- 1Fisheries College, Guangdong Ocean University, Zhanjiang, China
- 2Southern Marine Science and Engineering Guangdong Laboratory (Zhanjiang), Zhanjiang, China
The release strategy (choice of the species and locations stocked, releasing mode, and stocking size and times) is an important part of quantitative evaluations of marine fish stock enhancement, while optimization of the release strategy can contribute to assess the stocking success intended to alleviate declining fishery resources and to increase the income of fishers. In this study, a typical fish species released in the northern South China Sea, black sea bream Acanthopagrus schlegelii, was taken as the research object. The biological characteristics of this sparid were determined from samples collected from waters in the Zhanjiang estuary during June, July, and September 2020 to April 2021, and the data were applied to estimate its length frequency and its growth parameters using the ELEFAN I run in FiSAT II. We then simulated and evaluated the stocking effects of five scenarios under different release strategies, while assuming a fixed total quantity of released fish and a constant of mortality rate at different life stages. The results showed that (1) the breeding season of black sea bream in this region is mainly from December to March of the next year, which is also the period when most significant sexual reversal in this species occurs, and (2) the relationship between standard length and weight in black sea bream is W = 5.092 × 10–5 L2.906, L∞ = 54.39 cm, K = 0.15, and t0 = −0.967. (3) The recommended period to release black sea bream in Zhanjiang waters is from June to October. It appears more productive if the total quantity of fish released can be divided into two batches. The growth potential of released juvenile fish in this study was evaluated based on a density-dependent theory, and the stocking effect of released stocks was simulated with the consideration for biological parameters and field sampling technique. This study provides a reference for the optimization of fish release strategies in coastal waters.
Introduction
Overfishing, habitat degradation, and climate change exert continuous pressure on the global fishery (Jackson, 2001), with 34.2% of fisheries worldwide estimated to be overexploited in 2017 (FAO, 2020) and the proportion of fisheries deemed sustainable having decreased to 65.8% from 90% in 1974 (FAO, 2020). Sustainable fisheries contribute to the marine ecosystem functioning and to the livelihoods of the millions of fishermen, and provide a vital source of high quality of animal protein for human consumption. Ecosystem-based fishery management and the framework of responsible approach to stocking (Blankenship and Leber, 1995) hold tremendous potentials for increasing production for some capture fisheries, and many active steps such as the regulation of fishing efforts (Hammer and Truitt, 1942; Crutchfield, 1979), habitat restoration (Zaharia et al., 2014; Adams et al., 2019), and stock enhancement (Lorenzen et al., 2010; Johnston et al., 2018) have been put into practice.
Fish stock enhancement, which has been conducted worldwide since the 1970s, has been one of the most popular tools in fisheries management, but most studies have been in experimental stages (Kitada, 2018) and few reach their set goals (Li and Huang, 2011). The desired goals for stock enhancement include the enhancement of wild population, increase in food fish supplies, and improvement of fishermen’s livelihood (Ingram and De Silva, 2015). The outcomes of stock enhancement will in turn affect the efforts of ranching activities and financial supports to the artificial propagation (Kellison and Eggleston, 2004; Garlock et al., 2017).
It is impossible to realize the desired stocking goals if simply releasing large quantities of larvae/juvenile fish, as the success of stock enhancement is affected by factors such as stocking density, age and size of fish released, condition and health of fish, the timing of stocking, and the habitat suitability in an active adaptive management framework (Camp et al., 2013). Since the evaluation of stocking success (including ecological and economic aspects) (Bell et al., 2006; Kitada, 2018) is time-consuming and expensive, modeling the stocking programs with simulation can help us assess and improve the stocking programs (Ingram, and De Silva, 2015). The evaluation of stocking effects is also a part of fish stock assessment; most of the stock assessment techniques can be used to estimate the stocks and biological parameters of released populations, such as natural mortality, release mortality (Benaka et al., 2014), post-release survival, and recapture rate (Kitada et al., 1992; Støttrup et al., 2002; Lyu et al., 2021). The optimization of the release strategy must consider the technique for breeding the stocked species and fishing, and also the trade-offs between the costs of breeding and the benefits of stocking (Kellison and Eggleston, 2004). Research has dealt with the release strategies such as white sturgeon Acipenser transmontanus (Botsford and Hobbs, 1984), optimal release scenarios for summer flounder Paralichthys dentatus (Kellison and Eggleston, 2004), choosing the candidate stocking species (Garlock et al., 2017), and optimal stocking quantities and management actions to be taken after the release (Liu et al., 1994). Using the example of black seabream Acanthopagrus schlegelii, this study aims to contribute more effective stock enhancement using fish growth parameters. Most stocking programs are biomass oriented, whereby increases in catch and revenue are considered the most important expectations among fisheries and fishers, especially in developing countries/regions.
The black seabream is a warm-water demersal sparid found mainly in sand/silt-bottom or rocky-reef habitats of shallow coastal waters (Wu et al., 2011). It is a prominent aquaculture species owing to its rapid growth rate, high adaptability in coastal waters, and high-quality protein, which commands a higher market price (Wu et al., 2011). A sharp decrease in sparid catches has prompted the stocking of black seabream in Chinese waters (Guo et al., 2021), with the quantity reaching 70.15 million individuals in 2017 (Ministry of Agriculture and Rural Affairs of the People’s Republic of China et al., 2018), accounting for 0.27% of the annual total quantities of China (Tang, 2019). Previous studies have reported on the capture–recapture analyses of black seabream in Daya bay, northern South China Sea (SCS) (Lin et al., 2001; Liu et al., 2019). However, the growth potential of released juvenile fish and their contributions to population recruitment are largely unknown. Using examples of the wild stocks of black seabream in the coastal waters around the Zhanjiang estuary, northern SCS, we applied a fish growth model and the density-dependent theory to simulate release scenarios and the recruitment/stocking effects of the released stocks, with an aim to describe an optimal release scenario. This study offers an improved framework for quantitative analysis of marine fish stock enhancement.
