- Desert Agriculture and Ecosystems Program, Environment and Life Sciences Research Center, Kuwait Institute for Scientific Research, Kuwait City, Kuwait
Drought is the most critical limiting factor affecting plant survival, development, and adaptation in the desert ecosystem. Native desert plant species are adaptable natural biomass crops of the desert’s ecosystem. These native species use water efficiently and tolerate drought and other environmental constraints. In addition, they sustain moderate nutritional values that are essential in terms of maintaining optimum vegetation, development and productivity, and tolerating natural grazing. The objective of this study was to quantify the biomass production and nutritive values of three native desert grass species as influenced by drought stress, that is, 100, 75, 50, and 25% potential evapotranspiration (ETp). Three native desert grasses, viz., Cenchrus ciliaris, Lasiurus scindicus, and Pennisetum divisum were grown in pots, irrigated with fresh water in a greenhouse, and investigated for their drought tolerance and nutritive values. The results showed that the grasses differ in their responses to drought stress by controlling stomata conductance (gs). The gs increased significantly (p < 0.001) with adequate irrigation (100 and 75% ETp) and decreased with 50 and 25% ETp in all species. Under moderate drought stress (75 and 50% ETp), all desert grasses used the water efficiently and produced a considerable dry matter yield and stable amounts of nutritive value. At 50% ETp, all desert species showed significant increase (p < 0.001) in root biomass than shoots that increased water absorption to survive a drought environment. L. scindicus exhibited a significantly higher increase (p < 0.001) in root formation at 25% ETp than the 100% ETp treatment. In all the grass species, the protein contents increased as drought stress increased. No significant differences in fat content were detected under all irrigation conditions, while the ash content was significantly (p < 0.001) influenced by severe drought stress. Under natural desert conditions, these adjustments may reduce transpiration demand relative to water deprivation, leading to improved species establishment and supported adaptation to severe desert environments. Understanding the physiological responses and the requirements of desert plant species can be an excellent way to restore ecological functions and biodiversity conservation in a desert environment.
Introduction
Desert plants are frequently exposed to diversified environmental stresses, restricting the chance of reproduction, seedling establishment, and development. Drought and low soil moisture are considered the leading factors for plant survival in desert regions (North and Nobel 1998). Desert plants experience a fluctuating water supply during their life cycle in changing climates. Low and infrequent rainfall during the year exposes plants to periodic soil drying (Liang, et al., 2002). Plants in a desert ecosystem withdraw nutrients from senescing structures to develop tissues, a critical mechanism in order to reduce the dependence on nutrient uptake and, consequently, increase plant fitness in a low-nutrient environment (Lü et al., 2012). This critical mechanism with regard to determining desert plant fitness may rely particularly on reabsorbed nutrients due to poor soil fertility (Killingbeck, 1993; Han et al., 2013). Nutrient resorption by these desert plants may be severely affected by drought stress (Marchin et al., 2010), which may influence the nutritional value concentration of plants established under desert conditions (Wang et al., 2017). Therefore, the impact of drought stress on the nutritional content should receive significant attention in order to understand the responses and species requirements as a way of predicting the long-term consequences of their interactions with the environment and to ensure vigorous development of the ecological functions of the desert ecosystems.
Of all the environmental factors adversely affecting the ecology of desert terrestrial ecosystems, soil salinity, water availability, and temperature are probably the most important and common environmental problems (Piatt, 1976; Briedé and McKell, 1992; Breckle, 2002). Osmotic stress due to high levels of soil salinity may hinder nutrient resorption (Wang et al., 2017). Drought, in conjunction with salinity, may increase surface evaporation and pose the most severe environmental restriction to plant nutrient uptake and cycling (Hu and Schmidhalter, 2005). Long periods of drought, high temperatures, and increased soil salinity in a desert ecosystem may dramatically influence the eco-physiology of plants, limit their production, and influence their nutritive value (Madouh, 2013).
Drought leads to water deficits in the leaf tissue and affects many physiological processes. This can ultimately result in severe consequences for plant growth and survival (Vilagrosa et al., 2003). Water availability affects plants in several ways, and the plant responses to drought stress are complex (Chaves et al., 2002; Xiongwen, 2002). Desert plants normally cope with drought by either tolerance or avoidance mechanisms. Both these mechanisms contribute to the ability of a plant to survive drought. However, survival also depends on the frequency and severity of the drought episodes (Nilsen, et al., 1984; Larcher, 1995; Chaves et al., 2002; Chaves et al., 2003). Adaptation strategies include well-developed root systems, a shift in the allocation of assimilates from the shoot to root, closure of stomata in response to water stress, and the restriction of further water loss, osmotic adjustment, and certain changes in leaf morphology (Burghard and Riederer, 2003; Griffin et al., 2004).
One of the immediate responses to drought is the reduction in the stomata aperture. When water is limited, conductance decreases as a result of stomata closure (Zhang et al., 1997). Stomata closure is the first line of defense against desiccation since it is much quicker than adjustment in root growth and leaf area reduction. Some drought-tolerant species may be particularly effective at controlling the stomata aperture to allow for particular changes in CO2 intake and fixation, while reducing the volume of water lost through their pores. Other plants may open stomata rapidly when drought conditions are relieved, which allows them to maximize carbon gain and hence enhance survival (Atteya, 2003). Therefore, stomata closure has a great effect on photosynthesis and transpiration (Ehleringer and Cook, 1984; Zhang et al., 1997; Jones, 1998). By limiting transpiration, stomata closure can improve plant water-use efficiency and indirectly influence productivity (Maherali et al., 2003). Stomata closure response to drought may be due to root sensing and soil drying, producing a chemical signal which travels upward from the root to the leaves and leads to the closure of stomata (Gollan et al., 1986; Gowing et al., 1990; Davies and Zhang, 1991).
Root morphology is important for successful seedling establishment and for the survival of desert rangeland species. Root development is vital for the survival of most desert plants coping with limited water availability (Hassanyar and Wilson, 1978; Johnson and Aguirre, 1991). The capacity of roots to rapidly elongate allows root growth to advance beyond the soil drying front so that the plants can avoid desiccation (Aguirre and Johnson, 1991). Seedling survival in a drought-prone environment may depend upon a species being able to compensate for the negative effect of low water potential in the soil and atmosphere by adjusting root and shoot morphology and physiology mechanisms (Khalil and Grace, 1992). The capacity for adjustment, or acclimation, is presumed to be a complex genetic trait involving a range of physiological mechanisms (Khalil and Grace, 1992; Dias-Filho, 1995).
The desert ecosystem of Kuwait has diverse environmental constraints, including low rainfall, drought, high soil salinity, extreme temperatures, intense sunshine, low moisture retention, and infertile soil, all of which cumulatively impact plant growth and development. Despite these ecological restrictions, the natural flora is well-adapted to these adverse environmental conditions (Madouh and Al-Sabbagh, 2021). However, increased human and animal influences, along with extreme climatic conditions in the desert’s ecosystem, have contributed to substantial loss and depletion of native vegetation cover species. These conditions have resulted in the creation of vast degraded rangelands across the deserts of Kuwait (Omar, 1991; Zaman, 1997; Zaman, 2000; Misak et al., 2002). In order to diminish the risk of the failure of revegetation schemes, rangeland regeneration, and the variation of species requirements in the desert ecosystem, it is necessary to fully understand the factors involved in the process of adaptation and survival responses to severe environmental limitations (Piatt, 1976; Briedé and McKell, 1992). Because of the erratic precipitation and high evapotranspiration in arid lands, soil moisture is often inadequate for seed germination and seedling establishment (Le Houerou, 1996).
The researchers have identified several eco-physiological adaptive mechanisms associated with plants grown in drought environments. However, research on water-use strategies and mechanisms to control water loss compared with nutritive values for individual plant species under drought environments are scanty (Liang et al., 2002; Xiongwen, 2002; Wang et al., 2017). Native desert plants of Kuwait, particularly perennial grasses, are commonly found in extreme desert habitats. Madouh (2005), Madouh (2013) indicated that some of these species seem to resist drought stress naturally and maintain metabolic efficiency throughout the year, even under the harsh conditions of the desert ecosystem. In light of this, a greenhouse experiment was designed to determine how the desert plants Cenchrus ciliaris, Lasiurus scindicus, and Pennisetum divisum respond to drought stress in order to determine if the mechanisms for adaptation are common among these various species compared with their nutritional values (protein, fat, and ash). The results from the experiment will provide an in-depth understanding of the adaptation mechanisms to drought stress of these desert plant species and new evidence with regard to rangeland management and desert habitat conservation and restoration.
