- 1Southern California Coastal Water Research Project, Costa Mesa, CA, United States
- 2Geosyntec Consultants, Inc., Portland, OR, United States
- 3Orange County Public Works, Orange, CA, United States
- 4Center for Watershed Sciences, University of California, Davis, Davis, CA, United States
Flow alteration is a pervasive issue across highly urbanized watersheds that can impact the physical and biological condition of streams. In highly altered systems, flows may support novel ecosystems that may not have been found under natural conditions and reference-based environmental flow targets may not be relevant. Moreover, stream impairments such as altered channel morphology may make reference-based environmental flow targets less effective in supporting ecosystem functions. Here, we develop an approach for determining ecological flow needs in highly modified systems to support existing ecological uses utilizing the California Environmental Flows Framework (CEFF). CEFF was established to provide guidance on developing environmental flow recommendations across California’s diverse physical landscape and broad array of management contexts. This paper illustrates the application of CEFF in informing ecologically-based flow restoration in a highly altered region of South Orange County, California. The steps of CEFF were implemented including a stakeholder process to establish goals and provide input throughout the project; identifying the natural ranges of functional flow metrics, or distinct components of the natural flow regime that support ecosystem functions; refining ecological flow needs to account for altered channel morphology and the life history needs of riparian and fish species; and assessing flow alteration to inform management strategies. Key considerations and lessons learned are discussed in the context of developing ecological flow needs in highly altered systems including when non-flow related management actions (i.e., channel rehabilitation) are necessary to achieve ecological goals.
1 Introduction
Flow alteration is a pervasive issue across highly urbanized watersheds that can impact the physical and biological condition of streams (Poff and Zimmerman, 2010). However, addressing flow alteration and determining the ecological flows needed to support ecosystem functions can be a challenge in systems where alteration is widespread. Traditional reference-based approaches, such as a percentage of unimpaired flow (Richter et al., 2011), the Tennant method using a percentage of mean annual unimpaired flow (Tennant, 1976), or the Tessman method considering monthly unimpaired flow (Tessman, 1980), can be implemented to determine minimum environmental flows that serve as a management target. However, reference-based environmental flow targets may not be relevant nor realistic in systems that are far from reference. In highly altered river systems, such as streams with augmented flows from urban or wastewater discharges, altered flow regimes could support novel ecosystems and species, such as birds that did not occur under “natural” conditions (Luthy et al., 2015; Wolfand et al., 2021). In such systems, ecological flow needs, or quantifiable flow metrics that describe ranges of flows that must be maintained to support ecosystem functions, should be tailored to a suite of species of management concern that are representative of communities, rather than mimicking the entire natural flow regime (Tonkin et al., 2021).
Certain stream impairments, such as physical habitat alteration, water quality impairment, and biological interactions (i.e., invasive species), may alter the relationship between flow and ecology making the natural ranges of flow metrics less effective in supporting ecosystem functions. For example, natural flood flows may not inundate floodplains if the channel is deeply incised (Edwards et al., 2016), and thus the functions associated with floodplain inundation, such as riparian seed dispersal, fish breeding, and sediment deposition (Opperman et al., 2010; Yarnell et al., 2015), may not be supported. Similarly, high stream temperatures resulting from riparian vegetation loss may limit the functionality of summer baseflows for fish rearing if the temperatures exceed suitability thresholds (Cross et al., 2013; Kristensen et al., 2015; Abdi et al., 2021). Invasive species can further alter ecosystem functions, as shown for example by studies on the impacts of invasive bullfrogs in streams. Bullfrog tadpoles can outcompete native amphibian tadpoles by consuming large amounts of benthic algae and altering the dynamics of primary productivity in streams (Kupferberg, 1997), while adult bullfrogs increase the prevalence of disease that can decimate sensitive native amphibians (Adams et al., 2017). Ecological flow needs in highly altered systems should consider not only stream impairments, but also the needs of multiple species.
Holistic approaches have been developed that go beyond the needs of single species and consider the role of flow variability on ecosystem processes. For example, the Ecological Limits of Hydrologic Alteration (ELOHA) framework is an approach to identify ecological flow needs using relationships between flow and ecological outcomes (Poff et al., 2010). However, developing such relationships requires high data density on biological conditions as well as corresponding hydrologic data, which are seldom available at the same location. Additionally, the ELOHA framework does not consider mediating factors that can alter flow-ecology relationships, such as altered channel morphology or water quality impairments. The functional flows approach is a holistic approach that aims to manage and restore discrete components of the hydrograph that support key ecosystem functions and drive geomorphic and ecological processes (Yarnell et al., 2015). The functional flows approach presumes that restoring the natural ranges of functional flows will ultimately support ecosystem processes. However, the natural ranges for certain flow components may need to be adjusted to consider stream impairments and the needs of novel species and habitats. The development of designer flows that support novel ecosystems while balancing the needs of society are especially critical in urban areas (Acreman et al., 2014a; Tonkin et al., 2021).
The California Environmental Flows Framework (CEFF) uses a functional flows approach to develop ecological flow needs and environmental flow recommendations that balance water needs of multiple uses (Stein et al., 2021b). This approach can accommodate highly modified systems where the water demand for human uses may be high and mimicking the entire natural flow regime may be unrealistic. CEFF consists of three key sections that focuses on identifying the natural range of functional flows as a starting point (Section A; Grantham et al., 2022), developing refined ecological flow needs that consider physical and biological constraints (Section B), and producing a final set of balanced environmental flow recommendations that consider current hydrologic alteration and multiple uses of water (Section C). CEFF was developed to be applied across California’s diverse physical landscape and has the flexibility to be applied to a wide range of management challenges. However, there is a need to apply the conceptual approach of CEFF in real-world applications across a diversity of stream types, including groundwater-influenced systems (Yarnell et al., 2022) and highly urbanized streams, to inform future implementation of the framework.
This paper illustrates the utility of CEFF in developing ecological flow needs that consider altered physical habitat in a highly urbanized region of South Orange County (OC), California. This study provides a unique opportunity to pilot CEFF in a region where flow alteration is the highest priority concern, augmented urban runoff may be supporting novel ecosystems, and stormwater permits require that all nuisance dry-weather discharges into streams be eliminated under the Clean Water Act. Some considerations in Section C, including an alteration assessment and evaluation of an alternative management scenario, were conducted, but final environmental flow recommendations that consider human water uses were not produced. We explore how non-flow related management actions, such as instream channel rehabilitation of a widened reach, can influence ecological flow needs and produce more achievable flow targets.
The main objectives of this study were to:
1) Provide a demonstration of CEFF in an altered system.
2) Develop ecological flow needs that consider altered physical habitat and are supportive of key species of management concern.
3) Evaluate hydrologic alteration to inform management strategies.
4) Provide an example of how changes to channel form can help to achieve ecological flow needs.
Although CEFF was established for California, the approach developed in this study can be utilized in other places with highly modified systems. This study lays out a functional flows approach that uses natural flows as a starting point, identifies if the natural range of flows need to be modified based on stream impairments, and develops a set of holistic ecological flow needs that consider the natural variability of functional flows across all seasonal components of the hydrograph with special consideration to the landscape and the species in it.
2 Study Area
This study focuses on the San Juan Hydrologic Unit in South OC, California which includes several major streams including San Juan Creek, Trabuco Creek, Oso Creek, Aliso Creek, among others (Figure 1). Most of the upper tributaries of the major watersheds are undeveloped, while the lower portions of the watersheds are highly urbanized. The major land uses in the region include developed pervious land, single- and multi-family residential homes, transportation, and open space with low vegetation. The region is characterized by a Mediterranean climate with wet winters and dry summers. Long-term mean annual precipitation in the study region ranges from 722 to 299 mm (PRISM Climate Group, 2016). High priority areas where flow alteration may be associated with a biological decline were identified based on statewide bioassessment indices for macroinvertebrates and benthic algae (Irving et al., 2022). This paper focuses on a high priority area in lower Aliso Creek (see starred location in Figure 1) to illustrate the process and application of CEFF to develop ecological flow needs. However, the methods used in this study were chosen to allow for the evaluation to be applied at the regional scale, across a multitude of high priority stream reaches. The Lower Aliso study reach was selected for this study because it is subject to a potential decline in dry-weather flows from upstream outfall discharge diversions, has experienced urban-induced channel erosion, and is a soft-bottom reach of habitat importance for riparian and aquatic communities.
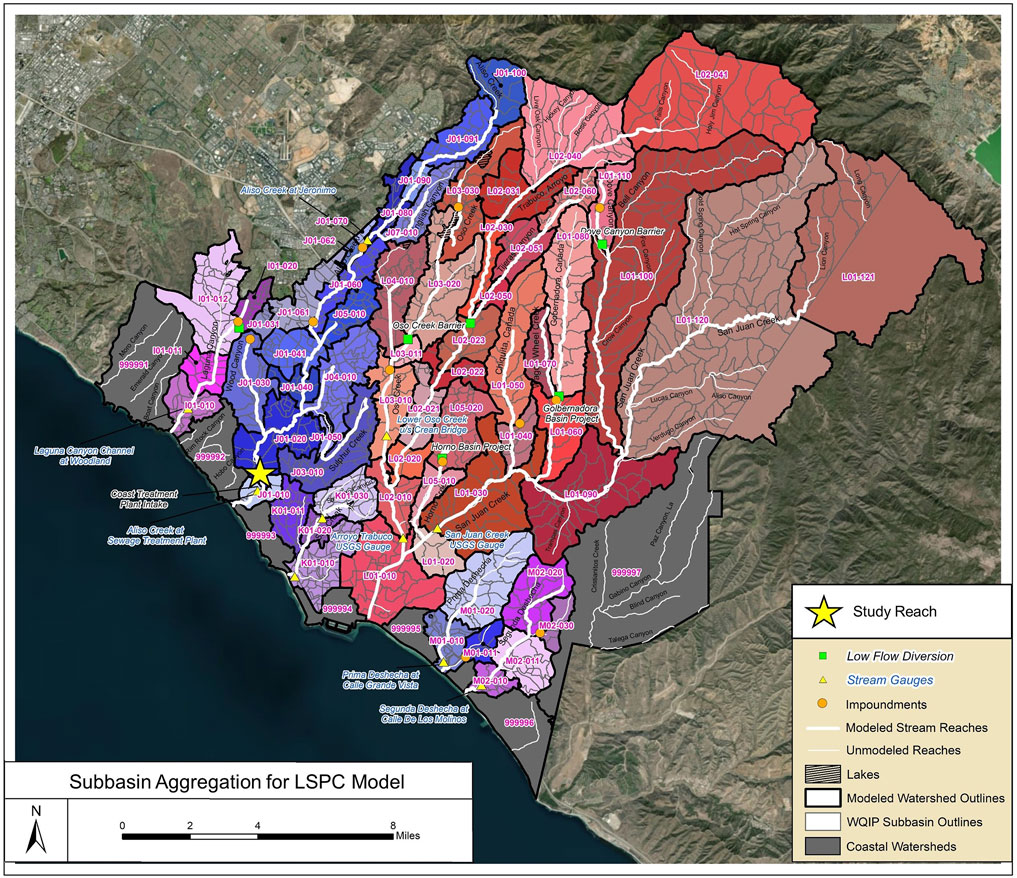
FIGURE 1. South OC WMA with modeled subbasins. This study focuses on the high priority reach of Lower Aliso Creek (star).
