- 1Jiangsu Key Laboratory for Biodiversity and Biotechnology, School of Biological Sciences, Nanjing Normal University, Nanjing, China
- 2Jiangsu Institute of Freshwater Fisheries, Nanjing, China
Nitrite and ammonia are two of the most common toxic nitrogenous pollutants in aquatic ecosystem, which can pose a serious threat to the health of aquatic organisms. Cladocerans, as an important part of freshwater ecosystem, will inevitably be harmed by these pollutants. To evaluate the combined toxic effects of these nitrogenous pollutants on cladocerans, we simulated 15 combinations of five nitrite concentrations (0, 0.5, 2, 4, 8 mg L−1) and three ammonia concentrations (0, 0.1, 1 mg L−1) to study the changes of life history traits of Daphnia pulex exposed to these combinations for 21 days. Results showed that under the combined stress of nitrite and ammonia, the survival time of D. pulex was shortened, the time to first batch of eggs and time to first brood were delayed, the body size and tail spine length at maturation were reduced, and the total offspring and the number of broods were decreased. There were some synergistically negative effects between the two nitrogenous pollutants. The presence of ammonia reduced the tolerance of D. pulex to nitrite, and vice versa. These findings provided new insights into the combined toxic effects of nitrite and ammonia on the life history traits of cladocerans, and were of great significance for understanding the population dynamics of specific species in cladocerans community under the condition of compound nitrogenous pollutants.
Introduction
The discharge from livestock and poultry breeding wastewater and the decomposition of dead aquatic organisms in natural waters can produce a large amount of nitrogenous pollutants, including ammonia and nitrite (Yang et al., 2011; Sun et al., 2012, 2013). Nitrite is the intermediate product of nitrification reaction. When oxygen is sufficient, it can be converted into nitrate with low toxicity under the action of microorganisms (van Kessel et al., 2015), but it can also be converted into ammonia with high toxicity under hypoxia (Tiso and Schechter, 2015). Therefore, ammonia and nitrite in some waters may be maintained at a high level together, causing serious harm to aquatic organisms (Yang et al., 2010; Lyu et al., 2013c; Wang et al., 2018). Specifically, nitrites can affect blood parameters by oxidizing Fe2+ in hemoglobin to Fe3+ (such as methemoglobin), thereby reducing the oxygen carrying capacity of blood (Kroupova et al., 2005), which will lead to asphyxia in severe cases. Studies on some cultured aquatic animals has showed that nitrite can cause a variety of physiological disorders (Jensen, 1996), resulting in abnormal metabolic function and a variety of diseases. For cladocerans, long-term exposure to environmentally relevant concentrations of nitrite will delay development, reduce fecundity, and even shorten survival time (Xiang et al., 2012).
Ammonia usually accumulates and even causes chronic toxicity at low concentration 0.01 mg L−1 NH3-N (Badiola et al., 2018). It has been reported that ammonia is a neurotoxin, which can cause significant changes in the level of amino acid in fish brain, especially for glutamate and glutamine (Iwata, 1988; Ip and Chew, 2010). There are many adverse effects of ammonia on aquatic organisms, such as slow growth (Atwood et al., 2000), damage to tissue structure (Xu et al., 2021), imbalance of immune function (Cheng et al., 2020), decreased fecundity (Visek, 1984), reduced osmotic regulation ability (Yang et al., 2010), disordered cell function (Xu et al., 2021). Zhu et al. (2015) studied the comprehensive effects of ammonia and microcystin on the life history characteristics of D. magna, and found that with the increase of ammonia concentration, the time to first batch of eggs and time to first brood of D. magna were delayed, and the number of total offspring decreased.
Cladocerans, an important component of zooplankton, play an irreplaceable role in transferring the energy fixed by primary producer algae to higher trophic levels, and are extremely critical to maintaining the health of aquatic ecosystem (Lyu et al., 2013a; Sun et al., 2022). Moreover, as cladocerans are easy to be cultured in the laboratory, have a short life cycle, and are sensitive to pollutants (Freitas and Rocha, 2011), they are usually used as a test species for ecotoxicological assays in waters (Sarma and Nandini, 2006). Although there have been many studies about the ecotoxicological response of cladocerans to a single nitrogenous pollutant ammonia or nitrite (Lyu et al., 2013b), there is still a lack of systematic research about the impact of simultaneous increase of nitrite and ammonia concentrations on the life history traits of cladocerans. In summer, the concentration of nitrite in some waters can reach 50 mg L−1 or higher (Kamstra et al., 1996), and the concentration of ammonia even can reach 46 mg L−1 (Wang et al., 2013). To reasonably evaluate the actual response of cladocerans to co-existing various nitrogenous pollutants, in this study, we exposed Daphnia pulex, a species of representative cladocerans, to simulated various combinations of different concentrations of nitrite and ammonia for 21 days, during which the life history traits were recorded. We propose a scientific hypothesis that nitrite and ammonia may interact with the life history traits of D. pulex, and the presence of one harmful nitrogenous pollutant can reduce the tolerance of D. pulex to another harmful nitrogenous pollutant. In water environment, the potential accumulation of ammonia and nitrite often co-exists, thus it was of practical significance to study the combined effect of these two pollutants on cladocerans.
Materials and methods
Culture of daphnia and algae
Daphnia pulex, originally isolated from a fish pond near Zhenjiang (32° 15 ‘58 ″ N, 119° 51′ four″ E), China (Huang et al., 2020), was used in this study. The D. pulex was maintained in our laboratory for more than 3 years under the controlled conditions (Huang et al., 2020): 25°C temperature, 40 µmol photons m−2 s−1 light intensity, 14 h:10 h light: dark cycle in COMBO medium (Kilham et al., 1998), and fed with Scenedesmus obliquus. The alga S. obliquus was cultured in 2 L sterile BG-11 medium (Gan et al., 2016), under the same conditions for about 15 days and centrifuged at 3000 g for 15 min after reaching the exponential growth stage. After diluted with ultra-pure water, the algae were stored in a refrigerator at 4°C as food for D. pulex in the following experiment.
