- 1Tecnologico de Monterrey, Escuela de Ingenieria y Ciencias, Zapopan, Mexico
- 2Department of Biology, Valparaiso University, Valparaiso, IN, United States
Sustainable waste management is a key component needed to achieve multiple of the Sustainable Development Goals established by the United Nations for the year 2030. Such waste management can reduce the degradation of superficial water sources and can contribute to the sustainable and efficient use of resources. Due to their usually high nutrient and organic loads, agro-industrial wastes pose a threat to soil and water resources and are a major contributor to greenhouse gas generation. In this study, a novel approach was proposed to assess the environmental sustainability of vinasse management practices, integrating an extensive physicochemical characterization of tequila vinasses, a GIS-based weighted overlay analysis and a scenario analysis. Mathematical models were applied for the determination of discharged pollutants (nitrogen, phosphorus, BOD5), as well as greenhouse gas emissions. This methodology was applied to an environmental sustainability assessment of the management practices of tequila vinasses, which are one of the main waste streams generated by the agro-industry in the Mexican state of Jalisco and are one of the main contributors to organic and nutrient loads affecting the water quality of Jalisco’s superficial water sources. Through this integrated approach, critical regions for the management of tequila vinasses were determined and an extensive physicochemical characterization of tequila vinasses was performed and applied to assess the environmental sustainability of four management scenarios for tequila vinasses. These results can be used by decision-makers for the implementation of public policy and infrastructure for the improvement of local and regional waste management systems. Additionally, these data may be used to increase the environmental sustainability of the tequila industry. The proposed methodology has the potential to be applied to different waste-intensive regions as well as different organic waste streams for the assessment of the environmental sustainability of specific management practices considering the local and regional context.
Introduction
The management of agro-industrial waste poses a great challenge for production-intensive (and waste-intensive) regions, as the inappropriate disposal of large volumes of solid organic waste and wastewater can cause many environmental and health problems (Madurwar et al., 2013; Bedoić et al., 2019). Consequently, waste management is a key component to achieving multiple of the United Nations (UN) Sustainable Development Goals (SDG) established for 2030. The sustainable management of waste contributes to integrated water resource management for the protection and restoration of water-related ecosystems and to achieving the sustainable and efficient use of natural resources (UN, 2017).
Tequila is a fermented alcoholic beverage exclusively made from Agave tequilana Weber var. azul (DOF, 2012b). Tequila has been given a protected geographical status that restrains where the agave plants, and tequila itself, can be produced. As a result, tequila and agave plants can only be produced in certain municipalities in the Mexican states of Jalisco, Michoacán, Tamaulipas, Nayarit, and Guanajuato, as shown in Figure 1 (DOF, 2012a; CRT, 2019). Tequila is one of the most commonly consumed alcoholic beverages both in Mexico and globally (Bowen and Zapata, 2009; CRT, 2021). Mexico produces a yearly estimate of 1,501,081 tons of A. tequilana Weber var. azul over an approximate surface area of 87,000 ha. The state of Jalisco alone contributes 75% of the national agave production (SIAP, 2020). The total tequila production in 2019 reached an all-time high with 374 million liters. Seventy percent of the produced tequila is destined for exportation and the remainder is distributed nationally (CRT, 2021).
Tequila production can be divided into four stages: 1) agave processing and sugar extraction, 2) fermentation, 3) distillation, and 4) maturation and bottling (Cedeño, 1995). Tequila vinasses are the liquid sludge generated during tequila production and are usually made at a 7–15:1 ratio of vinasse to tequila volume (España-Gamboa et al., 2011). While all four production stages have a direct effect on the quality, character and sensory characteristics of the produced tequila, only the first three can affect the physicochemical characteristics of the vinasses generated (Cedeño, 1995; López-López et al., 2010). Although vinasses are mainly generated at the distillation stage, effluents from other production stages may also be included (Cedeño, 1995). Tequila vinasses present high concentrations of total solids (TS) as a result of the sugar extraction processes, like mill extraction and diffusion extraction, prior to the fermentation stage (Íñiguez-Covarrubias and Peraza-Luna, 2007), as well as a result of extremely high chemical oxygen demand (COD) concentrations and high acidity (España-Gamboa et al., 2011). Tequila vinasses also contain high concentrations of phenolic compounds that give them a characteristic dark brown color (López-López et al., 2010). These characteristics make vinasses a great environmental concern, as their incorrect disposal can cause significant environmental impacts (López-López et al., 2010). This is especially a concern in regions with high rates of Tequila production, such as in the state of Jalisco (Macías, 1997).
The high agricultural and agro-industrial activities of the state of Jalisco have led to increased exploitation and pollution of its water resources (Montoya et al., 1997; Abrol and Sharma, 2012). Eutrophic conditions caused by high nutrient and organic matter loads have been reported for multiple waterbodies across the state. This is mainly due to excessive uncontrolled solid waste and wastewater discharges by agro-industrial activities, leading to anoxic conditions and toxic cyanobacterial blooms, which can damage wildlife and even pose a threat to nearby settlements (de Anda et al., 2019; Díaz-Torres et al., 2020; Gradilla-Hernández et al., 2020). In order to reduce this eutrophication, the management practices of both solid waste and wastewater must be improved to decrease the amounts of organic matter and nutrient discharge going into Jalisco’s hydrographic systems (Montoya et al., 1997; Abrol and Sharma, 2012; Jayme-Torres and Hansen, 2018). Additionally, Jalisco has officially declared an aim for the year 2030 to reduce its current GHG (greenhouse gas) emissions by 22%, of which 14% have been attributed to waste and wastewater management (INECC, 2018; SEMADET, 2018).
The evaluation of environmental sustainability of waste management practices has been accomplished based on the following inputs: public and stakeholder opinion (Sahar and Ahmad, 2020), overall waste production estimation and management costs analysis (Daian and Ozarska, 2009), combined Life Cycle Analysis (LCA) and Analytical Hierarchy Process (AHP) (Lijó et al., 2018), combined LCA and Resource Conservation Efficiency (RCE) determination (Kaufman et al., 2010), waste flow mapping (Kurdve et al., 2015), and carbon footprint assessment (Ibanescu et al., 2018), among others. However, most waste management environmental sustainability studies usually lack practical application, as they do not provide an integral approach based on the local geographic, environmental and socioeconomical conditions, while maintaining replicability for other regions or waste streams (Bani et al., 2009). New integrated methodologies are required to generate adaptative environmental sustainability assessments that can be easily applied by decision-makers to establish public policy and infrastructure to improve local and regional waste management systems, especially for waste-intensive regions.
As a contribution to the literature, this study presents a novel approach to spatially characterize the environmental impacts (nutrient and organic matter discharge and GHG production) of the tequila vinasses generated in the state of Jalisco. This work herein also quantitively assesses different management scenarios, combining the physicochemical characterization of vinasses collected in field, GIS-based multicriteria analysis and scenario analysis. Due to the lack of academic literature regarding the spatial variations of the physicochemical characteristics of tequila vinasses, an extensive physicochemical characterization of Tequila vinasses was carried out including samples from Tequila Production Units (TPU) across the state of Jalisco. In order to identify the most critical regions to establish vinasse management strategies, a weighted overlay analysis (WOA) was implemented to evaluate the potential pollution risk for the entire territory of the state, integrating five productive and environmental parameters: 1) vinasse production at the nearest tequila production unit (TPU), 2) total vinasse production for the municipality, 3) distance to the nearest TPU, 4) distance to the nearest water body and 5) distance to the nearest urban community. Furthermore, the environmental impacts generated as a result of the current management practices of tequila vinasses were evaluated in addition to the environmental benefits that could be gained if other management practices were implemented. This was accomplished using information generated through the physicochemical characterization and focusing on the critical regions previously determined through the WOA. Nutrient (nitrogen and phosphorus) and organic matter discharge estimations, as well as GHG production estimations were performed for the critical regions as well as for the entire state, based on the existing models reported for the management conditions of each scenario.
Methods
The general methodology used in the present study is shown in Figure 2.
Data Collection and Processing
The geographic information and the annual production of tequila production units (TPU), expressed in m3 year−1, was obtained from the National Statistic Directory of Economic Units in Mexico (DENUE by its acronym in Spanish) (INEGI, 2021a) and validated with official records provided by the Environment and Land Development Ministry of the state of Jalisco (SEMADET by its acronym in Spanish) (SEMADET, 2019b). When possible, this information was further corroborated directly with the producers and updated. TPUs were then classified into production categories according to the internal classification system of the Tequila Regulatory Council (CRT by its acronym in Spanish). This classification was based on the tequila production volumes presented in Table 1, and production records were used to estimate the production of vinasses for each individual TPU. The current management practices of vinasses within the TPUs in the state were also identified from official records (SEMADET, 2019b) in order to ascertain the most common practices by the production category according to the CRT classification system for the TPUs (Table 1).
Information related to the hydrographic structure, runoff network, digital elevation model, official municipal limits, and location of urban areas within the study region was obtained from the National Institute of Statistics and Geography (INEGI) (INEGI, 2021b). A geographical information system (GIS) was created on ArcGis 10.5 to integrate the data corresponding to the TPUs and the previously mentioned environmental and geographical parameters. All raster images had a 15 m × 15 m resolution.
Selection of Parameters for the WOA
The critical regions for the management of tequila vinasses were determined based on vinasse production records and environmental parameters. A weighted overlay analysis allows for the integration of multiple classified raster layers into a single weighted resulting raster based on a weighted index matrix to classify the evaluated parameters (Yazdani et al., 2015; Al-Anbari et al., 2018). A weighted index matrix was generated to classify and rank the rasters comprising each individual point within the territory of Jalisco according to their potential risk of environmental degradation as a result of inadequate management of vinasses. The following parameters were transformed into raster format for the weighted overlay analysis for the establishment of the critical regions: 1) vinasse production at the nearest TPU, 2) total vinasse production for the municipality, 3) distance to the nearest TPU, 4) distance to the nearest water body, and 5) distance to the nearest urban community. A similar approach was reported by (Díaz-Vázquez et al., 2020). These parameters were selected as they are commonly used for the evaluation of the pollution risk as well as the spatial suitability for the implementation of management strategies and infrastructure for organic waste and wastewaters (Comber et al., 2015; Mukherjee et al., 2015; Venier and Yabar, 2017; Díaz-Vázquez et al., 2020). These parameters are further explained in the following paragraphs.
(1) Vinasse production rate at the nearest TPU.
The rate of vinasse production at each individual TPU (V) was determined based on the mean, minimum, and maximum production ratio of vinasses (liter of vinasses per liter of tequila) (R) previously reported in the literature (Table 2) and the tequila production volume reported for each individual TPU (T), as shown in Eq. 1. The mean production rates of vinasses were used for the WOA based on the mean production ratio of vinasses (Rmean) reported in Table 2.
TPUs can act as both point and non-point sources of pollution to water sources, as the generated vinasses can be discharged directly from the TPUs into water bodies and municipal wastewater systems or they can be transported for field application as fertilizers and then be transported to water bodies by rainfall-runoff processes (Fuess and Garcia, 2014). Therefore, the vinasse production rate at the nearest TPU has a great influence on the pollution risk at every point in the territory.
(2) Total TPUs within the municipality
The total count of TPUs for each municipality in Jalisco was determined based on the TPU inventories. The TPU density within each municipality was included in the analysis as it is related to the density of vinasses that require to be disposed of within the municipal area. Additionally, according to current legislation, all waste of special management is the responsibility of the state, including vinasses (DOF, 1988; SADER, 1989). Therefore the state has the authority to set specific guidelines and regulations for the management of special wastes, as well as the right to establish strategies and region-specific programs in order to improve management of high priority waste, like vinasses (DOF, 1988; SADER, 1989). Higher densities of TPU can facilitate the implementation of pollution prevention strategies within specific regions or small clusters of TPUs rather than at a state level. Additionally, high densities of point sources can lead to the accumulation of pollutants in the areas nearby. Therefore, it is important to locate possible high-density regions in order to establish preventive measures and regulations to reduce pollution risk (Nobre et al., 2007).