Flow alteration and stream erosion are the highest priority water quality conditions identified for the region (County of Orange, 2021). Flow alteration is a pervasive issue across South OC due to the effects of historical farming and ranching and more current rapid urbanization over the past 50–70 years. Flashier hydrology has led to channel erosion issues (Hawley and Bledsoe, 2011; Hawley and Bledsoe, 2013), and many streams have shifted from a historically intermittent-ephemeral system to a more perennial system due to augmented baseflows from irrigation overspray. In some areas, these augmented flows now support sensitive species and habitats that were not historically present. To promote streamflow enhancement and habitat restoration, key implementation strategies have been identified through the South OC Watershed Management Area (WMA) Water Quality Improvement Plan including management of unnatural flows and restoration of 23,000 lineal feet (4.35 mi) of degraded stream habitat1. However, reduction of in-stream flows through flow management actions, drought, and water conservation, pose a potential threat to novel habitat and sensitive species that currently depend on these “non-reference” flows. For successful implementation, flow enhancement projects must consider how these factors may impact the flows needed to support key ecological functions.
Despite the widespread hydrologic alteration, streams in South OC currently support a combination of willow and riparian scrub communities, as well as federally listed bird species, such as the least Bell’s vireo (Vireo bellii pusillus), and fish species of special concern, such as the arroyo chub (Gila orcuttii). Although arroyo chub is not currently observed in Aliso Creek, there is a desire to rehabilitate stream conditions to be more conducive for sensitive aquatic species in the future. Additionally, the long-term viability of these species and habitats is uncertain in light of future changes in flow and channel conditions.
3 Methods
3.1 Stakeholder Engagement
A critical component to implementing CEFF is ongoing stakeholder engagement that seeks to integrate stakeholder values and local knowledge into the scientific process. We have collaborated closely with the County of Orange and all member agencies on our technical and stakeholder advisory group, which included federal and state resource and regulatory agencies, local water districts, non-governmental and private organizations, local watershed groups, and academic researchers. A total of 9 stakeholder meetings were held over the course of 2 years, where the group agreed upon management goals and project scope and provided valuable input on the overall technical approach. The overarching ecological management goals for this study, identified through the stakeholder process, were to improve stream flow conditions to benefit overall stream ecosystem health and to ultimately maintain or provide suitable habitat conditions for indicator species of management concern, willow and arroyo chub, which are representative of riparian and aquatic habitats. We also determined ecosystem functions for each functional flow component that should be supported by ecological flows to satisfy ecological management goals in the study area. Under the functional flows approach, all functional flow components should be maintained to achieve ecological management objectives. Therefore, at least one ecosystem function for each of the five functional flow components that are relevant to ecological management goals were identified.
3.2 Quantifying Functional Flows
3.2.1 Hydrologic Model
A continuous simulation Loading Simulation Program in C++ (LSPC) model was developed and calibrated to characterize current functional flow conditions across the South OC WMA (Figure 1). This model was then applied to estimate reference conditions. We did not use the predicted natural range of functional flow metrics produced by Grantham et al. (this issue) in this study because there was a lack of local reference gages used in the statewide models.
Model Forcing and Parameterization
Present-day conditions were simulated for 1993–2019 using LSPC. The model was forced by 16 continuous, hourly precipitation records and 2 continuous, hourly evapotranspiration records that spanned 1989–2019. The model was run for the entire span of these input time series, but the first 4 years of results were discarded to allow streamflows and aquifer storage to equilibrate from their initial conditions. Thus, the simulation period was 1993–2019. The study region was discretized into 73 modeled subbasins based on major tributary confluences, stream gage locations, channel type, bodies of water, low flow diversion locations, impoundments, and points of ecological interest. Land use for each subbasin was assigned in LSPC as “hydrologic response units” (HRUs), which were unique combinations of land use (County of Orange dataset), soil type (from National Resource Conservation Service (NRCS)), land slope (from County of Orange digital elevation model), and imperviousness (from the National Land Cover Database (NLCD) 2019)2. Characteristics of stream reaches were assigned using LiDAR datasets of channel morphology3, and major impoundments were included in the model after examination of available as-built or design drawings. Flow diversions were modeled based on data provided by local water utilities. Outdoor water usage (i.e., landscape irrigation) was estimated based on water usage and sanitary return flow data from 2015–2019, which accounts for current levels of water efficiency measures and includes the tail end of a 5-years drought which was from 2012–2016 (Lund et al., 2018).
Model Calibration
Stream gauging records were available during substantial portions of the simulated period for upper Aliso Creek, lower Aliso Creek, and lower Oso Creek from gages maintained by OC Public Works (OCPW), and lower Trabuco Creek (USGS Gage ID: 11047000). Additionally, dry-weather discharge measurements were measured by OCPW staff at 8 locations throughout the WMA on a monthly basis. The Lower Aliso and Trabuco Creek gages were used as the two primary stations for high resolution (hourly) calibration. Monthly dry weather flow measurements were used to support dry weather calibration. The period 2015–2019 was used as a calibration period for all reaches because it includes dry weather runoff reductions achieved through municipal water conservation efforts.
A good calibration during spring recession and low-flow periods was critical as these were key functional flow components. Additionally, understanding the source of dry season streamflow and matching this in the calibration was important to assess how conditions may change in a future with less outdoor water use and potentially less rainfall. To support this aspect of the calibration, a separate investigation was conducted where stable water isotope samples were collected from stream locations and known water sources (Lai, 2020b). In-stream stable water isotope data were compared to rainfall, groundwater, and imported potable water end-member samples to understand the source of streamflow (e.g., rainwater or imported water used in irrigation) following the methodology described in Lai (2020a) and Wallace et al. (2021). Lai (2020b) revealed that groundwater comprises a significant portion (65–80%) of dry weather flow in most stream reaches, and so model parameterizations of groundwater infiltration and storage were adjusted to match these observations from field samples. The model calibration parameters and targets at the lower Aliso Creek gage were: streamflow composition of 25–35% imported water in July based on isotope data described above, Nash-Sutcliffe Efficiency (NSE) and logarithmic NSE greater than 0.5, root-mean-squared error less than or equal to 0.7, and percent bias plus or minus 25% (Moriasi et al., 2007). Different streamflow composition targets were established for different stations based on the results of the water isotope investigation.
Reference Model Scenario
A reference condition model scenario was developed to quantify the natural range of functional flow metrics as a starting point (CEFF Section A) and to evaluate alteration of the current flow regime to inform management decisions (consideration for CEFF Section C). The reference condition scenario used the current climatic, soil, and slope conditions in the watershed. However, urban and agricultural land, imported water, water extraction, water impoundments, and other flow regulation systems were removed. This condition is not intended to represent a specific point in time but instead to serve as broad characterization of the natural flow variability in absence of anthropogenic disturbances.
3.2.2 Natural Ranges of Functional Flow Metrics (CEFF Section A)
This study evaluated hydrology across a suite of 24 functional flow metrics that describe the magnitude, timing, frequency, and duration of functional flow components identified for California streams (Yarnell et al., 2020). In California, functional flow components include the fall pulse flow, wet-season baseflow, peak flows, spring recession flow, and dry-season baseflow (Yarnell et al., 2020). For a description of functional flow metrics, see Supplementary Table S1. Modelled reference and current hourly flow timeseries from water year 1993–2019 were post-processed to mean daily flow, and functional flow metrics were quantified using the Functional Flows Calculator API client package in R (version 0.9.7.2, https://github.com/ceff-tech/ffc_api_client), which uses hydrologic feature detection algorithms developed by Patterson et al. (2020) and the Python functional flows calculator (https://github.com/NoellePatterson/ffc-readme). The functional flows calculator has difficulty detecting the timing of seasonal flow transitions (i.e., transition from dry-season to wet-season or wet-season to spring recession) if the annual hydrograph lacks seasonality. In such cases, the timing, duration, and magnitude metrics cannot be estimated for the water year. If timing values were not quantified with the calculator, we used the median timing value calculated across the period of record, to calculate the seasonal magnitude metrics for dry-season and wet-season baseflow and spring rate of change. The natural ranges of the flow metrics were defined as the 10th to 90th percentiles of the reference metric values calculated across the modelled time-period.
3.2.3 Determination of Non-flow Mediating Factors (CEFF Section A)
We conducted a literature review of existing watershed plans and stream studies on lower Aliso Creek to determine whether there were non-flow mediating factors, such as altered physical habitat, that could limit the effectiveness of the natural range of functional flow metrics in supporting ecosystem functions and identified which flow components may require additional consideration. The literature review included previous studies that assessed existing channel morphology and the potential for stream channel erosion to determine if the channel morphology was altered (i.e., the potential for channel incision, widening, and instability) and had the potential to limit functions associated with specific flow components. The focal flow components identified were subject to further analysis and refinement (CEFF Section B) to determine if the natural range of flows will be suitable for indicator species of management concern, including willow and arroyo chub, under current stream conditions and to develop refined ecological flow needs that consider altered stream conditions. For all other flow components with no limiting factors that would require additional refinements, the natural ranges of the flow metrics would be used as the ecological flow needs (CEFF Section A).
3.3 Refined Ecological Flow Needs for Components Requiring Additional Consideration (CEFF Section B)
For functional flow components with non-flow limiting factors, refined ecological flow needs were developed that considered the non-flow impairments and the life history needs of focal species. First, conceptual models were developed for the focal species that describe the relationship between functional flow components, mediating factor(s) (i.e., physical habitat), and ecosystem responses. Next, we conducted a literature review and compiled data to quantify the linkages in the conceptual model and develop habitat suitability relationships (3.3.1). We used hydraulic models to understand whether altered habitat conditions would provide suitable habitat for the focal species under various flow conditions (3.3.2). Finally, we determined refined ecological flow needs based on the hydraulic analysis (3.3.3).
3.3.1 Habitat Suitability Relationships
We evaluated whether the reference ecological flow needs would provide suitable habitat for arroyo chub (Gila orcutti) and Goodding’s black willow (Salix goodingii), both of which are indicator species of management concern that are representative of aquatic and riparian habitats. Arroyo chub are native to the streams of southern California, however have been extirpated in recent years due to habitat degradation, urbanization and fragmentation (Moyle et al., 1995; Benjamin et al., 2016). The willow are key components of riparian vegetation and provide important habitat for the endangered least Bell’s vireo (Vireo bellii pusillus). In highly urban areas of the study region, channelized reaches lack riparian habitat. Therefore, areas with augmented baseflows that support novel riparian habitat, may be of critical importance.
