- 1Department of Applied Chemistry, College of Sciences, China Agricultural University, Beijing, China
- 2Solid Waste and Chemicals Management Center, Ministry of Ecology and Environment, Beijing, China
- 3South China Institute of Environmental Sciences, Ministry of Ecology and Environment, Guangzhou, China
- 4Agricultural Ecology Institute, Fujian Academy of Agricultural Sciences, Fuzhou, China
- 5College of Agronomy and Biotechnology, China Agriculture University, Beijing, China
Little is known about whether the application of biogas residues in rice fields will affect the degradation of pesticides. This study investigated the dissipation behavior of the fungicide difenoconazole in paddy water and sediment after the application of a chemical fertilizer or biogas residues. The results showed that the application of biogas residues changes the dissipation of difenoconazole in both paddy water and sediment. The half-lives of difenoconazole in paddy water and sediment with biogas residues were 0.50 and 10.09 days, respectively, while the half-lives of difenoconazole in paddy water and sediment with chemical fertilizer were only 0.22 and 4.64 days, respectively. After biogas residues were applied in a paddy field, no significant changes in pH value of paddy water and sediment and soil microorganisms were observed, but soil organic matter decreased by 30%. The above studies suggest that biogas residues may affect the dissipation of pesticides in paddy field water and sediments, which provides a new focus on the scientific and rational use of biogas residues as organic fertilizer in rice fields.
Introduction
Pesticides are routinely used in integrated farm management programs to reduce possible losses. Pesticides undergo transformations in the environment when applied to crops. Despite beneficial effects on the global food supply and in maximizing the economic gain from agricultural activities, the use of pesticides can also cause environmental problems (Morrissey et al., 2015; Barron et al., 2017; Della Rossa et al., 2017; Souza et al., 2019). These persistent agrochemicals often cause health hazards to nontarget organisms, including animals and humans.
Azoles are versatile fungicides widely used to control fungal infestations in crops. Triazole fungicides are commonly used in agricultural systems against the main diseases of crops (Zubrod et al., 2019). Difenoconazole, the second molecule of triazole fungicide family to be released in the market, was developed by Switzerland Syngenta Corporation (Chowdhary and Meis, 2018). As a systemic sterol demethylation inhibitor (DMI), difenoconazole has a high ability to interfere with the mycelial growth and inhibit the spore germination of pathogens that ultimately results in inhibiting fungal growth. Difenoconazole is extensively used in a wide range of crops in many countries for its good control of various fungal diseases (Wang et al., 2008). However, the strong hydrophobicity and high bioaccumulation of difenoconazole may lead to undesirable side effects on ecological environment and human health (Chen et al., 2021b). Difenoconazole has been identified to show high toxicity to aquatic organisms such as Daphnia magna (Reproduction NOEC = 0.0056 mg a.s./L) according to the European Food Safety Authority (EFSA, 2011). Our previous study with zebrafish indicated that difenoconazole was able to cause hatching inhibition, abnormal spontaneous movement, slow heart rate, growth regression, and morphological deformities (Mu et al., 2013). Difenoconazole has been used as the main fungicide to control rice diseases for many years, which has a greater opportunity to contact and contaminate the water environment. Therefore, it has become important to check the dissipation behavior of difenoconazole in a paddy field environment.
Previous studies have investigated the dissipation of difenoconazole in rice, paddy water, and sediment (Wang et al., 2012). Considering that pesticide dissipation behavior in the open field is usually related to soil properties and microorganisms in the soil, the influence of different fertilizers on the dissipation dynamics of difenoconazole remains to be further investigated. Anaerobic digestion (AD) has been widely applied in the treatment of various wastes because of its ability to degrade organic solid wastes and generate biogas as renewable energy (de França et al., 2021; Tayibi et al., 2021). As a byproduct of AD, biogas residues is rich in nitrogen, phosphorus, and organic matter and could be used as organic fertilizer (Terhoeven-Urselmans et al., 2009; Tambone et al., 2010; Liu et al., 2011; Ohdoi et al., 2013; Riva et al., 2016; Abelenda et al., 2021), which can sustainably replace inorganic fertilizer (Akhiar et al., 2017; Sigurnjak et al., 2017). Many studies have been carried out to investigate biogas residues as an alternative to chemical fertilizer to improve paddy soil fertility (Ohdoi et al., 2013). The effect of biogas residue application on soil properties has been widely reported in the literature (Odlare et al., 2014; de França et al., 2021; Jurgutis et al., 2021). Therefore, it is of great significance to investigate the influence of biogas residue application on the dissipation dynamics of pesticides in paddy fields.
The present study was performed in open rice fields in Guangze, Fujian Province, China. The purpose was to investigate the effect of biogas residues on the dissipation behavior of difenoconazole in paddy fields.