Materials and Methods
Sample Collection and Processing
A total of 273 wild black seabream A. schlegelii were collected from the wharf landings of fishermen who used gillnets, fishing tackle, or trawls in the sea near the Zhanjiang estuary, in June, July, and September of 2020 to April 2021. The fish samples were taken back to the laboratory for biological analysis, the standard length (L) and body mass (W) of the black sea bream were measured, and the stages of gonadal maturity were determined (Holden and Raitt, 1974). The length was accurate to 1 mm, and the body mass was accurate to 0.1 g.
Simulation Model
Estimation of the increase in body mass of released fish was based on biological data from black sea bream collected in the field. Simulation of the number and biomass of released stocks were based on the relationship between stocking density and mortality rates. To optimize the stocking effects of constant total quantity of released fish, we simulated five stocking scenarios with the months as the interval unit (Table 1). For simplicity of calculation, we assumed that the total number of fish released is 50,400, and the stocking times is 1, 2, 3, 4, and 7, respectively, in scenarios I, II, III, IV, and V, and then simulated the stocking effect in a maximum 6-month period and six more months after release. Density-dependent theory in ecology usually maintains a linear, inverse relationship between the population mortality and population density (Roughgarden, 1971; Herrando-Pérez et al., 2012; Lee and Strauss, 2016). Therefore, differences in stock density during a single release may lead to varied mortality rates. Combined with the total number of fish released and their estimated natural mortality rates, the changes of the number of released fish stocks and their corresponding weight in each period could be simulated. Refer to the Ricker model to predict exponential yield (Ricker, 1975). Finally, the total biomass (weight/quantity) of the released fish was accumulated, and the effects of the stocking scenarios were evaluated.

TABLE 1. Simulated stocking scenarios under different intervals of release with the constant of total quantity of fish released.
Hypothesis of the Simulation Model
The model assumes the following:
1) The mortality rate of released fish is restricted only by density. Although studies have shown that the mortality rate of released fish in wild conditions can vary with age and size (Lorenzen, 2000), in this study, the release interval of different batches was relatively short (months), and therefore, the effect of the size difference between the batches of released fish on mortality was ignored.
2) There is no significant interaction between wild and released fish stocks in stocking waters during this study.
Data Analysis
Estimation of Biological Parameters
The power function was used to fit the body length–weight relationship of the black sea bream stock (Ricker, 1973) as follows:
where W is body mass (g), L is the length (mm), a is the condition factor, and b is the growth index. The larger value of a indicates the good growth conditions of fish and the value of b can test if the fish grow uniformly, and changes in the value may be related to the nutritional conditions and life stages of the fish.
Following the Snedecor statistical method of grouping by body length (Snedecor, 1980; Chen et al., 2008):
where CI is class interval, R is the range between the maximum and the minimum of body length, and SD is the standard deviation of body length. The body length data were grouped in increments of 18.5 cm, and the dominant group accounted for more than 10% of the total sample.
The ELEFAN I method of FiSAT II was used to analyze the body length frequency, estimate the growth parameters such as asymptotic body length (L∞) and growth rate (K), and fit the von Bertalanffy growth equation of black sea bream (Munro and Pauly, 1983) (Eqs 3, 4) is obtained by combining Eqs 1–3.
The t0 value was estimated using Pauly’s (Pauly, 1979) empirical equation:
Natural Mortality Rate of Released Fish Stocks
In certain areas, the natural mortality rate increased with an increase in juvenile fish density (Minto et al., 2008). The functional response curve (Lindholm et al., 2001) reflects the density–mortality relationship (Figure 1) and the number of juvenile fish released, then:
where m is the monthly natural mortality rate. α is the constant of the specific habitat (which has little effect on the research results and thus 0.5 is taken for simplicity), x is the number of fish released/1,000, and z is the theoretical maximum mortality that can be achieved under the curve. The variable x is the density of released fish, but it is difficult to quantify due to the random and rapid dispersal of released fish in stocking waters. Therefore, the number of fish released is used to replace x, and the formula is modified accordingly. According to a simulated 30-day survival rate of black sea bream experiment (Zhou et al., 2020), the initial monthly mortality rate of 2,400 black sea breams was 24.5%, and thus z is approximately 0.45 in Eq. 6.
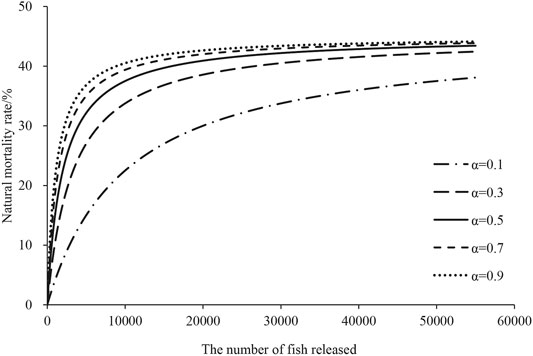
FIGURE 1. The relationship between the natural mortality rate and the number of fish released based on a density-dependent theory. α is the constant of the specific habitat.
The number of released fish was calculated as:
where Nt is the survived number of fish at month (t), and Nt-1is the number of fish released in previous month (t−1).