Arroyo Chub (Gila orcuttii)
The data collated for arroyo chub consisted of fish abundance and associated measurements of depth and velocity (Wulff et al., 2017a; Wulff et al., 2017b). The fish abundance and hydraulic data on depth and velocity were collected from 17 50 m reaches in 2015 (Wulf et al., 2017a) and 20 50 m reaches in 2016 (Wulff et al., 2017b). At each reach, fish abundance data were collected through a combination of seine netting, snorkeling and electrofishing techniques. Fish abundance, depth, and velocity (at 0.6 of the depth) data were collected where fish abundance data were collected. Reach habitat data were measured at transects positioned perpendicular to flow at every 10 m throughout the reach. Depth and velocity measurements were taken at each of 10 equidistant points along each transect. Depth was measured with a graduated wading rod. Velocity was measured with an electronic flow meter in the upstream direction. The hydraulic data where fish were located were defined as fish presences and reach habitat data where fish were not found were defined as fish absences. Limited data were available that described different life stages of chub, therefore individuals of all lengths were included in the model.
Following the procedure for developing fish species models in Stein et al. (2021a). Each hydraulic variable was modelled separately with either fish abundance or presence/absence. In brief, habitat suitability models were built for chub and velocity by first calculating a frequency histogram of fish abundance and velocity. A probability density curve was calculated from the histogram following a normal distribution probability function. To remove the accumulative probability values usually attained from this calculation, the habitat data were centered around the mean and scaled to 1 standard deviation. To maintain intuitiveness of the curve, the scaled habitat data were transformed back to their raw values. This results in a maximum potential probability value of 0.4 (vs. 1.0) because the total area under the curve represents the full range of probabilities. The habitat suitability model for the hydraulic variable depth was developed by applying Generalized Linear Models (GLMs) with binomial error distribution (1,0) with logit link function. The abundance data were transformed into presence/absence data.
Goodding’s Black Willow (Salix gooddingii)
We developed a suite of habitat rules used to identify ecological flow ranges for willow seedling and adult. Seedling mortality increases in both very wet and very dry conditions (Vandersande et al., 2001; Tallent-Halsell & Walker, 2002; Stein et al., 2021a) and with increased shear stress (Pasquale et al., 2014). Seedlings are dependent on soil water availability until their roots can reach the water table. Periodic high flows are important drivers of soil water content but are most suitable for seedling establishment early on in the growing season as large floods can scour the soil resulting in mortality (Woods and Cooper, 2005). However, the peak flow metrics that are related to scour do not typically occur during the critical growing period of April to September. We did not develop ecological flow needs for willow that correspond to the peak flows, assuming that the reference-based values will be a suitable target. Adult willows require flows to inundate the overbank area seasonally. Although they can withstand some large floods, these areas should not remain inundated for prolonged periods which may result in mortality or impaired growth (Hosner and Boyce, 1962; Nilsson, 1987; Bendix, 1999). For adult willows, we used a wet-season and dry-season baseflow lower threshold necessary to maintain at least 3 cm of depth of flow in the active channel, under the assumption that roots can reach the water table and used a maximum flow threshold at the channel capacity to limit overbank inundation and oversaturated soils in the overbanks. We also developed habitat criteria for the spring recession start magnitude to ensure that the lower limit will provide flows that will inundate the overbank to provide soil moisture in the overbanks prior to the start of the dry-season and ensure lateral connectivity to the floodplain for riparian seed dispersal. With these factors in mind, we determined flow ranges by applying a suite of rules developed (Table 1).
3.3.2 Stream Hydraulics
A one-dimensional hydraulic analysis, rather than a data- and resource-intensive two-dimensional analysis, was implemented to allow for flexibility in applying these methods across a multitude of reaches in the study region. Overall, the hydraulic analysis was conducted to evaluate whether altered habitat conditions would provide suitable habitat for the focal species under various flow conditions. Rating curves were developed in R statistical programming version 4.0.2 (R Core Team 2020) to apply to the simulated flow timeseries to produce timeseries of hydraulic data for depth and average velocity at discrete channel sub-sections. First, channel geometry and reach characteristics, including slope (0.01) and field-verified Manning’s roughness n (0.035), were taken from Orange County’s LiDAR-derived channel geometry cross sectional dataset4 near the outlet of the model subbasins. The channel cross section was split into geomorphically-distinct sub-sections (e.g., left floodplain, left overbank, main channel, right overbank) where channel hydraulics were estimated. To build the rating curves, hydraulic variables need to be estimated for a range of flows at various water surface elevations. We identified 200 water surface elevations, using the minimum bed elevation and the maximum floodplain elevation at capacity as the range, that were used to calculate discharge, ranging from 0 to 101 cfs, and associated hydraulics. For every water surface elevation, velocity and discharge were estimated across hundreds of micro-sections of the channel geometry using Manning’s equation. Micro-sections were defined by the change in topography in the cross sectional profile. Total discharge was determined by summing the discharges from each channel sub-section. For each channel sub-section, maximum and average depth and mean velocity were determined for every water surface elevation. Rating curve functions were determined for each hydraulic variable based on a least-squares fit.
3.3.3 Ecological Flow Needs to Support Species
Ecological flow needs were determined for the functional flow components based on the habitat ruleset for willow and the habitat suitability relationships for arroyo chub and compared to the reference ecological flows identified in CEFF Section A. Habitat suitability curves for depth and velocity for arroyo chub were related to the flow at each cross-sectional sub-section by applying the rating curve for each hydraulic variable in the habitat suitability curve and flow in the stream. The flow associated with the hydraulic value for a medium probability threshold of 50%, which was an agreed-upon criteria by the stakeholder and technical advisory groups, was determined for each hydraulic variable to create a target flow range. Hydraulic flow ranges were combined for each sub-section to develop ranges of integrative ecological flow needs. On occasions where flow ranges for depth and velocity did not overlap, the range of the variable least supported by the current flow range (limiting hydraulic factor) was used. The flow ranges developed for willow and arroyo chub represent the refined ecological flow needs.
The refined ecological flow needs, from CEFF Section B, and the natural range of the flow metrics for the remaining components that were not refined, from CEFF Section A, were combined to make up the overall ecological flow needs for all functional flow components. In developing the overall ecological flow needs, we evaluated whether the natural range of flow metrics will be suitable for the indicator species, to ensure that the holistic functional flow needs will be supportive of the ecological management goals for the region.
3.4 Alteration Assessment to Inform Flow Management (Consideration in CEFF Section C)
Prioritizing parts of the annual hydrograph to tailor management actions can be difficult when alteration is widespread across broad spatial scales and multiple aspects of the annual hydrograph. The extent of current hydrologic alteration was evaluated based on deviation from the reference ranges and deviation from the refined ecological flow needs developed. Alteration was assessed across all functional flow metrics by comparing the distribution of metric values under current and reference conditions. By utilizing the distribution of functional flows across the full period of record, as opposed to a year-by-year comparison, this approach evaluated the general trends in flow conditions over time. First, the 10th, 50th (median), and 90th percentiles were calculated for both reference and current functional flow metric values. Next, we applied the criteria illustrated in Figure 2 to assign an alteration status for each metric by comparing the median current value to the 10th and 90th percentile range of reference values and evaluating the percentage of years that current flow metric values fall within the 10th and 90th percentile range of reference values. The three alteration categories assigned were likely altered, likely unaltered, and indeterminate and the direction of alteration was categorized as high or low and early or late. For the focal flow components with specific flow needs for willow and arroyo chub, we utilized the same alteration criteria but used the refined ecological flow needs instead of the reference ranges.
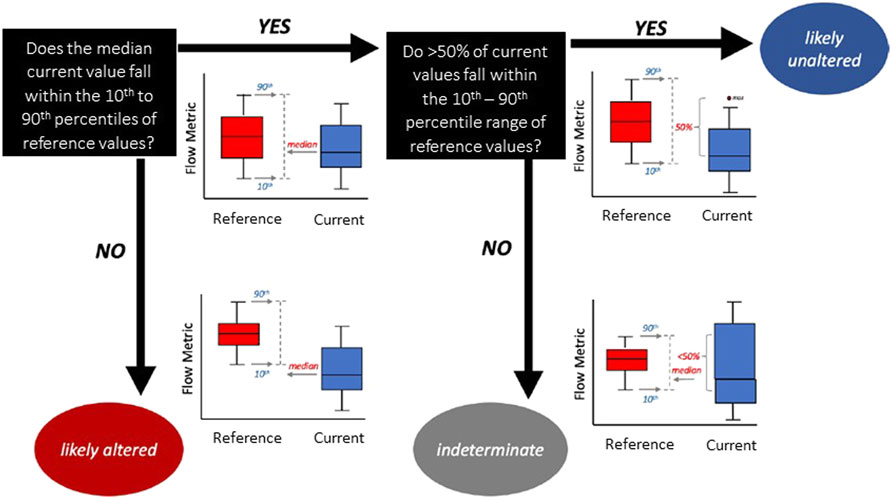
FIGURE 2. Criteria for assigning alteration status adapted from CEFF Appendix J (in review). Alteration was evaluated based on the deviation of current flows from reference conditions and the deviation of current flows from refined ecological flow needs identified.
3.5 Adjusting Ecological Flow Needs Based on Design of Restored Channel (Consideration in CEFF Section C)
Given the possibility that altered channel morphology may limit ecological functionality of reference flows, we evaluated scenarios for channel rehabilitation that may better support ecologic functions under reference and current flow conditions. In this example, we designed an alternative channel geometry with a low-flow channel within the main channel to provide suitable depths for arroyo chub (depth of at least 0.53 m total in the main channel throughout the reach) and a top width (1.5 m) that allows for seasonal inundation of an inset floodplain for willow. We developed new rating curves and identified revised ecological flow needs for willow and arroyo chub. We compared the flow ranges under current and reference conditions with the ecological flow needs under existing and “restored” channel conditions. We also evaluated the suitability of hydraulic conditions under both the current geometry and the alternative channel design to illustrate how non-flow actions, such as channel rehabilitation, could achieve ecological flow needs.
4 Results
4.1 Hydrologic Modeling
Model performance relative to calibration targets is shown in Table 2 for the lower Aliso Creek gauge, which was located at the study reach. Scatter plots of modeled mean monthly flow and mean daily flow showed a good fit to observed data over multiple orders of magnitude for the lower Aliso Creek gage (Figure 3).
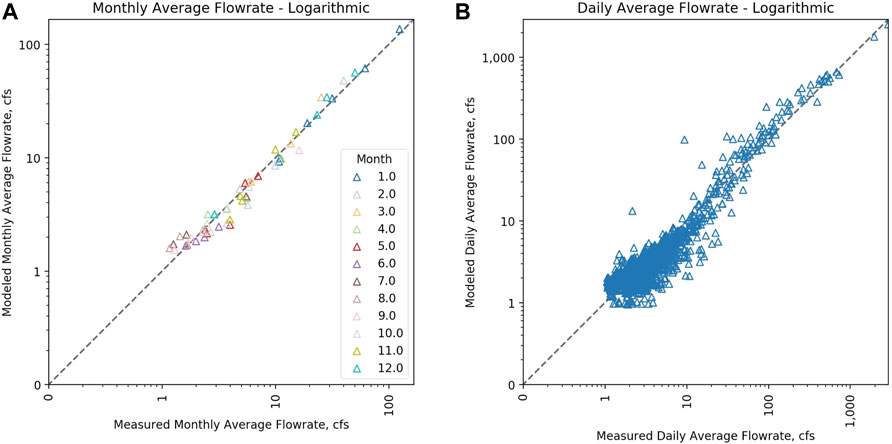
FIGURE 3. Comparison of monthly (A) and daily average (B) simulated streamflow to observed streamflow at the lower Aliso Creek gage between 1 October 2014 and 20 May 2019.
