- 1Postgraduate Program in Water Resources, Federal University of Mato Grosso, Cuiabá, Brazil
- 2Sanitary and Environmental Engineering Course, Federal University of Mato Grosso, Cuiabá, Brazil
- 3School of Engineering, University of Mount Union, Alliance, OH, United States
- 4W.K. Kellogg Biological Station, Department of Integrative Biology, Michigan State University, East Lansing, MI, United States
- 5Cary Institute of Ecosystem Studies, Millbrook, NY, United States
Hydroelectric facilities often release water at variable rates over the day to match electricity demand, resulting in short-term variability in downstream discharge and water levels. This sub-daily variability, known as hydropeaking, has mostly been studied at large facilities. The ongoing global proliferation of small hydropower (SHP) facilities, which in Brazil are defined as having installed capacities between 5 and 30 MW, raises the question of how these facilities may alter downstream flow regimes by hydropeaking. This study examines the individual and cumulative effects of hydropower facilities on tributaries in the upland watershed of the Pantanal, a vast floodplain wetland system located on the upper Paraguay River, mostly in Brazil. Simultaneous hourly discharge measurements from publicly available reference and downstream gage stations were analyzed for 11 reaches containing 24 hydropower facilities. Most of the facilities are SHPs and half are run-of-river designs, often with diversion channels (headraces). Comparison of daily data over an annual period, summarized by indicators of hydrological alteration (HA) that describe the magnitude, frequency, rate of change, and duration of flows, revealed differences at sub-daily scales attributable to hydropeaking by the hydropower facilities. Results showed statistically significant sub-daily HA in all 11 reaches containing hydropower facilities in all months. Discharge indicators that showed the highest percentage of days with increased variability were the mean rates of rise and fall, amplitude, duration of high pulses, maximum discharge, and number of reversals. Those that showed higher percentages of decreased variability included minimum discharge, number of high pulses, duration of stability, and number of low pulses. There was no correlation between HA values and physical characteristics of rivers or hydropower facilities (including installed capacity), and reaches with multiple facilities did not differ in HA from those with single facilities. This study demonstrates that SHPs as well as larger hydropower facilities cause hydrological alterations attributable to hydropeaking. Considering the rapid expansion of SHPs in tropical river systems, there is an urgent need to understand whether the ecological impacts of hydropeaking documented in temperate biomes also apply to these systems.
Introduction
Small hydropower (SHP) facilities are the most common kind of hydroelectric dams being built around the world, and although they are generally viewed as less environmentally harmful than larger dams, there has been little research to support that assertion, particularly in tropical and subtropical regions where most new SHPs are being constructed (Mbaka and Mwaniki, 2015; Couto and Olden, 2018). Reflecting the widespread assumption that SHPs have lower environmental and social impacts than larger dams, many countries have enacted policies that promote SHPs, including less stringent environmental impact assessments. In Brazil and many other countries, multiple SHPs may be distributed in series along river systems, raising concerns about their cumulative effects on rivers and downstream ecosystems (Kibler and Tullos, 2013; Athayde et al., 2019).
Even dams that are small may affect channel morphology, sediment transport, and deposition (Baker et al., 2011; Olden, 2016; Couto and Olden, 2018). Where significant impoundments exist relative to the size of the stream, artificially warm or cold water released downstream can negatively affect the aquatic biota (Zaidel et al., 2021). Dams and weirs associated with SHPs represent physical barriers for migratory species that rely on connected rivers to move upstream to spawn, to access floodplains, and for downstream migrations (Ovidio and Philippart, 2002; Santucci et al., 2005; Pompeu et al., 2012; Couto et al., 2021), and passage through turbines can harm or kill larval and adult fishes, shrimp, and other aquatic animals (DuBois and Gloss, 1993; Benstead et al., 1999).
A well-known effect of larger hydroelectric dams is the release of water at variable rates over the course of the day (i.e., sub-daily) to accommodate variation in electricity demand, and the resultant short-term variability in downstream velocity, discharge, and water levels is known as load following or hydropeaking (Bejarano et al., 2017). The ecological impacts of hydropeaking have mostly been studied at large facilities in North America and Europe, where mitigation measures have been designed to protect against stranding of fishes and maintain minimum flows to avoid desiccation of fish eggs (Moreira et al., 2019).
The ongoing global proliferation of small hydropower (SHP) facilities, which in Brazil are defined as having installed capacities between 5 and 30 MW [Aneel (Agência Nacional De Energia Elétrica), 2016], raises the question of how downstream flow regimes may be altered. Hydrological effects of SHPs are of particular concern in the upland watershed of the Pantanal, a world-renowned floodplain wetland system located mostly in Brazil. While the effects of hydropeaking are unlikely to extend into the floodplains due to longitudinal attenuation (Collischonn et al., 2019), the unnatural sub-daily variability in river flow regimes in reaches downstream of SHP facilities could affect behavior and reproduction of fishes that migrate upstream from the Pantanal (Campos et al., 2020), in addition to resident fishes and other aquatic and riparian organisms.
Existing and proposed hydropower facilities in tributaries to the Pantanal are depicted in Figure 1. As of 2018 there were 47 hydropower facilities in operation (hereafter “current hydropower facilities”), the majority of which are SHPs, with an additional 138 projects under construction, planned, proposed, or identified by the government as prospective sites (hereafter “future hydropower facilities”) (Agência Nacional de Águas, 2018). Most of these SHPs present diversion designs, where a low dam with a small or non-existent reservoir diverts river water into an artificial channel for as much as several km to a powerhouse farther down the river valley (Oliveira et al., 2020). The majority of the river discharge is normally diverted, leaving the natural channel with little as 10% of the discharge. The SHP designs that lack a large reservoir are “run-of-river” facilities inasmuch as they cannot alter discharge except on short time scales (Csiki and Rhoads, 2010; Kaunda et al., 2012). Many of the SHPs are located on lower-order rivers but some are on larger rivers with low elevational gradients.
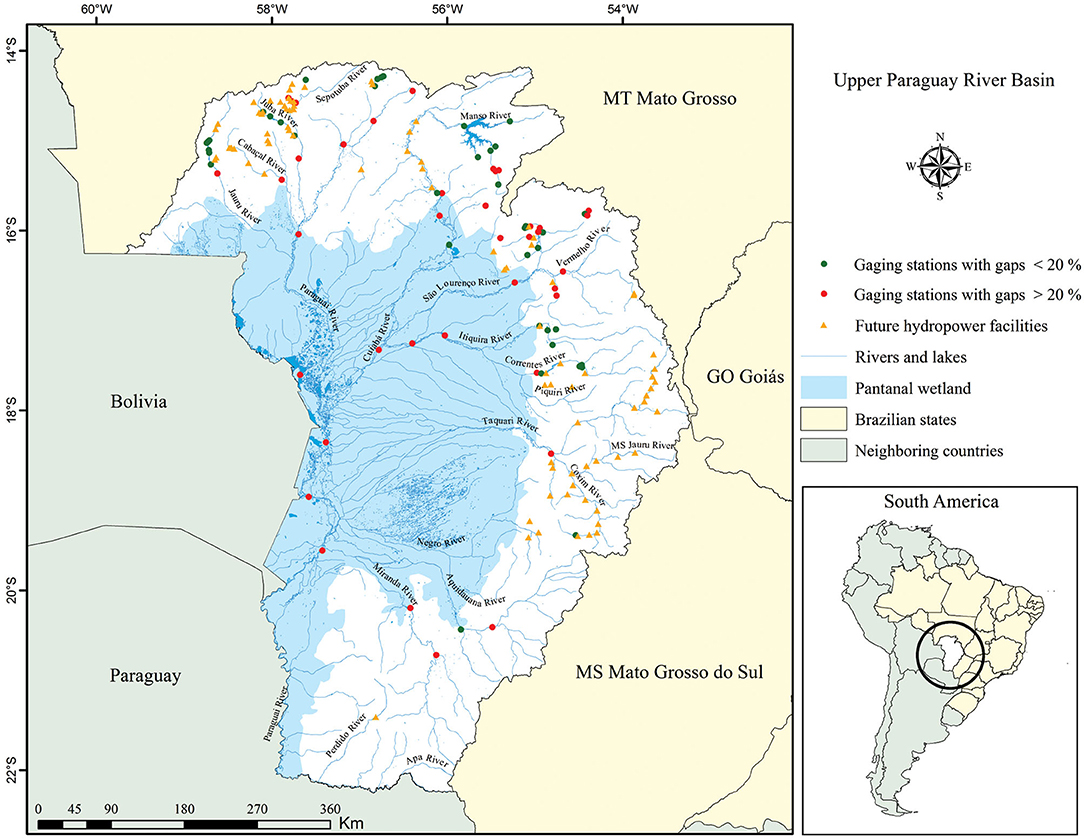
Figure 1. Map of the Upper Paraguay River Basin showing the distribution of river gaging stations upstream and downstream of currently operating hydropower facilities, indicating the stations with data gaps of <20% that allowed upstream-downstream comparisons for 24 hydropower facilities. The map also shows future hydropower projects that are either under construction, planned, or identified as potential sites for hydropower development in the Pantanal watershed by either the Brazilian National Electric Energy Agency (ANEEL) or the state environmental agencies (depending on location).
