- 1College of Resources and Environmental Sciences, China Agricultural University, Beijing, China
- 2Huaneng Renewables Corporation Limited, Beijing, China
- 3College of Resources and Environment, Southwest University, Chongqing, China
- 4Department of Plant Nutrition, College of Resources and Environmental Sciences, Huazhong Agricultural University, Wuhan, China
- 5College of Resources and Environmental Sciences, Nanjing Agricultural University, Nanjing, China
- 6The Sustainable Soils and Grassland Systems Department, Rothamsted Research, Harpenden, United Kingdom
The Yangtze River Basin (YRB) crosses three economic zones and major agricultural regions of eastern, central, and western China. Increasing non-point source pollution, caused by excessive nitrogen (N) inputs to farms, is one of the main causes of water contamination in the YRB. To improve N fertilizer use efficiency, we conducted a field experiment using 15N-labeled urea at three sites located in upstream, midstream, and downstream regions of the YRB to evaluate the impacts of improved fertilizer management on crop yield, fertilizer N recovery, and losses in three crop rotations: rice–wheat (RW), rice–vegetable (RV) [tuber mustard at Jiangjin (Chongqing), cabbage at Shayang (Hubei), and savoy cabbage at Rugao (Jiangsu)] and maize–wheat (MW). Applying only 50% of the traditional application of N and P fertilizer maintained the wheat yield at Jiangjin and Shayang and savoy cabbage yield at Rugao. However, it caused a 27% reduction of the wheat yield at Rugao. The 15N recovery showed that 27% of the fertilizer N was retained in the soil and that 25% less fertilizer N was lost to the environment compared to the traditional fertilizer application. Improved fertilizer management would reduce the environmental cost of farming in the YRB, but with some consequences to winter crop yields.
Introduction
The Yangtze River Basin (YRB) accounts for about one-fifth of the total land area of China, but its output of wheat (Triticum aestivum L.) and vegetables comprised 62% and 71%, respectively, of China’s production in 2020 (National Bureau of Statistics of China, 2021; Ministry of Agriculture of the People’s Republic of China, 2021). These high yields are due to the suitable weather, the fertile soil, and the typically-used double cropping systems such as rice–wheat, rice–vegetables, and maize–wheat (Timsina and Connor, 2001; Liu et al., 2005). From 2011 to 2020, the annual wheat grain production in China increased by 13%, from 118.57 to 134.25 million tons; the average wheat grain yield per unit area increased by 18%, from 4.89 to 5.74 t ha−1; the annual vegetable production increased by 25%, from 597.67 to 749.12 million tons; and the average vegetable yield per unit area increased by 15%, from 30.43 to 34.87 t ha−1 (Ministry of Agriculture of the People’s Republic of China, 2021). Nitrogen (N) fertilizer has played a vital role in these increases. However, the over-application of N fertilizer, while maintaining crop productivity, has greatly damaged the environment, causing eutrophication (Tilman et al., 2001; Fowler et al., 2013), air pollution from ammonia (NH3) volatilization (Gu et al., 2014; Liu M. et al., 2017), global warming from emissions of nitrous oxide (N2O) (Liu et al., 2004; Gao et al., 2014), and enhanced N deposition from the atmosphere (Liu et al., 2013) causing soil acidification (Matsuyama et al., 2005; Guo et al., 2010). The “Action plan for zero-growth fertilizer use by 2020”, issued by the Ministry of Agriculture of the People’s Republic of China in 2015, is aimed at improving fertilizer use efficiency by reducing unnecessarily large N inputs but still ensuring the effective supply of major agricultural products such as grain and so promoting sustainable agricultural development (Liu et al., 2016).
Food supplies in the south of China are dominated by rice and those in the north are dominated by wheat, of which 23% of the total is produced in the middle and lower YRB and in southwestern China (Wang et al., 2009). Jiangsu’s agriculture is based on grain production with rice and wheat as the main crop, which comprises 15% of the wheat produced in the YRB. Hubei is also a major agricultural region based on grain production, with rice as the main crop. In Chongqing and Sichuan, N applications to winter wheat were 150–718 kg ha−1 (Meng et al., 2013; Duan et al., 2014; Bouraima et al., 2016; Zhou et al., 2016) with applications as high as 240 kg N ha−1 in Hubei province (Hu et al., 2015; Yi et al., 2015). In Jiangsu, 90–375 kg N ha−1 were applied to wheat (Jiang et al., 2006; Ma et al., 2010; Zhao et al., 2016) and, generally, 234–870 kg N ha−1 were applied to vegetables (including open field and greenhouse) because they are such an important part of the diet (Liang et al., 2013; Gai et al., 2016; Min and Shi, 2018).
According to the investigations and surveys in these regions, the traditional N fertilizer input (famers’ practice) is 300 kg N ha−1. However, 150–180 kg N ha−1 is the recommended N application rate for cereal crops (including wheat) to obtain the maximum profit (Zhu and Chen, 2002). An integrated study by Ju et al. (2009) supported the view that a reduction of 30%–50% of the fertilizer N application was possible without yield loss of wheat in the North China Plain and Taihu Lake Region of China. Min et al. (2012) reported that a 40% reduction of fertilizer N inputs can reduce N leaching and maintain the yield of intensive greenhouse vegetables. Goh and Vityakon (1983) found that an application of only 300 kg N ha−1 can achieve high yields of spinach. We therefore conducted experiments to test whether a reduction in the N application rate from 300 and 600 kg N ha−1 to 150 and 300 kg N ha−1 would maintain wheat grain yields and vegetable yields in typical local crop rotations and reduce the environmental impact at three sites in the YRB.
Food security is the prime consideration of the Chinese Government because of the increasing population, currently more than 1.4 billion, but, as noted above, pollution is an increasing concern. The Ministry of Agriculture and Rural Affairs of the People’s Republic of China reported that the average fertilizer apparent recovery of rice, wheat, and maize was only 35.2% in 2015. Therefore, research is needed to increase N use efficiency while at least maintaining yields. This paper reports research on the winter production of typical multiple cropping rotations of rice–wheat, rice–vegetables, and maize–wheat. 15N-labeling experiments at three sites in upstream, midstream, and downstream regions of the YRB region continued the same fertilizer treatments and management practices as in the summer season (Wang et al., 2019). Crops planted in winter (wheat and vegetables) have special characteristics (e.g., dry-farming in a rotation; higher fertilizer applications in the vegetable season; cold climatic conditions affecting N dynamics in soils and utilization by crops). The fate of N and its effects on the yield of summer crops have been reported previously (Wang et al., 2019). However, the fate of 15N-labeled fertilizer in winter season crops rotated with summer crops (e.g., rice and maize) has not been addressed to date. This paper reports the results for the winter season crops with the following objectives: 1) to research if crop yields can be maintained or even increased with reduced and more efficient fertilizer inputs; 2) to quantify fertilizer N recovery by the crops and soil and measure N utilization efficiency in the wheat and vegetable growing seasons.
Materials and Methods
Experimental Site
The field experiments were conducted at three sites in the YRB in 2016–2017: the agronomic experimental station in Jiangjin County, Chongqing, China (106°11′E and 29°03′N) (upstream at 285 m altitude), the agronomic experimental station in Shayang County, Jingmen, Hubei, China (112°18′E and 30°43′N) (midstream at 87.3 m altitude), and the agricultural science research institute of Rugao County, Nantong, Jiangsu, China (120°29′E and 32°22′N) (downstream at 4 m altitude). Jiangjin, Shayang, and Rugao are typical agricultural counties in upstream, midstream, and downstream YRB. The Jiangjin site is a long-term experiment owned by Southwest University; Shayang belongs to Huazhong Agricultural University, while Rugao is the experimental site of Nanjing Agricultural University. The properties of the 0–40 cm soil layers at the three sites before transplanting are shown in Table 1. The so-named “Purple soil” is distributed widely in upstream YRB (Mo et al., 2005). The hydragric anthrosol at Shayang is a special type of anthropogenically formed soil developed by long-term flooding, puddling, and rice planting according to the World Reference Base for Soil Resources (WRB). Fluvisol is fluviatile deposits with evident stratification and is widely distributed in downstream YRB. The greatly improved multiple cropping index (Xu et al., 2019) has shortened the alternating time of dry and wet cycles, which induces secondary gley formation and develops into the soil type corresponding to a Gleyic fluvisol in WRB.
