary Table S3) because the rest did not meet the requirements established in the methodology. The most astringent selection filter of the articles was the experimental design, since it was established that the studies should include a control group in a non-stressful condition. In addition, some of the articles did not contain information on the standard deviation or did not have results related to the plant biomass (dry weight), since they focused on the evaluation of the activity of several genes and enzymes involved in tolerance to heavy metals (Supplementary Table S4).
The inoculum density used in the studies varied between 1 × 104 – 1 × 1012 CFU/ml, with most studies reporting a density of 1 × 108 CFU/ml (Table 1). None of the articles reported symptoms of pathogenicity in host plants due to inoculum application. The most widely used strain in the studies was Sphingomonas SAMR12 (n = 4), although it is not the most tested bacterial genus or the strain that conferred the greatest benefit.
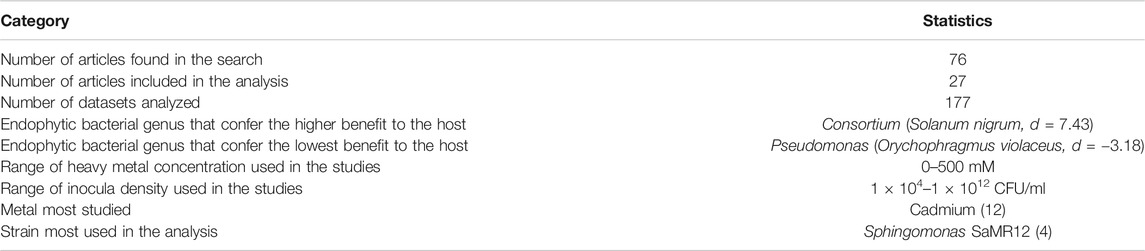
TABLE 1. General statistics of articles found in the databases about the effect of endophytic bacteria on plant heavy metal tolerance.
In our meta-analysis we included studies that evaluated a variety of heavy metals with phytotoxic effect; the most studied metal was Cadmium (37%), followed by Zinc (14%), Copper and Lead (11%) (Figure 3A). None of the articles analyzed evaluated the simultaneous contamination of soil by several heavy metals—although some evaluated the individual application of different elements in the same experiment. The metals were applied at concentrations in the range of 0–500 mM (Table 1).
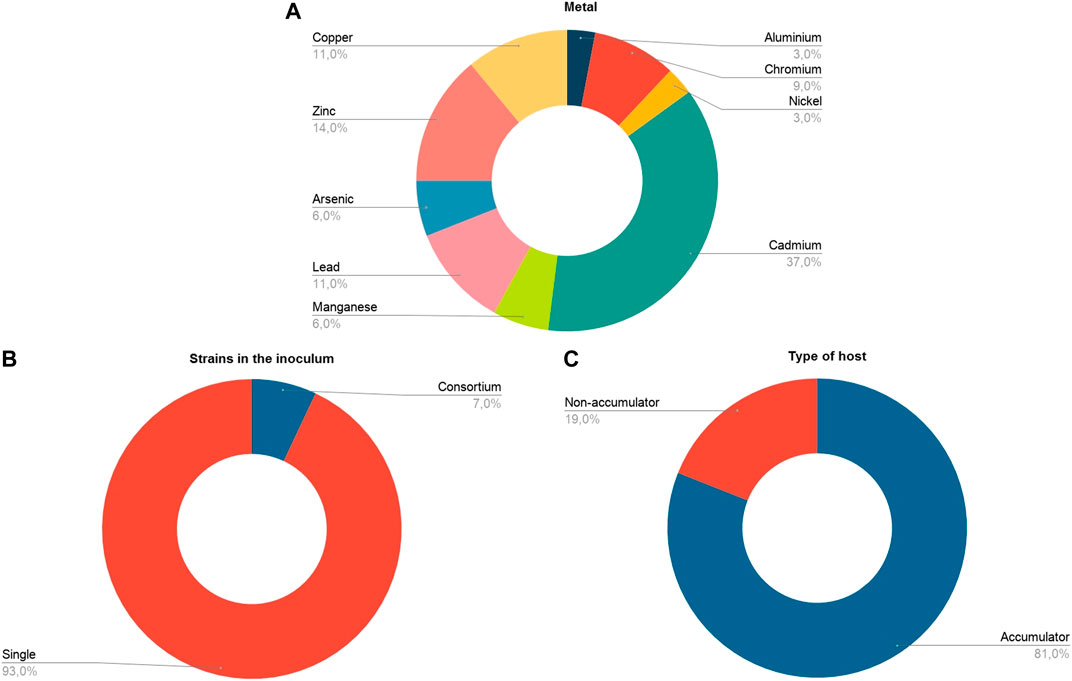
FIGURE 3. General information about the studies included in the analysis (n = 27) for (A) Type of metal. (B) Strains in the inoculum. (C) Host type in terms of its metal accumulation capacity.