(3) Distance to the nearest TPU
The distance from every point in the territory in Jalisco to the nearest TPU was determined using the Euclidean distance tool in ArcGIS 10.5. High production rates of vinasses, and organic waste in general, imply elevated transport costs due to the high water content of vinasses. As a consequence, vinasses tend to be disposed of and accumulated in areas nearby the TPUs in an attempt to reduce disposal costs (Carral et al., 2011; Shahmoradi and Isalou, 2013; Fuess and Garcia, 2017; Díaz-Vázquez et al., 2020). This leads to soil degradation, waterbody eutrophication and, in extreme cases, health hazards for nearby populations (Fuess and Garcia, 2014; Chowdhary et al., 2018).
(4) Distance to the nearest waterbody
The distance from every point in the territory in Jalisco to the nearest water body or water flow were determined using the Euclidean distance tool in ArcGIS 10.5 based on the hydrological data available for the state of Jalisco (INEGI, 2021b). The disposal of vinasses can have a direct effect on the water quality of nearby superficial water bodies (Carral et al., 2011; Shahmoradi and Isalou, 2013). Therefore, it is important to determine how close the evaluated point in the territory is to the nearest superficial waterbody.
(5) Distance to the nearest urban community
The distances from every point in the territory of Jalisco to the nearest urban area were determined using the Euclidean distance tool in ArcGIS 10.5 based on the geographical data available for the state of Jalisco (INEGI, 2021b). The disposal of vinasses to local sewage systems can overload the treatment capacity of local wastewater treatment plants due to the high concentration of pollutants in vinasses. While this is a common management strategy used by TPUs located within urban areas (Beltrán et al., 2001), current legislation requires that each TPU is responsible for the management and disposal of their wastewaters and should not transfer this responsibility to municipal wastewater treatment plants (DOF, 1988; SADER, 1989). Additionally, the accumulation of untreated vinasses in soils and water bodies can generate foul odors due to organic matter degradation (Carral et al., 2011; Shahmoradi and Isalou, 2013).
Development of Classified Raster Images
Raster images were constructed for the five selected parameters. The raster pixels within each raster image of each selected parameter were classified into non-dimensional values ranging from 1 to 5 according to the ranges presented in Table 3. These values were assigned based on how each of the selected parameters increases the risk of environmental pollution from the inadequate management of vinasses at every point in the territory of Jalisco. 1 was assigned to the areas with the lowest pollution risk, and 5 represents the highest pollution risk (Al-Anbari et al., 2018; Díaz-Vázquez et al., 2020).
To evaluate the overall risk of pollution as a result of the inadequate management of vinasses, at every point in the territory, weights were assigned to each parameter based on the literature and studies that evaluate similar parameters (Yager, 1988; Al-Hanbali et al., 2011; Shahmoradi and Isalou, 2013; Vaissi and Sharifi, 2019; Díaz-Vázquez et al., 2020). A WOA was performed using the five generated classified raster images according to Eq. 2, where RF represents the risk factor for every individually analyzed pixel (1–5 scale), Wi represents the assigned weight for the selected parameter and Si represents the reclassified raster value of the selected parameter (1–5) for the analyzed pixel. Weights were distributed evenly between all parameters based on previous studies (Yager, 1988; Al-Anbari et al., 2018; Vaissi and Sharifi, 2019; Díaz-Vázquez et al., 2020). The WOA was performed on ArcGIS 10.5 using the Weighted Overlay Analysis tool.
The resulting classified raster, with a 15 m × 15 m resolution, represents the overall pollution risk in each point of the territory (each pixel on the map) considering all five selected parameters expressed on a 1–5 scale. Contiguous municipalities showing zones with a pollution risk of 5 were classified as critical regions.
Collection of Vinasses and Physicochemical Characterization
The physicochemical characterization of vinasses was required due to the lack of information regarding the spatial variability of tequila vinasse physicochemical characteristics in the existing literature. Vinasses were collected from 20 TPUs distributed across the previously identified critical regions. A stratified sampling was performed to include all TPU size categories within the study. 5 L grab samples were taken the same day that these were produced, transported in refrigeration (4°C) for less than 5 h and kept at 4°C until further processing within the next 24 h, as established by the applicable regulation (DOF, 1997). No composite samples were prepared as all samples were analyzed individually in duplicate. Hydrogen potential (pH) was measured at the collection site by the potentiometric method while total suspended solids (TSS), settleable solids (SS), biological oxygen demand (BOD5), total Kjeldahl nitrogen (TKN), total phosphorus (TP), and fat oil and grease (FOG) were determined upon arrival by the Analytical and Metrologic Services Unit (USAM by its by its acronym in Spanish) of the Centro de Investigación y Asistencia Tecnológica del Estado de Jalisco (CIATEJ). TSS and SS were determined by gravimetric methods, BOD5 was determined by the respirometric method, TKN was determined using the Kjeldahl method, TP was determined by the persulfate digestion–molybdovanadate method, and FOG was determined by the Soxhlet extraction method. All determinations were made following the Federation, W. E., and American Public Health Association methods (Eaton et al., 2005).
A physicochemical characterization was required for the evaluation of the management scenarios. One-way ANOVAs and Tukey tests were performed on Minitab 19.2020.1 to determine significant spatial differences between the sampled vinasses collected from different regions of the state of Jalisco considering each of the water quality parameters (pH, BOD5, FOG, TKN, TP, and TSS). The same analyses were performed to determine possible differences in the vinasses produced by different TPU size categories (Table 1).
Management Scenarios Analysis
Four scenarios (A, B, C, and D) were proposed based on the most frequent vinasse management practices reported for Jalisco and according to the productive records provided by SEMADET (SEMADET, 2019b) as well as the most effective vinasse management practices reported in the literature (López-López et al., 2010; España-Gamboa et al., 2011; Robles-González et al., 2012; Moraes et al., 2017). Complete scenario descriptions are shown in Table 4.
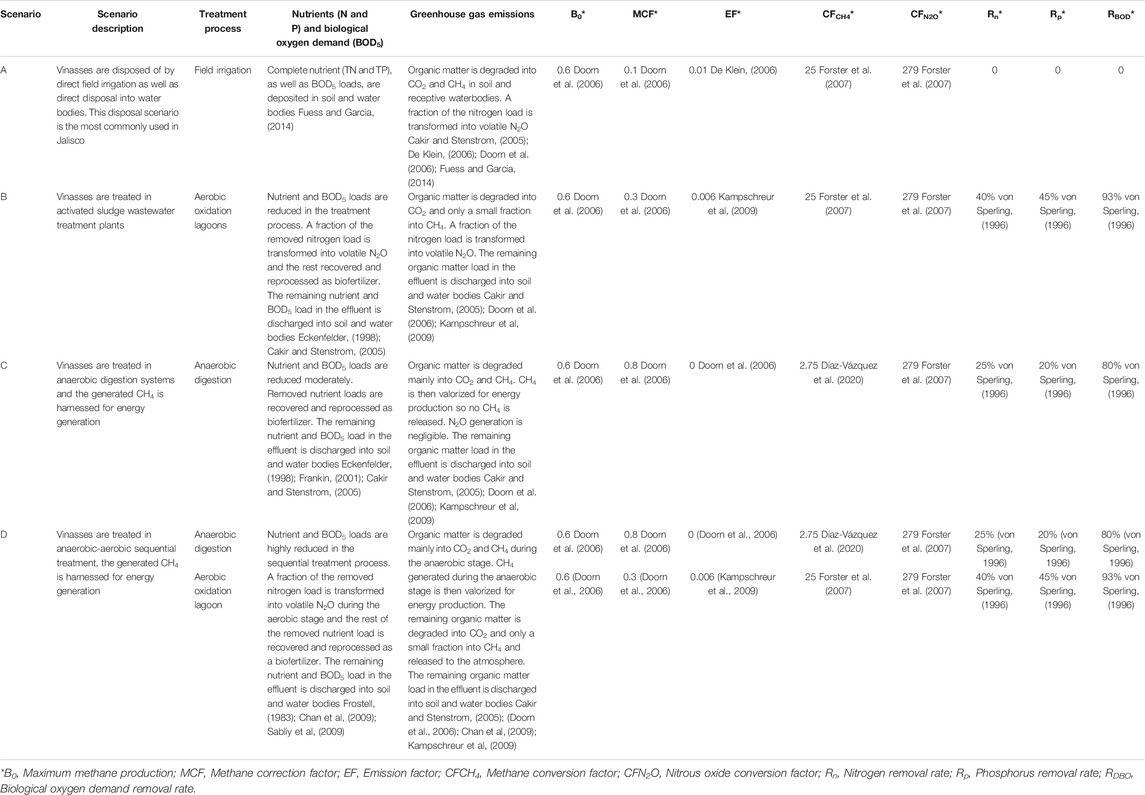
TABLE 4. Scenario description and parameters used for the calculation of the nitrogen and phosphorus released to the environment and the CO2eq emissions for each proposed scenario.
The potential nutrient and organic matter release into the environment, as well as the potential GHG emissions (CO2eq) from the disposal of the vinasses within the critical regions, were determined based on the characterization of vinasses performed in the present work for the four proposed scenarios (A, B, C, and D).
Nutrient Release to the Environment
The total vinasse production, TN production, and TP production were estimated based on the total production volumes reported for each individual TPU and the physicochemical characterization of the sampled vinasses using the minimum, mean and maximum values (for both vinasse production rates and physicochemical parameters) as shown in Table 1 and Table 6, for each of the critical regions. These estimations are total nutrient productions without the differentiation of the bioavailability.
The total nitrogen (NT) (Mg year−1) potential release was determined according to Eq. 3 for each scenario. This was based on the total vinasses generated (V) (m3 year−1) in each critical region and the mean, minimum, and maximum nitrogen concentration in the sampled vinasses (N) (Mg m−3) displayed in Table 6. The nitrogen load (ND) (Mg year −1) in the discharged effluent was determined according to Eq. 4, where RN (nondimensional) represents the nitrogen removal rate under each scenario.
The total phosphorus (PT) (Mg year−1) potential release was determined following the same method used for the calculation of the total nitrogen potential release, as shown in Eq. 5, where V is the total vinasse generation (m3 year−1) in each critical region and P is the phosphorus (mean, minimum, and maximum) composition of the sampled vinasses (Mg m−3) as displayed in Table 6. The phosphorus load in the discharged effluent (PD) (Mg year−1) was determined according to Eq. 6, where RP (Nondimensional) represents the phosphorus removal rate under each scenario.
Organic Matter Release and Greenhouse Gas Emissions
The mean, minimum and maximum organic matter content present in the untreated vinasses and the discharged effluent for each respective scenario was calculated according to Eqs. 7, 8, respectively. BODT (Mg year−1) represents the total BOD5 content in the untreated vinasses according to BOD5 (Mg m−3) values shown in Table 4. These were obtained as a result of the physicochemical characterization of the sampled vinasses. BODD (Mg year−1) represents the remaining BOD5 present in the discharged effluent after the proposed treatment, according to the specific organic matter removal factors of each process as reported in the literature (Table 4).
The mean, minimum and maximum GHG emissions (GHGi) (Mg CO2eq year−1) generated though the proposed scenarios were calculated for each scenario (A, B and C, and D, respectively) according to Eqs. 9–11, using the mean, minimum and maximum values from the vinasse production rates and the physicochemical characterization. These equations were adapted from the IPCC Guidelines for National Greenhouse gas inventories based on process specific factors, summarized in Table 4 (Doorn et al., 2006). The factors proposed by the IPCC are region specific in most cases and, thus, the Latin-America values were used when available. When not available a mean parameter was used, as suggested by the IPCC (De Klein, 2006; Doorn et al., 2006).
Bo (nondimensional) is the most commonly used methane production capacity in the calculation of GHG inventories, which indicates the fraction of organic matter that can be transformed to CH4 and tends to be independent of the degradation process and its conditions, assumed as 0.6 (Doorn et al., 2006). MCF (nondimensional) represents the methane conversion factor used to estimate the conversion of BOD5 into methane under specific degradation conditions (Doorn et al., 2006). V (m3 year−1) represents the generation of vinasses in the individual TPU. NT (Mg year−1) represents the nitrogen concentration in the untreated effluent, and ND (Mg year−1) represents the nitrogen present in the discharged effluent after the proposed treatment. The fraction of nitrogen transformed and released into the atmosphere as N2O is expressed as EF (nondimensional). Both CFCH4 (nondimensional) and CFN2O (nondimensional) represent the conversion factors used to transform the CH4 and N2O into CO2eq, respectively. Eqs. 9–11 were adapted from the equations proposed by De Klein, (2006) and Doorn et al. (2006), as they are the most common methods for estimating GHG resulting from waste management and agricultural practices (De Klein, 2006; Doorn et al., 2006). The GHG estimation for scenarios C and D includes only the direct GHG generated by organic matter and nutrient degradation; it does not include possible GHG reductions due to energetic need reduction or even energetic surplus due to the energetic revalorization of CH4.