In light of the ongoing construction and planning of future SHPs in the Pantanal watershed, there is an urgent need to understand how numerous SHPs on the tributaries may, in aggregate, alter the transport of water, sediments, and nutrients from the uplands into the Pantanal, and as well produce enough barriers to the upstream migration of fishes from the Pantanal to impede their reproduction and reduce their populations. In recognition of these needs, the present study is part of a multidisciplinary research program that has examined many dimensions of the issues surrounding hydroelectric facilities in the tributaries of the Pantanal, including hydrology (this study), sediment transport (Fantin-Cruz et al., 2020), water quality (Oliveira et al., 2020; Fantin da Cruz et al., 2021), and fish and fisheries (Campos et al., 2020; Ely et al., 2020). In this study, evidence for hydropeaking is evaluated based on discharge patterns in river gages downstream of 11 reaches containing a total of 24 hydropower facilities compared to simultaneous measurements at reference gages not influenced by the facilities. Comparison of hourly data, summarized by indicators of hydrological alteration, reveals differences at sub-daily scales that may be attributable to the hydropower facilities and aspects of their design. Accordingly, relationships between the observed hydrological alterations and the hydraulic and hydrological characteristics of rivers and hydropower facilities were also examined. These included installed potential, mean discharge, watershed area, reservoir area, hydraulic residence time, diverted natural channel length, and dam design. The paper ends with recommendations on further research to better understand how hydropeaking by small hydropower facilities may affect the aquatic biota of downstream reaches.
Materials and Methods
Study Site
This study examines rivers in the Brazilian portion of the uplands in the Upper Paraguay River basin that drain to the Pantanal wetland. The Pantanal lies mostly within Brazil, and drains southward via the Paraguay River. The uplands (150–1,400 m a.s.l.), which represent 59% of the basin area and lie mainly to the east and north of the Pantanal, include sloping terrain favoring rapid runoff and high sediment production. The Pantanal floodplains (80–150 m a.s.l.) are subject to extensive seasonal inundation by overflow of river inflows originating in the uplands as well as delayed drainage of local rainfall (Hamilton et al., 1996). According to the Köppen-Geiger climate classification, the climate of the region is tropical savanna, with average annual precipitation in the uplands ranging from 1,200 to 1,800 mm. About 80% of the annual rainfall occurs in the rainy season from October to April (Gonçalves et al., 2011).
The native vegetation in the uplands is Cerrado savanna, but extensive areas are now converted to cropland (29% of the upland watershed area analyzed in this study) or pasture (22%). Human population density in the rural municipalities is low with mostly <10 inhabitants km−2. Cuiabá city and its environs, situated along the Cuiabá River <50 km upstream of the Pantanal, is the largest urban area, which together with three other medium-sized cities located in the uplands has about 1,260,000 inhabitants.
Study Reaches, Data Sources, and Processing
The study region has data for 108 gaging stations with sub-daily measurements, of which 80 have rating curves to estimate discharge from stage and the remainder recorded only stage with no discharge measurements, and were installed at dams. Of the 80 stations with discharge data, 40 had sufficiently complete records for our analysis (i.e., gaps amounting to <20% of the year) and met our quality checks (Figure 1). These stations permitted upstream-downstream comparisons for the 24 hydropower facilities whose characteristics are shown in Table 1. Six of the 24 hydropower facilities were bounded by gaging stations, whereas the other 18 are sequentially arranged within 5 reaches, in which case we call them “cascades,” and thus we evaluate 11 reaches in this study. The watersheds above the 24 hydropower facilities range in area from 148 to 5,775 km2, and the rivers range in long-term mean discharge from 4.5 to 108 m3 s−1.
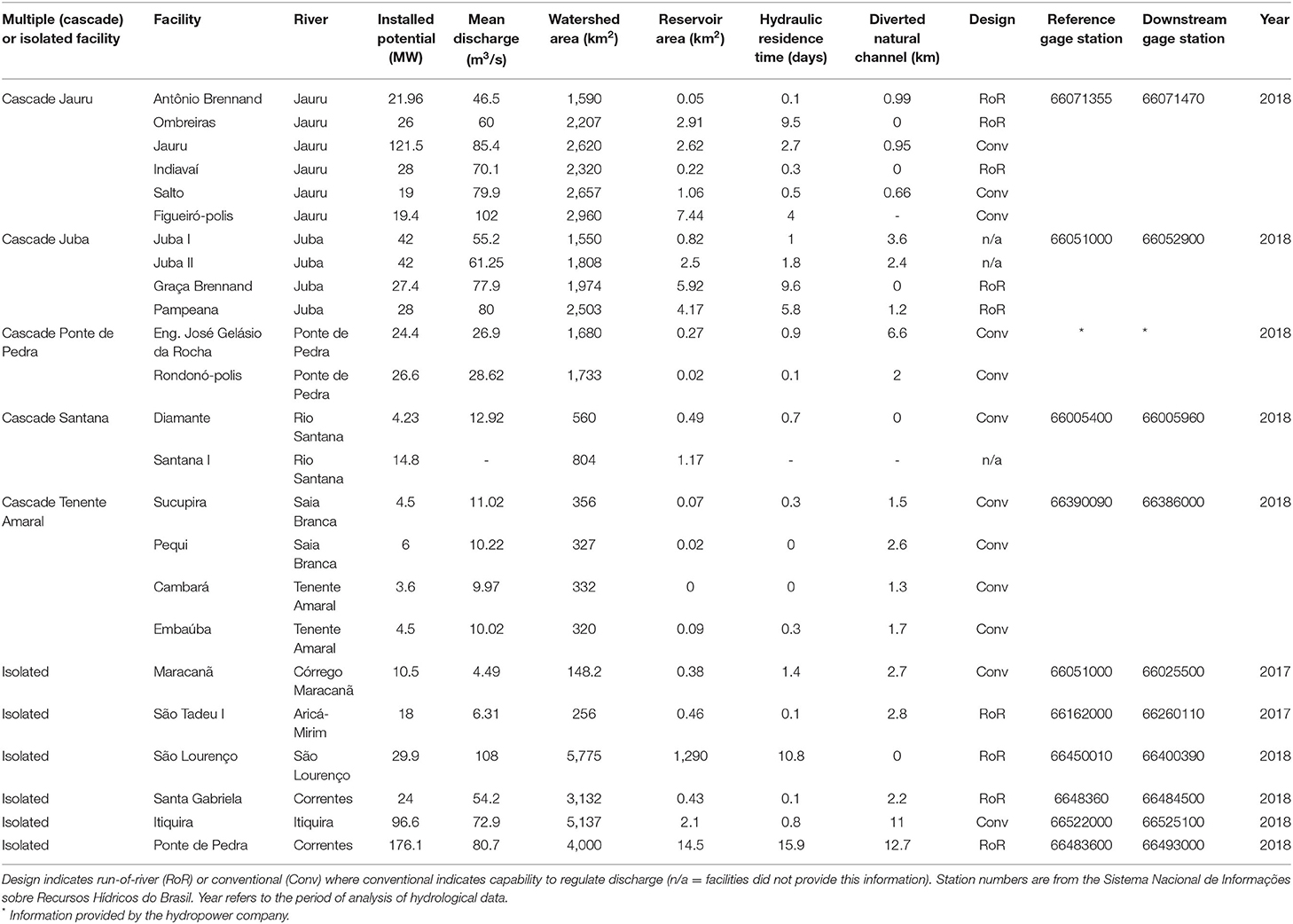
Table 1. Locations and characteristics of the hydropower facilities and river discharge gage stations.