Materials and Methods
Seed Collection and Harvesting
Field visits were made to a confined and protected area in the Kuwait Institute for Scientific Research Station for Research and Innovation (KSRI) (29.14°N, 47.62°E, and 149 m above the sea level) located in Kabd, Al Jahra Governorate, Kuwait (Figure 1). The mean annual rainfall in the area was 118 mm, mostly occurring in winter months. The ecosystem which was used for seed collection is described as the desert plain. Three native perennial desert grasses C. ciliaris, L. scindicus, and P. divisum were identified. The identification was based on their adaptation and tolerance levels to desert environmental conditions (drought and heat stress). Palatability (in terms of animal fodder), digestibility, and nutritional values were also considered desirable fodder qualities when it came to selecting the plant species.
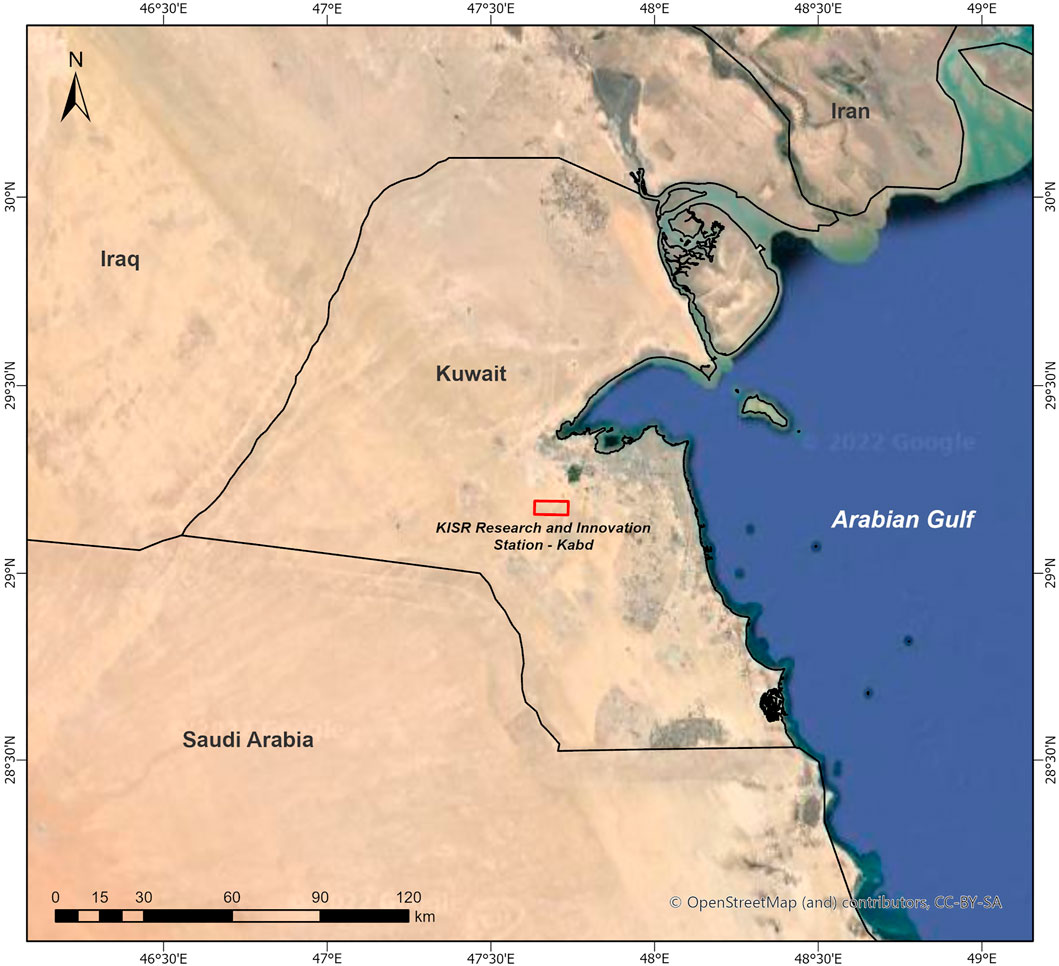
FIGURE 1. Map of Kuwait showing the location of the KISR (Kuwait Institute for Scientific Research) Research and Innovation Station—Kabd, Al-Jahra Governorate, Kuwait.
Seed collection and harvesting were undertaken during the mature and ripened stage to ensure plant quality and development. Seeds were collected manually. The seeds were fully cleaned and kept in cold storage for future use. Percentage of seed germination and viability tests were conducted on the cleaned seeds to ensure high rates of germination prior to the execution of the experiment.
Collection of Soil Samples and Experimental Assay Method
A bulk soil sample was collected from the area from which seeds were collected in the form of a 0–30 cm soil surface of a mixed, hyperthermic Typic Torripsamments (Soil Survey Staff, 2014a) soil taxa, the dominant desert soil in Kuwait (KISR, 1999). The soil was air-dried and passed through a 2-mm sieve (fine earth fraction) and then used to fill the pots. Plastic pots of 3.1 L volume capacity were used. The soil was analyzed for basic chemicals [EC of soil saturation extract (ECe), pH of saturated soil paste (pHs)], and physical (soil texture, bulk density and porosity, Munsell soil color, and field capacity) and organic matter using standard soil analytical procedures (Soil Survey Staff, 2014b). Fresh water (EC 215 μS/cm) was used for irrigation. The experiment was conducted in a greenhouse. The seeding was carried out in plastic pots (16.5 cm diameter and 14.6 cm length ∼3.1-L volume). 4.5 kg soil was added to each pot before the initiation of the experiment.
Growth Condition
The experiment was conducted in a greenhouse to evaluate the responses to drought stress of three native desert species. Greenhouse temperatures and humidity levels were monitored daily and were maintained at a 23° to 27°C temperature range for optimizing seed germination requirements. Uniform-sized seeds of three grasses were separated from the bulk sample for unification. Four seeds of each species were directly seeded in each pot and were germinated over a period of 2 weeks. After the 4th leaf stage, seedlings were thinned, and one plant in each pot was retained to complete the experiment. The irrigation level was determined by measuring potential evapotranspiration (ETp) by simulating a class A pan in the green house. Fresh irrigation water was used every day. Drip-irrigation was controlled via independent solenoids with a controller (ESP Modular, Rain Bird, United States) for each treatment. Pressure-compensated drippers (2 L/h. LBS. Rain Bird, United States) were used in the irrigation system with one drip nozzle per pot. This irrigation system provided precise irrigation to a large number of plants.
Drought-Imposed Treatment and Water Status
Immediately, when seedlings had been developed to the true fourth leaf stage, four irrigation treatments were applied to measure and investigate the influence of drought stress on growth development, productivity, and survival. The seedlings of all three plant species were compared for their productivity under the four irrigation treatments (no drought, two rates of moderate drought, and severe drought). The water application of the no-drought treatment involved full water status, that is, a potential evapotranspiration rate of 100% ETp, whereas the two moderate-drought regimes were equivalent to 75 and 50% ETp. In the case of severe-drought treatment, the watering level was maintained at 25% ETp. All plant species were irrigated every day. The ETp was measured weekly and calculated on daily basis irrigation, and all seedlings grown under the four irrigation regimes were brought back to the desired level of each irrigation treatment until the termination of the experiment.
The soil moisture content was measured using a moisture probe meter (MPM-160-B) provided by ICT International Pty Ltd. Australia. The moisture measurement probe was inserted vertically into the soil at two positions within the pots. The water content associated with each moisture regime treatment was monitored at weekly intervals before and after irrigation and was undertaken throughout the experiment. The water content was measured in mV unit.
Stomatal Conductance (gs)
The abaxial leaf conductance to water vapor diffusion at the surface of the fully developed leaves was measured with a diffusion porometer (Model SC-1, Leaf Porometer, Decagon Devices). Stomatal conductance (gs) measurements were taken for 9 weeks, starting immediately after the imposition of the different moisture regime treatments. Measurements of gs were made during the day between 9.00 and 12.00 h. The data of stomatal conductance were collected for six plants (replicates) for each plant species in each treatment combination and repeated across two positioned blocks within the greenhouse.
Aboveground and Belowground Biomass
At the end of the experiment, the aboveground biomass was harvested from each treatment/replication and weighed immediately in the greenhouse to avoid moisture loss. Later, the biomass was dried at 70°C in an oven to constant weight. The plant roots were carefully harvested and washed with tap water to remove soil particles. The root dry weight (g) was determined for each plant across all species and treatments.