Field Experiment Design
The field experiment compared three rotations during the wheat/vegetable production season: rice–wheat (RW), rice–vegetables (RV) (the vegetables were tuber mustard at Jiangjin, cabbage at Shayang, and savoy cabbage at Rugao), and maize–wheat (MW). Each rotation comprised three treatments: no N fertilizer as the control (C), reduced N fertilizer (R), and traditional N fertilizer (T). Fertilizers for wheat and vegetables were applied as shown in Table 2, based on previous studies in Chongqing (Ju et al., 2009; Su et al., 2017; Hao et al., 2019). Plot sizes were 8 m × 5 m at Jiangjin, 5.5 m × 4.5 m at Shayang, and 10 m × 4 m at Rugao, arranged in randomized blocks in four adjacent fields at the three sites.
Microplots (0.5 m × 0.5 m) fenced with a stainless steel frame (0.3 m high, and driven 0.2 m deep into the soil to avoid surface runoff and lateral contamination) were installed at the side of each traditional fertilizer treatment and reduced fertilizer treatment plot, 1 m from the edge of the plots. 15N-labeled urea (abundance 5.14%; Shanghai Chem-Industry Institute) was applied to the microplots at the same N rate as the main plots and at the same time. Applications of P and K fertilizer and management practices on the microplots were the same as on the corresponding main plots. Each treatment had three replicates. Crop varieties were Chuanmai 45, Zhengmai 9023, and Yangmai 16 wheat at Jiangjin, Shayang, and Rugao, respectively.
Plant and Soil Sampling and Analysis
Agricultural management practices for the wheat at the three sites, tuber mustard (Brassica juncea var. tumida L.) at Jiangjin, cabbage (Brassica rapa pekinensis L.) at Shayang, and savoy cabbage (Brassica oleracea L. var. capitata L.) at Rugao, are shown in Table 3. The wheat and vegetables were planted at 1.74–2.18 × 106 plants ha−1 and 9 × 104 plants ha−1, respectively. The recent (2006–2015) weather conditions (e.g., average precipitation and temperature during the wheat and vegetable growing period) in the three regions are shown in Figure 1.
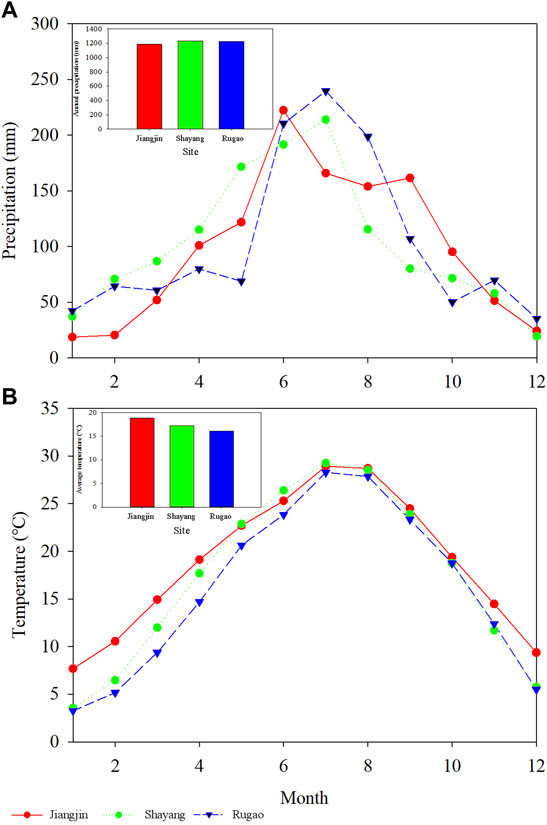
FIGURE 1. Average precipitation (A) and temperature (B) at the experimental sites from 2006 to 2015.
The yields of wheat grain and vegetables were determined by harvesting all of each plot. Plant samples from microplots were carefully collected by hand using a sickle and a small shovel and separated into grain, straw, and roots. Aboveground parts were harvested first and then whole roots were dug out and washed with distilled water. All samples were dried at 65°C in a forced air oven and ground to pass a 250-μm (65-mesh) screen. Samples from the microplots, including four hills of wheat and one hill of vegetables, were finely ground in a ball mill for 15N analysis.
Soil samples were collected from 0–40 cm depths from all plots and microplots after harvesting the wheat and vegetables using a 3-cm inner diameter tube auger and divided into two layers (0–20 cm, 20–40 cm). All soil samples were air-dried and ground to pass a 150-μm (100-mesh) screen. Soil samples from microplots were finely ground in a ball mill. Grain, straw, roots, and soil samples were analyzed for total N content by the Kjeldahl method and 15N abundance by mass spectrometry (Delta Plus XP, Thermo Finnigan, Pittsburg, PA). Tuber mustard has stems, leaves and roots. Usually, the stem is eaten but the leaves are discarded. All of the aboveground parts of cabbage and savoy cabbage can be eaten.
15N Analysis
The percentages of fertilizer N recovered in wheat/vegetable grain/edible part, straw and roots and in the soil from the microplots at harvest were calculated using Eqs. 1–4, where the 15N atom% excesses were corrected for background abundance [0.3663% (Cabrera and Kissel, 1989)].
Statistical Analysis
Statistical analyses were made using SPSS 23 (Statistical Product and Service Solutions Inc., Chicago, IL, United States). A two-way analysis of variance (ANOVA) was conducted to compare measured crop and soil data (biomass yield and fertilizer N recovery) by standard procedures on a randomized plot design for the rice/maize season within the following effects: crop rotation (RW, RV, and MW), fertilizer (C, R, and T), and crop rotation × fertilizer. Different small letters mean statistically significant differences at p < 0.05 between the different rotations at the same site according to the Tukey HSD test. Microsoft Office Excel 2013 was used to process the data. OriginPro 2017 was used to draw the figures.
Results
Crop Yields
Yields of wheat in the RW and MW rotations and of vegetables in the RV rotation are shown in Figure 2. The interactions of crop rotation and fertilizer rate had a significant effect on yield at Jiangjin and Rugao. At Jiangjin (Chongqing), the traditional fertilizer input resulted in a 90% increase (by 1.96 t ha−1) and 31% decrease (by 1.67 t ha−1) in the wheat yields in the RW and MW rotations compared with no fertilizer control, respectively. Reduced fertilizer caused a 116% and 3% increase in RW (2.23 t ha−1) and MW (2.47 t ha−1) wheat yields, respectively, compared to the zero N control. In the RV rotation, traditional fertilizer input and reduced fertilizer input produced 370% (13.99 t ha−1) and 214% (9.34 t ha−1) increases in tuber mustard yield relative to the control, respectively. However, although the traditional fertilizer input resulted in a bigger yield increase, it also increased the variability of yields.
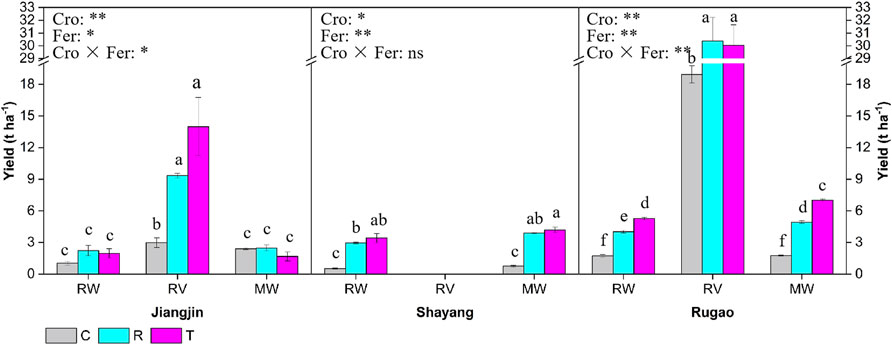
FIGURE 2. Wheat and vegetable yields at Jiangjin, Shayang, and Rugao. Note: RW = Rice–Wheat rotation. RV = Rice–Vegetable rotation. MW = Maize–Wheat rotation. Error bars present the standard error of the measurements from three replicates. C = no N fertilizer input. R = reduced fertilizer input. T = traditional fertilizer input. Different small letters above the bars denote significance at the 5% level. Cro = crop system. Fer = fertilization. Cro × Fer = the interactions of crop rotation and fertilization. ns, * and ** mean no significance, p < 0.05 and p < 0.01.
At Shayang (Hubei), the traditional fertilizer input increased (p < 0.05) wheat grain yield in the RW and MW rotations by 541% (3.42 t ha−1) and 459% (4.19 t ha−1), respectively, over the control. Reduced fertilizer input significantly increased yields over the control by 453% (2.95 t ha−1) for RW and 420% (3.90 t ha−1) for MW, but there were no significant differences between the traditional and reduced treatments. Because of an abnormal amount of precipitation at transplanting, which could not be handled by the drainage system in the concrete frame, flooding of the RV cabbage at Shayang resulted in no yield.