Most of these hydropower facilities can be considered small, although five have installed capacities above the Brazilian government's regulatory definition of small hydropower as <30 MW installed capacity, and two of those exceed 100 MW. Two of those that exceed 30 MW (Juba I and II, 42 MW each) have dams and reservoirs similar in size to the SHPs. One of the SHPs (São Lourenço, 29 MW) creates a reservoir comparable in size to larger facilities such as the largest one studied here, Ponte de Pedra (176 MW). Thus, installed capacity is an imperfect indicator of the potential environmental effects of these facilities (Couto and Olden, 2018). Hence, we analyze the SHPs and larger facilities together in this study to examine similarities and differences in their downstream hydrological effects.
All facilities have dams that form reservoirs, which range in area from 0.01 to 14.5 km2, in volume from 0.035 to 111 hm3, and in hydraulic residence time from 0.1 to 15.9 days. Twelve of the 24 are run-of-river designs, nine have the capacity to regulate discharge (labeled as conventional in Table 1), and information on design was unavailable for the other three. Most (17) of the facilities divert water from the natural channel into headraces for distances ranging from 0.66 to 12.7 km.
Figure 1 shows the distribution of existing and future hydropower facilities as well as available river gage stations considered in this study. Discharge data were downloaded from a public portal called the Sistema Nacional de Informações sobre Recursos Hídricos do Brasil (http://www.snirh.gov.br/hidrotelemetria). We used only stations with high-frequency measurements (i.e., every hour or more often). Discharge time series were screened for gaps, defined as either zero discharge (none of these rivers are intermittent) or missing date, time, and/or discharge data within the temporal sequence. For cases with missing discharge data, the sequential dates and times were added to enable us to estimate the percentage of missing data. Each discharge time series was inspected for outliers that were obviously unrealistic, as well as for abrupt changes that might reflect equipment problems, and in these cases the suspect data were replaced with gaps. For further analysis we selected only time series with gaps amounting to <20% of the total times, and gaps were excluded from statistical summaries. We analyzed data from 2018 where possible, though in some cases we had to use 2017 data because data gaps in 2018 amounted to >20%.
We analyzed discharge time series where stations existed both upstream and downstream of one or more hydropower facilities, which in many cases were measurements made by the hydropower companies as required for environmental compliance. Only stations with discharge data, as opposed to just water level as is often measured at the dams, were selected. Hereafter we use the term reference in place of upstream because not all cases presented a gaging station immediately upstream of the hydropower facility. In some cases we had to use a reference station well upstream, but not downstream of other hydroelectric facilities, and in one case we had to use a station on a downstream tributary with similar watershed features and discharge (the Vermelho River below the SHP São Lourenço). Discharge data were standardized to the mean annual discharge to facilitate comparisons across river reaches of different discharge rates (Bejarano et al., 2017). This standardization assumes that there is a fixed proportionality between flows at the reference site and downstream flows in a particular river reach.
Indicators of Flow Regime Alteration
We calculated sub-daily flow regime metrics from discharge data at 1-h intervals, based on the methods of Greimel et al. (2016), Timpe and Kaplan (2017), and Bejarano et al. (2017). These methods adapt the widely used Indicators of Hydrological Alteration approach (IHA; Richter et al., 1996) to produce sub-daily Indicators of Hydrological Alteration including 11 indicators that describe the magnitude, frequency, rate of change, and duration of flows. The indicators were calculated at daily time scales based on pairwise comparisons of temporally matched data from the reference site and downstream of hydropower facilities (Table 2).
For a particular hydrological indicator, the difference between the reference site and downstream of the hydropower facility was considered significant in a particular month only when the monthlong series of daily values showed statistically significant differences based on the non-parametric Mann-Whitney U-test (α ≤ 0.05).
For each of the indicators in Table 2 that showed significant differences between the reference and downstream sites in a particular month, we evaluated the hydrological alteration (HA) attributable to the hydropower facilities following the method described by Timpe and Kaplan (2017):
where HA is the median percent alteration in the indicator, Mdown is the median daily value of the indicator downstream of the hydropower facility, and Mref is the median daily value of the indicator at the reference site. Equation (1) was computed at daily intervals, from which monthly medians of HA were determined. Significant positive values of HA indicate an increase in the indicator from the reference site to downstream, negative values indicate a decrease, and in some cases the medians of the distributions were equal but the Mann-Whitney test indicated significant differences in the distributions of daily values around the median.
To facilitate HA comparisons among reaches with hydropower facilities, we calculated the overall HA across all indicators and months for each hydropower facility (Timpe and Kaplan, 2017). We summed the absolute values of the monthly HA values that were statistically significant, then divided that sum by the total number of months with data (including months that did not show significant differences between the reference and downstream sites in a particular month, effectively counting them as zero HA values).
We evaluated the effects of hydraulic and hydrological characteristics at each hydropower facility (Table 1) on the monthly HA values as well as the overall HA using the Spearman's rank correlation coefficient (α ≤ 0.05). Where more than one hydropower facility existed between the upstream and downstream gage stations, we examined correlations by two alternative approaches—using just the characteristics of the most downstream hydropower facility or using the sum of characteristics of all of the facilities in the reach (except in the case of discharge). The Mann-Whitney test was then employed to determine whether the number of indicators with significant HA values as well as the sub-daily HA values differed between those two approaches (α ≤ 0.05).
Results
Hydrological Alteration at Sub-daily Time Scales
Example hydrographs comparing reference and downstream stations for reaches with particularly marked HA show clear sub-daily variation imposed by the facilities (Figure 2). This variation occurs with a visible diel periodicity below the Santa Gabriela (Figures 2E,F) and Maracanã (Figures 2C,D) hydropower facilities, but is more irregular below the multiple facilities in the Jauru (Figures 2A,B) and Juba cascades (Figures 2G,H). These examples comparing hydrographs above and below reaches with the highest overall HA values reveal variable diel patterns of alteration ranging from irregular with high and low pulses of brief duration (Jauru and Juba cascades) to relatively regular with higher and lower periods lasting longer (SHP Maracanã and SHP Santa Gabriela), and these examples provide an indication of the magnitude of sub-daily variation that can be induced by the hydropower facilities (Figure 2). The magnitude of discharge variability would likely be accompanied by significant changes in the wetted area of channels downstream of these facilities, particularly during low discharge periods.