The choice of the host plant in the studies was inclined towards plants with innate capacity to accumulate heavy metals (hyperaccumulators), since the non-hyperaccumulating plants corresponded only to 19% of the sample, compared to 81% corresponding to the hyperaccumulating plants (Figure 2C). A marked bias was also observed with respect to the number of strains used in the inoculum; very few studies evaluated the effect of bacterial consortia (7%, n = 2) (Figure 3B). In general, the tendency has been to evaluate the effect of a single bacterial species.
As for the data groups that used only one bacterial strain (n = 170), 20 bacterial genera were evaluated, being Enterobacter (21%) and Bacillus (12%) the most investigated genera. Figure 4 shows the seven genera that were studied in more than one data set. The category “Others,” which represents 30%, includes the remaining 13 genera among which are Mesorhizobium, Methylobacterium, Acinetobacter, Ralstonia, Agrobacterium, etc., which were only included in one data set (n = 1). Interestingly, although the genus Enterobacter stands out as the most representative, there are no indications that it confers greater benefits to the host (Table 1).
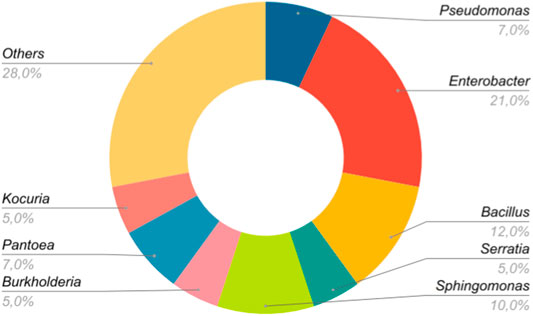
FIGURE 4. Most representative bacterial genera used in the experiments. The genera used in only one study (n = 1) were grouped in the category “Others.”
Effect Size
In general, there is a significant and positive effect of inoculation with endophyte bacteria on the biomass of host plants under both stressed (d = 1,179) and non-stress conditions (d = 1,043). It was determined that for the analysis there is no publication bias. The fail-safe number obtained was 10,866, quite high compared to the criterion (145). For all groups the effect size values were above zero (Figure 5), which supports the hypothesis that endophytic bacteria are able to promote metal stress tolerance in a wide variety of plants.
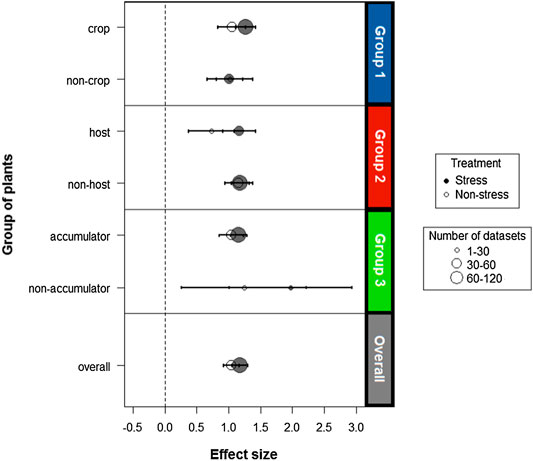
FIGURE 5. Analysis of the size of the cumulative effect under stress caused by different metals in the functional plant groups. The open and closed symbols present the effect sizes of endophytes on host plants in the absence and presence of stress, respectively. The size of the symbols indicates the number of combined studies to calculate the measurements. The overall summary effect sizes without (n = 54) and with stress (n = 123) are presented in gray. The horizontal error bars represent the ±95% confidence intervals (CIs). If the CIs do not cross the vertical dotted lines (d = 0), the effect size for a combination of a certain functional group is significant at p < 0.05.
For all three groups of plants the response to inoculation differed between stress and non-stress treatments. There was a general trend towards a larger effect size in the stressed plants compared to the non-stressed plants. However, there is an exception for non-cultivable plants (d = 1,019 and d = 1,015) and non-host plants (d = 1,182 and d = 1,159) whose results do not differ between plants exposed to heavy metals and control plants. Hence, an overlap of effect size points for these groups is shown in Figure 5.
