- 1Shaanxi Key Laboratory of Ecological Restoration in Shaanbei Mining Area, Yulin University, Yulin, Shaanxi, China
- 2State Key Laboratory of Soil Erosion and Dryland Farming on the Loess Plateau, Northwest A&F University, Yangling, Shaanxi, China
Laboratory-simulated experiments under a one-way wind erosion–rain erosion sequence were conducted to investigate the effect of S. psammophila roots on wind and water erosion processes and quantify its erosion-reducing potential. With the collected soil of sandy loam and planted shrub of S. psammophila, 16 soil boxes including bare and root-permeated soils were arranged in March 2017 and conducted in August 2017. With the wind speeds of 11 and 14 m s−1 and rainfall intensities of 60 and 100 mm h−1, two levels of interaction (11 m s−1 × 60 mm h−1 and 14 m s−1 × 100 mm h−1) were designed. The particle-size composition and sediment transport flux were examined in the former wind tunnel experiments, and the runoff hydrodynamic parameters and runoff and water erosion rates were determined in the following rainfall tests. The sediment reduction effect by roots (%) was used to quantify the erosion-reducing potential of roots. The results demonstrated that in the former wind tunnel experiments, compared with the bare soils, the root-permeated soils showed a slight coarsening of surface soil and had 18.03% and 35.71% less sediment transport flux at wind speeds of 11 and 14 m s−1, respectively. In the following rainfall tests, S. psammophila roots weakened the hydrodynamic intensity of overland flow and decreased runoff and water erosion rates by 13.34%, 30.70% and 4.44%, 43.72% at rainfall intensities of 60 and 100 mm h−1, respectively. Different from the water erosion process of bare soils, which showed an increased fluctuated trend, the root-permeated soils presented a steady increase in the early stage of rainfall and then a decrease-stable trend at the mid and end of rainfall. In the wind tunnel–rainfall experiments, the sediment reduction effect by Salix psammophila roots showed 24.37% and 39.72% at levels of 11 m s−1 × 60 mm h−1 and 14 m s−1 × 100 mm h−1, respectively. This kind of study may provide more insights into understanding ecological impacts of sandy vegetation construction on the water–wind crisscrossed erosion region of the Chinese Loess Plateau and also sandy land.
Introduction
On the Loess Plateau of China, the water–wind crisscrossed erosion region (35°20’∼40°10’ N, 103°33’∼113°53’ E), where wind and water erosion occurred alternately in temporal and distributed crisscross accelerating spatial variability, is the most serious erosion zone and the coarse sediment source area in the Yellow River (Wang et al., 2016; Tuo et al., 2018). Decades of practice had proved that vegetation played an important role in preventing wind or water erosion in this area (Wang et al., 2017; Lu et al., 2019). It is commonly known that controlling soil erosion by vegetation is mainly attributed to the combined effects of above-ground parts and below-ground roots (Zhang et al., 2012a; Zhao et al., 2017). As to the erosion-reducing effect of above-ground parts such as canopy (Thompson et al., 2015), stem (Mu et al., 2019), and also leaf litter (Liu et al., 2017), it has always been of great concern due to its significant weakening of air momentum or rainfall energy and increasing of wind or water erosion resistance. With respect to the below-ground roots, which has direct and close contact with the soils, owing to its great contribution to the improvement of soil infiltration (Wang et al., 2017), soil shear strength (Hao et al., 2020), and soil structure (Hallett et al., 2009), it was often more pronounced in retarding water erosion, especially for the concentrated flow erosion (Gyssels et al., 2005; Vannoppen et al., 2015). Through analyzing numerous relevant studies, it could be easily found that, over the last several decades, the knowledge of relationship between plant roots and soil erosion has been mostly focused on the root effects on water erosion (Ghidey and Alberts, 1997; Mamo and Bubenzer, 2001; Li et al., 2017; Hao et al., 2021), and the information or direct experiment data concerning the influence of roots on wind erosion are very limited.
In the water–wind crisscrossed erosion region of the Loess Plateau, due to the severe complex erosion by wind and water, it was often observed that a part of the below-ground roots was exposed to air, especially for the shrubs (e.g., S. psammophila) grown on the windward slope of a moving dune or semi-fixed dune (Figure 1) (Chen and Zhao, 2015; Zhang, 2018). According to Dong (2005), under the certain combination of exposed roots and flow velocity, a sooner or later phenomenon of air flowing around the exposed roots occurred, which increased the sectional resistance coefficient and thus decreased the surface flow velocity. Furthermore, among the exposed roots, the internal air turbulence produced and then enlarged collision frequency between soil particles and the exposed roots. As a result, some soil particles might be captured by the exposed roots. Most recently, a few studies conducted a simulated wind tunnel experiment and investigated the wind erosion resistance of roots. Cao (2013) initiatively created soil samples of 8 cm × 8 cm × 3 cm (length × width × height) dimensions, which were mixed into roots (death) of Setaria viridis, and observed that such “roots” could reduce soil loss by 24.73%. Thereafter, Li (2016) used the soil samples with the mixed “roots” which came from the fiber of Trachycarpus fortunei and pine needle and stalk of Sorghum bicolor, and the reported results further pronounced that the roots should be believed to play an effective and reliable role in mitigating wind erosion. These aforementioned studies are well known and accepted. However, the achieved knowledge about the root effects on wind erosion was only confined to the simulated roots, which could not exactly represent the interactions between roots and soils. In addition, there is a lack of information about root effects on water erosion followed by wind erosion since both the types of erosion occur alternately throughout the year in the water–wind crisscrossed erosion region of the Chinese Loess Plateau. Therefore, it is essential to provide more insights into this topic for the in-depth understanding of sandy plant root reinforcement on soil.