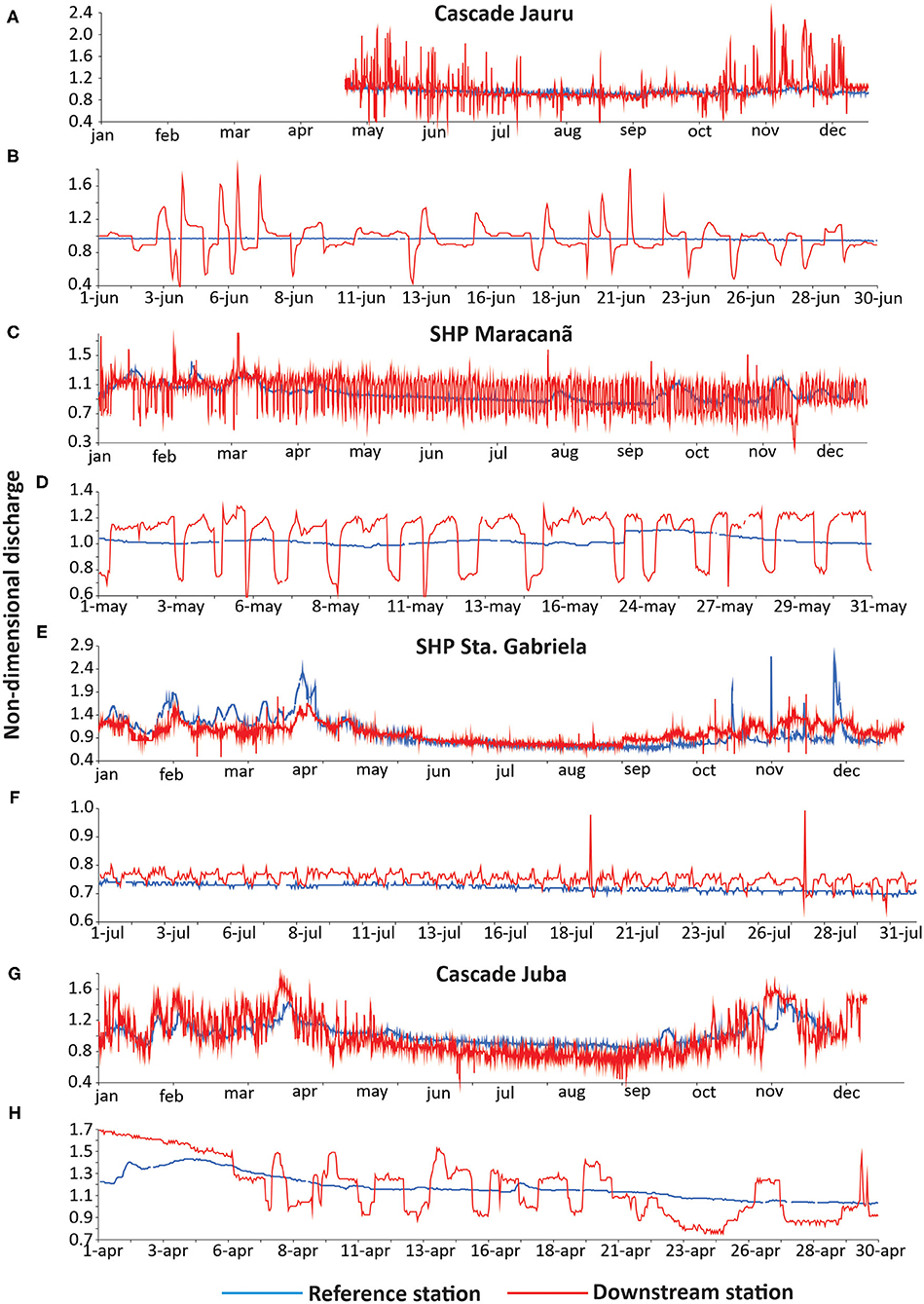
Figure 2. Hydrographs showing examples of hydrological alteration by small hydropower (SHP) facilities during the 2018 calendar year (2017 for Maracanã) and in representative months of that year during the season of lower discharge. Red lines show discharge downstream of the hydropower facilities and blue lines show the reference discharge station. All discharge data are standardized to the mean annual discharge to facilitate comparisons among rivers. (A,B) Cascade Jauru on the Jauru River in Mato Grosso State; (C,D) SHP Maracanã on the Córrego Maracanã (a tributary of the Sepotuba River); (E,F) SHP Santa Gabriela on the Correntes River; and (G,H) Cascade Juba on the Juba River.
Analysis of 11 indicators of hydrological alteration in 11 reaches containing a total of 24 hydropower facilities, most of which are SHPs, provides clear evidence of sub-daily variability that can be attributed to hydropeaking by dam operations (Figures 3, 4). Almost all of the indicators showed significant differences between gages at reference sites and gages downstream of the reaches in most months over the year of analysis. The greatest alterations involved the rates of change (rises and falls) in discharge and the magnitudes of minimum flows (often lower) and maximum flows (often higher). The duration of stable periods decreased in most cases. The hydrological alteration was most marked at the height of the dry season (Aug–Oct) but was apparent in all months. There were more than twice as many significantly positive values of monthly HA (and thus increased variability) than negative ones (49 vs. 22% overall) (Figure 3A). Discharge indicators that showed the highest percentage of increases (positive HA values) were the mean rates of rise and fall, amplitude, duration of high pulses, maximum discharge, and number of reversals. Those that showed higher percentages of decreases than increases include minimum discharge, number of high pulses, duration of stability, and number of low pulses.
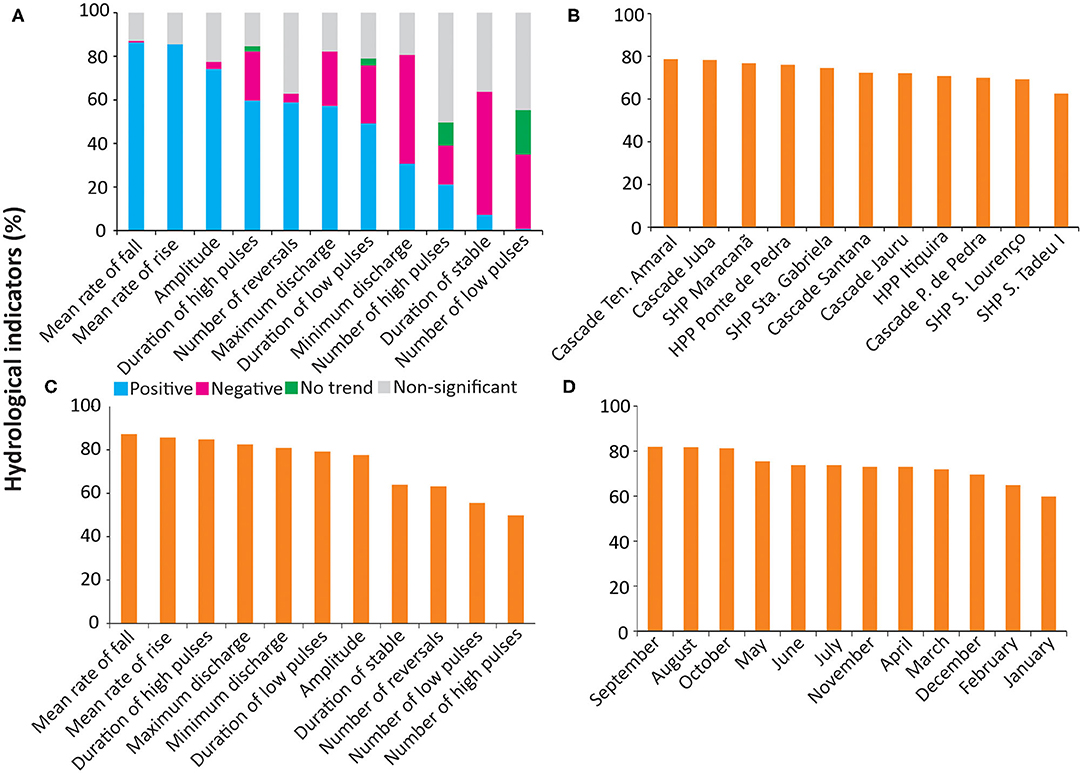
Figure 3. Summary of the sub-daily indicators of hydrological alteration (HA): (A) Relative proportions of the monthly median values of HA showing significant positive values (increasing), negative (decreasing), no trend (indistinguishable medians) but with statistically significant differences in data distributions, and non-significant monthly medians; (B) Percentages of the hydrological indicators that showed statistically significant differences based on comparisons of daily values of each month, arranged by hydropower facility; (C) the same arranged by the indicators in Table 2; and (D) the same arranged by month.