Calculation of Resource Quantity
Biomass (B) is related to individual body mass and population at a given time (Zhan, 1995) (Eq. 8):
From Eqs 2–6, the biomass of black seabream released was calculated as:
For scenarios with multiple releases, the previously released population will impact the calculation of mortality for the post-release population. When calculating the resource change under a multi-stage release strategy, the number of fish released should be added to the number of surviving fish in the previously released groups:
where Bt is biomass at time t, Nt is the survived number of fish at month (t), Nt-1 is the number of released fish that survived in the previous month (t−1), and xi is the total number of live fish in multiple batches in the last month/1,000.
Results
Standard Length and Body Mass
A total of 273 black sea bream samples were collected in this study. The standard length (SL) range was 74–406 mm, and the average was 235.65 SL. The dominant body-length groups were 129–148 mm (12.09%) and 259–277 mm (10.62%) (Figure 2).
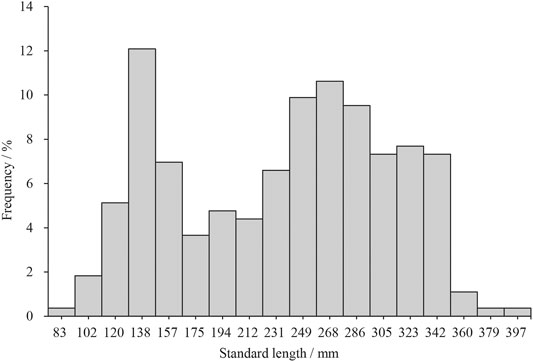
FIGURE 2. Frequency distribution of Acanthopagrus schlegelii body length from Zhanjiang estuary waters, northern South China Sea.
Gonadal Development and Sex Determination
The gonadal stages of the black seabream were evaluated and a coefficient of sexual maturity was calculated for fish in the sea area near the Zhanjiang estuary. The breeding period of the fish was from December to March of the next year. Among the 273 fish sampled, 131 females, 88 males, and six hermaphrodites were visually identifiable as the gonad differentiation was obvious. Monthly variation in the stages of gonadal development denoting males and females is shown in Figure 3. Gonads in stages IV and V appeared from December to March.
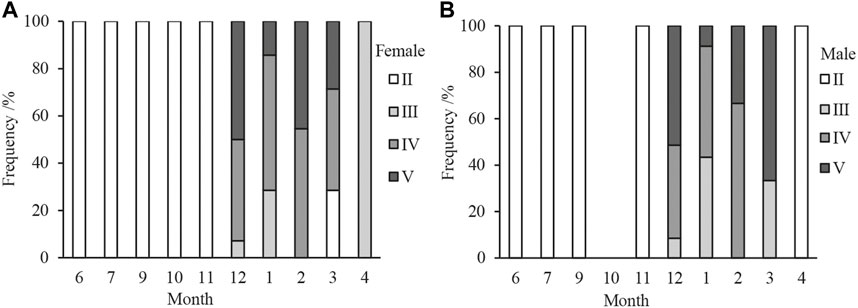
FIGURE 3. Monthly variations in gonad stages denoting males and females of the Acanthopagrus schlegelii sampled from Zhanjiang estuary waters. (A,B) represented the males and females respectively.
Length–Weight Relationship
The relationship between the body length and body mass showed a more-or-less equivalent increase in black sea bream in this sea area. The annual body mass relationship of black sea bream was W = 5.092 × 10–5 L2.906 (R2 = 0.971) (Table 2). Comparing the body length and weight parameters in different seasons, the condition factor a was winter > spring > autumn > summer. The power index b is summer > autumn > winter > spring (Table 2). The b value in summer is greater than 3, reflecting positive allometric growth; the b value in autumn and winter is less than 3, indicating negative allometric growth.
Biological Parameter
The von Bertalanffy growth parameters were obtained by the ELEFAN I method using the time-series and body length frequency data of black sea bream (Figure 4) as follows: asymptotic body length L∞ = 54.39 cm, growth rate K = 0.15, and t0 = −0.967.
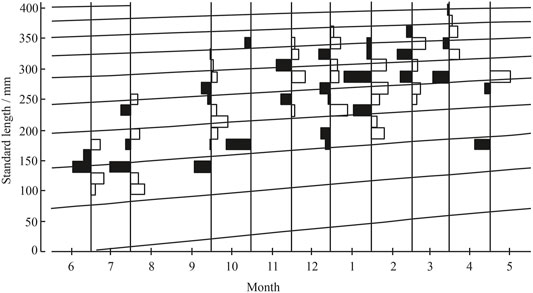
FIGURE 4. Length frequency distribution data and growth curves estimated using ELEFAN method for Acanthopagrus schlegelii.
Variation of the Biomass Under Release Scenarios
Changes in the number and biomass of black sea bream (Table 3; Figures 5, 6) indicated that dividing the release of black seabream into two batches was best. Five kinds of release scenarios were simulated (Figure 7), with each releasing a total of 50,400 juvenile black sea bream but at different intervals. The survival and biomass of the twice release strategies (dividing the total quantity of released fish into two batches) in December accounted for 15.81% of the total release, and the remaining strategies decreased with more release events. A one-time release strategy had the worst effect because of the high mortality rate of released stocks, and the remaining amount is 1.78 and 10.57%. Trends in the biomass and the quantity of released juvenile fish were similar. Because the individual’s natural growth rate is lower than the rate of natural mortality, all release curves showed a downward trend. One release–effect curve decreased monthly after a maximum in the first month, and the other release–effect curves exhibited peaks because of multiple release events. After the last releasing (in July), the stocks and biomass of the juvenile fish under the different release scenarios decreased at different rates because of no further supplement to the existing stocks.