Materials and methods
Chemical material
Difenoconazole (25%) emulsifiable concentrate (EC) and difenoconazole stock standard solutions of 100 mg/L in acetone were supplied by the College of Science, China Agricultural University.
Biogas residues and chemical fertilizer
Biogas residues were produced during the anaerobic digestion from a biogas plant fed with pig dung, which is located in Guangze, Fujian Province, China. The basic properties of biogas residues from biogas plant fed with pig dung are shown in Table 1.
Field experiment
Field experiments were performed in Guangze, Fujian Province, China, in 2020. Two different treatments were chemical fertilizer treatment and biogas residue treatment (biogas residue total nitrogen input was 153.75 kg/ha equivalent to chemical fertilizer). Nitrogen input was 97.5 kg/ha before rice transplanting on June 1, 2020, and nitrogen input was 56.25 kg/ha after manuring on July 7, 2020, both with chemical fertilizer treatment and biogas residue treatment. Nitrogen content of chemical fertilizer is about 12%; therefore, rice transplantation was made, and after manuring, chemical fertilizer input was 813 and 469.5 kg/ha, separately. Nitrogen content of biogas residues is about 0.04%; therefore, after rice transplantation and manuring, biogas residue input was 243.75 and 139.09 t/ha, separately. The total experiment plot was 300 m2 (Each plot was 50 m2, and chemical fertilizer treatment and biogas residues treatment were repeated three times, respectively). A buffer area of 50 m2 was used to separate the plots of different treatments.
Difenoconazole EC (25%) was sprayed evenly in the above two experiment plots using a SeeSa SX-MD16E-2 backpack sprayer at an active ingredient dose of 45 g a.i./ha. Paddy sediment was collected randomly using a sealing bag from the bottom mud surface to measure difenoconazole concentration. Paddy water was collected randomly using plastic bottles and then mixed in plastic containers to measure difenoconazole concentration. Paddy water and sediment samples were collected from each plot at 2 h (August 31, 2020), 1 day ( September 1, 2020), 3 days ( September 3, 2020), 7 days ( September 7, 2020), 14 days (September 12, 2020), and 21 days (September 21, 2020) after difenoconazole application. Paddy soil was collected randomly using a soil auger to a depth of 10 cm from the land surface to measure pH value and soil organic matter, and soil microorganisms and soil samples were collected from each plot on May 24 and October 26, 2020. Five sediment, water, and soil samples were taken from each plot. All samples were stored at −20°C until analyzed within 1 month.
Preparation of water sample
Water sample (5 ml) was transferred into a 50-ml centrifuge tube, and 10 ml of ethyl acetate was added. The solution was vortexed for 2 min and subsequently centrifuged at 4,000 r/min for 5 min. The supernatant ethyl acetate layer was transferred into a 50-ml heart-shaped bottle. Ethyl acetate (10 ml) was added to the water sample again, and the above extraction operation was repeated once. The supernatant ethyl acetate in the heart-shaped bottle was vacuum evaporated to dryness. The residue was then dissolved with 1 ml of acetonitrile and then filtered through a 0.22-μm membrane filter into an autosampler vial and analyzed by LC–MS.
Preparation of sediment sample
Homogenized sediment sample (2 g) was transferred into a 50-ml centrifuge tube, and then 5 ml of HPLC-grade water and 10 ml of ethyl acetate were added. Further preparation of these samples was the same as for the water samples described above.
Apparatus
All samples were detected by LC-MS/MS of Shimadzu. The ion source was electrospray ionization (ESI), and Agilent POROSHELL 120EC-C18 (2.7 μm × 2.1 mm × 50 mm) was employed at room temperature. The flow rate of acetonitrile/H2O (0.1%HCOOH) = 70/30 (V/V) was 0.2 ml·min−1. Selected multiple reaction monitoring (MRM) as the detection mode in triple-quadrupole mass spectrometry. The relevant parameters and the limit of detection of this method are shown in Table 2.
Measurement of pH value, soil organic matter, and soil microorganisms
The pH of paddy water was measured directly using a calibrated pH meter. The pH of paddy soil is measured according to the following operations: 20 g of naturally dried soil was transferred into a 250-ml Erlenmeyer flask, and 100 ml of deionized water was added. The solution was shaken for 30 min and subsequently placed overnight. The supernatant layer was transferred into a beaker and measured using a calibrated pH meter. The pH measurement of paddy water and soil was repeated three times.
The soil organic matter assay was performed as described. Naturally dried soil (0.5 g) passing a 0.5-mm-diameter sieve was transferred into a glass beaker and 5 ml of K2Cr2O7 (0.8 mol/L) was added. The solution was thoroughly mixed, and 5 ml of concentrated sulfuric acid was added then heated to 185°C–190°C. The solution was kept boiling for 5 min and was made to undergo static cool down. O-phenanthrolin indicator (three to four drops) was added to the cooled solution and titrated with FeSO4 (0.2 mol/L) until the solution turns brown red. The blank test was carried out with silica instead of soil samples.