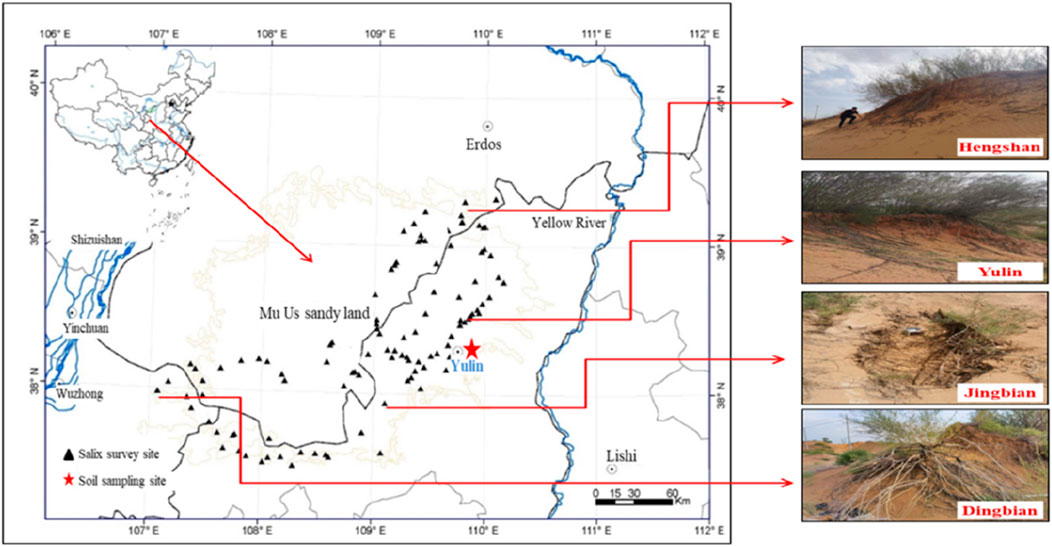
FIGURE 1. Distribution of the S. psammophila survey site and soil sampling site in the water–wind crisscrossed erosion region of the Chinese Loess Plateau.
Salix psammophila, which is widely distributed in this area, is recognized as a typical and dominant shrub species of wind breaking and sand fixation. In the present study, a simulated experiment of wind tunnel first and rainfall thereafter was conducted, and the primary objectives were to i) investigate the effect of S. psammophila roots on the former wind erosion (particle-size composition and sediment transport flux) and the following water erosion (runoff hydrodynamic characteristics and runoff and water erosion rates) and ii) quantify the erosion-reducing potential of S. psammophila roots in the wind tunnel–rainfall experiments. It was hypothesized that the soils under different designs would make some differences in their soil resistance to wind and/or water erosion, and it was believed that the erosion-reducing potential of roots would be achieved.
Materials and methods
Experimental soil and equipment
The experimental soil for this study was a typical sandy loam soil with 12.77% clay (<2 μm), 21.17% silt (2 –50 μm), and 66.06% sand (50 μm–2 mm), collected from the upper 20 cm soil layer in an area of S. psammophila land without plant cover at Yulin County, Shaanxi Province, China (Figure 1). The collected soil was air-dried to a moisture content of approximately 1.5% and then sieved (a 5-mm sieve) after the removal of visible stones, weeds, roots, and other debris. A kind of metal box (length = 110 cm, width = 70 cm, and depth = 35 cm), with the dual function of a movable and adjustable slope gradient, was constructed to hold the collected soils for wind tunnel and rainfall experiments (Figure 2). The metal box was filled at a bulk density of 1.30 g cm−3, which was similar to the field conditions, in layer by layer (every 5 cm layer) to a total depth of 35 cm. After filling and placing in a shelter for 15 days so as to allow the natural sedimentation of soils, the experimental box was planted with the cuttings (20 cm length) taken from S. psammophila and sprayed evenly with 2 L tap water at intervals of 7 days.
The simulated experiments were conducted at the Simulation Hall of the State Key Laboratory of Soil Erosion and Dryland Farming on the Loess Plateau in the Institute of Soil and Water Conservation, Chinese Academy of Sciences and Ministry of Water Resources, Yangling. A wind tunnel and a rainfall simulator were used for simulating wind and water erosion (Figure 2). The wind tunnel, with dimensions of 24 m × 1.2 m × 1 m (length × width × height), consisted of a driving system (fan section, wind regulation, and rectification section) and a measuring system (experimental section, sand collection, and wind diversion section). The uniformity of the produced airflow was >99%, and the wind speed could be continuously switched from 0 to 15 m s−1 by adjusting frequency of the matching inverter (0 –50 Hz) (Wang et al., 2014). For collection of wind-blown particles at different heights and thus measurement of the sediment transport flux profile, a multi-opening sampler equipped with 15 removable rectangular sand chambers (length = 15 cm, width = 3 cm, and height = 1 cm) was set in the center of the sand collection section close to the wind outlet of the experimental box (Dong et al., 2004). The rainfall simulator with the nozzle height of 16 m was used, and the rainfall intensity could be precisely controlled by adjusting the aperture of the nozzle and water pressure (Pan and Shangguan, 2006).