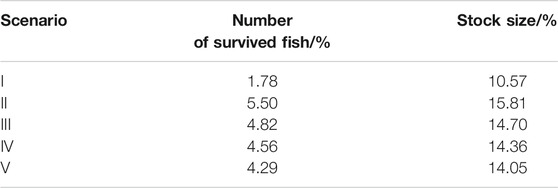
TABLE 3. Simulation of percentages of the survived fish and stock size to the total amount of fish, respectively, 6 months after release finished under scenarios I–V.
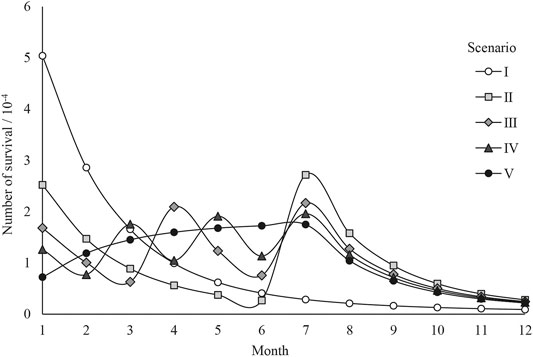
FIGURE 5. Changes in the number survival of released fish under scenarios. Scenario I to V indicated that the total number of fish were released in one, two, three, four, and seven events, respectively, with different intervals during 6 months.
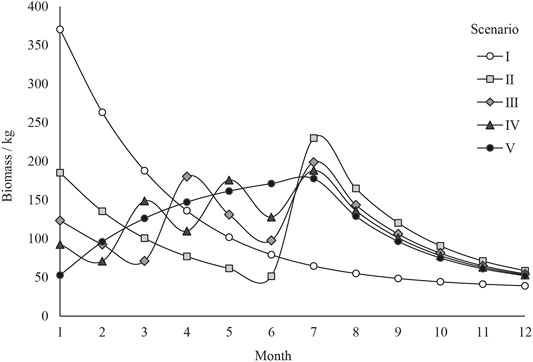
FIGURE 6. Changes in the biomass of survived fish under scenarios. Scenario I to V indicated that the total number of fish were released in one, two, three, four, and seven events, respectively, with different intervals during 6 months.
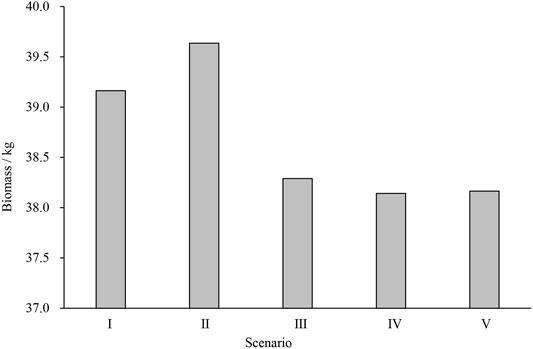
FIGURE 7. Stocking effects of the released fish on biomass replenishing under scenarios. Scenarios I to V indicated that the total number of fish were released in one, two, three, four, and seven events, respectively, with different intervals during 6 months.
Discussion
Optimization of Stocking Strategies
In this study, the effects of different scenarios were compared based on growth parameters and the estimated mortality rate. The stocking effect of twice release strategy is the best among the five releasing strategies (Figure 7). In a practical fish stocking program, in order to achieve the effect of population replenishment, the number of released fish is usually very large (Tang, 2019), and the preferable released strategy needs to be optimized on species accordingly. The simulation model of Yamashita et al. (2017) of an optimum stocking density of juvenile flounder Paralichthys olivaceus showed that the most important factors were prey density and the number of wild juvenile flounder. If the population size is relatively high in a certain sea area, the natural regulation within the population would lead to a slower growth rate of the fish and an increase in mortality (Liu et al., 1994). In the stocking practice, multiple release sites can be selected in the target stocking sea area to reduce the drastic increase of mortality rate due to overhigh stocking density.
Studies have shown that the survival rate of released marine fish varied with seasons (Mckinnell and Lundqvist, 2000). Most of the release work of black seabream in the northern SCS has begun in summer, and is expected to be finished in autumn every year. However, insufficient attention is paid to the actual effects of both the stocking interval and the quantity of fish released in a single event, with fish being released once to many times every year (Liang et al., 2010; Liu et al., 2019). Zhou (2004) summarized the releases of black seabream in the Xiangshan estuary from 1992 to 1994, which were mainly released from June to October, and the recapture rate was about 4.4%–6.05%. In all, the total released quantity of black sea bream subsequently increased year by year, with good economic benefits. However, few quantitative studies on costs and benefits of stock enhancement have been reported, with some qualitative reports. The recapture rate of black seabream in Daya bay in November 2014 was 7.76%, exceeding that record in June 2015 (3.66%) and July 2016 (2.49%), but that the better recapture effect in November was mainly due to the larger size of the released stocks (Liu et al., 2019). Xu et al. (2008) suggested that to avoid the phenomenon of black sea bream being caught immediately after stocking, releases are more appropriate in the closed fishing season, generally in summer and autumn—a time when the development of the released fish corresponds with the natural population. Based on the body length–weight relationship in this study, the b value was higher in summer, following the closed season for fishing in the SCS, which begins in May (Lin and Cheng, 2009). Therefore, June to October would be more appropriate for the release of black sea bream in Zhanjiang estuary waters. The swimming and disperse ability of black sea bream after release is strong, and the number of recaptures appears to be highest at 3–5 months after release, and decrease sharply thereafter (Liu et al., 2019). In combination with the optimal release season (June–October), the optimum release interval of black sea bream in the Zhanjiang estuary waters would be 5 months, with the fish first released in June, and again in October.