Nutritive Quality Measurements
The plant samples were ground in a mill (Thomas Scientific; GE motor industrial system) and passed through a 1.0-mm screen. The analytical methods of the Association of Official Analytical Chemists (AOAC International, 1995) were used for plant analysis. The proximate composition of the nutritive value as indicated by crude protein, fat, and ash was determined for all plant species from each treatment using samples oven-dried at 50°C for 7 days.
The percentage of crude protein was estimated by multiplying N% with a standard factor (6.25). Nitrogen percentage was determined after digesting the samples and using the Kjeldahl apparatus. Petroleum ether (60°–80°C) was used to dissolve the fat in the sample. The dissolved fat is then recovered by evaporating the solvent, and the crude fat percentage was then calculated gravimetrically. For ash percent determination, a known weight of the sample was ignited at 600°C for 6 h, and the sample residue was then weighed gravimetrically.
Statistical Analysis and Experimental Design
The experimental design consisted of three desert plant species, four irrigation treatments, and two replicate blocks with eight individuals for each treatment. The arrangement of the experiment design was a split-plot under randomized block design, with the main plots being the irrigation regimes and the subplots, species interaction. Statistical analysis was performed at the end of the experiment using IBM SPSS Statistics (Version 24) software by pooling the data from the whole host or separated based on the individual species, as appropriate. All reported values were in the form of the means of eight replicates of each treatment.
Results
Characteristics of Soil and Water Used
The sandy desert soil is slightly calcareous (7% CaCO3 equivalents), very slightly saline (ECe 2.84 dS/m), moderately alkaline (pHs 7.55), low in organic matter (< 1%), and light yellowish brown (10 YR 6/4) based on the Munsell color notation, porous (31%), bulk density (1.60 g/cm3), field capacity (8%), and of a sandy texture (sand 96%; silt + clay = 4%). This soil represents the dominant desert soils found in Kuwait (KISR, 1999; Omar and Shahid, 2013). Fresh water (EC 200 μS/cm and pH 7.02) was used for irrigating the plants.
Water-Use Efficiency and Productivity Under Drought Stress
The differences among the volumetric soil water content treatments of no drought (100% ETp), moderate drought (75%, 50% ETp), and severe drought (25% ETp) measured before and after irrigation were significantly different (p < 0.001) for all desert plant species at the start and end of the imposed irrigation regimes (Figure 2). Throughout the course of the treatments, and for all plant species, the water content of the 100% ETp regime remained between 743.6 and 866.2 mV, between 686 and 735 mV for the 75% ETp regime, and between 401.4 and 570 mV for the 50% ETp; whereas in the case of the 25% ETp regime, the moisture content was stabilized between 207.2 and 453.2 mV throughout the experiment (d.f. = 89 for each treatment). This shows that the soil moisture content decreased as the drought conditions increased.
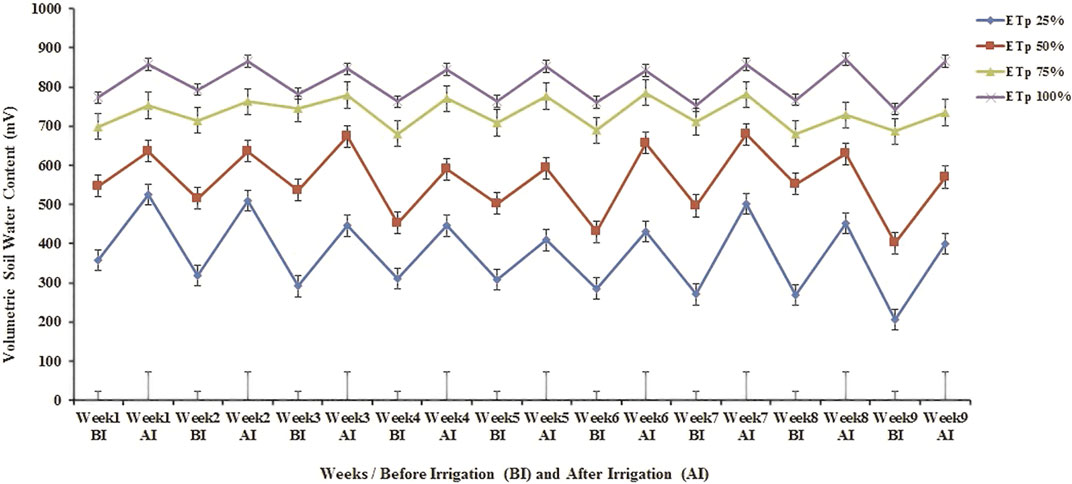
FIGURE 2. Effects of various irrigation regimes on volumetric soil water content (mV) measured by a moisture meter probe in all treatments during a 9-week period. Bars (┬) represent the Least Significant Difference values (LSD) for comparisons between treatments at p < 0.001 and p < 0.05. d. f. = 89.
Stomatal Conductance
The stomatal conductance (gs) varied noticeably under the different irrigation treatments of 100, 75, 50, and 25% ETp in all desert plant species. The stomatal conductance increased with adequate irrigation and decreased in limited irrigation conditions for all plant species. The differences in the stomatal conductance of the various plants species were constantly statistically significant under different irrigation treatments (Figure 3). During the course of the experiment, the plant species grown under 75, 50, and 25% ETp treatments adapted to drought stress by partial closure of the stomata, as shown by the reduction in conductance, compared to well-watered plants of 100% ETp treatment. However, under severe drought conditions (25% ETp), significant reduction (p < 0.001) in the stomatal conductance occurred which led to an almost complete stomatal closure in all plant species (Figure 3). In C. ciliaris, the stomatal conductance (gs) before and after irrigation in case of 50 and 25% ETp was significantly (p < 0.001) different from that of 100% ETp irrigation treatment (Figure 3), whereas in the case of L. scindicus, stomatal conductance (at 50 and 25% ETp) was significantly different from both 75 and 100% ETp. In P. divisum, significant reduction in stomatal conductance was observed in the case of drought-stressed treatments (ETp 25, 50, and 75%) compared with ETp 100%. The C. ciliaris exposed to 100 and 75% ETp showed a higher mean stomatal conductance of 34.43 and 35.88 mmol m−2 s−1, respectively, than that exposed to 50 and 25% ETp (23.78 and 16.25 mmol m−2 s−1 respectively) (Figure 3). Overall, L. scindicus grown at 50% ETp demonstrated better control of stomatal conductance than in the other three irrigation treatments, with mean values of 17 and 35 mmol m−2 s−1 for before and after irrigation, respectively. A similar trend was found in P. divisum at 50 and 75% ETp. In C. ciliaris, the stomatal conductance (gs) associated with the 25% ETp treatment was significantly lower (p < 0.001) than that of the other three irrigation treatments. In contrast, in the case of L. scindicus and P. divisum at 25% ETp, stomata remained partially closed before and after irrigation. In all plants, the stomatal conductance was considerably reduced at 25% ETp (Figure 3), while at 100% ETp, it was the highest.
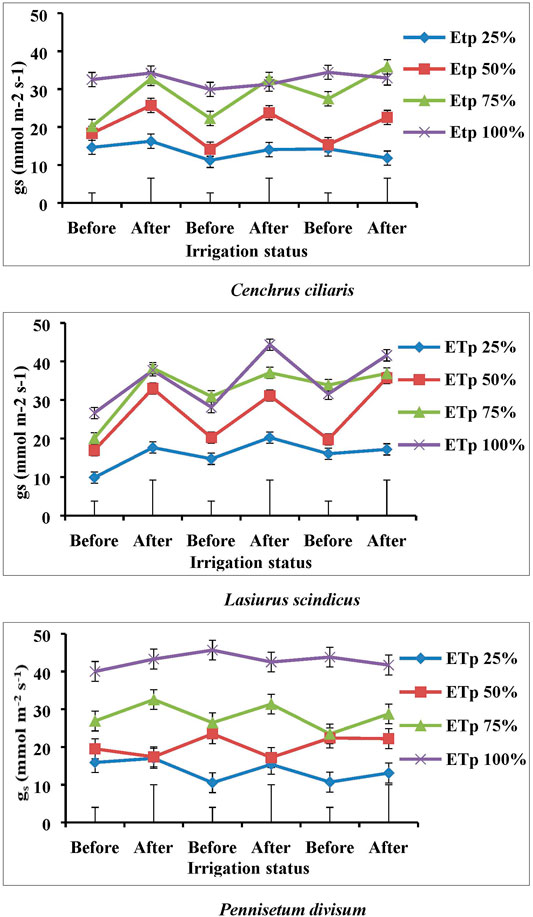
FIGURE 3. Effect of different irrigation regimes on the stomatal conductance (gs) of Cenchrus ciliaris, Lasiurus scindicus, and Pennisetum divisum. Bars (┬) represent the Least Significant Difference values (LSD) for comparisons between treatments at p < 0.001 and p < 0.05. d. f = 95.