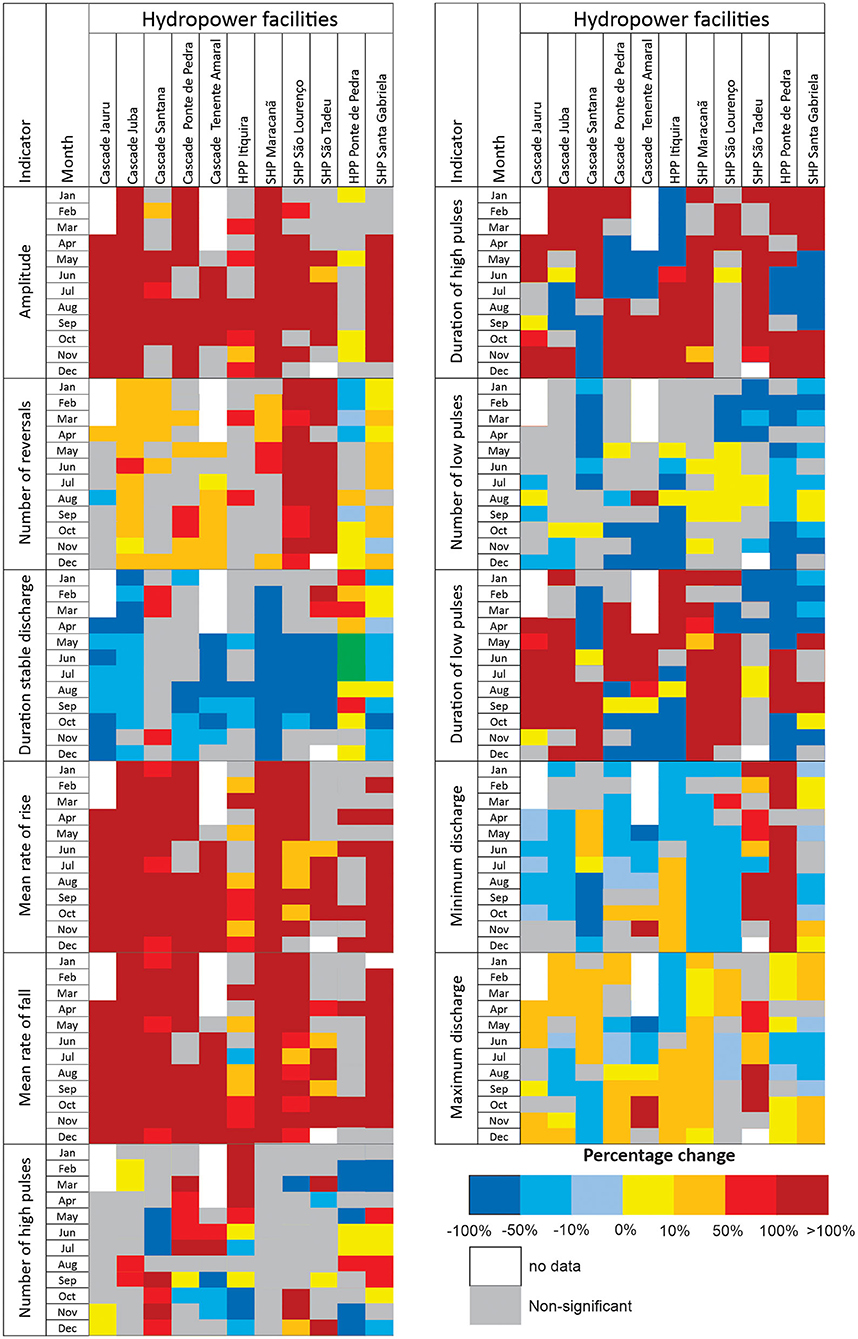
Figure 4. Percentage change in sub-daily indicators of hydrological alteration for each study reach by month, showing only cases where there was a statistically significant difference (Mann-Whitney test) between the upstream reference site and the downstream site.
The hydropower facilities that most strongly altered downstream hydrology were the Cascade Tenente Amaral (sum, 19.5 MW) and the Cascade Juba (sum, 139.4 MW), each located on rivers with those names. In both of these reaches, 78% of the paired comparisons of monthly indicators showed significant alteration based on the Mann-Whitney test (Figure 3B). The indicators that showed the most frequent alterations were the mean rates of rise and fall in discharge, with 91 and 89% showing significant alterations, respectively. Indicators that were least often significant include the numbers of high and low discharge pulses (58 and 51%, respectively) (Figure 3C). The highest percentages of statistically significant sub-daily hydrological alterations occurred during months of lower discharge and particularly from August through October, although the percentages exceeded 50% in all months (Figure 3D). The full temporal resolution of the data summarized in Figure 3 is depicted in Figure 4.
Among the 11 reaches, the overall HA varied by >4-fold among the reaches analyzed (Figure 5). The greatest overall HA occurred in the Jauru River reach containing the six hydropower facilities (Jauru Cascade in Table 1: 423%), followed by the Maracanã (302%), Santa Gabriela (229%) and Juba Cascade (181%) reaches. The overall HA did not vary in rank order of installed capacity; the lowest overall HA values were found for two of the larger facilities in terms of installed capacity (Itiquira and SHP São Lourenço), whereas the highest overall HA was found for the Jauru cascade containing six facilities with one particularly large one.
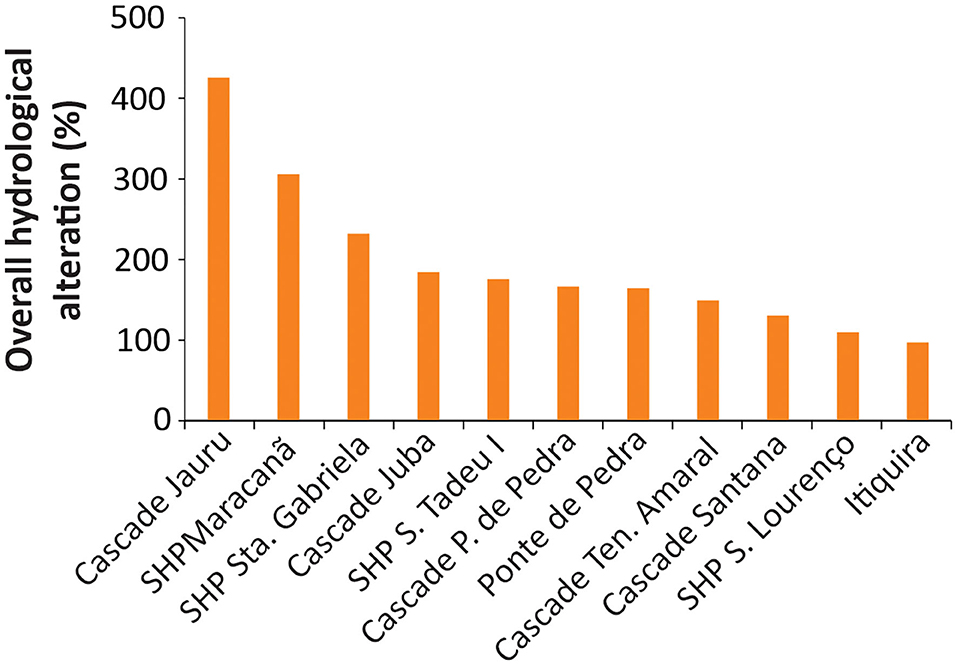
Figure 5. Overall hydrological alteration (HA overall) across all indicators and months for each hydropower facility (in the five reaches containing more than one facility, the labels show the name of the facility that is closest to the downstream gaging station).
Relationships Between Hydrological Alteration and Characteristics of Rivers and Facilities
The physical characteristics of rivers and facilities in the 11 reaches (Table 1) were not significantly correlated with the number of indicators that changed significantly between upstream and downstream (Figure 3A), nor with the monthly HA values (Figures 3C, 4) (statistical results not shown). In addition, comparison between reaches containing more than one hydropower facility with those containing a single facility showed no significant differences in either the percentage of significant indicators of hydrological alteration (Figure 6A) or the overall HA (Figure 6B), and therefore no evidence for cumulative impacts.