4.2 Ecological Flow Needs
4.2.1 Functional Flow Components and Ecosystem Functions (CEFF Section A)
Ecosystem functions that must be supported by ecological flows to achieve management goals were identified for each functional flow component (Table 3). All flow components support a broad range of functions important to overall riparian and aquatic ecosystem health. For example, the wet-season baseflow increases shallow groundwater, which is important for riparian habitat, and supports migration, spawning, and residency of aquatic organisms. While the dry-season baseflow maintains riparian soil moisture during the growing period and suitable water temperatures for aquatic species.
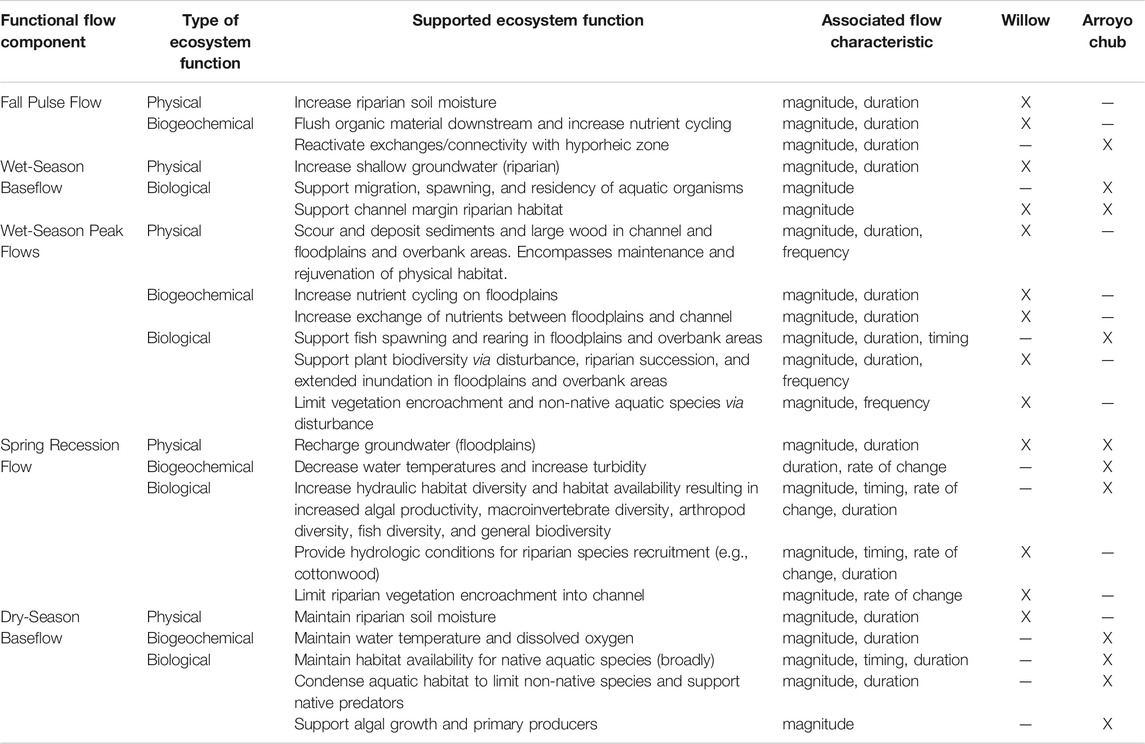
TABLE 3. Functions associated with functional flow components and species of management concern, willow and arroyo chub. Table adapted from (Yarnell et al., 2020).
4.2.2 Identifying Non-flow Limiting Factors (CEFF Section A)
Channel Morphology
In lower Aliso Creek, portions of the reach have been identified to have clear bank instabilities and major hydromodification impacts due to increases in peak flows from upstream urbanization (County of Orange, 2021). Channel incision and widening via fluvial erosion and mass failure are the primary channel responses to altered flood hydrology in lower Aliso Creek (Collison and Garrity, 2009). In some areas where incision and subsequent widening have decreased the longitudinal slope, the channel was vertically stable and slightly aggregational, as evidenced by the age of riparian trees observed on the inset floodplain (Collison and Garrity, 2009; Tetra Tech, 2014). Excessive channel widening in lower Aliso Creek, however, has resulted in infrastructure failure of sewer lines and the adjacent road (Tetra Tech, 2012). Although there is limited space for future development in the contributing watershed and minimal potential for future changes to peak flows, additional bank failure and channel widening are likely to occur in locations where banks are nearly vertical, composed of unconsolidated alluvium, and contain tension cracks (Tetra Tech, 2010; Tetra Tech, 2014).
Altered channel morphology, including channel widening and instability, may be a factor that could limit functionality of the natural range of flow metrics for the spring recession flow, wet-season baseflow, and dry-season baseflow component (Table 4). For example, the widened channel could potentially limit baseflows from providing necessary depths to support migration, spawning, and residency of aquatic organisms. The widened channel could also limit the natural range of the spring recession flow from inundating the floodplain, which is necessary for riparian seed dispersal and providing adequate soil moisture prior to the dry-season. However, the functionality of the natural range of the fall pulse flows and peak flows may not be limited as these higher flows within the widened channel can provide a range of depths and velocities that promote scour, deposition, inundation, and floodplain connectivity. Therefore, the refined ecological flow needs developed in Section B of CEFF considered altered channel morphology as the primary limiting factor to ensure that the associated stream functions can be supported.
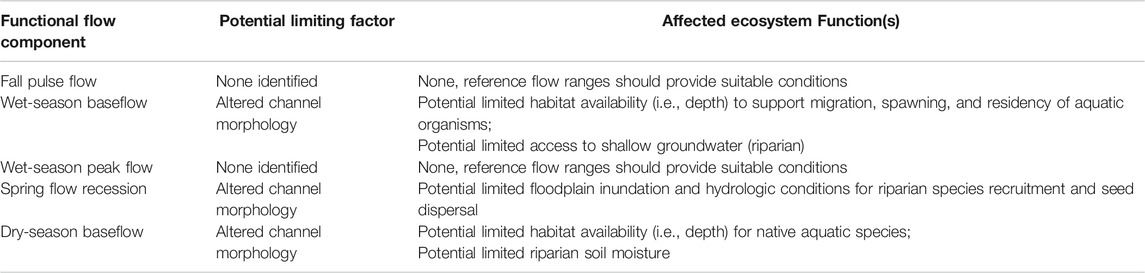
TABLE 4. Potential non-flow limiting factors that may alter the relationship between the natural range of functional flow metrics and their intended functions for each functional flow component.
4.2.3 Determining Ecological Flow Needs (CEFF Section B)
Habitat Suitability Relationships
Conceptual models to determine ecological flow needs for the focal flow components considered altered channel morphology and the life history needs of focal species, willow (Figure 4) and arroyo chub (Figure 5). Habitat suitability models for arroyo chub survival developed for the hydraulic variable velocity and depth (deviance = 265.84, p < 0.001) are shown in Figure 6. The velocity of 0.49 m/s was associated with the maximum probability, and 0.19–0.79 m/s was the velocity range associated with the medium probability of 0.2. The depth associated with the medium probability of 0.5 was 53 cm, which served as our lower limit of the ecological flow needs.
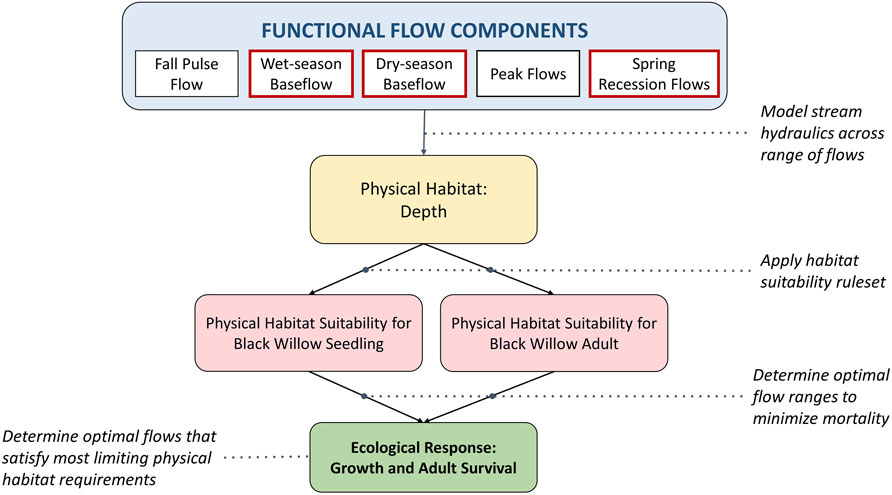
FIGURE 4. Conceptual model for refining ecological flow needs for wet-season and dry-season baseflow and spring recession flow components based on black willow.
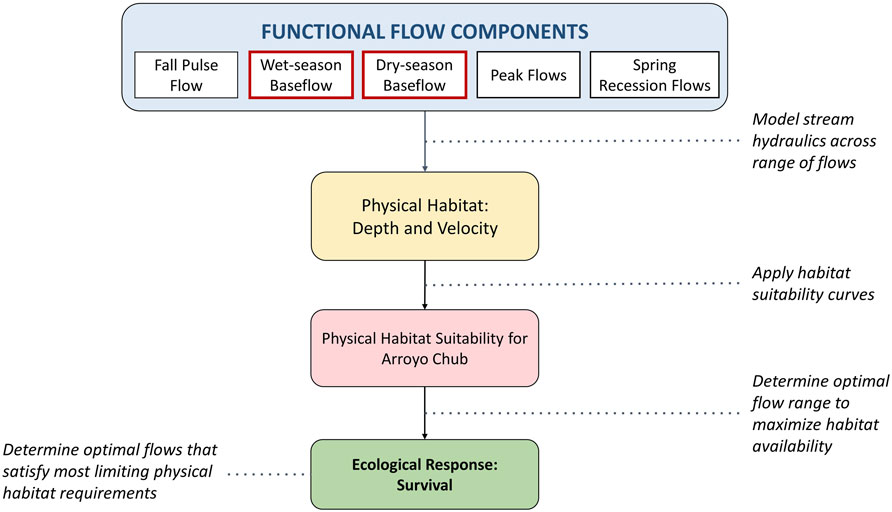
FIGURE 5. Conceptual model for refining ecological flow needs for dry-season and wet-season baseflow components based on arroyo chub.