Results
Characterization of the Tequila Production Sector and of Current Producer Management Practices
The total production of tequila in Jalisco is accomplished by 158 TPUs, from which 109, 17, 14, and 18 units are categorized as micro, small, medium, and large, respectively. This is according to the internal classification system used by the CRT, which is based on the tequila production volume shown in Table 1. The mean estimated vinasse production rate in Jalisco was found to be 2’645,655.69 m3 year −1. The TPUs classified as large contribute 84.3% of the state vinasse production, while the remaining 15.7% is generated by micro, small and medium TPUs contributing with 1.7, 4.1, and 9.8% respectively. Almost 80% of the state’s vinasse production is accomplished by only seven municipalities: Tequila, Zapotlanejo, Atotonilco el Alto, Amatitan, Arandas, Tlaquepaque, and Tototlan. The complete vinasse production and size distribution for the municipalities responsible for 80% of the vinasses within the state is displayed in Table 5. Tequila and Amatitan are located within the middle-western region of the state, Atotonilco, Arandas and Tototlan belong to the eastern region, and Zapotlanejo and Tlaquepaque are located in the central region of the state.
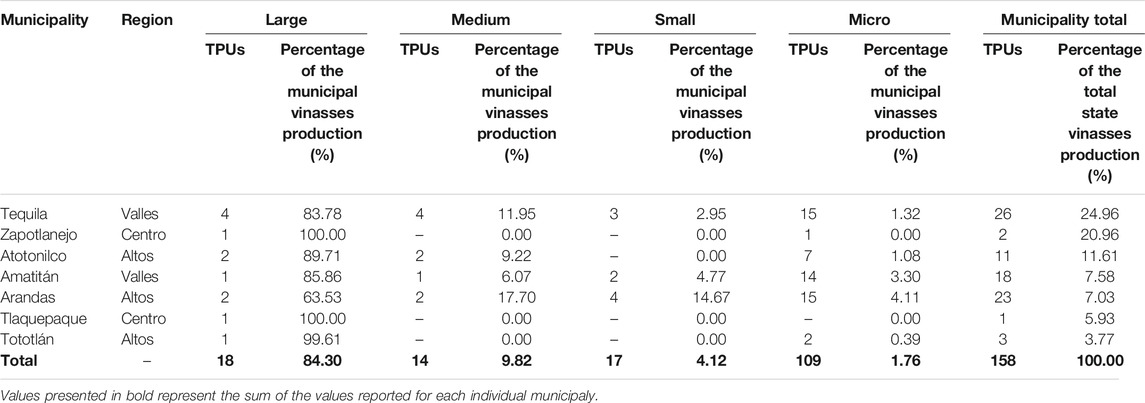
TABLE 5. TPUs and vinasses production within the municipalities responsible for 80% of the vinasses within the state of Jalisco.
Most small, micro, and medium TPUs used pH neutralization and direct field application as their management practices for vinasses, while large TPUs used a variety of treatment alternatives, mainly categorized as biological treatment (aerobic or anaerobic) and, with a lower frequency, sequential anaerobic-aerobic treatment.
Critical Region Determination
Five classification maps were generated, each corresponding to one of the selected parameters (Figure 3) used to evaluate the risk of environmental degradation due to inadequate management of tequila vinasses. High values (5), which indicated a higher risk of pollution, were located within the middle-western and eastern regions of the state of Jalisco. This can be attributed to the higher density of TPUs, and their high tequila production rates, within these regions as well as the abundance of urban settlements across these areas.
As a result of the WOA, a classification map was obtained to identify the critical regions (Figure 4) weighting all five selected parameters. Two main critical regions were found to be located in the western region of the state (Valles region) and in the eastern region of the state (Altos region). These critical regions, Valles and Altos, have the highest possible risk of environmental pollution as a result of nutrient and organic matter release and the highest potential to produce GHG emissions resulting from poor management of the tequila vinasses. The contiguous municipalities displaying critical zones (Figure 4) were selected and clustered, as shown in Figure 5. The Valles critical region comprises the municipalities of Tequila, Amatitan, and El Arenal, while the Altos critical region consists of the municipalities of Tepatitlan de Morelos, Atotonilco el Alto, Arandas, and Jesus Maria, as displayed in Figure 5. The municipalities of Tequila, Amatitan, Atotonilco el Alto, and Arandas belong to the municipalities responsible for 80% of vinasse production in the state, as shown in Table 5.
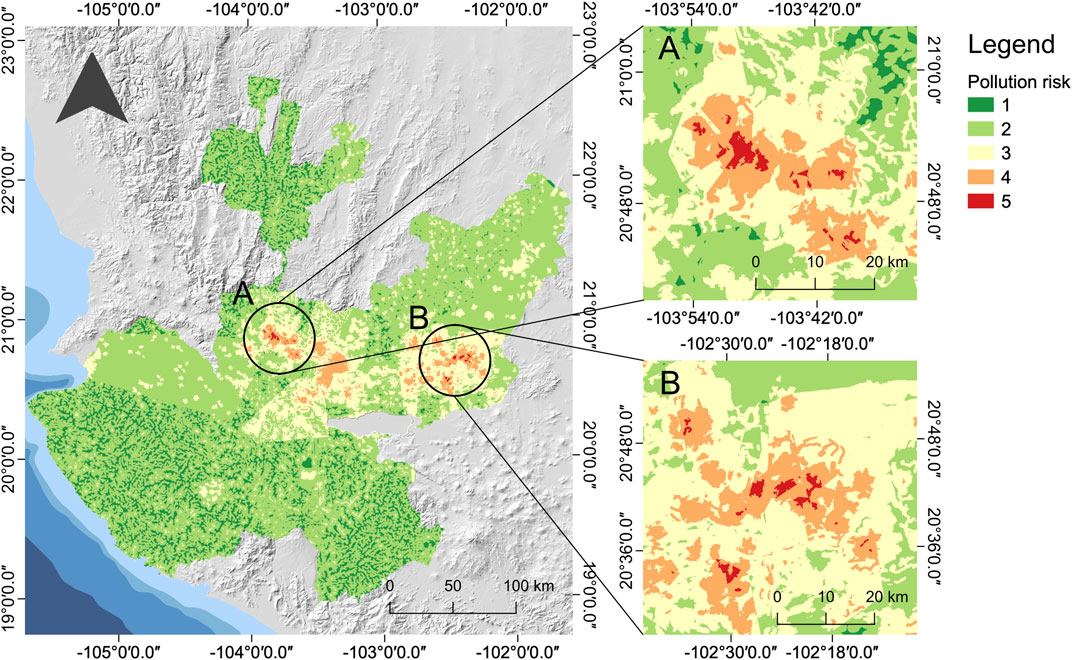
FIGURE 4. Critical zones determined within the middle-western (A) and eastern (B) areas of the state of Jalisco.
Characterization of Vinasses
The results of the physicochemical characterization, summarized by descriptive statistics, of vinasses collected at 20 TPUs distributed across the critical regions are displayed in Table 6. Additionally, based on the current Mexican legislation (DOF, 1997), the newly proposed Mexican legislation (DOF, 2018), the European Union water directive (Council directive, 2014) and the EPA guidelines for centralized waste treatment point discharges (EPA, 2021), the permissible limits are shown to compare the results to the applicable federal discharge guidelines as well as to international standards. While the physicochemical characteristics of vinasses from other alcoholic production processes (molasses, sugarcane, sugar beet, etc.) are well documented (España-Gamboa et al., 2011; Christofoletti et al., 2013; Chowdhary et al., 2018; Mikucka and Zielińska, 2020), there is a lack of information regarding tequila vinasses because most of the existing reports on the physicochemical characteristics have been obtained from single production units and thus fail to represent the variability in vinasse characteristics resulting from a wide variety of productive processes used across the state (Íñiguez-Covarrubias and Peraza-Luna, 2007; López-López et al., 2010).
The pH values of the sampled vinasses exhibited the lowest coefficient of variation (CV = 5%) while FOG displayed the highest coefficient of variation of all analyzed parameters (CV = 158%). This indicates that variations within the tequila production process have a great influence on the FOG content of the discharged vinasses. TP and SS also presented elevated coefficients of variation, at 156 and 133%, respectively.
According to Table 6, vinasses surpass the permissible discharge limits established in the current Mexican legislation for all of the regulated parameters. Furthermore, neither of the vinasse samples were within acceptable limits for any of the seven analyzed parameters according to current legislation. Therefore, a treatment process would be required in order to fulfill the current local legislation standards (DOF, 1997). The local permissible limits for wastewater discharge are currently under a revision process since a new legislation was recently proposed (DOF, 2018). New lower permissible limits may be established within the short term due to the new legislation proposal, which would increase the need for effective wastewater management strategies in order to fulfill the new standards that not only reduce current permissible limits but add new parameters to be regulated, as is the case of color and toxicity (DOF, 2018). Both international discharge guidelines presented in this study (Table 6) exhibit lower limits than the current local legislation, making it unacceptable to discharge untreated vinasses under those guidelines (Council directive, 2014; EPA, 2021).
The mean concentrations of phosphorus and nitrogen (42.01 mg L−1 and 131.63 mg L−1) were closer to the current permissible limits, 40 and 110% higher than the current legislation, in comparison to the mean concentrations for other parameters. However, when compared to international standards, tequila vinasses display concentrations of phosphorus and nitrogen that were 2,100 and 400% higher than the permissible limits set for the European Community (Council directive, 2014). BOD5 is the water quality parameter that most highly exceeded the current local and international guidelines, reflecting the extreme organic load in the vinasses and emphasizing the need for effective vinasse management practices in order to prevent environmental degradation.
From the seven analyzed parameters, significant mean differences between the vinasses produced by TPUs of different size categories were only found when considering SS (p = 0.011). No significant differences were found between the vinasses produced in the different regions (Altos and Valles) of the state of Jalisco, indicating there is no difference between the vinasses generated in either of the tequila producing regions.
Critical Regions and Scenarios Analysis
Table 7 displays the estimated vinasse production volumes for the municipalities within the established critical regions. The complete scenario-based estimations for organic matter, nutrient and GHG release is shown in Tables 8, 9 for both Altos and Valles critical regions as well as for the entire state of Jalisco.
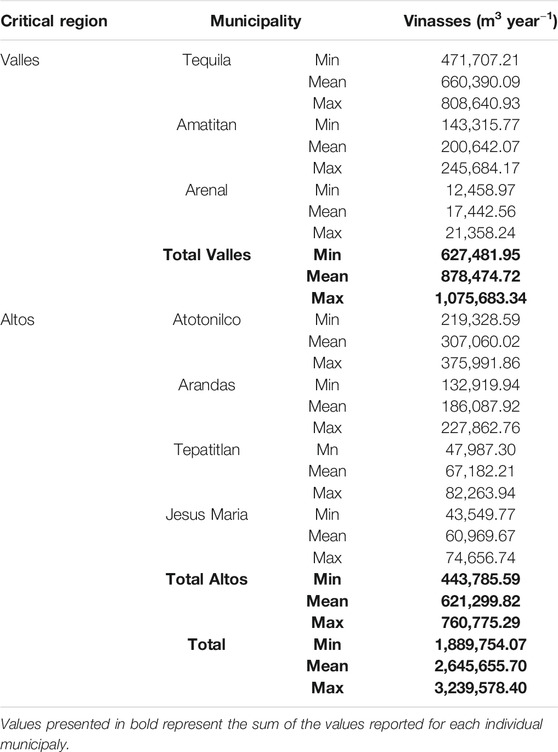
TABLE 7. Estimated vinasse production rates for the municipalities within the established critical regions.