At Rugao (Jiangsu), the traditional fertilizer application produced 207% (by 5.28 t ha−1) and 300% (by 7.02 t ha−1) increases (p < 0.05) in RW and MW wheat yields, respectively, compared to no N control. Reduced fertilizer input resulted in 134% (by 4.02 t ha−1) and 182% (by 4.94 t ha−1) increases in grain yield for RW and MW, respectively, compared to the control. The traditional fertilizer input also increased the yield in the RW and MW rotations by 31% and 42%, respectively, over the R treatment. Savoy cabbage receiving the traditional fertilizer rate (yield = 30.04 t ha−1) and reduced fertilizer rate (yield = 30.38 t ha−1) in the RV rotation yielded 59% and 60% more, respectively, than the controls, but there was no significant difference between the T and R treatments.
The Recovery of 15N-Labeled Fertilizer in the Crops
The atom % 15N recovery in wheat (grain, straw and roots) in the RW and MW rotations and in the vegetables in the RV rotation (leaves, stem, and roots) are shown in Figure 3. There were significant interactions between the crop rotation and fertilizer in determining the 15N recovery in grain, straw, and roots at Jiangjin, with the average recovery in the grain, straw, and roots of wheat from the T treatment at Jiangjin being only 7%, 8%, and 1%, respectively; i.e., very low. Recoveries in the R treatment were 11%, 12%, and 1% in the grain, straw, and roots, respectively. 15N recoveries from the R treatment in the stem, leaves, and roots of tuber mustard were 25%, 11%, and 2%, respectively, and those from the T treatment were 16%, 5%, and 1%, respectively. Recoveries in the grain and stem from the R treatment at Jiangjin were significantly higher (by 34, 75 and 55%) than those from the T treatment in the RW, MW (grain), and RV (stem) rotations, respectively. The recoveries from the R treatment in the straw and leaves of wheat and cabbage at Rugao were higher (p < 0.05) than those from the T treatments in the RW (by 67%), MW (by 27%), and RV (by 136%). The 15N recoveries in the roots of crops receiving reduced fertilizer inputs were 43% and 76% higher than those receiving the traditional fertilizer input in the RW and RV rotations, respectively.
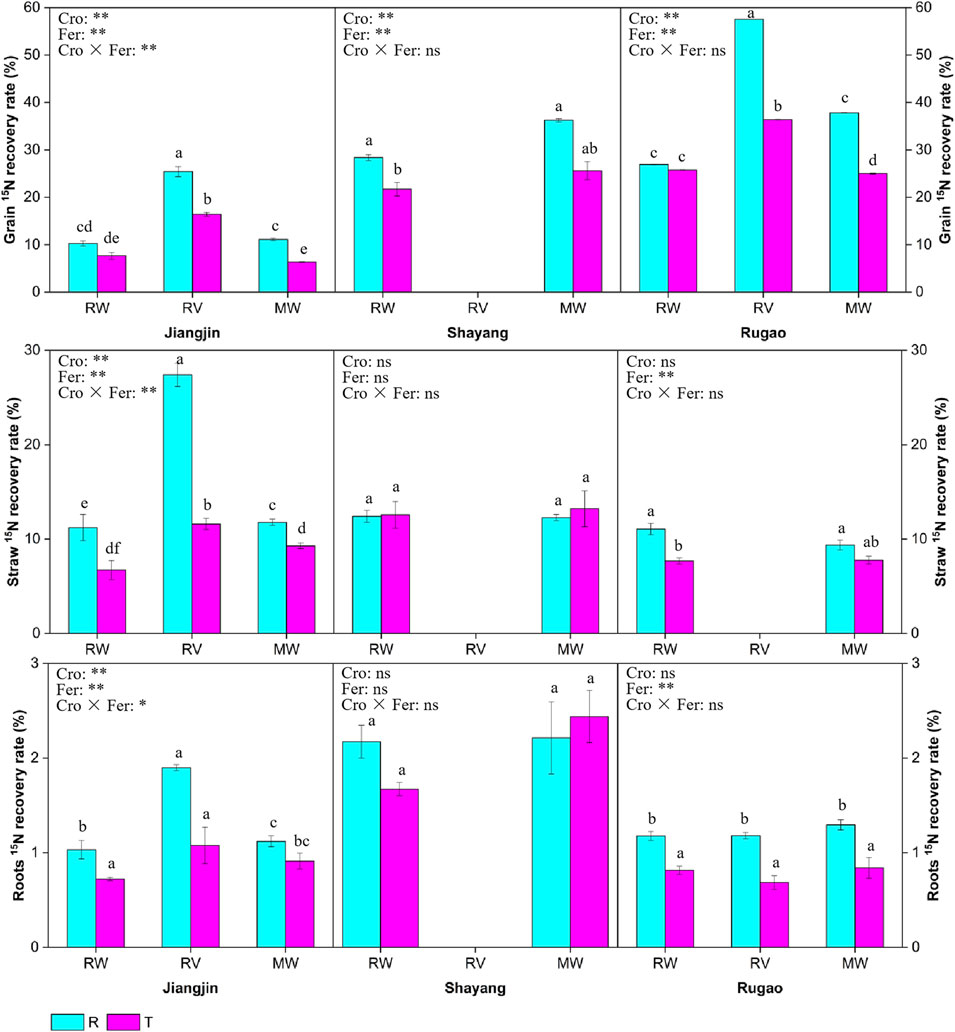
FIGURE 3. 15N recoveries in wheat/vegetable grain/stem/leaves, straw and roots in the three crop rotations at Jiangjin, Shayang and Rugao. Note: RW = rice–wheat rotation. RV = rice–vegetable rotation. MW = maize–wheat rotation. Error bars present the standard error of the measurements from three replicates. R = reduced fertilizer input. T = traditional fertilizer input. Different small letters above the bars denote significance at the 5% level. Cro = crop system. Fer = fertilization. Cro × Fer = the interactions of crop rotation and fertilization. ns, * and ** mean no significance, p < 0.05 and p < 0.01.
At Shayang, the average recoveries in the grain, straw, and roots of wheat receiving the traditional fertilizer input were 20%, 10%, and 2%, respectively, and the average recoveries in those receiving reduced fertilizer input were 25%, 112%, and 2%, respectively. The total 15N recoveries in the grain from the R treatment on the RW and MW rotations were significantly higher (by 31% and 19%, respectively) than those receiving traditional fertilizer input. There were no significant differences between the 2 N rates in the recoveries in the straw and roots.
At Rugao, the average recoveries in grain, straw, and roots of wheat receiving the traditional fertilizer rate were 25%, 8%, and 1%, respectively; in the R treatment, they were 32%, 10%, and 1%, respectively. There were no significant differences between the 2 N treatments in the recoveries in the grain in the RW rotation, but more 15N was recovered in the straw and roots in RW. Total 15N recoveries in the grain, straw, and roots of the R treatment were significantly higher (by 51%, 21%, and 54%, respectively) than those grown with the traditional fertilizer input in the MW rotation. The percentage 15N recoveries in leaves and roots of savoy cabbage were 58% and 1%. The percentage recovery from the T treatment was 36% and 1% and that from the R treatment 58% and 73%, i.e., higher (p < 0.05) compared to the T treatment.
Distribution of 15N-Labeled Fertilizer N in Soil
The recoveries of 15N fertilizer in the soil are shown in Figure 4. There were significant interactions of the crop rotation with fertilizer rate in the 0–20 and 0–40 cm soil layers at Jiangjin and Rugao. At Jiangjin, the percentage recoveries of 15N in the RW, MW, and RV rotations were 17%, 15%, and 36% in the 0–20 cm soil layer and 7%, 7%, and 9% in the 20–40 cm layer in the T treatment over the whole wheat/vegetable season. The percentages of the total soil recoveries in the shallow (0–20 cm) soil layer were 70%, 69%, and 80% in the RW, MW, and RV rotations, respectively. In the R treatment, the recoveries in the RW, MW, and RV rotations were 19%, 20%, and 19%, respectively, in the 0–20 cm soil and 10%, 7%, and 9%, respectively, in the 20–40 cm layer. The percentages of total recovery in the shallow soil layer were therefore 67%, 68%, and 73%, respectively, in the R treatment. The recovered 15N residues in the soil from the R treatments were 20% and 26% higher, respectively, in the RW and MW rotations but 38% lower in RV rotation compared with the T treatment.