Soil microorganisms were analyzed using Illumina miseq platform (Chen et al., 2021a). DNA from different samples was extracted using the E.Z.N.A.® Soil DNA Kit (Omega Bio-tek, Norcross, GA, USA) according to the instructions of the manufacturer. Primers 338F (5′-ACT CCT ACG GGA GGC AGC AG-3′) and 806R (5′-GGA CTA CHV GGG TWT CTA AT-3′) were designed to amplify the V3–V4 region gene (Fadrosh et al., 2014). PCR amplification was performed in a total volume of 20 µl of reaction mixture containing 10 ng of template DNA, 4 µl of 5*FastPfu buffer, 2 µl of dNTPs (2.5 mM), 0.8 µl of each primer (5 µM), 0.4 µl of FastPfu polymerase, and PCR-grade water to adjust the volume. PCR amplification conditions were 30 s at 95°C, 30 s at 55°C, and 30 s at 72°C (27 cycles) (ABI GeneAmp® 9700).
The PCR products were confirmed with 2% agarose gel electrophoresis. The PCR products were purified by AxyPrep DNA Gel Extraction Kit (Axygen Biosciences, Union City, CA, USA) and quantified by QuantiFluor™-ST (Promega, USA).
The PE 2*300 library was constructed from purified amplified fragments according to standard operating procedure of Illumina MiSeq (Illumina, San Diego, CA, USA).
In brief: 1) connect the “Y”-shaped joint; 2) remove the self-connecting segment by magnetic bead screening; 3) enrichment of templates by PCR amplification; 4) denature to produce single-stranded DNA fragments by NaOH. Sequencing was performed using Illumina Miseq PE300.
Trimmomatic software was used for quality control of original sequences, and FLASH software was used as a splicing tool: 1) 50 bp was used as the removal criterion, and base back-end sequences with average mass value lower than 20 were subtracted; 2) when sequences on both ends are splicing, the maximum error ratio between overlap is 0.2, and the length should be greater than 10 bp; 3) barcode was accurately matched, and the number of base mismatches of primers was ≤2.
The UPARSE software (version 7.1) http://drive5.com/uparse/ was used for OTU sequence clustering (similarity ≥97%) at the same time removing the single sequence and chimeras. RDP classifier (http://rdp.cme.msu.edu/) was used to make species classification annotation for each sequence and compared with the Silva database (SSU123). The threshold of comparison was 70%.
Statistical analysis
The dissipation of difenoconazole has been generally expressed in terms of DT50, i.e., the time after which 50% of the difenoconazole has disappeared. DT50 values were obtained by fitting first-order kinetics to observe degradation patterns, expressed by the equation: C = C0 e−kt, where C is the chemical concentration at time t (d), C0 is the initial concentration, and k is the first-order rate constant (d−1), which is independent of C and C0. The DT50 of difenoconazole was calculated using Hoskins formula (DT50 = ln2 k−1) for each location (Hoskins, 1961).
Soil organic matter (%) = [(V0−V) × C × 0.003 × 1.724 × f]/W × 100%where, C is the molar concentration of FeSO4 (mol/L), V0 is the volume of the FeSO4 solution consumed by the blank test (ml), V is the volume of the FeSO4 solution consumed by the soil sample test (ml), 0.003 is the molar mass of a quarter of a carbon atom (g/mol) and factor of equivalence (1 L/1,000 ml), 1.724 is the empirical coefficient of converting soil organic carbon into soil organic matter, f is the oxidation correction coefficient (f = 1.1), and W is the dry weight of the tested soil sample (g).
Results
Results of pH, soil organic matter, and soil microorganisms
There was no obvious change in pH of paddy water and sediment before and after fertilizer application with pH around 6.0. However, significant changes in soil organic matter were observed between the two treatment plots with chemical fertilizer and biogas residues. The soil organic matter decreased by 29% in the chemical fertilizer treatment plot, while a higher reduction rate of 34.8% in the soil organic matter was observed in biogas residue treatment plot (Figure 1), indicating that under nitrogen equivalent condition, the application of chemical fertilizer contains more phosphorus and potassium to preserve soil fertility, while there is less nutrient content in biogas residues, and the paddy rice absorbed more from the soil, leading to more decrease in organic matter in biogas residue treatment. Zhang (2017) indicated that fertilizers provide nutrients for agriculture system and increase soil fertility.