Preparation and determination of nitrite and ammonia
We used sodium nitrite (NaNO2) and ammonium chloride (NH4Cl) to simulate the mixtures of the two nitrogenous pollutants. NaNO2 (CAS No. 7362–00–0, analytical pure) and NH4Cl (CAS No. 12125–02–9, analytical pure, 99.5% purity) were purchased from Aladdin (Shanghai, China). Before the experiment, 200 mg L−1 and 2 g L−1 of NaNO2 and NH4Cl stock solution were prepared by dissolving NaNO2 and NH4Cl in de-chlorinated water, respectively, which were diluted to the required concentrations in proportion during the experiment. The accurate concentrations were determined by colorimetric method and Nessler reagent spectrophotometry (Wu and Cao, 2013). The concentrations of NH3-N were calculated using the equation NH3=
Experimental design
The third brood of neonates (<24 h) from the synchronously female D. pulex was used in the experiment. During the experiment, neonates were randomly placed into 50 ml beakers, one for each beaker, which made it easy to record life history traits. According to the environmentally relevant concentrations of nitrogenous pollutants occurred in some specific waters (Kamstra et al., 1996; Wang et al., 2013), we set up 15 treatments, i.e. the combinations of five nitrite concentrations (0, 0.5, 2, 4, 8 mg L−1) and three ammonia concentrations (0, 0.1, 1 mg L−1). We set more concentrations for nitrite to fit the change trend of life history traits using appropriate function with ecological significance, whereas the range of ammonia concentration was wide, representing no pollution, light pollution, and heavy pollution levels, respectively. Each treatment had ten replicates, and the experiment lasted for 21 days. During the experiment, some key life history traits that can fully show the survival, development, and reproduction of D. pulex were recorded every day: survival number, times to first batch of eggs and first brood, sizes at first batch of eggs and first brood, spine lengths and relative spine lengths at first batch of eggs and first brood, number of broods, total offspring per female, and number of offspring per brood. Although nitrite can be converted into nitrate due to bacteria, this experiment cannot be carried out under sterile conditions, as cladocerans cannot survive and develop in a bacteria-free environment (Akbar et al., 2020), and actually bacteria exist under natural conditions. Thus, to ensure the relative stability of nitrite and ammonia concentrations during the whole experimental period, fresh COMBO media with corresponding concentrations and 2 mg C L−1 S. obliquus were replaced every 48 h (Lyu et al., 2013b; Cao et al., 2014) for each replicate. All experiments were conducted under the conditions as described above.
Data analysis
All data were presented in the form of mean values ±SE. Two-way ANOVA and Hoc Tukey’s test were used to analyze the comprehensive effects of different nitrite and ammonia concentrations on survival, development, and reproduction of D. pulex. The three parameter Sigmoidal model