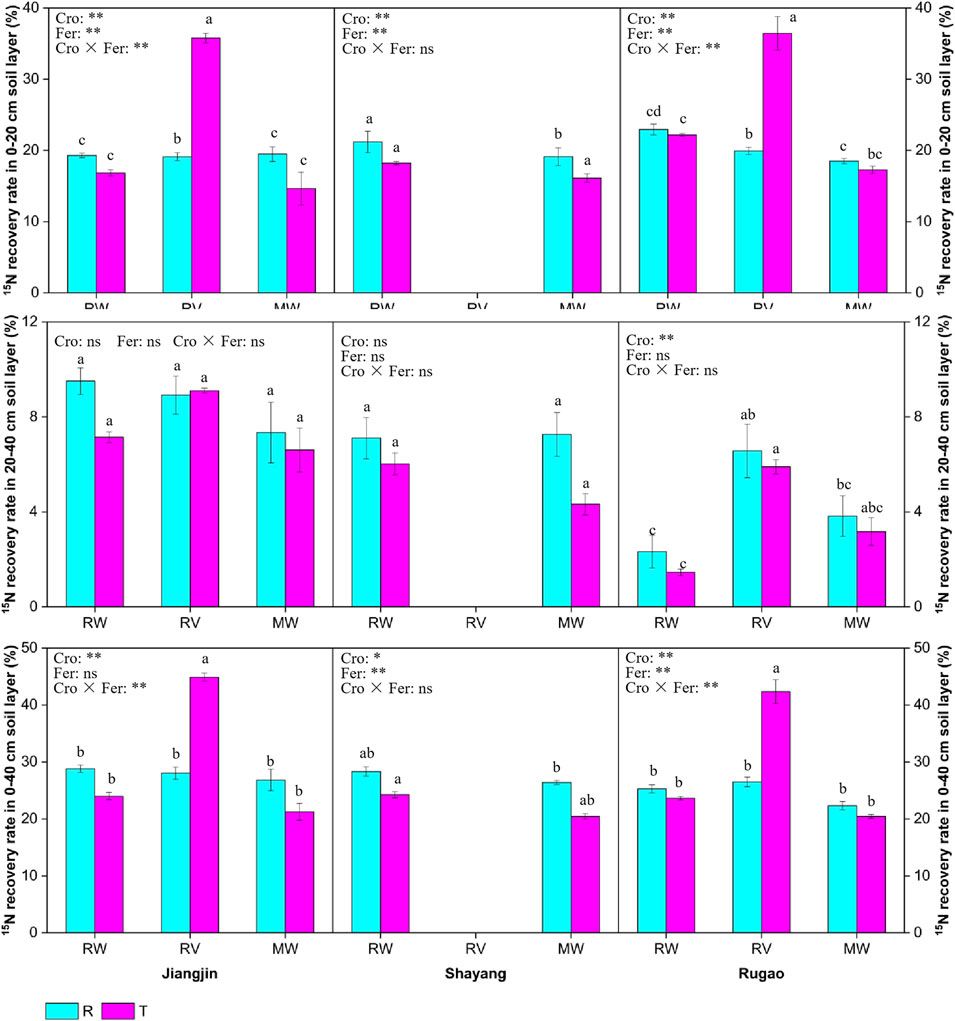
FIGURE 4. 15N recovery in the 0–40 cm soil layer during the wheat/vegetable season at Jiangjin, Shayang, and Rugao. Note: RW = rice–wheat rotation. RV = rice–vegetable rotation. MW = maize–wheat rotation. Error bars present the standard error of the measurements from three replicates. R = reduced fertilizer input. T = traditional fertilizer input. Different small letters above the bars denote significance at the 5% level. Cro = crop system. Fer = fertilization. Cro × Fer = the interactions of crop rotation and fertilization. ns, * and ** mean no significance, p < 0.05 and p < 0.01.
At Shayang, 18%, 21%, 16%, and 19% of the residual 15N was found in the 0–20 cm soil layer in the RWT (traditional fertilizer rate), RWR (reduced fertilizer rate), MWT, and MWR treatments, respectively. 15N recovery in the surface layer was increased significantly by reducing the fertilizer application. Recovery in the 20–40 cm soil layer in the RW and MW rotations was 6% and 4% for the T treatment and 7% and 7% for the R treatment. The percentages of the total recovery of 15N in the surface soil layer were 75% and 75% in the RW rotation receiving the traditional and reduced fertilizer rates, respectively, and 79% and 73% in the MW rotation receiving the traditional and reduced fertilizer input, respectively. Reducing the fertilizer rate increased 15N recovery in the soil by 17% and 29% in the RW and MW rotations, respectively, compared to the traditional fertilizer rates used by farmers.
At Rugao, the rate of N applied had no significant effect on the amount of 15N recovered in the soil in the wheat/vegetable season. Of the total 15N recovered, 94%, 91%, 85%, 83%, 86%, and 75% were in the surface 0–20 cm of soil in the RW (T), RW (R), MW (T), MW (R), RV (T), and RV (R) treatments, respectively.
Losses of 15N-Labeled Urea During the Wheat/Vegetable Season
The loss of 15N was calculated as the difference between the 15N applied and its measured uptake by wheat (grain, straw, and roots), tuber mustard (stem, leaves, and roots), savoy cabbage (leaves and roots), and the residue in the soil (Table 4). In our experiment, a significant decrease in total N loss (i.e., the N unaccounted for) was observed in improved fertilizer treatments relative to traditional fertilizer management of the three cropping systems in winter, with total N losses of winter-season crops (wheat and vegetables) reduced by 60%–67%, 58%–68%, and 64%–69% at Jiangjin, Shayang, and Rugao, respectively. Across all three sites in the YRB, improved (i.e., reduced) fertilizer rates decreased N losses to the environment by 63% and 66% from wheat and vegetable rotations. According to the two-way ANOVA (Table 5), N loss was mainly determined by crop rotation (p < 0.05) at the three sites, but fertilizer rate had a significant effect at Jiangjin.
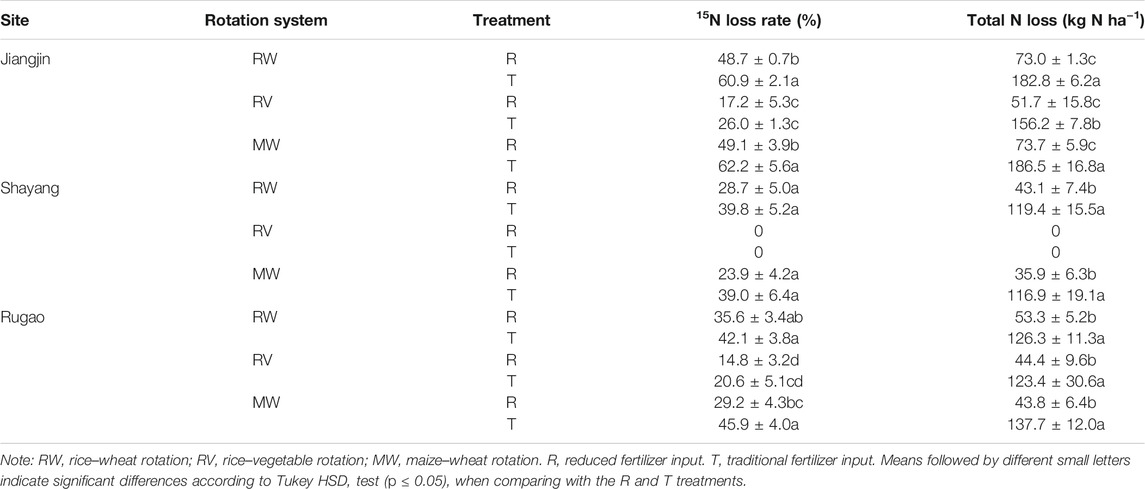
TABLE 4. Average N losses as percentages and amounts from the crop rotations in the YRB (mean ± SD).
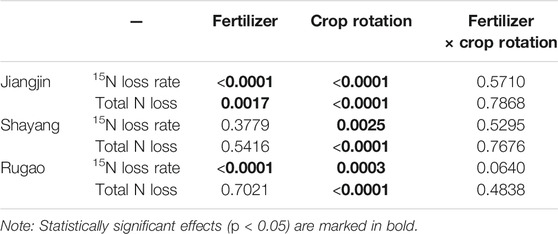
TABLE 5. ANOVA results (p values) for the individual effects of interactions of fertilizer (R and T) and crop rotation (RW, RV, and MW) on15N loss and total N loss.