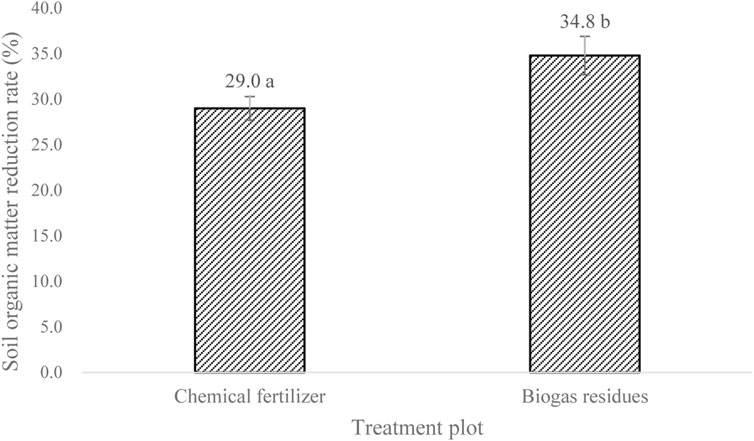
FIGURE 1. Paddy soil organic matter reduction rate. Error bar is S.D. (N = 3). Different lowercase letters denote significant differences (p < 0.05), analyzed by ANOVA followed by Duncan multiple comparison test.
There was also a slight difference in the species of soil microorganisms between the two treatment plots (Figure 2). The main dominant phyla were Proteobacteria, Chloroflexi, Acidobacteriota, and Actinobacteriota, indicating that the application of biogas residues has no effect on the diversity of soil microorganisms.
Dissipation of difenoconazole in paddy water and sediment
The results of difenoconazole dissipation in paddy water are shown in Figure 3. The initial concentrations of difenoconazole were 3.177 μg/L in chemical fertilizer treatment plot and 2.575 μg/L in biogas residue treatment plot, respectively. The dissipation dynamics of difenoconazole in paddy water could be described by the first-order kinetic equation: C = 3.1770e−3.1915t (chemical fertilizer treatment plot) and C = 2.5537e−1.3868t (biogas residue treatment plot), respectively. The dissipation half-life (DT50) of difenoconazole calculated from the regression equation was 0.22 day (chemical fertilizer treatment plot) and 0.50 day (biogas residue treatment plot), respectively.
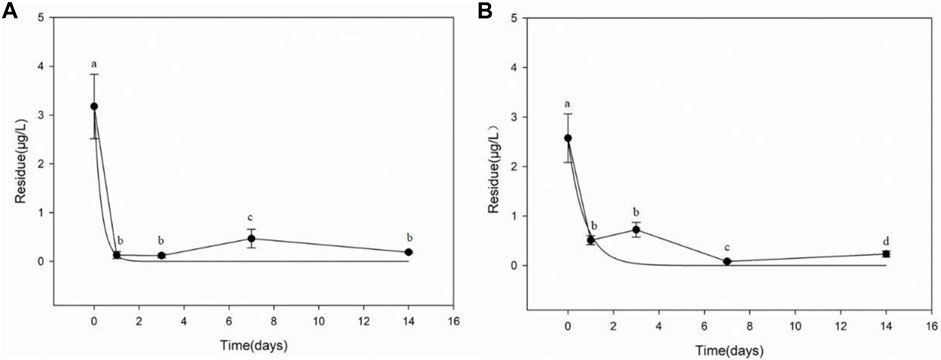
FIGURE 3. Dissipation of difenoconazole in paddy water. (A) Chemical fertilizer treatment plot. (B) Biogas residue treatment plot. Error bar is S.D. (N = 3). Different lowercase letters denote significant differences (p < 0.05), analyzed by ANOVA followed by Duncan multiple comparison test.
Figure 4 shows the dissipation results for difenoconazole in paddy sediment. The initial residues in sediment were 0.796 μg/kg in chemical fertilizer treatment plot and 2.145 μg/kg in biogas residue treatment plot, respectively. A sharp increase in difenoconazole residue occurred from 2 h to 1 day in both treatment plots. After reaching peak in 1 day, a gradual degradation of difenoconazole was observed in accordance with first-order kinetics. The dissipation dynamics of difenoconazole in paddy soil could be described by the equations: C = 2.4804e−0.1495x (chemical fertilizer treatment plot) and C = 2.9403e−0.0687x (biogas residue treatment plot), respectively. The DT50 of difenoconazole in paddy soil were 4.64 days (chemical fertilizer treatment plot) and 10.09 days (biogas residues treatment plot), respectively.