Experimental design and process
The examined experimental treatments in the present study were bare soils (0 cuttings per box) and S. psammophila root-permeated soils (5, 10, and 15 cuttings per box), which were arranged in mid-March 2017 and conducted on August 12–26, 2017. Considering the characteristics of soil erosion in this area that showed an alternating cycle of winter–spring wind erosion and summer–autumn water erosion (Song et al., 2006), this experiment was designed with the wind tunnel first and rainfall thereafter under a one-way wind erosion–rain erosion sequence. Two wind speeds (11 and 14 m s−1) and rainfall intensities (60 and 100 mm h−1) were selected, and two levels of interaction between wind speed and rainfall intensity (11 m s−1 × 60 mm h−1 and 14 m s−1 × 100 mm h−1) were designed. The wind speed of 11 m s−1 represents a typical wind event and that of 14 m s−1 reflects the maximum monthly average wind speed. The rainfall intensities of 60 and 100 mm h−1 were representatives of low- and high-intensity erosion events in the study area, respectively (Tuo et al., 2016). A total of 16 boxes were prepared, and 16 wind tunnel–rainfall events were performed as 2 levels of interaction (11 m s−1 × 60 mm h−1 and 14 m s−1 × 100 mm h−1) × 4 planting densities (0, 5, 10, and 15 cuttings per box) × 2 replications.
For investigation of individual root effects on soil erosion, it was thought practicable to cut off the above-ground parts of the plant (Zhou and Shangguan, 2007; Zhang et al., 2012a). Therefore, before the wind tunnel tests, the above-ground parts of S. psammophila were cut off to the ground level using a pruner and its growth characteristics (height, diameter, and biomass) were measured (Table 1). Then, the experimental boxes were carefully pushed into the wind tunnel. The soil surface of the box was aligned exactly parallel to the floor of the experimental section and then exposed to the wind at designated wind speeds for 20 min. After each wind tunnel test, the collected wind-blown sediments were transferred into iron containers for oven-drying at 105°C to determine the sediment transport flux. Thereafter, the wind-blown box was carefully taken out of the wind tunnel and positioned at the appropriate location in the rain hall where the rainfall intensity and effective area were calibrated. Before rainfall tests, surface soil samples (0–1 cm) were collected and its particle composition was measured using a Mastersizer 2000 laser diffraction device. Then, the slope gradient of the experimental box was adjusted to 15, and the rainfall duration was set to 60 min after runoff was initiated. The KMnO4 dye technique was used to measure cross-section average flow velocity (Zhao et al., 2015). Runoff and the scoured sediments were collected every 3 min using the plastic buckets at the box outlet. After each rainfall test, all the plastic runoff-collection buckets were weighed and placed for a period of time. Then, the upper clear liquid was drained off, and the lower sediments were transferred into iron containers for oven-drying at 105° C. In addition, all root segments were carefully excavated and washed, and root characteristics such as root density (g m−3), root length density (mm−3), and root surface area density (cm2 m−3) were determined (Table 1). In wind tunnel–rainfall experiments, several indicators related to wind or water erosion were also measured and calculated (Table 2).
Statistical analysis
In the present study, the mean of each treatment (bare soil and S. psammophila root-permeated soil) was used for data analysis. The descriptive parameters were analyzed using Excel 2017, and the figures were created using SigmaPlot 14.0.
Results and discussion
Particle-size composition and sediment transport flux in wind tunnel tests
Wind erosion preferentially removes finer particles and thus coarsens soil texture (Zhao et al., 2006; Zhang et al., 2007). Table 3 illustrates that, after the wind tunnel tests, both bare and root-permeated soils showed a decrease in clay and silt contents and an increase in sand content to different degrees. However, our results also observed that the increase in the sand content in root-permeated soils (7.18%–10.07%) was less than that in bare soils (8.89%–11.72%), implying that roots weakened the coarsening of surface soil to a certain extent.
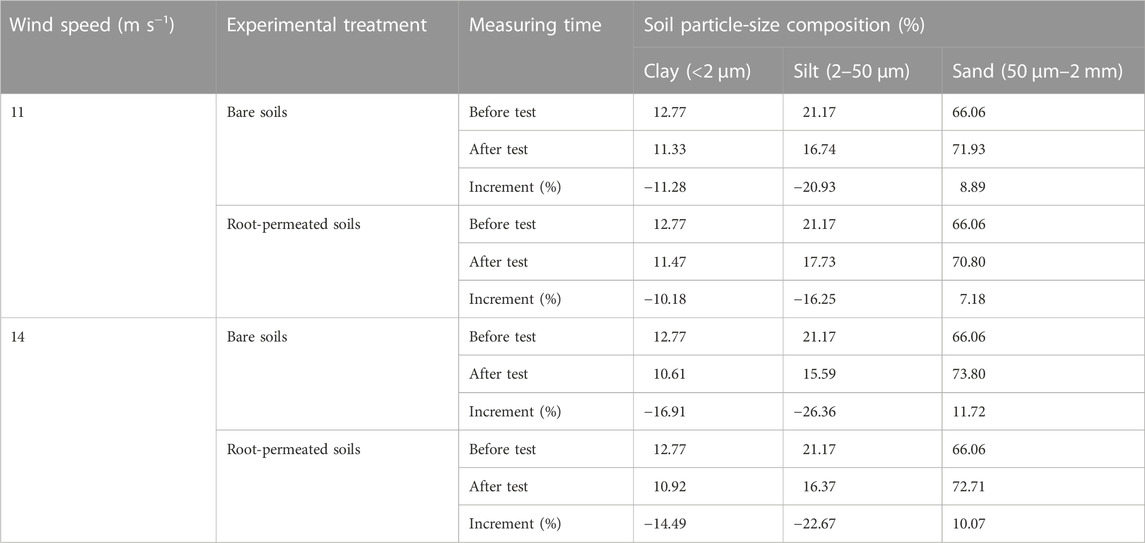
TABLE 3. Particle-size composition of experimental soils (0–1 cm) before and after wind tunnel tests.