Although a similar effect size value was obtained for all plant groups, non-accumulating plants show a better performance under heavy metal stress conditions compared to the other groups. This group, in spite of having a reduced sample size (n = 5), reports the highest effect size (d = 1,972 and d = 1,243 for the stress and control treatments, respectively), a value that exceeds that of the other groups and the total sample (Figure 5).
In relation to the three most toxic heavy metals, it was found that 13 of the 19 plant species and 17 of the 20 bacterial genera have been evaluated for these metals, covering most of the research conducted in this area. The species Brassica napus and Solanum nigrum are those in which the effect of more than one bacterial genus has been evaluated on tolerance to more than one of the three heavy metals analyzed, as shown in Figure 6. Of the 35 combinations plotted, six had a negative effect on the plant host.
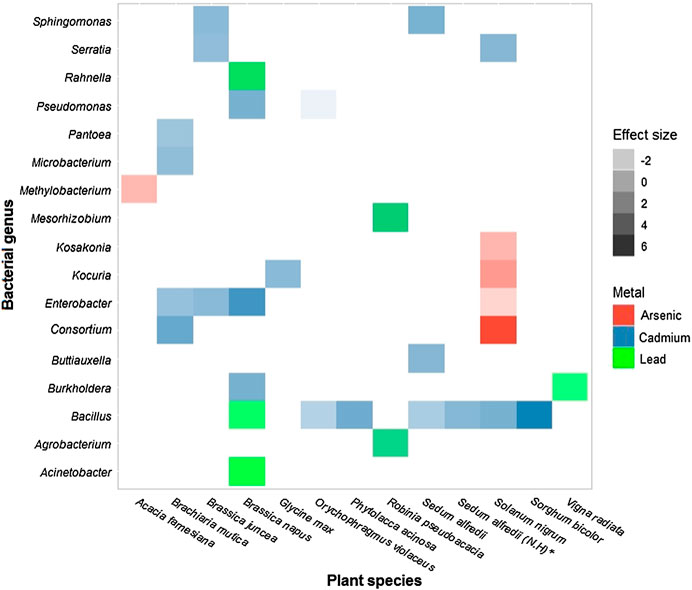
FIGURE 6. Size of the effect of the bacterial genus on plant species under stress from the three most toxic metals: Cadmium (
A version of Figure 6 that covers all the host plants and bacterial genera used in the studies included in the analysis, without discriminating by the type of metal used as a stress agent, only includes 43 combinations of the 380 possible combinations (19 × 20 = 380), indicating that only 11% of the combinations have been described in the literature (Supplementary Figure S1). The maximum value of effect size was obtained by the combination Consortium-Solanum nigrum under stress by Arsenic (d = 6.05) and the minimum effect size was obtained by the combination of Pseudomonas and Orychophragmus violaceus in the presence of Cadmium (d = −3.18) (Figure 6). This coincides with what is reported in Table 1 for the genera that confer the greatest and least benefit to the host.
Discussion
General Statistical Information
The publication of articles related to the positive effects of endophytic bacteria on plant growth and plant tolerance to heavy metal toxicity has a tendency to increase over time, demonstrating the growing interest in this topic. The trend observed in Figure 2 coincides with that found by Rho et al. (2018) for other types of stress, and is expected to continue in the coming years. Thus reflecting the recognition of endophytic bacteria as a promising alternative to replace traditional cultivation practices, while improving soil properties and plant productivity (Tiwari and Lata, 2018).
Although the trend is for more articles to be published on this topic, many of the studies published to date do not have complete experimental designs. As can be seen in Figure 1 and Supplementary Table S4, about 40 articles were discarded because they did not include an adequate control to compare against stress conditions. The lack of this information limits the analysis and understanding of the real impact of the research, since the evaluation of the effect of endophytic bacteria on host plants under stress and non-stress conditions allows a correct comparison and estimation of the size of the inoculation effect. The estimation of the effect size allows a better interpretation of the results, in terms of efficacy, that a certain treatment has under different conditions (Coe and Soto, 2003).
Interestingly, there are not many studies evaluating the effect of bacterial consortia on host plant response to heavy metals. This is an important difference from the findings of Rho et al. (2018), who reported a preponderance of studies of bacterial and fungal consortia, based on the hypothesis that they exhibit a positive synergistic effect. This trend has not been followed for heavy metals (Figure 3B). However, it should be noted that our study focused on the effect of endophytic bacteria, and therefore articles evaluating the effect of bacteria-fungal consortia were not included in the analysis.