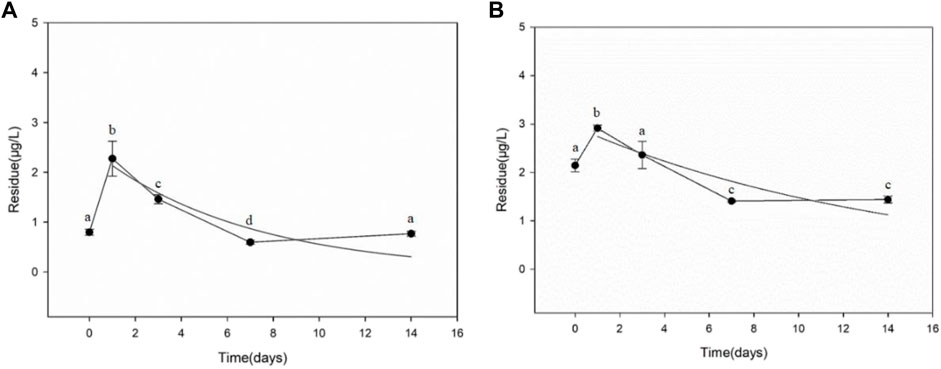
FIGURE 4. Dissipation of difenoconazole in paddy sediment. (A) Chemical fertilizer treatment plot. (B) Biogas residue treatment plot. Error bar is S.D. (N = 3). Different lowercase letters denote significant differences (p < 0.05), analyzed by ANOVA followed by Duncan multiple comparison test.
Discussion
As an important agricultural production material, the pesticides benefit a lot in increasing the agricultural production. However, the current pesticide utilization rate in China is only about 40%, and about 60% of pesticides enter the environment (Shuqin and Fang, 2018), which may pose a threat to the ecological environment. Therefore, the rapid degradation of pesticides after entering the environment is very important to environmental safety. The current research on the negative impact of biogas residues on the environment mainly focuses on heavy metal and antibiotic pollution (Pu et al., 2018; Xu et al., 2019; Liu et al., 2020; Tang et al., 2020; He et al., 2021; Lu et al., 2021). Relatively, little is known whether biogas residues have an impact on pesticide degradation after being used as fertilizer in farmland. It has been reported that the application of biogas residues can significantly improve the physical and chemical properties of soil (Zhu et al., 2012; Wang et al., 2016). However, the dissipation behavior of pesticides in the environment is usually related to soil properties, such as pH, soil organic matter, and soil microorganisms. Therefore, the effect of biogas residues applied in farmland on pesticide dissipation behavior needs further investigation.
In the present paper, we investigate the dissipation behavior of the difenoconazole in rice fields applied with chemical fertilizer or biogas residues. Pesticides can be adsorbed and degraded by organic matter that contains microorganisms (Godeau et al., 2021), which makes it more important to investigate the changes in the organic matter and microorganisms, and their roles in the effect of pesticide dissipation. We observed longer DT50 of difenoconazole in paddy sediment and water in rice fields applied with biogas residues than with chemical fertilizers, in accordance with the severe decrease in organic matter after treatment with biogas residues (Figure 1). Although biogas residues did not change the species of microorganisms in paddy sediment (Figure 2), we speculate that biogas residues may affect the degradation ability of soil microorganisms to difenoconazole. Since the ability of soil microorganisms to degrade pesticides is related to the content of organic matter that contains energy and nutrient supplies for microorganisms in soil, which influences the microbial activities (Holatko et al., 2021). The decline in soil organic matter in paddy field with biogas residues may not provide sufficient carbon source and energy for soil microorganisms, resulting in the decline of soil microbial activities, and thus, their ability to degrade difenoconazole is also reduced. Another possibility of longer DT50 of difenoconazole with biogas residues is that slow biodegradation of difenoconazole may occur under certain conditions. In water–sediment systems, slow degradation from the system was reported; half-life is 8 months. Meanwhile, difenoconazole contains chromophores, which may be susceptible to direct photolysis by sunlight (Environmental Abiotic Degradation1). Grain yield with biogas residues is 8,158.05 kg/ha, 7.5% higher than 7,590.6 kg/ha of chemical fertilizers. Moreover, biogas residues contain microelements, such as calcium, magnesium, iron, etc., which help crops grow more flourishing than chemical fertilizers. We observed that rice crops with biogas residues are more flourishing than with chemical fertilizers, which may block the light to impact photodecomposition. Therefore, we speculate that DT50 of difenoconazole is longer with biogas residues than with chemical fertilizers could be photolysis.
Conclusion
As organic fertilizer, biogas residues can replace chemical fertilizers to provide nutrients for crops. However, our research found that biogas residues may affect the dissipation of the fungicide difenoconazole in rice fields. Difenoconazole has a longer half-life in paddy water and sediment where biogas residues were applied. This study provides a new focus on the scientific and rational use of biogas residues as organic fertilizer in rice field.
Data Availability Statement
The datasets presented in this study can be found in online repositories. The name of the repository and accession number can be found below: National Center for Biotechnology Information (NCBI) BioProject, https://www.ncbi.nlm.nih.gov/bioproject/, PRJNA795026.
Author Contributions
Investigation: LY, YW, YX, and XY. Methodology: XM and XY. Data curation: XM, XLu, and YX. Conceptualization: LY, XM, and SP. Writing–original draft: LY and XM. Writing, reviewing, and editing: LY, SP, and XM. Formal analysis: YW; SP, and YX. Supervision: XLi. Funding acquisition: XLi.