Aboveground Biomass Production
Differences in the fresh and dry biomass weight above soil were observed among all desert plants species and in terms of various irrigation treatments. All plant species have shown the highest increase in fresh and dry weight at 75% ETp treatment, with differences being always significant (p < 0.001) (Figure 4) in the C. ciliaris and P. divisum plant species, but in the case of L. scindicus, the differences were not consistently significant among all the irrigation treatments. The lowest fresh and dry weight was recorded in all plant species at 25% ETp irrigation treatment.
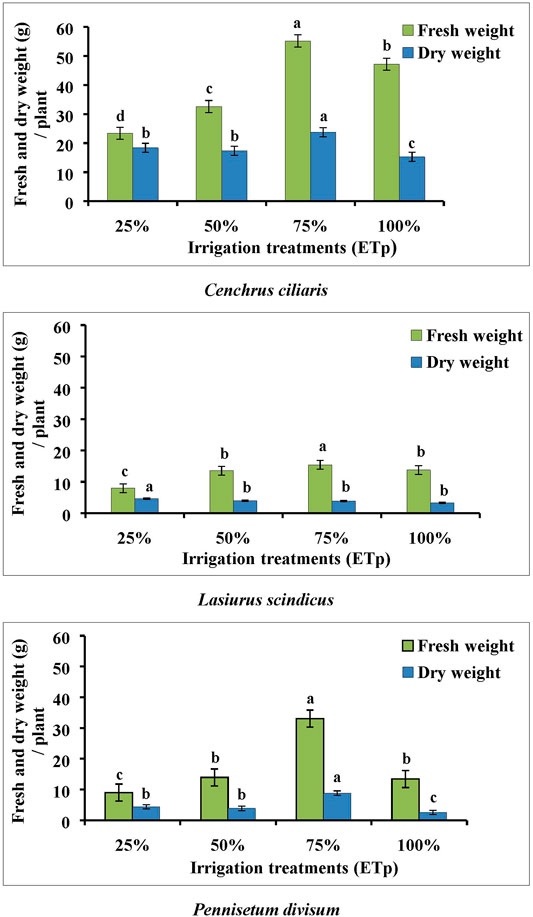
FIGURE 4. Effect of different irrigation regimes on mean fresh and dry weight measurement of Cenchrus ciliaris, Lasiurus scindicus, and Pennisetum divisum. Bars labeled with different letters present significant difference between treatments at p < 0.001 and p < 0.05. d. f = 16.
In C. ciliaris, the maximum mean values of the fresh and dry weight production of shoots was 55.18 and 23.75 g/plant, respectively, for 75% ETp treatment. In P. divisum, at 50% ETp and 100% ETp treatments, fresh weight production of shoots was lower than that found in the case of 75% ETp treatment, with means of 13.93 and 13.38 g/plant, respectively. In L. scindicus, the dry weight yield was nearly similar in terms of various irrigation treatments, but in case of P. divisum, at 25% ETp treatment, the dry weight production was greater (mean 4.4 g/plant) than that in the 50% ETp and 100% ETp treatments (3.8 and 2.55 g/plant, respectively) (Figure 4).
Belowground Biomass Production
Significant differences (p < 0.001) in root dry weight were recorded in the three desert plants species at the four irrigation levels of 100, 75, 50, and 25% ETp, showing the influence of different irrigation regimes. The root growth of all three plant species increased with reduced irrigation treatments compared to 100% ETp, except for C. ciliaris at 25% ETp, and these differences were considerable. Visual observations showed that a large proportion of roots for all three plants species was grown in 50 and 25% ETp at the base of the container, while at 100% ETp treatment (C. ciliaris), a considerable number of lateral roots were developed. The root growth of C. ciliaris was significantly (p < 0.001) greater in the 75 and 50% ETp irrigation treatments (Figure 5) than that of 100% ETp, and the mean values of the root mass were 17.85 and 22.05 g/plant. However, under the 100 and 25% ETp irrigation treatments, the mean root growth was 8 and 7.1 g/plant, respectively (Figure 5).
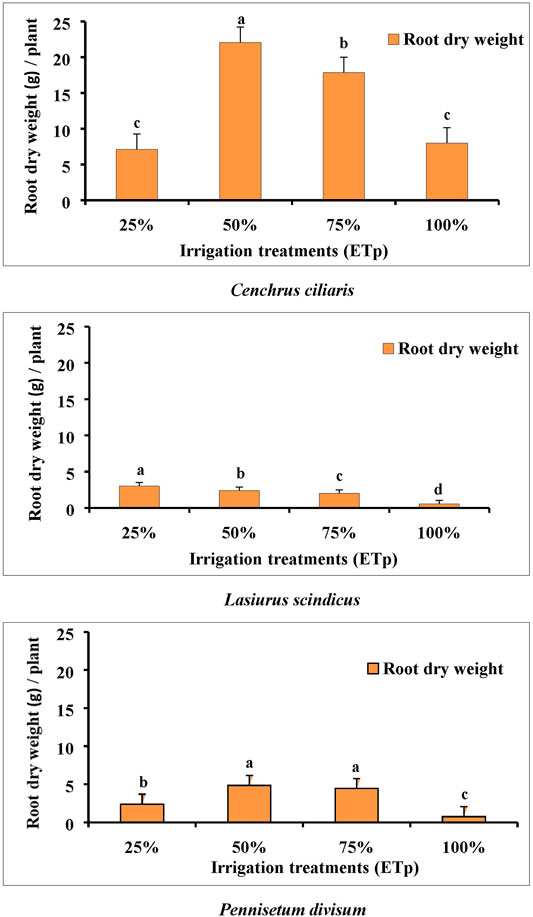
FIGURE 5. Effect of different irrigation regimes on mean root dry weight measurement of Cenchrus ciliaris, Lasiurus scindicus, and Pennisetum divisum. Bars labeled with different letters present significant difference at p < 0.001 and p < 0.05 between treatments. d. f = 16.
In L. scindicus, the root growth showed significant differences among all the different irrigation treatments (Figure 5). Overall, the root dry mass was poor under all irrigation levels, but was the highest at 25% ETp. The maximum mean value recorded was 3 g/plant at 25% ETp, while the minimum was 0.5 g/plat at 100% ETp (Figure 5).
The trend with regard to the root growth of P. divisum was similar to that of C. ciliaris, with a considerable difference (p < 0.001) in the root dry weight produced in the 75 and 50% ETp irrigation treatments compared to 100% ETp. The root dry weight of P. divisum was less than that of the roots of C. ciliaris and higher than that of L. scindicus, except at 25% ETp. The maximum mean values recorded were 0.75, 4.47, 4.84, and 2.4 g/plant at 100, 75, 50, and 25% ETp, respectively (Figure 5).
Nutritive Quality Determinations
The nutritive quality (protein, fat, and ash) of the three native plant species (C. ciliaris, L. scindicus, and P. divisum) irrigated at 100, 75, 50, and 25% ETp varied between each plant species (Figure 6). Overall in all three plant species, the protein concentration increased significantly (p < 0.001), and the ash content decreased with increased drought. The fat concentration remained relatively similar under various irrigation statuses (Figure 6).
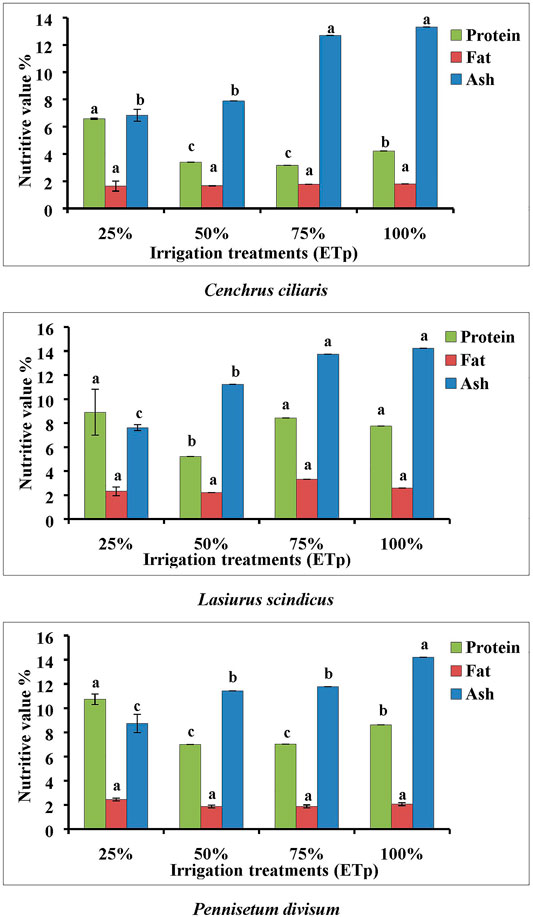
FIGURE 6. Effect of different irrigation regimes on mean protein, fat, and ash percentage of Cenchrus ciliaris, Lasiurus scindicus, and Pennisetum divisum. Bars labeled with different letters present significant difference at p < 0.001 and p < 0.05 between treatments. d. f = 16.