Results
Survival time
Two-way ANOVA showed that nitrite and ammonia significantly reduced the survival time of D. pulex respectively, and there was a significant interaction between the two nitrogenous pollutants (Table 1). When ammonia concentration was 0 mg L−1, low concentration nitrite (0–4 mg L−1) had no significantly negative effect on the survival days of D. pulex (Figure 1A), whereas in the case of 8 mg L−1 nitrite, some maternal D. pulex died, and the survival time decreased to 17.1 d, which was significantly different from the low nitrite concentration treatments. When the ammonia concentration increased to 0.1 and 1 mg L−1, the survival time decreased significantly with the increase of nitrite concentration, and the average survival time under 8 mg L−1 nitrite decreased to 14 days and 10.3 days respectively, and there was a significant difference among the low concentration nitrite treatments.
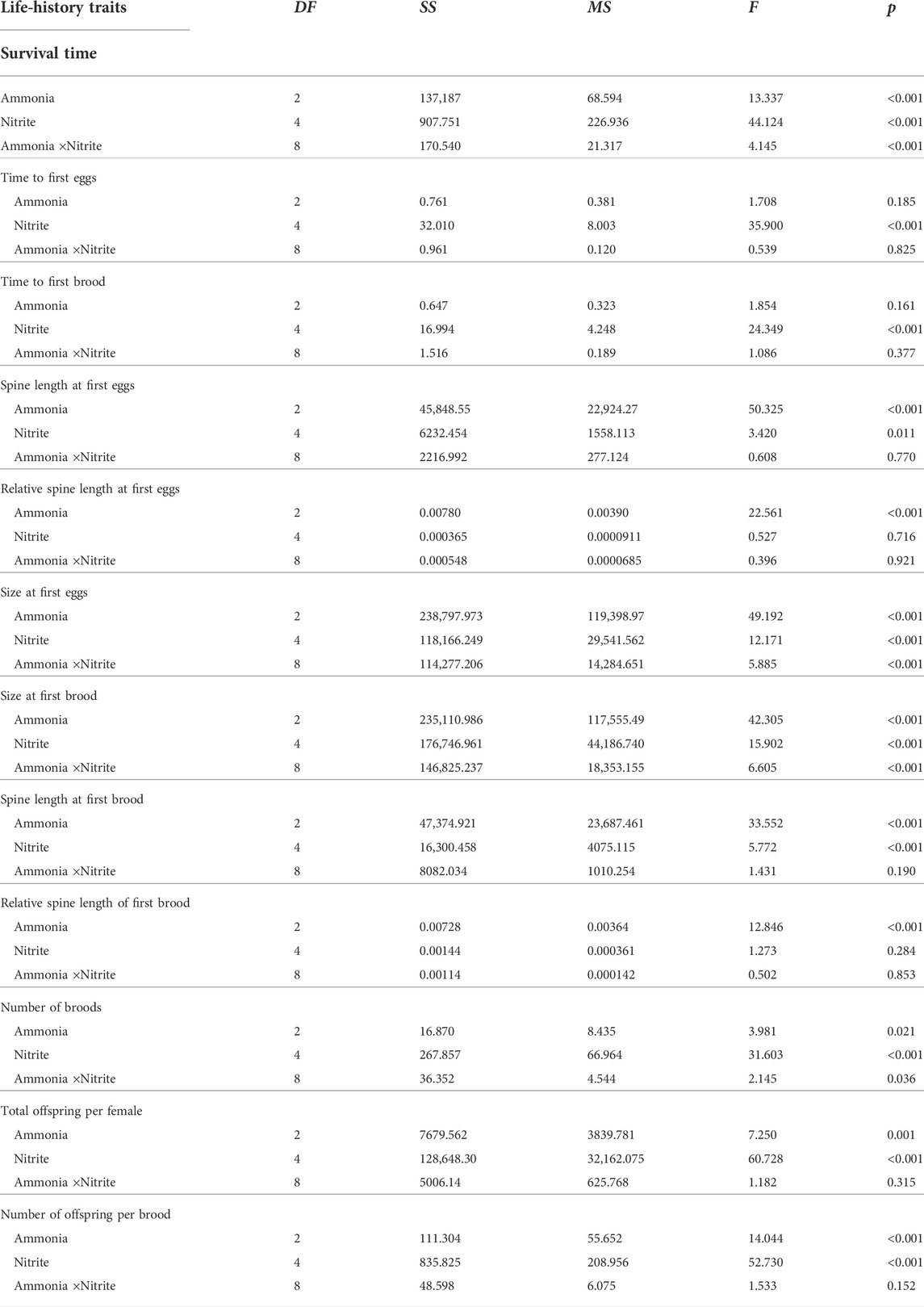
TABLE 1. Two-way ANOVA results of survival, development, and reproductive traits of D. pulex under different ammonia and nitrite concentrations.
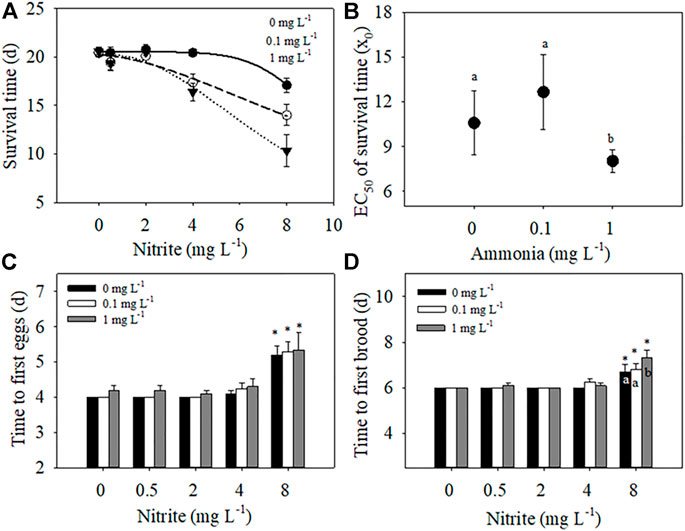
FIGURE 1. Effects of different nitrite concentrations (0, 0.5, 2, 4, 8 mg L−1) and ammonia concentrations (0, 0.1, 1 mg L−1) on the survival time, time to first eggs, and time to first brood of D. pulex. A three-parameter Sigmoidal model
According to the relationship between survival time and nitrite concentration fitted by Sigmoidal model, the EC50 value of nitrite for survival time under the highest ammonia concentration treatment was significantly lower than that under 0 mg L−1 and 0.1 mg L−1 ammonia (Figure 1B), which indicated that the presence of ammonia impaired the tolerance of D. pulex to nitrite, resulting in D. pulex more sensitive to nitrite. Compared with the treatment of different ammonia concentrations under the same nitrite concentration, the decrease amplitude was larger with the increase of nitrite concentration under the same ammonia concentration, which meant that the toxic effect of nitrite also reduced the tolerance of D. pulex to ammonia (Figure 1A).
Development to maturity
The increase of nitrite and ammonia concentration delayed the time to first batch of eggs and time to first brood of D. pulex, but there was no interaction between nitrite and ammonia (Table 1). At the same ammonia concentration, with the increase of nitrite concentration, the time to first batch of eggs and the time to first brood gradually delayed. At the concentration of 8 mg L−1 nitrite, the delay effect reached the maximum, with the time to first batch of eggs delayed by 1.2, 1.3, and 1.3 days, respectively (Figure 1C), and the time to first brood delayed by 0.7, 0.8, and 1.3 days (Figure 1D), respectively. According to the results of two-way ANOVA, although both nitrite and ammonia delayed development, the toxic effect of nitrite on development time was stronger than ammonia in the concentration range of this experiment.