Funding
This work was supported by Zero-Waste City Pilots of Guangze County to evaluate “Zero-Waste Agriculture” performance on research on the establishment of breeding and planting circular ecological agriculture model and environmental risk.
Conflict of Interest
The authors declare that the research was conducted in the absence of any commercial or financial relationships that could be construed as a potential conflict of interest.
Publisher’s Note
All claims expressed in this article are solely those of the authors and do not necessarily represent those of their affiliated organizations, or those of the publisher, the editors, and the reviewers. Any product that may be evaluated in this article, or claim that may be made by its manufacturer, is not guaranteed nor endorsed by the publisher.
Acknowledgments
The authors acknowledge the technical support from Dr. Changsheng Li of the College of Agronomy and Biotechnology, China Agriculture University.
Footnotes
1https://pubchem.ncbi.nlm.nih.gov/compound/86173#section<Environmental-Fate-Exposure-Summary
References
Abelenda, A. M., Semple, K. T., Lag-Brotons, A. J., Herbert, B. M. J., Aggidis, G., and Aiouache, F. (2021). Kinetic Study of the Stabilization of an Agro-Industrial Digestate by Adding wood Fly Ash. Chem. Eng. J. Adv. 7, 100127. doi:10.1016/j.ceja.2021.100127
Akhiar, A., Battimelli, A., Torrijos, M., and Carrere, H. (2017). Comprehensive Characterization of the Liquid Fraction of Digestates from Full-Scale Anaerobic Co-digestion. Waste Manage. 59, 118–128. doi:10.1016/j.wasman.2016.11.005
Barron, M. G., Ashurova, Z. J., Kukaniev, M. A., Avloev, H. K., Khaidarov, K. K., Jamshedov, J. N., et al. (2017). Residues of Organochlorine Pesticides in Surface Soil and Raw Foods from Rural Areas of the Republic of Tajikistan. Environ. Pollut. 224, 494–502. doi:10.1016/j.envpol.2017.02.031
Chen, M., Qin, Y., Deng, F., Zhou, H., Wang, R., Li, P., et al. (2021a). Illumina MiSeq Sequencing Reveals Microbial Community Succession in Salted Peppers with Different Salinity during Preservation. Food Res. Int. 143, 110234. doi:10.1016/j.foodres.2021.110234
Chen, S., Cai, L., Zhang, H., Zhang, Q., Song, J., Zhang, Z., et al. (2021b). Deposition Distribution, Metabolism Characteristics, and Reduced Application Dose of Difenoconazole in the Open Field and Greenhouse Pepper Ecosystem. Agric. Ecosyst. Environ. 313, 107370. doi:10.1016/j.agee.2021.107370
Chowdhary, A., and Meis, J. F. (2018). Emergence of Azole Resistant Aspergillus fumigatus and One Health: Time to Implement Environmental Stewardship. Environ. Microbiol. 20 (4), 1299–1301. doi:10.1111/1462-2920.14055
de França, A. A., von Tucher, S., and Schmidhalter, U. (2021). Effects of Combined Application of Acidified Biogas Slurry and Chemical Fertilizer on Crop Production and N Soil Fertility. Eur. J. Agron. 123, 126224. doi:10.1016/j.eja.2020.126224
Della Rossa, P., Jannoyer, M., Mottes, C., Plet, J., Bazizi, A., Arnaud, L., et al. (2017). Linking Current River Pollution to Historical Pesticide Use: Insights for Territorial Management? Sci. Total Environ. 574, 1232–1242. doi:10.1016/j.scitotenv.2016.07.065
EFSA (2011). Conclusion on the Peer Review of the Pesticide Risk Assessment of the Active Substance Difenoconazole. Parma, Italy: European Food Safety Authority.
Fadrosh, D. W., Ma, B., Gajer, P., Sengamalay, N., Ott, S., Brotman, R. M., et al. (2014). An Improved Dual-Indexing Approach for Multiplexed 16S rRNA Gene Sequencing on the Illumina MiSeq Platform. Microbiome 2 (1), 6. doi:10.1186/2049-2618-2-6
Godeau, C., Morin-Crini, N., Staelens, J.-N., Martel, B., Rocchi, S., Chanet, G., et al. (2021). Adsorption of a Triazole Antifungal Agent, Difenoconazole, on Soils from a Cereal Farm: Protective Effect of Hemp Felt. Environ. Tech. Innovation 22, 101394. doi:10.1016/j.eti.2021.101394
He, L., Zhu, Q., Wang, Y., Chen, C., He, M., and Tan, F. (2021). Irrigating Digestate to Improve Cadmium Phytoremediation Potential of Pennisetum Hybridum. Chemosphere 279, 130592. doi:10.1016/j.chemosphere.2021.130592
Holatko, J., Hammerschmiedt, T., Kintl, A., Danish, S., Skarpa, P., Latal, O., et al. (2021). Effect of Carbon-Enriched Digestate on the Microbial Soil Activity. Plos One 16 (7), e0252262. doi:10.1371/journal.pone.0252262
Hoskins, W. M. (1961). Mathematical Treatment of the Rate of Loss of Pesticide Residues. FAO. Plant Prot. Bull. 9, 163–168.