The height profile of wind-eroded sediment transport flux is the reflection of blown sand particles that move in different trajectories and thus is the basis for a better understanding of the characteristics of the wind erosion process (Dong et al., 2003; Dong and Qian 2007). Figure 3 shows the characteristics of sediment transport flux at different heights above surface soil. At the designed wind speeds of 11 and 14 m s−1, both bare and root-permeated soils presented a similar height profile that sediment transport flux decreased with the increasing ground height. This height profile of wind-eroded sediment transport flux has been widely reported in many other studies (Tuo et al., 2016; Li et al., 2021). However, it should also be noted that, compared with the bare soils, root-permeated soils had always shown a low sediment transport flux at different ground heights, which indicated that roots effectively enhanced soil resistance to wind erosion and thus reduced soil loss to a certain extent. In our study, it was obviously observed that the soil surface of all the experimental soils was sheared with different degrees, and some linear fringes or rills were created that oriented from the wind direction (Figure 2). Interestingly, compared with the bare soils, the root-permeated soils showed less numbers and shallow depth of linear fringes or rills, which implied that roots weakened the shaping effect of wind erosion on soil surface micro-topography. By observing and analyzing, our statistical data demonstrated that the sediment reduction effect by roots recorded 18.03% at a wind speed of 11 m s−1, and this value increased to as high as 35.71% as the wind speed was increased up to 14 m s−1.
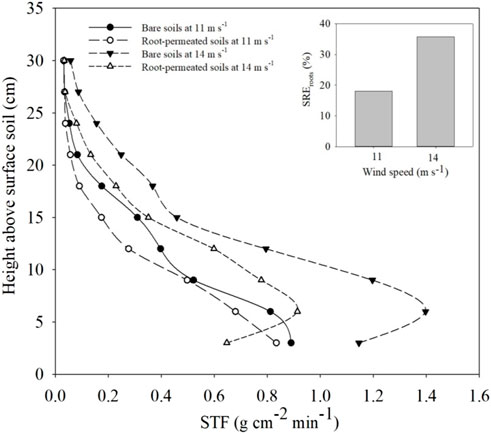
FIGURE 3. Sediment transport flux (STF) at different ground heights and the sediment reduction effect by roots (SREroots, %) at wind speeds of 11 and 14 m s−1.
Runoff hydrodynamic characteristics in rainfall tests
A quantitative understanding of runoff hydraulics such as flow velocity, Reynolds number, and drag coefficient could well explain and clarify the water erosion process and sediment yield (Govers, 1992; Lenoard and Richard, 2004). Table 4 shows the hydrodynamic characteristics of runoff for bare and root-permeated soils which suffered the former wind erosion. At a wind speed of 11 m s−1 combined with the rainfall intensity of 60 mm h−1, compared with bare soils, the flow velocity, Reynolds number, and unit stream power in root-permeated soils decreased by 21.79%, 13.18%, and 21.49%, respectively. In addition, the root-permeated soils also showed a higher flow depth and drag coefficient (11.34% and 88.89% increments compared to bare soils, respectively). These observations indicated that S. psammophila roots had a positive effect on weakening the hydrodynamic intensity of runoff. However, such root effects were seemed to be less pronounced as the wind speed and rainfall intensity increased (14 m s−1 × 100 mm h−1). This could be attributed to the more significant effect of rainfall intensity on runoff hydrodynamic intensity (Zhang et al., 2012b).
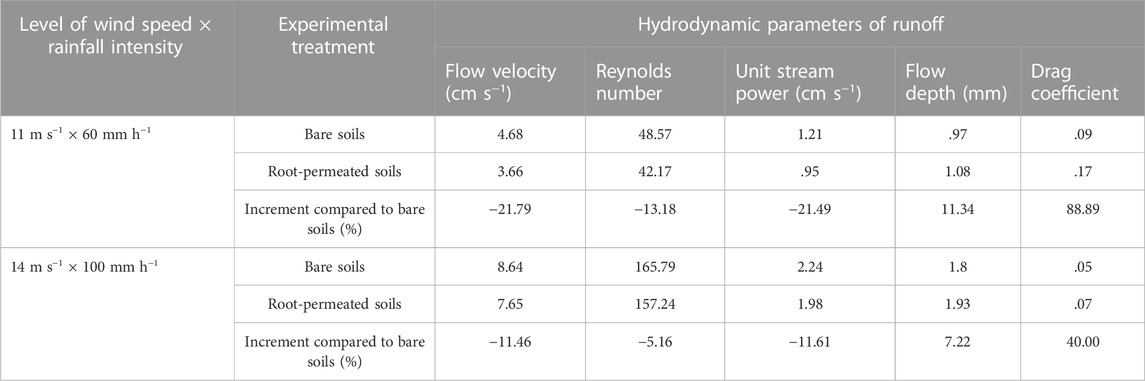
TABLE 4. Hydrodynamic parameters of runoff for bare and root-permeated soils in simulated rainfall following wind tunnel tests.