Fate of 15N-Labeled Urea During the Wheat/Vegetable Season
The fate of 15N-labeled urea in wheat/vegetable season under the different rotations at the three sites is shown in Figure 5. At the conclusion of the experiment at Jiangjin, the average percentage of recovery of 15N in wheat was 16% and 24% with the traditional and reduced fertilizer inputs, respectively, i.e., very low. The average recovery from the soil was 23% and 28% with the traditional and reduced fertilizer input, respectively. Thus, fertilizer 15N loss to water and air (62%) under the traditional fertilizer input was reduced by 21% by reducing the fertilizer input (actual loss = 49%), and by 34% (from 26 to 17%) under the wheat rice–vegetable rotation.
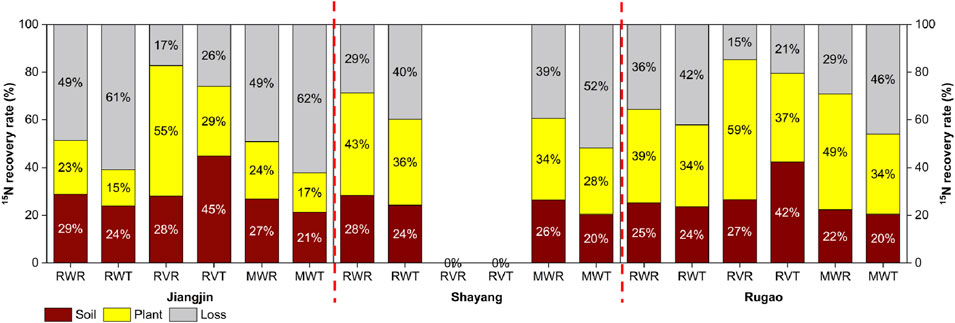
FIGURE 5. 15N recoveries in the crops at the end of the rotations, soil, and losses. Note: RW = rice–wheat rotation. RV = rice–vegetable rotation. MW = maize–wheat rotation. RWR = reduced fertilizer input in RW rotation, RWT = traditional fertilizer input in RW rotation, the same as RV and MW rotations in Jiangjin, Shayang, and Rugao.
At Shayang, the average N uptakes by the wheat were 32% (T) and 39% (R). The average 15N recoveries from the soil under wheat were 22% and 27% in the T treatment and R treatment, respectively, so the N loss to the environment was reduced by 26%.
At Rugao, the average fertilizer 15N recovered in the wheat was 34% with the traditional fertilizer input and 44% with the reduced fertilizer input, with average recoveries from the soil of 22% (T) and 24% (R). Thus, fertilizer N loss was reduced by 27% by reducing the fertilizer input. Savoy cabbage utilized 36% and 58% of the N applied and left 22% and 24% in the soil at the traditional and reduced fertilizer rates, respectively; a 28% reduction in loss to the environment.
Discussion
The average wheat yield at Jiangjin obtained by reducing the fertilizer application (2.35 ± 0.76 t ha−1) was higher but not significantly different from that using the traditional fertilizer input (1.81 ± 0.82 t ha−1). All treatments at Jiangjin yielded less than in previous experiments in the Sichuan Basin (2.74 ± 0.93 t ha−1) (Duan et al., 2014; Zhou et al., 2016) due to fusarium head blight (FHB). The disease mainly occurs in warm, humid climates (such as Jiangjin, which has no useful tools or sufficient experience for FHB control) and can lead to a loss of yield and quality (significant yield losses on more than 5 million ha per year in China are caused by FHB) (Goswami and Kistler, 2004; Cowger et al., 2016; Liu Y. Y. et al., 2017; Chen et al., 2019). In Shayang county, the grain yield was 3.80 ± 0.69 t ha−1 with the traditional fertilizer rate and 3.42 ± 0.53 t ha−1 with the reduced rate; higher than those reported in the literature (2.85 ± 0.81 t ha−1) (Hu et al., 2015; Yi et al., 2015). In contrast, the yield of wheat at Rugao was 6.15 ± 0.97 t ha−1 with the traditional fertilizer rate and 4.48 ± 0.54 t ha−1 with the reduced rate. The average of these (5.32 t ha−1) was comparable with that reported by Jiang et al. (2006) and Ma et al. (2010). However, this is less than that recommended for food security (7.12 t ha−1) by Chen et al. (2014). It might therefore be necessary to decrease the area of wheat planted in Jiangjin and Shayang and focus on other more productive crops (e.g., a green manure crop in Jiangjin and oilseed rape in Shayang), based on our results and predicted climate change. Statistics show that the cultivated area and yield of wheat in Chongqing has decreased: from 2011 to 2020, the cultivated wheat in Chongqing decreased by 80%, from 90,530 to 18,520 ha, and the annual wheat grain production decreased by 78%, from 0.27 to 0.06 million tons (Ministry of Agriculture of the People’s Republic of China, 2021). In the rice–vegetable rotation at Jiangjin, the traditional fertilizer input produced a lower output (13.99 ± 2.77 t ha−1) than that measured in a previous study (29.90 ± 6.56 t ha−1) (Bai et al., 2009), and yields were much more variable. Reducing the fertilizer input reduced the yield (9.34 ± 0.21 t ha−1) but not significantly and yields were more stable. The average yield of savoy cabbage in the RV rotation at Rugao (30.21 ± 2.70 t ha−1) was also less than that in earlier experiments (48.69 ± 17.45 t ha−1) (Franczuk et al., 2010; Gai et al., 2016; Maucieri et al., 2017) and there was no significant difference between the two fertilizer levels (30.04 ± 2.00 t ha−1 with traditional fertilizer and 30.38 ± 2.30 t ha−1 with reduced fertilizer).
Vegetables had even greater yield variability than wheat across the three sites, suggesting significant risks if wheat was replaced by vegetables in winter. Specifically, the Jiangjin site is not suitable for wheat while the Shayang site is not suitable for vegetables if there is no drainage system (no harvested yield) in winter. Rugao achieved the highest wheat and vegetable yields of all the sites, reflecting the influence of soil fertility (Rugao > Shayang > Jiangjin), climate (sunshine in winter: Rugao > Shayang > Jiangjin; precipitation in winter: Rugao < Shayang < Jiangjin), and good field management [Rugao (hired/professional farmers) > Shayang (half-professional farmers) > Jiangjin (smallholder farmers)].
Figures 3, 4 show clearly how the 15N recovered in the crops (wheat and vegetables) was influenced by fertilizer input. Reducing the fertilizer input resulted in higher fertilizer N uptake in the grain and smaller losses, except for the recoveries in the straw and roots of the MW rotation at Shayang. At the same time, N recovery was very low in wheat at Jiangjin due to the low yield caused by disease. Previous research had also demonstrated that reducing N inputs can increase N efficiency and decrease environmental impacts (Chen et al., 2014; Yi et al., 2015). Also, returning wheat straw to the soil can increase SOC stock (Hao et al., 2013; Guan et al., 2015), water capture and retention, microbial C and microbial N, the activities of cellulase and catalase (Li et al., 2018), and microbial and invertebrate activity (in particular earthworms) (van Gestel et al., 2003) and decrease soil pH (Walter et al., 1996). A wheat–straw mulch on upland crops can ameliorate salinization, prevent water and wind erosion of soil (Dong and Qian, 2002), and reduce N uptake by weeds (Liu et al., 2005). We found that 15N recovery in wheat/vegetable grain, straw, and roots in the YRB was increased by 41%, 48%, and 43% by reducing the fertilizer rate compared with the traditional fertilizer input used by farmers. We therefore recommend the reduction of the amount of fertilizer applied and returning the stubble to the field to improve soil quality and increase the recovery of fertilizer N.
The average fertilizer 15N recoveries in the 0–20 cm soil layer were 16%, 17%, and 20% at the traditional fertilizer rate and 19%, 20%, and 21% with reduced fertilizer at Jiangjin, Shayang, and Rugao, respectively. The 15N recovered in topsoil under the vegetable crops receiving traditional and reduced fertilizer applications was 36% and 19%, respectively, at Jiangjin and 36% and 20%, respectively, at Rugao. Overall, the percentage recoveries of residual 15N in the topsoil (0–20 cm) out of the total recoveries in the 0–40 cm layer of soil under crops receiving the traditional and reduced fertilizer rates were 73% and 69%, respectively, at Jiangjin, 75% and 73%, respectively, at Shayang, and 88% and 83%, respectively, at Rugao. This was caused by runoff and leaching resulting from the abnormal amounts of precipitation during the application of basal fertilizer and topdressing. The higher soil residues observed at Jiangjin were because rainfall leaches N deep into the soil where soil colloids adsorb NH4+ (Xu et al., 2021). Also, soil pH values at Shayang and Rugao were 7.52 and 7.44, respectively, which resulted in more ammonia volatilization than at Jiangjin (Harrison and Webb, 2001; Huang et al., 2017).