Jurgutis, L., Šlepetienė, A., Amalevičiūtė-Volungė, K., Volungevičius, J., and Šlepetys, J. (2021). The Effect of Digestate Fertilisation on Grass Biogas Yield and Soil Properties in Field-Biomass-Biogas-Field Renewable Energy Production Approach in Lithuania. Biomass and Bioenergy 153, 106211. doi:10.1016/j.biombioe.2021.106211
Liu, C., Chen, Y., Li, X., Zhang, Y., Ye, J., Huang, H., et al. (2020). Temporal Effects of Repeated Application of Biogas Slurry on Soil Antibiotic Resistance Genes and Their Potential Bacterial Hosts. Environ. Pollut. 258, 113652. doi:10.1016/j.envpol.2019.113652
Liu, W. K., Yang, Q. C., Du, L. F., Cheng, R. F., and Zhou, W. L. (2011). Nutrient Supplementation Increased Growth and Nitrate Concentration of Lettuce Cultivated Hydroponically with Biogas Slurry. Acta Agriculturae Scand. Section B - Soil Plant Sci. 61 (5), 391–394. doi:10.1080/09064710.2010.482539
Lu, Y., Li, J., Meng, J., Zhang, J., Zhuang, H., Zheng, G., et al. (2021). Long-term Biogas Slurry Application Increased Antibiotics Accumulation and Antibiotic Resistance Genes (ARGs) Spread in Agricultural Soils with Different Properties. Sci. Total Environ. 759, 143473. doi:10.1016/j.scitotenv.2020.143473
Morrissey, C. A., Mineau, P., Devries, J. H., Sanchez-Bayo, F., Liess, M., Cavallaro, M. C., et al. (2015). Neonicotinoid Contamination of Global Surface Waters and Associated Risk to Aquatic Invertebrates: a Review. Environ. Int. 74, 291–303. doi:10.1016/j.envint.2014.10.024
Mu, X., Pang, S., Sun, X., Gao, J., Chen, J., Chen, X., et al. (2013). Evaluation of Acute and Developmental Effects of Difenoconazole via Multiple Stage Zebrafish Assays. Environ. Pollut. 175, 147–157. doi:10.1016/j.envpol.2012.12.029
Odlare, M., Pell, M., Arthurson, J. V., Abubaker, J., and Nehrenheim, E. (2014). Combined mineral N and Organic Waste Fertilization - Effects on Crop Growth and Soil Properties. J. Agric. Sci. 152 (1), 134–145. doi:10.1017/S0021859612001050
Ohdoi, K., Miyahara, S., Iwashita, K., Umeda, M., Shimizu, H., Nakashima, H., et al. (2013). Optimization of Fertilizer Application Schedule: Utilization of Digestate after Anaerobic Digestion as Liquid Fertilizer. IFAC Proc. Volumes 46 (4), 317–322. doi:10.3182/20130327-3-jp-3017.00072
Pu, C., Liu, H., Ding, G., Sun, Y., Yu, X., Chen, J., et al. (2018). Impact of Direct Application of Biogas Slurry and Residue in fields: In Situ Analysis of Antibiotic Resistance Genes from Pig Manure to fields. J. Hazard. Mater. 344, 441–449. doi:10.1016/j.jhazmat.2017.10.031
Riva, C., Orzi, V., Carozzi, M., Acutis, M., Boccasile, G., Lonati, S., et al. (2016). Short-term Experiments in Using Digestate Products as Substitutes for mineral (N) Fertilizer: Agronomic Performance, Odours, and Ammonia Emission Impacts. Sci. Total Environ. 547, 206–214. doi:10.1016/j.scitotenv.2015.12.156
Shuqin, J., and Fang, Z. (2018). Zero Growth of Chemical Fertilizer and Pesticide Use: China's Objectives, Progress and Challenges. J. Resour. Ecol. 9 (sp1), 50–58. doi:10.5814/j.issn.1674-764x.2018.01.006
Sigurnjak, I., Vaneeckhaute, C., Michels, E., Ryckaert, B., Ghekiere, G., Tack, F. M. G., et al. (2017). Fertilizer Performance of Liquid Fraction of Digestate as Synthetic Nitrogen Substitute in Silage maize Cultivation for Three Consecutive Years. Sci. Total Environ. 599-600, 1885–1894. doi:10.1016/j.scitotenv.2017.05.120
Souza, L. P., Faroni, L. R. D. A., Heleno, F. F., Pinto, F. G., Queiroz, M. E. L. R., and Prates, L. H. F. (2019). Difenoconazole and Linuron Dissipation Kinetics in Carrots under Open-Field Conditions. Ecotoxicology Environ. Saf. 168, 479–485. doi:10.1016/j.ecoenv.2018.10.070