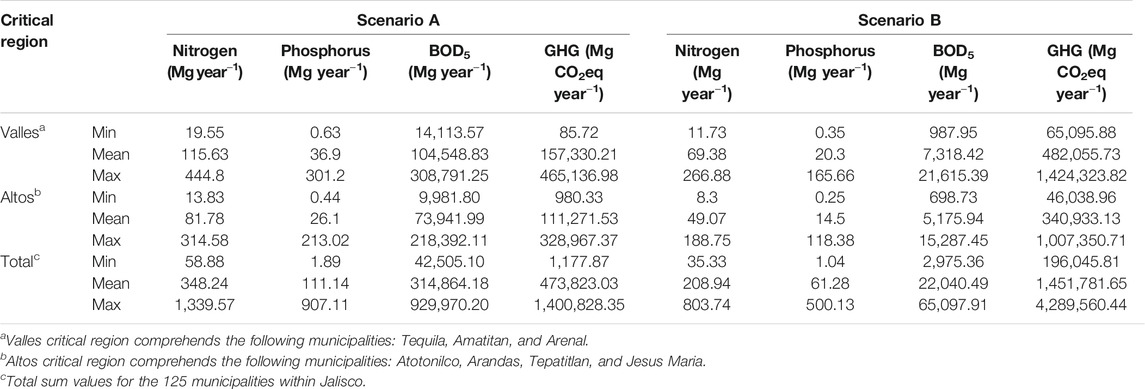
TABLE 8. Pollutant discharge potential values calculated for the established critical regions under scenarios A and B.
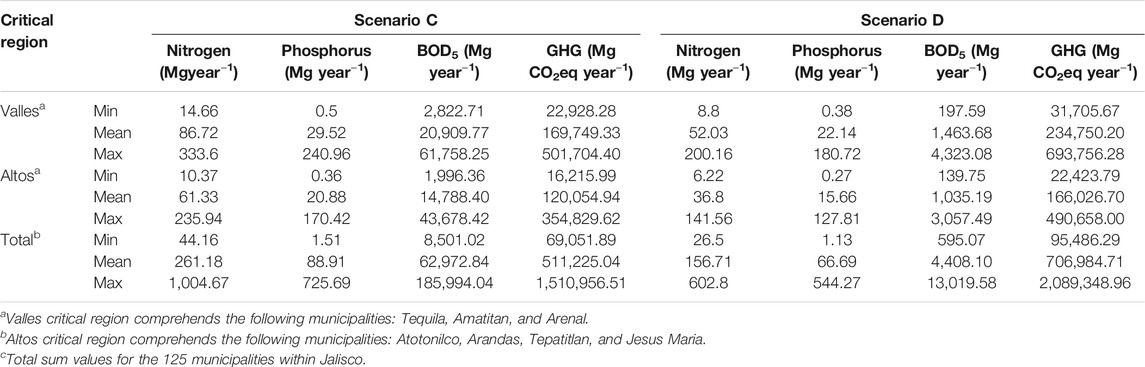
TABLE 9. Pollutant discharge potential values calculated for the established critical regions under scenarios C and D.
Discussion
Critical Regions for the Management of Vinasses
All of the tequila producers registered in the official records in Jalisco were classified in four categories, as shown in Table 1. Similar proportions of all categories (micro, small, medium, and large) were found for both critical regions, Valles and Altos (SEMADET, 2019b) While most of the vinasses generated in Jalisco are produced by large TPUs (83%), a great majority of these producers already report vinasse treatment systems that offer complete or partial treatment. Large producers generally have the resources and technical knowledge to implement effective wastewater management systems. However, medium, small, and micro-producers generally lack these resources and technical knowledge and thus release higher volumes of untreated vinasses discharged to soils and waterbodies across the state (SEMADET, 2019b).
Both the critical regions determined in this study displayed similar overall vinasse production volumes, as shown in Table 7. However, these regions present different spatial configurations. Most of the TPUs within the Valles critical region are in a smaller area (within a 5 km radius, approximately), which favors the implementation of centralized treatment units. This is not only because of the constant supply of vinasses, but due to the relatively short distances between most TPUs. The TPUs within the Altos critical region are more dispersed across the region (within a 30 km radius), which can be a disadvantage for the implementation of large-scale centralized treatment units due to higher transport costs (Flotats et al., 2009; Fuess and Garcia, 2014). Areas with lower densities of wastewater production may require different approaches in order to achieve effective and economical wastewater management like small-scale clustering or other diverse onsite treatment options (Massoud et al., 2009).
Besides being the top Tequila producer, Jalisco is the leading state for the production of crops and livestock in Mexico (SIAP and SAGARPA, 2018). This agricultural productivity increases the overall organic waste and nutrients released to the environment as a result of inadequate management practices (Montoya et al., 1997; Martinez Rivera, 2004; Jayme-Torres and Hansen, 2018; Díaz-Vázquez et al., 2020). Both critical regions identified in this study, Altos and Valles, display high agricultural and industrial activity that influences the soil and water quality of the region. Additionally, the tequila industry in these regions generates larger volumes of agro-industrial waste compared to other industrial categories and therefore has a great influence on the environmental degradation (Montoya et al., 1997; SEMADET, 2017; Jayme-Torres and Hansen, 2018). Furthermore, these regions are located within the Santiago river basin, which is known to be highly polluted with nitrogen, phosphorus, and organic matter (Abrol and Sharma, 2012). The spatial identification of critical regions can be a powerful tool to identify areas that require special policies or management in order to prevent environmental degradation or health hazards to nearby populations (Delivand et al., 2015; Díaz-Vázquez et al., 2020). Although it is out of the scope of the present work, the identification of waste-intensive regions would open up the possibility for the implementation of synergic waste management systems. Different waste streams could then be integrated and revalorized to higher levels than the ones from individual revalorization of waste streams. One example of this is the anaerobic co-digestion of waste, where the biogas yields from mixed waste stream feeds are significatively higher than the ones from individual waste feeds (Muhammad and Chandra, 2021; Rincón-Pérez et al., 2021).
Potential Environmental Effects of Untreated Vinasses
As shown in Table 6, the mean pH of the analyzed samples is within the range previously reported for tequila vinasses (3.4–4.5) (López-López et al., 2010). If vinasses are discharged without previous pH neutralization, the receptive soils and water bodies can be severely affected due to the high acidity of this agro-industrial waste (Fuess and Garcia, 2017). Acidification of soil and water is known to increase nutrient mobility, altering its uptake in crops as well as increasing its transport by runoff or leaching to receptive waterbodies or groundwater, respectively (World Health Organization, 2006). The most common method used by TPUs to regulate this parameter is to utilize neutralizing agents, such as CaO, CaOH2, and NaOH. However, special care must be taken, as large amounts of neutralizing agents can increase the content of specific ions in the effluent (Fuess and Garcia, 2017; Chowdhary et al., 2018), which over time can cause soil degradation, heavy metal transport, as well as damage to aquatic species due to increased ion concentrations in receptive waterbodies (Dickman and Christy, 2002; Fuess and Garcia, 2014; Fuess and Garcia, 2017).
The mean BOD5 value (Table 6) in the sampled vinasses (119,011.77 ± 104,097.12 mg L−1) was greater than previously reported values for tequila vinasses as well as for other vinasses (20,600–60,000 mg L−1) (López-López et al., 2010; España-Gamboa et al., 2011). The higher BOD5 concentrations in the sampled vinasses can be linked to the high TSS content also found in the characterized vinasses (9,875.80 ± 11,958.41). This is due to the fact that TSS in tequila vinasses are mainly composed of organic unfermentable solids like cellulose and pectin, which are present in the agave juice that was not degraded during the alcoholic fermentation stages (Íñiguez-Covarrubias and Peraza-Luna, 2007; España-Gamboa et al., 2011). The mean TSS content in the sampled vinasses (9,875.80 mg L−1) is above the higher limit reported by López-López (2000–8,000 mg L−1), possibly due to the mechanical extraction processes applied by the specific TPUs. Mechanical sugar extraction processes, such as mill extraction, increase the total unfermentable solid content in agave juice, which is discarded in the vinasse effluent during the distillation stage (Íñiguez-Covarrubias and Peraza-Luna, 2007; Tequila Sauza, 2021). The implementation of other sugar extraction processes, such as diffusion extraction, can reduce the solid load in the produced vinasses as well as increase sugar availability during the fermentation stage (Tequila Sauza, 2021). The TSS content in the vinasses represents an important fraction of a vinasse DBO5 and can directly have negative effects in both soils and receptive waterbodies if discharged without previous treatment. High BOD5 values can significantly reduce the dissolved oxygen levels in receptive waterbodies due to microbial proliferation (EPA, 2001). Over time, this increases GHG production due to the production of metabolic byproducts like CH4 and CO2 (Doorn et al., 2006). Significant differences were identified in the SS mean values. Micro and small producers generate lower volumes of vinasses compared to medium and large producers and usually store them for longer periods prior to treatment or disposal (SEMADET, 2019b). Therefore, the settleable solid content in the sampled vinasses could have been affected due to natural sedimentation during the time prior the sampling.
The TKN content (Table 6) in the analyzed vinasses (131.63 ± 97.59 mg L−1) was approximately three times higher than the previously reported values for tequila vinasses (20–50 mg L−1). However, it was still lower than the values reported in the literature for vinasses from mezcal production, which uses different varieties of agave; likewise, the TKN content reported herein was lower than in vinasses obtained from the fermentation of other substrates like corn, beet, and sugarcane (660–5,650 mg L−1, 2,000–2,680 mg L−1, 1,800–4,750 mg L−1, and 1,000–7,000 mg L−1, respectively) (Robles-González et al., 2012; Fuess and Garcia, 2017). An important fraction of the total nitrogen content in tequila vinasses is in an organic form, which reduces its potential as a crop fertilizer since it requires mineralization by soil microorganisms to be of use for crop fertilization (Fuess and Garcia, 2017). Organic nitrogen is mineralized in soils or water bodies, into ammonium which can be easily used by plants as a nitrogen source. However, high ammonium concentrations may lead to elevated concentrations of nitrites and nitrates in soil and waters, causing eutrophication, or may also cause increased N2O emissions through nitrification or denitrification processes (Kirchman, 2018). The mean phosphorus content of the tequila vinasses analyzed in the present study (42.01 mg L−1) corresponds to the values reported for tequila vinasses by España-Gamboa (40 mg L−1) (España-Gamboa et al., 2011). Tequila vinasses present lower phosphorus contents compared to vinasses obtained from other common substrates (71–1990 mg L−1) (sugar cane, sorghum, sugar beet, molasses, etc.), which can be attributed to the natural phosphorus content in these other substrates (España-Gamboa et al., 2011). Because phosphorus exhibits lower volatility compared to nitrogen, there is the risk of accumulation of phosphorus in soil and receptive waterbodies if vinasses are inadequately managed (World Health Organization, 2006) either by the direct discharge of or by the transport of accumulated phosphorus in the soil through superficial runoff and groundwater (Fuess and Garcia, 2017). The disposal of untreated vinasses by field irrigation or direct discharge to water bodies can be a powerful driver for phosphorus accumulation due to its excessive phosphorus content and reduced volatilization rates, leading to eutrophication of water bodies due to surface runoff and groundwater phosphorus transport (Carpenter et al., 1998; Fuess and Garcia, 2017).
The FOG mean values (Table 6) obtained in the present study (301.14 mg L−1) were 300% higher than the upper limit of the reported range by López-López for tequila vinasses (10–100 mg L−1) (López-López et al., 2010). The high coefficient of variation (Table 6) (CV = 158%) found for FOG in this study is of special interest since the lower measured values of FOG in the analyzed vinasses fall within the acceptable limits for both current and proposed legislation. The cooking process used for the agave heads may be related to the high variation in the FOG values measured in the analyzed vinasses since the agave heads cuticles release waxy compounds under heat treatment. Prior to sugar extraction by milling, agave heads are cooked mostly either by oven cooking or autoclaving (Cedeño, 1995). The use of diffusion extraction, on the other hand, may prevent the release of waxy compounds into the sugar-rich agave juice and can significantly reduce the content of FOG in the vinasses (Cedeño, 1995; Tequila Sauza, 2021). However, only a small fraction of producers have implemented extraction alternatives like diffusion (Cedeño, 1995; Tequila Sauza, 2021). When vinasses are inadequately managed and released to the environment untreated, the FOG present in them not only contributes to the overall BOD5 but can reduce gaseous transfer rates in both water bodies and soils and can cause clogging in transport and treatment piping (Husain et al., 2014).