Two-way ANOVA showed that nitrite and ammonia significantly inhibited the time to first batch of eggs and the size of maternal D. pulex at the first brood, and there was a significant interaction between nitrite and ammonia (Table 1). Compared with 0 mg L−1 nitrite, when nitrite concentration was 8 mg L−1 and ammonia concentration was 0, 0.1, and 1 mg L−1, the size of D. pulex at first batch of eggs decreased by 9.4%, 5.8%, and 9.5%, respectively, and the size at first brood decreased by 10.2%, 6.5%, and 9.9%, respectively. Similarly, the increase of nitrite and ammonia concentration also significantly inhibited the spine length and the relative spine length at first batch of eggs and first brood (Figure 2). When nitrite concentration was 8 mg L−1 and ammonia concentration was 0, 0.1, and 1 mg L−1, the spine length of D. pulex at first batch of eggs decreased by 4.7%, 6.5%, and 15.6%, respectively, and spine length at first brood decreased by 6.8%, 7.5%, and 15.7%, respectively. The results of two-way ANOVA showed that within the concentration range of this experiment, ammonia had a stronger toxic effect on the growth and development of D. pulex than nitrite (Table 1). In addition, under different nitrite concentrations (0, 0.5, 2, 4, 8 mg L−1) and ammonia concentrations (0, 0.1, 1 mg L−1), the size, spine length, relative spine length of D. pulex at first batch of eggs and first brood showed various trends (Figure 2). Compared with the treatment without nitrite and ammonia, the body size, spine length and relative spine length of D. pulex in other treatments showed a gradient decrease with the increase of the two nitrogenous pollutants (Figure 2).
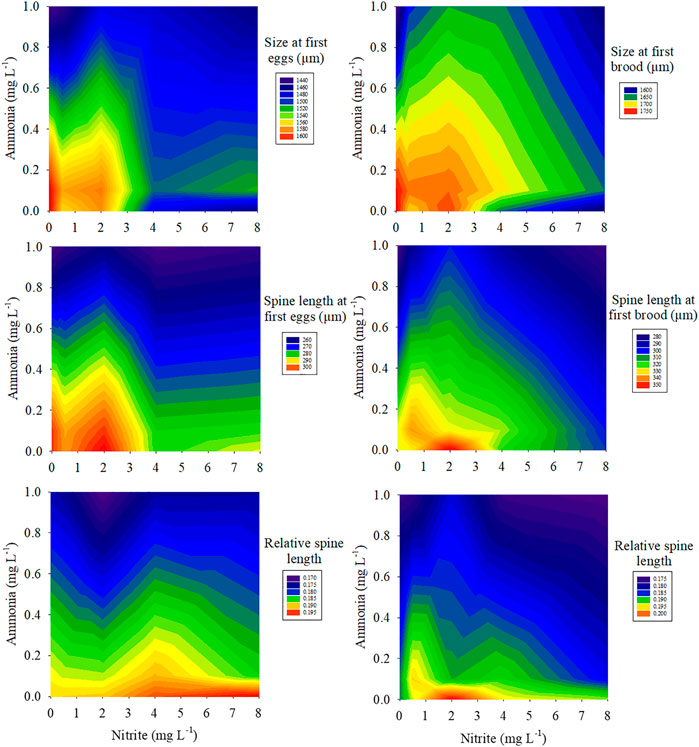
FIGURE 2. Combined effects of different nitrite concentrations (0, 0.5, 2, 4, 8 mg L−1) and ammonia concentrations (0, 0.1, 1 mg L−1) on size, spine length, relative spine length at first eggs and first brood.
Reproductive performances
Under the same ammonia concentration, with the increase of nitrite concentration, the total offspring per female and the number of offspring per brood showed a significant downward trend, and with the increase of ammonia concentration, the downward trend became more sharply (Figure 3). Compared with 0 mg L−1 nitrite, when nitrite concentration was 8 mg L−1 and ammonia concentration was 0, 0.1, and 1 mg L−1, the total offspring per female decreased by 68%, 71%, and 78%, respectively. In addition, nitrite and ammonia had significant inhibitory effects on the number of broods of D. pulex. The results of two-way ANOVA showed that there was an interaction between nitrite and ammonia (Table 1). Similarly, compared with 0 mg L−1 nitrite, when the nitrite concentration was 8 mg L−1 and the ammonia concentration was 0, 0.1, and 1 mg L−1, the total number of broods per individual for 21 days decreased by 33.3%, 50%, and 56.3%, respectively (Figure 3).
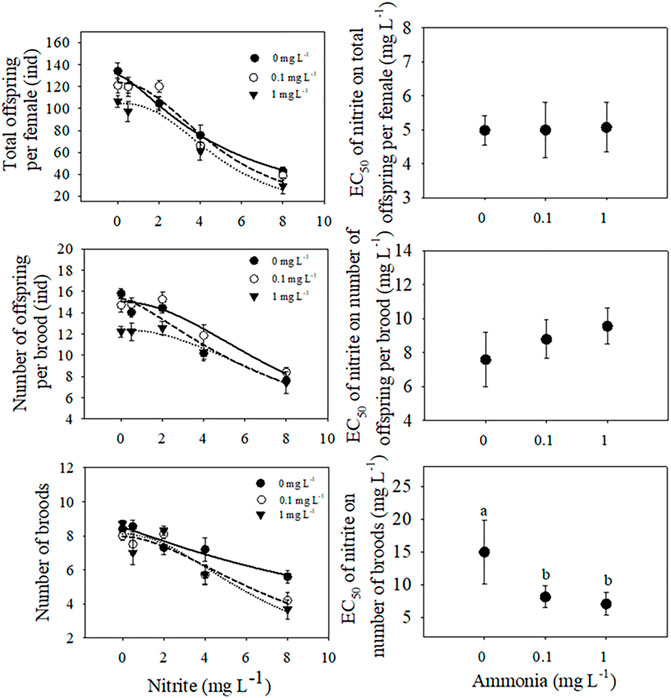
FIGURE 3. The total offspring number, the number of offspring per brood and the number of broods of D. pulex under different nitrite concentrations (0, 0.5, 2, 4, 8 mg L−1) and different ammonia concentrations (0, 0.1, 1 mg L−1). The three-parameter Sigmoidal model
Discussion
In recent years, the rise of nitrogenous pollutants has aroused widespread concern. Aquatic organisms face the stress of nitrogenous pollutants, among which nitrite and ammonia nitrogen are two of the most common and abundant pollutants in freshwater and coastal ecosystems (Howarth et al., 2000). Especially in water bodies with excess nutrients, it is found that high levels of nitrite and ammonia will cause serious physiological disorders of aquatic animals and lead to a large number of fish deaths (Medeiros et al., 2016). Through our experiment, we concluded that nitrite and ammonia had obvious toxic effects on D. pulex respectively, and nitrite and ammonia had significant interaction on some life history traits of D. pulex. Specially, the presence of ammonia reduced the tolerance of D. pulex to nitrite, and vice versa. The above results well confirmed our scientific hypothesis.