Factors Affecting the Stocking Effects
In this study, the release interval and natural mortality were considered to be the two most important factors on stocking effects. As for the release interval, a longer release cycle was expected to be better stocking results. The release interval constituted the biggest difference between the release strategies. Short intervals would amount to a more continuous release of the juvenile fish into the natural water, with each release event involving a relatively small number. However, shortened time intervals and insufficient dispersal of the previous batch of released fish allows for greater intraspecific competition among successive batches, with implications for survival. According to the density-dependent theory (Lorenzen and Enberg, 2002), increases in population density of the released fish lead to higher mortality in certain waters (Liu et al., 1994). With repeated releases, the increase in population density of the released fish leads to an overall high mortality rate, and thus the natural mortality for early released fish is high. In contrast, although secondary releases may involve a large number of fish, there will be less interaction owing to the longer interval, because half of them are released later, with a short period of natural morality, resulting in a larger number of final survivors. Using a yield-per-recruit model, Xu (2015) simulated a release and management strategy of Chinese white prawn Penaeus chinensis, and proposed that a postponed release time somewhat could achieve greater fishing yields. Therefore, appropriately extending the time interval between two releases but within the appropriate release period can reduce the impact of natural mortality on the released fish biomass.
Density-dependent mortality is a key consideration in the optimization of a release strategy, as the natural mortality importantly acts on the released fish. The natural mortality rates of fish populations vary greatly at different life stages and are difficult to estimate accurately (Zhan, 1995). Some research believed that the rates of natural mortality were underestimated for released fish. The release strategy simulation by Xu (2015) deployed a constant mortality rate and assumed that the natural mortality coefficient did not conform to actual changes in the parameter, and this could affect the strategy optimization results. Lindholm (2001) studied the response of cod Gadus morhua larvae to changes in sea-bottom habitat based on three types of response curves depicting mortality and population density, and found that the relationship between survival rate and density of the cod was similar regardless of the type of curve. The purpose of the present study was to optimize the releasing strategy for stocking black seabream, and thus only change in one type of mortality rate was selected. There is an obvious upper limit in a mortality curve. In the case of a large quantity of released fish, the mortality rate increased, but this change did not affect the increasing trend of the amount after releasing. Therefore, although the quantity of released fish stocked in two events (scenario II in Table 1) is still high, the mortality rate will not be significantly higher than that with the other release strategies. In addition, factors affect the mortality rate of released fish, and will exert stocking effects even in circumstances of unknown mortality of released fish, but it will affect the actual stocking effects. Thus, simulation results can still provide a reference for the optimization of the release strategies.
Implications of Condition Factors for Fish Stocking
The results indicate that the growth of black seabream is better in waters of the Zhanjiang estuary area than in Daya bay because of the value of parameter a (Eq. 1) was higher for fish in the Zhanjiang estuary (4.37 × 10–5), compared with that for those in Daya bay (1.55 × 10–5) (Chen et al., 2019). Seasonal variation in a revealed the trend winter > spring > fall > summer. In general, the highest value of a reflects the stage of gonad maturity (Zhan, 1995). The gonad with maturity stage IV or V in the black seabream was observed mainly from December to March of the next year, when the inflated gonad occupied three quarters or more of the body cavity.
The values of the growth parameters reflect the impacts of environmental changes on life conditions of fish (Froese and Pauly, 2021). The a value of the stocks correspondingly varied with the different life stages of the black seabream. The growth index b (2.906) indicates the growth of fish expressed as isauxesis for length and body mass. The value of b was close to that for the black seabream in Daya bay (Chen et al., 2019) and in Taiwanese waters (Chu et al., 2011). Seasonal variations in b revealed the descending trend from summer, fall, winter, to spring in order. The higher b value in summer was possibly attributable to the rapid growth in body mass and faster development of gonads (Zhan, 1995; Chen et al., 2019). This research found a lower b value in winter compared with that in summer and fall, which was possibly caused by a shortage of food and the high energy costs in the winter. Furthermore, higher seasonal fishing pressure may lead to allometric growth as well as a decrease in the quantity of bait fish (Stergiou and Moutopoulos, 2001).
Gonad Development and Sex Change
Male-to-female sex change in black seabream is possibly related to the body shape (Todd et al., 2016). The black seabream is male in the first 2 years (Lee et al., 2001). The popular theory for explaining the gynandromorphy is the size-advantage hypothesis (Munday et al., 2006; Kazancıoğlu and Alonzo, 2010), which hypothesizes that the sex change of fish stocks is adaptive with the total reproductivity of the whole population, and that the body shape determines the direction of the sex change. Under this assumption, males that change to females gain more individual advantages, such as a bigger body shape and higher fecundity, which are beneficial for survival of the whole population (Gemmell et al., 2019). It is imperative to take a systematic consideration, incorporating the reproductive characteristics into the optimization of stocking practice, to attain the desired stocking goals.
Conclusion
We put forward a method for optimizing the fish release strategies based on the biological characteristics, growth parameters, and mortality rates of the stocking population, which is constructive to the quantifying of fish stocking effects. Using the example of the black seabream released into the Zhanjiang estuary and adjacent waters, we established a framework to improve the stock enhancement practice. Further research is needed on the contribution of the released stocks to the replenishment of fish population, as well as their interactions with the wild stocks.
Data Availability Statement
The raw data supporting the conclusion of this article will be made available by the authors, without undue reservation.
Ethics Statement
The animal study was reviewed and approved by the Animal Experimental Ethics Committee of Guangdong Ocean University, China.
Author Contributions
SZ: Methodology and writing original draft preparation; LL: Fieldwork and experiments, data curation, and resources; XW: Supervision, conceptualization, reviewing and editing, and funding acquisition.
Conflict of Interest
The authors declare that the research was conducted in the absence of any commercial or financial relationships that could be construed as a potential conflict of interest.