The protein percentage of C. ciliaris increased significantly (p < 0.001) under severe drought conditions (25% ETp) compared with that under the other three treatments (100, 75, and 50% ETp).
In L. scindicus, there was no significant effect on the protein percentage under different irrigation statuses (Figure 6), although, at 50% ETp, the protein percentage was the lowest.
In P. divisum, the protein percentage increased drastically (p < 0.01) under severe drought conditions (25% ETp) at a mean of 10.7% compared to the 100, 75, and 50% ETp conditions with means of 8.6, 7, and 6.9, respectively (Figure 6). In all three plant species, no significant effect of irrigation regimes on fat concentration was recorded, with the fat percentage remaining almost similar under all irrigation treatments. Conversely, in all three plant species, the concentration of ash increased greatly with decreased drought (Figure 6). In L. scindicus, the ash concentration at 100, 75, 50, and 25% ETp was recoded as 14.2, 13.7, 11.3, and 7.6%, respectively (Figure 6). A similar decreasing trend was observed in the case of P. divisum with respect to irrigation regimes, where the lowest ash concentration (8.7%) was recorded at 25% ETp and the maximum at 100% ETp (14.2%).
Discussion
In the desert ecosystem, drought and other environmental constraints, that is, heat, wind, and salinity are among the most critical abiotic limiting factors affecting plant growth, development, and adaptation (Madouh, 2013; Luo et al., 2021). This research project was conducted with the aim of identifying some of the physiological responses of Kuwait native desert plants (grasses) to drought stress in terms of stomatal conductance, biomass (shoot and roots), and nutritional values (protein, fat, and ash). The growth responses, productivity, and nutritive values of three native desert grasses (C. ciliaris, L. sindicus, and P. divisum) were investigated under greenhouse conditions in terms of various irrigation treatments, that is, 100% ETp (no drought), 75 and 50% ETp (moderate drought), and 25% ETp (severe drought). The soil used in the experiment represented the dominant sandy soil found in the deserts of Kuwait (KISR, 1999; Omar and Shahid, 2013) and in the farming areas of Kuwait (Shahid et al., 2004). Thus, the adoption of the findings from the present study will have a direct impact on rangeland management, rehabilitation, and restoration as a means of improving desert ecosystem functions.
Stomatal Conductance
Several studies have reported that plants must regulate transpiration through stomatal or leaf conductance in order to avoid desiccation and maintain an efficient stomatal control to conserve water (Xiongwen, 2002; Aasamaa and Sõber, 2011). The impact of climate change, in the form of associated frequent drought episodes and an increased sensitivity to environmental change, may influence the stomatal mechanism in plants. The sensitivity and response time to drought differs between various plant species, and plants have developed several different mechanisms that increase desiccation tolerance and water retention (Aasamaa and Sõber, 2011; Arve et al., 2011). The responses of stomatal conductance (gs) of the three desert plants investigated varied remarkably under the different irrigation treatments of 100, 75, 50, and 25% ETp. The results have shown that stomatal conductance increases with adequate moisture level and decreases with limited irrigation for all of the plant species. Differences in the stomatal conductance of various plant species were constantly statistically significant under different moisture conditions (Figure 3).
During the course of the experiment, all three native plant species grown under 75, 50, and 25% ETp irrigation treatments adopted partial closure of stomata, as shown by the reduction of conductance, compared to that of well-watered plants (100% ETp treatment) (Figure 3). However, under severe drought conditions (25% ETp), significant reduction (p < 0.001) in stomatal conductance was noted from the beginning to the end of the experiment, followed by gradual reduction, leading to an almost complete stomatal closure in almost all plant species. The stomatal conductance of all three plant species increased when irrigation was applied and then decreased when soil water was depleted (prior to irrigation). This suggests that water applied to these treatments was sufficient to maintain stomatal behavior and continued growth in all three plant species. This indicates that stomatal conductance does not only necessarily increase with soil moisture but will also decrease when soil moisture is below a critical level (Baronti et al., 2014; Yang et al., 2021). Xiongwen (2002) suggested that the transpiration rate would not always decrease under moderate water stress, but drought-tolerant species should have a lower transpiration rate and lower water loss at low soil water content. When water is sufficient, the rate of transpiration influences the rate of soil water loss. High stomatal conductance is usually associated with rapid depletion of soil water and greater plant water deficit.
The most important quick response to drought stress and control of water loss in the desert environment is reducing the stomatal transpiration. Consequently, this can be considered an important survival mechanism. Stomatal conductance was the highest under no drought condition (100% ETp) and was the lowest under severe drought conditions (25% ETp). However, these three desert plant species demonstrated their ability, flexibility, and effectiveness in terms of regulating their stomatal conductance over water loss when exposed to moderate levels of drought stress (i.e., 75% and 50% ETp). It is likely that these species when exposed to moderate drought stress partially close their stomatal pores to increase the efficiency of water use and reduce the volume of water loss (Farooq et al., 2009; Arve et al., 2011). Stomatal conductance can also be viewed as a mechanism for coping with drought, along with several other mechanisms which work together, such as the transpiration rate reduction function and chemical signals from the plant stress hormone, abscisic acid (ABA) (Arve et al., 2011). Low conductance inhibits water loss and conserves water to avoid the effects of drought (Xiongwen, 2002). Knowing the species-specific water requirements of desert plants and how to decrease the adverse effects of drought stress by quick response to water deficit using the control of stomata mechanism, it would be possible to enhance the production protocol in terms of large-scale rehabilitation and restoration programs in degraded desert areas.
Growth Responses to Drought Stress
It is obvious that the exposure of these desert plant species to various irrigation deficit treatments in general negatively reduces the top growth and fresh and dry weight in terms of drought stress compared to there being no drought stress in almost all plant species. However, there is an exception in the case of 75% ETp, where the highest fresh biomass is recorded in all three plant species. Therefore, one of the findings of this project could be that 50 and 75% ETp irrigation treatments are optimal in terms of water requirement, whereby maximum production may be achieved (Figure 4). A further reduction of applied water is likely to reduce the fresh biomass. The top growth biomass of plant species reflects their ability to accumulate photosynthetic dry matter (Yang et al. 2021), but to variable degrees. The aboveground biomass differences were generally most noticeable at the final harvest. A reduction in the aboveground biomass among plant species is a normal response to drought stress (Kramer, 1995). Several authors have reported the reduction in leaf expansion and a decline in the net assimilation associated with drought stress (Sharp and Davies, 1985; Simane et al., 1993; Dias Filho, 1995; Dias Filho, 2000). The effect of water stress on the production of new leaves can influence cell expansion, resulting in smaller leaves, which in turn causes a reduction in photosynthetic capacity (Sharp and Davies, 1985; Kramer, 1995). The observation of this study demonstrated that different desert plant species responded differently, depending on their level of tolerance and avoidance of drought stress. All the plant species studied showed no considerable decline in the fresh and dry weight under two rates of moderate drought (75 and 50% ETp) (Figure 4). The shoot dry weight of C. ciliaris and L. scindicus was significantly (p < 0.001) reduced in the case of 50 and 25% ETp irrigation treatments, respectively, while P. divisum showed significant reduction in the shoot dry weight in the 25, 50, and 100% ETp irrigation treatments compared to that in the 75% ETp treatment. All plant species exhibited their capability to maintain top growth and photosynthesis despite the drought conditions (Dias Filho, 1995; Dias Filho, 2000). Furthermore, the precise reasons for P. divisum showing a significant difference in overall top growth and the full water treatment of the 100% ETp irrigation are unclear. However, the possible explanation may be that the 75% ETp treatment was the optimum water level for growth and development as the plant is adapted to dry desert conditions, suggesting that moderate drought is beneficial for plant adaptation and survival.