In this study, the survival rate of D. pulex decreased significantly with the increase of nitrite concentrations, and decreased more sharply under the condition of simultaneous ammonia stress, indicating that there was a certain synergy between the two nitrogenous pollutants. Some studies exposed Daphnia magna to ammonium, nitrite, nitrate and phosphate respectively for 7 days, and the mortality increased with the increase of nitrite and ammonium concentration (Serra et al., 2019), which was similar to the results observed in our experiment. In addition, long-term nitrite and ammonia exposure Daphnia obtusa and Daphnia similoides showed similar results (Xiang et al., 2012). According to the EC50 value of survival time obtained from Sigmoidal model, it can be found that the existence of ammonia not only shortened the life span of D. pulex, but also reduced the tolerance of D. pulex to nitrite. Conversely, nitrite also reduced the tolerance of D. pulex to ammonia. Nitrite can diffuse into the red blood cells of aquatic animals and oxidize the hemoglobin in the red blood cells into methemoglobin, thereby reducing the oxygen carrying capacity of the blood (Kroupova et al., 2005), destroying the ion regulation and respiratory system, leading to oxidative stress (Umbreit, 2007), finally suffocating aquatic animals. As for the non-ionic ammonia, it has good liposolubility and is easy to diffuse into aquatic organisms through cell membrane, causing damage to important organs including gill tissue, resulting in dyspnea, and inhibiting growth and development (Liu et al., 2014). The non-ionic ammonia directly affects the metabolism of enzymes, leading to disorder of enzyme metabolism, reducing immunity, stimulating aquatic organisms to produce a series of toxic reactions such as excitement and convulsion, and eventually leading to death. In addition, ammonia can inhibit ATP synthesis, resulting in that cladocerans do not have enough energy to maintain their own growth and reproduction (Fan et al., 2018).
When studying the effects of external stress on the life history traits of cladocerans, the time to first batch of eggs and body size at maturation are generally used as important indicators to show the development. In this study, the increase of nitrite and ammonia concentration affected the development of D. pulex, which was mainly manifested in the delay of the time to first batch of eggs and time to first brood, and the reduction of body size, tail spine length, and the relative tail spine length. When nitrite interacted with ammonia, the growth and development of D. pulex were more severely stressed. Within the nitrite concentration range less than 8 mg L−1, there was no delay in the time to first batch of eggs and the time to first brood of D. pulex. When the nitrite concentration was higher than 4 mg L−1, the body size and tail spine length of D. pulex at maturation decreased, and the inhibition effect was significant. When nitrite accumulates to a certain level, it will adversely affect the endocrine system (Panesar and Chan, 2001), excretion process (Cheng and Chen, 1998), and antioxidant system (Wang et al., 2017) of D. pulex, impair its normal physiological function and energy metabolism process, and cause the development of D. pulex to be inhibited. Hannas et al. (2010) have proved that nitrite will be toxic to the development and reproduction of cladocerans at environmentally relevant concentrations, which may be also because it is converted into nitric oxide in cells. Nitric oxide plays an important role in the regulation of multiple reproductive functions of vertebrates and invertebrates (Nighorn et al., 1998), and too high will cause many adverse consequences. Some studies have reported the negative effects of nitrite or ammonia stress on the development of aquatic organisms, for example, Yang et al. (2012) found that with the increase of ammonia concentration, the maturation time of D. magna were delayed, and fecundity was decreased.
Compared with the treatment without pollutants, nitrite and ammonia treatments significantly reduced the total offspring, the average offspring per brood and the number of broods of D. pulex. When nitrite concentration was 8 mg L−1 and ammonia concentration was 1 mg L−1, the number of broods decreased by 50%, which may be due to the reproductive strategy response of the maternal D. pulex to the stress. According to the EC50 value of brood number obtained from Sigmoidal model, we found that the presence of ammonia not only reduced the reproduction of D. pulex, but also decreased the tolerance of D. pulex to nitrite. Similarly, nitrite also reduced the tolerance of D. pulex to ammonia. We speculated that when cladocerans were exposed under adverse environmental stress, they would invest part of their energy to resist stress. Due to the combined effect of nitrite and ammonia, the energy consumption of D. pulex was increased, and the energy invested in growth, development, and reproduction was greatly reduced (Hanazato and Dodson, 1995). Similarly, studies have found that the development and reproductive characteristics of Daphnia similis are inhibited under the conditions of ammonia and hypoxia, i.e., the time to first brood is delayed, the body length is reduced, and the number of offspring is decreased (Lyu et al., 2013a). The toxicity of ammonia under the conditions of hypoxia and normoxia is different, and hypoxia will greatly increase the toxic effect of ammonia (Wajsbrot et al., 1991). This is similar to the phenomenon revealed in this experiment, i.e., the interaction between nitrite and ammonia may affect the balance of energy distribution. Nitrite and ammonia stress can reduce the metabolic rate of cladocerans, which increases the risk of death and reduces reproductive output due to the imbalance of energy distribution (Jensen, 1996; Ip and Chew, 2010; Medeiros et al., 2016; Fan et al., 2018). Therefore, the reproduction decreased due to the stress of nitrogenous pollutants, which eventually led to the decline of cladocerans population and affect the stability of aquatic ecosystem.