Publisher’s Note
All claims expressed in this article are solely those of the authors and do not necessarily represent those of their affiliated organizations, or those of the publisher, the editors, and the reviewers. Any product that may be evaluated in this article, or claim that may be made by its manufacturer, is not guaranteed or endorsed by the publisher.
Acknowledgments
We gratefully thank the National Key Research and Development Program of China (2020YFD0901103) and the Program the Southern Marine Science and Engineering Guangdong Laboratory (Zhanjiang) (ZJW-2019-06) for financial support. Cynthia Kulongowski assisted with editing the language of a draft of this manuscript. Our lab members Ling Qiu and Zijie Cui helped us finish the field work.
References
Adams, A. J., Shenker, J. M., Jud, Z. R., Lewis, J. P., Carey, E., and Danylchuk, A. J. (2019). Identifying Pre-spawning Aggregation Sites for Bonefish (Albula vulpes) in the Bahamas to Inform Habitat protection and Species Conservation. Environ. Biol. Fish. 102 (2), 159–173. doi:10.1007/s10641-018-0802-7
Bell, J. D., Bartley, D. M., Lorenzen, K., and Loneragan, N. R. (2006). Restocking and Stock Enhancement of Coastal Fisheries: Potential, Problems and Progress. Fish. Res. 80, 1–8. doi:10.1016/j.fishres.2006.03.008
Benaka, L. R., Sharpe, L., Anderson, L., Brennan, K., Budrick, J. E., Lunsford, C., et al. (2014). Fisheries Release Mortality: Identifying, Prioritizing, and Resolving Data Gaps. Silver Spring: NOAA Tech. Memo. NMFS-F/SPO-142, 84. U.S. Dep. Commerce.
Blankenship, H. L., and Leber, K. M. (1995). A Responsible Approach to marine Stock Enhancement. Am. Fish. Soc. Sym. 15, 167–175.
Botsford, L. W., and Hobbs, R. C. (1984). Optimal Fishery Policy with Artificial Enhancement through Stocking: california’s white sturgeon as an Example. Ecol. Model. 23 (4), 293–312. doi:10.1016/0304-3800(84)90126-1
Camp, E. V., Lorenzen, K., Ahrens, R. N. M., Barbieri, L., and Leber, K. M. (2013). Potentials and Limitations of Stock Enhancement in Marine Recreational Fisheries Systems: An Integrative Review of Florida's Red Drum Enhancement. Rev. Fish. Sci. 21, 388–402. doi:10.1080/10641262.2013.838075
Chen, D. F., Wang, T., Liu, Y., Xiao, Y. Y., Wang, J. J., and Li, C. H. (2019). Biological Study of Reproductive Characteristics of Acanthopagrus Schlegeli in Dava Bay. South China Fish. Sci. 15 (5), 41–47. doi:10.12131/20190051
Chen, J., Shi, Y., Li, M., Ding, W., and Niu, H. (2008). Molecular Cloning of Liver Angiotensinogen Gene in Ayu (Plecoglossus Altivelis) and mRNA Expression Changes upon Aeromonas Hydrophila Infection. Fish Shellfish Immunol. 24 (5), 659–662. doi:10.1016/j.fsi.2008.01.015
Chu, W. S., Wang, J. P., Hou, Y. Y., Ueng, Y. T., and Chu, P. H. (2011). Length-weight Relationships for Fishes off the Southwestern Coast of Taiwan. Afr. J. Biotechnol. 10 (19), 3945–3950. doi:10.5897/ajb11.1552
Crutchfield, J. A. (1979). Economic and Social Implications of the Main Policy Alternatives for Controlling Fishing Effort. J. Fish. Res. Bd. Can. 36 (7), 742–752. doi:10.1139/f79-112
FAO (2020). “The State of World Fisheries and Aquaculture 2020,” in Sustainability in Action (Rome, Italy). doi:10.4060/ca9229en
Froese, R., and Pauly, D. (2021). FishBase. Available at: www.fishbase.org,version(06/2021).
Garlock, T. M., Camp, E. V., and Lorenzen, K. (2017). Using Fisheries Modeling to Assess Candidate Species for marine Fisheries Enhancement. Fish. Res. 186, 460–467. doi:10.1016/j.fishres.2016.08.024
Gemmell, N. J., Todd, E. V., Goikoetxea, A., Ortega-Recalde, O., and Hore, T. A. (2019). Chapter Three - Natural Sex Change in Fish. Curr. Top. Developmental Biol. 134, 71–117. doi:10.1016/bs.ctdb.2018.12.014
Guo, J. T., Wang, T., Chen, D. F., Liu, Y., Quan, Q. M., Wang, J. J., et al. (2021). Feeding Habits of Acanthopagrus Schlegeli in the Daya bay. J. Fish. Sci. China. 28 (8), 1041–1050. doi:10.12264/JFSC2020-0576
Hammer, R. C., and Truitt, R. V. (1942). Control of Fishing Intensity in Maryland. Trans. Am. Fish. Soc. 71 (1), 144–148. doi:10.1577/1548-8659(1941)71[144:cofiim]2.0.co;2
Herrando-Pérez, S., Delean, S., Brook, B. W., and Bradshaw, C. J. A. (2012). Density Dependence: an Ecological Tower of Babel. Oecologia 170 (3), 585–603. doi:10.1007/s00442-012-2347-3
Holden, M. J., and Raitt, D. F. S. (1974). Manual of Fisheries Science Part 2-Mehtod of Resource Investigation and Their Application. Rome, Italy: Food and Agriculture Organization of the United Nations.
Ingram, B., and De Silva, S. (2015). “General Aspects of Stock Enhancement in Fisheries Developments,” in Perspectives on Culture-Based Fisheries Developments in Asia. Editors Sena S. De Silva, B. A. Ingram, and S. Wilkinson (Bangkok, Thailand: Network of Aquaculture Centres in Asia-Pacific), 27–37.