The formation of a strong root system is vital for the establishment, development, and productivity of desert plants. The root growth of all plant species showed significant statistical differences (p < 0.001), as was detected throughout the experiment. The root dry weight under 25, 50, and 75% ETp irrigation treatments was considerably higher in all plant species than those of the 100% ETp irrigation treatments (Figure 5), except in the case of C. ciliaris, where at 25% ETp, the root weight was insignificantly lower than at 100% ETp. In fact, water stress influenced the root weight of all the plant species examined in this experiment. The root growth dry weight of C. ciliaris and P. divisum was significantly increased in the 50 and 75% ETp irrigation treatments, while L. scindicus showed a significant increase in root dry weight in the 25 and 50% ETp irrigation treatments compared to that in the 100% ETp treatment. The increased root growth under various irrigation levels could be due to some stimulation of root growth due to moisture stress. Further investigation is needed to determine how the stimulation of root growth functioned in these species in drought stress environments.
Interestingly, the C. ciliaris and P. divisum plant species had greater root development under moderate drought conditions (50% ETp), as did L. scindicus at 25% ETp treatment throughout the experiment when compared to that under the 100% ETp treatment. Sharp and Davies (1985) reported that the rate of root development in the unexplored regions of the soil is sufficient to maintain an effective root system which can be extremely effective in terms of removing water from moist soil. It is likely that these three plant species developed more root systems under drier conditions as a result of water stress in order to improve their water absorption from deeper soil layers, resulting in a higher fresh biomass. This is also supported by Sharp and Davies, (1985), Dias Filho, (1995), Dias Filho, (2000) that a fast and vigorous root growth could help seedlings to compensate and compete for soil water use and to support the transpiration aboveground biomass (Simane et al., 1993). Numerous authors have reported an increase in the root-to-shoot ratio of plants exposed to drought stress Sharp and Davies, 1985; Simane et al., 1993; Dias Filho, (1995); and Dias Filho, (2000). Under limited moisture conditions, irrespective of the drought period, the biomass allocation to the roots was greater among all three plant species.
Nevertheless, plants growing in drought stress condition may have greater portioning into the root growth (Dias Filho, 1995; Dias Filho, 2000) in order to increase a seedling’s water absorption capability and its osmotic adjustment to improve photosynthesis and water uptake (Sharp and Davies, 1985). The increase in root growth and the greater root-to-shoot ratio are attributes associated with drought tolerance (Simane et al., 1993). In this study, all three plant species showed a shift in aboveground biomass allocation to the roots that may increase water uptake in drought conditions. Under drought stress conditions, a greater rooting depth and exploration of soil volume is commonly associated with higher biomass yields, greater drought tolerance, and enhanced plant water absorption. Tilman (1988) suggested that in nutrient-poor and water-limited environments, plants may increase root biomass allocation relative to shoot allocation.
Nutritive Values Vs. Drought Stress
Remarkable differences in crude protein percentages among the three species occurred under different irrigation treatments. Nonetheless, the crude protein percentage of all the desert plant species increases as drought stress increases (Madouh and Al-Sabbagh, 2021). Positive trends in crude protein concentration across irrigation levels were substantial for all three plant species with a decrease in the water supplied. With no exceptions, all three plants showed a significant increase in protein concentration under severe drought conditions (25% ETp). Jensen et al. (2010) reported a linear increase in crude protein concentration across five water levels, which was significant for all species supplied with decreasing amounts of water. These findings were consistent with the results in this study. The increase in nutritional value associated with drought stress is, in most cases, negated by the associated adverse tendency in terms of dry biomass yield with increased water stress (Jensen et al., 2010). It is obvious that the crude protein percentage of these desert plant species was positively affected by drought stress, indicating that the metabolic adjustments may have supported the adaptation to a severe natural environment (Xi et al., 2006; Yong et al., 2016; Luo et al., 2021). However, Lokko et al. (2007) reported that drought stress activates several responses, including variations in gene expression, productions of specific proteins, and high levels of metabolites. It is obvious from the present investigation that desert plants implement multiple adaptive and tolerance mechanisms in response to drought stress in the form of partial stomata closure, alterations in biomass allocation, and increasing protein content to maintain their metabolites in such a way so as to resist and avoid drought stress.
However, no consistent trends in fat concentration across various irrigation levels were detected among the plant species. The amount of irrigation did not affect the fat concentration, suggesting that drought stress has no influence on fat percentages which remained relatively stable under severe drought conditions (25% ETp). The unaffected and steady fat concentration of these desert plants under various reduced watering levels appears to be among several features indicative of a so far unknown adaptation function to drought stress at the cellular level (Gigon et al., 2004).
The ash concentration commonly represents the inorganic residue with minerals as its primary content (Ismail, 2017). The higher level of ash content could be attributed to the high mineral content of the leaves (Purwanti, et al., 2019; Osagie et al., 2021). The percentage of ash concentration measured under various irrigation levels decreased considerably with increased drought for all the desert plant species. However, there was a general increase in ash concentration of all the plants as irrigation amounts increased. This indicates that drought stress negatively affected the ash concentration (p < 0.001), which suggests lower mineral content in these plants, particularly in severe drought conditions (25% ETp). Under the moderate levels of drought stress, (75 and 50% ETp), all plants maintained a relatively stable ash content compared to that of well-watered plants. It is likely that the ash concentration yield of these desert plants was highly sensitive to drought stress, which may be viewed as another survival response favoring water absorption over nutrient uptake. In general, the nutritional value of the desert plants investigated under various water deficit conditions showed an increased value in terms of protein content as drought stress increased. In contrast, no significant differences in terms of fat content were detected under all the irrigation conditions, while the ash content was significantly influenced by severe drought stress.
Conclusion
From the present study, it is concluded that the investigated native desert plants are capable of enduring drought stress by adapting various physiological processes. The native desert plants moderated their responses by regulating stomata closure to control transpiration and CO2 uptake. This process increased the allocation of photosynthetic assimilation to roots that promoted greater root development and a higher root–shoot ratio by regulating the metabolism and accumulation of primary (proteins and lipids) and secondary metabolites (ash and phenolic compounds) in various plant parts to endure drought stress. This was substantiated by the increased crude protein in response to drought stress in all the tested desert plant species. Furthermore, the lower ash contents are indicative of sensitivity to drought stress. This suggests that these plants are capable of maintaining water absorption at the expense of nutrient uptake. The stimulation of these multiple adaptation responses allows them to conserve water and avoid the drought events for their survival and helps in stabilizing the biodiversity to sustain the desert ecosystem. The species-specific adaptation mechanism provides an excellent opportunity to not only restore normal ecological functions and encourage biodiversity conservation but also play a part in large-scale use of native plants for the restoration of degraded habitats, for rangeland management in water-scarce environments, and/or even these habitats can be used as pastures.
In the present study, irrigating the plants at 100% ETp (full irrigation) reduced the water use efficiency, whereas deficit irrigation at 75 and 50% ETp increased the water productivity (biomass yield per unit of water used). Deficit irrigations have been shown to induce drought tolerance by promoting the production and accumulation of certain metabolites in plant tissues. Such metabolites allow the plants to avoid or escape stress conditions. The results from the present study have opened interesting perspectives to better understand the links between desert habitat management, native desert plant production, and establishment in the field. This concludes that it is now possible to reduce water supply to native plants without affecting their biomass and increase water productivity. Furthermore, the tested desert plant species demonstrated their ability, flexibility, and effectiveness in terms of regulating their physiological responses to reduce water loss when exposed to moderate levels of drought stress, that is, 75% and 50% ETp. Therefore, reduced the watering (75 and 50% ETp) regime may be considered for native plant production and desert rehabilitation, especially for arid and semi-arid regions of low precipitation (< 250 mm).
Data Availability Statement
The original contributions presented in the study are included in the article/Supplementary Material, further inquiries can be directed to the corresponding author.
Author Contributions
TM administrated, designed, and acquired funding for the project. TM performed the experiments, analyzed the data, and wrote the manuscript.
Funding
The Kuwait Foundation for the Advancement of Sciences (KFAS) supported the project financially under project code: (2008-1207-08).
Conflict of Interest
The author declares that the research was conducted in the absence of any commercial or financial relationships that could be construed as a potential conflict of interest.
Publisher’s Note
All claims expressed in this article are solely those of the authors and do not necessarily represent those of their affiliated organizations, or those of the publisher, the editors, and the reviewers. Any product that may be evaluated in this article, or claim that may be made by its manufacturer, is not guaranteed or endorsed by the publisher.
Acknowledgments
The author wishes to acknowledge financial support of the Kuwait Foundation for the Advancement of Sciences (KFAS) and KISR management for providing facilities and for their kind support. The author would like to thank Dr. Shabbir A. Shahid for his technical review and valuable comments with regard to improving the manuscript.