Conclusion
The results of this study showed that environmentally relevant or high concentrations of nitrite and ammonia exposure had a significantly negative impact on the key life history traits of D. pulex. Under nitrite and ammonia stress, the survival time of D. pulex was shortened, the time to first batch of eggs and the time to first brood were delayed, the body size and tail spine length were reduced, and the number of offspring and broods were decreased. There was a certain synergistic negative effect between the two nitrogenous pollutants. The presence of ammonia reduced the tolerance of D. pulex to nitrite, and vice versa. In contrast, within the concentration range set in this experiment, nitrite may have a stronger toxic effect on the development and reproduction of D. pulex. As these two nitrogenous pollutants often co-exist in the water body, and can be transformed into each other, such study provided new insights into the combined toxic effects of nitrite and ammonia on the life history traits of cladocerans, which was of great significance to understand the population dynamics of cladocerans and the stability of aquatic ecosystem under the condition of compound nitrogenous pollutants.
Data availability statement
The raw data supporting the conclusions of this article will be made available by the authors, without undue reservation.
Author contributions
YS and JL conceived and designed the experiment. BY performed the experiments and analyzed the data with JL and KL. BY, YS, and ZY wrote the paper. All authors gave their final approval of the submitted and published versions.
Funding
The study was supported by National Natural Science Foundation of China (31730105) and the Priority Academic Program Development of Jiangsu Higher Education Institutions of China.
Conflict of interest
The authors declare that the research was conducted in the absence of any commercial or financial relationships that could be construed as a potential conflict of interest.
Publisher’s note
All claims expressed in this article are solely those of the authors and do not necessarily represent those of their affiliated organizations, or those of the publisher, the editors and the reviewers. Any product that may be evaluated in this article, or claim that may be made by its manufacturer, is not guaranteed or endorsed by the publisher.
References
Akbar, S., Gu, L., Sun, Y. F., Zhou, Q. M., Zhang, L., Lyu, K., et al. (2020). Changes in the life history traits of Daphnia magna are associated with the gut microbiota composition shaped by diet and antibiotics. Sci. Total Environ. 705, 135827. doi:10.1016/j.scitotenv.2019.135827
Atwood, H. L., Tomasso, J. R., Ronan, P. J., Barton, B. A., and Renner, K. J. (2000). Brain monoamine concentrations as predictors of growth inhibition in channel catfish exposed to ammonia. J. Aquat. Anim. Health 122 (1), 69–73. doi:10.1577/1548-8667(2000)012<0069:bmcapo>2.0.co;2
Badiola, M., Basurko, O. C., Piedrahita, R., Hundley, P., and Mendiola, D. (2018). Energy use in recirculating aquaculture systems (ras): A review. Aquac. Eng. 81, 57–70. doi:10.1016/j.aquaeng.2018.03.003
Cao, H. S., Lyu, K., Xiang, F. H., and Yang, Z. (2014). Life history responses of Daphnia similoides simultaneously exposed to microcystin-LR and ammonia and their post exposure recovery. Environ. Toxicol. Chem. 33, 2497–2505. doi:10.1002/etc.2701
Cheng, C. H., Ma, H. L., Deng, Y. Q., Feng, J., Jie, Y. K., and Guo, Z. X. (2020). Effect of ammonia on the immune response of mud crab (Scylla paramamosain) and its susceptibility to mud crab reovirus. Isr. J. Aquacult.-Bamid. 72, 1131765. doi:10.46989/001c.21468
Cheng, S. Y., and Chen, J. C. (1998). Effects of nitrite exposure on the hemolymph electrolyte, respiratory protein and free amino acid levels and water content of Penaeus japonicus. Aquat. Toxicol. 44 (1-2), 129–139. doi:10.1016/S0166-445X(98)00064-2
Emerson, K., Russo, R. C., Lund, R. E., and Thurston, R. V. (1975). Aqueous ammonia equilibrium calculations: Effect of pH and temperature. J. Fish. Res. Bd. Can. 32 (12), 2379–2383. doi:10.1139/f75-274
Fan, S. H., Wang, Y. Y., Zhang, Z. F., Lu, J., Wu, Z. Y., Shan, Q., et al. (2018). High expression of glutamate-ammonia ligase is associated with unfavorable prognosis in patients with ovarian cancer. J. Cell. Biochem. 119 (7), 6008–6015. doi:10.1002/jcb.26797
Freitas, E. C., and Rocha, O. (2011). Acute toxicity tests with the tropical cladoceran Pseudosida ramosa: The importance of using native species as test organisms. Arch. Environ. Contam. Toxicol. 60 (2), 241–249. doi:10.1007/s00244-010-9541-2
Gan, X. Y., Shen, G. Z., Xin, B., and Li, M. (2016). Simultaneous biological desalination and lipid production by Scenedesmus obliquus cultured with brackish water. Desalination 400, 1–6. doi:10.1016/j.desal.2016.09.012
Hanazato, T., and Dodson, S. I. (1995). Synergistic effects of low oxygen concentration, predator kairomone, and a pesticide on the cladoceran Daphnia pulex. Limnol. Oceanogr. 40 (4), 700–709. doi:10.4319/lo.1995.40.4.0700
Hannas, B. R., Das, P. C., Li, H., and LeBlanc, G. A. (2010). Intracellular conversion of environmental nitrate and nitrite to nitric oxide with resulting developmental toxicity to the crustacean Daphnia magna. Plos One 5 (8), e12453. doi:10.1371/journal.pone.0012453
Howarth, R., Anderson, D., Cloern, J., Elfring, C., Hopkinson, C., Lapointe, B., et al. (2000). Nutrient pollution of coastal rivers, bays, and seas. Issues Ecol. 7, 1–15.