Runoff and water erosion rates in rainfall tests
Dynamics of runoff and water erosion rates with rainfall duration for the bare and root-permeated soils are shown in Figure 4. As to the characteristics of runoff generation, it can be seen that both bare and root-permeated soils presented a similar variation trend that runoff rates gradually increased and then appeared to approach a steady value with increases in rainfall duration. However, it should not be ignored that, compared with the bare soils, the root-permeated soils always had a low runoff rates in the process of runoff generation. This observation confirmed the positive effects of below-ground roots on runoff reduction (Zhao et al., 2017). Yet, our statistic data also indicated that this contribution of roots to reductions in runoff decreased at a combination of higher wind speed and rainfall intensity. Specifically, compared with the bare soils, under a level of 11 m s−1 × 60 mm h−1, the runoff rate in root-permeated soils could be decreased by 13.34%, but this value was reduced to 4.44% at a level of 14 m s−1 × 100 mm h−1.
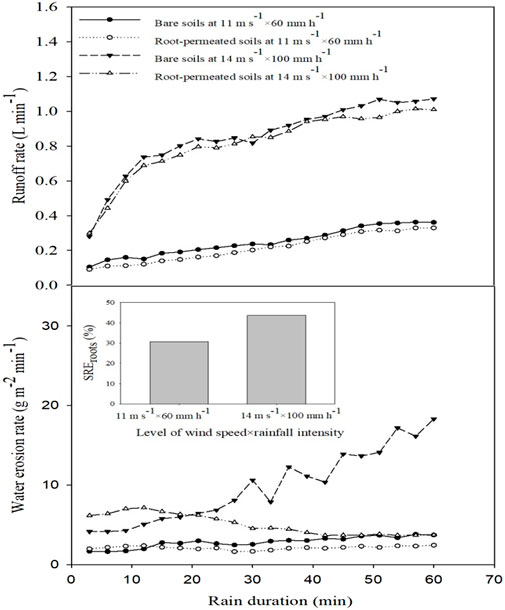
FIGURE 4. Average runoff rate and water erosion rate with rain duration and sediment reduction effect by roots (%).
Runoff was generally accompanied by sediment transportation. At a wind speed of 11 m s−1 combined with the rain intensity of 60 mm h−1, bare and root-permeated soils had similar trends that the water erosion rates enlarged slightly and steadily with rainfall duration. However, large variations were observed under the level of 14 m s−1 × 100 mm h−1. Specifically, for the bare soils, the water erosion rate increased noticeably in the first 9 –30 min which was attributed to the abundant detached soil particles and large numbers of linear fringes or rills caused by the former wind erosion (Tuo et al., 2016). After that, the water erosion rate tended to fluctuate at the mid and end of rainfall. This observation was roughly consistent with that found by Zhang et al. (2016) and Zhao et al. (2017) who reported that the sediment yield often fluctuated due to the irregular connections of rills and collapse of side walls, especially on a bare slope that was reshaped by wind erosion. Different from the bare soils, the water erosion rate in root-permeated soils increased steadily in the early stages of rainfall (the first 12 min), which was associated with the erodible soil particles on the surface flowed down the slope. As the rainfall continued, although the runoff rate became greater, the erosion rate gradually decreased and thereafter remained almost constant. In addition, it was also observed in our study that the erosion rate in root-permeated soils was higher than that in bare soils in the early stages of rainfall (the first 15 min), especially under the level of 14 m s−1 × 100 mm h−1. This phenomenon was probably because bare and root-permeated soils might have different water-erodible particles for flow transport after the former wind erosion. Compared with the root-permeated soils, the bare soils suffered much serious wind erosion, particularly at a wind speed of 14 m s−1 (Figure 3), which may provide less detached soil particles for flow transport in the early stages of rainfall (Tuo et al., 2012). As a consequence, a lower erosion rate in bare soils was observed when runoff was not strong enough. Our results showed that the existence of roots not only changed the water erosion process but also reduced the sediment yield (a reduction of 30.70% and 43.72% at rainfall intensities of 60 and 100 mm h−1, respectively).
In the whole wind tunnel–rainfall experiments, the erosion-reducing potential of S. psammophila roots showed 24.37% and 39.72% at the levels of 11 m s−1 × 60 mm h−1 and 14 m s−1 × 100 mm h−1, respectively. This result was mainly contributed to the protection and improvement of soils by roots in the wind and water erosion process. The roots are like a net wedging in soils mechanically, thus carpeting the ground. Because of its physical effects, roots could bind, enlace and enmesh soil particles, and then substantially reinforce the soil surface layer (Wang and Zhang, 2017; Shaurav et al., 2021). Furthermore, due to its biochemical effects, the produced exudates from roots adhered to soil particles and thus increased cementation between adjacent soil particles (Li et al., 2017). All of these direct or indirect actions of roots increased the soil wind or water erosion resistance (Guo et al., 2020). The present study investigated only one type of root and two levels of interaction between wind speed and rainfall intensity under a one-way wind erosion–rain erosion sequence; however, the effects of other types of roots (taproot system, fibrous root system, or even adventitious root system) (De Baets et al., 2007), multiple levels of interaction between wind speed and rainfall intensity, a sequence of alternating wind and water erosion (i.e., first wind erosion–first water erosion–second wind erosion–second water erosion) (Yang et al., 2019), and other conditions still remain unknown. These problems hinder a comprehensive understanding of the mechanism of how plant roots influence the erosion process and impede the estimation accuracy of the erosion-reducing potential of plant roots in arid and semi-arid regions. Therefore, more insights should be gained about the knowledge of erosion-reducing potential of roots under the complex erosion by wind and water in the future study.