The most studied heavy metal in the different works was cadmium (Figure 3A). According to Gill and Tuteja (2011) this is because cadmium is considered one of the most toxic heavy metals due to its high solubility in water and because low concentrations are required to generate harmful effects on plants. In addition, this element has toxic effects on human health and yet it is commonly released into the environment as a result of industrial activities (Tiwari and Lata, 2018). Although several metals were used in the articles analysed, others, such as mercury and beryllium, have been little studied, probably because they have similar effects to other elements and are of a rare nature.
Most of the studies conducted focused on phytoremediation and the use of endophytic bacteria as enhancers of heavy metal accumulation or removal. Because of this, in the analysis we found a tendency to use plants hyper accumulating heavy metals. Figure 3C shows that only 19% of the host plants used in the experiments are non-accumulating plants. This bias is due to the fact that some of the heavy metal hyper accumulating plants produce little biomass and exhibit slow growth (Rajkumar et al., 2009). Therefore, the experimental approach has been to increase the effectiveness of phytoremediation of these plants using either endophyte bacteria capable of promoting plant growth and/or increasing metal tolerance.
Nevertheless, it is important to understand whether the effects on plants with an innate ability to accumulate metals can be extrapolated to plants that do not accumulate heavy metals, which require even more of the protective functions that endophytic bacteria have against heavy metals. Few studies have used non-accumulating host plants and therefore a knowledge gap exists, opening opportunities for further research. Just as it is important to improve the ability to accumulate metals in hyper accumulating plant species, it is also necessary to increase the tolerance of non-accumulating plants of agronomic interest in order to expand the areas of cultivation to potentially arable land strongly affected by the presence of heavy metals.
Effect Size
The results obtained in our meta-analysis support the hypothesis that endophytic bacteria provide tolerance to heavy metals in host plants, regardless of the identity of the heavy metal, a finding consistent with that reported by Rho et al. (2018) and Larimer et al. (2010). Also, the measurement of the effect of inoculation obtained when comparing growth under stress and non-stress conditions was higher than 0, indicating that endophytic bacteria are indeed able to maintain their functionality and provide benefits to their host (Figure 5). However, 16 data sets corresponding to 9% of the total analyzed reported a detrimental effect on the host (Figure 6; Supplementary Table S5).
According to Nadeem et al. (2014), some endophytes have a negative effect on their host as a result of overproduction of auxins and other metabolic derivatives. However, 10 of the 16 data sets that reported a detrimental effect on the plant represented combinations of bacterial strains inoculated into plants other than their natural hosts. Mayerhofer et al. (2013) made a similar finding regarding fungal endophytes, describing that their positive effect may be host-specific. Interestingly, in our meta-analysis we found that the same combinations of bacteria that had an effect size value below 0 did not cause a detrimental effect when evaluated at higher or lower concentrations of heavy metals (Supplementary Table S5). These results suggest that the benefits provide by endophytic bacteria may be host-specific, and also depend on the intensity of the stress faced. In the case of drought, salinity, and nitrogen deficiency, low host specificity was established by Rho et al. (2018), that compared with the results obtained indicates that plant-bacteria interaction in the context of heavy metal stress may be more specific compared to the types of stress evaluated by Rho et al. (2018).
Despite the above, the efficacy of endophytic bacteria to increase tolerance to heavy metal stress—although host specific-is corroborated considering the low percentage of data with negative effect size and the evidence that they were able to promote the growth of the various groups of plants both under stress and without it (Figure 5). There is ample evidence that the beneficial effect of endophytic bacteria on their host plants is mediated by various mechanisms including the production of auxins, siderophores and other nutrient solubilizing compounds, increased activity of the enzyme ACC deaminase and even changes in root morphology (Sessitsch et al., 2013; Tiwari and Lata, 2018). Furthermore, Sessitsch et al. (2013) suggest that an increase in biomass could be directly associated with an increase in phytoextraction efficiency and stress tolerance to heavy metals.