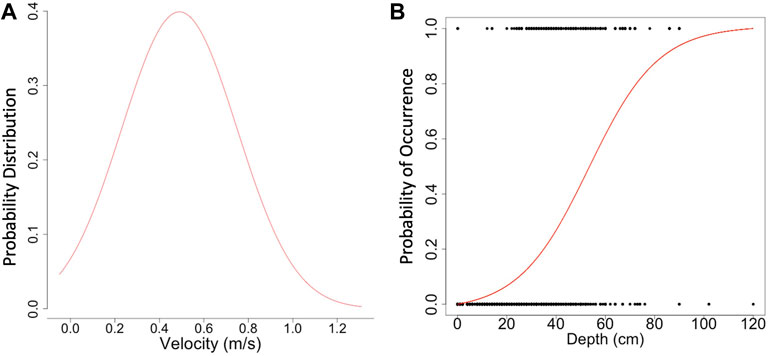
FIGURE 6. Suitability relationships for arroyo chub survival based on velocity (A) and depth (B). Data used to generate curves were from (Wulff et al., 2017a, Wulff et al., 2017a and 2017b).
Refined ecological flow needs were developed for the dry-season and wet-season baseflow magnitudes and the spring recession start magnitude based on the habitat suitability requirements for willow and arroyo chub (Table 5). Together, the natural and refined ranges of flow metric values represent the ecological flow needs, or the suite of functional flow metrics that can serve as a management goal. Under current channel morphology, the flow at the active channel capacity was 12 cfs. For willow adult, the ecological flow needs for wet-season and dry-season baseflow magnitude were 0.1–12 cfs. The natural range of the wet- and dry-season baseflow magnitude, 2 to 5 cfs and 0.5 to 4 cfs, respectively, would be suitable for willow adult. For willow adult and seedling, the ecological flow needs for the spring recession start magnitude was 33–528 cfs. Under the existing channel morphology, the reference lower limit of 5 cfs would not provide ecosystem functions associated with floodplain inundation and would need to be increased to 33 cfs to provide such functions. For arroyo chub, depth was the limiting hydraulic factor under the existing channel morphology. Both the wet-season and dry-season baseflow magnitude need to be at least 120 cfs to provide suitable depths in the existing channel morphology for arroyo chub. The minimum flow of 120 cfs is well beyond the baseflow ranges under current and natural conditions, 2 to 4.9 cfs and 0.3 to 3 cfs, respectively, and are only observed during storm events. Overall, the natural range of flow metrics would provide suitable conditions for willow but not for arroyo chub. We therefore slightly adjusted the refined ecological flow needs for willow to ensure that willows are supported (Table 5). Given that the refined ecological flow needs for arroyo chub were unrealistic management goals, we evaluated whether channel restoration could provide suitable habitat for chub under both natural and current flow conditions (see results in Adjusting Ecological Flow Needs Based on Design of Restored Channel (Consideration in CEFF Section C) Section).
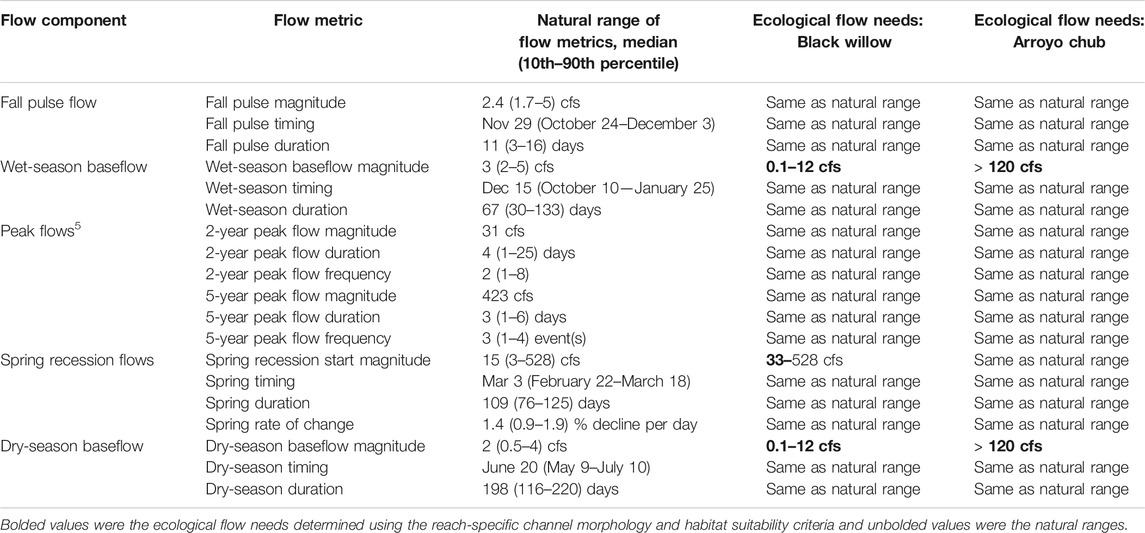
TABLE 5. Natural range of flow metrics from CEFF Section A and ecological flow needs for black willow and arroyo chub from CEFF Section B.
4.3 Alteration assessment to inform management (consideration in CEFF Section C)
Overall, the fall pulse flow and spring recession flow components had more than one flow characteristic that were likely altered compared to natural conditions (Table 6 and Supplementary Data Sheet S1 for current flow metric ranges). Although the spring recession start magnitude was classed as likely unaltered compared to reference conditions and the ecological flow needs for willow, the spring flow recession was quicker and had a larger rate of change compared to the natural range. Alteration based on the ecological flow needs for willow were likely unaltered for all relevant flow metrics, indicating that current flow conditions are suitable for willow. In contrast, the current flow conditions for the wet- and dry-season baseflow magnitude were determined as altered low based on the flow needs for arroyo chub, as the current baseflows were too shallow for chub.
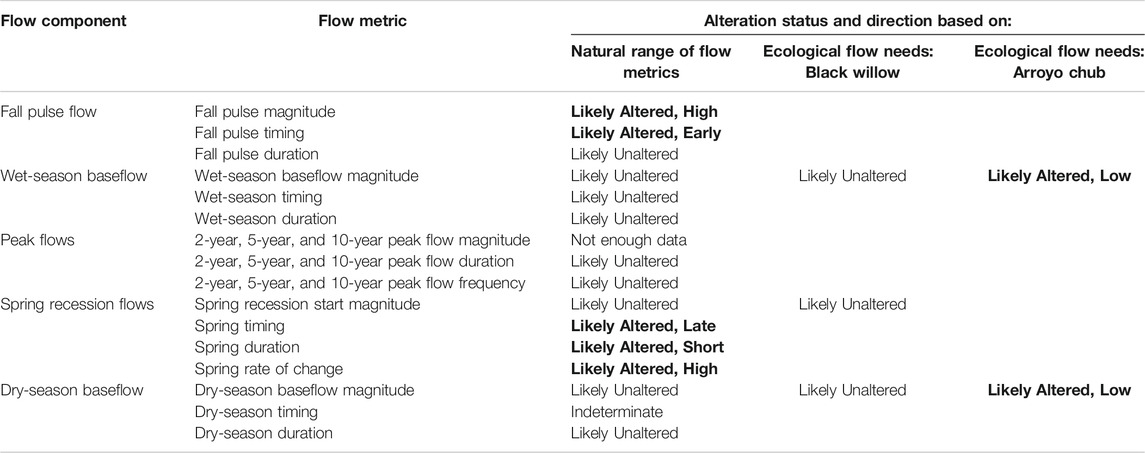
TABLE 6. Alteration status and direction for functional flow metrics comparing current flows to natural ranges and ecological flow needs for willow and arroyo chub. Likely altered statuses are bolded.
4.4 Adjusting Ecological Flow Needs Based on Design of Restored Channel (Consideration in CEFF Section C)
With the alternative channel design, the ecological flow needs for the wet- and dry-season baseflows will allow for slightly less water needed to support willow (Figure 7). The wet- and dry-season baseflow magnitude lower limit for willow adult decreased from 0.11 cfs under the existing channel morphology to 0.09 cfs under the alternative channel design. Both flow limits are below the current wet- and dry-season baseflow range of 3.6–6.3 cfs and 2.4 to 5 cfs, respectively. The ecological flow needs for willow spring recession start magnitude, which is defined by the flow associated with overbank inundation, decreased from 33 cfs under existing channel morphology to 18 cfs under the alternative channel design, which is closer to the natural median value of 15 cfs.
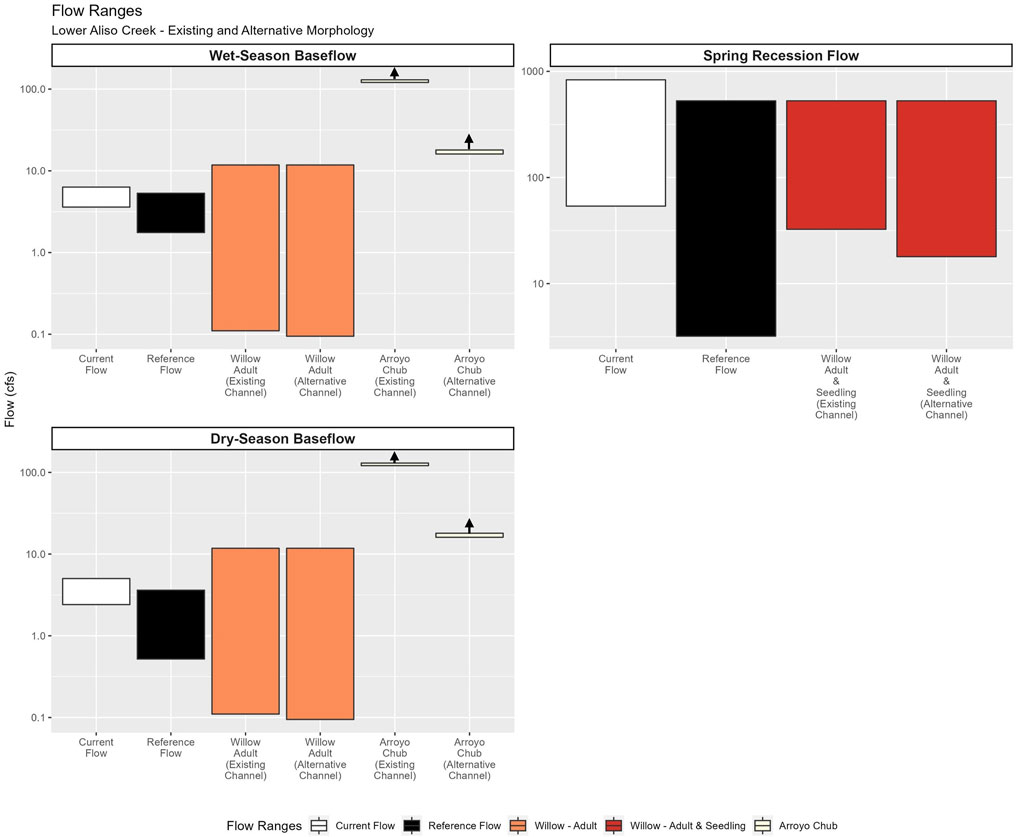
FIGURE 7. Flow ranges for dry-season baseflow, spring recession flow, and wet-season baseflow magnitudes under current and reference conditions and refined ecological flow needs for focal species, willow and arroyo chub, developed for the existing channel morphology and a “restored” alternative channel design. Current flow conditions are suitable for willow seedling and adult under existing and alternative channel geometries but are too low for arroyo chub.