Conclusion
The roots of S. psammophila clearly mitigated soil erosion in the water–wind crisscrossed erosion region of the Chinese Loess Plateau. Based on the simulated experiments of the wind tunnel first and rainfall thereafter, the present study indicated that S. psammophila roots weakened the coarsening of surface soil and decreased the sediment transport flux at different heights in the former wind erosion and reduced runoff and water erosion rates in the following water erosion process. Roots also changed the water erosion process and showed a trend that the water erosion rate increased steadily in the early stage of rainfall and then it gradually decreased and thereafter remained almost constant at the mid and end of rainfall. The erosion-reducing potential of S. psammophila roots could reach up to 24.37% and 39.72% at the levels of 11 m s−1 × 60 mm h−1 and 14 m s−1 × 100 mm h−1, respectively. These results are useful in revealing the mechanism of impacts of sandy vegetation construction on the water–wind crisscrossed erosion region of the Chinese Loess Plateau and also sandy land (Zhou and Shangguan, 2007).
Data availability statement
The raw data supporting the conclusion of this article will be made available by the authors, without undue reservation.
Author contributions
ZZ and WY designed and wrote this article, QL and HS conducted the experiment, and ZF and JZ analyzed the data.
Funding
This research was jointly supported by the National Natural Science Foundation of China (Grant Nos 41907059, 42067018, and 42267071), the Doctoral Research Foundation of Yulin University (18GK07 and 20GK18), and the Scientific and Technological Projects of Yulin City (CXY-2022-87).
Acknowledgments
The authors are also grateful to the reviewers and editors for their constructive comments and suggestions.
Conflict of interest
The authors declare that the research was conducted in the absence of any commercial or financial relationships that could be construed as a potential conflict of interest.
Publisher’s note
All claims expressed in this article are solely those of the authors and do not necessarily represent those of their affiliated organizations, or those of the publisher, the editors, and the reviewers. Any product that may be evaluated in this article, or claim that may be made by its manufacturer, is not guaranteed or endorsed by the publisher.
References
Cao, X. Y. (2013). A wind-tunnel study of roots against wind erosion. Shaanxi, China: Shaanxi Normal University.
Chen, G. P., and Zhao, W. Z. (2015). Age structure and dynamics of Salix psammophyla branches in southern edge of the Mu Us Sandy land. Journal of Desert Research. 35, 1521–1526. doi:10.7522/j.issn.1000-694X.2015.00001
De Baets, S., Poesen, J., Knape, A., and Galindo, P. (2007). Impact of root architecture on the erosion-reducing potential of roots during concentrated flow. Earth Surface Processes and Landforms. 32, 13231345. doi:10.1002/esp.1470
Dong, Z. B., Sun, H. Y., and Zhao, A. G. (2004). WITSEG sampler: A segmented sand sampler for wind tunnel test. Geomorphology 59, 119–129. doi:10.1016/j.geomorph.2003.09.010
Dong, Z. B., Liu, X. P., Wang, H. T., Zhao, A. G., and Wang, X. M. (2003). The flux profile of a blowing sand cloud: A wind tunnel investigation. Geomorphology 49, 219–230. doi:10.1016/S0169-555X(02)00170-8
Dong, Z. B., and Qian, G. Q. (2007). Characterizing the height profile of the flux of wind-eroded sediment. Environmental Geology. 51, 835–845. doi:10.1007/s00254-006-0363-5
Dong, Z. B. (2005). Research achievements in Aeolian physics in China for the last five decades. Journal of Desert Research. 25, 293–305. 1000-694X(2005)03-0293-13
Ghidey, F., and Alberts, E. E. (1997). Plant root effects on soil erodibility, splash detachment, soil strength, and aggregate stability. American Society of Agricultural and Biological Engineers ASAE) 40, 129–135. doi:10.13031/2013.21257
Govers, G. (1992). Relationship between discharge, velocity and flow area for rills eroding loose, non-layered materials. Earth Surface Processes Landforms (Earth Earth Surface Processes Land) 17, 515–528. doi:10.1002/esp.3290170510
Guo, P., Xia, Z., Gao, F., Islam, S., Xu, W., Li, M., et al. (2020). Evaluating the contribution of the physical and biochemical effects of root on detachment for the coarse-textured soil from the three Gorges Reservoir, China. Eurasian Soil Science. 53, 1260–1269. doi:10.1134/S1064229320090069
Gyssels, G., Poesen, J., Bochet, E., and Li, Y. (2005). Impact of plant roots on the resistance of soils to erosion by water: A review. Progress in Physical Geography. 29, 189–217. doi:10.1191/0309133305pp443ra
Hallet, D., Feeney, D. S., Bengough, A. G., Rillig, M. C., Scrimgeour, C. M., and Young, I. M. (2009). Disentangling the impact of AM fungi versus roots on soil structure and water transport. Plant and Soil. 314, 183196. doi:10.1007/s11104-008-9717-y
Hao, H. X., Di, H. Y., Jiao, X., Guo, Z. L., and Shi, Z. L. (2020). Fine roots benefit soil physical properties key to mitigate soil detachment capacity following the restoration of eroded land. Plant Soil 446, 487–501. doi:10.1007/s11104-019-04353-x
Hao, H. X., Qin, J. H., Sun, Z. X., Guo, Z, L., and Wang, J. G. (2021). Erosion-reducing effects of plant roots during concentrated flow under contrasting textured soils. Catena 203, 105378. doi:10.1016/j.catena.2021.105378
Huang, C. A., and Yang, Z. D. (2003). Sediment transport and flow intensity indexes. Journal of. Journal of Hydraulic Engineering. 6, 1–7. doi:10.13243/j.cnki.Slxb.2003.06.001
Leonard, J., and Richard, G. (2004). Estimation of runoff critical shear stress for soil erosion from soil shear strength. Catena 57, 233–249. doi:10.1016/j.catena.2003.11.007
Li, C. (2016). Effect of roots content on wind erosion under simulated wind-tunnel experiments. Shaanxi, China: Shaanxi Normal University.