Huang, J., Li, Y. R., Zhou, Q. M., Sun, Y. F., Zhang, L., Gu, L., et al. (2020). Non-toxic and toxic Microcystis aeruginosa reduce the tolerance of Daphnia pulex to low calcium in different degrees: Based on the changes in the key life-history traits. Chemosphere 248, 126101. doi:10.1016/j.chemosphere.2020.126101
Ip, Y. K., and Chew, S. F. (2010). Ammonia production, excretion, toxicity, and defense in fish: A review. Front. Physiol. 1, 134. doi:10.3389/fphys.2010.00134
Iwata, K. (1988). Nitrogen metabolism in the mudskipper, Periophthalmus cantonens: Changes in free amino acids and related compounds in various tissues under conditions of ammonia loading, with special reference to its high ammonia tolerance. Comp. Biochem. Physiology Part A Physiology 91 (3), 499–508. doi:10.1016/0300-9629(88)90625-1
Jensen, F. B. (1996). Uptake, elimination and effects of nitrite and nitrate in freshwater crayfish (Astacus astacus). Aquat. Toxicol. 34 (2), 95–104. doi:10.1016/0166-445X(95)00030-8
Kamstra, A., Span, J. A., and Weerd, J. H. (1996). The acute toxicity and sublethal effects of nitrite on growth and feed utilization of European eel, Anguilla anguilla (L.). Aquac. Res. 27 (12), 903–911. doi:10.1111/j.1365-2109.1996.tb01250.x
Kilham, S. S., Kreeger, D. A., Lynn, S. G., Goulden, C. E., and Herrera, L. J. H. (1998). Combo: A defined freshwater culture medium for algae and zooplankton. Hydrobiologia 377 (1-3), 147–159. doi:10.1023/a:1003231628456
Kroupova, H., Machova, J., and Svobodova, Z. (2005). Nitrite influence on fish: A review. Veterinarni Med. 50 (11), 461–471. doi:10.17221/5650-vetmed
Liu, Y., Jiang, D. S., Li, Y. J., Zhang, R. F., and Cui, Y. B. (2014). Influence of environmental factors on the acute toxicity of ammonia to Corbicula fluminea and Limnodrilus hoffmeisteri. Res. Environ. Sci. 27 (9), 1067–1073. doi:10.13198/j.issn.1001-6929.2014.09.16
Lyu, K., Cao, H. S., Chen, R., Wang, Q. Q., and Yang, Z. (2013a). Combined effects of hypoxia and ammonia to Daphnia similis estimated with life-history traits. Environ. Sci. Pollut. Res. 20 (8), 5379–5387. doi:10.1007/s11356-013-1555-7
Lyu, K., Wang, Q. Q., Chen, R., Lu, Q. L., and Yang, Z. (2013b). Inter-specific differences in survival and reproduction of cladocerans to nitrite gradient and the ecological implications. Biochem. Syst. Ecol. 48, 151–156. doi:10.1016/j.bse.2012.12.002
Lyu, K., Zhu, X. X., Wang, Q. Q., Chen, Y. F., and Yang, Z. (2013c). Copper/zinc superoxide dismutase from the cladoceran daphnia magna: Molecular cloning and expression in response to different acute environmental stressors. Environ. Sci. Technol. 47 (15), 8887–8893. doi:10.1021/es4015212
Medeiros, R. S., Lopez, B. A., Sampaio, L. A., Romano, L. A., and Rodrigues, R. V. (2016). Ammonia and nitrite toxicity to false clownfish Amphiprion ocellaris. Aquacult. Int. 24 (4), 985–993. doi:10.1007/s10499-015-9965-9
Nighorn, A., Gibson, N. J., Rivers, D. M., Hildebrand, J. G., and Morton, D. B. (1998). The nitric oxide–cGMP pathway may mediate communication between sensory afferents and projection neurons in the antennal lobe ofManduca sexta. J. Neurosci. 18, 7244–7255. doi:10.1523/jneurosci.18-18-07244.1998
Panesar, N. S., and Chan, K. W. (2001). Decreased steroid hormone synthesis from inorganic nitrite and nitrate: Studies in vitro and in vivo. Toxicol. Appl. Pharmacol. 169 (3), 222–230. doi:10.1006/taap.2000.9079
Sarma, S. S. S., and Nandini, S. (2006). Review of recent ecotoxicological studies on cladocerans. J. Environ. Sci. Health Part B 41 (8), 1417–1430. doi:10.1080/03601230600964316
Serra, T., Soler, M., Pous, N., and Colomer, J. (2019). Daphnia magna filtration, swimming and mortality under ammonium, nitrite, nitrate and phosphate. Sci. Total Environ. 656, 331–337. doi:10.1016/j.scitotenv.2018.11.382
Sun, H. J., Lu, K., Minter, E. J. A., Chen, Y. F., Yang, Z., and Montagnes, D. J. S. (2012). Combined effects of ammonia and microcystin on survival, growth, antioxidant responses, and lipid peroxidation of bighead carp Hypophthalmythys nobilis larvae. J. Hazard. Mat. 221, 213–219. doi:10.1016/j.jhazmat.2012.04.036
Sun, H., Wang, W., Geng, L., Chen, Y., and Yang, Z. (2013). In situ studies on growth, oxidative stress responses, and gene expression of juvenile bighead carp (Hypophthalmichthys nobilis) to eutrophic lake water dominated by cyanobacterial blooms. Chemosphere 93 (2), 421–427. doi:10.1016/j.chemosphere.2013.05.022
Sun, Y. F., Yu, B., Lei, Y. L., Qin, S. S., Lyu, K., and Yang, Z. (2022). Differential comprehensive effects of food quality and ZnO nanoparticles on the key traits of early life history of Daphnia magna. Front. Environ. Sci. 10, 918570. doi:10.3389/fenvs.2022.918570