The differences presented between previous studies and the present work can be attributed to the larger pool of sampled vinasses since the present study characterized 20 different TPUs across the state of Jalisco compared to individual characterizations performed by Lopez-Lopez, Íñiguez-Covarrubias, and España-Gamboa (Íñiguez-Covarrubias and Peraza-Luna, 2007; López-López et al., 2010; España-Gamboa et al., 2011), which may not represent the complete diversity of TPUs and their vinasses. López-López et al. (2010) reported the only physicochemical characterization of Tequila vinasses available in the literature but neither indicated the amount/location of TPUs sampled nor the descriptive statistics from the results.
Critical Regions and Scenarios Analysis
Scenario A (field application) is based on the most common management in Jalisco, while scenarios B (aerobic treatment), C (anaerobic treatment), and D (sequential anaerobic-aerobic treatment) are not widely implemented in Jalisco but are generally applied for the management of vinasses in other regions (López-López et al., 2010; España-Gamboa et al., 2011).
Scenario A: Field Irrigation
Because scenario A (field application) assumes no treatment previous to the vinasse’s disposal, the complete nitrogen, phosphorus, and organic matter loads are released to the soil through irrigation (348.24 Mg year−1, 111.14 Mg year,−1, and 314,864.18 Mg year−1, respectively) (Table 8). Although the estimated GHG emissions for scenario A (473,823.03 Mg year−1) (Table 8) are the lowest of the proposed scenarios, the GHG production rates in scenario A are highly dependent on the environmental conditions (temperature, oxygen, nutrient concentration, microorganism populations, etc.) on which the organic matter and nutrients present in the soils and waterbodies are degraded. The estimated GHG emissions for scenario A are likely an underestimation since the emission factors used assume complete aerobic degradation. Moreover, due to the elevated organic content, vinasses are prone to generate partially anaerobic conditions upon applications, which would significatively increase overall GHG estimations (De Klein, 2006; Doorn et al., 2006; Fuess and Garcia, 2014). Additionally, GHG estimations from scenarios C and D do not include possible GHG reductions due to external energy demand reduction, which would reduce overall GHG estimations, lowering the GHG estimation difference between scenario A and scenarios C and D. The mean GHG production estimated for scenario A represents 82.2% of the total GHG production estimate through wastewater management reported for the state of Jalisco (SEMADET, 2019a) and 1.5% of the state’s total GHG emissions (SEMADET, 2019a). However, due to lack of information, it is not clear if the GHG resulting from wastewater management previously estimated for Jalisco includes only urban wastewaters or if it also includes agro-industrial wastewater, therefore explaining the low reported wastewater management GHG values in the state.
Field application, proposed in scenario A, is the most common disposal method of vinasses globally (Moraes et al., 2017). As shown in Table 8, field irrigation of vinasses has several financial advantages, such as low investment and overall disposal costs, in addition to savings in chemical fertilizer or other fertilizer sources for crops. However, this management practice also comes with strong disadvantages that reduce its environmental sustainability due to possible environmental impacts generated. Plants mainly assimilate nitrogen in the form of nitrate. Therefore, most of the nitrogen (85–90%) present in vinasses cannot be directly assimilated by plants, reducing the fertilization potential of untreated vinasses (Fuess and Garcia, 2017). Increased nitrogen content in untreated vinasses leads to high volatilization rates by denitrification increasing N2O generation (World Health Organization, 2006). Phosphorus displays higher stability compared to nitrogen and can be accumulated on the soil´s surface if regularly applied, making it prone to be transported by runoff during rain events (World Health Organization, 2006). Vinasses typically do not contain significant heavy metal concentrations, but the increased soil acidity caused by the regular application of vinasses can increase the mobility of metals present in the soil, causing the accumulation of metals in receptive waterbodies and groundwater (Fuess and Garcia, 2017).
To reduce transport costs, a common practice for tequila producers is to dispose of the vinasses in their own crop fields (SEMADET, 2019b). Since agave is one of the most cultivated crops within both of the critical regions, a great majority of the irrigated vinasses are disposed of in agave fields. However, agave plants display higher soil erosion rates compared to other crops due to their rainfall-concentrating leaf structure, which leads to higher nutrient and solid transport in runoff coming from agave fields (Abrol and Sharma, 2012). Therefore, counterproductive effects can be seen due to excessive vinasse application on crops, as proposed in scenario A. This is due to changes in soil structure and salinity, nutrient bioavailability and microbial communities (World Health Organization, 2006; Fuess and Garcia, 2017).
Jalisco has a total cultivated surface of 1,649,784.9 Ha in 2019 (SIAP, 2020), with an estimated fertilizer consumption of 54.79 kg Ha−1 and 25.74 kg Ha−1 of nitrogen and phosphorus, respectively (FAO, 2021). While the fertilizer requirements of Jalisco largely surpass nitrogen and phosphorus production from tequila vinasses, which represent only 0.03 and 2.5% of the total nitrogen and phosphorus demand respectively, the production of vinasses in the Valles and Altos critical regions and the distribution of the generated vinasses for its use in other regions of the state by fertigation may be economically unfavorable due to increased transport costs (Fuess and Garcia, 2014).
Scenario B: Aerobic Treatment
The aerobic treatment of wastewater (scenario B) is one of the most common processes applied globally for the treatment of organically polluted wastewater since it has proven to be an effective way to remove both organic matter and nutrients from organic wastewaters (SEMADET, 2019b). A downside of this process is the intensive energy requirement to achieve the aeration rates required for the system to operate properly (Rantala and Väänänen, 1985; Eckenfelder, 1998). This energetic demand increases overall treatment costs (Rantala and Väänänen, 1985; Cakir and Stenstrom, 2005). From the four proposed scenarios, the aerobic treatment (scenario B) had the highest GHG emission estimates with a mean of 1,451,781.65 Mg year−1 while having the second lowest nitrogen and phosphorus disposed volumes (208.94 and 61.28 Mg, respectively) (Table 8). GHG production from aerobic systems tends to be proportional to the organic load of the wastewater feed (Cakir and Stenstrom, 2005). Aerobic treatment of highly polluted wastewaters, like vinasses, tends to demand elevated oxygen concentrations in order to allow for the aerobic degradation of organic matter. If operated under non-ideal conditions (low aeration, low mixing, low retention times, etc.), partial anaerobic conditions can be generated within the treatment system, which increases GHG release (Doorn et al., 2006). Since none of the CH4 generated by the aerobic process is captured and combusted, the complete CH4 load is released to the atmosphere, increasing overall GHG estimations for the scenario and increasing the GHG production difference against other proposed management scenarios (Díaz-Vázquez et al., 2020).
Scenario C: Anaerobic Treatment
Scenario C had a mean GHG estimate of 511,225.04 Mg year−1 (Table 9), which represents a 96% reduction compared to the values estimated for scenario B. This was due to CH4 combustion and transformation into CO2 rather than direct release as CH4. Although scenario C displayed lower GHG and a significant reduction of the discharged nitrogen and phosphorus load (208.94 Mg year−1 and 61.28 Mg year−1, respectively) (Table 9), the implementation of individual anaerobic systems requires high investment from producers, limiting its implementation to medium and big producers (Anderson et al., 2013). The implementation of centralized anaerobic digestion systems can offer the possibility to micro and small producers to treat their vinasses through greener alternatives, which bypass the required high initial investments but do not significatively increase treatment costs (Díaz-Vázquez et al., 2020). However, the operation of a centralized anaerobic digestion system can present difficulties due to the heterogeneous nature of the vinasses generated by multiple production units as well as the presence of other pollutants due to non-ideal wastewater management systems within the production units. Alterations in the feed composition can cause changes in the microbial populations within the anaerobic system leading to reduction in treatment efficiency and even complete shutdown of the system (Naik et al., 2014). This heterogenicity in the vinasses can be regulated by strictly monitoring the incoming vinasses in order to prevent undesired pollution and to maintain stable feed conditions to prevent inhibitory or toxic effects on microbial population within the anaerobic digester (Álvarez et al., 2010).
The use of effective nutrient (nitrogen and phosphorus) recovery systems, as proposed in scenarios B, C, and D, not only prevents the excessive discharge of nutrients into the soil and water but additionally presents the opportunity to reduce treatment cost by valorizing the recovered nutrients and allowing for a controlled application of nutrients in crop fields. By concentrating the recovered nutrients in both aerobic and anaerobic sludge, the volume of the nutrient mixture is reduced, simultaneously reducing transport and application costs, which in time allows these recovered nutrients to be applied in fields further from the production site, increasing its economical competitivity against other fertilizer sources (Fuess and Garcia, 2014; Díaz-Vázquez et al., 2020).
Scenario D: Sequential Anaerobic-Anaerobic Treatment
Scenario D displayed the maximum nutrient (nitrogen and phosphorus) and BOD5 reduction and the third lowest GHG production out of the four proposed scenarios (156.71 Mg, 66.69 MG, and 706,984.71 Mg, respectively). The higher GHG production through scenario D in comparison to scenario C can be explained by the addition of an aerobic stage, which slightly increases both CH4 and N2O production even when the BOD5 and nutrient loads entering the aerobic system are reduced by the previous anaerobic stage (Cakir and Stenstrom, 2005). Anaerobic digestion of effluents with high organic loads tends to result in lower overall GHG generated compared to other treatment alternatives, even reaching negative values due to the use of produced biogas for energy generation and the consequent reduction of required energy during the treatment process, contrary to aerobic treatment processes in which wastewaters with a high organic load tend to increase overall GHG generation and energy consumption (Cakir and Stenstrom, 2005). The GHG reduction by CH4 energetic revalorization is not accounted for in scenarios C and D, meaning both have the potential to produce even lower GHG emission rates.
Table 10 displays a comparison of the reported advantages and disadvantages for each of the management strategies proposed in the present work.
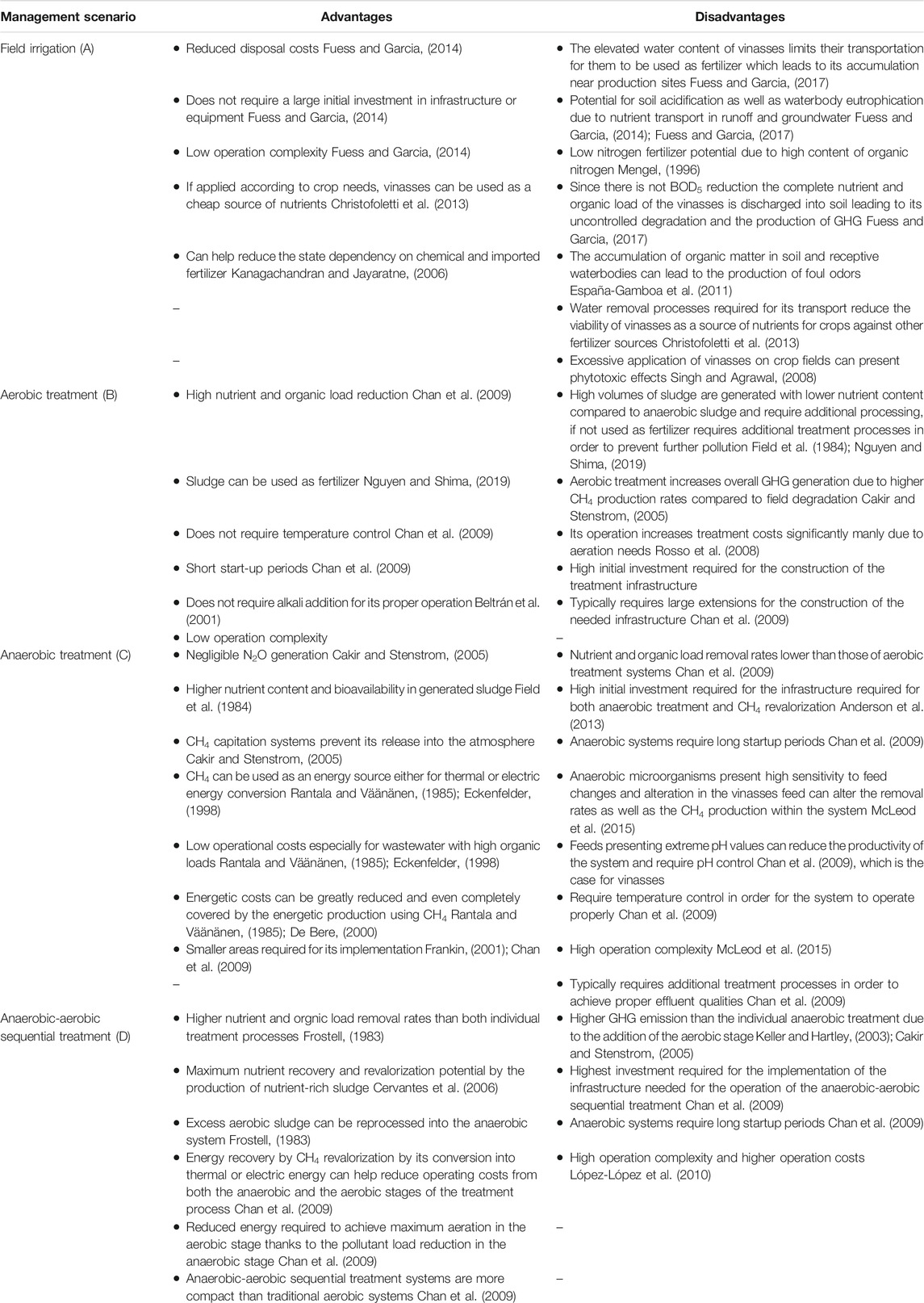
TABLE 10. Comparison between the advantages and disadvantages of the proposed vinasses management scenarios.