Although the general trend is to investigate bacterial effect on accumulating plants, it is the non-accumulating plants that benefit most from the interaction as shown in Figure 5. Non-accumulating plants are naturally more affected when they are subjected to stress, so the benefits of plant-bacteria interaction are more evident in these organisms. Lindblom et al. (2014) found that the non-accumulating specie showed positive and significant growth response to bacterial inoculation compared to the accumulating specie that did not respond. It has also been described that non-accumulating plants tend to have a higher growth potential (Chandra and Kumar, 2018), which explains their higher growth response compared to accumulating plants. The results show the importance of research on non-accumulating plants response to inoculation with endophytes.
We found that Brassica napus and Solanum nigrum are the species most studied for the three most toxic heavy metals (Figure 6). Both are model species for hyperaccumulators due to their short growth cycle and abundant biomass, so there is a wealth of information about their tolerance level and defense mechanisms against metal stress (Mourato et al., 2015; Shi et al., 2016). Also, Brassica napus is an edible plant that for its phytoextraction capacity, has been useful to understand metal contamination in the food chain (Mourato et al., 2015).
Figure 6 confirms the trend to study metal of high toxicity and with adverse effects on human health. More than half of the host plants and bacterial genera included in our meta-analysis have been evaluated for the metals cadmium, arsenic and lead, which are the three most toxic of the nine heavy metals analyzed (Figure 5). But, it is necessary to continue expanding the literature related to this field since, as shown in Supplementary Figure S1, less than 15% of the possible combinations of plant-endophytic bacteria have been studied.
The maximum value of the effect size was for a combination that integrated a bacterial consortium (Table 1; Figure 6). This supports the hypothesis raised in other meta-analyses (Larimer et al., 2010; Rho et al., 2018), which proposes that the interaction of several symbionts/variants maximizes the benefits, so it would be advisable to direct the research towards it. For this reason, although there has been a clear tendency not to evaluate the effect of bacterial consortia (Figure 3B), already described previously by other authors (Sessitsch et al., 2013), the results suggest that it should be modified over time.
In conclusion, our study supports that endophytic bacteria are a sound alternative for increasing heavy metal stress tolerance, regardless of the metal used as a stress agent and with low host specificity. Of course, there are exceptions that should not be overlooked and require further studies, for example, some bacterial strains have a detrimental effect on plant growth or their effect is host-specific and dose-dependent. Our meta-analysis showed a strong tendency to evaluate 1) single-strain inoculum and 2) hyper-accumulating plants as hosts. However, we found clear evidence that bacterial consortia maximize benefits compared to individual strains and that non-accumulating plant species gain greater advantages from the symbiotic association, so the trends should change. On the other hand, it is of great importance to continue research in this area since only 11% of the possible plant-bacteria combinations have been reported in the literature. At the same time, most of the articles lack complete experimental designs which make difficult the analysis of the information. Other variables other than biomass should be investigated because, although it is an indicator of stress tolerance and is directly related to accumulation capacity, there is currently a great deal of information related to the effects of bacteria on gene expression and on the enzymatic function of the plant to be exploited. Finally, the positive effect of bacteria determined in this document may be limited by the restriction to studies conducted under controlled conditions, which avoids estimating the precise impact on natural systems.
Data Availability Statement
The original contributions presented in the study are included in the article/Supplementary Material, further inquiries can be directed to the corresponding author.
Author Contributions
TG-H: conceived the idea and contribute to the writing of the manuscript. VF-F: performed the data collection, statistical analysis and was the major contributor in writing the manuscript. TG-H and SM-R: revised the manuscript and gave guidance throughout the process of this study. All authors read and approved the summited and final version of the manuscript to be published.
Funding
SM-R master studies were financially supported by a grant from Universidad Icesi and the CIBioFi Project, funded by the Colombian Science, Technology and Innovation Fund-General Royalties System (Fondo CTeI-SGR), Gobernación del Valle del Cauca, and COLCIENCIAS, under contract No. BPIN 2013000100007.
Conflict of Interest
The authors declare that the research was conducted in the absence of any commercial or financial relationships that could be construed as a potential conflict of interest.
Acknowledgments
This is a short text to acknowledge the contributions of specific colleagues, institutions, or agencies that aided the efforts of the authors.
Supplementary Material
The Supplementary Material for this article can be found online at: https://www.frontiersin.org/articles/10.3389/fenvs.2020.603668/full#supplementary-material.