Changes to the channel morphology could substantially reduce the ecological flow needs to support arroyo chub for the wet- and dry-season baseflow magnitudes. Ecological flow needs would be reduced from >120 cfs under the existing channel morphology to >16 cfs under the alternative channel design.
Habitat suitability for arroyo chub with the existing channel was 0.25% (+/- 0.34) of time during summer and 3.48% (+/- 0.35) of time during winter. In the restored channel, current baseflows were still not high enough to provide suitable depths during the winter and summer, however, habitat suitability for chub increased to 0.88% (+/- 0.9) of time over the summer and 10.1% (+/- 0.91) of time over winter. The most limiting physical habitat requirements for arroyo chub survival was depth associated with the dry-season and wet-season baseflow magnitude, as velocity was suitable under existing and restored channel conditions.
Suitability for willow adult with the existing channel was 99.1% +/- 0.3 of time during the summer and 85% (+/- 2.09) during the winter. In the restored channel, suitability for willow adult only minimally increased to 99.2% (+/- 0.23) during summer and increased to 88.6% (+/- 1.01) during the winter. The spring recession start magnitude was suitable for willow adult and seedling for 80.1% of the modeled years with the existing and the restored channels.
5 Discussion
5.1 Study Limitations
We provide a simplistic one-dimensional hydraulic analysis of physical habitat suitability at a high priority stream reach to develop ecological flow needs that could be implemented at other priority stream reaches, with the primary goal of illustrating the process and application of CEFF in an altered system. The alternative channel design evaluated here was not intended to be a recommended design for channel rehabilitation, but rather an illustration of how changes to the channel morphology could be tailored to provide more suitable physical habitat conditions for species of management concern, even without changing the flow regime. A more detailed two-dimensional hydraulic model is recommended for the design of channel rehabilitation projects and to evaluate the spatial variability of hydraulics at larger spatial scales. Future evaluations should also consider the importance of in-stream habitat heterogeneity for fish including availability to low-flow refugia (Magoulick and Kobza, 2003). In intermittent streams or during times of drought, fish can oversummer in perennial pools that provide suitable refugia (Magoulick and Kobza, 2003). There may be other limiting factors including water quality and stream temperature, substrate composition, interactions with invasive species, food availability, among others, that should be considered in a comprehensive habitat suitability analysis. Moreover, future research could couple a comprehensive population viability model (Anderson et al., 2006; Shenton et al., 2012; Tonkin et al., 2018), models based on guilds of species that share similar flow needs (Merritt et al., 2010), or flow ecology models based on community responses (Irving et al., 2022; Mazor et al., 2018) with the eco-hydraulic analysis. Additionally, we utilized a more simplified hydraulic analysis to be applied at multiple high priority stream reaches in the South OC region. This approach allows for the development of ecological flow needs at the regional scale.
Although this study focuses on developing ecological flow needs, multiple additional steps need to be taken to develop balanced environmental flow recommendations that account for ecological and non-ecological water uses. Prior to implementation of flow management actions, a trade-offs analysis that considers the consequences of multiple alternative management scenarios on ecological and non-ecological management objectives is recommended. For example, a Multi-Criteria Decision Analysis (MCDA) could be used to quantify socio-economic and environmental tradeoffs of multiple management scenarios (Barton et al., 2020) and can form the basis for developing environmental flow recommendations among multiple stakeholders.
5.2 Importance of physical habitat on developing ecological flow needs
In highly altered systems where channel morphology has been altered via excess incision or widening, for example, the relationship between physical habitat characteristics such as depth, velocity, and shear stress, and flow will change, making it critical to consider altered channel morphology when developing ecological flow needs. Ecological flow needs based solely on the natural flow regime may not provide suitable physical habitat conditions to support species in areas where stream channel alterations have occurred. In this study, the natural range of baseflows and peak flows would provide suitable conditions for willow adult, even with the widened channel morphology. However, the natural lower limit of the spring recession start magnitude would not support seasonal floodplain inundation. In highly incised streams, the natural ranges of peak flows, for example, may not inundate the floodplain (Edwards et al., 2016) and important floodplain functions and processes associated with lateral connectivity such as seed dispersal and spawning (Hayes et al., 2018; Yarnell and Thoms, this issue), may not be supported.
In the current widened channel, the natural ranges of baseflows would not provide suitable depths for arroyo chub in the main channel, even with the existing augmented baseflows. Moreover, current baseflow conditions would be too shallow to support arroyo chub with the alternative channel geometry. If restoring flow and hydraulic conditions for arroyo chub is a priority in this reach, channel rehabilitation, including provisions for suitable refugia under low-flow conditions, are likely necessary, in addition to flow management. Likewise, critical physical habitat features such as shallow edgewater habitats that provide slow moving, warmer water and refuge for tadpoles and other aquatic organisms may no longer be accessible or present in highly enlarged stream reaches (Wheeler et al., 2015). In addition to the importance of lateral connectivity, longitudinal connectivity of the stream network and the availability of low-flow refugia, such as perennial pools, are important considerations when determining if the natural ranges of flows can support ecological functions.
Flow ecology relationships can be used to develop ecological flow needs, but in highly altered systems with physical habitat degradation, consideration of altered channel morphology should be taken. Direct relationships between multiple aspects of flow and ecological response, used in holistic frameworks such as ELOHA (Poff et al., 2010), can be used to develop regional-scale ecological flow needs if data on flow are characterized at every site where biological data is collected. These direct statistical relationships between flow and ecological response, however, do not consider altered channel morphology and subsequent flow needs derived from these relationships at streams with altered morphology may not be adequate. In other words, a flow in a stream with a natural morphology may be ecologically protective but that same flow in an enlarged stream, may not be. These direct flow ecology relationships could be developed for different stream geomorphic types, as in the ELOHA framework, but there is seldom the data density of both hydrology and ecology collected specifically at enlarged geomorphic stream types.
A multitude of physical habitat suitability methods can be used to directly link flow, hydraulics, and ecology (Ahmadi-Nedushan et al., 2006) and can be especially useful in developing ecological flow needs in streams with altered channel morphology. For example, the Instream Flow Incremental Methodology (IFIM) and the physical habitat simulation model, PHABSIM, which uses IFIM as a basis (Stalnaker et al., 1995; Bovee et al., 1998) are widely used to evaluate how physical habitat characteristics, such as depth and velocity, vary with flow and are combined with habitat suitability criteria to determine the amount of available habitat across the stream network. However, PHABSIM has been criticized because it cannot be used to evaluate flow variability over time and cannot consider seasonal functional flow metrics that are associated with timing and duration which are key components of the flow regime (Railsback, 2016). More sophisticated two-dimensional hydraulic modeling of continuous flow could be applied to evaluate habitat suitability both laterally across the channel and floodplain, longitudinally, and temporally. However, such approaches require detailed topographic information of stream and floodplain morphology, require higher computing power and time, and could therefore be an infeasible approach to develop ecological flow needs across multiple stream reaches. Detailed hydraulic analyses also typically consider the needs of single species and may focus solely on one part of the annual hydrograph, such as dry-season baseflow, which have the potential to result in ecological flow needs that optimize conditions for one species at the expense of others (Acreman et al., 2014b; Tonkin et al., 2021).
In this study, we lay out a functional flows approach that is broadly protective of ecosystem functions, considers altered channel morphology, and could be applied to other modified systems. Although our hydraulic analysis to develop ecological flow needs for arroyo chub is similar to PHABSIM, the functional flows approach goes beyond specifying flow needs that correspond solely to baseflows by encompassing the natural range of flow variability across multiple seasonal flow components that are tied to a range of ecosystem functions (see Table 3). Moreover, we illustrated how designing flow targets based solely on a single species, may negatively impact other species. For example, baseflow targets for arroyo chub under the widened channel morphology would be too high for willow and could lead to excess sediment transport that could negatively impact bugs and algae. Channel restoration may be necessary so that the natural, current, or future range of flows can be functional for chub, willow, and other species of management concern. The approach we developed was designed to be simplistic enough, in terms of data requirement and computing power, to be implemented across multiple stream reaches, inclusive of all seasonal flow components that are broadly protective of overall stream health, and takes special consideration of the landscape and the species in it.
5.3 Lessons Learned From Implementing CEFF
CEFF provides flexible guidance that can be used to support important habitats, even if they are not natural, and consider alterations to the landscape. In this study, we developed ecological flow needs for species of management concern that may be indicative of aquatic and novel riparian habitat and considered channel widening from the altered urban landscape. Through Section B, multiple approaches could be used to develop ecological flow needs including traditional hydraulic analyses similar to PHABSIM, as illustrated with arroyo chub, by using habitat suitability criteria that directly relate the life history needs of species to aspects of the seasonal flow components, as illustrated with willow, or more complex hydraulic approaches that were not explored in this study. Regardless of the method used in Section B, the key to CEFF is implementing a functional flows approach that considers all seasonal flow components and aspects of the annual hydrograph that are linked to ecosystem functions and the natural variability of these components. The full set of ecological flow needs developed through CEFF combines ecological flow needs from Section A, based on the natural ranges, and the refined ecological flow needs related to ecological management goals from Section B.
In highly altered systems, the alteration evaluation of CEFF Section C may be critical to inform future management decisions. For example, we found that the ecological flow needs developed for arroyo chub would never be achievable under current flow conditions and the existing channel morphology due to channel widening. Even with the current augmented baseflows, the lower limit of the ecological flow needs only occur during high flow storm events. If the goal of restoring habitat for chub was a priority, non-flow related management actions, such as channel rehabilitation, should be implemented to provide more achievable ecological flow targets and improve physical habitat conditions. Moreover, CEFF could be used to inform the design of channel rehabilitation projects to provide more suitable conditions for species that are regulated and alternative non-flow related management strategies aimed at achieving ecological flow needs.
CEFF uses a functional flows approach that provides a mechanism to determine if there may be optimal times of the year that additional water could be used for other uses. In this study, ecological flow needs for wet- and dry-season baseflow for willow are below the current augmented baseflow ranges, indicating that there may be opportunities to divert and reuse a portion of the augmented baseflows while still supporting willow. There may be more capacity for water to be used for other uses during winter stormflows when flows are highly augmented, well beyond the ecological flow needs. In the effluent-dominated Los Angeles River, for example, there was more capacity for wastewater reuse to occur during the wet-season compared to the dry-season, as reductions to the dry-season baseflow magnitude could impact novel ecosystems and recreational uses (Wolfand et al., 2022).
6 Conclusion
This paper demonstrates the utility of CEFF in developing ecological flow needs that consider altered physical habitat and the needs of species of management concern. We developed a functional flows approach that can be implemented in other areas with highly modified streams. First, we identified which seasonal functional flow components may be impacted by altered channel morphology and require further consideration. For the flow components being refined, we used a simplistic hydraulic analysis that relates flow, hydraulics, and habitat suitability to the life history needs of multiple species of management concern. For flow components that were likely not impacted by altered morphology, we developed flow needs based on the natural range of flow variability over time for multiple key components of the hydrograph that are hypothesized to be protective of overall stream health. Together, the refined and reference-based ecological flow needs developed represented a holistic set of ecological flow recommendations for all seasonal components of the annual hydrograph. Moreover, by developing ecological flow needs for multiple species, we illustrated how designing flow targets based solely on a single species, may negatively affect other species.