Integrated Management Approaches
The implementation of effective treatment systems, either aerobic (scenario B), anaerobic (scenario C), or sequential anaerobic-aerobic (scenario D), usually requires large investments, which limit the producers that can afford such investments and the corresponding operation costs (Díaz-Vázquez et al., 2020). The implementation of centralized treatment units allows micro and small productive units to access effective treatment systems reducing the initial investment needed for its implementation. Additionally, TPUs located within urban areas, which is the case of most productive units located within the municipality of Tequila (Valles critical region), allows access to treatment systems that would require offsite construction due to spatial constraints, reducing the required investment and reducing the strain of local wastewater treatment plants caused by vinasse discharge into municipal wastewater systems (Flotats et al., 2009; Massoud et al., 2009).
The logistics required for the operation of centralized treatment units can significatively increase treatment costs, especially in waste with elevated water content, which is the case of tequila vinasses. This needs to be taken into consideration by decision-makers in order to prevent an unnecessary treatment cost increase due to logistics (Díaz-Vázquez et al., 2020). The establishment of centralized treatment units within critical regions allows the guarantee of a constant supply of wastewater to maintain the economic viability of the treatment systems and protect the natural resources and services of sensitive regions from degradation, which is the case for most basins within Jalisco (de Anda and Shear, 2001; Martinez Rivera, 2004; Abrol and Sharma, 2012; Jayme-Torres and Hansen, 2018). This is not only applicable to tequila vinasses but for most primary production wastes and byproducts generated in the state, as is the case of livestock production waste and dairy processing wastewater (Díaz-Vázquez et al., 2020).
Since vinasses are generated by most alcoholic beverage and bioethanol production processes, regions in Brazil, Japan, the United States as well as many countries within the European Union that are known for intensive alcoholic production, face similar problems to those found in Jalisco (España-Gamboa et al., 2011). Several other practices have been proposed globally for the management of vinasses, in addition to the ones proposed in the previously discussed scenarios. Some examples include use as animal feed, treatment by electrocoagulation, coagulation-flocculation and advanced oxidation (Ozone) as well as biorefinery approaches using either bacteria, fungi, or algae for the simultaneous treatment and recovery of metabolites, biomass or energy (Waliszewski et al., 1997; López-López et al., 2010; España-Gamboa et al., 2011; Christofoletti et al., 2013; Mikucka and Zielińska, 2020). Several novel processes have been developed on a laboratory scale that may offer new alternatives with promising effects on the quality of generated vinasses, as they use hydrolytic enzymes to increase substrate fermentability and decrease the discarded vinasses organic load (Ávila-Fernández et al., 2009; Waleckx et al., 2011). These alternative management practices could be analyzed following the methodology proposed here to assess their environmental sustainability applied to Tequila vinasses. It would be important to pay special attention to the scale and economic viability for the implementation of such practices, as well as to the local and regional context.
The existence of strong producer associations and certification organizations for TPUs at state and national levels can assist in the establishment of centralized treatment units. They can act as intermediaries between policymakers, local authorities, academia, technology suppliers and producers in order to establish the best management practices and coordinate efforts to improve the Tequila production sector and achieve international environmental standards.
Conclusion
The proposed methodology integrates both physicochemical characterization and GIS-based spatial analysis in order to evaluate management scenarios for the determination of possible environmental impacts derived from management practices, either current or proposed. This can be a powerful tool for policymakers and local authorities to evaluate and propose local or regional integral waste management programs, policies and infrastructure. It likewise has the potential to incentivize sustainable practices among productive sectors. This approach can be applied to other regions or other waste-producing sectors in order to determine best management practices in a local and regional context.
The proposed methodology could be improved by the generation of site-specific and waste-specific emission, removal, and conversion factors in order to estimate nutrient and organic load discharge as well as greenhouse gas emissions with higher accuracy based on regional and geographical characteristics, either for environmental (soil and water) degradation of waste or for the evaluation of specific treatment alternatives. It would also be useful to include the integration of multiple organic waste streams into the analysis model. This would open up the possibility to evaluate coprocessing alternatives that may prove to have a more positive environmental impact compared to individual management strategies, especially for dense and highly productive regions, such as the Mexican state of Jalisco.
Data Availability Statement
The raw data supporting the conclusions of this article will be made available by the authors, without undue reservation.
Author Contributions
Conceptualization, MG-H and DD-V; methodology, DD-V; software, DD-V; validation, MG-H and CS-G; investigation, DD-V; resources, MG-H; data curation, DD-V; writing—original draft preparation, DD-V; writing—review and editing, MG-H, DC-N, DO-N, and CS-G; visualization, DD-V; supervision, MG-H, DC-N, and CS-G; project administration, MG-H and CS-G; funding acquisition, MG-H and CS-G.
Funding
This research was funded by the Innovation, Science and Technology Ministry of the State of Jalisco (SICYT by its acronym in Spanish) and the Science and Technology State Council (COECyTJAL by its acronym in Spanish) through Jalisco’s Scientific Development Found (FODECIJAL by its acronym in Spanish), assigned as the Project 7947: “Vinazas tequileras como sustratos para la producción de aditivos alimenticios de alto valor proteico para animales monogástricos.”
Conflict of Interest
The authors declare that the research was conducted in the absence of any commercial or financial relationships that could be construed as a potential conflict of interest.
Acknowledgments
The authors acknowledge the administrative support of “Secretaría de Medio Ambiente y Desarrollo Territorial de Jalisco,” “Valparaiso University,” and the “Instituto Tecnológico y de Estudios Superiores de Monterrey, campus Guadalajara.”
Abbreviations
B0, Methane production capacity; BOD5, Biological oxygen demand; BODD, Biological oxygen demand load in the discharged effluent; BODT, Total Biological oxygen demand; CFCH4, CO2eq conversion factor for methane; CFN2O, CO2eq conversion factor for nitrous oxide; EF, N2O Emission factor; FOG, Fat oil and grease; MCF, Methane conversion factor; N, Nitrogen concentration; ND, Nitrogen load in the discharged effluent; NT, Total nitrogen; P, Phosphorus concentration; PD, Phosphorus load in the discharged effluent; pH, Hydrogen potential; PT, Total phosphorus; R, Vinasses production ratio; RBOD, Biological oxygen demand removal rate; RF, Risk factor; RN, Nitrogen removal rate; RP, Phosphorus removal rate; S, Reclassified raster value of the selected parameter; SS, Settleable solids; T, Tequila production volume; TKN, Total Kjeldahl nitrogen; TSS, Total suspended solids; V, Vinasses production volume; W, Weight of a selected parameter.
References
Abrol, V., and Sharma, P. (2012). Resource Management for Sustainable Agriculture. Rijeca, Croatia: BoD – Books on Demand. doi:10.5772/51304
Al-Anbari, M., Thameer, M., and Al-Ansari, N. (2018). Landfill Site Selection by Weighted Overlay Technique: Case Study of Al-Kufa, Iraq. Sustainability. 10 (4), 999. doi:10.3390/su10040999
Al-Hanbali, A., Alsaaideh, B., and Kondoh, A. (2011). Using GIS-Based Weighted Linear Combination Analysis and Remote Sensing Techniques to Select Optimum Solid Waste Disposal Sites within Mafraq City, Jordan. Jgis. 03 (04), 267–278. doi:10.4236/jgis.2011.34023
Álvarez, J. A., Otero, L., and Lema, J. M. (2010). A Methodology for Optimising Feed Composition for Anaerobic Co-digestion of Agro-Industrial Wastes. Bioresour. Tech. 101 (4), 1153–1158. doi:10.1016/j.biortech.2009.09.061
Anderson, R. C., Hilborn, D., and Weersink, A. (2013). An Economic and Functional Tool for Assessing the Financial Feasibility of Farm-Based Anaerobic Digesters. Renew. Energ. 51, 85–92. doi:10.1016/j.renene.2012.08.081
Ávila-Fernández, Á., Rendón-Poujol, X., Olvera, C., González, F., Capella, S., Peña-Álvarez, A., et al. (2009). Enzymatic Hydrolysis of Fructans in the Tequila Production Process. J. Agric. Food Chem. 57 (12), 5578–5585. doi:10.1021/jf900691r
Bani, M. S., Rashid, Z. A., Hamid, K. H. K., Harbawi, M. E., Alias, A. B., and Aris, M. J. (2009). The Development of Decision Support System for Waste Management; a Review. Int. J. Chem. Mol. Eng. 3 (1), 17–24. Available at: https://publications.waset.org/7145/the-development-of-decision-support-system-for-waste-management-a-review.
Bedoić, R., Ćosić, B., and Duić, N. (2019). Technical Potential and Geographic Distribution of Agricultural Residues, Co-products and By-Products in the European Union. Sci. Total Environ. 686, 568–579. doi:10.1016/j.scitotenv.2019.05.219
Beltrán, F. J., Álvarez, P. M., Rodríguez, E. M., Garcia-Araya, J. F., and Rivas, J. (2001). Treatment of High Strength Distillery Wastewater (Cherry Stillage) by Integrated Aerobic Biological Oxidation and Ozonation. Biotechnol. Prog. 17 (3), 462–467. doi:10.1021/bp010021c
Bowen, S., and Zapata, A. V. (2009). Geographical Indications, Terroir, and Socioeconomic and Ecological Sustainability: The Case of Tequila. J. Rural Stud. 25 (1), 108–119. doi:10.1016/j.jrurstud.2008.07.003
Cakir, F. Y., and Stenstrom, M. K. (2005). Greenhouse Gas Production: A Comparison between Aerobic and Anaerobic Wastewater Treatment Technology. Water Res. 39 (17), 4197–4203. doi:10.1016/j.watres.2005.07.042
Carpenter, S. R., Caraco, N. F., Correll, D. L., Howarth, R. W., Sharpley, A. N., and Smith, V. H. (1998). Nonpoint Pollution of Surface Waters with Phosphorus and Nitrogen. Ecol. Appl. 8 (3), 559–568. doi:10.1890/1051-0761(1998)008[0559:NPOSWW]2.0.CO;2
Carral, E., Bello, M., and López-Mosquera, M. E. (2011). GIS-based Dairy-Industry Sludge Application Plan in Galiza (NW Spain). Proced. Environ. Sci. 9, 27–32. doi:10.1016/j.proenv.2011.11.006
Cedeño, M. C. (1995). Tequila Production. Crit. Rev. Biotechnol. 15 (1), 1–11. doi:10.3109/07388559509150529
Cervantes, F. J., Pavlostathis, S. G., and Haandel, A. V. (2006). Advanced Biological Treatment Processes for Industrial Wastewaters. London, United Kingdom: IWA Publishing.