Yan et al. (2014) pointed out that N residues in soil following high N inputs can be taken up by subsequent crops and so should be considered as part of the available N. However, we found that fertilizer 15N recovery in the soil of a wheat crop was significantly increased by 23% and 23% by reducing the fertilizer rate at Jiangjin and Shayang; the increase was 8% at Rugao, which was not significant. Thus, reducing N applications is still advisable. In contrast, the very high demand for N by vegetable crops resulted in less N remaining in the soil under the R treatment compared to the traditional fertilizer rate.
Overall, reducing the application of synthetic N fertilizer to a wheat/vegetable rotation in the YRB will reduce N losses to the environment (including runoff, leaching, and gaseous loss) (Tian et al., 2007; Wang et al., 2007; Qiao et al., 2012; Xu et al., 2012; Xue et al., 2014; Zhong et al., 2016; Zhang et al., 2017). We observed the maximum fertilizer N loss from the traditional fertilizer application to the MW rotation and the minimum loss from the reduced fertilizer application to the RW rotation (MW at Rugao). The large 15N loss at Jiangjin resulted from the heavier rainfall over the growing period generating more N losses via surface runoff and leaching (Xie et al., 2019; Liu et al., 2020). Also, the higher temperature at Jiangjin triggers more NH3 volatilization (Xu et al., 2021). More fertilizer N remained in the vegetable plots than in the wheat plots after harvest of both crops in Jiangjin and Rugao. This can be explained by the much earlier harvest date of vegetables at Jiangjin and/or two additional topdressings at both sites (see Table 3), providing more opportunities for 15N labeled fertilizer to be retained in the soils growing vegetables.
Conclusion
Research in the winter growing season at three sites in the YRB supports our conclusion that reducing the application of N fertilizer is an effective way of balancing sustainable crop yields for a secure food supply and environmental benefit. A 50% reduction of the N applied to wheat and vegetables maintained the wheat yields at Jiangjin and Shayang and vegetable yields at Jiangjin and Rugao. However, the yields of wheat at Rugao were reduced. More (27%) fertilizer N was retained in the soil (0–40 cm) and recycled to the next rice/maize crop, and there was a 25% reduction of fertilizer N loss compared with traditional N use. This confirms the importance of reducing N inputs to crop rotations in the YRB. Moreover, the yield gap of three sites proved that the agricultural infrastructure (e.g., drainage system) and management (e.g., hired/professional farmers) have substantial effects on yield in the YRB. More research is needed to optimize crop rotations across the whole crop production season for better whole system productivity, N use efficiency, and less environmental impact. Such integrated analyses are vital if the YRB is to adapt to predicted global climate change and regional green development within the Yangtze Economic Zone. In the future, we aim to quantify all N inputs (including atmospheric deposition and manures as well as fertilizer) and outputs (including crop uptake, losses to surface and groundwater, and gaseous loss) and, synthesizing the relevant data (including yield and future food demands), to recommend optimal rotations for the different regions of the YRB.
Data Availability Statement
The original contributions presented in the study are included in the article/Supplementary Material, further inquiries can be directed to the corresponding author.
Author Contributions
SW, FSZ, and XJL contributed to the conception of the study; SW, LSY, SPL, and BS performed the experiment; SW, KG, and XJL contributed significantly to analysis and manuscript preparation; SW performed the data analyses and wrote the manuscript; SW, XJS, JWL, SWG, JBS, KG, and XJL helped perform the analysis with constructive discussions.
Funding
This study was supported by the State Key Basic Research Programme (2017YFD0200100), the National Natural Science Foundation of China (41425007), the Sino-UK CINAg program (BBSRC project BB/N013468/1), and the High-level Team Project of China Agricultural University.
Conflict of Interest
SW was employed by the company Huaneng Renewable Corporation Limited.
The remaining authors declare that the research was conducted in the absence of any commercial or financial relationships that could be construed as a potential conflict of interest.
Publisher’s Note
All claims expressed in this article are solely those of the authors and do not necessarily represent those of their affiliated organizations, or those of the publisher, the editors, and the reviewers. Any product that may be evaluated in this article, or claim that may be made by its manufacturer, is not guaranteed or endorsed by the publisher.
Acknowledgments
We would like to thank Southwest University, Huazhong Agricultural University, Nanjing Agricultural University, and Key Lab of Plant-Soil Interaction, MOE, Center for Resources, Environment and Food Security, College Resources and Environmental Sciences, China Agricultural University, Beijing, China for the support of the field and lab work. We are grateful to the help from Xin Ma, Minmin Su, Tianxiang Hao, Xiaoqing Cui, Wei Wang, and Chongjing Guo at China Agricultural University.
References
Bai, B. Y., Zhao, J. K., Wei, S. Q., and Shi, X. J. (2009). Response of Different Varieties of Tuber Mustard to Nitrogen Fertilizer. J. Anhui Agric. Sci. 37, 237–238. doi:10.13989/j.cnki.0517-6611.2009.01.149
Bouraima, A.-K., He, B., and Tian, T. (2016). Runoff, Nitrogen (N) and Phosphorus (P) Losses from Purple Slope Cropland Soil under Rating Fertilization in Three Gorges Region. Environ. Sci. Pollut. Res. 23, 4541–4550. doi:10.1007/s11356-015-5488-1
Cabrera, M. L., and Kissel, D. E. (1989). Review and Simplification of Calculations in15N Tracer Studies. Fertilizer Res. 20, 11–15. doi:10.1007/bf01055396
Chen, H., Wu, Q., Zhang, G., Wu, J., Zhu, F., Yang, H., et al. (2019). Carbendazim-resistance of Gibberella Zeae Associated with fusarium Head Blight and its Management in Jiangsu Province, China. Crop Prot. 124, 104866. doi:10.1016/j.cropro.2019.104866
Chen, X., Cui, Z., Fan, M., Vitousek, P., Zhao, M., Ma, W., et al. (2014). Producing More Grain with Lower Environmental Costs. Nature 514, 486–489. doi:10.1038/nature13609
Cowger, C., Weisz, R., Arellano, C., and Murphy, P. (2016). Profitability of Integrated Management of Fusarium Head Blight in North Carolina winter Wheat. Phytopathology 106 (8), 814–823. doi:10.1094/phyto-10-15-0263-r
Dong, Z.-y., and Qian, B. F. (2002). Field Investigation on Effects of Wheat-Straw/corn-Stalk Mulch on Ecological Environment of upland Crop farmland. Jzus 3, 209–215. doi:10.1631/jzus.2002.0209
Duan, Y.-h., Shi, X.-j., Li, S.-l., Sun, X.-f., and He, X.-h. (2014). Nitrogen Use Efficiency as Affected by Phosphorus and Potassium in Long-Term Rice and Wheat Experiments. J. Integr. Agric. 13, 588–596. doi:10.1016/s2095-3119(13)60716-9
Fowler, D., Coyle, M., Skiba, U., Sutton, M. A., Cape, J. N., Reis, S., et al. (2013). The Global Nitrogen Cycle in the Twenty-First century. Philos. Trans. R. Soc. Lond. B Biol. Sci. 368, 20130164. doi:10.1098/rstb.2013.0164