Jackson, J. B. C., Kirby, M. X., Berger, W. H., Bjorndal, K. A., Botsford, L. W., Bourque, B. J., et al. (2001). Historical Overfishing and the Recent Collapse of Coastal Ecosystems. Science 293 (5530), 629–637. doi:10.1126/science.1059199
Johnston, F. D., Allen, M. S., Beardmore, B., Riepe, C., Pagel, T., Hühn, D., et al. (2018). How Ecological Processes Shape the Outcomes of Stock Enhancement and Harvest Regulations in Recreational Fisheries. Ecol. Appl. 28 (8), 2033–2054. doi:10.1002/eap.1793
Kazancıoğlu, E., and Alonzo, S. H. (2010). A Comparative Analysis of Sex Change in Labridae Supports the Size Advantage Hypothesis. Evolution 64 (8), 2254–2264. doi:10.1111/j.1558-5646.2010.01016.x
Kellison, G. T., and Eggleston, D. B. (2004). Coupling Ecology and Economy: Modeling Optimal Release Scenarios for Summer Flounder (Paralichthys Dentatus) Stock Enhancement. Fish. B-noaa. 102 (1), 78–93.
Kitada, S. (2018). Economic, Ecological and Genetic Impacts of marine Stock Enhancement and Sea Ranching: a Systematic Review. Fish. Fish. 19 (3), 511–532. doi:10.1111/faf.12271
Kitada, S., Taga, Y., and Kishino, H. (1992). Effectiveness of a Stock Enhancement Program Evaluated by a Two-Stage Sampling Survey of Commercial Landings. Can. J. Fish. Aquat. Sci. 49, 1573–1582. doi:10.1139/f92-175
Lee, D. E., and Strauss, M. K. L. (2016). “Giraffe Demography and Population Ecology,” in Reference Module in Earth Systems and Environmental Sciences. Amsterdam, Netherlands: Elsevier.
Lee, Y.-H., Du, J.-L., Yueh, W.-S., Lin, B.-Y., Huang, J.-D., Lee, C.-Y., et al. (2001). Sex Change in the Protandrous Black Porgy,Acanthopagrus Schlegeli: A Review in Gonadal Development, Estradiol, Estrogen Receptor, Aromatase Activity and Gonadotropin. J. Exp. Zool. 290 (7), 715–726. doi:10.1002/jez.1122
Li, L., and Huang, S. (2011). A Study on Management of Stock Enhancement in China. J. Shanghai Ocean Univ. 20 (5), 765–772.
Liang, J., Wang, W. D., Lin, G. Z., Xu, K. D., and Guo, A. (2010). Effect and Assessment of Enhancement Release of Nibea Japonica and Sparus Macrocephalus in Artificial Reef Habitat Waters of Zhoushan, Zhejiang. J. Fish. Sci. China. 17 (5), 1075–1084.
Lin, J. B., Chen, T., Chen, L., and Guo, J. F. (2001). The Techniques of Sparus Marocephalus Tagged and Released in Daya bay. J. Fish. China. 25 (1), 79–83.
Lin, L. S., and Cheng, J. H. (2009). Effects of the Prolonged Summer Closed Fishing Period on Fisheries in East China Sea. J. Dalian Ocean Univ. 24 (1), 12–16. doi:10.3969/j.issn.1000-9957.2009.01.003
Lindholm, J. B., Auster, P. J., Ruth, M., and Kaufman, L. (2001). Modeling the Effects of Fishing and Implications for the Design of marine Protected Areas: Juvenile Fish Responses to Variations in Seafloor Habitat. Conservation Biol. 15 (2), 424–437. doi:10.1046/j.1523-1739.2001.015002424.x
Liu, Y. C., Gao, Y. F., Qiu, S. Y., and Liang, X. M. (1994). On the Optimum Released Number of Prawn Larvae for Multiplication in Jiaozhou Bay. Qilu Fish 11 (2), 27–30.
Liu, Y., Yang, C., Shan, B., Sun, D., Liu, S., Li, T., et al. (2019). Investigation of a Mark-Recapture Method of Black Porgy, Acanthopagrus Schlegelii, in Daya Bay Using Plastic Oval Tags. J. Fish. Sci. China 26 (1), 63–70. doi:10.3724/sp.j.1118.2019.18252
Lorenzen, K. (2000). Allometry of Natural Mortality as a Basis for Assessing Optimal Release Size in Fish-Stocking Programmes. Can. J. Fish. Aquat. Sci. 57 (12), 2374–2381. doi:10.1139/f00-215
Lorenzen, K., and Enberg, K. (2002). Density-dependent Growth as a Key Mechanism in the Regulation of Fish Populations: Evidence from Among-Population Comparisons. Proc. R. Soc. Lond. B 269 (1486), 49–54. doi:10.1098/rspb.2001.1853
Lorenzen, K., Leber, K. M., and Blankenship, H. L. (2010). Responsible Approach to marine Stock Enhancement: an Update. Rev. Fish. Sci. 18 (2), 189–210. doi:10.1080/10641262.2010.491564
Lyu, S., Lin, K., Zeng, J., Liu, Y., Chen, Z., and Wang, X. 2021). Fin‐spines Attachment, a Novel External Attachment Method for the Ultrasonic Transmitters on Hard Fin‐spines Fish (Sparidae). J. Appl. Ichthyol. 2021; 37: 227– 234.doi:10.1111/jai.14164
Mckinnell, S. M., and Lundqvist, H. (2000). Unstable Release Strategies in Reared Atlantic salmon,Salmo salarL. Fish. Management Ecol. 7 (3), 211–224. doi:10.1046/j.1365-2400.2000.00172.x
Ministry of Agriculture and Rural Affairs of the People’s Republic of China (2018). National Fisheries Technology Extension Center, China Society of Fisheries. Beijing: China Agriculture Press. China Fishery Statistical Yearbook.