Chan, Y. J., Chong, M. F., Law, C. L., and Hassell, D. G. (2009). A Review on Anaerobic-Aerobic Treatment of Industrial and Municipal Wastewater. Chem. Eng. J. 155 (1), 1–18. doi:10.1016/j.cej.2009.06.041
Chowdhary, P., Raj, A., and Bharagava, R. N. (2018). Environmental Pollution and Health Hazards from Distillery Wastewater and Treatment Approaches to Combat the Environmental Threats: A Review. Chemosphere 194, 229–246. doi:10.1016/j.chemosphere.2017.11.163
Christofoletti, C. A., Escher, J. P., Correia, J. E., Marinho, J. F. U., and Fontanetti, C. S. (2013). Sugarcane Vinasse: Environmental Implications of its Use. Waste Manag. 33 (12), 2752–2761. doi:10.1016/j.wasman.2013.09.005
Comber, A., Dickie, J., Jarvis, C., Phillips, M., and Tansey, K. (2015). Locating Bioenergy Facilities Using a Modified GIS-Based Location-Allocation-Algorithm: Considering the Spatial Distribution of Resource Supply. Appl. Energ. 154, 309–316. doi:10.1016/j.apenergy.2015.04.128
Council directive (2014). Council Directive Concerning Urban Waste Water Treatment. Available at: http://data.europa.eu/eli/dir/1991/271/2014-01-01.
CRT, C. R. del. T. (2019). Declaratoria. Available at: https://www.crt.org.mx/index.php/es/pages-3/declaratoria.
CRT, C. R. del. T. (2021). Estadisticas CRT. Available at: https://www.crt.org.mx/EstadisticasCRTweb/.
Daian, G., and Ozarska, B. (2009). Wood Waste Management Practices and Strategies to Increase Sustainability Standards in the Australian Wooden Furniture Manufacturing Sector. J. Clean. Prod. 17 (17), 1594–1602. doi:10.1016/j.jclepro.2009.07.008
de Anda, J., Díaz-Torres, J. d. J., Gradilla-Hernández, M. S., and de la Torre-Castro, L. M. (2019). Morphometric and Water Quality Features of Lake Cajititlán, Mexico. Environ. Monit. Assess. 191 (2), 92. doi:10.1007/s10661-018-7163-8
de Anda, J., and Shear, H. (2001). “Nutrients and Eutrophication in Lake Chapala,” in The Lerma-Chapala Watershed: Evaluation and Management. Editors A. M. Hansen, and M. van Afferden (Boston: Springer US), 183–198. doi:10.1007/978-1-4615-0545-7_8
De Bere, L. (2000). Anaerobic Digestion of Solid Waste: State-Of-The-Art. Water Sci. Tech. 41 (3), 283–290. doi:10.2166/wst.2000.0082
De Klein, C. (2006). IPCC Guidelines for National Greenhouse Gas Inventories, Chapter 11. Available at: https://www.ipcc-nggip.iges.or.jp/public/2006gl/spanish/pdf/4_Volume4/V4_11_Ch11_N2O&CO2.pdf.
Delivand, M. K., Cammerino, A. R. B., Garofalo, P., and Monteleone, M. (2015). Optimal Locations of Bioenergy Facilities, Biomass Spatial Availability, Logistics Costs and GHG (Greenhouse Gas) Emissions: A Case Study on Electricity Productions in South Italy. J. Clean. Prod. 99, 129–139. doi:10.1016/j.jclepro.2015.03.018
Díaz-Torres, O., Lugo-Melchor, O. Y., de Anda, J., Gradilla-Hernández, M. S., Amézquita-López, B. A., and Meza-Rodríguez, D. (2020). Prevalence, Distribution, and Diversity of Salmonella Strains Isolated from a Subtropical Lake. Front. Microbiol. 11. doi:10.3389/fmicb.2020.521146
Díaz-Vázquez, D., Alvarado-Cummings, S. C., Meza-Rodríguez, D., Senés-Guerrero, C., de Anda, J., and Gradilla-Hernández, M. S. (2020). Evaluation of Biogas Potential from Livestock Manures and Multicriteria Site Selection for Centralized Anaerobic Digester Systems: The Case of Jalisco, México. Sustainability 12 (9), 3527. doi:10.3390/su12093527
Dickman, C., and Christy, M. (2002). Effects of Salinity on Tadpoles of the green and golden bell Frog (Litoria Aurea). Amphib Reptilia 23 (1), 1–11. doi:10.1163/156853802320877582
DOF, D. O. de. la. F. (1997). NOM-001-SEMARNAT-1996, NOM-002-SEMARNAT-1996 y NOM-003-SEMARNAT-1997. Available at: http://www.conagua.gob.mx/CONAGUA07/Publicaciones/Publicaciones/SGAA-15-13.pdf.
DOF, D. O. de. la. F. (2018). PROY-NOM-001-SEMARNAT-2017. Available at: https://www.dof.gob.mx/nota_detalle.php?codigo=5510140&fecha=05/01/2018.
DOF, D. O. de. la. F. (2012a). NORMA Oficial Mexicana NOM-006-SCFI-2012, Bebidas Alcohólicas-Tequila-Especificaciones. Available at: http://www.dof.gob.mx/nota_detalle.php?codigo=5282165&fecha=13/12/2012.
DOF, D. O. de. la. F. (2012b). NOM-006-SCFI-2012. Available at: http://www.dof.gob.mx/nota_detalle.php?codigo=5282165&fecha=13/12/2012.
Doorn, M., Towprayoon, S., Sonia, M., Irving, W., Palmer, C., Pipatti, R., et al. (2006). IPCC Guidelines for National Greenhouse Gas Inventories, Chapter 6. Available at: https://www.ipcc-nggip.iges.or.jp/public/2006gl/pdf/5_Volume5/V5_6_Ch6_Wastewater.pdf.
A. D. Eaton American Public Health Association, American Water Works Association, & Water Pollution Control Federation (2005). Standard methods for the examination of water and wastewater. 21st Edn. American Public Health Association.
Eckenfelder, W. (1998). Anaerobic versus Aerobic Treatment in the U.S.A. Available at: http://patoczka.net/Jurek%20Pages/Papers/Anaerobic%20vs%20Aerobic%20Treatment.pdf.
EPA (2001). Parameters of Water Quality, Interpretation and Standards. Available at: https://www.epa.ie/pubs/advice/water/quality/Water_Quality.pdf.
EPA, E. P. A. (2021). Code of Federal Regulations: PART 437—CENTRALIZED WASTE TREATMENT POINT SOURCE CATEGORY [Text]. Electron. Code Fed. Regul. (Ecfr). Available at: https://www.ecfr.gov/.
España-Gamboa, E., Mijangos-Cortes, J., Barahona-Perez, L., Dominguez-Maldonado, J., Hernández-Zarate, G., and Alzate-Gaviria, L. (2011). Vinasses: Characterization and Treatments. Waste Manag. Res. 29 (12), 1235–1250. doi:10.1177/0734242X10387313
FAO (2021). FAOSTAT. Available at: http://www.fao.org/faostat/en/?#data/.
Field, J. A., Caldwell, J. S., Jeyanayagam, S., Reneau, R. B., Kroontje, W., and Collins, E. R. (1984). FERTILIZER RECOVERY FROM ANAEROBIC DIGESTERS. Trans. Am. Soc. Agric. Eng. 27 (6), 1871–1876, 1881. Available at: https://arizona.pure.elsevier.com/en/publications/fertilizer-recovery-from-anaerobic-digesters.
Flotats, X., Bonmatí, A., Fernández, B., and Magrí, A. (2009). Manure Treatment Technologies: On-Farm versus Centralized Strategies. NE Spain as Case Study. Bioresour. Tech. 100 (22), 5519–5526. doi:10.1016/j.biortech.2008.12.050
Forster, P., Ramaswamy, V., Artaxo, P., Berntsen, T., Betts, R., Fahey, D. W., et al. (2007). Changes in Atmospheric Constituents and in Radiative Forcing. Chapter 2. Clim. Change 2007. Phys. Sci. Basis. Available at: http://inis.iaea.org/Search/search.aspx?orig_q=RN:39002468.
Frankin, R. J. (2001). Full-scale Experiences with Anaerobic Treatment of Industrial Wastewater. Water Sci. Tech. 44 (8), 1–6. doi:10.2166/wst.2001.0451
Frostell, B. (1983). Anaerobic-Aerobic Biological Treatment of Starch Industry Waste Waters. Starch/Stärke 35 (6), 185–189. doi:10.1002/star.19830350602
Fuess, L. T., and Garcia, M. L. (2014). Implications of Stillage Land Disposal: A Critical Review on the Impacts of Fertigation. J. Environ. Manage. 145, 210–229. doi:10.1016/j.jenvman.2014.07.003
Fuess, L. T., Rodrigues, I. J., and Garcia, M. L. (2017). Fertirrigation with Sugarcane Vinasse: Foreseeing Potential Impacts on Soil and Water Resources through Vinasse Characterization. J. Environ. Sci. Health A 52 (11), 1063–1072. doi:10.1080/10934529.2017.1338892
Gradilla-Hernández, M. S., de Anda, J., Garcia-Gonzalez, A., Montes, C. Y., Barrios-Piña, H., Ruiz-Palomino, P., et al. (2020). Assessment of the Water Quality of a Subtropical Lake Using the NSF-WQI and a Newly Proposed Ecosystem Specific Water Quality Index. Environ. Monit. Assess. 192 (5), 1–19. doi:10.1007/s10661-020-08265-7
Husain, I. A. F., Alkhatib, M. a. F., Jammi, M. S., Mirghani, M. E. S., Zainudin, Z. B., and Hoda, A. (2014). Problems, Control, and Treatment of Fat, Oil, and Grease (FOG): A Review. J. Oleo Sci. 63, 747–752. doi:10.5650/jos.ess13182
Ibanescu, D., Cailean, D., Teodosiu, C., and Fiore, S. (2018). Assessment of the Waste Electrical and Electronic Equipment Management Systems Profile and Sustainability in Developed and Developing European Union Countries. Waste Manag. 73, 39–53. doi:10.1016/j.wasman.2017.12.022
INECC, I. N. de. E. y. C. (2018). INECC reitera su compromiso ante el Acuerdo de París con rutas de mitigación al cambio climático. Available at: http://www.gob.mx/inecc/prensa/inecc-reitera-su-compromiso-ante-el-acuerdo-de-paris-con-rutas-de-mitigacion-al-cambio-climatico.
INEGI, I. N. de. E. y. G. (2021a). Directorio Nacional de Unidades Económicas. DENUECensos Económicos 2014; Instituto Nacional de Estadística y Geografía. INEGIAvailable at: https://www.inegi.org.mx/app/mapa/denue/default.aspx.
INEGI, I. N. de. E. y. G. (2021b). Oferta de información estadística a nivel nacional y por entidad federativa. Instituto Nacional de Estadística y Geografía. INEGIAvailable at: https://www.inegi.org.mx/datos/.
Íñiguez-Covarrubias, G., and Peraza-Luna, F. (2007). Reduction of Solids and Organic Load Concentrations in Tequila Vinasses Using a Polyacrylamide (Pam) Polymer Flocculant. Revista Internacional de Contaminación Ambiental 23 (1), 17–24. Available at: https://www.redalyc.org/pdf/370/37023102.pdf.