Tiso, M., and Schechter, A. N. (2015). Nitrate reduction to nitrite, nitric oxide and ammonia by gut bacteria under physiological conditions. Plos One 10 (3), e0119712. doi:10.1371/journal.pone.0119712
Umbreit, J. (2007). Methemoglobin—it's not just blue: A concise review. Am. J. Hematol. 82, 134–144. doi:10.1002/ajh.20738
van Kessel, M., Speth, D. R., Albertsen, M., Nielsen, P. H., Op den Camp, H. J. M., Kartal, B., et al. (2015). Complete nitrification by a single microorganism. Nature 528 (7583), 555–559. doi:10.1038/nature16459
Visek, W. J. (1984). Ammonia: Its effects on biological systems, metabolic hormones, and reproduction. J. Dairy Sci. 67 (3), 481–498. doi:10.3168/jds.S0022-0302(84)81331-4
Wajsbrot, N., Gasith, A., Krom, M. D., and Popper, D. M. (1991). Acute toxicity of ammonia to juvenile gilthead seabream Sparus aurata under reduced oxygen levels. Aquaculture 92, 277–288. doi:10.1016/0044-8486(91)90029-7
Wang, J., Tang, H. X., Zhang, X. X., Xue, X. F., Zhu, X. X., Chen, Y. F., et al. (2018). Mitigation of nitrite toxicity by increased salinity is associated with multiple physiological responses: A case study using an economically important model species, the juvenile obscure puffer (Takifugu obscurus). Environ. Pollut. 232, 137–145. doi:10.1016/j.envpol.2017.09.026
Wang, L., Jie, H., Guo, H., Li, L., Rong, T. J., Zhang, D., et al. (2017). The synergistic effects of waterborne microcystin-LR and nitrite on hepatic pathological damage, lipid peroxidation and antioxidant responses of male zebrafish. Environ. Pollut. 235, 197–206. doi:10.1016/j.envpol.2017.12.059
Wang, S., Liu, X., and Wu, L. (2013). Effects of temperature and free ammonia concentration on nitrification between the aerobic granular and the floccular sludges. J. Beijing Univ. Technol. 39 (2), 297–303. doi:10.1016/j.biortech.2005.03.032
Wu, H. Z., and Cao, A. E. (2013). “Preparation and adding methods of nessler's reagent Having effects on determination of water quality ammonia nitrogen,” in 2. Int. C. En. Env. Prot. (ICEEP), 1362–1366.
Xiang, F. H., Geng, L. L., Lu, K., Zhang, J., Minter, E. J. A., and Yang, Z. (2012). Effect of long-term nitrite exposure on the cladoceran Daphnia obtusa: Survival, moults, and reproduction. Biochem. Syst. Ecol. 41, 98–103. doi:10.1016/j.bse.2011.12.006
Xu, Z. K., Cao, J., Qin, X. M., Qiu, W. Q., Mei, J., and Xie, J. (2021). Toxic effects on bioaccumulation, hematological parameters, oxidative stress, immune responses and tissue structure in fish exposed to ammonia nitrogen: A review. Animals 11, 11113304. doi:10.3390/ani11113304
Yang, W., Xiang, F. H., Sun, H. J., Chen, Y. F., Minter, E., and Yang, Z. (2010). Changes in the selected hematological parameters and gill Na+/K+ ATPase activity of juvenile crucian carp Carassius auratus during elevated ammonia exposure and the post-exposure recovery. Biochem. Syst. Ecol. 38 (4), 557–562. doi:10.1016/j.bse.2010.06.005
Yang, Z., Lu, K., Chen, Y. F., and Montagnes, D. J. S. (2012). The interactive effects of ammonia and microcystin on life-history traits of the cladoceran Daphnia magna: Synergistic or antagonistic? Plos One 7, e32285. doi:10.1371/journal.pone.0032285
Yang, Z., Xiang, F., Minter, E. J. A., Lü, K., Chen, Y., and Montagnes, D. J. S. (2011). The interactive effects of microcystin and nitrite on life-history parameters of the cladoceran Daphnia obtusa. J. Hazard. Mat. 190 (1), 113–118. doi:10.1016/j.jhazmat.2011.03.002
Keywords: ammonia, Daphnia pulex, development, life history, nitrite, reproduction
Citation: Yu B, Lyu K, Li J, Yang Z and Sun Y (2022) Combined toxic effects of nitrite and ammonia on life history traits of Daphnia pulex. Front. Environ. Sci. 10:1019483. doi: 10.3389/fenvs.2022.1019483
Received: 15 August 2022; Accepted: 14 October 2022;
Published: 26 October 2022.
Edited by:
Hai Ocean Xu, Jiangsu University, ChinaReviewed by:
Huihui Chen, Nanjing Institute of Geography and Limnology (CAS), ChinaAndreas Heyland, University of Guelph, Canada
Copyright © 2022 Yu, Lyu, Li, Yang and Sun. This is an open-access article distributed under the terms of the Creative Commons Attribution License (CC BY). The use, distribution or reproduction in other forums is permitted, provided the original author(s) and the copyright owner(s) are credited and that the original publication in this journal is cited, in accordance with accepted academic practice. No use, distribution or reproduction is permitted which does not comply with these terms.
*Correspondence: Jiajia Li, anNsaWpqQDE2My5jb20=; Yunfei Sun, c3VueXVuZmVpQG5qbnUuZWR1LmNu