Minto, C., Myers, R. A., and Blanchard, W. (2008). Survival Variability and Population Density in Fish Populations. Nature 452 (7185), 344–347. doi:10.1038/nature06605
Munday, P., Buston, P., and Warner, R. (2006). Diversity and Flexibility of Sex-Change Strategies in Animals. Trends Ecol. Evol. 21 (2), 89–95. doi:10.1016/j.tree.2005.10.020
Munro, J., and Pauly, D. (1983). A Simple Method for Comparing the Growth of Fishes and Invertebrates. Fishbyte 1 (1), 5–6.
Pauly, D. (1979). Theory and Management of Tropical Multispecies Stocks: A Review, with Emphasis on the Southeast Asian Demersal Fisheries. Manila: ICLARM.
Ricker, W. E. (1975). Computation and Interpretation of Biological Statistics of Fish Populations. Bull. Fish. Res. Bd. Can. 191, 1–382.
Ricker, W. E. (1973). Linear Regressions in Fishery Research. J. Fish. Res. Bd. Can. 30 (3), 409–434. doi:10.1139/f73-072
Roughgarden, J. (1971). Density-Dependent Natural Selection. Ecology 52, 453–468. doi:10.2307/1937628
Snedecor, G. W. (1980). Statistical Methods. Seventh Editionseventh. Ames, Iowa: Iowa State University Press.
Stergiou, K. I., and Moutopoulos, D. K. (2001). A Review of Length-Weight Relationships of Fishes from Greek marine Waters. WorldFish 24 (1&2), 23–39.
Støttrup, J. G., Sparrevohn, C. R., Modin, J., and Lehmann, K. (2002). The Use of Releases of Reared Fish to Enhance Natural Populations: A Case Study on Turbot Psetta Maxima (Linné, 1758). Fish. Res. 59, 161–180. doi:10.1016/S0165-7836(01)00413-1
Tang, Q. S. (2019). Strategic Research on the Proliferation of Fishery Resources in China EEZs. Beijing: China Ocean Press. (in Chinese).
Todd, E. V., Liu, H., Muncaster, S., and Gemmell, N. J. (2016). Bending Genders: the Biology of Natural Sex Change in Fish. Sex. Dev. 10 (5-6), 223–241. doi:10.1159/000449297
Wu, R. X., Liu, J., Fan, J. R., and Zhao, Y. J. (2011). A Review on the Nomenclature and Taxonomic Status of the Black Porgy, Acanthopagrus Schlegelii (Perciformes: Sparidae). Mar. Sci. 35 (5), 117–119.
Xu, H. L. (2015). Optimization of Stocking Strategy in Fisheries -taking Chinese Shrimp Fishery as an Example. Shanghai: Shanghai Ocean Univ.
Xu, K. D., Zhou, Y. D., Wang, W. D., Xue, L. J., Zhang, H. L., He, Z. T., et al. (2008). The Tagging and Releasing experiment of Sparus Macrocephalus (Basilewsky) in the Zhoushan Sea Area. J. Shanghai Ocean Univ. 17 (1), 93–97.
Yamashita, Y., Kurita, Y., Yamada, H., Miller, J. M., and Tomiyama, T. (2017). A Simulation Model for Estimating Optimum Stocking Density of Cultured Juvenile Flounder Paralichthys olivaceus in Relation to Prey Productivity. Fish. Res. 186, 572–578. doi:10.1016/j.fishres.2016.08.018
Zaharia, T., Maximov, V., Radu, G., Anton, E., Spinu, A., and Nenciu, M. (2014). Reconciling Fisheries and Habitat protection in Romanian Coastal marine Protected Areas. Sci. Mar. 78 (S1), 95–101. doi:10.3989/scimar.04028.25b
Zhou, Y. B., Chen, P. M., Feng, X., and Yuan, H. R. (2020). Study on the Survival Rate of Main marine Economic Species during Initial Enhancement and Releasing Stages in Guangdong Province. J. Anhui. Agr. Sci. 48 (22), 103–140.
Keywords: stock enhancement, simulation model, optimization of releasing strategy, Acanthopagrus schlegelii, northern South China Sea
Citation: Zhang S, Lin L and Wang X (2021) Optimization of a Marine Fish Release Strategy: A Case Study of Black Sea Bream Acanthopagrus schlegelii in the Zhanjiang Estuary, Northern South China Sea. Front. Environ. Sci. 9:779544. doi: 10.3389/fenvs.2021.779544
Received: 19 September 2021; Accepted: 16 November 2021;
Published: 14 December 2021.
Edited by:
Changliang Ke, Chinese Academy of Fishery Sciences (CAFS), ChinaReviewed by:
Kui Zhang, Chinese Academy of Fishery Sciences (CAFS), ChinaBilin Liu, Shanghai Ocean University, China
Rijin Jiang, Zhejiang Marine Fisheries Research Institute, China
Yong Liu, South China Sea Fisheries Research Institute (CAFS), China
Copyright © 2021 Zhang, Lin and Wang. This is an open-access article distributed under the terms of the Creative Commons Attribution License (CC BY). The use, distribution or reproduction in other forums is permitted, provided the original author(s) and the copyright owner(s) are credited and that the original publication in this journal is cited, in accordance with accepted academic practice. No use, distribution or reproduction is permitted which does not comply with these terms.
*Correspondence: Xuefeng Wang, Xuefeng1999@126.com