Tambone, F., Scaglia, B., D’Imporzano, G., Schievano, A., Orzi, V., Salati, S., et al. (2010). Assessing Amendment and Fertilizing Properties of Digestates from Anaerobic Digestion through a Comparative Study with Digested Sludge and Compost. Chemosphere 81 (5), 577–583. doi:10.1016/j.chemosphere.2010.08.034
Tang, Y., Wang, L., Carswell, A., Misselbrook, T., Shen, J., and Han, J. (2020). Fate and Transfer of Heavy Metals Following Repeated Biogas Slurry Application in a rice-wheat Crop Rotation. J. Environ. Manage. 270, 110938. doi:10.1016/j.jenvman.2020.110938
Tayibi, S., Monlau, F., Marias, F., Thevenin, N., Jimenez, R., Oukarroum, A., et al. (2021). Industrial Symbiosis of Anaerobic Digestion and Pyrolysis: Performances and Agricultural Interest of Coupling Biochar and Liquid Digestate. Sci. Total Environ. 793, 148461. doi:10.1016/j.scitotenv.2021.148461
Terhoeven-Urselmans, T., Scheller, E., Raubuch, M., Ludwig, B., and Joergensen, R. G. (2009). CO2 Evolution and N Mineralization after Biogas Slurry Application in the Field and its Yield Effects on spring Barley. Appl. Soil Ecol. 42 (3), 297–302. doi:10.1016/j.apsoil.2009.05.012
Wang, K., Wu, J. X., and Zhang, H. Y. (2012). Dissipation of Difenoconazole in rice, Paddy Soil, and Paddy Water under Field Conditions. Ecotoxicology Environ. Saf. 86, 111–115. doi:10.1016/j.ecoenv.2012.08.026
Wang, Y. Q., Han, W. B., Zhao, Y. Z., Chen, H., Wang, Y. X., and Hu, S. L. (2016). Effects of Biogas Slurry on Soil Physical and Chemical Properties. J. Anhui Agr Sci. 44 (1), 193–195. doi:10.3969/j.issn.0517-6611.2016.01.064
Wang, Z. H., Yang, T., Qin, D. M., Gong, Y., and Ji, Y. (2008). Determination and Dynamics of Difenoconazole Residues in Chinese Cabbage and Soil. Chin. Chem. Lett. 19 (8), 969–972. doi:10.1016/j.cclet.2008.04.028
Xu, Z.-M., Wang, Z., Gao, Q., Wang, L.-L., Chen, L.-L., Li, Q.-G., et al. (2019). Influence of Irrigation with Microalgae-Treated Biogas Slurry on Agronomic Trait, Nutritional Quality, Oxidation Resistance, and Nitrate and Heavy Metal Residues in Chinese Cabbage. J. Environ. Manage. 244, 453–461. doi:10.1016/j.jenvman.2019.04.058
Zhang, F. S. (2017). Scientific Understanding of the Role of Fertilizers. China Agr Technol. Ext 33 (1), 16–19. doi:10.3969/j.issn.1002-381X.2017.01.005
Zhu, Y. L., Na, W., Xi, D. B., and Zhao, X. Y. (2012). Effects of Application of Biogas Slurry of Pig Dung on Physical and Chemical Properties of Soil. J. Anhui Agr Sci. 40 (31), 15202–15203. doi:10.3969/j.issn.0517-6611.2012.31.043
Keywords: difenoconazole, biogas residues, dissipation, paddy water, paddy sediment, paddy soil
Citation: Yu L, Wen Y, Luo X, Xiang Y, Yuan X, Pang S, Ma X and Li X (2022) Effects of Biogas Residues on Dissipation of Difenoconazole in Paddy Sediment System Under Field Conditions. Front. Environ. Sci. 10:814438. doi: 10.3389/fenvs.2022.814438
Received: 13 November 2021; Accepted: 20 January 2022;
Published: 14 March 2022.
Edited by:
Yue Geng, Ministry of Agriculture and Rural Affairs, ChinaReviewed by:
Germán Giácoman Vallejos, Universidad Autónoma de Yucatán, MexicoChuanjiang Tao, Ministry of Agriculture and Rural Affairs, China
Copyright © 2022 Yu, Wen, Luo, Xiang, Yuan, Pang, Ma and Li. This is an open-access article distributed under the terms of the Creative Commons Attribution License (CC BY). The use, distribution or reproduction in other forums is permitted, provided the original author(s) and the copyright owner(s) are credited and that the original publication in this journal is cited, in accordance with accepted academic practice. No use, distribution or reproduction is permitted which does not comply with these terms.
*Correspondence: Xuefeng Li, OTEwMzBAY2F1LmVkdS5jbg==