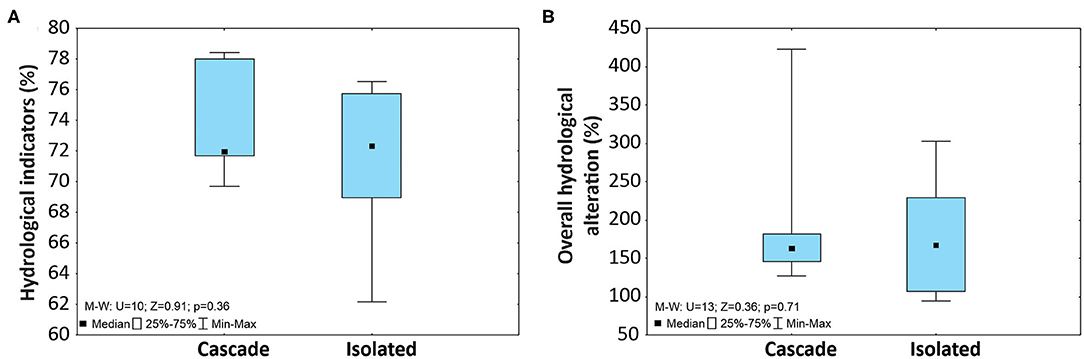
Figure 6. Comparison between reaches containing more than one hydropower facility (cascades) with those containing a single facility (isolated) showing no significant differences in either (A) the percentage of significant indicators of hydrological alteration or (B) the overall HA.
Discussion
Our results are consistent with earlier studies that evaluated hydrological alterations by subsets of these hydropower facilities. Timpe and Kaplan (2017) analyzed indicators of hydrological alteration at multiannual time scales below 33 hydropower facilities, 16 of which were SHPs, across the Amazon and Upper Paraguay River basins, including several of the facilities we analyze here as well as the much larger reservoir created by the Manso Dam (212 MW) on the Cuiabá River. Although lowland hydropower facilities with the largest dams and reservoirs induced the greatest alterations, Timpe and Kaplan noted that for reaches containing single facilities that were either large dams or SHPs, the SHPs induced alterations similar in magnitude to the large dams when scaled to installed capacity (i.e., % HA per MW). Ely et al. (2020), in a multiyear analysis of indicators of hydrological alteration, found that high and low pulse counts as well as the number of reversals were the most frequent dam-induced impacts in the Upper Paraguay River basin.
Other studies have examined individual hydropower facilities in the upper Paraguay River basin. Braun-Cruz et al. (2021) reported evidence of hydropeaking by the Itiquira hydropower facility on the Itiquira River, which was included in the present study. The downstream hydrological effects of the much larger Manso Dam were recently evaluated in detail by Jardim et al. (2020). Fantin-Cruz et al. (2015) analyzed IHA at multiannual time scales attributable to the Ponte de Pedra hydropower facility, also one of our study sites and our largest facility in terms of installed capacity (176 MW). Seven indicators were significantly altered by Ponte de Pedra—magnitude of lowest monthly flow, minimum flows of 1, 3, and 7 days, maximum flow of 90 days, and counts of high and low pulses—and the reservoir released higher flows during the dry season.
Sub-daily hydrological alterations attributable to hydropeaking have been documented below hydropower facilities elsewhere throughout the world (Bejarano et al., 2017), though only in a few studies of modern SHPs (e.g., Lu et al., 2018; Xiao et al., 2019). Hydrological alterations by SHPs tend to occur over short time scales as the number of active turbines is increased during high demand in the day and reduced at night, particularly below run-of-river facilities with relatively small reservoir volumes that depend on managing discharge to meet short-term variation in electricity demand (Bevelhimer et al., 2015). Many of these SHPs are diversion designs in which most of the discharge is directed into a headrace leading to the powerhouse, and thus fluctuations in discharge through the turbines may cause opposite fluctuations in the diverted portion of the natural channel. In contrast, large dams and reservoirs tend to dampen seasonal variation in outflow discharge, releasing more water during the dry season and attenuating short-term discharge peaks resulting from precipitation or snowmelt (Magilligan and Nislow, 2005).
The ecological implications of hydropeaking for downstream ecosystems are little known for tropical rivers (Jumani et al., 2018), but have been studied in temperate zones with negative impacts increasingly demonstrated for riparian and aquatic plants (Bejarano et al., 2018), macroinvertebrates (Kennedy et al., 2016; Leitner et al., 2017; Schulting et al., 2019), and especially for fishes (Vollset et al., 2016; Boavida et al., 2017; Costa et al., 2019; Rocaspana et al., 2019; Vehanen et al., 2019). As a result of increasing awareness of these impacts, abrupt changes in water level and velocity associated with hydropeaking have received increasing regulatory attention (Hauer et al., 2017, Hayes et al., 2019, Moreira et al., 2019), particularly in rivers supporting important fisheries. In the case of the Itiquira hydropower facility in the upper Paraguay River, Braun-Cruz et al. (2021) provided circumstantial evidence that a fish kill involving stranding was linked to hydropeaking by the dam operations.
In conclusion, we have demonstrated that many SHPs, as well as somewhat larger hydropower facilities, cause hydrological alterations on sub-daily time scales attributable to hydropeaking to meet varying energy demand. In our data set, these hydrological alterations were not correlated with characteristics of the river reaches or the facilities. In addition to those variables, prediction of hydrological alterations caused by hydropeaking would likely require information on operating procedures at each facility, which was not available to us.
Considering the rapid expansion of small hydropower development in tropical environments (Couto and Olden, 2018), there is an urgent need to understand whether the ecological impacts of hydropeaking documented in temperate biomes also apply to these systems. This will be challenging because life cycles and behavior of the aquatic biota in tropical rivers in relation to river hydrology are less well-understood, and even migration routes of fishes that support socioeconomically valuable fisheries are incompletely known (Campos et al., 2020). It is obvious that the aquatic biota will likely be harmed by abrupt decreases in water levels causing stranding of fishes and other aquatic animals as well as the temporary emergence of aquatic substrata that would otherwise remain underwater. However, changes in depth and wetted area of the river channel as a result of hydropeaking depend on channel morphology (Moreira et al., 2019), information that is lacking for the rivers we study here, as it is for most other regions of the world where SHPs are proliferating. If negative impacts are revealed, research will be needed on the costs vs. benefits of mitigation of these changes by altering dam operations, as for example those proposed for the SHP Ponte de Pedra by Fantin-Cruz et al. (2015). In addition to mitigating impacts of existing SHPs, planning for new SHP locations and designs needs to consider how the resultant hydrological modifications may negatively affect migratory fishes and other aquatic animals, not only by producing barriers and directing most of the flow through turbines, but also altering downstream hydrology. Such planning should be conducted at the scale of entire river basins to minimize negative impacts on migratory populations (Couto and Olden, 2018; Lange et al., 2018; Couto et al., 2021).
Data Availability Statement
The raw data supporting the conclusions of this article will be made available by the authors, without undue reservation.
Author Contributions
This study was conceived and carried out by IF-C, PG, PZ, and SH. IF-C, GS, and RB developed the computational routine for the high frequency time series analysis. Field work and data analysis were conducted by IF-C, JF, EU, EM, and HT. The paper was written mainly by IF-C, JF, and SH. All authors contributed to the article and approved the submitted version.
Funding
Funding was provided by the Brazilian National Water Agency (Case 062/ANA/2016), the Foundation for Research Support in the State of Mato Grosso (FAPEMAT Case 0249639/2017), the Brazilian National Council for Scientific and Technological Development (CNPq, Case 435543/2018-0), and the Critical Ecosystem Partnership Fund (Grant Agreement CEPF-104254). Logistical and technical support was provided by the Federal University of Mato Grosso (UFMT).
Conflict of Interest
The authors declare that the research was conducted in the absence of any commercial or financial relationships that could be construed as a potential conflict of interest.