References
Ahemad, M., and Khan, M. S. (2012). Alleviation of fungicide-induced phytotoxicity in greengram [Vigna radiata (L.) Wilczek] using fungicide-tolerant and plant growth promoting pseudomonas strain. Saudi J. Biol. Sci. 19 (4), 451–459. doi:10.1016/j.sjbs.2012.06.003
Chandra, R., and Kumar, V. (2018). “Hyperaccumulator versus nonhyperaccumulator plants for environmental waste management,” in Phytoremediation of environmental pollutants. 1st Edn, Editors R. Chandra, N. K. Dubey, and V. Kumar (Boca Raton, FL: CRC Press), 43–80. Available at:https://www.researchgate.net/publication/321463234_Hyperaccumulator_versus_Nonhyperaccumulator_Plants_for_Environmental_Waste_Management (Accessed May 27, 2020).
Coe, R., and Soto, C. (2003). Magnitud del efecto: Una Guía para investigadores y usuarios. Rev. Psicol. 21 (1), 145–177. doi:10.18800/psico.200301.006
Farh, M. E., Kim, Y. J., Sukweenadhi, J., Singh, P., and Yang, D. C. (2017). Aluminium resistant, plant growth promoting bacteria induce overexpression of aluminium stress related genes in arabidopsis thaliana and increase the ginseng tolerance against aluminium stress. Microbiol. Res. 200, 45–52. doi:10.1016/j.micres.2017.04.004
Gill, S. S., and Tuteja, N. (2011). Cadmium stress tolerance in crop plants. Plant Signal. Behav. 6 (2), 215–222. doi:10.4161/psb.6.2.14880
Hernández-Rodríguez, A., Rives-Rodríguez, N., Díaz-de la Osa, A., de la Fe-Pérez, Y., Pijeira-Fernández, G., and Divan-Baldani, V. L. (2017). Caracterización de bacterias diazotróficas asociativas con actividad promotora del crecimiento vegetal en Oryza sativa L. Rev. Cubana Cienc. Biol. 5 (2), 1–9.
Johnston, D., and Raizada, M. N. (2011). “Plant and endophyte relationships: nutrient management,” in Comprehensive biotechnology. 2nd Edn, Editor M. Moo-Young (Saint Louis: Elsevier), 713–727. Available at: https://www.researchgate.net/profile/David_Johnston-Monje/publication/215674365_Plant_and_Endophyte_Relationships/links/5a96b2c6a6fdccecff0a2a98/Plant-and-Endophyte-Relationships.pdf (Accessed October 15, 2019).
Kumar, S., and Trivedi, P. K. (2015). “Heavy metal stress signaling in plants,” in Plant metal interaction: Emerging remediation techniques. Amsterdam: Elsevier Inc., 585–603.
Larimer, A. L., Bever, J. D., and Clay, K. (2010). The interactive effects of plant microbial symbionts: a review and meta-analysis. Symbiosis 51 (2), 139–148. doi:10.1007/s13199-010-0083-1
Liberati, A., Altman, D. G., Tetzlaff, J., Mulrow, C., Gøtzsche, P. C., Ioannidis, J. P., et al. (2009). The PRISMA statement for reporting systematic reviews and meta-analyses of studies that evaluate health care interventions: explanation and elaboration. J. Clin. Epidemiol. 62, e1. doi:10.1136/bmj.b270010.1016/j.jclinepi.2009.06.006
Lindblom, S. D., Fakra, S. C., Landon, J., Schulz, P., Tracy, B., Pilon-Smits, E. A., et al. (2014). Inoculation of selenium hyperaccumulator Stanleya pinnata and related non-accumulator Stanleya elata with hyperaccumulator rhizosphere fungi--investigation of effects on Se accumulation and speciation. Physiol. Plantarum 150 (1), 107–118. doi:10.1111/ppl.12094
Ma, Y., Oliveira, R. S., Freitas, H., and Zhang, C. (2016). Biochemical and molecular mechanisms of plant-microbe-metal interactions: relevance for phytoremediation. Front. Plant Sci. 7, 918. doi:10.3389/fpls.2016.00918
Mayerhofer, M. S., Kernaghan, G., and Harper, K. A. (2013). The effects of fungal root endophytes on plant growth: a meta-analysis. Mycorrhiza 23 (2), 119–128. doi:10.1007/s00572-012-0456-9
Miller, S. A., and Forrest, J. L. (2001). Enhancing your practice through evidence-based decision making: PICO, learning how to ask good questions. J. Evid. Base Dent. Pract. 1, 136–141. doi:10.1016/S1532-3382(01)70024-3
Moher, D., Liberati, A., Tetzlaff, J., and Altman, D. G. (2009). Preferred reporting items for systematic reviews and meta-analyses: the PRISMA statement. PLoS Med. 6 (7), e1000097. doi:10.1371/journal.pmed.1000097