Jayme-Torres, G., and Hansen, A. M. (2018). Nutrient Loads in the River Mouth of the Río Verde basin in Jalisco, Mexico: How to Prevent Eutrophication in the Future Reservoir?. Environ. Sci. Pollut. Res. 25 (21), 20497–20509. doi:10.1007/s11356-017-0334-2
Kampschreur, M. J., Temmink, H., Kleerebezem, R., Jetten, M. S. M., and van Loosdrecht, M. C. M. (2009). Nitrous Oxide Emission during Wastewater Treatment. Water Res. 43 (17), 4093–4103. doi:10.1016/j.watres.2009.03.001
Kanagachandran, K., and Jayaratne, R. (2006). Utilization Potential of Brewery Waste Water Sludge as an Organic Fertilizer. J. Inst. Brewing 112 (2), 92–96. doi:10.1002/j.2050-0416.2006.tb00236.x
Kaufman, S. M., Krishnan, N., and Themelis, N. J. (2010). A Screening Life Cycle Metric to Benchmark the Environmental Sustainability of Waste Management Systems. Environ. Sci. Technol. 44 (15), 5949–5955. doi:10.1021/es100505u
Keller, J., and Hartley, K. (2003). Greenhouse Gas Production in Wastewater Treatment: Process Selection Is the Major Factor. Water Sci. Tech. 47 (12), 43–48. doi:10.2166/wst.2003.0626
Kirchman, D. L. (2018). Processes in Microbial Ecology. Oxford, United Kingdom: Oxford University Press. doi:10.1093/oso/9780198789406.001.0001
Kurdve, M., Shahbazi, S., Wendin, M., Bengtsson, C., and Wiktorsson, M. (2015). Waste Flow Mapping to Improve Sustainability of Waste Management: A Case Study Approach. J. Clean. Prod. 98, 304–315. doi:10.1016/j.jclepro.2014.06.076
Lijó, L., Frison, N., Fatone, F., González-García, S., Feijoo, G., and Moreira, M. T. (2018). Environmental and Sustainability Evaluation of Livestock Waste Management Practices in Cyprus. Sci. Total Environ. 634, 127–140. doi:10.1016/j.scitotenv.2018.03.299
López-López, A., Davila-Vazquez, G., León-Becerril, E., Villegas-García, E., and Gallardo-Valdez, J. (2010). Tequila Vinasses: Generation and Full Scale Treatment Processes. Rev. Environ. Sci. Biotechnol. 9 (2), 109–116. doi:10.1007/s11157-010-9204-9
Macías, A. M. (1997). ORGANIZACIÓN DE LA INDUSTRIA DEL TEQUILA. Carta Económica Reg. 54, 3–11. doi:10.32870/cer.v0i54.7643
Madurwar, M. V., Ralegaonkar, R. V., and Mandavgane, S. A. (2013). Application of Agro-Waste for Sustainable Construction Materials: A Review. Construction Building Mater. 38, 872–878. doi:10.1016/j.conbuildmat.2012.09.011
Martinez Rivera, L. (2004). Watershed Management to Control Pollution in the Ayuquila River, Jalisco, Mexico. All Graduate Theses and DissertationsAvailable at: https://digitalcommons.usu.edu/etd/6613.
Massoud, M. A., Tarhini, A., and Nasr, J. A. (2009). Decentralized Approaches to Wastewater Treatment and Management: Applicability in Developing Countries. J. Environ. Manage. 90 (1), 652–659. doi:10.1016/j.jenvman.2008.07.001
McLeod, J. D., Othman, M. Z., Beale, D. J., and Joshi, D. (2015). The Use of Laboratory Scale Reactors to Predict Sensitivity to Changes in Operating Conditions for Full-Scale Anaerobic Digestion Treating Municipal Sewage Sludge. Bioresour. Tech. 189, 384–390. doi:10.1016/j.biortech.2015.04.049
Mengel, K. (1996). Turnover of Organic Nitrogen in Soils and its Availability to Crops. Plant Soil 181 (1), 83–93. doi:10.1007/BF00011295
Mikucka, W., and Zielińska, M. (2020). Distillery Stillage: Characteristics, Treatment, and Valorization. Appl. Biochem. Biotechnol. 192 (3), 770–793. doi:10.1007/s12010-020-03343-5
Montoya, H. A., Contreras, C. S., and Garcia, V. M. (1997). Estudio Integral de la Calidad del Agua en el Estado de Jalisco. Guadalajara, Mexico: Comision estatal del agua.
Moraes, B. S., Petersen, S. O., Zaiat, M., Sommer, S. G., and Triolo, J. M. (2017). Reduction in Greenhouse Gas Emissions from Vinasse through Anaerobic Digestion. Appl. Energ. 189, 21–30. doi:10.1016/j.apenergy.2016.12.009
Muhammad, M. B., and Chandra, R. (2021). Enhancing Biogas and Methane Production from Leaf Litter of Neem by Co-digestion with Vegetable Waste: Focus on the Effect of Tannin. Biomass and Bioenergy 147, 106007. doi:10.1016/j.biombioe.2021.106007
Mukherjee, D., Cromley, R. G., Shah, F. A., and Bravo-Ureta, B. E. (2015). Optimal Location of Centralized Biodigesters for Small Dairy Farms: A Case Study from the United States. Int. J. Sust. Energ. Plann. Manag. 8, 3–16. doi:10.5278/ijsepm.2015.8.2
Naik, L., Gebreegziabher, Z., Tumwesige, V., Balana, B. B., Mwirigi, J., and Austin, G. (2014). Factors Determining the Stability and Productivity of Small Scale Anaerobic Digesters. Biomass and Bioenergy 70, 51–57. doi:10.1016/j.biombioe.2014.01.055
Nguyen, T.-B., and Shima, K. (2019). Composting of Sewage Sludge with a Simple Aeration Method and its Utilization as a Soil Fertilizer. Environ. Manage. 63 (4), 455–465. doi:10.1007/s00267-017-0963-8
Nobre, R. C. M., Rotunno Filho, O. C., Mansur, W. J., Nobre, M. M. M., and Cosenza, C. A. N. (2007). Groundwater Vulnerability and Risk Mapping Using GIS, Modeling and a Fuzzy Logic Tool. J. Contaminant Hydrol. 94 (3), 277–292. doi:10.1016/j.jconhyd.2007.07.008
Rantala, P., and Väänänen, P. (1985). Cost Comparison of Aerobic and Anaerobic Wastewater Treatment Systems. Water Sci. Tech. 17 (1), 255. doi:10.2166/wst.1985.0020
Rincón-Pérez, J., Celis, L. B., Morales, M., Alatriste-Mondragón, F., Tapia-Rodríguez, A., and Razo-Flores, E. (2021). Improvement of Methane Production at Alkaline and Neutral pH from Anaerobic Co-digestion of Microalgal Biomass and Cheese Whey. Biochem. Eng. J. 169, 107972. doi:10.1016/j.bej.2021.107972
Robles-González, V., Galíndez-Mayer, J., Rinderknecht-Seijas, N., and Poggi-Varaldo, H. M. (2012). Treatment of Mezcal Vinasses: A Review. J. Biotechnol. 157 (4), 524–546. doi:10.1016/j.jbiotec.2011.09.006
Rosso, D., Larson, L. E., and Stenstrom, M. K. (2008). Aeration of Large-Scale Municipal Wastewater Treatment Plants: State of the Art. Water Sci. Tech. 57 (7), 973–978. doi:10.2166/wst.2008.218
Sabliy, L., Kuzminskiy, Y., Gvozdyak, P., and Łagód, G. (2009). Anaerobic and Aerobic Treatment of Wastewater of Milk Plants. Proc. ECOpole 3 (2). Available at: http://yadda.icm.edu.pl/yadda/element/bwmeta1.element.baztech-be474944-3e6f-41f7-b01f-cd2deecddbff.
SADER, S. de. A. y. D. R. (1989). Ley Estatal del Equilibrio Ecológico y la Protección al Ambiente. Available at: https://info.jalisco.gob.mx/gobierno/documentos/3089.
Sahar, I., and Ahmad, I. (2020). Waste Management Analysis from Economic- Environment Sustainability Perspective. Int. J. Scientific Tech. Res. 8, 1540–1543.
SEMADET (2017). Programa estatal para la prevencion y gestion integral de residuos del estado de Jalisco. Available at: https://semadet.jalisco.gob.mx/sites/semadet.jalisco.gob.mx/files/programa_estatal_de_residuos_2017-2022.pdf.
SEMADET, S. de. M. A. y. D. T. (2018). Programa Estatal de Acción ante el Cambio Climático (PEACC) | Secretaría de Medio Ambiente y Desarrollo Territorial. Available at: https://semadet.jalisco.gob.mx/gobernanza-ambiental/cambio-climatico/programa-estatal-de-accion-ante-el-cambio-climatico-peacc.
SEMADET (2019a). Inventario Estatal de Emisiones de Gases y Compuestos de Efecto Invernadero de Jalisco. Available at: https://transparencia.info.jalisco.gob.mx/sites/default/files/INVENTARIO%20(1).pdf.
SEMADET (2019b). Listados de las Cédulas de Operación Anual. Available at: https://semadet.jalisco.gob.mx/medio-ambiente/calidad-del-aire/listados-de-las-cedulas-de-operacion-anual.
Shahmoradi, B., and Isalou, A. (2013). Site Selection for Wastewater Treatment Plant Using Integrated Fuzzy Logic and Multicriteria Decision Model: A Case Study in Kahak District. J. Adv. Environ. Health Res. 1, 51–61. doi:10.22102/jaehr.2013.40125
SIAP (2020). SIACON. gob.Mx. Available at: http://www.gob.mx/siap/documentos/siacon-ng-161430.
SIAPSAGARPA (2018). Atlas Agroalimentario 2018. Available at: https://nube.siap.gob.mx/gobmx_publicaciones_siap/pag/2018/Atlas-Agroalimentario-2018.
Singh, R. P., and Agrawal, M. (2008). Potential Benefits and Risks of Land Application of Sewage Sludge. Waste Manag. 28 (2), 347–358. doi:10.1016/j.wasman.2006.12.010
Tequila Sauza (2021). Making Tequila. Available at: https://www.casasauza.com/en/making-tequila.
UN, U. N. (2017). “Transforming Our World: The 2030 Agenda for Sustainable Development,” in A New Era in Global Health. Editor W. Rosa (Springer Publishing Company). doi:10.1891/9780826190123.ap02
Vaissi, S., and Sharifi, M. (2019). Integrating Multi-Criteria Decision Analysis with a GIS-Based Siting Procedure to Select a Protected Area for the Kaiser's Mountain Newt, Neurergus kaiseri (Caudata: Salamandridae). Glob. Ecol. Conservation 20, e00738. doi:10.1016/j.gecco.2019.e00738
Venier, F., and Yabar, H. (2017). Renewable Energy Recovery Potential towards Sustainable Cattle Manure Management in Buenos Aires Province: Site Selection Based on GIS Spatial Analysis and Statistics. J. Clean. Prod. 162, 1317–1333. doi:10.1016/j.jclepro.2017.06.098
von Sperling, M. (1996). Comparison Among the Most Frequently Used Systems for Wastewater Treatment in Developing Countries. Water Sci. Tech. 33 (3), 59–72. doi:10.1016/0273-1223(96)00301-0
Waleckx, E., Mateos-Diaz, J. C., Gschaedler, A., Colonna-Ceccaldi, B., Brin, N., García-Quezada, G., et al. (2011). Use of Inulinases to Improve Fermentable Carbohydrate Recovery during Tequila Production. Food Chem. 124 (4), 1533–1542. doi:10.1016/j.foodchem.2010.08.007
Waliszewski, K. N., Romero, A., and Pardio, V. T. (1997). Use of Cane Condensed Molasses Solubles in Feeding Broilers. Anim. Feed Sci. Tech. 67 (2), 253–258. doi:10.1016/S0377-8401(97)00004-7
World Health Organization (2006). WHO | Guidelines for the Safe Use of Wastewater, Excreta and Greywater—Volume 2. World Health OrganizationAvailable at: http://www.who.int/water_sanitation_health/publications/gsuweg2/en/.
Yager, R. R. (1988). On Ordered Weighted Averaging Aggregation Operators in Multicriteria Decisionmaking. IEEE Trans. Syst. Man. Cybern. 18 (1), 183–190. doi:10.1109/21.87068
Keywords: integrated waste management, agro-industrial waste, tequila vinasses, GIS analysis, scenario analysis, physicochemical characterization, integrated methodology
Citation: Díaz-Vázquez D, Carrillo-Nieves D, Orozco-Nunnelly DA, Senés-Guerrero C and Gradilla-Hernández MS (2021) An Integrated Approach for the Assessment of Environmental Sustainability in Agro-Industrial Waste Management Practices: The Case of the Tequila Industry. Front. Environ. Sci. 9:682093. doi: 10.3389/fenvs.2021.682093
Received: 17 March 2021; Accepted: 09 June 2021;
Published: 25 June 2021.
Edited by:
C. Marjorie Aelion, University of Massachusetts Amherst, United StatesReviewed by:
Christel Baum, University of Rostock, GermanyRosa Rodriguez, Facultad de Ingeniería–Universidad Nacional de San Juan, Argentina
Copyright © 2021 Díaz-Vázquez, Carrillo-Nieves, Orozco-Nunnelly, Senés-Guerrero and Gradilla-Hernández. This is an open-access article distributed under the terms of the Creative Commons Attribution License (CC BY). The use, distribution or reproduction in other forums is permitted, provided the original author(s) and the copyright owner(s) are credited and that the original publication in this journal is cited, in accordance with accepted academic practice. No use, distribution or reproduction is permitted which does not comply with these terms.
*Correspondence: Misael Sebastián Gradilla-Hernández, bXNncmFkaWxsYUB0ZWMubXg=