Acknowledgments
We are grateful for support in field activities and access to secondary data provided by the State Secretariats for the Environment of Mato Grosso (SEMA-MT) and Mato Grosso do Sul (IMASUL), the Prosecutor's Office of the State of Mato Grosso (MP-MT), the Brazilian Association for Clean Energy generation (ABRAGEL), and the Union of Construction, Generation, Transmission and Distribution of Electrical Energy and Gas in the State of Mato Grosso (SINDENERGIA). We also appreciate field assistance by Valdeci Oliveira, Josias Campos, Adriano Dias, and Luiz Amaro.
References
Agência Nacional de Águas (2018). Conjuntura dos Recursos Hídricos no Brasil 2018: Informe anual. Available online at: http://arquivos.ana.gov.br/portal/publicacao/Conjuntura2018.pdf (accessed 3 June, 2020).
Aneel (Agência Nacional De Energia Elétrica) (2016). Resolução Normativa N 745, 22 de Novembro de 2016. Brasil: Ministério de Minas e Energia. 228:27.
Athayde, S., Duarte, C. G., Gallardo, A. L. C. F., Moretto, E. M., Sangoi, L. A., Dibo, A. P. A., et al. (2019). Improving policies and instruments to address cumulative impacts of small hydropower in the Amazon. Energy Policy 132, 265–271. doi: 10.1016/j.enpol.2019.05.003
Baker, D. W., Bledsoe, B. P., Albano, C. M., and Poff, N. L. (2011). Downstream effects of diversion dams on sediment and hydraulic conditions of Rocky Mountain streams. River Res. Appl. 27, 388–401. doi: 10.1002/rra.1376
Bejarano, M. D., Jansson, R., and Nilsson, C. (2018). The effects of hydropeaking on riverine plants: a review. Biol. Rev. 93, 658–667. doi: 10.1111/brv.12362
Bejarano, M. D., Sordo-Ward, A., Alonso, C., and Nilsson, C. (2017). Characterizing effects of hydropower plants on sub-daily flow regimes. J. Hydrol. 550, 186–200. doi: 10.1016/j.jhydrol.2017.04.023
Benstead, J. P., March, J. G., Pringle, C. M., and Scatena, F. N. (1999). Effects of a low-head dam and water abstraction on migratory tropical stream biota. Ecol. Appl. 9, 656–668. doi: 10.1890/1051-0761(1999)009[0656:EOALHD]2.0.CO;2
Bevelhimer, M. A., McManamay, R. A., and O'Connor, B. (2015). Characterizing sub-daily flow regimes: implications of hydrologic resolution on ecohydrology studies. River Res. Appl. 31, 867–879. doi: 10.1002/rra.2781
Boavida, I., Harby, A., Clarke, K. D., and Heggenes, J. (2017). Move or stay: habitat use and movements by Atlantic salmon parr (Salmo salar) during induced rapid flow variations. Hydrobiologia 785, 261–275. doi: 10.1007/s10750-016-2931-3
Braun-Cruz, C. C., Tritico, H. M., Beregula, R. L., Girard, P., Zeilhofer, P., Ribeiro, L. S., et al. (2021). Evaluation of hydrological alterations at the sub-daily scale caused by a small hydroelectric facility. Water 13:206. doi: 10.3390/w13020206
Campos, M. M., Tritico, H. M., Girard, P., Zeilhofer, P., Hamilton, S. K., and Fantin-Cruz, I. (2020). Predicted impacts of proposed hydroelectric facilities on fish migration routes upstream from the Pantanal wetland (Brazil). River Res. Applic. 36, 1–13. doi: 10.1002/rra.3588
Collischonn, W., Paz, A. R., Melo, M. M. M., and Jardim, P. F. (2019). Potenciais impactos de barragens sobre o regime hidrológico nos rios da RH Paraguai. Elaboração de Estudos de Avaliação dos Efeitos da Implantação de Empreendimentos Hidrelétricos na Região Hidrográfica do Rio Paraguai. Agência Nacional de Águas, Brasilia (DF). Available online at: https://www.ana.gov.br/gestao-da-agua/planejamento-dos-recursos-hidricos/plano-de-recursos-hidricos-rio-paraguai/grupo-de acompanhamento/19a-reuniao-do-gap-1/relatorio-de-andamento_hidrologia_parte1-1.pdf (accessed 3 June, 2020).
Costa, M. J., Fuentes-Perez, J. F., Boavida, I., Tuhtan, J. A., and Pinheiro, A. N. (2019). Fish under pressure: examining behavioural responses of Iberian barbel under simulated hydropeaking with instream structures. PLoS ONE 1:e0211115. doi: 10.1371/journal.pone.0211115
Couto, T. B. A., Messager, M. L., and Olden, J. D. (2021). Safeguarding migratory fish via strategic planning of future small hydropower in Brazil. Nat. Sustain. doi: 10.1038/s41893-020-00665-4 Available online at: https://www.nature.com/articles/s41893-020-00665-4
Couto, T. B. A., and Olden, J. D. (2018). Global proliferation of small hydropower plants – science and policy. Front. Ecol. Environ. 16:1746. doi: 10.1002/fee.1746
Csiki, S., and Rhoads, B. L. (2010). Hydraulic and geomorphological effects of run-of-river dams. Prog. Phys. Geogr. 34, 755–780. doi: 10.1177/0309133310369435
DuBois, R. B., and Gloss, S. P. (1993). Mortality of juvenile American shad and striped bass passed through Ossberger crossflow turbines at a small-scale hydroelectric site. N. Am. J. Fish. Mgmt. 13, 178–185. doi: 10.1577/1548-8675(1993)013<0178:MOJASA>2.3.CO;2
Ely, P., Fantin-Cruz, I., Tritico, H. M., Girard, P., and Kaplan, D. (2020). Dam-induced hydrologic alterations in the rivers feeding the Pantanal. Front. Environ. Sci. 8:579031. doi: 10.3389/fenvs.2020.579031
Fantin da Cruz, R., Hamilton, S. K., Tritico, H. M., Fantin-Cruz, I., Maimone de Figueiredo, D., and Zeilhofer, P. (2021). Water quality impacts of small hydroelectric power plants in a tributary to the Pantanal floodplain, Brazil. River Res. Appl. 37, 448–461. doi: 10.1002/rra.3766
Fantin-Cruz, I., Oliveira, M. D., Campos, J. A., Campos, M. M., Ribeiro, L. S., Mingoti, R., et al. (2020). Further development of small hydropower will significantly reduce sediment transport to the Pantanal Wetland of Brazil. Front. Environ. Sci. 8:577748. doi: 10.3389/fenvs.2020.577748
Fantin-Cruz, I., Pedrollo, O., Girard, P., Zeilhofer, P., and Hamilton, S. K. (2015). Effects of a diversion hydropower facility on the hydrological regime of the Correntes River, a tributary to the Pantanal floodplain, Brazil. J. Hydrol. 531, 810–820. doi: 10.1016/j.jhydrol.2015.10.045
Gonçalves, H. C., Mercante, M. A., and Santos, E. T. (2011). Hydrological cycle. Braz. J. Biol. 71, 241–253. doi: 10.1590/S1519-69842011000200003
Greimel, F., Zeiringer, V., Höller, N., Grün, B., and Godina, R. (2016). A method to detect and characterize sub-daily flow fluctuations. Hydrol. Proc. 30, 2063–2078. doi: 10.1002/hyp.10773
Hamilton, S. K., Sippel, S. J., and Melack, J. M. (1996). Inundation patterns in the Pantanal Wetland of South America determined from passive microwave remote sensing. Arch. Hydrobiol. 137, 1–23.