Mourato, M. P., Moreira, I. N., Leitão, I., Pinto, F. R., Sales, J. R., and Martins, L. L. (2015). Effect of heavy metals in plants of the genus Brassica. Int. J. Mol. Sci. 16, 17975. doi:10.3390/ijms160817975
Mustafa, G., and Komatsu, S. (2016). Toxicity of heavy metals and metal-containing nanoparticles on plants. Biochim. Biophys. Acta 1864 (8), 932–944. doi:10.1016/j.bbapap.2016.02.020
Nadeem, S. M., Ahmad, M., Zahir, Z. A., Javaid, A., and Ashraf, M. (2014). The role of mycorrhizae and Plant Growth Promoting Rhizobacteria (PGPR) in improving crop productivity under stressful environments. Biotechnol. Adv. 32, 429–448. doi:10.1016/j.biotechadv.2013.12.005
Newsham, K. K. (2011). A meta-analysis of plant responses to dark septate root endophytes. New Phytol. 190 (3), 783–793. doi:10.1111/j.1469-8137.2010.03611.x
Perez, C. A., Perez, C. C., and Chamorro, A. L. (2013). Diversidad de bacterias endofitas asociadas a cultivo de arroz en el departamento de cordoba-colombia. Estudio preliminar. Rev. Colombiana Cienc. Anim. 5 (1), 83–92. doi:10.24188/recia.v5.n1.2013.473
R Core Team (2020). R: a language and environment for statistical computing. Vienna, Austria: R Foundation for Statistical Computing. Available at: www.R-project.org.
Rajkumar, M., Ae, N., and Freitas, H. (2009). Endophytic bacteria and their potential to enhance heavy metal phytoextraction. Chemosphere, 77, 153. doi:10.1016/j.chemosphere.2009.06.047
Rejeb, I. B., Pastor, V., and Mauch-Mani, B. (2014). Plant responses to simultaneous biotic and abiotic stress: molecular mechanisms. Plants (Basel) 3, 458. doi:10.3390/plants3040458
Rho, H., Hsieh, M., Kandel, S. L., Cantillo, J., Doty, S. L., and Kim, S. H. (2018). Do endophytes promote growth of host plants under stress? A meta-analysis on plant stress mitigation by endophytes. Microb. Ecol. 75 (2), 407–418. doi:10.1007/s00248-017-1054-3
Rosenberg, M. S., Adams, D. C., and Gurevitch, J. (2000). MetaWin: statistical software for metanalysis. Version 2. Sunderland, MA: Sinauer Associates.
Schneider, C. A., Rasband, W. S., and Eliceiri, K. W. (2012). NIH image to ImageJ: 25 years of image analysis. Nat. Methods 9, 671. doi:10.1038/nmeth.2089
Sessitsch, A., Kuffner, M., Kidd, P., Vangronsveld, J., Wenzel, W. W., Fallmann, K., et al. (2013). The role of plant-associated bacteria in the mobilization and phytoextraction of trace elements in contaminated soils. Soil Biol. Biochem. 60, 182. doi:10.1016/j.soilbio.2013.01.012
Shi, P., Zhu, K., Zhang, Y., and Chai, T. (2016). Growth and cadmium accumulation of Solanum nigrum L. seedling were enhanced by heavy metal-tolerant strains of Pseudomonas aeruginosa. Water Air Soil Pollut. 227 (12), 1–11. doi:10.1007/s11270-016-3167-6
Singh, S., Parihar, P., Singh, R., Singh, V. P., and Prasad, S. M. (2016). Heavy metal tolerance in plants: role of transcriptomics, proteomics, metabolomics, and ionomics. Front. Plant Sci. 6, 1143. doi:10.3389/fpls.2015.01143
Soldan, R., Mapelli, F., Crotti, E., Schnell, S., Daffonchio, D., Marasco, R., et al. (2019). Bacterial endophytes of mangrove propagules elicit early establishment of the natural host and promote growth of cereal crops under salt stress. Microbiol. Res. 223–225, 33–43. doi:10.1016/j.micres.2019.03.008
Srivastava, S., Verma, P. C., Chaudhry, V., Singh, N., Abhilash, P. C., Kumar, K. V., et al. (2013). Influence of inoculation of arsenic-resistant Staphylococcus arlettae on growth and arsenic uptake in Brassica juncea (L.) czern. Var. R-46. J. Hazard Mater. 262, 1039–1047. doi:10.1016/j.jhazmat.2012.08.019
Sukweenadhi, J., Kim, Y. J., Choi, E. S., Koh, S. C., Lee, S. W., Kim, Y. J., et al. (2015). Paenibacillus yonginensis DCY84(T) induces changes in Arabidopsis thaliana gene expression against aluminum, drought, and salt stress. Microbiol. Res. 172, 7–15. doi:10.1016/j.micres.2015.01.007
Tanya Morocho, M., and Leiva-Mora, M. (2019). Microorganismos eficientes, propiedades funcionales y aplicaciones agrícolas. Centro Agrícola 46 (2), 93–103. Available at: http://scielo.sld.cu/scielo.php?script=sci_abstract&pid=S0253-57852019000200093 (Accessed March 28, 2020).