Results highlighted how non-flow management actions, such as stream channel rehabilitation, may produce achievable ecological flow needs and more suitable physical habitat conditions. Although the augmented dry-season baseflow magnitude is currently supporting the novel riparian habitat, the widened channel could get restored to provide suitable habitat in the main channel for fish and seasonal inundation of an inset floodplain, for example, to support willow riparian habitat. In highly degraded streams, non-flow related management actions in addition to flow management may be necessary to improve the habitat conditions for species that are regulated.
Although CEFF was developed for California streams, the flexible and non-prescriptive guidance that uses a functional flows approach allows for the framework to inform the development of ecological flow needs and environmental flow recommendations in other regions and across a multitude of management contexts. Additional studies implementing CEFF across a diversity of stream types and management applications are needed in order to refine the framework and improve the development of environmental flow regulations that are protective of overall stream health. Future studies should also consider how future changes in climate and water use may impact the ecological flows needed to support ecosystem functions and stream health over time.
Data Availability Statement
The datasets presented in this study can be found in online repositories. The names of the repository/repositories and accession number(s) can be found in the article/Supplementary Material.
Author Contributions
KT led the preparation of this manuscript and the CEFF evaluation. RW, AP, JF contributed to hydrologic model development. KI contributed to species model development and evaluation. ES, KT, KI, AA, RW, CR, GS contributed to the conceptualization of the project design. KT, ES, SY contributed to the CEFF application framing. All authors contributed to writing this manuscript.
Funding
Funding for this work was provided by OCPW under Agreement #MA-080-18010919 and by the California Wildlife Conservation Board’s Stream Flow Enhancement Program (Proposition 1) under Grant Agreement #WC-1849AB.
Conflict of Interest
AP, RW, and JF are employed by Geosyntec Consultants, Inc.
The remaining authors declare that the research was conducted in the absence of any commercial or financial relationships that could be construed as a potential conflict of interest.
Publisher’s Note
All claims expressed in this article are solely those of the authors and do not necessarily represent those of their affiliated organizations, or those of the publisher, the editors and the reviewers. Any product that may be evaluated in this article, orclaim that may be made by its manufacturer, is not guaranteed or endorsed by the publisher.
Acknowledgments
We would like to thank the members of the South OC Flow Ecology Special Study Stakeholder and Technical Advisory Groups for valuable feedback on the overall project scope and technical approach and members of the California Environmental Flows Framework technical team for providing input and review of this manuscript. We would also like to thank Anne Holt from Southern California Coastal Water Research Project for providing enhancements to the functional flow metric calculations.
Supplementary Material
The Supplementary Material for this article can be found online at: https://www.frontiersin.org/articles/10.3389/fenvs.2022.787631/full#supplementary-material
Supplementary Data Sheet S1 | Current range of functional flow metrics from the 10th percentile to the 90th percentile. For all units and flow metric descriptions, see Supplementary Table S1.
Footnotes
1https://www.southocwqip.org/pages/flow-ecology-study.
3https://www.ocgis.com/ocpw/IllicitDischarge/.
4Dataset available at: https://www.ocgis.com/ocpw/IllicitDischarge/.
5The 10-years peak flow metrics are not shown
References
Abdi, R., Rogers, J. B., Rust, A., Wolfand, J. M., Philippus, D., Taniguchi-Quan, K., et al. (2021). Simulating the thermal Impact of Substrate Temperature on Ecological Restoration in Shallow Urban Rivers. J. Environ. Manage. 289, 112560. doi:10.1016/j.jenvman.2021.112560
Acreman, M., Arthington, A. H., Colloff, M. J., Couch, C., Crossman, N. D., Dyer, F., et al. (2014a). Environmental Flows for Natural, Hybrid, and Novel Riverine Ecosystems in a Changing World. Front. Ecol. Environ. 12 (8), 466–473. doi:10.1890/130134
Acreman, M. C., Overton, I. C., King, J., Wood, P. J., Cowx, I. G., Dunbar, M. J., et al. (2014b). The Changing Role of Ecohydrological Science in Guiding Environmental Flows. Hydrological Sci. J. 59 (3–4), 433–450. doi:10.1080/02626667.2014.886019
Adams, A. J., Kupferberg, S. J., Wilber, M. Q., Pessier, A. P., Grefsrud, M., Bobzien, S., et al. (2017). Extreme Drought, Host Density, Sex, and Bullfrogs Influence Fungal Pathogen Infection in a Declining Lotic Amphibian. Ecosphere 8, e01740. doi:10.1002/ecs2.1740
Ahmadi-Nedushan, B., St-Hilaire, A., Bérubé, M., Robichaud, É., Thiémonge, N., and Bobée, B. (2006). A Review of Statistical Methods for the Evaluation of Aquatic Habitat Suitability for Instream Flow Assessment. River Res. Applic. 22 (5), 503–523. doi:10.1002/rra.918
Anderson, K. E., Paul, A. J., McCauley, E., Jackson, L. J., Post, J. R., and Nisbet, R. M. (2006). Instream Flow Needs in Streams and Rivers: the Importance of Understanding Ecological Dynamics. Front. Ecol. Environ. 4 (6), 309–318. doi:10.1890/1540-9295(2006)4[309:ifnisa]2.0.co;2
Barton, D. N., Sundt, H., Bustos, A. A., Fjeldstad, H.-P., Hedger, R., Forseth, T., et al. (2020). Multi-criteria Decision Analysis in Bayesian Networks - Diagnosing Ecosystem Service Trade-Offs in a Hydropower Regulated River. Environ. Model. Softw. 124, 104604. doi:10.1016/J.ENVSOFT.2019.104604
Bendix, J. (1999). Stream Power Influence on Southern Californian Riparian Vegetation. J. Vegetation Sci. 10 (2), 243–252. doi:10.2307/3237145
Benjamin, A., May, B., O'Brien, J., and Finger, A. J. (2016). Conservation Genetics of an Urban Desert Fish, the Arroyo Chub. Trans. Am. Fish. Soc. 145, 277–286. doi:10.1080/00028487.2015.1121925
Bovee, K. D., Lamb, B. L., Bartholow, J. M., Stalnaker, C. B., Taylor, J., and Henriksen, J. (1998). Stream Habitat Analysis Using the Instream Flow Incremental Methodology. Fort Collins, CO: USGS/BRD-Information and Technology Report.
Collison, A., and Garrity, N. (2009). Memorandum: Aliso Creek Stabilization Project Review. Submitted to Kenneth Frank, City of Laguna Beach. San Francisco, California: Prepared by Philip Williams & Associates.
County of Orange (2021). South Orange County Watershed Management Area Water Quality Improvement Plan - Version 1.3. OrangeCalfornia: Submitted to the San Diego Regional Water Quality Control Board.
Cross, B. K., Bozek, M. A., and Mitro, M. G. (2013). Influences of Riparian Vegetation on Trout Stream Temperatures in Central Wisconsin. North Am. J. Fish. Manag. 33 (4), 682–692. doi:10.1080/02755947.2013.785989
Edwards, B. L., Keim, R. F., Johnson, E. L., Hupp, C. R., Marre, S., and King, S. L. (2016). Geomorphic Adjustment to Hydrologic Modifications along a Meandering River: Implications for Surface Flooding on a Floodplain. Geomorphology 269, 149–159. doi:10.1016/J.GEOMORPH.2016.06.037
Grantham, T. E., Carlisle, D. M., Howard, J., Lane, B., Lusardi, R., Obester, A., et al. (Forthcoming 2022) Modeling Functional Flows in California’s Rivers. Front. Environ. Sci. - Freshw. Sci. 9.
Hawley, R. J., and Bledsoe, B. P. (2013). Channel Enlargement in Semiarid Suburbanizing Watersheds: A Southern California Case Study. J. Hydrol. 496, 17–30. doi:10.1016/j.jhydrol.2013.05.010
Hawley, R. J., and Bledsoe, B. P. (2011). How Do Flow Peaks and Durations Change in Suburbanizing Semi-arid Watersheds? A Southern California Case Study. J. Hydrol. 405 (1–2), 69–82. doi:10.1016/j.jhydrol.2011.05.011
Hayes, D. S., Brändle, J. M., Seliger, C., Zeiringer, B., Ferreira, T., and Schmutz, S. (2018). Advancing towards Functional Environmental Flows for Temperate Floodplain Rivers. Sci. Total Environ. 633, 1089–1104. doi:10.1016/j.scitotenv.2018.03.221
Hosner, J. F., and Boyce, S. G. (1962). Tolerance to Water Saturated Soil of Various Bottomland Hardwoods. For. Sci. 8 (2), 180–186. doi:10.1093/forestscience/8.2.180
Irving, K., Taniguchi-Quan, K. T., Aprahamian, A., River, C., Sharp, G., Mazor, R. D., et al. (2022). Application of Flow Ecology Analysis to Inform Prioritization for Stream Restoration and Management Actions. Front. Environ. Sci. - Freshw. Sci. 9. doi:10.3389/fenvs.2021.787462
Kristensen, P., Kristensen, E., Riis, T., Anette, A., Larsen, S., Verdonschot, P., et al. (2015). Riparian forest as a Management Tool for Moderating Future thermal Conditions of lowland Temperate Streams. Inland Waters 5 (1), 27–38. doi:10.5268/IW-5.1.751
Kupferberg, S. J. (1997). Bullfrog (Rana catesbeiana) Invasion of a California River: The Role of Larval Competition. Ecology 78 (6), 1736. doi:10.2307/2266097
Lai, C.-T. (2020a). Stable Water Isotope Analysis at Pilot Outfalls in Orange County, California. Orange, CA: Technical Report for Orange County Public Works, 1–20.
Lai, C.-T. (2020b). Stable Water Isotope Analysis to Quantify Potable Water Contribution to Streamflow in Orange County, California. Orange, CA: Technical Report for Orange County Public Works, 1–16.
Lund, J., Medellin-Azuara, J., Durand, J., and Stone, K. (2018). Lessons from California’s 2012–2016 Drought. J. Water Resour. Plann. Manag. 144, 04018067. doi:10.1061/(asce)wr.1943-5452.0000984
Luthy, R. G., Sedlak, D. L., Plumlee, M. H., Austin, D., and Resh, V. H. (2015). Wastewater-effluent-dominated Streams as Ecosystem-Management Tools in a Drier Climate. Front. Ecol. Environ. 13 (9), 477–485. doi:10.1890/150038
Magoulick, D. D., and Kobza, R. M. (2003). The Role of Refugia for Fishes during Drought: A Review and Synthesis. Freshw. Biol. 48, 1186–1198. doi:10.1046/j.1365-2427.2003.01089.x
Mazor, R. D., May, J. T., Sengupta, A., Mccune, K. S., Bledsoe, B. P., and Stein, E. D. (2018). Tools for Managing Hydrologic Alteration on a Regional Scale: Setting Targets to Protect Stream Health. Freshw. Biol. 63, 786–803. doi:10.1111/fwb.13062
Merritt, D. M., Scott, M. L., Leroy Poff, N., Auble, G. T., and Lytle, D. A. (2010). Theory, Methods and Tools for Determining Environmental Flows for Riparian Vegetation: Riparian Vegetation-Flow Response Guilds. Freshw. Biol. 55, 206–225. doi:10.1111/j.1365-2427.2009.02206.x
Moriasi, D. N., Arnold, J. G., Van Liew, M. W., Bingner, R. L., Harmel, R. D., and Veith, T. L. (2007). Model Evaluation Guidelines for Systematic Quantification of Accuracy in Watershed Simulations. Trans. ASABE 50 (3), 885–900. doi:10.13031/2013.23153
Moyle, P. B., Yoshiyama, R. M., Williams, J. E., and Wikramanayake, E. D. (1995). Fish Species of Special Concern in California. Rancho Cordova, CA: California Department of Fish and Game.