References
Aasamaa, K., and Sõber, A. (2011). Stomatal Sensitivities to Changes in Leaf Water Potential, Air Humidity, CO2 Concentration and Light Intensity, and the Effect of Abscisic Acid on the Sensitivities in Six Temperate Deciduous Tree Species. Environ. Exp. Bot. 71, 72–78. doi:10.1016/j.envexpbot.2010.10.013
Aguirre, L., and Johnson, D. A. (1991). Root Morphological Development in Relation to Shoot Growth in Seedlings of Four Range Grasses. J. Range Manag. 44, 341–346. doi:10.2307/4002396
AOAC International (1995). Official Methods of Analysis of AOAC International. 16th ed., 2. Arlington, VA, USA: Association of Analytical Communities.
Atteya, A. M. (2003). Alteration of Water Relations and Yield of Corn Genotypes in Response to Drought Stress. Bulg. J. Plant Physiol. 29, 63–76.
Baronti, S., Vaccari, F. P., Miglietta, F., Calzolari, C., Lugato, E., Orlandini, S., et al. (2014). Impact of Biochar Application on Plant Water Relations in Vitis vinifera (L.). Eur. J. Agron. 53, 38–44. doi:10.1016/j.eja.2013.11.003
Breckle, S. W. (2002). “Salinity, Halophytes and Salt Affected Natural Ecosystems,” in Salinity: Environment - Plants - Molecules. Editors A. Läuchli, and U. Lüttge (Dordrecht: Springer). doi:10.1007/0-306-48155-3_3
Briedé, J.-W., and McKell, C. M. (1992). Germination of Seven Perennial Arid Land Species, Subjected to Soil Moisture Stress. J. Arid Environments 23, 263–270. doi:10.1016/s0140-1963(18)30515-9
Burghardt, M., and Riederer, M. (2003). Ecophysiological Relevance of Cuticular Transpiration of Deciduous and evergreen Plants in Relation to Stomatal Closure and Leaf Water Potential. J. Exp. Bot. 54, 1941–1949. doi:10.1093/jxb/erg195
Chaves, M. M., Pereira, J. S., Maroco, J., Rodrigues, M. L., Ricardo, C. P., Osório, M. L., et al. (2002). How Plants Cope with Water Stress in the Field. Photosynthesis and Growth. Ann. Bot. 89 (7), 907–916. doi:10.1093/aob/mcf10510
Chaves, M. M., Maroco, J. P., and Pereira, J. S. (2003). Understanding Plant Responses to Drought–From Genes to the Whole Plant. Funct. Plant Biol. 30, 239–264. doi:10.1071/FP02076
Davies, W. J., and Zhang, J. (1991). Root Signals and the Regulation of Growth and Development of Plants in Drying Soil. Annu. Rev. Plant Physiol. Plant Mol. Biol. 42, 55–76. doi:10.1146/annurev.pp.42.060191.000415
Dias Filho, M. B. (1995). Root and Shoot Growth in Response to Soil Drying in Seedlings of Four Amazonian Weedy Species. Revista Brasileira de Fisiologia Vegetal 7 (1), 53–59.
Dias-Filho, M. B. (2000). Growth and Biomass Allocation of the C4 Grasses Brachiaria Brizantha and B. Humidicola under Shade. Pesq. Agropec. Bras. 35 (12), 2335–2341. doi:10.1590/s0100-204x2000001200003
Ehleringer, J. R., and Cook, C. S. (1984). Photosynthesis in Encelia Farinosa Gray in Response to Decreasing Leaf Water Potential. Plant Physiol. 75 (3), 688–693. doi:10.1104/pp.75.3.688
Farooq, M., Wahid, A., Kobayashi, N., Fujita, D., and Basra, S. M. A. (2009). “Plant Drought Stress: Effects, Mechanisms and Management,” in Sustainable Agriculture. Editors E. Lichtfouse, M. Navarrete, P. Debaeke, S. Véronique, and C. Alberola (Dordrecht: Springer). doi:10.1007/978-90-481-2666-8_12
Gigon, A., Matos, A.-R., Laffray, D., Zuily-Fodil, Y., and Pham-Thi, A.-T. (2004). Effect of Drought Stress on Lipid Metabolism in the Leaves of Arabidopsis thaliana (Ecotype Columbia). Ann. Bot. 94 (3), 345–351. doi:10.1093/aob/mch150
Gollan, T., Richards, R., Rawson, H., Passioura, J., Johnson, D., and Munns, R. (1986). Soil Water Status Affects the Stomata1. Funct. Plant Biol. 13, 459. doi:10.1071/pp9860459
Gowing, D. J. G., Davies, W. J., and Jones, H. G. (1990). A Positive Root-Sourced Signal as an Indicator of Soil Drying in Apple,Malus X domesticaBorkh. J. Exp. Bot. 41 (12), 1535–1540. doi:10.1093/jxb/41.12.1535
Griffin, J. J., Ranney, T. G., and Pharr, D. M. (2004). Heat and Drought Influence Photosynthesis, Water Relations, and Soluble Carbohydrates of Two Ecotypes of Redbud (Cercis canadensis). J. Am. Soc. Hortic. Sci. 129, 497–502. doi:10.21273/jashs.129.4.0497
Han, W., Tang, L., Chen, Y., and Fang, J. (2013). Relationship between the Relative Limitation and Resorption Efficiency of Nitrogen vs Phosphorus in Woody Plants. PLoS ONE 8 (12), e83366. doi:10.1371/journal.pone.0083366
Hassanyar, A. S., and Wilson, A. M. (1978). Drought Tolerance of Seminal Lateral Root Apices in Crested Wheatgrass and Russian Wildrye. J. Range Manag. 31, 254–258. doi:10.2307/3897594
Hu, Y., and Schmidhalter, U. (2005). Drought and Salinity: A Comparison of Their Effects on mineral Nutrition of Plants. Z. Pflanzenernähr. Bodenk 168, 541–549. doi:10.1002/jpln.200420516
Ismail, B. P. (2017). “Ash Content Determination,” in Food Analysis Laboratory Manual. Food Science Text Series (Cham: Springer). doi:10.1007/978-3-319-44127-6_11
Jensen, K. B., Waldron, B. L., Peel, M. D., and Robins, J. G. (2010). Nutritive Value of Herbage of Five Semi-irrigated Pasture Species across an Irrigation Gradient. Grass Forage Sci. 65, 92–101. doi:10.1111/j.1365-2494.2009.00723.x
Johnson, D. A., and Aguirre, L. (1991). Effect of Water on Morphological Development in Seedlings of Three Range Grasses: Root Branching Patterns. J. Range Manag. 44, 355–360. doi:10.2307/4002398
Jones, H. (1998). Stomatal Control of Photosynthesis and Transpiration. J. Exp. Bot. 49, 387–398. doi:10.1093/jexbot/49.suppl_1.387
Khalil, A. A. M., and Grace, J. (1992). Acclimation to Drought in Acer Pseudoplatanus L. (Sycamore) Seedlings. J. Exp. Bot. 43 (257), 1591–1602. doi:10.1093/jxb/43.12.1591
Killingbeck, K. T. (1993). Nutrient Resorption in Desert Shrubs. Revista Chilena de Historia Nat. 66, 345–355.
KISR (1999). Soil Survey for the State of Kuwait. Reconnaissance Survey: Volume II. Kuwait: Kuwait Institute for Scientific Research.
Kramer, K. (1995). Modelling Comparison to Evaluate the Importance of Phenology for the Effects of Climate Change on Growth of Temperate-Zone Deciduous Trees. Clim. Res. 5, 119–130. doi:10.3354/cr005119
Le, A., S, T., Je, O., and Kk, T. (2011). “Stomatal Responses to Drought Stress and Air Humidity,” in Abiotic Stress in Plants - Mechanisms and Adaptations. Editors S. Arun, and B. Venkateswarlu (London: IntechOpen). doi:10.5772/24661
Le Houerou, H. N. (1996). Drought Tolerant and Water Efficient Trees and Shrubs for Rehabilitation of Tropical and Subtropical Arid Lands of Africa and Asia. Land Husbandry 1, 43–64.