Franczuk, J., Zaniewicz-Bajkowska, A., Kosterna, E., Rosa, R., Pniewska, I., and Olszewski, W. (2010). The Effect of Cover Plants on the Yield and Content of Selected Components of Cabbage Varietes. Acta Scientiarum Polonorum-Hortorum Cultus 9, 23–30. doi:10.1109/MVHI.2010.54
Gai, X., Liu, H., Zhai, L., Tan, G., Liu, J., Ren, T., et al. (2016). Vegetable Yields and Soil Biochemical Properties as Influenced by Fertilization in Southern China. Appl. Soil Ecol. 107, 170–181. doi:10.1016/j.apsoil.2016.06.001
Gao, B., Ju, X., Su, F., Meng, Q., Oenema, O., Christie, P., et al. (2014). Nitrous Oxide and Methane Emissions from Optimized and Alternative Cereal Cropping Systems on the North China Plain: A Two-Year Field Study. Sci. Total Environ. 472, 112–124. doi:10.1016/j.scitotenv.2013.11.003
Goh, K. M., and Vityakon, P. (1983). Effects of Fertilisers on Vegetable Production 1. Yield of Spinach and Beetroot as Affected by Rates and Forms of Nitrogenous Fertilisers. New Zealand J. Agric. Res. 26, 349–356. doi:10.1080/00288233.1983.10427042
Goswami, R. S., and Kistler, H. C. (2004). Heading for Disaster: Fusarium Graminearum on Cereal Crops. Mol. Plant Pathol. 5, 515–525. doi:10.1111/j.1364-3703.2004.00252.x
Gu, B., Sutton, M. A., Chang, S. X., Ge, Y., and Chang, J. (2014). Agricultural Ammonia Emissions Contribute to China's Urban Air Pollution. Front. Ecol. Environ. 12, 265–266. doi:10.1890/14.wb.007
Guan, S., Dou, S., Chen, G., Wang, G., and Zhuang, J. (2015). Isotopic Characterization of Sequestration and Transformation of Plant Residue Carbon in Relation to Soil Aggregation Dynamics. Appl. Soil Ecol. 96, 18–24. doi:10.1016/j.apsoil.2015.07.004
Guo, J. H., Liu, X. J., Zhang, Y., Shen, J. L., Han, W. X., Zhang, W. F., et al. (2010). Significant Acidification in Major Chinese Croplands. Science 327, 1008–1010. doi:10.1126/science.1182570
Hao, T., Zhu, Q., Zeng, M., Shen, J., Shi, X., Liu, X., et al. (2019). Quantification of the Contribution of Nitrogen Fertilization and Crop Harvesting to Soil Acidification in a Wheat-maize Double Cropping System. Plant Soil 434, 167–184. doi:10.1007/s11104-018-3760-0
Hao, X. X., Yang, C. B., Yuan, Y. R., Han, X. Z., Li, L. J., and Jiang, H. (2013). Effects of Continuous Straw Returning on Organic Carbon Content in Aggregates and Fertility of Black Soil. Chin. Agric. Sci. Bull. 29, 263–269. doi:10.11924/j.issn.1000-6850.2013-0286
Harrison, R., and Webb, J. (2001). A Review of the Effect of N Fertilizer Type on Gaseous Emissions. Adv. Agron. 73, 65–108. doi:10.1016/s0065-2113(01)73005-2
Hu, C., Li, S.-L., Qiao, Y., Liu, D.-H., and Chen, Y.-F. (2015). Effects of 30 Years Repeated Fertilizer Applications on Soil Properties, Microbes and Crop Yields in Rice-Wheat Cropping Systems. Ex. Agric. 51, 355–369. doi:10.1017/s0014479714000350
Huang, M., Zhou, X., Chen, J., Cao, F., Jiang, L., and Zou, Y. (2017). Interaction of Changes in pH and Urease Activity Induced by Biochar Addition Affects Ammonia Volatilization on an Acid Paddy Soil Following Application of Urea. Commun. Soil Sci. Plant Anal. 48, 107–112. doi:10.1080/00103624.2016.1253725
Jiang, D., Hengsdijk, H., Dai, T.-B., de Boer, W., Jing, Q., and Cao, W.-X. (2006). Long-term Effects of Manure and Inorganic Fertilizers on Yield and Soil Fertility for a winter Wheat-maize System in Jiangsu, China. Pedosphere 16, 25–32. doi:10.1016/s1002-0160(06)60022-2
Ju, X.-T., Xing, G.-X., Chen, X.-P., Zhang, S.-L., Zhang, L.-J., Liu, X.-J., et al. (2009). Reducing Environmental Risk by Improving N Management in Intensive Chinese Agricultural Systems. Proc. Natl. Acad. Sci. 106, 3041–3046. doi:10.1073/pnas.0813417106
Li, Z., Schneider, R. L., Morreale, S. J., Xie, Y., Li, C., and Li, J. (2018). Woody Organic Amendments for Retaining Soil Water, Improving Soil Properties and Enhancing Plant Growth in Desertified Soils of Ningxia, China. Geoderma 310, 143–152. doi:10.1016/j.geoderma.2017.09.009
Liang, L. Z., Zhao, X. Q., Yi, X. Y., Chen, Z. C., Dong, X. Y., Chen, R. F., et al. (2013). Excessive Application of Nitrogen and Phosphorus Fertilizers Induces Soil Acidification and Phosphorus Enrichment during Vegetable Production in Yangtze River Delta, China. Soil Use Manage 29, 161–168. doi:10.1111/sum.12035
Liu, C., Wang, L., Cocq, K. L., Chang, C., Li, Z., Chen, F., et al. (2020). Climate Change and Environmental Impacts on and Adaptation Strategies for Production in Wheat-rice Rotations in Southern china. Agric. For. Meteorology 292-293, 108136. doi:10.1016/j.agrformet.2020.108136
Liu, M., Song, Y., Zhou, T., Xu, Z., Yan, C., Zheng, M., et al. (2017). Fine Particle pH during Severe Haze Episodes in Northern China. Geophys. Res. Lett. 44, 5213–5221. doi:10.1002/2017gl073210
Liu, X., Ai, Y., Zhang, F., Lu, S., Zeng, X., and Fan, M. (2005). Crop Production, Nitrogen Recovery and Water Use Efficiency in rice-wheat Rotation as Affected by Non-flooded Mulching Cultivation (NFMC). Nutr. Cycl Agroecosyst 71, 289–299. doi:10.1007/s10705-004-6801-4
Liu, X., Vitousek, P., Chang, Y., Zhang, W., Matson, P., and Zhang, F. (2016). Evidence for a Historic Change Occurring in China. Environ. Sci. Technol. 50, 505–506. doi:10.1021/acs.est.5b05972
Liu, X., Walsh, M., Ju, X., Zhang, F., Schimel, D., and Ojima, D. (2004). NO and N 2 O Fluxes from Agricultural Soils in Beijing Area*. Prog. Nat. Sci. 14, 489–494. doi:10.1080/10020070412331343841
Liu, X., Zhang, Y., Han, W., Tang, A., Shen, J., Cui, Z., et al. (2013). Enhanced Nitrogen Deposition over China. Nature 494, 459–462. doi:10.1038/nature11917
Liu, Y. Y., Sun, H. Y., Li, W., Xia, Y. L., Deng, Y. Y., Zhang, A. X., et al. (2017). Fitness of Three Chemotypes of Fusarium Graminearum Species Complex in Major winter Wheat-Producing Areas of China. PLoS ONE 12, e0174040. doi:10.1371/journal.pone.0174040
Ma, E., Zhang, G., Ma, J., Xu, H., Cai, Z., and Yagi, K. (2010). Effects of rice Straw Returning Methods on N2O Emission during Wheat-Growing Season. Nutr. Cycl Agroecosyst 88, 463–469. doi:10.1007/s10705-010-9369-1
Matsuyama, N., Saigusa, M., Sakaiya, E., Tamakawa, K., Oyamada, Z., and Kudo, K. (2005). Acidification and Soil Productivity of Allophanic Andosols Affected by Heavy Application of Fertilizers. Soil Sci. Plant Nutr. 51, 117–123. doi:10.1111/j.1747-0765.2005.tb00014.x
Maucieri, C., Nicoletto, C., Caruso, C., Sambo, P., and Borin, M. (2017). Effects of Digestate Solid Fraction Fertilisation on Yield and Soil Carbon Dioxide Emission in a Horticulture Succession. Ital. J. Agron. 12, 116–123. doi:10.4081/ija.2017.800
Meng, H.-q., Xu, M.-g., Lü, J.-l., He, X.-h., Li, J.-w., Shi, X.-j., et al. (2013). Soil pH Dynamics and Nitrogen Transformations under Long-Term Chemical Fertilization in Four Typical Chinese Croplands. J. Integr. Agric. 12, 2092–2102. doi:10.1016/s2095-3119(13)60398-6
Min, J., and Shi, W. (2018). Nitrogen Discharge Pathways in Vegetable Production as Non-point Sources of Pollution and Measures to Control it. Sci. Total Environ. 613-614, 123–130. doi:10.1016/j.scitotenv.2017.09.079
Min, J., Zhang, H., and Shi, W. (2012). Optimizing Nitrogen Input to Reduce Nitrate Leaching Loss in Greenhouse Vegetable Production. Agric. Water Manage. 111, 53–59. doi:10.1016/j.agwat.2012.05.003
Ministry of Agriculture of the People's Republic of China (2021). China Agiculture Yearbook. Beijing: China Agriculture Press.