Nilsson, C. (1987). Distribution of Stream-Edge Vegetation along a Gradient of Current Velocity. J. Ecol. 75 (2), 513–522. doi:10.2307/2260430
Opperman, J. J., Luster, R., McKenney, B. A., Roberts, M., and Meadows, A. W. (2010). Ecologically Functional Floodplains: Connectivity, Flow Regime, and Scale1. J. Am. Water Resour. Assoc. 46 (2), 211–226. doi:10.1111/j.1752-1688.2010.00426.x
Pasquale, N., Perona, P., Francis, R., and Burlando, P. (2014). Above-ground and Below-groundSalixdynamics in Response to River Processes. Hydrol. Process. 28, 5189–5203. doi:10.1002/hyp.9993
Patterson, N. K., Lane, B. A., Sandoval-Solis, S., Pasternack, G. B., Yarnell, S. M., and Qiu, Y. (2020). A Hydrologic Feature Detection Algorithm to Quantify Seasonal Components of Flow Regimes. J. Hydrol. 585, 124787. doi:10.1016/J.JHYDROL.2020.124787
Poff, N. L., Richter, B. D., Arthington, A. H., Bunn, S. E., Naiman, R. J., Kendy, E., et al. (2010). The Ecological Limits of Hydrologic Alteration (ELOHA): A New Framework for Developing Regional Environmental Flow Standards. Freshw. Biol. 55 (1), 147–170. doi:10.1111/j.1365-2427.2009.02204.x
Poff, N. L., and Zimmerman, J. K. H. (2010). Ecological Responses to Altered Flow Regimes: A Literature Review to Inform the Science and Management of Environmental Flows. Freshw. Biol. 55 (1), 194–205. doi:10.1111/j.1365-2427.2009.02272.x
Railsback, S. F. (2016). Why it Is Time to Put PHABSIM Out to Pasture. Fisheries 41, 720–725. doi:10.1080/03632415.2016.1245991
Richter, B. D., Davis, M. M., Apse, C., and Konrad, C. (2011). A Presumptive Standard for Environmental Flow Protection. River Res. Applic. 28 (8), 1312–1321. doi:10.1002/rra.1511
Shenton, W., Bond, N. R., Yen, J. D. L., and Mac Nally, R. (2012). Putting the “Ecology” into Environmental Flows: Ecological Dynamics and Demographic Modelling. Environ. Manage. 50 (1), 1–10. doi:10.1007/s00267-012-9864-z
Stalnaker, C., Lamb, B. L., Henriksen, J., Bovee, K., and Bartholow, J. (1995). The Instream Flow Incremental Methodology: A Primer for IFIM. Washington, DC: Biological Report - US Department of the Interior, National Biological Service.
Stein, E. D., Wolfand, J. M., Abdi, R., Irving, K., Hennon, V., Taniguchi-Quan, K. T., et al. (2021a). Assessment of Aquatic Life Use Needs for the Los Angeles River: Los Angeles River Environmental Flows Project. Costa Mesa, CA. Retrieved from https://ftp.sccwrp.org/pub/download/DOCUMENTS/TechnicalReports/1154_LARiverAquaticLifeUses.pdf.
Stein, E. D., Zimmerman, J., Yarnell, S. M., Stanford, B., Lane, B., Taniguchi-Quan, K. T., et al. (2021b). The California Environmental Flows Framework: Meeting the Challenges of Developing a Large-Scale Environmental Flows Program. Front. Environ. Sci. - Freshw. Sci. 9 doi:10.3389/fenvs.2021.769943
Tallent-Halsell, N. G., and Walker, L. R. (2002). Responses of Salix Gooddingii and Tamarix Ramosissima to Flooding. Wetlands 22, 776–785. doi:10.1672/0277-5212(2002)022[0776:rosgat]2.0.co;2
Tennant, D. L. (1976). Instream Flow Regimens for Fish, Wildlife, Recreation and Related Environmental Resources. Fisheries 1, 6–10. doi:10.1577/1548-8446(1976)001<0006:ifrffw>2.0.co;2
Tessman, S. A. (1980). Environmental Assessment, Technical Appendix E, in Environmental Use Sector Reconnaissance Elements of the Western Dakotas Region of South Dakota Study. South Dakota: Brookings.
Tetra Tech (2010). Aliso Creek F4 Geomorphic Assessment. Los Angeles District. Irvine, California: Prepared for the U.S. Army Corps of Engineers.
Tetra Tech (2014). Aliso Creek Mainstem Geomorphic Baseline Assessment County of Orange. CaliforniaLos Angeles District. Irvine, California: Prepared for U.S. Army Corps of Engineers.
Tetra Tech (2012). Lower Aliso Creek Erosion Assessment. Prepared for South Orange County Wastewater Authority. California: Dana Point.
Tonkin, J. D., Merritt, D. M., Olden, J. D., Reynolds, L. V., and Lytle, D. A. (2018). Flow Regime Alteration Degrades Ecological Networks in Riparian Ecosystems. Nat. Ecol. Evol. 2 (1), 86–93. doi:10.1038/s41559-017-0379-0
Tonkin, J. D., Olden, J. D., Merritt, D. M., Reynolds, L. V., Rogosch, J. S., and Lytle, D. A. (2021). Designing Flow Regimes to Support Entire River Ecosystems. Front. Ecol. Environ. 19, 326–333. doi:10.1002/fee.2348
Vandersande, M. W., Glenn, E. P., and Walworth, J. L. (2001). Tolerance of Five Riparian Plants from the Lower Colorado River to Salinity Drought and Inundation. J. Arid Environments 49 (1), 147–159. doi:10.1006/JARE.2001.0839
Wallace, S., Biggs, T., Lai, C.-T., and McMillan, H. (2021). Tracing Sources of Stormflow and Groundwater Recharge in an Urban, Semi-arid Watershed Using Stable Isotopes. J. Hydrol. Reg. Stud. 34, 100806. doi:10.1016/j.ejrh.2021.100806
Wheeler, C. A., Bettaso, J. B., Ashton, D. T., and Welsh, H. H. (2015). Effects of Water Temperature on Breeding Phenology, Growth, and Metamorphosis of Foothill Yellow-Legged Frogs (Rana Boylii): A Case Study of the Regulated Mainstem and Unregulated Tributaries of California's Trinity River. River Res. Applic. 31, 1276–1286. doi:10.1002/rra.2820
Wolfand, J., Taniguchi-Quan, K. T., Abdi, R., Gallo, E., Irving, K., Philippus, D., et al. (2022). Balancing Water Reuse and Ecological Support Goals in an Effluent Dominated River. Manuscript Submitted for Publication.
Woods, S. W., and Cooper, D. J. (2005). Hydrologic Factors Affecting Initial Willow Seedling Establishment along a Subalpine Stream, Colorado, U.S.A. Arctic, Antarctic, Alpine Res. 37 (4), 636–643. doi:10.1657/1523-0430(2005)037[0636:hfaiws]2.0.co;2
Wulff, M. L., Brown, L. R., and May, J. (2017a). Native Fish Population and Habitat Study, Santa Ana River, California, 2015. Sacramento, CA: U.S. Geological Survey Data Release. doi:10.5066/F72B8W48
Wulff, M. L., Brown, L. R., and May, J. T. (2017b). Native Fish Population and Habitat Study, Santa Ana River, California, 2016 (Ver. 2.0, August 2017). Sacramento, CA: U.S. Geological Survey Data Release. doi:10.5066/F7K072H3
Yarnell, S. M., Petts, G. E., Schmidt, J. C., Whipple, A. A., Beller, E. E., Dahm, C. N., et al. (2015). Functional Flows in Modified Riverscapes: Hydrographs, Habitats and Opportunities. BioScience 65 (10), 963–972. doi:10.1093/biosci/biv102
Yarnell, S. M., Stein, E. D., Webb, J. A., Grantham, T., Lusardi, R. A., Zimmerman, J., et al. (2020). A Functional Flows Approach to Selecting Ecologically Relevant Flow Metrics for Environmental Flow Applications. River Res. Applic 36 (2), 318–324. doi:10.1002/rra.3575
Yarnell, S. M., and Thoms, M. (Forthcoming 2022). Geomorphic Processes in Maximizing Environmental Flow Functionality and Creating Resilience. Front. Environ. Sci. - Freshw. Sci. 9.
Yarnell, S. M., Willis, A., Obester, A., Peek, R. A., Lusardi, R. A., Zimmerman, J., et al. (2022). Functional Flows in Groundwater-Influenced Streams: An Application of the California Environmental Flows Framework to Determine Ecological Flow Needs. Front. Environ. Sci. - Freshw. Sci. 9. doi:10.3389/fenvs.2021.788295
Keywords: functional flows, environmental flows, urban streams, channel alteration, flow ecology, flow management, channel rehabilitation
Citation: Taniguchi-Quan KT, Irving K, Stein ED, Poresky A, Wildman Jr RA, Aprahamian A, Rivers C, Sharp G, Yarnell SM and Feldman JR (2022) Developing Ecological Flow Needs in a Highly Altered Region: Application of California Environmental Flows Framework in Southern California, USA. Front. Environ. Sci. 10:787631. doi: 10.3389/fenvs.2022.787631
Received: 01 October 2021; Accepted: 10 January 2022;
Published: 21 February 2022.
Edited by:
Carlo Camporeale, Politecnico di Torino, ItalyReviewed by:
Maria Filomena Magalhães, University of Lisbon, PortugalGorazd Urbanič, Urbanzero Institute for Holistic Environmental Management, Slovenia
Copyright © 2022 Taniguchi-Quan, Irving, Stein, Poresky, Wildman Jr, Aprahamian, Rivers, Sharp, Yarnell and Feldman. This is an open-access article distributed under the terms of the Creative Commons Attribution License (CC BY). The use, distribution or reproduction in other forums is permitted, provided the original author(s) and the copyright owner(s) are credited and that the original publication in this journal is cited, in accordance with accepted academic practice. No use, distribution or reproduction is permitted which does not comply with these terms.
*Correspondence: Kristine T. Taniguchi-Quan, a3Jpc3RpbmV0cUBzY2N3cnAub3Jn