Li, Q., Kang, F. R., Zhang, Z., Ma, C. Y., and Nan, W. G. (2021). Simulated experiment on wind erosion resistance of Salix residual in the agro-pastoral ecotone. Frontiers in Environmental Science. 9, 574883. doi:10.3389/fenvs.2021.574883
Li, Q., Liu, G. B., Zhang, Z., Tuo, D. F., Bai, R. R., and Qiao, F. F. (2017). Relative contribution of root physical enlacing and biochemistrical exudates to soil erosion resistance in the Loess soil. Catena 153, 61–65. doi:10.1016/j.catena.2017.01.037
Li, Z. B., Qin, B. S., Kang, W., Li, P., Li, W., and Wei, W. (2008). Indoor experimental studies on hydrodynamic characteristics of runoff in rill erosion procession steep slope. Transactions of the Chinese Society of Agricultural Engineering CSAE) 6, 64–68. doi:10.3901/JME.2008.09.177
Liu, W. J., Luo, Q. P., Lu, H. J., Wu, J. N., and Duan, W. P. (2017). The effect of litter layer on controlling surface runoff and erosion in rubber plantations on tropical mountain slopes, SW China. Catena 149, 167–175. doi:10.1016/j.catena.2016.09.013
Lu, R., Liu, Y. F., Jia, C., Huang, Z., Liu, Y., He, H. H., et al. (2019). Effects of mosaic-pattern shrub patches on runoff and sediment yield in a wind-water erosion crisscross region. Catena 174, 199–205. doi:10.1016/j.catena.2018.11.022
Mamo, M., and Bubenzer, G. D. (2001). Detachment rate, soil erodibility and soil strength as influenced by plant roots: Part II. Field study. Tran21sactions of the American Society of Agricultural Engineers ASAE) 44, 1167–1174. doi:10.13031/2013.6445
Mu, H. L., Yu, X. J., Fu, S. H., Yu, B. F., Liu, Y. N., and Zhang, G. H. (2019). Effect of stem basal cover on the sediment transport capacity of overland flows. Geoderma 337, 384–393. doi:10.1016/j.geoderma.2018.09.055
Pan, C., and Shangguan, Z. (2006). Runoff hydraulic characteristics and sediment generation in sloped grassplots under simulated rainfall conditions. Journal of Hydrology 331, 178–185. doi:10.1016/j.jhydrol.2006.05.011
Shaurav, A., Tanvir, M., Eric Joseph, W., Cody, R., David, H., William, B. P., et al. (2021). In-situ assessment of soil-root bonding strength to aid in preventing soil erosion. Soil and Tillage Research. 213, 105140. doi:10.1016/j.still.2021.105140
Song, Y., Yan, P., and Liu, L. Y. (2006). A review of the research on complex erosion by wind and water. Journal of Geographical Sciences. 16, 231–241. doi:10.1007/s11442-006-0212-1
Thompson, A., Davis, J. D., and Oliphant, A. J. (2015). Surface runoff and soil erosion under Eucalyptus and oak canopy. Earth Surface Processes and Landforms 41, 1018–1026. doi:10.1002/esp.3881
Tuo, D. F., Xu, M. X., and Gao, G. Y. (2018). Relative contributions of wind and water erosion to total soil loss and its effect on soil properties in sloping croplands of the Chinese Loess Plateau. Science of the Total Environment. 633, 1032–1040. doi:10.1016/j.scitotenv.2018.03.237
Tuo, D. F., Xu, M. X., Gao, L. Q., Zhang, S., and Liu, S. H. (2016). Changed surface roughness by wind erosion accelerates water erosion. Journal of Soils and Sediments 16, 105–114. doi:10.1007/s11368-015-1171-x
Tuo, D. F., Xu, M. X., Zheng, S. Q., Li, Q., and Pei, H. M. (2012). Effects of wind and water double erosion on characteristics of runoff and sediment from slope lands. Transactions of the Chinese Society of Agricultural Engineering. 28, 142–148. doi:10.3969/j.issn.1002-6819.2012.18.021
Vannoppen, W., Vanmaercke, M., De Baets, S., and Poesen, J. (2015). A review of the mechanical effects of plant roots on concentrated flow erosion rates. Earth-Science Reviews. 150, 666–678. doi:10.1016/j.earscirev.2015.08.011
Wang, B., and Zhang, G. H. (2017). Quantifying the binding and bonding effects of plant roots on soil detachment by overland flow in 10 typical grasslands on the Loess Plateau. Soil Science Society of America Journal. 81, 1567–1576. doi:10.2136/sssaj2017.07.0249