Hauer, C., Holzapfel, P., Leitner, P., and Graf, W. (2017). Longitudinal assessment of hydropeaking impacts on various scales for an improved process understanding and the design of mitigation measures. Sci. Total Environ. 575, 1503–1514. doi: 10.1016/j.scitotenv.2016.10.031
Hayes, D. S., Moreira, M., Boavida, I., Haslauer, M., Unfer, G., Zeiringer, B., et al. (2019). Life stage-specific hydropeaking flow rules. Sustainability 11:1547. doi: 10.3390/su11061547
Jardim, P. M., Melo, M. M. M., Ribeiro, L. C., Collischonn, W., and Paz, A. R. (2020). A modeling assessment of large-scale hydrologic alteration in South American Pantanal due to upstream dam operation. Front. Environ. Sci. 8:567450. doi: 10.3389/fenvs.2020.567450
Jumani, S., Rao, S., Kelkar, N., Machado, S., Krishnaswamy, J., and Vaidyanathan, S. (2018). Fish community responses to stream flow alterations and habitat modifications by small hydropower projects in the Western Ghats biodiversity hotspot, India. Aquat. Conserv. Mar. Freshw. Ecosyst. 28, 979–993. doi: 10.1002/aqc.2904
Kaunda, C. S., Kimambo, C. Z., and Nielsen, T. K. (2012). Hydropower in the context of sustainable energy supply: a review of technologies and challenges. ISRN Renew. Energy 2012, 1–15. doi: 10.5402/2012/730631
Kennedy, T. A., Muehlbauer, J. D., Yackulic, C. B., Lytle, D. A., Miller, S. W., Dibble, K. L., et al. (2016). Flow management for hydropower extirpates aquatic insects, undermining river food webs. Bioscience 66, 561–575. doi: 10.1093/biosci/biw059
Kibler, K. M., and Tullos, D. D. (2013). Cumulative biophysical impact of small and large hydropower development in Nu River, China. Water Resour. Res. 49, 3104–3118. doi: 10.1002/wrcr.20243
Lange, K., Meier, P., Trautwein, C., Schmid, M., Robinson, C. T., Weber, C., et al. (2018). Basin-scale effects of small hydropower on biodiversity dynamics. Front. Ecol. Environ. 16:1823. doi: 10.1002/fee.1823
Leitner, P., Hauer, C., and Wraf, W. (2017). Habitat use and tolerance levels of macroinvertebrates concerning hydraulic stress in hydropeaking rivers – a case study at the Ziller River in Austria. Sci. Total Environ. 575, 112–118. doi: 10.1016/j.scitotenv.2016.10.011
Lu, W., Lei, H., Yang, D., Tang, L., and Miao, Q. (2018). Quantifying the impacts of small dam construction on hydrological alterations in the Jiulong River basin of Southeast China. J. Hydrol. 567, 382–392. doi: 10.1016/j.jhydrol.2018.10.034
Magilligan, F. J., and Nislow, K. H. (2005). Changes in hydrologic regime by dams. Geomorphology 71, 61–78. doi: 10.1016/j.geomorph.2004.08.017
Mbaka, J. G., and Mwaniki, M. W. (2015). A global review of the downstream effects of small impoundments on stream habitat conditions and macroinvertebrates. Environ. Rev. 23, 257–262. doi: 10.1139/er-2014-0080
Moreira, M., Hayes, D. S., Boavida, I., Schletterer, M., Schmutz, S., and Pinheiro, A. (2019). Ecologically-based criteria for hydropeaking mitigation. A review. Sci. Total Environ. 657, 508–1522. doi: 10.1016/j.scitotenv.2018.12.107
Olden, J. D. (2016). “Challenges and opportunities for fish conservation in dam-impacted waters,” in Conservation of Freshwater Fishes, eds G. P. Closs, M. Krkosek, and J. D. Olden (Cambridge: Cambridge University Press), 107–148.
Oliveira, M. D., Fantin-Cruz, I., Campos, J. A., Campos, M. M., Mingoti, R., Souza, M. L., et al. (2020). Further development of small hydropower may alter nutrient transport to the Pantanal Wetland of Brazil. Front. Environ. Sci. 8:577793. doi: 10.3389/fenvs.2020.577793
Ovidio, M., and Philippart, J. C. (2002). The impact of small physical obstacles on upstream movements of six species of fish: synthesis of a 5-year telemetry study in the River Meuse basin. Hydrobiologia 483, 55–69. doi: 10.1023/A:1021398605520
Pompeu, P. S., Agostinho, A. A., and Pelicice, F. M. (2012). Existing and future challenges: the concept of successful fish passage in South America. River Res. Appl. 28, 504–512. doi: 10.1002/rra.1557
Richter, B. D., Baumgartner, J. F., Powell, J., and Braun, D. (1996). A method for assessing hydrologic alteration with ecosystems. Cons. Biol. 10, 1163–1174. doi: 10.1046/j.1523-1739.1996.10041163.x
Rocaspana, R., Aparicio, E., Palau-Ibars, A., Guillem, R., and Alcaraz, C. (2019). Hydropeaking effects on movement patterns of brown trout (Salmo trutta L.). River Res. Appl. 35, 646–655. doi: 10.1002/rra.3432
Santucci, V. J. Jr., Gephard, S. R., and Pescitelli, S. M. (2005). Effects of multiple low-head dams on fish, macroinvertebrates, habitat, and water quality in the Fox River, Illinois. N. Am. J. Fish. Mgmt. 25, 975–992. doi: 10.1577/M03-216.1
Schulting, L., Feld, C. K., Zeiringer, B., Hudek, H., and Graf, W. (2019). Macroinvertebrate drift response to hydropeaking: an experimental approach to assess the effect of varying ramping velocities. Ecohydrology 12:12. doi: 10.1002/eco.2032
Timpe, K., and Kaplan, D. (2017). The changing hydrology of a dammed Amazon. Sci. Adv. 3, 1–13. doi: 10.1126/sciadv.1700611
Vehanen, T., Louhi, P., Huusko, A., Mäki-Petäys, A., Meer, O., Orell, P., et al. (2019). Behaviour of upstream migrating adult salmon (Salmo salar L.) in the tailrace channels of hydropeaking hydropower plants. Fish. Manag. Ecol. 27, 41–51. doi: 10.1111/fme.12383
Vollset, K. W., Skoglund, H., Wiers, T., and Barlaup, B. T. (2016). Effects of hydropeaking on the spawning behaviour of Atlantic salmon Salmo salar and brown trout Salmo trutta. J. Fish Biol. 88, 2236–2250. doi: 10.1111/jfb.12985
Xiao, X., Chen, X., Zhang, L., Lai, R., and Liu, J. (2019). Impacts of small cascaded hydropower plants on river discharge in a basin in Southern China. Hydrol. Proc. 33, 1420–1433. doi: 10.1002/hyp.13410
Keywords: hydroelectricity, dams, load following, tropical, hydrology, index of hydrological alteration
Citation: Figueiredo JSMC, Fantin-Cruz I, Silva GMS, Beregula RL, Girard P, Zeilhofer P, Uliana EM, Morais EB, Tritico HM and Hamilton SK (2021) Hydropeaking by Small Hydropower Facilities Affects Flow Regimes on Tributaries to the Pantanal Wetland of Brazil. Front. Environ. Sci. 9:577286. doi: 10.3389/fenvs.2021.577286
Received: 29 June 2020; Accepted: 03 March 2021;
Published: 25 March 2021.
Edited by:
Angela Helen Arthington, Griffith University, AustraliaReviewed by:
Teresa Ferreira, University of Lisbon, PortugalAntónio Pinheiro, Universidade de Lisboa, Portugal
Copyright © 2021 Figueiredo, Fantin-Cruz, Silva, Beregula, Girard, Zeilhofer, Uliana, Morais, Tritico and Hamilton. This is an open-access article distributed under the terms of the Creative Commons Attribution License (CC BY). The use, distribution or reproduction in other forums is permitted, provided the original author(s) and the copyright owner(s) are credited and that the original publication in this journal is cited, in accordance with accepted academic practice. No use, distribution or reproduction is permitted which does not comply with these terms.
*Correspondence: Ibraim Fantin-Cruz, aWJyYWltZmFudGluQGdtYWlsLmNvbQ==