Mo, B., Zhu, B., Gao, M. R., and Liu, G. C. (2005). Analyzing the Characteristics and Influence Factors of Erosion and Sediment Production of Purple Soil Shale. Res. Soil Water Conservation 12, 129–131. doi:10.3969/j.issn.1005-3409.2005.01.038
National Bureau of Statistics of China (2021). China Statistical Yearbook. Beijing: China Statistics Press. Available at: http://www.stats.gov.cn/.
Qiao, J., Yang, L., Yan, T., Xue, F., and Zhao, D. (2012). Nitrogen Fertilizer Reduction in rice Production for Two Consecutive Years in the Taihu Lake Area. Agric. Ecosyst. Environ. 146, 103–112. doi:10.1016/j.agee.2011.10.014
Su, M., Kuang, F., Lv, Y., Shi, X., Liu, X., Shen, J., et al. (2017). Nitrous Oxide and Methane Emissions from Paddy Soils in Southwest China. Geoderma Reg. 8, 1–11. doi:10.1016/j.geodrs.2016.12.003
Tian, Y.-H., Yin, B., Yang, L.-Z., Yin, S.-X., and Zhu, Z.-L. (2007). Nitrogen Runoff and Leaching Losses during rice-wheat Rotations in Taihu Lake Region, China. Pedosphere 17, 445–456. doi:10.1016/s1002-0160(07)60054-x
Tilman, D., Fargione, J., Wolff, B., D'Antonio, C., Dobson, A., Howarth, R., et al. (2001). Forecasting Agriculturally Driven Global Environmental Change. Science 292, 281–284. doi:10.1126/science.1057544
Timsina, J., and Connor, D. J. (2001). Productivity and Management of rice-wheat Cropping Systems: Issues and Challenges. Field Crops Res. 69, 93–132. doi:10.1016/s0378-4290(00)00143-x
van Gestel, C. A. M., Kruidenier, M., and Berg, M. P. (2003). Suitability of Wheat Straw Decomposition, Cotton Strip Degradation and Bait-Lamina Feeding Tests to Determine Soil Invertebrate Activity. Biol. Fertil. Soils 37, 115–123. doi:10.1007/s00374-002-0575-0
Walter, I., Miralies de Imperial, R., and Bigeriego, M. (1996). Long Term Effects of Wheat Straw Incorporation Compared with Burning on Wheat Yield and Soil Properties, Springer Netherlands. Fertilizers Environ. 66, 463–465. doi:10.1007/978-94-009-1586-2_79
Wang, F., He, Z., Sayre, K., Li, S., Si, J., Feng, B., et al. (2009). Wheat Cropping Systems and Technologies in China. Field Crops Res. 111, 181–188. doi:10.1016/j.fcr.2008.12.004
Wang, X.-Z., Zhu, J.-G., Gao, R., Yasukazu, H., and Feng, K. (2007). Nitrogen Cycling and Losses under rice-wheat Rotations with Coated Urea and Urea in the Taihu Lake Region. Pedosphere 17, 62–69. doi:10.1016/s1002-0160(07)60008-3
Wang,Yang, S. M., Yang, M., Liao, S., Sheng, W., Shi, X., Lu, J., et al. (2019). Yield and the 15 N Fate in Rice/Maize Season in the Yangtze River Basin. Agron.j. 111, 517–527. doi:10.2134/agronj2018.06.0379
Xie, H., Dong, J., Shen, Z., Chen, L., Lai, X., Qiu, J., et al. (2019). Intra- and Inter-event Characteristics and Controlling Factors of Agricultural Nonpoint Source Pollution under Different Types of Rainfall-Runoff Events. Catena 182, 104105. doi:10.1016/j.catena.2019.104105
Xu, J., Peng, S., Yang, S., and Wang, W. (2012). Ammonia Volatilization Losses from a rice Paddy with Different Irrigation and Nitrogen Managements. Agric. Water Manage. 104, 184–192. doi:10.1016/j.agwat.2011.12.013
Xu, W. Y., Jin, J. X., Jin, X. B., Xiao, Y. Y., Ren, J., Liu, J., et al. (2019). Analysis of Changes and Potential Characteristics of Cultivated Land Productivity Based on MODIS EVI: A Case Study of Jiangsu Province, China. Remote Sensing 11 (17), 2041. doi:10.3390/rs11172041
Xu, X., Ouyang, X., Gu, Y., Cheng, K., Smith, P., Sun, J., et al. (2021). Climate Change May Interact with Nitrogen Fertilizer Management Leading to Different Ammonia Loss in China's Croplands. Glob. Change Biol. 27, 6525–6535. doi:10.1111/gcb.15874
Xue, L., Yu, Y., and Yang, L. (2014). Maintaining Yields and Reducing Nitrogen Loss in rice-wheat Rotation System in Taihu Lake Region with Proper Fertilizer Management. Environ. Res. Lett. 9, 115010. doi:10.1088/1748-9326/9/11/115010
Yan, X. Y., Ti, C. P., Vitousek, P., Chen, D. L., Leip, A., Cai, Z. C., et al. (2014). Fertilizer Nitrogen Recovery Efficiencies in Crop Production Systems of China with and without Consideration of the Residual Effect of Nitrogen. Environ. Res. Lett. 9, 095002. doi:10.1088/1748-9326/9/9/095002
Yi, Q., He, P., Zhang, X. Z., Yang, L., and Xiong, G. Y. (2015). Optimizing Fertilizer Nitrogen for Winter Wheat Production in Yangtze River Region in China. J. Plant Nutr. 38, 1639–1655. doi:10.1080/01904167.2015.1061547
Zhang, M., Tian, Y., Zhao, M., Yin, B., and Zhu, Z. (2017). The Assessment of Nitrate Leaching in a rice-wheat Rotation System Using an Improved Agronomic Practice Aimed to Increase rice Crop Yields. Agric. Ecosyst. Environ. 241, 100–109. doi:10.1016/j.agee.2017.03.002
Zhao, B., Ata-Ui-Karim, S. T., Yao, X., Tian, Y., Cao, W., Zhu, Y., et al. (2016). A New Curve of Critical Nitrogen Concentration Based on Spike Dry Matter for Winter Wheat in Eastern China. PLoS ONE 11, e0164545. doi:10.1371/journal.pone.0164545
Zhong, Y., Wang, X., Yang, J., Zhao, X., and Ye, X. (2016). Exploring a Suitable Nitrogen Fertilizer Rate to Reduce Greenhouse Gas Emissions and Ensure rice Yields in Paddy fields. Sci. Total Environ. 565, 420–426. doi:10.1016/j.scitotenv.2016.04.167
Zhou, M., Zhu, B., Brüggemann, N., Dannenmann, M., Wang, Y., and Butterbach-Bahl, K. (2016). Sustaining Crop Productivity while Reducing Environmental Nitrogen Losses in the Subtropical Wheat-maize Cropping Systems: A Comprehensive Case Study of Nitrogen Cycling and Balance. Agric. Ecosyst. Environ. 231, 1–14. doi:10.1016/j.agee.2016.06.022
Keywords: wheat/vegetable, 15N-labeled, yield, nitrogen fate, Yangtze River Basin
Citation: Wang S, Yang LS, Liao SP, Sun B, Shi XJ, Lu JW, Guo SW, Shen JB, Zhang FS, Goulding K and Liu XJ (2022) Impact of Nutrient Management on Wheat/Vegetable Yields and the Fate of 15N-Labeled Fertilizer in the Yangtze River Basin. Front. Environ. Sci. 10:772972. doi: 10.3389/fenvs.2022.772972
Received: 09 September 2021; Accepted: 14 February 2022;
Published: 28 March 2022.
Edited by:
Silvana Munzi, University of Lisbon, PortugalReviewed by:
Fumiaki Takakai, Akita Prefectural University, JapanMaria Amélia Martins-Loução, University of Lisbon, Portugal
Copyright © 2022 Wang, Yang, Liao, Sun, Shi, Lu, Guo, Shen, Zhang, Goulding and Liu. This is an open-access article distributed under the terms of the Creative Commons Attribution License (CC BY). The use, distribution or reproduction in other forums is permitted, provided the original author(s) and the copyright owner(s) are credited and that the original publication in this journal is cited, in accordance with accepted academic practice. No use, distribution or reproduction is permitted which does not comply with these terms.
*Correspondence: Xue Jun Liu, bGl1MzEwQGNhdS5lZHUuY24=