Wang, J. H., Li, Z. B., Yao, W. Y., and Dong, G. T. (2017). Influence of vegetation on runoff and sediment in wind-water erosion crisscross region in the upper Yellow River of China. Chinese Geographical Science. 27, 569–576. doi:10.1007/s11769-016-0829-7
Wang, L., Shi, Z. H., Wu, G. L., and Fang, N. F. (2014). Freeze/thaw and soil moisture effects on wind erosion. Geomorphology 207, 141–148. doi:10.1016/j.geomorph.2013.10.032
Wang, S. A., Fu, B. J., Piao, S. L., Lü, Y. H., Ciais, P., Feng, X. M., et al. (2016). Reduced sediment transport in the Yellow River due to anthropogenic changes. Nature Geoscience. 9, 38–41. doi:10.1038/ngeo2602
Yang, H. M., Zou, X. Y., Wang, J. A., and Shi, P. J. (2019). An experimental study on the influences of water erosion on wind erosion in arid and semi-arid regions. Journal of Arid Land 11, 208–216. doi:10.1007/s40333-019-0097-3
Zhang, C. L., Zou, X. Y., Yang, P., Dong, Y. X., Li, S., Wei, X. H., et al. (2007). Wind tunnel test and 137Cs tracing study on wind erosion of several soils in Tibet. Soil and Tillage Research. 94, 269–282. doi:10.1016/j.still.2006.08.002
Zhang, G. H., Liu, G. B., and Wang, G. L. (2012a). Effects of canopy and roots of patchy distributed Artemisia capillaris on runoff, sediment, and the spatial variability of soil erosion at the plot scale. Soil Science. 177, 409–415. doi:10.1097/SS.0b013e3182539713
Zhang, G. H., Liu, G. B., Wang, G. L., and Wang, Y. X. (2012b). Effects of patternedArtemisia capillarison overland flow velocity under simulated rainfall. Hydrological Processes. 26, 3779–3787. doi:10.1002/hyp.9338
Zhang, Q. Y., Fan, J., and Zhang, X. P. (2016). Effects of simulated wind followed by rain on runoff and sediment yield from a sandy loessial soil with rills. Journal of Soils and Sediments 16, 2306–2315. doi:10.1007/s11368-016-1470-x
Zhang, Z. (2018). Effect of Salix psammophyla roots on soil anti-erosion and its mechanisms in the water-wind erosion crisscross region on the Loess Plateau. Shaanxi, China: Northwest A&F University.
Zhao, C. H., Gao, J. E., Huang, Y. F., Wang, G. Q., and Xu, Z. (2017). The contribution of Astragalus adsurgens roots and canopy to water erosion control in the water-wind crisscrossed erosion region of the loess plateau, China. Land Degradation & Development. 28, 265–273. doi:10.1002/ldr.2508
Zhao, C. H., Gao, J. E., Huang, Y. F., Wang, G. Q., and Zhang, M. J. (2015). Effects of vegetation stems on hydraulics of overland flow under varying water discharges. Land Degradation & Development. 27, 748–757. doi:10.1002/ldr.2423
Zhao, H. L., Yi, X. Y., Zhou, R. L., Zhao, X. Y., Zhang, T. H., and Drake, S. (2006). Wind erosion and sand accumulation effects on soil properties in Horqin Sandy Farmland, Inner Mongolia. Catena 65, 71–79. doi:10.1016/j.catena.2005.10.001
Keywords: Salix roots, wind tunnel, rainfall simulation, sediment transport flux, water erosion rate, sediment reduction effect
Citation: Zhang Z, Yu W, Li Q, Sun H, Feng Z and Zou J (2023) Erosion-reducing potential of Salix psammophila roots in the water–wind crisscrossed erosion region of the Chinese Loess Plateau: A simulated investigation. Front. Environ. Sci. 10:1109174. doi: 10.3389/fenvs.2022.1109174
Received: 27 November 2022; Accepted: 21 December 2022;
Published: 06 January 2023.
Edited by:
Zheng Gang Guo, Lanzhou University, ChinaReviewed by:
Jianhua Xiao, Northwest Institute of Eco-Environment and Resources (CAS), ChinaBaojun Zhang, Institute of Mountain Hazards and Environment (CAS), China
Copyright © 2023 Zhang, Yu, Li, Sun, Feng and Zou. This is an open-access article distributed under the terms of the Creative Commons Attribution License (CC BY). The use, distribution or reproduction in other forums is permitted, provided the original author(s) and the copyright owner(s) are credited and that the original publication in this journal is cited, in accordance with accepted academic practice. No use, distribution or reproduction is permitted which does not comply with these terms.
*Correspondence: Weijie Yu, eXV3ZWlqQG53c3VhZi5lZHUuY24=