Tirry, N., Tahri Joutey, N., Sayel, H., Kouchou, A., Bahafid, W., Asri, M., et al. (2018). Screening of plant growth promoting traits in heavy metals resistant bacteria: prospects in phytoremediation. J. Genet. Eng. Biotechnol. 16 (2), 613–619. doi:10.1016/j.jgeb.2018.06.004
Tiwari, S., and Lata, C. (2018). Heavy metal stress, signaling, and tolerance due to plant-associated microbes: an overview. Front. Plant Sci. 9, 452. doi:10.3389/fpls.2018.00452
Viechtbauer, W. (2010). Conducting meta-analyses in R with the metafor package. J. Stat. Software 36 (3), 1–48. doi:10.18637/jss.v036.i03
Wang, W., Vinocur, B., and Altman, A. (2003). Plant responses to drought, salinity and extreme temperatures: towards genetic engineering for stress tolerance. Planta 218, 1. doi:10.1007/s00425-003-1105-5
White, J. F., Kingsley, K. L., Zhang, Q., Verma, R., Obi, N., Dvinskikh, S., et al. (2019). Review: endophytic microbes and their potential applications in crop management. Pest Manag. Sci. 75 (10), 2558–2565. doi:10.1002/ps.5527
Yan, X., Wang, Z., Mei, Y., Wang, L., Wang, X., Xu, Q., et al. (2018). Isolation, diversity, and growth-promoting activities of endophytic bacteria from tea cultivars of Zijuan and Yunkang-10. Front. Microbiol. 9, 1848. doi:10.3389/fmicb.2018.01848
Zaets, I., and Kozyrovska, N. (2012). “Heavy metal resistance in plants: a putative role of endophytic bacteria,” in Toxicity of heavy metals to legumes and bioremediation. Editors A. Zaidi, P. A. Wani, M. S. Khan (Vienna: Springer), 203–217.
Zhang, Y. F., He, L. Y., Chen, Z. J., Zhang, W. H., Wang, Q. Y., Qian, M., et al. (2011). Characterization of lead-resistant and ACC deaminase-producing endophytic bacteria and their potential in promoting lead accumulation of rape. J. Hazard Mater. 186 (2–3), 1720–1725. doi:10.1016/j.jhazmat.2010.12.069
Keywords: endophytic bacteria, effect size, heavy-metal stress, meta-analysis, plant biomass
Citation: Franco-Franklin V, Moreno-Riascos S and Ghneim-Herrera T (2021) Are Endophytic Bacteria an Option for Increasing Heavy Metal Tolerance of Plants? A Meta-Analysis of the Effect Size. Front. Environ. Sci. 8:603668. doi: 10.3389/fenvs.2020.603668
Received: 25 September 2020; Accepted: 21 December 2020;
Published: 25 January 2021.
Edited by:
Markus Puschenreiter, University of Natural Resources and Life Sciences Vienna, AustriaReviewed by:
Ihsan Ullah, King Abdulaziz University, Saudi ArabiaAdeline Su Yien Ting, Monash University Malaysia, Malaysia
Copyright © 2021 Franco-Franklin, Moreno-Riascos and Ghneim-Herrera. This is an open-access article distributed under the terms of the Creative Commons Attribution License (CC BY). The use, distribution or reproduction in other forums is permitted, provided the original author(s) and the copyright owner(s) are credited and that the original publication in this journal is cited, in accordance with accepted academic practice. No use, distribution or reproduction is permitted which does not comply with these terms.
*Correspondence: Thaura Ghneim-Herrera, tghneim@icesi.edu.co