Liang, Z., Zhang, F., Shao, M., and Zhang, J. (2002). The Relations of Stomatal Conductance, Water Consumption Growth Rate to Leaf Water Potential during Soil Drying and Re-watering Cycle of Wheat (Triticum aestivum). Bot. J. Acad. Sci. 43, 187–192. doi:10.7016/BBAS.200207.0187
Lokko, Y., Anderson, J. V., Rudd, S., Raji, A., Horvath, D., Mikel, M. A., et al. (2007). Characterization of an 18,166 EST Dataset for Cassava (Manihot Esculenta Crantz) Enriched for Drought-Responsive Genes. Plant Cel Rep 26, 1605–1618. doi:10.1007/s00299-007-0378-8
Lü, X.-T., Freschet, G. T., Flynn, D. F. B., and Han, X.-G. (2012). Plasticity in Leaf and Stem Nutrient Resorption Proficiency Potentially Reinforces Plant-Soil Feedbacks and Microscale Heterogeneity in a Semi-arid Grassland. J. Ecol. 100, 144–150. doi:10.1111/j.1365-2745.2011.01881.x
Luo, Y., Chen, Y., Peng, Q., Li, K., Mohammat, A., and Han, W. (2021). Nitrogen and Phosphorus Resorption of Desert Plants with Various Degree of Propensity to Salt in Response to Drought and saline Stress. Ecol. Indicators 125, 107488. doi:10.1016/j.ecolind.2021.107488
Madouh, Tareq A., and Al-Sabbagh, Tareq A. Nutritional Quality and Adaptation of Several Native Plant Species of Kuwait to the Farming System As Potential Livestock Feed. Int J Envi & Eart Scie 2021, 2: 1–8. doi:10.33140/jahr.04.01.01
Madouh, T. A. (2013). Development and Utilization of Desert Forages for Sustainable Livestock Production under kuwait Conditions (FA078C). Kuwait: Kuwait Institute for Scientific Research. Final Report, KISR 11626.
Madouh, T. A. (2005). Effect of Drought on Growth and Development of Perennial Desert Species for Rehabilitation of Degraded Areas of Kuwait (United Kingdom: Reading University). [Ph.D. Thesis].
Maherali, H., Johnson, H. B., and Jackson, R. B. (2003). Stomatal Sensitivity to Vapour Pressure Difference over a Subambient to Elevated CO2 Gradient in a C3/C4 Grassland. Plant Cel Environ 26, 1297–1306. doi:10.1046/j.1365-3040.2003.01054.x
Marchin, R., Zeng, H., and Hoffmann, W. (2010). Drought-deciduous Behavior Reduces Nutrient Losses from Temperate Deciduous Trees under Severe Drought. Oecologia 163, 845–854. doi:10.1007/s00442-010-1614-4
Misak, R. F., Al-Awadhi, J. M., Omar, S. A., and Shahid, S. A. (2002). Soil Degradation in Kabd Area, Southwestern Kuwait City. Land Degrad. Dev. 13, 403–415. doi:10.1002/ldr.522
Nilsen, E. T., Sharifi, M. R., and Rundel, P. W. (1984). Comparative Water Relations of Phreatophytes in the Sonoran Desert of California. Ecology 65, 767–778. doi:10.2307/1938049
North, G. B., and Nobel, P. S. (1998). Water Uptake and Structural Plasticity along Roots of a Desert Succulent during Prolonged Drought. Plant Cel Environ. 21, 705±713. doi:10.1046/j.1365-3040.1998.00317.x
Omar, S. A. S. (1991). Dynamics of Range Plants Following 10 Years of protection in Arid Rangelands of Kuwait. J. Arid Environments 21, 99–111. doi:10.1016/s0140-1963(18)30732-8
Omar, S. A. S., and Shahid, S. A. (2013). “Reconnaissance Soil Survey for the State of Kuwait,” in Developments in Soil Classification, Land Use Planning and Policy Implications: Innovative Thinking of Soil Inventory for Land Use Planning and Management of Land Resources. Editors S. A. Shahid, F. K. Taha, and M. A. Abdelfattah (Dordrecht Heidelberg New York London: Springer), 85–107. doi:10.1007/978-94-007-5332-7_3
Omar, S. A. (2000). Vegetation of Kuwait: A Comprehensive Illustrative Guide to the Flora and Ecology of the Desert of Kuwait. Kuwait: Kuwait institute for Scientific Research.
Osagie, M. A., Orobator, N. O., Orobator, S. E., and Azeke, A. M. (2021). Effects of Moringa Oleifera Leaf Powder on Some Chemical, Phytochemical and Antioxidant Properties of Bread: Potential Control for NCDs. Delhi: Akinik Publications. doi:10.22271/phyto.2021.v10.i3a.14069
Piatt, J. R. (1976). Effects of Water Stress and Temperature on Germination of True Mountainmahogany. J. Range Manag. 29, 138–140. doi:10.2307/3897411
Purwanti, E., Prihanta, W., and Fauzi, A. (2019). “Nutritional Content Characteristics of Dolichos Lablab L. Accessions in Effort to Investigate Functional Food Source,” in [Conference Proceedings] 6th International Conference on Community Development (ICCD) (Paris: Atlantis Press). doi:10.2991/iccd-19.2019.45
Shahid, S. A., Omar, S. A. S., Jamal, M. E., Shihab, A., and Burezq, H. (2004). Soil Survey for Farm Planning in Northern Kuwait. Kuwait J. Sci. Eng. 31 (1), 43–57.
Sharp, R. E., and Davies, W. J. (1985). Root Growth and Water Uptake by maize Plants in Drying Soil. J. Exp. Bot. 36, 1441–1456. doi:10.1093/jxb/36.9.1441
Simane, B., Struik, P. C., Nachit, M. M., and Peacock, J. M. (1993). Ontogenetic Analysis of Yield Components and Yield Stability of Durum Wheat in Water-Limited Environments. Euphytica 71-71, 211–219. doi:10.1007/bf00040410
Soil Survey Staff (2014b). Kellogg Soil Survey Laboratory Methods Manual. Lincoln Nebraska: U.S. Department of Agriculture, Natural Resources Conservation Service. Soil Survey Investigations Report No. 42, Version 5.0. R. Burt and Soil Survey Staff.
Soil Survey Staff (2014a). Keys to Soil Taxonomy. 12th Edition. Washington, DC: US Department of Agriculture, Natural Resources Conservation Service, US Government Printing Office.
Tilman, D. (1988). “Plant Strategies and the Dynamics and Structure of Plant Communities,” in Monographs in Population Biology (Princeton,NJ: Princeton University Press), 26, 360.
Vilagrosa, A., Cortina, J., Gil-Pelegrin, E., and Bellot, J. (2003). Suitability of Drought-Preconditioning Techniques in Mediterranean Climate. Restor Ecol. 11, 208–216. doi:10.1046/j.1526-100x.2003.00172.x
Wang, L., Wang, L., He, W., An, L., and Xu, S. (2017). Nutrient Resorption or Accumulation of Desert Plants with Contrasting Sodium Regulation Strategies. Sci. Rep. 7 (1), 17035. doi:10.1038/s41598-017-17368-0
Xiongwen, C. (2002). Change of Water Vapor Exchange Properties of 3 Tree Species under Drought. J. Arid Environments 51, 423–435. doi:10.1006/jare.2001.0964
Yang, Y., Wu, J., Du, Y., Gao, C., Pan, X., Tang, D. W. S., et al. (2021). Short- and Long-Term Straw Mulching and Subsoiling Affect Soil Water, Photosynthesis, and Water Use of Wheat and maize. Front.Agron. 3, 63. doi:10.3389/fagro.2021.708075
Yong, Y. H., Zhang, X., Wang, S. M., and Wu, L. (2016). Salt Accumulation in Vegetative Organs and Ecological Stoichiometry Characteristics in Typical Halophytes in Xinjiang, China. Chin. J. Plant Ecol. 40, 1267–1275. doi:10.17521/cjpe.2016.0146
Zaman, S. (1997). Effects of Rainfall and Grazing on Vegetation Yield and Cover of Two Arid Rangelands in Kuwait. Envir. Conserv. 24, 344–350. doi:10.1017/s0376892997000453
Keywords: drought stress, nutritive value, desert biodiversity, stomatal conductance, water use efficiency, eco-physiological responses, restoration with desert restoration, desert plants
Citation: Madouh TA (2022) Eco-Physiological Responses of Native Desert Plant Species to Drought and Nutritional Levels: Case of Kuwait. Front. Environ. Sci. 10:785517. doi: 10.3389/fenvs.2022.785517
Received: 29 September 2021; Accepted: 08 March 2022;
Published: 14 April 2022.
Reviewed by:
Xiaodong Gao, Northwest A&F University, ChinaRaafat Misak, Desert Research Center, Egypt
Edited by:
Rasheed Adeleke, North-West University, South AfricaCopyright © 2022 Madouh. This is an open-access article distributed under the terms of the Creative Commons Attribution License (CC BY). The use, distribution or reproduction in other forums is permitted, provided the original author(s) and the copyright owner(s) are credited and that the original publication in this journal is cited, in accordance with accepted academic practice. No use, distribution or reproduction is permitted which does not comply with these terms.
*Correspondence: Tareq A. Madouh, dG1hZG91aEBraXNyLmVkdS5rdw==